- 1Sierra Nevada Aquatic Research Laboratory, University of California Natural Reserve System, Mammoth Lakes, CA, United States
- 2Marine Science Institute, University of California, Santa Barbara, CA, United States
Saline lakes worldwide are undergoing drying, and as lake levels fall and areas contract, salinities increase. There is a critical need for data on salinity impacts to guide conservation for recovery of the aquatic productivity that supports migratory and breeding birds that depend on these habitats. Brine flies are key sources of food to these birds and are adapted for life in saline waters owing to their capacity for osmotic regulation. The sublethal effects on growth, development and reproduction were determined in experiments and field observations with the alkali fly Cirrula hians from alkaline lakes of differing salinity. The cost of osmoregulation to fitness from rising salinity was exhibited in slower growth rates of larvae, smaller size at maturity of pupae, reduced adult emergence success, and lower fecundity. The results identify a salinity management range of 25 to 100 g L−1 that would optimize life history traits and productivity of this insect as a food source for birds.
Introduction
Saline lakes worldwide are in crisis as lake levels and habitat areas have declined owing to histories of diversion of inflowing rivers and streams that otherwise would offset the evaporation of water from these closed-basin ecosystems (Wurtsbaugh et al., 2017). As salinities rise at the same time, the aquatic invertebrate life of these lakes that serve as key food resources to myriad migratory and breeding waterbirds are increasingly threatened (Jehl, 1994; Rubega and Robinson, 1996; Andrei et al., 2009). Adult brine flies congregate along shorelines of saline lakes in super-abundance (tens of thousands per square meter), while larvae and pupae stages are aggregated in shallow water where they often secure themselves on rocky substrata or feed on mats of algae (Herbst, 1988; Herbst and Bradley, 1993). Their contagious distribution thus allows easy foraging on all life stages by shorebirds in particular (Elphick and Rubega, 1995; Strauss et al., 2002; Senner et al., 2018; Frank and Conover, 2021). Along with the abstraction of stream inflows, and exacerbated by ongoing drought related to climate change (Seager et al., 2007), these rapidly desiccating habitats are in critical need of clear criteria for multiple-use management of salinity levels to maintain productivity and ecological integrity in balance with consumptive uses (e.g., Wurtsbaugh and Sima, 2022). Salinization may also be a contributing factor to the long-term decline noted for North American migratory shorebirds (37% loss, Rosenberg et al., 2019).
Halophilic organisms often do not so much as love salt as they have evolved means to tolerate it. In seawater where there is usually little variation in external salinity (around 34 g L−1, excepting estuaries and some tidal habitats), marine invertebrates simply live with it, that is, their internal solute content conforms to the environment (osmoconformers, Prosser, 1973). Inland saline water environments, however, can vary considerably in chemical composition and dissolved ion concentration, and can reach salinities well in excess of 200 g L−1, to saturation and in anionic content of chloride, carbonates and sulfate (Hardie and Eugster, 1970). Common halophilic invertebrates such as brine flies and brine shrimp usually cope with varied salinity through osmoregulation (Bradley, 2009). Keeping metabolic homeostasis requires regulation of a stable internal osmotic concentration. There can be no question that osmotic homeostasis of intra- and extra-cellular fluids requires energy as ATP generated from mitochondria amassed in cells that power active transport in trans-membrane enzyme ion pumps that remove solutes. The extent to which this limits other physiological processes is critical to the success of these organisms in inhabiting saline environments. Why live in such extremes? If freshwater habitats are full of a diversity of other aquatic life, salt tolerance may provide an extremophile escape from the rigors of coping with competition and predation and often enables achieving high levels of productivity—but at what cost is the subject of the present study. The energy demands of osmoregulation relative to the supply of food over a range of external salinity may be the essential determinants of population productivity where ecological limitations are absent.
The aquatic larvae of Cirrula hians Say (Diptera: Ephydridae, formerly placed in the genus Ephydra) osmoregulate over a wide range of salinity (Herbst et al., 1988), and must therefore vary in expenditure of energy in maintenance of internal osmotic homeostasis. Although the physiological tolerance and cellular mechanisms associated with ion and water balance have been investigated in this and other species of saline water insects (e.g., Barnby, 1987; Bradley, 1987), only a few studies have investigated the net cost of these processes to the growth and development of such organisms. For example, increased salinity has been found to limit growth, prolong development, and reduce size at maturity in other osmoregulators such as brine shrimp and brine mosquitoes (Nayar, 1969; Dana and Lenz, 1986).
Also known by the common name, the alkali fly, C. hians inhabits alkaline salt lakes in western North America (Wirth, 1971) that contain high concentrations of bicarbonate and carbonate anions at high pH (Herbst, 1988). Larvae use a specialization of the Malpighian tubules to concentrate and excrete carbonate in the form of calcium carbonate microspheres (Herbst and Bradley, 1989a). Development occurs through three larval instars that feed on benthic algae and cyanobacteria in the shallow littoral zone (Herbst and Bradley, 1993; Bradley and Herbst, 1994) and attach to hard calcareous substrates as pupae, emerging as adults to gather in dense bands along lake shorelines. The alkali fly is often the most abundant occupant of these habitats along with the related species Ephydra gracilis, found primarily in chloride-dominated water chemistry (Aldrich, 1912; Collins, 1980a; Herbst, 1999).
The objectives of this study were to investigate both the developmental and reproductive costs of increasing salinity and how these may be further altered by varied food supply. Over a wide range of experimental salinity treatments, growth of larvae from Mono Lake (California) was measured as was the duration to and size at maturity of resulting pupae and success of emergence of adult flies from these pupae. Experiments at varied salinities with flies raised from the egg stage were also conducted with and without algal food limitations. In addition, field studies contrasting life stages of C. hians populations coming from Mono Lake with those from the less saline Lake Abert (Oregon) allowed contrast of life history traits (adult size and fecundity), and relative biochemical food values, under natural conditions of differing salinity.
Methods
Environmental setting
Mono Lake, in east-central California, and Lake Abert, in south-central Oregon are two of the largest closed-basin salt lakes of the western Great Basin high-altitude desert (Herbst, 1988). These are alkaline lakes (mainly CO3−2 and HCO3− anions, also Cl− and SO4−2), pH near 10, and salinity of Mono at ~90 g L−1 (grams per liter total dissolved solutes) and Abert at ~25 g L−1 at the time of these studies (field and lab work were all conducted 1983–1987). Being a shallow lake of smaller volume, the water levels and salinity of Abert are more prone to fluctuate than the deeper Mono Lake, but both have histories of diversions of inflowing streams that have concentrated salts because of evaporative losses exceeding the inflow of freshwater to these terminal lakes (Figure 1).
