- 1Behaviour and Ecology Lab, Department of Biological Sciences, Indian Institute of Science Education and Research, Kolkata, India
- 2Laboratoire d’Ethologie Expérimentale et Comparée, Université Sorbonne Paris Nord, Villetaneuse, France
Introduction: The cognitive abilities of animals play a crucial role in their capacity to adapt to changing environments. Experiments to understand cognition are usually performed on animals such as non-human primates, canids, or corvids. These studies often use food as the motivating factor. However, in the current study, we used a new paradigm to investigate the ability of ponerine ants, who are solitary foragers, to solve a nature-inspired problem. As pupae are the most invested brood item and are directly associated with the colony’s fitness, we designed an experiment that presented a challenge to colony relocation by imposing restrictions on the transportation of pupae to the new nest.
Methods: We used a narrow nest entrance through which an adult could enter, but it rendered the passage of an adult holding a pupa virtually impossible. Through this, we examined the capacity of these ants to overcome the “pupal insertion” challenge by investigating eight colonies involving 425 pupal insertions performed by 104 uniquely labeled ants.
Results: We found that 80% of all pupae were inserted into the nest by employing a novel cooperative behavior: one ant pushed the pupa while the other ant(s) inside the nest pulled it. Even though individual ants showed high variability in their performance regarding pupal insertion, the initial three successive insertions showed a significant improvement in their performance. Such improvement was also documented at the colony level, with shorter durations and fewer attempts for subsequent pupal insertions.
Discussion: Our study demonstrated that ants can overcome difficult environmental challenges through innovation and subsequently improve their performance without training or reward.
Introduction
Animals learn and integrate information in constantly changing environments to enhance their survival and fitness. Cognitive abilities constitute adaptive traits that allow organisms to learn and integrate information to perform tasks, such as foraging, nesting, selecting breeding sites, avoiding predators, acquiring mates, or communicating optimally. The cognitive abilities of primates, including humans, and other vertebrates have been extensively explored (Kummer and Goodall, 1985; Marler, 1991; Bateson, 2002; Sol et al., 2002; Sol et al., 2005; Reader and Laland, 2003; Amiel et al., 2011; Benson-Amram and Holekamp, 2012; Cole and Quinn, 2012; Pritchard et al., 2016; Preiszner et al., 2017; Huebner et al., 2018; Chow et al., 2021; Santana and Garcia-Mijares, 2022).
The cognitive capacities of insects, which comprise the largest group of invertebrates, have, however, been explored to a much lesser extent, possibly due to their simple nervous systems and miniature brains (Chittka and Rossi, 2022). Nevertheless, recent findings suggest that the complexity of insect societies is not solely based on innate behavior, as they exhibit some features of social intelligence, like vertebrates, including recognition of individuals, object manipulation learning, cultural traditions, and emotion-like states. This highlights the need to explore the cognitive abilities of social insects in unusual situations, which could even be experimentally generated but possibly never examined earlier (Giurfa et al., 2001; Giurfa, 2013; Giurfa, 2019; Reznikova, 2018; Chittka and Rossi, 2022).
Ants are eusocial insects and all 12,000 known species of ants exhibit the hallmarks of eusociality, including reproductive division of labor, cooperative alloparental brood care, and overlapping generations (Hölldobler and Wilson, 1990). Ants, living in complex societies, are expected to have faced many challenges, but they appear to have been successful over their 150 million years on Earth, despite the changing environments that they must have confronted (Cole, 1985; Anderson and McShea, 2001). Some of the insights into the cognitive abilities of Hymenoptera have been obtained, for example, the cognitive differences between ants performing mass foraging and solitary foraging. Solitary hunters, such as members of the genus Myrmecia and subgenus Serviformica, are much more flexible than mass-foraging ants, as they independently search for food and make important decisions (Reznikova, 2018). Honeybee foragers can count, optimize paths, innovate, and even self-evaluate their chances of solving a task (Giurfa et al., 2001; Lihoreau et al., 2012; Perry and Barron, 2013; Loukola et al., 2017; Howard et al., 2018). Experiments have also been performed on insects under laboratory conditions to understand their ability to learn and retain their memory of coping with various environmental challenges (Papaj and Lewis, 1992; Collett et al., 2013; Giurfa, 2013; , 2019; Feinerman and Korman, 2017; Perry et al., 2017).
However, many laboratory-based mechanistic studies fail to consider the ecological contexts, within which animals have to solve specific problems. This limitation reduces the scope of insect-based cognition research in several ways, as it may hinder the ability of insects to perform cognitive tasks, as observed in Drosophila, honeybees, and bumblebees (Bateson et al., 2011; Yang et al., 2013). The current opinion in insect cognition research thus emphasizes prioritizing ecologically inspired experiments, which can be generated by closely observing insects in their natural habitat and understanding their real-life problems. Such experiments should be relevant to the umwelt of the organisms and should pose meaningful challenges (Lihoreau et al., 2019). At the collective level, for instance, social insects, such as bees and ants, generally make precise decisions for selecting their nesting site, sources of food, and solving mazes or transporting large food items as a group, rather than alone (Goss et al., 1989; Beckers et al., 1990; Czaczkes and Ratnicks, 2013; Sasaki et al., 2013; Mukhopadhyay et al., 2019). Hence, there is a need to understand the mechanisms that enhance performance in animal groups, when performing such tasks; some factors, such as learning from other group members or increased complementarity among group members, may contribute to such improvement in task performance (Collet et al., 2023).
The current study draws inspiration from observing Diacamma indicum colonies in their natural habitat. D. indicum is a metallic black, one-centimeter-long ant, found in India, Sri Lanka, and possibly Japan. They reside in colonies of approximately 85 adult ants, brood, and a single reproductively active female, known as the gamergate (Annagiri, 2021). When there is a disturbance at their nest or a better nesting option available, they relocate to a new nest, using tandem running recruitment. In tandem running, an informed ant (“leader”) recruits only one nestmate (“follower”) and walks her to the destination while maintaining tactile contact throughout the journey. This form of recruitment is used to bring nest mates to the new nest, to food sources or to slave colonies to raid them (Wilson, 1959; Franklin, 2014; Kaur et al., 2017).
During our earlier observations on colony relocation, we observed several tandem leaders and their followers accumulating at a narrow concrete crevasse, forming the new nest’s entrance, as it was a challenge to enter this narrow opening with their pupa (MP, pers. obs.). Generally, when ants relocate into a subterranean nest, the entrance dimension and other features of the nest, such as the tunnels and chambers, are often enlarged by excavating the surrounding soil. However, this may not always be possible, as we have seen in D. indicum colonies, residing at other locations, such as crevices inside tree trunks, bamboo stems, brick walls, or concrete slabs, which may pose various degrees of challenges to the ants in their efforts to redesign their new nest (Bhattacharyya et al., 2021). Observing the colony’s struggle to insert the pupa, we were inspired to design an experiment that utilized a narrow nest entrance, thus posing a cognitive challenge to the relocating colonies.
Our experimental design was unique in several ways. First, relocation is a goal-oriented and self-motivated process that forms an essential component in the life history of several social insects. It is often an adaptive response to factors, such as competition, nest deterioration, or colony expansion (McGlynn, 2012). Delaying the relocation process would expose the colony to both biotic and abiotic stress for relatively longer periods of time. Therefore, it becomes crucial to execute the relocation process efficiently to minimize the colony’s exposure to stress factors. Second, unlike other learning experiments, no training sessions were conducted. Colonies experienced the challenge at the start of relocation and had an opportunity to perform insertion as many times as the number of pupae within their colony. They were then tested with additional pupae in the control and manipulation experiments. Third, instead of using food, the most commonly used reward in cognition experiments, we utilized pupae, the most valuable item among the brood, as the source of motivation. Pupae require urgent and careful attention, as any damage to them during transportation would result in the loss of the investment in time, social care, and resources for the colony. Unlike food, which can be carried back piecemeal, pupae have to be kept intact. Pupae eventually eclose to forming the next generation of workers or reproductives for the colony and are thus linked to the colony’s fitness, making it unlikely that they would be abandoned. We, therefore, hypothesized that pupa transporters are likely to face much higher selection pressures than would food transporters because more is at stake for the former.
The combination of these features, we believe, creates a new paradigm to explore the cognitive abilities of these superorganisms, in general. More specifically, we examined how individual D. indicum ants and colonies were able to address this cognitive challenge and the manner in which they solved the problem.
Materials and methods
Ant colony collection and maintenance
We collected 26 colonies of the ponerine ant Diacamma indicum from their natural habitat in Mohanpur, Nadia district, West Bengal state, India (22° 57’ 47” N, 88° 31’ 38” E) from April to June 2021 for our cognitive experiments. We used the water-flooding method (Kaur et al., 2012) to collect the colonies from their subterranean nests. After the experiments, we released the colonies back into their natural habitat though not necessarily at the exact spots, where we had collected them but at nearby locations. We conducted qualitative observations to ensure that the ants relocated to a temporary shelter on their own but did not systematically track them later.
The collected colonies comprised adults (mean of 69.68 ± SD of 13.63, range = 41 to 96) and various stages of brood. We kept the ants inside a large plastic box (28.5 x 21.5 x 12 cm) with plaster-of-Paris (PoP) coating at its base. We provided the colonies with a separate artificial nest of a plastic Petri plate (radius = 45 mm) with a PoP coating at the bottom as well. The cover of the Petri plate was used as the roof of the artificial nest, which had a single entrance hole of diameter 1.7 cm. We covered the roof with a layer of red cellophane paper to make the nest chamber dark. The colonies were provided ant cake and water ad libitum and occasionally (about 2 times a week), small pieces of freshly killed earthworm (Bhatkar and Whitcomb, 1970). We maintained the colonies in a light:dark cycle of 12:12 h at an ambient temperature of 26°C and 70% relative humidity.
