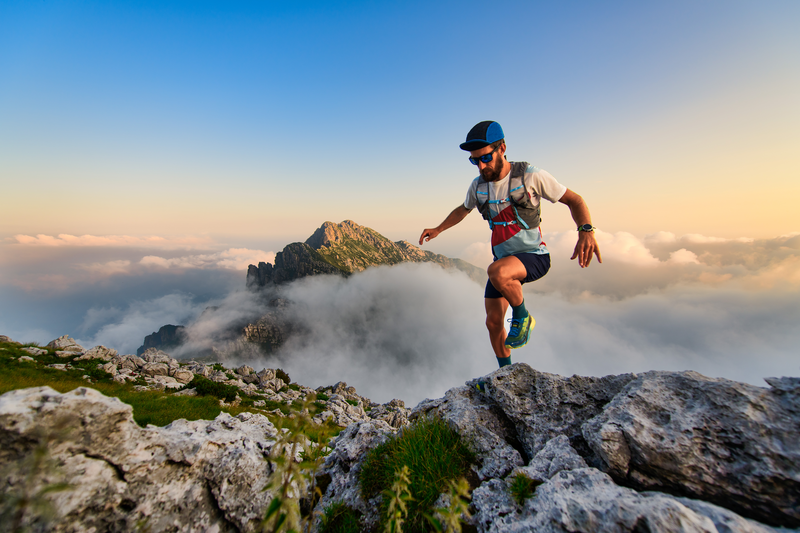
95% of researchers rate our articles as excellent or good
Learn more about the work of our research integrity team to safeguard the quality of each article we publish.
Find out more
ORIGINAL RESEARCH article
Front. Ecol. Evol. , 20 April 2023
Sec. Environmental Informatics and Remote Sensing
Volume 11 - 2023 | https://doi.org/10.3389/fevo.2023.1141155
This article is part of the Research Topic Advanced Methods for Interpreting Geological and Geophysical Data View all 36 articles
The frequency of mudflow disasters induced by rainfall in the Loess Plateau is increasing with the occurrence of global warming. The initial water content is one of the basic properties of soil, which affects the initiation of loess mudflow. In this work, the field study of the debris flow gullies in Yan’an City, Shaanxi Province, China, was conducted, and the main factors that induce gully loess mudflow were summarized. Based on the investigation results, a flume model was designed to carry out flume tests with different initial soil water contents. The experimental results demonstrate the following. (1) Different initial soil water contents lead to different soil failure models. The damage of soil by water flow when the soil water content is in the range of 0−5% is mainly gully erosion; that within the range of 10−15% is mainly rill surface erosion; that within the range of 20−25% is mainly dam breach failure. (2) When the water content of loess is equal to or less than 5% or equal to or greater than 20%, soil can promote the formation of loess mudflow, and the destruction of soil is more likely to cause mudflow disasters. In contrast, when the water content is within 10−15%, loess mudflow is not easily produced. The research results of the initial water content provide not only theoretical support for the study of loess mudflow disasters, but also a reference for the prevention and control of loess mudflow disasters in the Loess Plateau.
The Loess Plateau is one of the most fragile geological environments in China (Xin et al., 2015). The frequency of extreme rainfall in this region is increasing with the occurrence of global warming (He, 2008; Shi et al., 2012; Jia et al., 2014; Zhao A. Z. et al., 2017; Zhang et al., 2019). Rainstorm disaster is one of the natural disasters that usually occur on a global scale, and it is the main cause of soil erosion (Zhou and Wang, 1992; Li et al., 2010; Yang et al., 2023a), and are also an important factor in the occurrence of landslides, debris flows, and other geological disasters (Liu et al., 2023; Yang et al., 2023b; Zhao et al., 2023). Loess mudflow is a common geological disaster in the Loess Plateau. The content of sand in the composition of solid material is between 10 and 20%, and more than 98% of the particles are smaller than 2 mm (Wu, 2014). Compared with debris flow, loess mudflow is characterized by some particularities. However, it also erupted suddenly and violently, which greatly threatens the personal and property safety of the people. For example, in July 2013, Yan’an City in Shaanxi Province suffered continuous rainstorms and triggered mudflow disasters, which resulted in more than 10 casualties (Huang et al., 2014a,b).