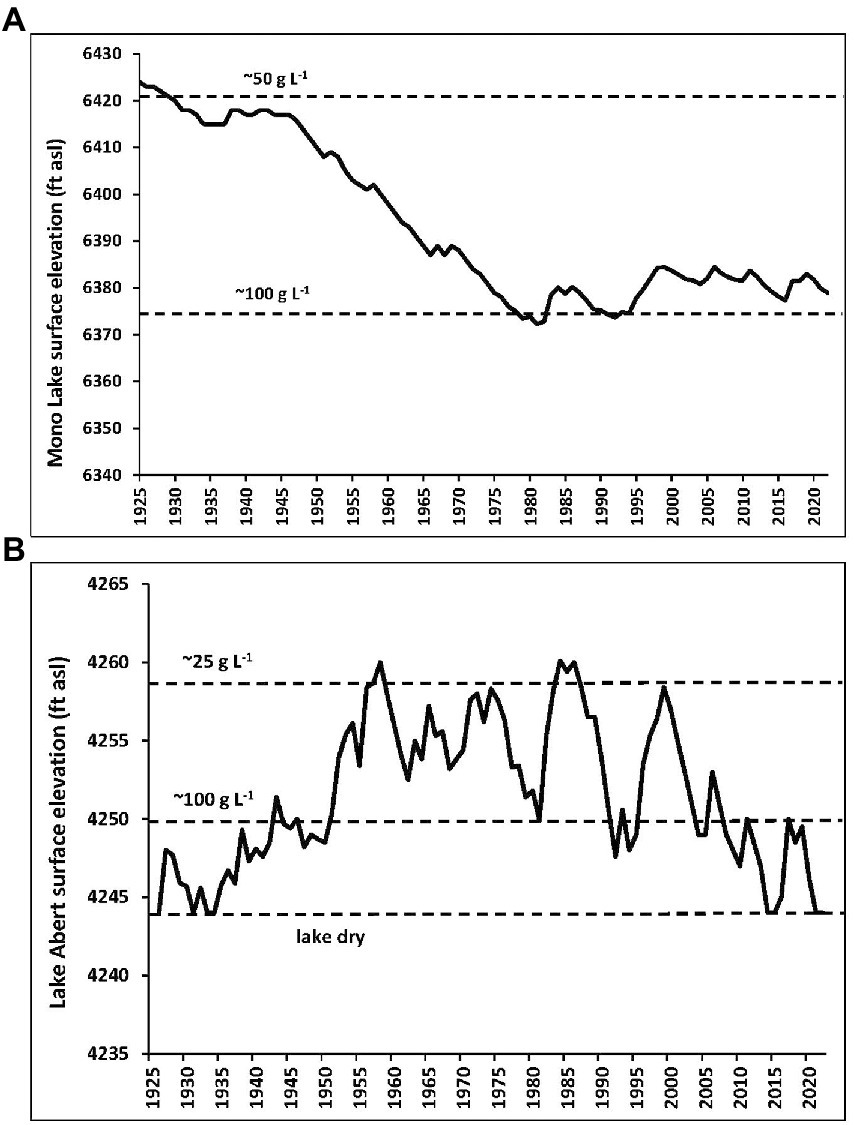
Figure 1. Historical trends in the surface elevation of Mono Lake (A) and Lake Abert (B), showing key salinity levels associated with lake elevation (feet above sea level, asl). Mono Lake levels and salinity: https://www.monobasinresearch.org/data/levelyearly.php (Mono Basin Clearinghouse), and (Herbst, 1988). Lake Abert levels and salinity: (Larson et al., 2016).
Larval growth experiments
The influence of osmotic stress on growth rates, development time, and size at maturity of pupae was examined by exposure of third instar larvae to experimental treatment salinities. Third instars were staged to be of equal age between treatments by rearing field-collected second instar larvae (from shallow near-shore waters using an aquarium net) at the treatment salinity and then isolating newly molted third instars into separate culture dishes to initiate individual trials at salinities of 10, 50, 100, and 150 g L−1. To prepare this salinity range, filtered lake water (0.45 micron membrane filters) was either diluted with distilled water or concentrated by evaporation in large shallow pans over several weeks, with salinities adjusted to a specific gravity corresponding to the desired salinity (as documented in Herbst, 1988). The lower salinity of 10 g L−1 is nearly iso-osmotic with the hemolymph (blood) of larvae (Herbst et al., 1988), while the upper salinity of 150 g L−1 was chosen based on preliminary scope of response data showing first instar larvae do not survive longer than 72 h at 200 g L−1 but some will survive exposures at 150 g L−1. Each larva was contained in a 60 × 15 mm plastic culture dish with lid, in 15 ml of treatment water, held in humidified boxes in an environmental chamber at 20°C on a 14:10 L:D light:dark cycle. Food was provided from fresh lab-cultures consisting of a mix of diatoms and cyanobacteria (mostly Nitzschia frustulum and Oscillatoria spp.) that were grown in nutrient-enriched (Guillard F/2 seawater medium) water only at 50 g L−1 so that food quality was held constant. Algae cultures used for feeding were harvested after about 1 week by centrifuging, and the pellet drawn into small syringes and delivered equally into the culture dishes. Food was also supplemented with algae cultured on nutrient-enriched 1% agar pour plates, provided to larvae as small disks. Food was provided regularly as depleted and fecal pellets were removed with a pipette, and treatment water replaced daily to prevent fouling. Third instar growth rates were measured at intervals for each larva over the initial 2–3 weeks after molt (at 3, 6, 9, and 16 days). Each larva was rinsed briefly in distilled water, blotted dry, and weight determined within 10–15 s to ±10 micrograms using a digital Cahn Electrobalance, then returned to culture dishes. As third instars continued to develop, the time from molt to pupa formation (pupariation) was recorded for all maturing to that stage. The size at maturity of pupae was then measured with a microscope eyepiece reticle (to 0.01 mm) at the maximum dorso-ventral width of the puparium (between the 3rd and 4th prolegs).
Interaction of food supply and salinity on growth and development
The effect of food supply on larval survival and development was evaluated in experiments using individuals isolated as newly-hatched first instar larvae. Eggs were obtained from 20 adults reared in the lab (size range 4.8–5.8 mm) from field-collected pupae at Mono Lake (netted from pupae detached from rock in near-shore shallows), harvesting eggs laid into an algae-sediment substrate in screened jars which held the emerged mix of females with male adult flies. Food supply to isolated first instar larvae was provided in the form of algal-microbial mats collected from the field (suctioning off surface sediments in shallows) regularly in protected areas of submerged shallows in Mono Lake. This consisted typically of a mix of diatoms (Nitzschia spp.), green algae (Ctenocladus circinnatus), cyanobacteria (mostly Oscillatoria) and undetermined bacteria. Collected food was first homogenized in a low-speed blender, centrifuged, and the pellet delivered by small syringe into multi-well culture plates with a single larva in 2 mL treatment water. For the first 2 days after hatching, all larvae were given unlimited access to food. Thereafter, larvae were randomly assigned to daily food ration, or a ration of 2 days with food followed by 2 days without. In each case, these were conducted in replicates at three salinity levels—50, 100 and 125 g L−1 (larvae could not complete development at 150 g L−1). Water was replaced daily to prevent fouling, and the experiments were again conducted at 20°C and 14:10 L:D photoperiod. Survivorship curves were plotted for each food availability x salinity treatment, along with records of the time to pupa formation (pupariation). Adult emergence success as a function of pupa size was determined both from the pupae formed from this experiment and the larval growth study, and supplemented with data from a parallel experiment with Abert larvae, and field-collected pupae from both Mono Lake and Lake Abert.