To enable individual identification, we color-coded all the ants in a colony with non-toxic enamel paint (Testors®, Rockford, IL, USA) on their first and second thoracic segments and abdomen. Colonies were given at least 24 h to acclimate to laboratory conditions before experimentation. We used 16 colonies for the experiments, with eight in the control and eight in the manipulation groups. We collected pupae from an additional 10 donor colonies to provide extra pupae for the second phase of the experiments. This was necessary due to the overall limited availability of pupae. As D. indicum is known to steal pupae from neighboring colonies during nest relocation in the wild (Paul and Annagiri, 2019), collecting pupae in this manner mimicked natural conditions rather than being an artificial stimulus.
We collected 433 pupae from all 26 colonies (16 ± 12.98, range = 0 to 59 pupae/colony) for the control and manipulation experiments, for each of which observations were conducted in two phases: (a) “relocation” and (b) “test”. The test phase was performed immediately after the relocation phase and consisted of providing additional pupae at the old nest. To ensure a sufficient supply of pupae in the test phase, we allowed colonies to carry an average of 12.37 (± 3.24) pupae in the relocation phase while about twice the number of pupae were supplied in the test phase (28 ± 4.20).
The number of adult ants (female workers) was similar in both the control (N = 8, mean ± SD of 74.5 ± 7.27, range = 41 to 96) and manipulation colonies (N = 8, 64.87 ± 17.1, range = 62 to 84; Mann-Whitney U-test, U = 15.5, p = 0.09). Each colony was used only once, for either the control or the manipulation experiment which ensured that each ant encountered the experimental setup for the first time at the start of this experiment.
Experimental set-up
We constructed an experimental arena, using plexiglass, which was filled with 1–2 cm of tap water (see Supplementary Figure S1 for the set-up and Figure S2 for the procedure). The new nest was placed 75 cm away from the old nest, with the entrance and roof of the nests above the water level and both were connected by a plexiglass bridge (75 × 2.5 cm) to ensure that the nests remained dry and the upper surface was above water level. This distance of 75 cm was chosen to simulate the relocation range of D. indicum in its natural habitat (Kaur et al., 2012). Thus, the ants could access the new nest and the bridge but could not escape due to the surrounding water. We performed two experiments using this setup: a control experiment and a manipulative experiment.
The nest entrance size in the control experiment was similar to that in the natural habitat (16.21 ± 0.11 mm; Bhattacharyya and Annagiri, 2019; Bhattacharyya et al., 2021). In the manipulative relocation experiment, however, the nest entrance size was narrower (3.40 ± 0.05 mm). This allowed for the easy movement of a single ant due to its flexible body but made it virtually impossible for an adult ant, holding a pupa in its mandible, to enter the new nest. We thus created this narrow nest entrance to present a cognitive challenge to the colonies regarding brood transport, particularly with reference to pupae, which represent the final and most invested developmental stage in these holometabolous insects. The pupae are typically blackish-brown and non-flexible, making them easy to spot in the colony (pupal width: 2.60 ± 0.20 mm, range = 2.24 to 3.16 mm, n = 22 pupae). By making the entrance of dimension 3.40 ± 0.05 mm, we found that pupae could be dropped into the entrance by aligning the pupal axis vertically with the entrance. The ants could not, however, march into the new nest while holding a pupa in their mandibles, as they usually do during tandem running (Kaur and Sumana, 2014).
Relocation phase and test phase
Relocation phase
To start each trial, we placed the colonies inside the experimental arena and allowed them to settle down for a few minutes (about 15 minutes). We then positioned a white light (9-watt LED bulb) above the old nest and removed its roof to stimulate the ants to explore the arena for potentially new nests.
Once the ants discovered the new nest, the adult females began tandem running into the new nest. The brood was transported by either carrying them in the mandibles of the transporters or of the leader or follower while tandem running. The latter method of transportation is known as couple adult-brood transport. If any males or stored resources were present, they were also carried in the mandibles (Kaur and Sumana, 2014).
Test phase
At the end of the relocation phase, we initiated the test phase. The end of the relocation phase was defined as when the last pupa of the ant colony had been transported to the new nest, with more than 90% of all the adults and pupae already inside the new nest, or when no transport occurred from the old nest for at least 10 min. In the test phase, we rotated the nest entrance away from the bridge by a random angle in the anti-clockwise direction, in multiples of 45°. This was done to prompt ants to search for the displaced entrance instead of using the exact location, as in the relocation phase, thus allowing us to test whether the ants had learned to overcome a specific challenge or if they could generalize the method to be followed. Colonies were provided with at least 25 more pupae in the test phase to assess if the ants could efficiently insert pupae, based on their experience during the colony relocation phase.
Behavioral observations
We used a Sony® Handycam (HDR-CX200) to video-record the proceedings at the new nest. An ethogram was created to catalog behaviors directly related to pupal transport into the new nest (Supplementary Table 1). The data were then carefully entered into Microsoft® Excel sheets for further analysis. Across all replicates, we noted the time of discovery of the new nest and the identity of the first brood transporter or tandem leader. The last tandem run or brood transport was recorded to mark the end of the transport phase.
We noted whether ants successfully inserted pupae into the narrow nest entrance or if they failed to do so, along with the participating ants’ identity and the duration of the event. We observed that the insertion of a pupa occurred either individually, without the aid of other ants, or cooperatively, with multiple ants actively participating in the process of pupal insertion. The former was called individual insertion while the latter was termed cooperative insertion (Supplementary Table 1). When multiple ants were involved, some ants initiated the process and pushed the pupa into the nest entrance while others pulled the pupa from inside the nest. The identity of the pushers and pullers and the duration of each insertion were noted. Some ants performed both the push and pull actions but, at different pupal insertion events, they only acted as either a pusher or a puller. Ants frequently failed to insert pupae into the nest entrance in the manipulative experiments. We compared the number of failed attempts before successful pupal insertion by the final pushers, between the relocation and the test phases.
In both experiments, we calculated the pupal transport time by dividing the total number of pupae in the relocation and test phases by the respective transport time in each phase. This normalization accounted for the variation in the number of pupae transported across replicates. We also measured the latency to take the first insertion attempt on a pupa after it was transported to the roof of the new nest, in both the relocation and the test phases of the manipulation experiment.
Statistical analysis
To test for differences between groups, we used the Mann-Whitney U-test to compare transport time (control vs manipulation experiments), pupal transport time (control vs manipulation experiments, during relocation and test phases), and failed attempts of pupal insertion (relocation vs test phases during manipulation experiments). We used the Friedman test to compare the different insertion modes during the relocation phase (control and manipulation experiments).
The specialization of the workforce into puller, pusher, or multi-tasker ants was estimated using the Kruskal-Wallis test, followed by a post-hoc test (Dwass-Steel-Critchlow-Fligner test) for pairwise comparison among them. In the manipulation experiments, we also performed the Wilcoxon paired-sample test for mean latency before each pupal insertion (relocation vs test phases).
We constructed a generalized linear mixed model (GLMM) to estimate total pupal insertion time. We used the R package ‘glmmTMB’ with the Poisson family, with pusher identity as a random factor (Brooks et al., 2017). The puller data were fed into this GLMM as a factor (one, two, or three pullers) to estimate the impact of the number of puller ants on insertion time.
Finally, we constructed another GLMM for failed attempts of pupal insertion as a response variable. The dataset for failed attempts contained excess zeros, so we used the R package ‘pscl’ with the function ‘zeroinfl’ and a negative binomial family for the zero-inflated regression model (Jackman et al., 2015).
We used the number of pullers and phase (relocation or test phase) as explanatory variables for both models. Colony identity and individual pusher identity were taken as random effects. We also analyzed inter- and intra-individual variation amongst the ants for their ability to successfully insert pupae during the manipulation experiments. We prepared a list of ants, who made at least three pupal insertions (N = 43 ants) and another list of all ants with a maximum of six pupal insertions (N = 13). The performance of these ants was analyzed by constructing GLMMs, with pupae inserted in sequence and failed attempts as fixed effects and pusher identity as a random effect (Hertel et al., 2020).
Furthermore, we examined the correlation, if present, between the sequence of pupal insertion attempts at the colony level and the number of attempts taken for insertion during the manipulation experiments, using Spearman’s correlation. We conducted all statistical analyses in R Studio with software R (version 4.0.4). Unless mentioned otherwise, we accepted the alternate hypothesis for p < 0.05, and reported the mean and standard deviation values, unless stated otherwise.
Results
Colony relocation and transport time
During the experiments, all ant colonies relocated cohesively to the new nests, along with their adults and brood (N = 8 control colonies and 8 manipulation colonies). The brood remained uninjured and there were no instances of cannibalism during the experiments. The transport time for the entire colony, including adults and brood, was significantly longer in the manipulation group (4890 ± 2330 s), as compared to the control group (1173 ± 400 s; Mann-Whitney U-test, U = 0, N = 8 for both control and manipulation groups, p < 0.01; Figure 1A). Additionally, the transportation time for pupae was significantly higher in the manipulation group (389 ± 143 s), compared to the control group (100 ± 42 s; U = 1, N = 8 colonies, p < 0.01; Figure 1B).