Many studies have been conducted to investigate the initiation mechanism of loess mudflow. Research shows that rainfall (Jiang et al., 2002; Chen and Wang, 2013), agricultural irrigation (Wu et al., 2011; Lin, 2013; Zhao, 2013; Zhao J. F. et al., 2017), and groundwater (Xi et al., 2017) are important reasons for the development of loess mudflow. However, there are no definitive conclusions regarding the initial conditions of the development of loess mudflow. Iverson et al. (2000) and Hu et al. (2014, 2015a,b) found that different initial conditions have a very important impact on the development of debris flows. Therefore, it is necessary to study the influence of differences in initial conditions on the development of loess mudflow.
The initial soil water content is one of the basic properties of soil, and affects the initiation of loess mudflow. The research shows that soil erosion is affected by many aspects, among which water is the most important factor leading to soil erosion (Meshram et al., 2022). Soil with different initial water contents exhibits different erosion phenomena under other related factors, which leads to different types of debris flow disasters. Cui et al. (2003) found that the change in the soil water content caused by early rainfall plays an important role in the formation of debris flow. Zhou carried out experiments on debris flow induced by artificial rainfall with a self-made flume model. The relationship between the rainfall intensity and water content was analyzed, and the effects of different initial soil water contents on the initiation of sand debris flow were studied (Zhou et al., 2012, 2016). Hu et al. (2015b) carried out flume experiments and found that the initial soil water content can affect the permeability coefficient and internal mechanical properties of soil, which led to significantly different types in the initiation of debris flow. The effect of antecedent rainfall on the initiation of debris flow was then simulated by different initial soil water contents (Xu, 2014; Zhu, 2014). Pan et al. (2017) carried out the glacial debris flow initiation test of natural moraine soil with different initial water content, and approximately simulated the initiation process of debris flow formed by melting water scouring moraine under different early rainfall, and studied the influence of initial water content on the initiation of glacial debris flow.
In summary, the initial soil water content is an important initial condition for studying the initiation mechanism of debris flow. However, there are no definitive conclusions on the initiation of loess mudflow. Therefore, in the present research, the gully loess mudflow test under different initial water contents is carried out, and the failure characteristics of soil under different initial soil water contents were analyzed. The research results will provide theoretical support for the prevention and control of loess mudflow disasters.
The test takes the loess mudflow channel in Yan’an City, Shaanxi Province as the research object. The main parameters of mudflow disasters in the Yan’an area are obtained based on a field investigation and report (Huang, 2015). The gullies of debris flow in Yan’an are small watershed gullies with an average watershed area of 4.08 km2, an average gully length of 3.03 km (0.34−7.1 km), an elevation difference of 54−349 m, and an average gradient of 21.2% (1.94−32.83%). According to the similarity principle, a scale of 1:850 was selected to design the flume model, the main parameters of which are reported in Table 1. The flume model (Figure 1) is a channel model composed of transparent materials with a length of 3.6 m and a trapezoidal cross-sectional area (with a bottom width of 0.1 m, an upper floor width of 1.02 m, and a height of 0.39 m). The slope is 21.2%. The channel is divided into three parts, namely the catchment area, soil accumulation area, and running area. Moreover, in order to increase the friction between the transparent material and the test soil, sandpaper is pasted at the contact area between the sink and the pile soil to increase the friction between the bottom groove and the side (Hu et al., 2014, 2015a,b). A flow pump is used to supply water during the experiments, and the water supply rate is 0.3 ± 0.01 L/s. The design of the water flow rate is scaled according to the flow rate in the Yan’an small watershed with a design frequency of 2%.