Adult fecundity and proximate chemical analysis of food value between lakes
To determine the relationship between size of adult females and fecundity, summer field-collections from both Mono and Abert Lake were made in sweep-nets along shores, and the abdomens of preserved female flies dissected and number of eggs in ovaries counted in each. The relative food value to birds of larvae, pupae and adults from both lakes was also determined from field-collections of all these life stages, and proximate chemical analysis performed as follows. Ash was determined by dry weight difference before and after combustion at 550°C for 3 h. Total organic nitrogen was determined by the micro-Kjeldahl method (APHA Standard Methods). Total protein was estimated from nitrogen content by the conversion factor of 6.25. Water soluble protein was determined by the dye-binding assay of Bradford (1976). Water soluble carbohydrate was determined spectrophotometrically by reaction with phenol (Kochert, 1978). Lipid was determined by weight before and after Soxhlet extraction for 20–24 h in 2:1 chloroform:methanol. Caloric content was calculated from protein, carbohydrate, and lipid by standard conversion factors.
Results
Osmotic stress and larval growth and development
The net average growth rate over the initial 16 days from molt for third instar larvae decreased with salinity (Figure 2). Average growth rate was similar at 10 and 50 g L−1 (from 0.77 to 0.74 mg d−1, respectively), but decreased significantly at 100 and 150 g L−1 (0.48 to 0.34 mg d−1, two-tailed t-tests of pairwise comparisons of slopes). Initial molt weight of third instars from second instars also decreased with salinity (points on the Y-intercept).
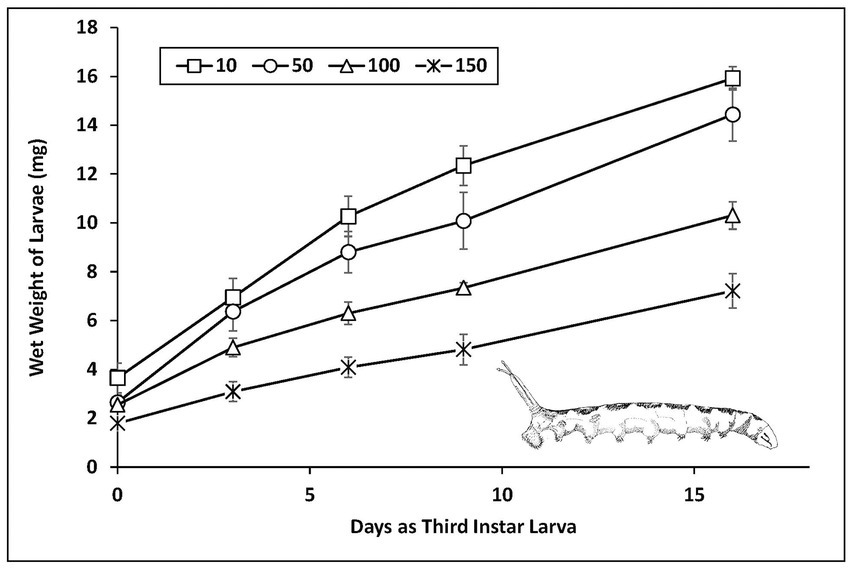
Figure 2. Growth rates of third instar alkali fly larvae exposed to a range of salinities of Mono Lake water. The number of larvae tracked through the 16th day of weighing trials were 12, 6, 7 and 4 at 10, 50, 100 and 150 g L−1 (standard error for each data point).
Duration of third instar development to pupation was prolonged with increased salinity (Figure 3). Development required on average about 3–4 weeks at 10 g L−1, 4–5 weeks at 50 g L−1, 5–6 weeks at 100 g L−1, and more than 6 weeks at 150 g L−1. Even though development time was extended in response to increased salinity, this did not result in achieving equal sizes at maturity. Puparium width decreased with increased salinity (Figure 4; ANOVA with analysis of variance Fisher’s least significant difference test of multiple comparisons of means by salinity: 10 = 50 > 100 > 150). Noted also on Figure 4 is the threshold pupa size (1.6–1.7 mm width) at which adult emergence success drops from greater than 75% to less than 50% (Figure 5). Almost no emergence occurs below a size of 1.5 mm width, but when successful took 10–14 days regardless of salinity treatment. In sum then, salinity increase leads to reduced larval growth rates, prolonged development, smaller size at maturity, and reduced success of adult emergence from smaller pupae.
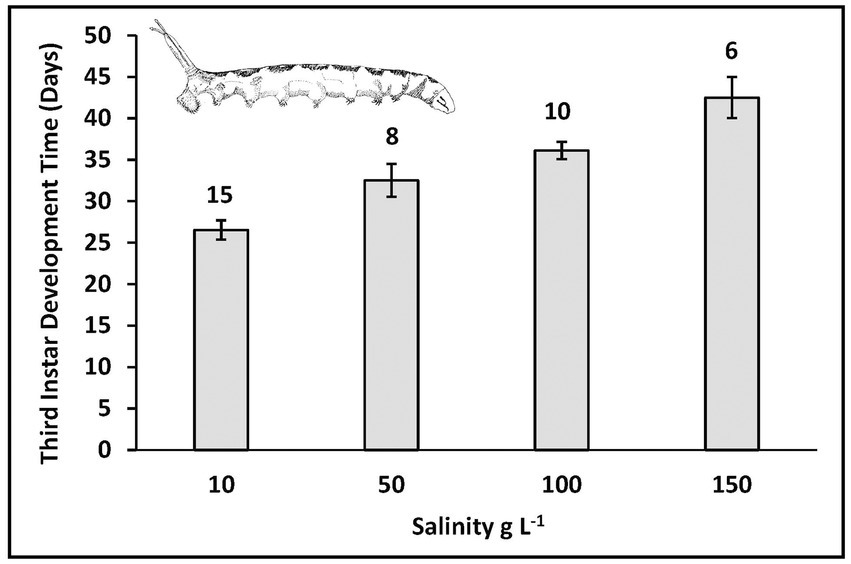
Figure 3. Development time of third instar larvae from molt to pupariation at varied salinities of Mono Lake water. Number of larvae tracked to pupariation, and standard errors for each bar.
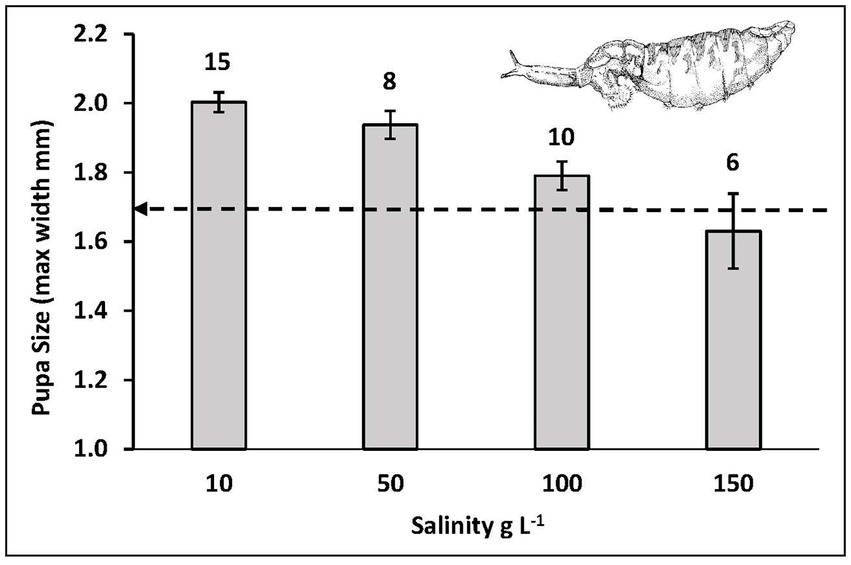
Figure 4. Size at maturity of pupae forming from third instar growth experiment at varied salinities of Mono Lake water (dashed line indicates threshold below which emergence success falls below 50%, in Figure 5). Standard error and sample size as in Figure 3 above each bar.