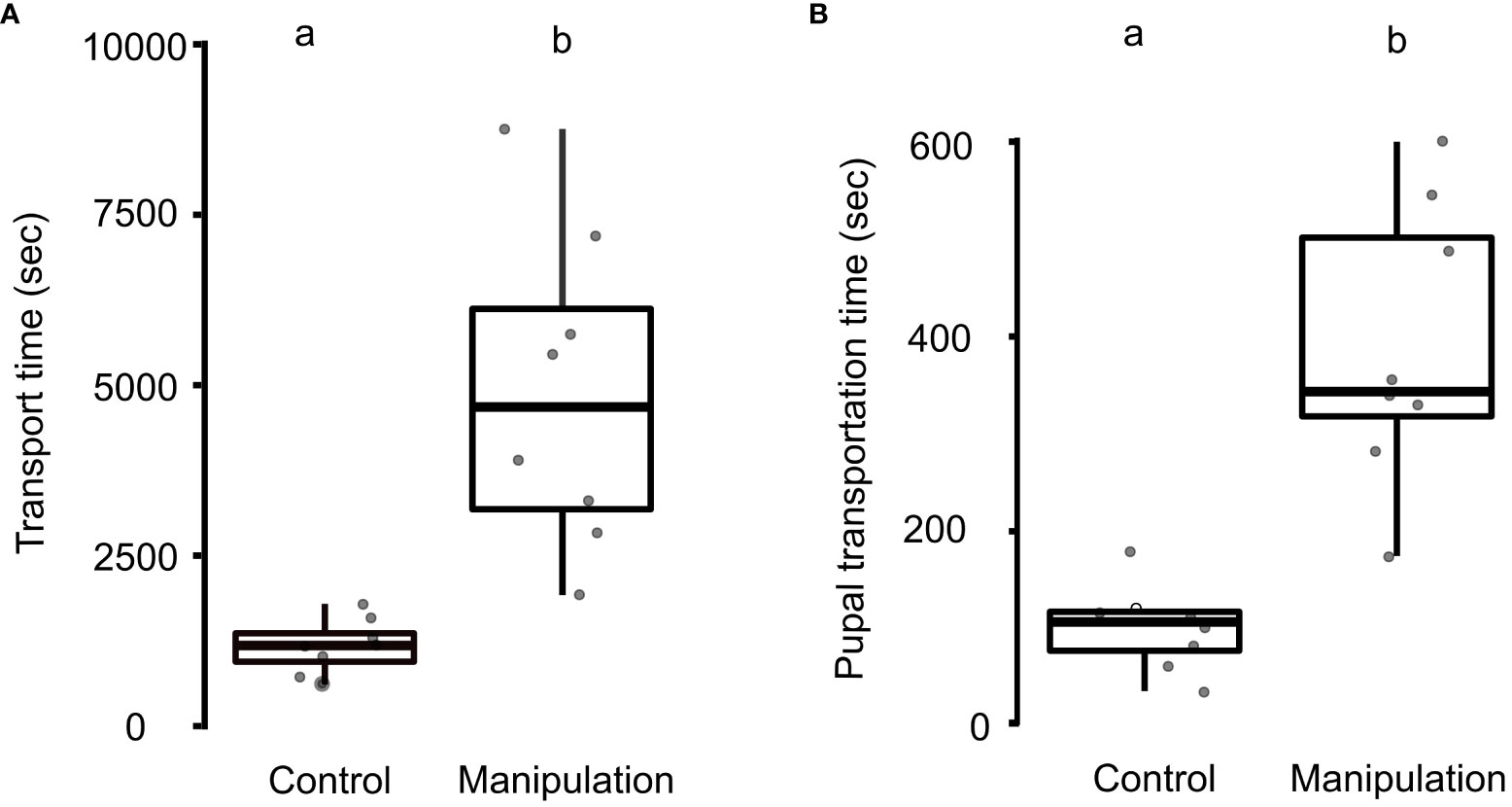
Figure 1 Transport time during nest relocation. (A) A boxplot with jitters for transport time of colony (adults and brood) during the control and manipulation experiments. Transport time was higher in the manipulation experiment (Mann-Whitney U-test, p < 0.01). (B) A boxplot with jitter for pupal transportation time (total relocation time/number of pupae transported) in the control and manipulation experiments (p < 0.01). Different letters placed over the boxplots denote statistically significant differences among the groups. A transverse line inside the box represents the median; a p-value of ≤ 0.05 was taken as a cut-off for statistically significant differences.
Modes of pupal insertion
We observed various pupal insertion behaviors during the experiments and recorded them in the ethogram (Supplementary Table 1; Figure 2). In the control experiments, ants transporting the brood quickly entered the nest entrance while holding a pupa in their mandibles (ITHP; Figure 3A). However, in the manipulation experiments, ants faced a challenge due to the narrow nest entrance, which did not allow simultaneous entry for both the adult and the pupa. To overcome this, they had to vertically align the pupal axis to the entrance, push the pupa inside and release it from their mandibles simultaneously (ITPP; Figure 3B). Sometimes, an ant attempted to align and push the pupa at the narrow entrance while another ant pulled it from the nest floor, as shown in Figure 3C (COIP).
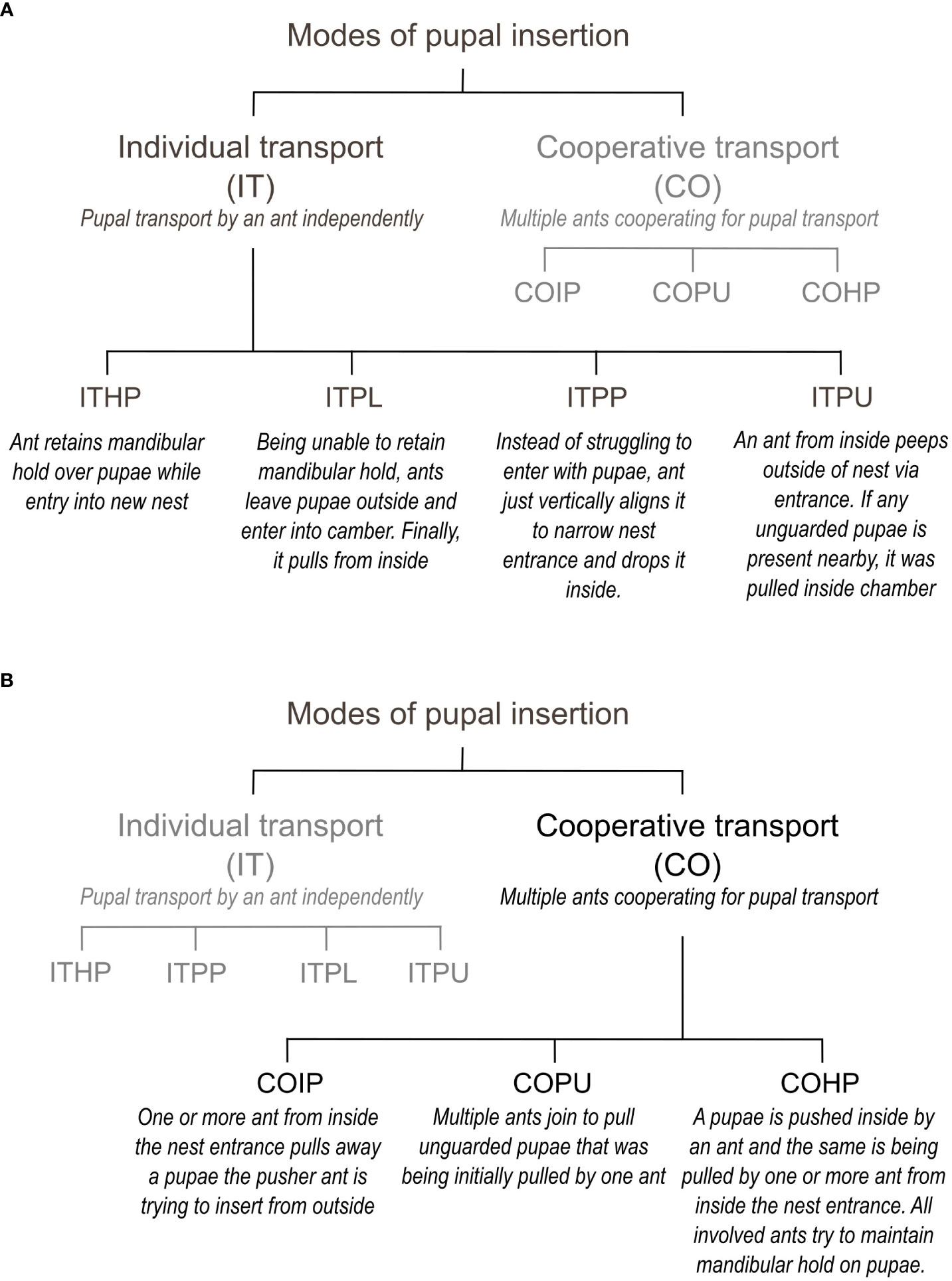
Figure 2 Ethogram explaining the different modes of pupal insertion. (A) Individual transport. (B) Cooperative transport.