The materials used in the experiments were sourced from a watershed in Baota District, Yan’an City, Shaanxi Province (109°31′07 ′′E, 36°29′58′′N). The basic physical and mechanical parameters were measured, and were reported in Table 2 (Yang et al., 2019). The grading curve was obtained by screening the test materials (Figure 2). The flume model was scaled proportionally to the field conditions. The test materials were then similarly translated in the flume experiments to avoid the influence of the larger particle size of the accumulated soil on the experimental result. However, the particle size of loess is small, and the content of particles smaller than 0.075 cm was found to reach 53.97% (Figure 2). Therefore, the field loess soil samples were used directly in the experiments (Yuan et al., 2015).
The measured dry bulk density of the loess sample was 11.6 kN/m3. The water content of the experimental soil samples was changed with a gradient of 5% to ensure that the water content was not affected by the bulk density. The soil initial water content of each group is reported in Table 3. The soil water content of the samples in different positions was measured before the experiments to ensure that the soil water content and bulk density of the soil samples met the experimental requirements. When the soil water content met the requirements, the soil layering step was carried out. During laying, the piled soil was successively divided into layers of 5, 10, 10, and 5 cm. After each layer of soil was laid, a cutting ring was used to sample the soil to ensure that the soil water content and bulk density met the requirements. After all the soil was laid and the other parameters were found to have met the requirements, the experiments were immediately initiated and carried out within 20 min to avoid water evaporation.
During the experiments, the fluid produced was collected in plastic containers. The time interval of the fluid collection in each container was recorded by a stopwatch, and the volume and mass of the fluid in the container were then measured.
The experimental phenomenon of the development of loess mudflow under different soil water contents was determined via flume model experiments. To ensure the accuracy and repeatability of the experiments, three repeated experiments were carried out. The water content of the repeated experiments was 10%, which was close to the field sampling data. The volume concentration measured during the repeated experiments is presented in Figure 3.
Based on the comparison of volume concentration in the repeated experiments, the change rules in each group were found to be roughly the same, but there were some minor differences. The differences between repeated experiments were mainly caused by the comprehensive action of many factors involved in the occurrence of debris flow. Moreover, experimental error may also have affected the experimental results. Based on the comparison of the experimental parameters, the volume concentration in the repeated experiments fluctuated within the range of 2.61−7.02%, and the volume concentration decreased with time, the overall fluctuation range is not large. In summation, some differences were found in the sequence and process of the experiments under the same conditions. However, from the perspective of the movement process, the changes in the parameters of the experimental products met certain conditions. These findings all demonstrate that the experimental results obtained by using the indoor flume model have certain reliability.
Different initial soil water contents led to different types of soil failure. The failure of the experimental soil can be roughly divided into three types, namely gully erosion, rill surface erosion, and dam breach failure.
When the water content of the soil was between 0 and 5%, the damage caused to the soil by the water flow was primarily gully erosion. When the water flowed over the soil, the water could not infiltrate quickly. Most of the water flow on the soil surface formed a flow similar to surface runoff, which constantly eroded the surface of the soil, and then formed deeper undercut gullies. With the continuation of undercutting erosion, headward erosion occurred. The undercut gullies extended to the back and eventually formed narrow erosion gullies. The entire process of the experiment with the initial water content of 0% is presented in Figure 4 and lasted for 400 s. After the start of the experiment, the water flow accumulated continuously behind the piled soil and overflowed from the upper part of the soil at 109 s (Figure 4B). The loess particles were carried out by the overflowing water flow, and obvious gullies were formed on the free surface of the soil in a short period (Figure 4C). Then, the obvious under erosion of the soil occurred. It was then found that headward erosion occurred in the backward direction of the soil (Figure 4D). Under the effects of undercutting erosion and headward erosion, the soil developed from small gullies to large gullies. The soil on the right side of the gullies collapsed at 387 s (Figure 4E). It was obvious that the soil on the collapse surface was still dry, indicating that the permeability of dry loess was very poor. At the end of the experiment at 400 s, the soil did not change greatly.
Figure 4. The experimental process with the initial water content of 0%. In this figure describes the test process when the water content is 0%. For (A,F), it is the beginning and result node of the test, and (B–E) is the important node in the test process.