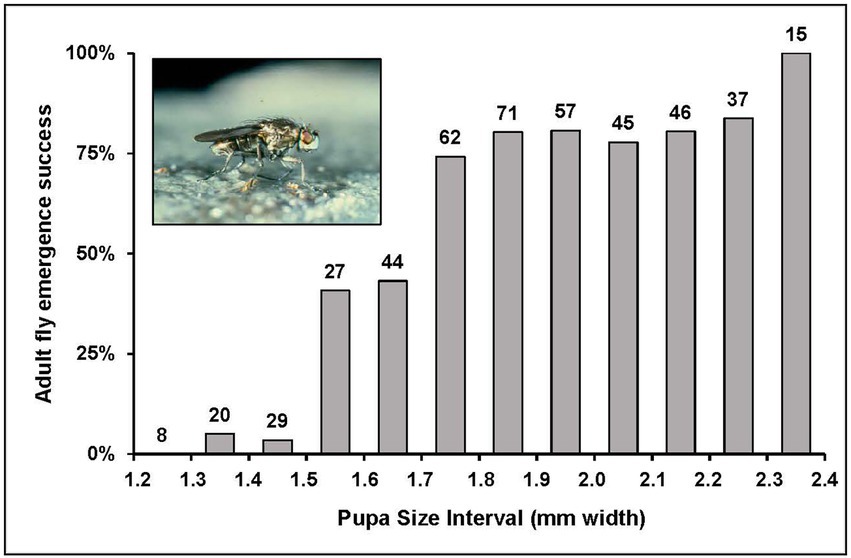
Figure 5. Percent adult emergence success from pupae of varied size, with standard error and sample size above each bar. Pupae derived from experimental exposures of larvae to varied salinity along with pupae collected from both Mono Lake and Lake Abert.
Combined effects of food limitation and salinity on survival and development
Survivorship curves of Mono Lake larvae showed that rearing at reduced algal food availability resulted in substantial mortality, especially among early instars (Figure 6). The detrimental effects of food limitation were more pronounced at elevated salinities of 100 and 125 g L−1 where no larvae were capable of surviving unless food was available at all times. Only at 50 g L−1 were larvae able to pupate on a reduced food ration but even so, this experiment was terminated beyond 160 days duration. The larvae that had pupated in the reduced food availability level at 50 g L−1 were delayed by nearly 3 months relative to those that had been fed full-time and were significantly smaller (average of 1.37 mm, n = 4 vs. 1.67 mm, n = 30), and most pupae perished if they formed after 3 months. At the full-time food availability level at 50 g L−1 86% of larvae survived to pupate and started forming after 42 days, with all forming before 3 months emerging as adults. When food was reduced only 12% of larvae survived to pupate and these were delayed by over 2 months. At higher salinities and daily food ration, onset of pupa formation was also delayed until after 75 days at 100 g L−1 and 90 days at 125 g L−1. Early stage larvae (instars 1 and 2) took 23 days on average to molt to the third instar at 50 g L−1 but took twice as long (46 days) when food ration was reduced. At higher salinities with daily food, early instars required 35–37 days on average to reach the third instar stage.
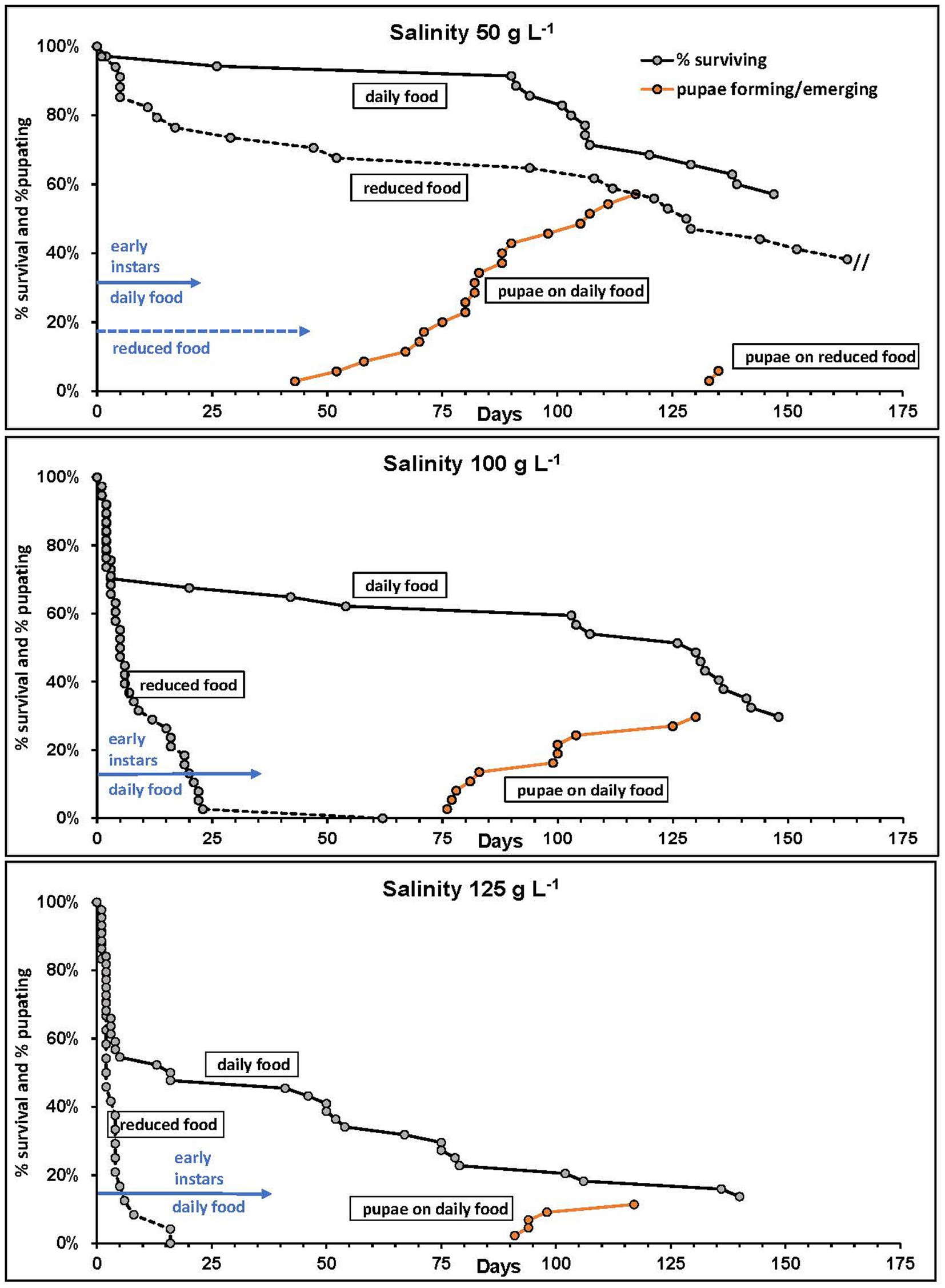
Figure 6. Mono Lake alkali fly larval survivorship and development time to pupation at varied levels of salinity and food ration. Curves refer to algal food supplied daily or reduced to 2 of 4 days. Initial sample size of of first instar larvae in each salinity at daily and reduced food ration, respectively: 50 (35,34), 100 (37,38), 125 (44,24). Experiment at reduced food level in 50 g L−1 was terminated after 160 days due to slow growth.