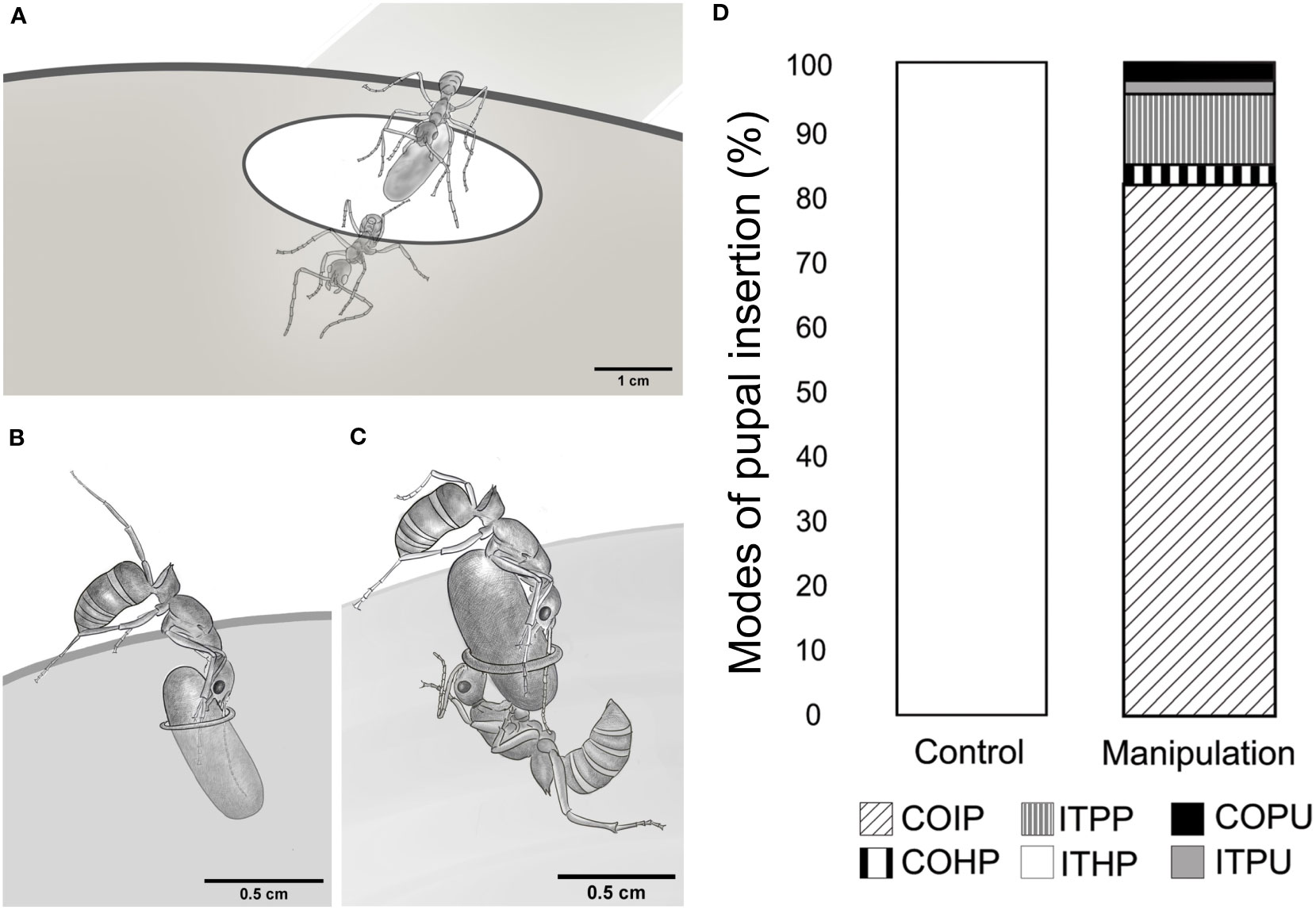
Figure 3 Modes of pupal insertion, observed in control and manipulation experiments. (A) Drawing, depicting a relocating tandem pair entering through a nest entrance, similar to that in the natural habitat. The pupa is held in the follower’s mandibles. (B) Sketch, depicting an individual brood transporter ant, inserting the pupa through the narrow nest entrance in the manipulation experimental set-up (ITPP). (C) Sketch, depicting the cooperative effort by one pusher and one puller ant to insert the pupa through a narrow nest entrance in the manipulative experiment (COIP). (D) Stacked bar chart, depicting the proportion of pupal insertions, performed using different modes in the control and manipulation experiments. – Individual transport by holding a pupa, ITHP; Individual transport by posting the pupa into the nest, ITPP; Individual transport by pulling unguarded pupa, ITPU; Cooperative insertion of pupa, COIP; Cooperative insertion by pulling unguarded pupa, COPU; Cooperative insertion by holding pupa, COHP.
ITHP was the only mode of pupal transport observed in the control experiments (100%). In contrast, cooperative insertion was the most common mode used in manipulation experiments (COIP, 81.18%) while individual transport was observed in a small number of cases (ITPP, 10.89%). Apart from these two behaviors, a few others were observed. For instance, pulling an unguarded pupa from close to the edges of the nest entrance into the nest with the puller being inside the nest (ITPU, 1.98%) or cooperative insertion of an unguarded pupa by more than one puller from the inside (COPU, 2.97%) was also observed. Note that ITPU and COPU did not involve a pusher. We also observed the cooperative insertion of a pupa, where the pusher ant moved into the nest while holding one end of the pupa; simultaneously, another ant held and pulled the pupa from inside the nest (COHP, 2.97%; Figure 3D).
Behavioral plasticity among ants
Ants involved in pupal insertions were classified into three groups, based on their roles. The first group comprised ants that exclusively pushed the pupa inside the nest (pusher ants), constituting 60.5 ± 13.3% of the ants. The second group consisted of ants that pulled the pupa inside the nest (puller ants), accounting for 72.4 ± 12.8% of the ants. The third group included ants that engaged in both pushing and pulling actions (multi-tasker ants), constituting a relatively small proportion of the ants at 40.5 ± 11.6% (Figure 4A).
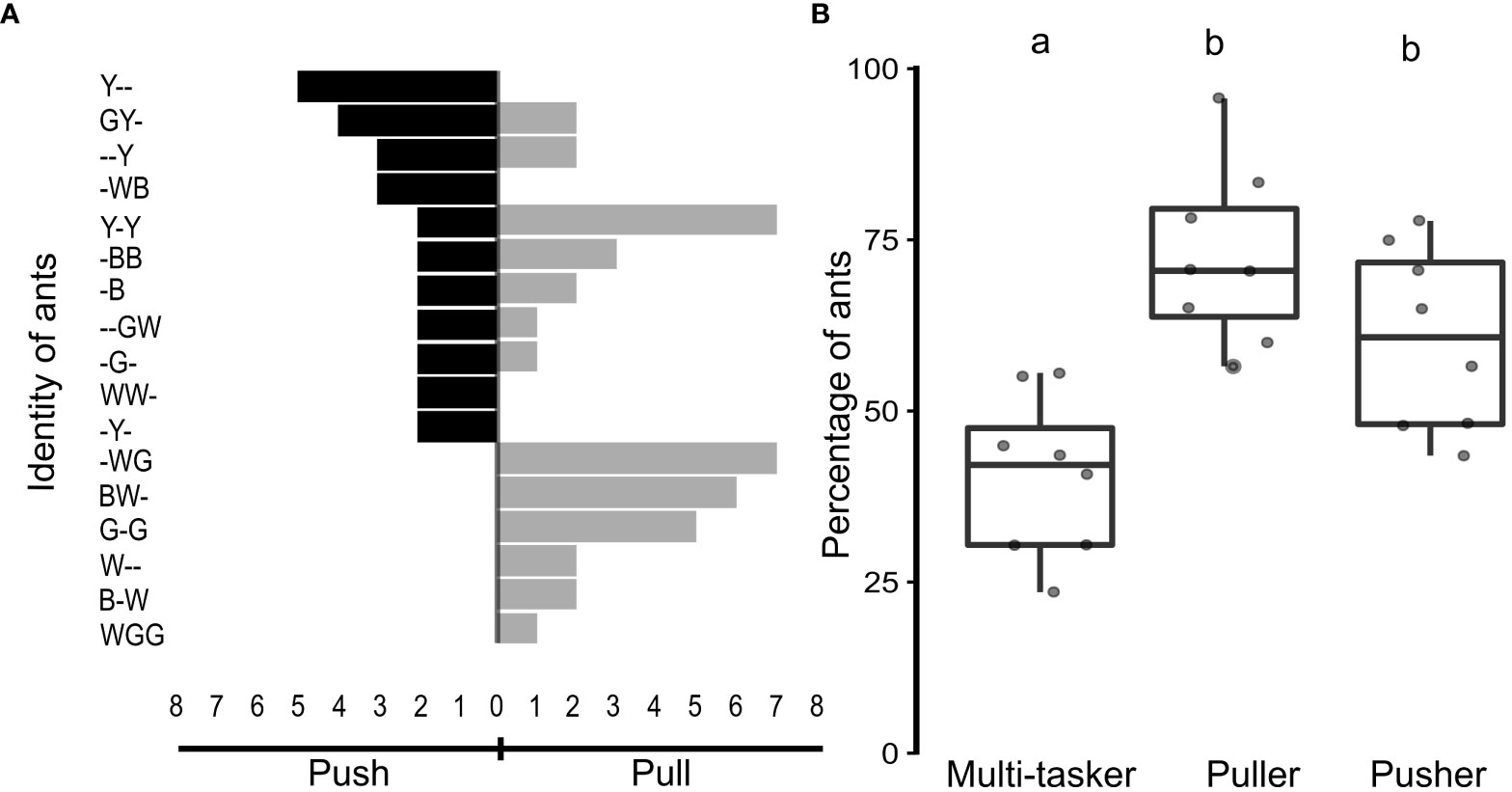
Figure 4 Behavioral plasticity among worker ants. (A) Tornado chart, depicting the identity and actions of workers in a representative ant colony from a manipulative experiment. Black horizontal bars represent the number of pupae-pushing events performed (left-side extension) by individual ants, as mentioned on the Y-axis. Grey horizontal bars represent the number of pupae-pulling events performed (right-side extension) by individual ants. Note that if a particular ant had performed both pushing and pulling events (“multi-tasker”), it has black and grey extensions. (B) Percentage of ants, performing both pulling and pushing of pupae (multi-taskers), only pulling or only pushing during the manipulation experiments (n = 8 colonies), depicted as boxplots with jitter. Different alphabets on the boxplots depict statistically significant differences, with the cut-off for significance being a p-value ≤ 0.05.