The rill surface erosion damage mainly occurred in the soil water content of 10 ∼ 15%. When the water flowed through the piled soil, the water still could not infiltrate in time. The sediment carrying capacity of the water was low. Thus, a flow channel could not be formed rapidly, which contributed to a large area of water flowing through the soil surface (Figure 5). Numerous small gullies were then formed on the soil surface with the continuation of the experiment.
Collapse damage occurred when the water content was between 20 and 25%. Due to the high water content of the soil, the strength of the soil was relatively low. The loess flocculated into clusters with a high water content, which led to the development of a large number of cracks between the soils. A large amount of water then flowed into the soil, and the soil water content further increased. Therefore, the strength of the soil continued to decline. When the strength was below a certain threshold, dam breach failure occurred (Figure 6).
To further explore the effect of the soil water content on the development of mudflow, the bulk density of the experimental products was measured. The relationship between the bulk density and the water content in each experiment is exhibited in Figure 7, from which it can be seen that the bulk density decreased gradually with the continuation of time. The maximum bulk density in each experiment is presented in Figure 8.
It can be seen from Figure 8 that with the increase of the soil water content, the effect of the water content on the initiation of mudflow was first restrained and then promoted. When the soil water content was low, there was a lack of water cohesion between the soil, resulting in low soil strength. Thus, the soil could easily be lost under the action of water flow. When the soil water content was high, the matrix suction of the soil was low, which led to the decrease of soil cohesion. Thus, the soil could easily be destroyed. To further explore the relationship between the soil water content and the maximum bulk density, the data were fitted and analyzed by Origin software. The optimal fitting model given by Eq. (1) was then obtained.
In Table 4, SS and MS are, respectively, the regression square sum and the mean square deviation, and F is the F-test distribution value. Moreover, P is a coefficient used to determine whether the regression fitting model has significant statistical significance, and is usually compared with α = 0.05; if the P-value is less than 0.05, the correlation of the fitting model is determined to be statistically significant. Finally, R2 and R2 (adj) are, respectively, the unadjusted and adjusted correlation coefficients. In general, R2 (adj) should be greater than 80%. The larger the value of R2 (adj), the higher the goodness of the model fitting data.
Based on the theoretical analysis of the mathematical statistics, it can be determined from Table 4 that the correlation coefficients R2 and R2 (adj) of the fitting model were both more than 95%, indicating that the relationship between the model and the experimental results was good. Therefore, the fitting model was found to meet the requirements of statistical theory.
The values of two groups of parallel experiments were substituted into Eq. (1) to validate its effectiveness. The comparative analysis results with the measured values are reported in Table 5, from which it can be seen that there was little difference between the theoretical values of the maximum bulk density of the parallel experiments and the measured values, the relative error was less than 5%. Therefore, Eq. (1) can be used to better predict and calculate the maximum bulk density values that may occur at different initial water contents.
Table 5. The verification results of the model of the relationship between the soil water content and the maximum bulk density.
The loess mudflow gullies in Yan’an City, Shaanxi Province, China, were selected as the research object of this study. Based on the field investigation and the survey data, the flume model was designed to carry out flume experiments with different initial soil water contents, and the following conclusions were obtained.
(1) Different initial soil water contents led to different soil failure modes. The failure of the experimental soil can be roughly divided into three types, namely gully erosion (within the soil water content range of 0−5%), rill surface erosion (within the soil water content range of 10−15%), and dam breach (within the soil water content range of 20−25%).
(2) When the water content of loess was equal to or less than 5% or equal to or greater than 20%, the maximum bulk density of the experimental products was found to be relatively high, and mudflow occurred in some instances, indicating that the soil has a promoting effect on the formation of loess mud flow. When the soil water content was between 10 and 15%, the maximum bulk density of the experimental products was low, and mudflow was not obviously formed. Therefore, loess mudflow cannot easily occur when the soil is damaged.
(3) A quantitative analysis demonstrated that the relationship between the maximum bulk density of each experiment and the soil water content was as follows: γm max = 0.019w2−0.084w+12.98. This model was verified by parallel experiments, and the error was less than 5%.