Size and fecundity of adult females and proximate chemical analysis of all life stages
A sampling of field-collected adult flies from both Mono and Abert showed that as body size of adult females increases, so does the number of eggs in the ovaries (Figure 7). Eggs were always of constant size, so this was not affected by field or lab conditions. For this wedge-shaped distribution, it is the upper limits with respect to body size that reflects potential maximum fecundity because many of the field-collected females no doubt had already begun laying some eggs.
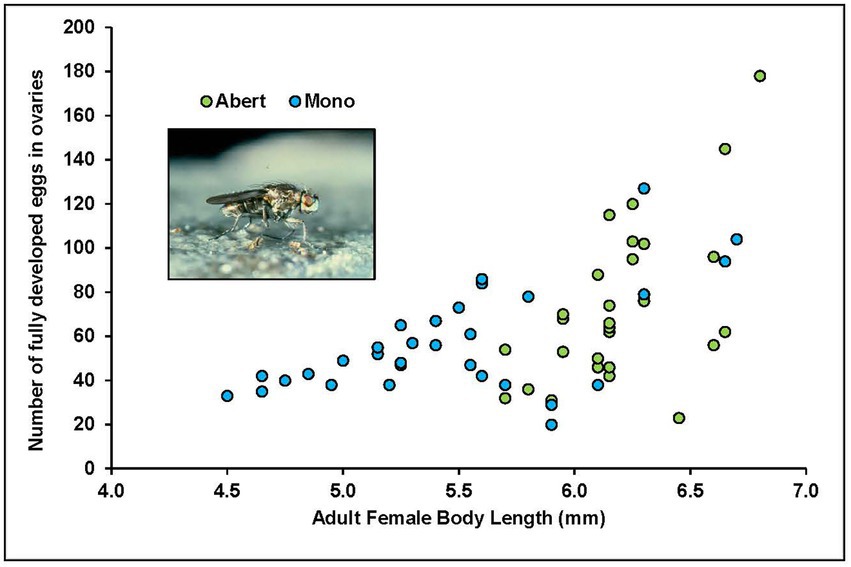
Figure 7. Fecundity of field-collected adult females from both Mono and Abert, from dissections of abdomen and counts of eggs present. Abert green points, Mono blue points.
Pupae and adults of C. hians from Lake Abert were significantly larger than their Mono Lake counterparts, consistent with the lower salinity of Abert, but had similar digestible chemical composition (Table 1, as percent water soluble fractions of protein, carbohydrate, and lipid). Third instar larvae were more variable in composition, most notable being a higher ash content in Mono larvae (24.5% vs. 9.0% at Abert), and lower lipid content (16.3% vs. 28.0% at Abert). For contrast, data from Artemia collected at Mono Lake are also reported (Table 1; Enzler et al., 1974) and indicates these have lesser lipid and caloric content than aquatic larvae or pupae of C. hians, but similar food value as adult flies.
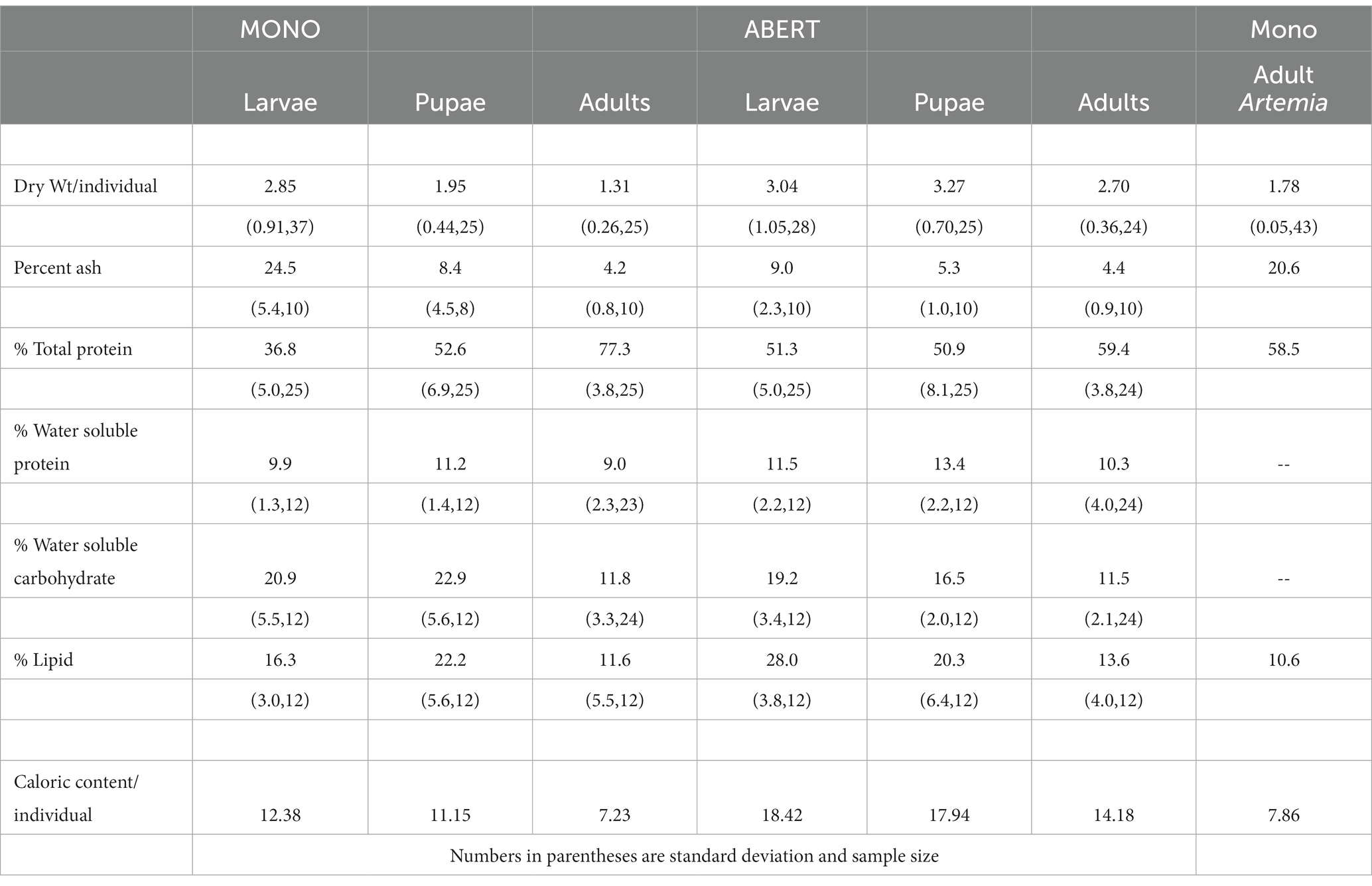
Table 1. Potential food value to birds foraging at lakes of differing salinity. Indicated here by proximate chemical analysis comparing life stages of Ephydra hians collected from Mono Lake with same from Lake Abert, and to Artemia brine shrimp from Mono Lake.