Upon comparing the percentage of ants involved in the different tasks, the pushers, pullers, and multi-taskers were significantly different in numbers (Kruskal-Wallis test, χ2 = 13.7, N = 8 colonies, p < 0.05; Figure 4B). Pairwise comparisons, using the Dwass-Steel-Critchlow-Fligner test, showed that the percentage of multi-tasker ants was significantly lower than those that exclusively pulled or pushed the pupae (puller vs multi-tasker, w = −4.76, p < 0.05; pusher vs multi-tasker, w = −3.64, p < 0.05; Figure 4B). The percentage of ants that exclusively performed the push or pull action, however, remained comparable (w = −2.16, p = 0.27; Figure 4B).
It was observed that only one pusher ant could push one pupa at a given time while one or more pullers could simultaneously pull it inside the nest. In most cases, however, a single pusher–puller combination was sufficient to successfully complete the task (1 pusher: 1 puller, 67.7%; 1 pusher: 2 pullers, 19.62%; 1 pusher: 3 pullers, 2.22%; 1 pusher: no puller, 10.74%; Figure S3).
Dynamics of pupal insertion
Several variables were examined to investigate the mechanism of pupal insertion and whether experience improved performance in this task, as measured by latency, the number of failed attempts, pupal insertion time, the number of pullers involved, and their impact on the process.
Latency was measured by analyzing the first ten pupal transports to the new nest site in the relocation and test phases of the manipulation experiments. After completing the relocation phase, the nest entrance was randomly rotated in multiples of 45°, and the ants were initially seen to search for the entrance. Upon finding the entrance, they aligned the pupa and quickly inserted it inside. The latency for insertion attempts per pupa was significantly higher in the relocation phase (355 ± 366 s), compared to the test phase (59 ± 80 s; Wilcoxon paired-sample test, W = 33, N = 8 colonies, p = 0.03; Figure 5A).
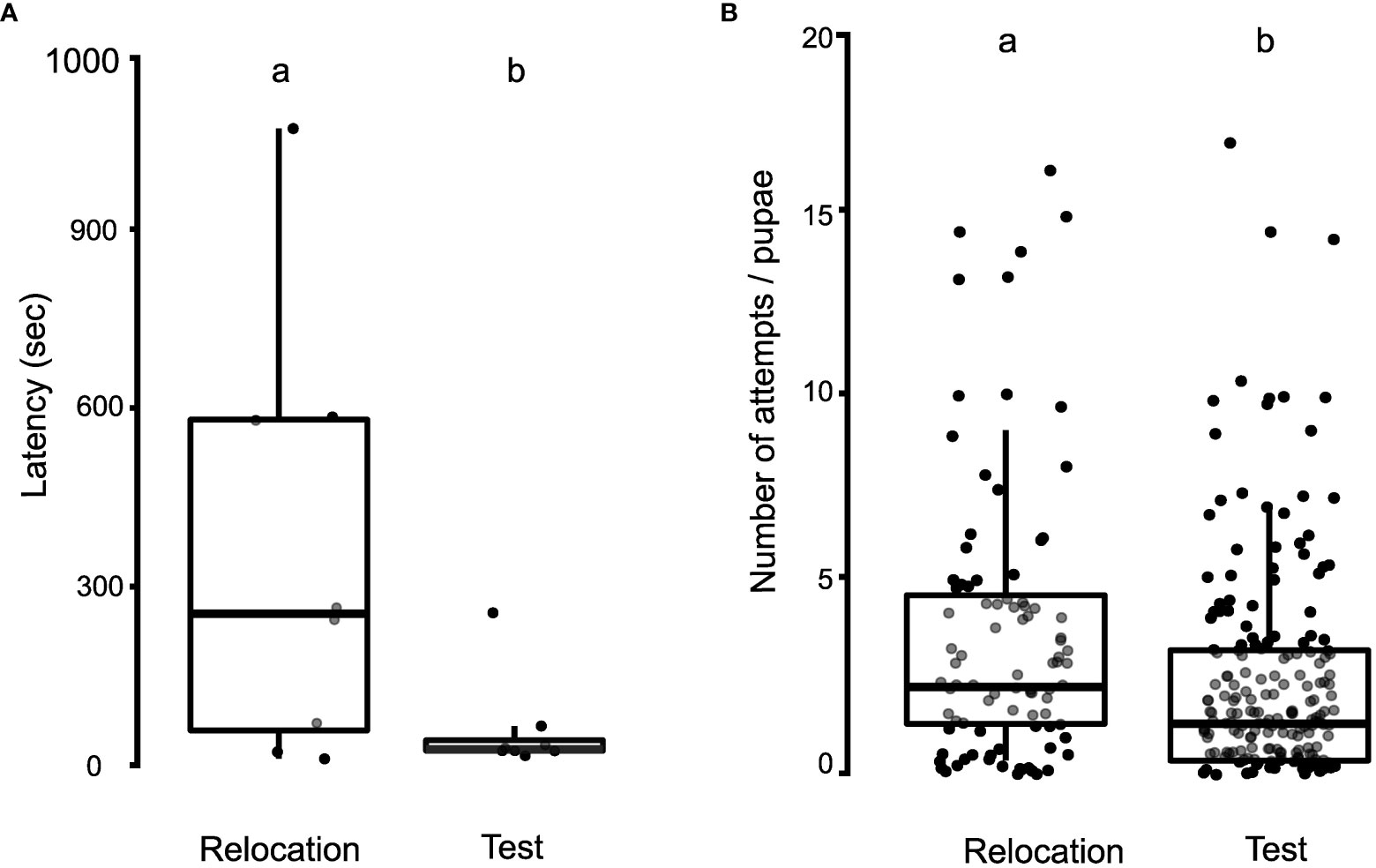
Figure 5 Dynamics of pupal insertion during the relocation and test phases of the manipulation experiments. (A) Boxplot with jitter, depicting the comparison of latency for pupal insertion during the relocation and test phases. (B) Boxplot, depicting the comparison of the number of attempts required for successful pupal insertion in the relocation and test phases of the manipulation experiment. Different alphabets on the boxplots depict statistically significant differences among the different categories with the cut-off for significance being a p-value of ≤ 0.05.
Failed attempts were quantified by examining the number of attempts taken to successfully insert each pupa during the manipulation experiments. Following an unsuccessful attempt, ants generally tried to insert the pupae repeatedly. The number of attempts taken to insert each pupa was significantly lower in the test phase (2.31 ± 2.93 attempts/pupa) than in the relocation phase (3.50 ± 3.79 attempts/pupa; Negative Binomial and Zero-Inflated Mixed Models, z = 2.27, p = 0.02; Figure 5B).
Pupal insertion time and the presence of puller ants were also investigated. In contrast to the relocation phase, the test phase of the manipulation experiments took a significantly shorter time for pupal insertion (GLMM, z = −70.33, p < 0.01). Furthermore, the presence of pullers had a significant impact on pupal insertion time (147.15 ± 262.16 s), as compared to no pullers (289.72 ± 831.16 s; GLMM, z = −33.40, p < 0.01). However, the presence of more than one puller significantly increased the insertion time: two pullers (172.33 ± 326.83 s; GLMM, z = 16.13, p < 0.01), three pullers (174.66 ± 125.71 s; GLMM, z = 3.01, p < 0.01) while insertions involving a single puller took the least amount of time (138.91 ± 244.38 s).
Individual- and colony-level efficiency
After evaluating the efficiency of pupal insertion by individual ants, we observed that pusher ants, who had successfully inserted three or more pupae (N = 43 ants) showed a significant decrease in their unsuccessful attempts (GLMM, z = −2.29, p = 0.02). This suggests that ants were able to improve their ability to insert pupae over time. We did not observe a significant reduction in failed attempts when monitoring all ants that had successfully inserted six pupae. However, sample size was small (N = 14) for this analysis (GLMM, z = −1.5, p = 0.12; Figures 6A, B). The presence or absence of pullers did not also significantly affect failed attempts of pupal insertions (GLMM, z = 0.96, p = 0.33).