This research explored the influence of the initial soil water content on the failure mode of soil in the development of loess mudflow. The results revealed that the initial soil water content has an obvious influence on the formation process of loess mudflow. However, while the failure types of soil were found to be distinguished, the critical water content of these failure types was not determined. Therefore, further research on the critical soil water content for soil failure should be carried out. The research results provide not only technical support for the study of loess mudflow in Yan’an, but also a theoretical reference for the prevention and control of mudflow disasters in this area.
The raw data supporting the conclusions of this article will be made available by the authors, without undue reservation.
ZY contributed to the all aspects of this work, wrote the manuscript text, and revised and finalized it. MC processed and analyzed the data. JZ collected the data and samples in the field. PD, NH, and YY reviewed the manuscript and revised and finalized it. All authors contributed to the article and approved the submitted version.
This work was supported by the National Natural Science Foundation of China (Grant No. 41861134008), the Muhammad Asif Khan Academician Workstation of Yunnan Province (Grant No. 202105AF150076), the Key R&D Program of Yunnan Province (Grant No. 202003AC100002), and the General Program of Basic Research plan of Yunnan Province (Grant No. 202001AT070043).
PD was employed by China Construction Science and Technology Group Co., Ltd.
The remaining authors declare that the research was conducted in the absence of any commercial or financial relationships that could be construed as a potential conflict of interest.
All claims expressed in this article are solely those of the authors and do not necessarily represent those of their affiliated organizations, or those of the publisher, the editors and the reviewers. Any product that may be evaluated in this article, or claim that may be made by its manufacturer, is not guaranteed or endorsed by the publisher.
Chen, H. X., and Wang, J. D. (2013). Experimental research about the rainfall’s effects on mudflow initiation. J. North. Univ. Nat. Sci. Edit. 43, 447–450. doi: 10.16152/j.cnki.xdxbzr.2013.03.014
Cui, P., Yang, K., and Chen, J. (2003). Contribution of early rainfall to debris flow formation: taking Jiangjiagou debris flow formation as an example. China Soil Water Conserv. Sci. 2003, 11–15. doi: 10.16843/j.sswc.2003.01.006
He, X. B. (2008). Clay illuviation in a holocene palaeosol -sequence in the chinese loess plateau. Berlin Heidelberg: Springer.
Hu, W., Xu, Q., Rui, C., Huang, R. Q., Van Asch, T. W. J., Zhu, X., et al. (2015a). An instrumented flume to investigate the initiation mechanism of the post-earthquake huge debris flow in the southwest of China. Bull. Eng. Geol. Environ. 74, 393–404. doi: 10.1007/s10064-014-0627-3
Hu, W., Xu, Q., Van Asch, T. W. J., Zhu, X., and Xu, Q. Q. (2014). Flume tests to study the initiation of huge debris flows after the wenchuan earthquake in S-W China. Eng. Geol. 182, 121–129. doi: 10.1016/j.enggeo.2014.04.006
Hu, W., Xu, Q., Wang, G. H., Van Asch, T. W. J., and Hicher, P.-Y. (2015b). Sensitivity of the initiation of debris flow to initial soil moisture. Landslides 12, 1139–1145. doi: 10.1007/s10346-014-0529-2
Huang, Y. H. (2015). Detailed survey and comprehensive research report of geological hazards in Yan’an.
Huang, Y. H., Feng, W., and Li, Z. G. (2014a). Characteristics and geological disaster mode of the rainstorm happened on July 3. 2013 in Yanan area of Shaanxi province. J. Catastrophol. 29, 54–59.
Huang, Y. H., Wu, W. Y., Feng, W., and Li, Z. G. (2014b). Main types and characteristics of the geo-hazards triggered by heavy rain on July 3 in Yan’an,Shaanxi. Northwest. Geol. 47, 140–146.