Discussion
The important applications of this study are to provide insights to why and where birds are using saline lakes for foraging. The alkali fly can be an abundant inhabitant of saline Great Basin lakes and ponds and this research shows the underlying basis for how population demography, productivity and distribution can be related to the physiological costs of living over gradients of varied salinity. The strength of this research derives from combining lab and field observations along with contrasts between lakes with differing salinity regimes. This approach is further validated because the studies follow salinity effects over the full life cycle of this insect (larvae to pupae and adults) and verifies experimental results with field observations. A limitation of the study is that it applies only to this insect, and other salt lake invertebrates with differing tolerances of salinity and water chemistry may show different optima. However, the general principle of developmental costs associated with osmoregulation will still apply.
Growth and development of larvae and pupae under osmotic stress
Assimilated food is allocated according to differing conditions of quantity and quality of food consumed, and how energetic needs and trade-offs are divided among metabolic regulatory functions, and requirements for growth, storage, and reproduction (the principle of allocation sensu Sibly and Calow, 1986). This partitioning determines life history traits such as development time, size at maturity, and fecundity along with the capacity for adaptation to varied environmental conditions. The gradual inhibition of larval growth observed with increased salinity level is consistent with the expectation that increased osmoregulatory costs creates a deficit in resources that can be allocated for growth (Figures 2–4). Larval growth was limited not only by slower rates of weight gain with increasing salinity, but also because second instars acclimated to treatment salinities produced smaller size of third instars at molt. This is a conservative estimate then of how salinity can disrupt growth over all larval stages. Indeed, the experiments using larvae reared from egg hatching on showed how severely survivorship and development rates are curtailed (Figure 6).
At a salinity near iso-osmotic (10 g L−1) where the theoretical cost of osmoregulation should be at a minimum, the highest growth rates, shortest development time and largest size at maturity were achieved. As growth rates are inhibited with increase in salinity it is conceivable that some compensation for lost growth could occur by extending development time. Such prolonged growth at higher salinity does indeed occur (Figure 3) but not enough to maintain a constant body size (Figure 4). At the highest salinity (150 g L−1), this size was actually below a threshold at which emergence success of adult flies from pupae falls below 50% (Figure 5).
Although the lowest salinity tested appears to be the condition under which these salt lake insects would most thrive, in nature these are conditions where other less salt-tolerant insects and other invertebrates (such as amphipods, damselflies, corixids) can also occupy these habitats and restrict population productivity due to competition and predation (Herbst, 1988, 2001, 2006). Given these ecological constraints, the experiments reported here, and observations of comparative population ecology, the salinity optimum for productivity of the alkali fly appears to be in the range of 25–100 g L−1, with a maximum near 50 g L−1.
Food limitation substantially reduced survival and delayed maturation of larvae, and when combined with increased salinity from 50 to 100 and 125 g L−1, prevented any further development to the pupa stage. The food limits imposed in these experiments were severe—with food provided only half the time larvae were trying to grow. While this may be an unrealistic situation, it does show the importance of food supply in offsetting the effects of salinity stress, but may still represent how salinity can reduce the quantity and quality of algae food resource available. In unialgal cultures of the filamentous green algae C. circinnatus, growth rates in lake water from Mono or Abert were reduced by half or more as salinity increased from 50 to 75 or 100 g L−1 and also resulted in changes in the growth form of this species, commonly found at low to moderate salinities in saline lakes (Herbst and Castenholz, 1994). In mixed algae growing in experimental field mesocosms, the production of an assemblage of diatoms, Ctenocladus, and cyanobacteria decreased by far more than half and diatom diversity reduced as salinities of Mono Lake water were increased from 50 to 75 or 100 g L−1 (Herbst and Blinn, 1998). Lab studies of mixed algae and microbial cultures from both lakes indicated somewhat broader salinity tolerance (Herbst and Bradley, 1989b), possibly due to greater salt tolerance of the cyanobacteria present. Altogether, these studies suggest algae food resource restrictions may not be an unreasonable expectation as salinities increase. The primarily diatom-based cultures provided as food in growth experiments has been determined to provide for the best larval growth (Bradley and Herbst, 1994), but even when fed ad libitum still does not compensate for salinity limitations on survival and development (Figure 6).
The life history correlation of decreasing survivorship of larvae with slower growth rates and smaller size at maturity conforms to how fruit flies (Drosophila) respond to crowding or poor food conditions, and is a predictable “reaction norm” to stress (Stearns and Koella, 1986). This variable growth pattern whereby size at maturity is flexible can be interpreted as an adaptation for survival in the face of osmotic stress in brine flies. The brine fly E. gracilis (nee Ephydra cinerea) living in Great Salt Lake (Utah) also shows plasticity in development and smaller size at maturity when stressed by low food availability (Collins, 1980b). If direct effects of salinity on development depend on osmoregulatory costs, then high hemolyph osmotic concentration would lower the gradient between internal and external fluids and should confer greater resistance to the stress of increased salinity on growth and survival. This appears to be exactly the case with E. gracilis larvae, having a hemolymph osmolality approximately three-fold higher than in C. hians, and are capable of living in much higher salinities, up to and beyond 200 g L−1 salinity of the sodium chloride-rich waters of Great Salt Lake (Nemenz, 1960; Herbst, 1999). Although they have a greater salt tolerance, E. gracilis also show reduced size at maturity with increased salinity (Herbst, 2006), but do not occur in the alkaline water chemistry of salt lakes such as Mono or Abert. Plasticity in growth and development then may permit some degree of adaptability to salinity stress, but not without a cost that would result in demographic limitations on population productivity.
Corroborating field observations
The altered life history traits produced under experimental treatments of increased salinity are consistent with observed differences in these characteristics from collections at Mono Lake and Lake Abert (Table 1). Adults and pupae from the less saline Abert (~25 g L−1) were significantly larger than specimens collected from Mono Lake at the same time (~90 g L−1). Adult flies collected from both lakes covered a broad range of body sizes and egg-laying capacity was obviously dependent on the general relationship to body size (Honĕk, 1993). This important life history attribute then is related to how both salinity and food limitations can delay the time of reproduction through prolonged development and maturation to the pupa stage, and lower fecundity owing to reduced pupa size from which smaller adult size will result. Smaller adults will emerge from smaller pupae, so these limitations on reproduction extend the influence of rising salinity in demographic constraints on productivity.
Although the proximate analysis of chemical composition of field-collected specimens showed percent digestible (water soluble) fractions of tissue to be similar in pupae and adults from both lakes, third instar larvae differed. Abert larvae stored significantly more lipid, important both in growth and later vitellogenesis in adults, but also giving them a higher caloric content and fat storage value to birds consuming them. Mono larvae had not only lower lipid content but higher indigestible ash fraction, likely related to the greater inorganic fraction of CaCO3 concretions stored in the lime glands of larvae from more saline waters. To the extent that prey type and capture by birds is similar between lakes, foraging on any life stage of the flies would be less productive at Mono than Abert under these conditions (as in Herbst, 2006).