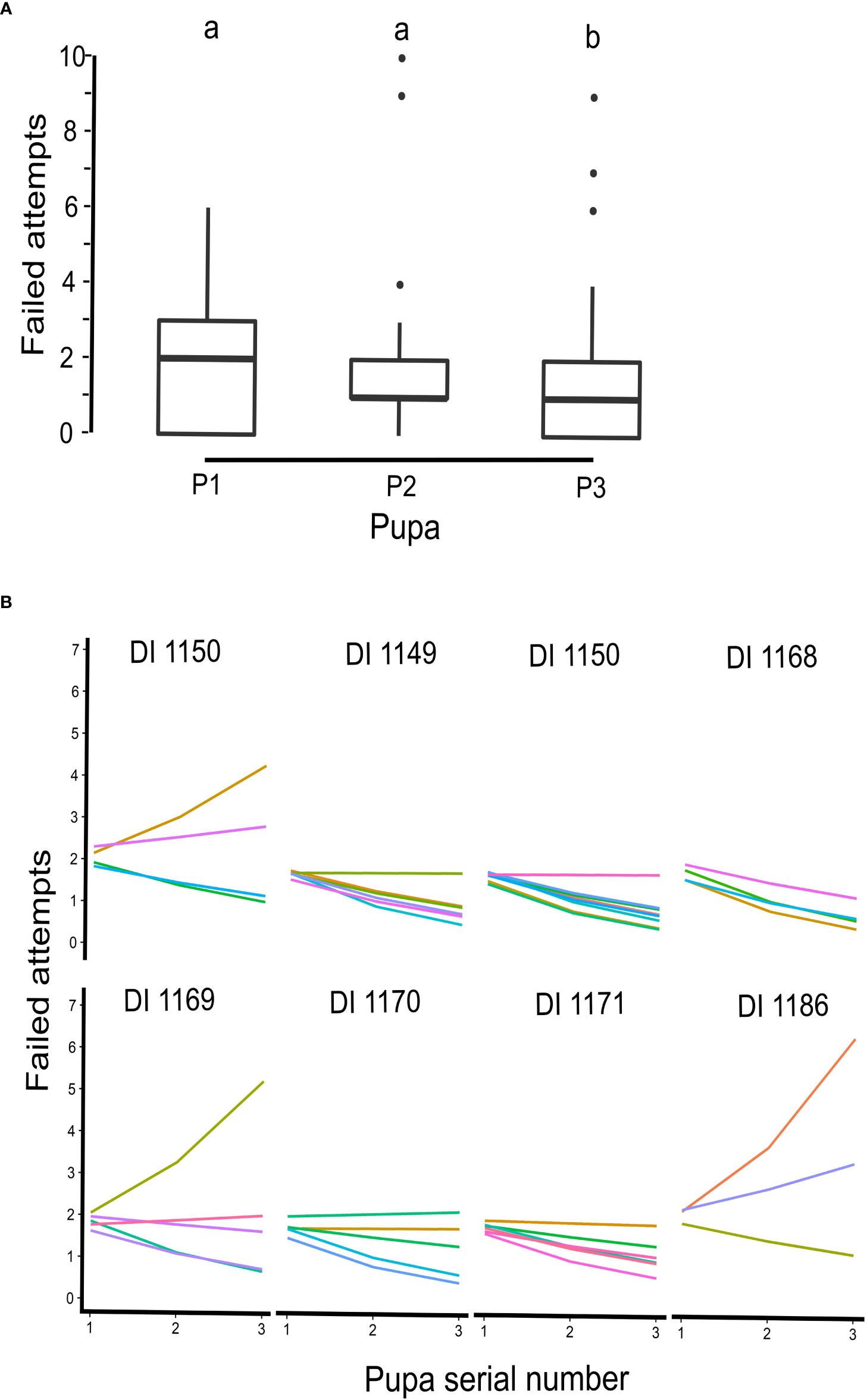
Figure 6 Failed attempts of pusher ants at three successive pupal insertions. (A) Boxplot, representing failed attempts, before a successful pupal insertion in a sequence from P1 to P3, by pusher ants. The number of failed attempts decreased with each successive pupal insertion (from P1 to P3) and the decline was statistically significant (GLMM, z = −2.29, p = 0.02). (B) Inter- and intra-individual efficiency of pusher ants from all eight ant colonies, on which manipulation experiments were conducted, plotted according to their colony identity. Most pusher ants (represented by different colored lines) showed a significant decrease in failed attempts for subsequent pupal insertions. A few outliers, however, went against the trend.
At the colony level, we found a non-significant correlation between the pupal insertion sequence and the number of failed attempts for that pupa during the relocation phase (Spearman’s correlation, ρ = −0.29, p = 0.41; Figure 7A). We however found a significant negative correlation between the pupal insertion sequence and the number of failed attempts during the test phase (ρ = −0.43, p = 0.03; Figure 7B).
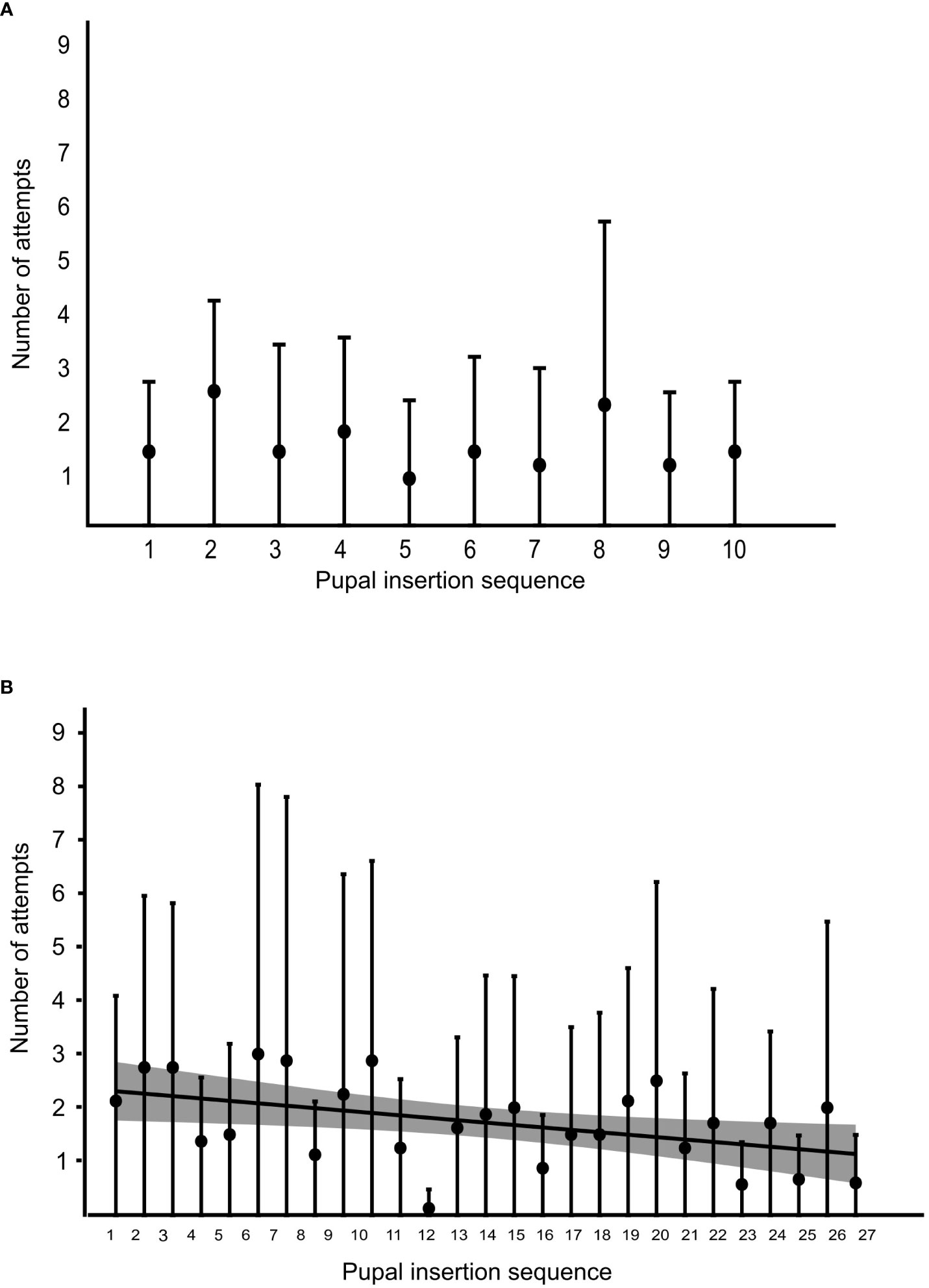
Figure 7 The number of attempts for subsequent pupal insertion through a narrow nest entrance. (A) Scatter plot, showing the mean number of attempts required (represented by circles) and their standard deviation (represented by whiskers) across eight ant colonies to successfully insert the pupa during the relocation phase, counted before a successful insertion, across successive pupal insertions at the colony level (Spearman’s correlation, ρ = −0.29, p = 0.41). (B) Scatter plot, showing the mean number of attempts (represented by black circles) and their standard deviation (represented by whiskers) required across the colonies to successfully insert the pupa during the test phase, measured across successive pupae at the colony level (ρ = −0.43, p = 0.03, shaded area, near the coefficient line, representing 95% confidence intervals).
Discussion
Behavioral innovations are critical for organisms to explore, access, and utilize new resources, enabling them to survive in changing environments and occupy new ecological niches (Reader and Laland, 2003; Sol et al., 2005; Liker and Bókony, 2009; Benson et al., 2012; Thornton and Samson, 2012; Griffin and Guez, 2014; Reader et al., 2016). Although extensive research has been conducted on behavioral innovation and problem-solving in primates and birds, relatively few studies have been conducted on social insects (Reader and Laland, 2003; Sol et al., 2005). Jean-Henri Faber, a pioneering scientist, thus wrote, “What happens in this little brain of Hymenoptera? Are their abilities similar to ours? Is there a thought? What problem, if we could solve it; what chapter of psychology, if we could write it!” (Translated from Fabre, 1882).
To explore the cognitive abilities of Hymenopteran insects, we therefore investigated whether D. indicum ants could overcome a cognitive challenge that confronted them. We used a novel paradigm that did not involve separate training or rewards and found that these ants could cooperate and successfully deciphered how to insert pupae through narrow nest entrances. Furthermore, their efficiency improved with experience, at both the individual and colony levels. It is true that not all animals can overcome challenges posed to them, as even motivated individuals may face difficulties in problem-solving, due to a lack of suitable motor action or physical abilities (Griffin and Guez, 2014). However, D. indicum ants overcame the challenge by performing a novel emergent behavior – a cooperative insertion of pupa, with one ant pushing and the other ant(s) pulling it from inside the nest. This behavior is distinctively novel and innovative, as D. indicum is known to be a solitary forager and transporter of food, and these ants typically transport brood individually (Kaur and Sumana, 2014).