Iverson, R., Reid, M., Iverson, N., LaHusen, R., Logan, M., Mann, J., et al. (2000). Acute sensitivity of landslide rates to initial soil porosity. Science 290, 513–516. doi: 10.1126/science.290.5491.513
Jia, W. X., Zhang, Y. S., and Li, Z. S. (2014). Spatial and temporal change of precipitation extremes in Qilian mountains and Hexi corridor in recent fifty years. Sci. Geogr. Sin. 34, 1002–1009. doi: 10.13249/j.cnki.sgs.2014.08.002
Jiang, X. W., Zhang, J., Zou, Y. Q., and Liu, Y. J. (2002). Discussion on the dynamic features and dynamic mechanism of storm debris flow in loess area. Chin. J. Geol. Hazard Control 13, 29–33.
Li, Z., Zheng, F. L., and Liu, W. Z. (2010). Analysis on the spatiotemporal changes of extreme precipitation events on the loess plateau from 1961 to 2007. J. Nat. Res. 25, 291–299.
Lin, X. Y. (2013). Study on formation mechanism of loess landslide and mud flow In heifangtai irrigation area, gansu. Ph. D, thesis. Yan’an, Shanxi, China: Chang’an University.
Liu, Z. Q., Yang, Z. Q., Chen, M., Xu, H. H., Yang, Y., Zhang, J., et al. (2023). Research hotspots and frontiers of mountain flood disaster: bibliometric and visual analysis. Water 15:673. doi: 10.3390/w15040673
Meshram, S. G., Ilderomi, A. R., Sepehri, M., and Santos, C. A. G. (2022). Flood prioritization based on fuzzy best worse multi-criteria decision-making method. Arab J. Geosci. 15:1374. doi: 10.1007/s12517-022-10570-z
Pan, L., Wei, X. L., Zhang, Y. F., Li, B., and Yang, X. L. (2017). Influence of initial water content on glacial debris flow triggering process. J. Soil Water Conservat. 31, 116–122. doi: 10.13870/j.cnki.stbcxb.2017.06.020
Shi, J. S., Wu, S. R., Zhang, Y. S., and Wang, T. (2012). Integrated landslide mitigation strategies study for global change in China. Geol. Rev. 58, 309–318. doi: 10.16509/j.georeview.2012.02.018
Wu, C. X., Xu, L., Dai, F. C., Min, H., Tan, G. H., Qi, G. L., et al. (2011). Topographic features and initiation of earth flows on heifangtai loess plateau. Rock Soil Mechan. 32, 1767–1773. doi: 10.16285/j.rsm.2011.06.019
Wu, X. J. (2014). Study on the formation mechanism of debris flow (mud flow) in Yan’an. Ph. D, thesis. Yan’an, Shanxi, China: Northwest University.
Xi, Y., Li, T. L., and Xing, X. L. (2017). Analysis of triggering mechanism of loess landslide mudflow induced by seepage of irrigation channels. J. Earth Sci. Environ. 39, 135–142.
Xin, P., Wu, S. R., Shi, J. S., Wang, T., and Shi, L. (2015). Comment on the progress in, problems and countermeasure on mudflow induced by rainfall. Geol. Rev. 61, 485–493. doi: 10.16509/j.georeview.2015.03.002
Xu, Q. Q. (2014). Exploration on the cause of the formation of loose debris after the earthquake—considering the effects of slope, discharge and initial water content. Ph. D, thesis. Chengdu, Sichuan, China: Chengdu University of Technology.