Conclusion: Conservation, or not
Since 1994, partial protection of stream inflows to Mono have been in place intended to raise lake elevation to 6,292 ft. (about 75 g L−1). With intervening drought, however, this has not been achieved. Without these protections ordered by the State Water Board of California the lake level would by now have dropped to around 6,350 ft. (in excess of 150 g L−1). Abert has no such protections in place and recently has been near dry, all but for inches of water near freshwater seeps at the northeast edge of the lakebed. With a shallower profile and smaller volume than Mono, restoring inflows of the Chewaucan River to Abert could raise lake levels and reduce salinity to viable conditions (Figure 1B), with flies re-inhabiting the lake from refuge habitats created by salinity gradients within lakebed margin seeps and springs. At Mono, where some stream diversions to the Los Angeles aqueduct are still permitted, leaving those to flow to the lake would achieve the mandated water level in about 30 years (Mono Lake Committee, 2022).
Other saline lakes of the Great Basin are in similarly dire condition, with lake levels falling to historic lows at the Great Salt Lake and Walker Lake in Nevada (Herbst et al., 2013). Impaired survival of the benthic littoral assemblage of midges and damselfly nymphs at Walker Lake over lower ranges of salt concentration than are optimal for brine flies illustrates the fact that salinity management is not “one size fits all.” Conservation of saline lake ecosystem networks should consider the ranges of water chemistry, native aquatic life in each, and historic conditions with and without diversions of inflowing rivers and streams that sustain productivity, diversity, and habitat value to birds (Wurtsbaugh et al., 2017). Major shifts in the utilization and connections among saline wetlands for migratory waterbirds of the Great Basin appears to be related to reduced availability and salinization of these habitats (Haig et al., 2019). In addition to their value as food resource to birds visiting saline lakes, these halophilic invertebrates are of concern in their own right, often with prolific populations being limited to just the few larger more perennial lakes. Where present in smaller and refuge aquatic habitats they may be subject to the genetic bottlenecks of small populations and temporary availability of optimal environmental conditions. Conservation of large perennial saline lake habitats provides protection for multiple ecosystem types and species.
Although the drying of Owens Lake (California) is sometimes cited as a cautionary tale for the demise of other saline lake ecosystems, it is actually an example of how rapidly the ecological values of these lakes can be recovered. The Owens basin had been regarded as a problematic dry lakebed emitting dust pollution during wind storms, but under the requirements of federal air quality standards, the Los Angeles Department of Water and Power was ordered to control dust emissions from the Owens dry playa. Using a system of flood irrigation, not only was dust pollution abated in large part, but in the process shallow saline water habitat was recreated in which algae and brine flies flourished (Herbst and Prather, 2014). When proper water quality returns so does the life of saline lakes. The lake now hosts an annual bird festival.1 Add water and they will come.
Data availability statement
The raw data supporting the conclusions of this article will be made available by the authors, without undue reservation.
Author contributions
The author confirms being the sole contributor of this work and has approved it for publication.
Acknowledgments
I am grateful to Faye Cummins for her excellent artwork in Figures 2–4 depicting larvae and pupae of the alkali fly. Portions of this work are drawn from the PhD dissertation of the author (Herbst, 1986).
Conflict of interest
The author declares that the research was conducted in the absence of any commercial or financial relationships that could be construed as a potential conflict of interest.
Publisher’s note
All claims expressed in this article are solely those of the authors and do not necessarily represent those of their affiliated organizations, or those of the publisher, the editors and the reviewers. Any product that may be evaluated in this article, or claim that may be made by its manufacturer, is not guaranteed or endorsed by the publisher.
Footnotes
References
Aldrich, J. M. (1912). The biology of some western species of the dipterous genus Ephydra. J. New York Entomol. S 20, 77–99.
Andrei, A. E., Smith, L. M., Haukos, D. A., Surles, J. G., and Johnson, W. P. (2009). Foraging ecology of migrant shorebirds in saline lakes of the southern Great Plains. Waterbirds 32, 138–148. doi: 10.1675/063.032.0117
Barnby, M. A. (1987). Osmotic and ionic regulation of two brine fly species (Diptera: Ephydridae) from a saline hot spring. Physiol. Zool. 60, 327–338. doi: 10.1086/physzool.60.3.30162286
Bradford, M. M. (1976). A rapid and sensitive method for the quantitation of microgram quantities of protein utilizing the principle of protein-dye binding. Anal. Biochem. 72, 248–254. doi: 10.1016/0003-2697(76)90527-3
Bradley, T. J. (1987). Physiology of osmoregulation in mosquitoes. Annu. Rev. Entomol. 32, 439–462. doi: 10.1146/annurev.en.32.010187.002255
Bradley, T. J., and Herbst, D. B. (1994). Growth and survival of larvae of Ephydra hians say on unialgal diets. Environ. Entomol. 23, 276–281. doi: 10.1093/ee/23.2.276
Collins, N. C. (1980a). Population ecology of Ephydra cincerea Jones (Diptera: Ephydridae), the only benthic metazoan of the great salt Lake, U.S.a. Hydrobiologia 68, 99–112. doi: 10.1007/BF00019696
Collins, N. C. (1980b). Developmental responses to food limitation as indicators of environmental conditions for Ephydra cinerea Jones (Diptera). Ecology 61, 650–661. doi: 10.2307/1937431
Dana, G. L., and Lenz, P. H. (1986). Effects of increasing salinity on an Artemia population from mono Lake, California. Oecologia 68, 428–436. doi: 10.1007/BF01036751
Elphick, C. S., and Rubega, M. A. (1995). Prey choices and foraging efficiency of recently fledged California gulls at mono Lake, California. Great Basin Nat. 55, 363–367.
Enzler, L., Smith, V., Lin, J. S., and Olcott, H. S. (1974). Lipids of mono Lake, California, brine shrimp (Artemia salina). J. Agric. Food Chem. 22, 330–331. doi: 10.1021/jf60192a017
Frank, M. G., and Conover, M. R. (2021). Foraging behavior of red-necked (Phalaropus lobatus) and Wilson’s (P.tricolor) phalaropes on great salt Lake, Utah. Wilson J. Ornithol. 133, 538–551.
Haig, S. M., Murphy, S. P., Matthews, J. H., Arismendi, I., and Safeeq, M. (2019). Climate-altered wetlands challenge waterbird use and migratory connectivity in arid landscapes. Sci. Rep. 9:4666. doi: 10.1038/s41598-019-41135-y
Hardie, L. A., and Eugster, H. P. (1970). The evolution of closed-basin brines. Mineral Soc. Am. Spec. Pap. 3, 273–290.
Herbst, D. B. (1986). Comparative studies of the population ecology and life history patterns of an alkaline salt lake insect: Ephydra (Hydropyrus) hians say (Diptera: Ephydridae). PhD dissertation. Corvallis OR: Oregon State University.
Herbst, D. B. (1988). Comparative studies of the population ecology and life history patterns of an alkaline salt Lake insect: Ephydra (Hydropyrus) hians say (Diptera: Ephydridae) at mono Lake (California) and Abert Lake (Oregon). Hydrobiologia 158, 145–166. doi: 10.1007/BF00026273
Herbst, D. B. (1999). Biogeography and physiological adaptations of the brine fly genus Ephydra (Diptera: Ephydridae) in saline waters of the Great Basin. Great Basin Nat. 59, 127–135.