Typically, in the case of a new nest entrance that is narrower than optimum, ants try to widen the entrance (SA, pers. obs.). When the substrate is soft, as for soil or decaying wood, this behavior can successfully enlarge the nest entrance by mandibular action. However, in the current set-up, the nest entrance, constructed from rigid plastic, did not allow the ants’ mandibles to modify it and thus, this became a hindrance to brood transportation. However, ants continued to bite the edges of the nest entrance, probably trying to solve the new problem with an existing solution, as is the case with higher primates that use already existing behavioral patterns to solve new problems (Kummer and Goodall, 1985). In the manipulation experiments, the ants took four times longer to relocate the colony, indicating that the narrow nest entrance indeed posed a challenge. Transportation from the old nest to the roof of the new nest was, however, qualitatively similar to what occurred in the control experiments. Ants were seen crowding the entrance and accumulating on the roof, with pupae held in their mandibles (Supplementary Figure 4), as they repeatedly tried to enter through the narrow entrance, still holding the pupae. Occasionally, after multiple unsuccessful trials, they would leave the pupae on the roof, enter the new nest, explore it, and then return to attempt the pupal insertion again. As ants neither had prior exposure to this challenge nor received any reward from experimenters for successfully inserting the pupae, their self-motivation and innovation were examined.
Interestingly, although individual ants attempted to introduce a pupa into the narrow nest entrance, they often could not complete the task. Due to the unsuitable alignment, the pupa got stuck at the entrance. Even when the ant aligned a pupa correctly and inserted more than 60% of the pupae into the entrance, their inability to let go of the pupae from their mandibles prevented them from successfully posting the pupa. Thus, only a small percentage of pupal insertions were through individual transport (ITPP mode). In the control experiments, all pupal transport was performed by individual transporters holding a pupa each (ITHP) but, in the manipulation experiments, the majority of the insertions were through cooperative transport, in which one ant pushed the pupa through the nest entrance while another ant pulled that pupa from inside the nest (COIP). Such a shift in behavior can be truly termed “innovation”. Cooperative transport was, however, not seen in this species for pupae, as the control experiments did not show a single cooperative insert. On facing a challenge, however, the ants showed “a new or modified learned behavior not previously found in a population”, which is the definition of innovation, as recorded in other social insects like bumblebees and Aphaenogaster ants (Fellers and Fellers, 1976; Reader and Laland, 2003; Kaufman and Kaufman, 2015; Chittka and Rossi, 2022). To understand the possible steps involved in this innovation, we thus constructed a flow chart, inspired by Kaufman and Kaufman (2015) (Figure 8).
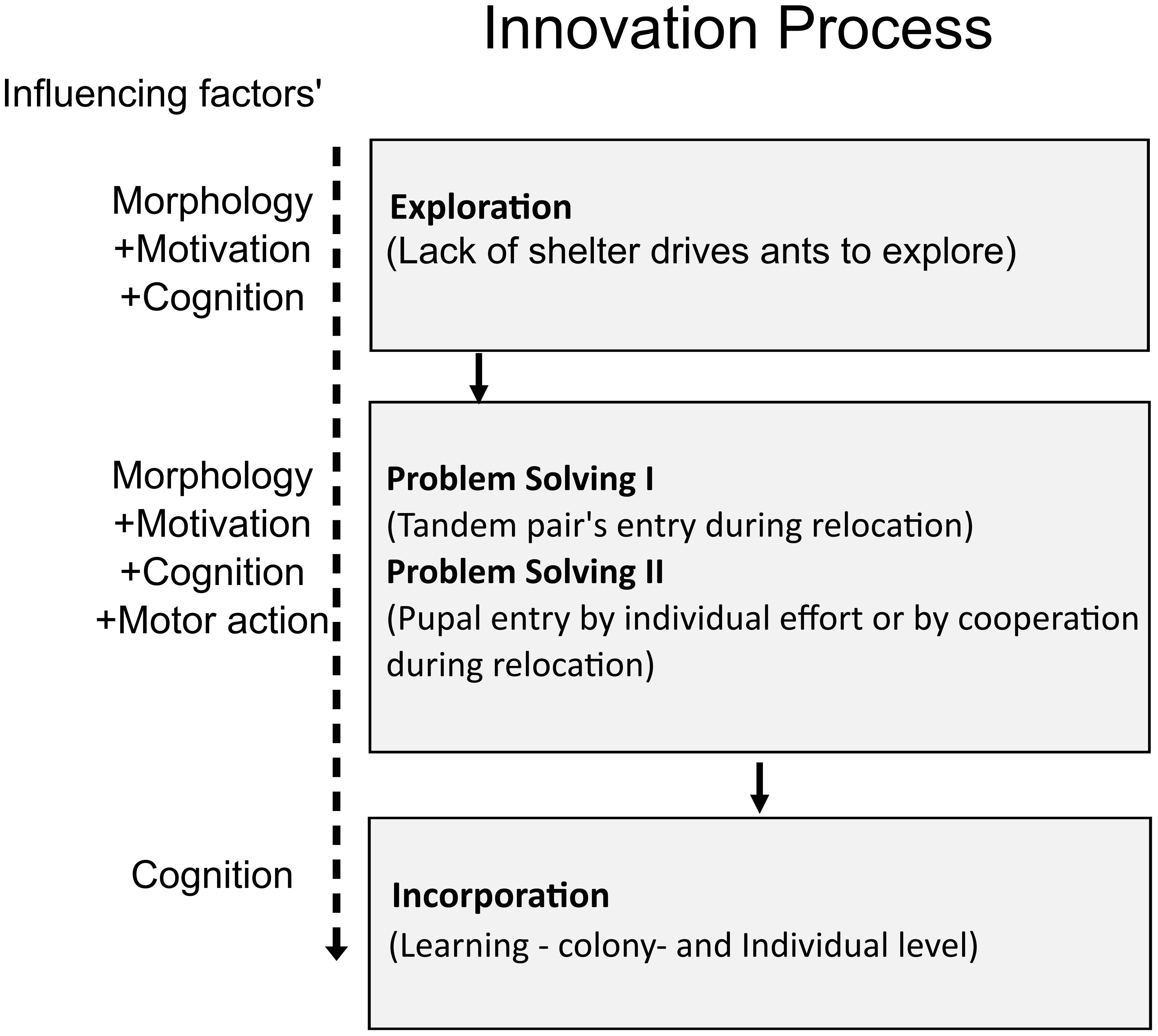
Figure 8 Flow chart describing the steps of innovation that the ants followed to overcome the experimental challenge and prepared following inputs into the innovation process by Kaufman and Kaufman (2015).
During pupal transportation, only one ant acted as the pusher most of the time while one to three ants participated in pulling the pupa from inside the new nest. The most efficient method of pupa transportation involved a single puller, as the time taken to insert a pupa was lowest with a combination of one pusher and one puller (Supplementary Figure S3). Previous research on Novomessor cockerelli ants, transporting food, indicated that they predominantly pulled the load individually and also cooperatively (Buffin and Pratt, 2016). However, in the current experiment with D. indicum ants, both pushing and pulling were performed equally by individual ants, possibly because the pupae were relatively light but required careful handling to avoid damage during transportation. During the experiments, in fact, no pupa was observed to be damaged or cannibalized by nestmates. Even though minor injuries may not have been detected by unaided human eyes, ants typically notice any such damage or infection and cannibalize the injured brood (Maák et al., 2020). It was observed, nevertheless, that most ants specialized either as pushers or pullers, with only about 40% of the ants exhibiting behavioral flexibility by performing both tasks.
An increase in latency has been noted when birds or primates perform problem-solving tasks (Griffin and Guez, 2014). Similarly, in the current experiment, our study ants showed a relatively higher latency for pupal transport through the narrow entrance of the nest. During the relocation phase, the crowding, seen at the entrance, with multiple workers entering and exiting the entrance for a tandem run, may have increased the latency for pupal transportation. However, the main factor, influencing latency, appeared to be the inability of the ants to transport pupae through the narrow entrance, as multiple individuals kept trying to do so, leading to several failed attempts. To quantify this, we analyzed the number of failed attempts before the focal ant successfully inserted a given pupa. At the individual level, we found that ants, in their third pupal insertion, took 19% fewer attempts, indicating that they improved their performance in this task with time. However, a significant improvement was not seen when the first six attempts by individual ants were examined, possibly due to its relatively small sample size or its complex dynamics while these insertions were performed, as staged in a natural context.
Further, at the colony level, we analyzed the number of attempts required to insert the pupae across the sequence of successful insertions and found a significantly negative correlation during the test phase. A comparable improvement was, however, not seen during the relocation phase, possibly because of all the other activities that simultaneously occurred at the nest entrance during this time. The colonies had thus clearly learned to perform this task better with experience.
In conclusion, the current study aimed to investigate the problem-solving abilities of an ant society, using a nature-inspired design that utilized its brood as motivation. Our findings suggest that these innovative ants could indeed solve the given problems and even improve their performance with experience. Generalizing further, the ants’ capabilities to innovate and adapt to changing environments could be expected to significantly impact their survival, particularly in circumstances, such as relocation, which threaten the whole colony, including the brood. Further studies involving other social insects and ecologically-inspired designs that consider the organisms’ umwelt may uncover more insights into the intricate workings of these superorganism brains.