Yang, Z. Q., Ding, P., Yu, D. C., Yang, Y., Zhu, Y. Y., and Han, Y. S. (2019). Shear strength of Yan’an loess interpreted by porosity. Mount. Res. 37, 392–399. doi: 10.16089/j.cnki.1008-2786.000432
Yang, Z. Q., Wei, L., Liu, Y. Q., He, N., Zhang, J., and Xu, H. H. (2023a). Discussion on the relationship between debris flow provenance particle characteristics, gully slope, and debris flow types along the Karakoram Highway. Sustainability 15:5998. doi: 10.3390/su15075998
Yang, Z. Q., Zhao, X. G., Chen, M., Zhang, J., Yang, Y., Chen, W. T., et al. (2023b). Characteristics, dynamic analyses and hazard assessment of debris flows in Niumiangou Valley of Wenchuan County. Appl. Sci. 13:1161. doi: 10.3390/app13021161
Yuan, B., Chen, W. W., Tang, Y. Q., Li, J. P., and Yang, Q. (2015). Experimental study on gully-shaped mud flow in the loess area. Environ. Earth Sci. 74, 759–769. doi: 10.1007/s12665-015-4080-9
Zhang, F. Y., Yan, B. B., Feng, X. M., Lan, H. X., Kang, C., Lin, X. S., et al. (2019). A rapid loess mudflow triggered by the check dam failure in a bulldoze mountain area, Lanzhou, China. Landslides 16, 1981–1992. doi: 10.1007/s10346-019-01219-2
Zhao, A. Z., Zhu, X. F., and Pan, Y. Z. (2017). Spatiotemporal variations of extreme precipitation events in the loess plateau from 1965 to 2013. J. Beijing Normal Univ. Nat. Sci. 53, 43–50. doi: 10.16360/j.cnki.jbnuns.2017.01.009
Zhao, J. F. (2013). Heifangtai loess landslide-muddy flow formation mechanism caused by irrigation. Ph. D, thesis. Xi’an, Shanxi, China: Chang’an University.
Zhao, J. F., Huang, J. Y., Hou, X. K., Li, T. L., and Lin, X. Y. (2017). Analysis of a flow-slide in heifangtai induced by irrigation. J. Catastrophol. 32, 60–66.
Zhao, X. G., Yang, Z. Q., Meng, X. R., Wang, S. B., Li, R., Xu, H. H., et al. (2023). Study on mechanism and verification of columnar penetration grouting of time-varying Newtonian fluids. Processes 11:1151. doi: 10.3390/pr11041151
Zhou, J., Du, Q., and Li, C. N. (2016). Model test of rainfall intensity influence on debris flow starting. J. Nat. Dis. 25, 104–113. doi: 10.13577/j.jnd.2016.0312
Zhou, J., Gao, B., Zhang, J., and Jia, M. C. (2012). Influence of initial water content on sandy debris flow starting process. Chin. J. Rock Mechan. Eng. 31, 1042–1048.
Zhou, P. H., and Wang, Z. L. (1992). Study on rainstorm of soil erosion on the loess plateau. J. Soil Water Conservat. 1992, 1–5. doi: 10.13870/j.cnki.stbcxb.1992.03.001
Keywords: initial soil water content, flume experiments, loess, loess mudflow, soil failure mechanism
Citation: Yang Z, Chen M, Zhang J, Ding P, He N and Yang Y (2023) Effect of initial water content on soil failure mechanism of loess mudflow disasters. Front. Ecol. Evol. 11:1141155. doi: 10.3389/fevo.2023.1141155
Received: 10 January 2023; Accepted: 03 April 2023;
Published: 20 April 2023.
Edited by:
Ahmed M. Eldosouky, Suez University, EgyptReviewed by:
Kamal Abdelrahman, King Saud University, Saudi ArabiaCopyright © 2023 Yang, Chen, Zhang, Ding, He and Yang. This is an open-access article distributed under the terms of the Creative Commons Attribution License (CC BY). The use, distribution or reproduction in other forums is permitted, provided the original author(s) and the copyright owner(s) are credited and that the original publication in this journal is cited, in accordance with accepted academic practice. No use, distribution or reproduction is permitted which does not comply with these terms.
*Correspondence: Pan Ding, dG1hYzExMDI1QDE2My5jb20=; Na He, aG42MTg4NkAxNjMuY29t; Yi Yang, MjkxOTg0NzIzMEBxcS5jb20=
Disclaimer: All claims expressed in this article are solely those of the authors and do not necessarily represent those of their affiliated organizations, or those of the publisher, the editors and the reviewers. Any product that may be evaluated in this article or claim that may be made by its manufacturer is not guaranteed or endorsed by the publisher.
Research integrity at Frontiers
Learn more about the work of our research integrity team to safeguard the quality of each article we publish.