Herbst, D. B. (2001). Gradients of salinity stress, environmental stability and water chemistry as a templet for defining habitat types and physiological strategies in inland salt waters. Hydrobiologia 466, 209–219. doi: 10.1023/A:1014508026349
Herbst, D. B. (2006). Salinity controls on trophic interactions among invertebrates and algae of solar evaporation ponds in the Mojave Desert and relation to shorebird foraging and selenium risk. Wetlands 26, 475–485. doi: 10.1672/0277-5212(2006)26[475:SCOTIA]2.0.CO;2
Herbst, D. B., and Blinn, D. W. (1998). Experimental mesocosm studies of salinity effects on the benthic algal community of a saline lake. J. Phycol. 34, 772–778. doi: 10.1046/j.1529-8817.1998.340772.x
Herbst, D. B., and Bradley, T. J. (1989a). A Malpighian tubule lime gland in an insect inhabiting alkaline salt lakes. J. Exp. Biol. 145, 63–78. doi: 10.1242/jeb.145.1.63
Herbst, D. B., and Bradley, T. J. (1989b). Salinity and nutrient limitations on growth of benthic algae from two alkaline salt lakes of the western Great Basin (USA). J. Phycol. 25, 673–678. doi: 10.1111/j.0022-3646.1989.00673.x
Herbst, D. B., and Bradley, T. J. (1993). A population model for the alkali fly at mono Lake: depth distribution and changing habitat availability. Hydrobiologia 267, 191–201. doi: 10.1007/BF00018801
Herbst, D. B., and Castenholz, R. W. (1994). Growth of the filamentous geen alga Ctenocladus circinnatus (Chaetophorales: Chlorophyceae) in relation to environmental salinity. J. Phycol. 30, 588–593. doi: 10.1111/j.0022-3646.1994.00588.x
Herbst, D. B., Conte, F. P., and Brookes, V. J. (1988). Osmoregulation in an alkaline salt lake insect Ephydra (Hydropyrus) hians say (Diptera: Ephydridae) in relation to water chemistry. J. Insect Physiol. 34, 903–909. doi: 10.1016/0022-1910(88)90125-4
Herbst, D. B., and Prather, M. (2014). Owens Lake—from dustbowl to mosaic of salt water habitats. Lake Line Mag. 34, 34–38.
Herbst, D. B., Roberts, S. W., and Medhurst, R. B. (2013). Defining salinity limits on the survival and growth of benthic insects for the conservation management of saline Walker Lake, Nevada, USA. J. Insect Conserv. 17, 877–883. doi: 10.1007/s10841-013-9568-6
Honĕk, A. (1993). Intraspecific variation in body size and fecundity in insects: a general relationship. Oikos 66, 483–492. doi: 10.2307/3544943
Jehl, J. R. (1994). Changes in saline and alkaline lake avifaunas in western north American in the past 150 years. Stud. Avian Biol.-Ser. 15, 258–272.
Kochert, G. (1978). “Carbohydrate determination by the phenol-sulphuric acid method,” in Handbook of Phycological Methods, Physiological and Biochemical Methods. eds. J. A. Hellebust and J. S. Craigie (Cambridge, England: Cambridge University Press), 96–97.
Larson, R., Eilers, J., Kreuz, K., Pecher, W. T., DasSarma, S., and Dougill, S. (2016). Recent desiccation-related changes at Lake Abert, Oregon: a terminal alkaline salt lake. West N Am. Nat. 76, 389–404. doi: 10.3398/064.076.0402
Mono Lake Committee. (2022). Modeling mono Lake (scenarios for water diversions). https://www.monolake.org/today/raising-mono-lake-requires-a-new-plan/.
Nayar, J. K. (1969). Effects of larval and pupal environmental factors on biological status of adults at emergence in Aedes taeniorhynchus (Wied.). B Entomol. Res. 58, 811–827. doi: 10.1017/S0007485300056054
Nemenz, H. (1960). On the osmotic regulation of the larvae of Ephydra cinerea. J. Insect Physiol. 4, 38–44. doi: 10.1016/0022-1910(60)90066-4
Prosser, C. L. (Ed.) (1973). “Comparative animal physiology” in Environmental Physiology, vol. 1. 3rd ed (Philadelphia, PA: WB Saunders Co.)
Rosenberg, K. V., Dokter, A. M., Blancher, P. J., Sauer, J. R., Smith, A. C., Smith, P. J., et al. (2019). Decline of the north American avifauna. Science 366, 120–124. doi: 10.1126/science.aaw1313
Rubega, M. A., and Robinson, J. A. (1996). Water salinization and shorebirds: emerging issues. Int. Wader Stud. 9, 45–54.
Seager, R., Ting, M., Held, I., Kushnir, Y., Lu, J., Vecchi, G., et al. (2007). Model projections of an imminent transition to a more arid climate in southwestern North America. Science 316, 1181–1184. doi: 10.1126/science.1139601
Senner, N. R., Moore, J. N., Seager, S. T., Dougill, S., Kreuz, K., and Senner, S. E. (2018). A salt lake under stress: relationships among birds, water levels and invertebrates at a Great Basin saline lake. Biol. Conserv. 220, 320–329. doi: 10.1016/j.biocon.2018.02.003
Sibly, R. M., and Calow, P. (1986). Physiological Ecology of Animals: An Evolutionary Approach. (Oxford. Blackwell Scientific).
Stearns, S. C., and Koella, J. C. (1986). The evolution of phenotypic plasticity in life-history traits: predictions of reaction norms for age and size at maturity. Evolution 40, 893–913. doi: 10.1111/j.1558-5646.1986.tb00560.x
Strauss, E. A., Ribic, C. A., and Shuford, W. D. (2002). Abundance and distribution of migratory shorebirds at mono Lake, California. Western Birds 33, 222–240.
Wirth, W. W. (1971). The brine flies of the genus Ephydra in North America (Diptera: Ephydridae). Ann. Entomol. Soc. Am. 64, 357–377. doi: 10.1093/aesa/64.2.357
Wurtsbaugh, W. A., Miller, C., Null, S. E., DeRose, R. J., Wilcock, P., Hahnenberger, M., et al. (2017). Decline of the world’s saline lakes. Nat. Geosci. 10, 816–821. doi: 10.1038/ngeo3052
Keywords: osmoregulation, salinity, Ephydra, Mono Lake, salt lakes, Cirrula hians, Lake Abert
Citation: Herbst DB (2023) Developmental and reproductive costs of osmoregulation to an aquatic insect that is a key food resource to shorebirds at salt lakes threatened by rising salinity and desiccation. Front. Ecol. Evol. 11:1136966. doi: 10.3389/fevo.2023.1136966
Edited by:
Jacquelyn K. Grace, Texas A&M University, United StatesReviewed by:
Brianna R. Beechler, Oregon State University, United StatesJulien Pétillon, University of Rennes 1, France
Copyright © 2023 Herbst. This is an open-access article distributed under the terms of the Creative Commons Attribution License (CC BY). The use, distribution or reproduction in other forums is permitted, provided the original author(s) and the copyright owner(s) are credited and that the original publication in this journal is cited, in accordance with accepted academic practice. No use, distribution or reproduction is permitted which does not comply with these terms.
*Correspondence: David B. Herbst, aGVyYnN0QHVjc2IuZWR1