Data availability statement
The raw data supporting the conclusions of this article will be made available by the authors, without undue reservation.
Ethics statement
This study was conducted under the prevalent animal care regulations in India. The model organism was not endangered and was not collected from any legally protected area. After experimentation, the ant colonies were released back into their natural habitat.
Author contributions
The idea for the experiment emerged from an observation made by MP. Conceptualization was by SA. Fund acquisition was by SA. The experimental design was by SA, MP, and SH. Data acquisition was conducted by MP and SH. Interpretation of data was by SA, SM, MP, and SH. Statistical analysis was done by SM and MP. Visualization was done by SH, MP, and SA. The original draft of the manuscript was prepared by MP, and it was reviewed and edited by SA. Supervision was by SA. All authors contributed to the article and approved the submitted version.
Funding
Funding for experimental expenditure was received from the Science and Engineering Research Board, Government of India – SERB Project (EMR/2017/001457) – and the Indian Institute of Science Education and Research Kolkata (IISER-K), India. SH and SM received IISER-K fellowships. MP received a DST/INSPIRE fellowship (IF170031) from the Department of Science and Technology, Government of India.
Acknowledgments
We appreciate Basudev Ghosh for his efforts in collecting ant colonies, carefully maintaining them in the laboratory, and releasing them back into their natural habitat after experimentation. Bishwarup Paul, Rubina Mondal, and Anoop Karunakaran provided valuable inputs into the statistical analysis and revision of the manuscript, some of which we incorporated and for which we sincerely thank them. MP acknowledges the selfless efforts of Kristin Sainani of the Stanford School of Medicine for providing an excellent course on “Writing in Sciences, SOM-Y0010” freely on an online platform and which helped him write the first draft of this manuscript. We also thank the two reviewers and the editor for their helpful suggestions on an earlier version of this manuscript.
Conflict of interest
The authors declare that the research was conducted in the absence of any commercial or financial relationships that could be construed as a potential conflict of interest.
Publisher’s note
All claims expressed in this article are solely those of the authors and do not necessarily represent those of their affiliated organizations, or those of the publisher, the editors and the reviewers. Any product that may be evaluated in this article, or claim that may be made by its manufacturer, is not guaranteed or endorsed by the publisher.
Supplementary material
The Supplementary Material for this article can be found online at: https://www.frontiersin.org/articles/10.3389/fevo.2023.1135068/full#supplementary-material
References
Amiel J. J., Tingley R., Shine R. (2011). Smart moves: effects of relative brain size on establishment success of invasive amphibians and reptiles. PloS One 6, e18277. doi: 10.1371/journal.pone.0018277
Anderson C., McShea D. W. (2001). Individual versus social complexity, with particular reference to ant colonies. Biol. Rev. 76, 211–237. doi: 10.1017/S1464793101005656
Annagiri S. (2021). Diacamma In: Starr C.K. (eds) Encyclopedia of Social Insects. Springer, Cham. doi: 10.1007/978-3-030-28102-1_35
Bateson M. (2002). Recent advances in our understanding of risk-sensitive foraging preferences. Proc. Nutr. Soc 61, 509–516. doi: 10.1079/PNS2002181
Bateson M., Desire S., Gartside S. E., Wright G. A. (2011). Agitated honeybees exhibit pessimistic cognitive biases. Curr. Biol. 21, 1070–1073. doi: 10.1016/j.cub.2011.05.017
Beckers R., Deneubourg J.-L., Goss S., Pasteels J. M. (1990). Collective decision making through food recruitment. Ins. Soc 37, 258–267. doi: 10.1007/BF02224053
Benson-Amram S., Kolekamp K. E. (2012). Innovative problem solving by wild spotted hyenas. Proc. R. Soc B 279, 4087–4095. doi: 10.1098/rspb.2012.1450
Bhatkar A., Whitcomb W. H. (1970). Artificial diet for rearing various species of ants. Florida Entomologist, 229–232. doi: 10.2307/3493193
Bhattacharyya K., Kolay S., Annagiri S. (2021). The structure and importance of nest mounds in a tropical ant Diacamma indicum. Ecol. Entomol. 46, 1324–1332. doi: 10.1111/een.13079
Bhattacharyya K., Annagiri S. (2019). Characterization of nest architecture of an Indian ant Diacamma indicum (Hymenoptera: Formicidae). J. Insect. Sci. 19, 9. doi: 10.1093/jisesa/iez083
Brooks M. E., Kristensen K., Van Benthem K. J., Magnusson A., Berg C. W., Nielsen A., et al. (2017). glmmTMB balances speed and flexibility among packages for zero-inflated generalized linear mixed modeling. R J. 9 (2), 378–400. doi: 10.32614/RJ-2017-066
Buffin A., Pratt S. C. (2016). Cooperative transport by the ant novomessor cockerelli. Insectes Soc. 63, 429–438. doi: 10.1016/j.tics.2022.04.001
Chittka L., Rossi N. (2022). Social cognition in insects. Trends Cogn. Sci. 26, 578–592. doi: 10.1016/j.tics.2022.04.001
Chow P. K. Y., Clayton N. S., Steele M. A. (2021). Cognitive performance of wild eastern gray squirrels (Sciurus carolinensis) in rural and urban, native, and non-native environments. Front. Ecol. Evol. 9, 615899. doi: 10.3389/fevo.2021.615899
Cole B. J. (1985). Size and behaviour in ants: constraints on complexity. Proc. Natl. Acad. Sci. 82, 8548–8551. doi: 10.1073/pnas.82.24.8548
Cole E. F., Quinn J. L. (2012). Personality and problem-solving performance explain competitive ability in the wild. Proc. R. Soc. B: Biol. Sci. 279, 1168–1175. doi: 10.1098/rspb.2011.1539
Collet J., Morford J., Lewin P., Bonnet-Lebrun A. S., Sasaki T., Biro D. (2023). Mechanisms of collective learning: how can animal groups improve collective performance when repeating a task? Philos. Trans. R. Soc. B 378 (1874), 20220060. doi: 10.1098/rstb.2022.0060
Collett M., Chittka L., Collett T. S. (2013). Spatial memory in insect navigation. Curr. Biol. 23, R789–R800. doi: 10.1016/j.cub.2013.07.020
Czaczkes T., Ratnieks F. (2013). Cooperative transport in ants (Hymenoptera: formicidae) and elsewhere. Myrmecol News 18, 1–11.
Fabre J.-H. (1882). Nouveaux souvenirs entomologiques: études sur l’instinct et les moeurs des insectes. Paris: Librairie Ch. Delagrave.
Feinerman O., Korman A. (2017). Individual versus collective cognition in social insects. J. Exp. Biol. 220, 73–82. doi: 10.1242/jeb.143891
Fellers J. H., Fellers G. M. (1976). Tool use in a social insect and its implications for competitive interactions. Science 192, 70–72. doi: 10.1016/j.beproc.2014.08.027
Franklin E. L. (2014). The journey of tandem running: the twists, turns and what we have learned. Insectes Sociaux 61, 1–8. doi: 10.1007/s00040-013-0325-3
Giurfa M. (2013). Cognition with few neurons: higher-order learning in insects. Trends Neurosci. 36, 285–294. doi: 10.1016/j.tins.2012.12.011
Giurfa M. (2019). Honeybees foraging for numbers. J. Comparat. Physiol. A. 205, 439–450. doi: 10.1007/s00359-019-01344-2
Giurfa M., Zhang S., Jenett A., Menzel R., Srinivasan M. v. (2001). The concepts of “sameness” and “difference” in an insect. Nature 410, 930–933. doi: 10.1038/35073582
Goss S., Aron S., Deneubourg J.-L., Pasteels J. M. (1989). Selforganized shortcuts in the Argentine ant. Naturwissenschaften 76, 579–581. doi: 10.1007/BF00462870
Griffin A. S., Guez D. (2014). Innovation and problem solving: a review of common mechanisms. Behav. Process. 109, 121–134. doi: 10.1016/j.beproc.2014.08.027
Hertel A. G., Niemelä P. T., Dingemanse N. J., Mueller T. (2020). A guide for studying among-individual behavioural variation from movement data in the wild. Mov Ecol. 8, 30. doi: 10.1186/s40462-020-00216-8
Howard S. R., Avarguès-Weber A., Garcia J. E., Greentree A. D., Dyer A. G. (2018). Numerical ordering of zero in honey bees. Science 360, 1124–1126. doi: 10.1126/science.aar4975
Huebner F., Fichtel C., Kappeler P. M. (2018). Linking cognition with fitness in a wild primate: fitness correlates of problem-solving performance and spatial learning ability. Philos. Trans. R. Soc B. 373, 20170295. doi: 10.1098/rstb.2017.0295
Jackman S., Tahk A., Zeileis A., Maimone C., Fearon J., Meers Z., et al. (2015). Package “pscl”. Polit. Sci. Comput. Lab. 18, 93–97. https://cran.microsoft.com/snapshot/2015-03-01/web/packages/pscl/pscl.pdf.
Kaufman A. B., Kaufman J. C. (2015). Animal creativity and innovation (Cambridge, MA: Academic Press).
Kaur R., Anoop K., Sumana A. (2012). Leaders follow leaders to reunite the colony: relocation dynamics of an Indian queenless ant in its natural habitat. Anim. Behav. 83, 1345–1353. doi: 10.1016/j.anbehav.2012.02.022
Kaur R., Joseph J., Anoop K., Sumana A. (2017). Characterization of recruitment through tandem running in an Indian queenless ant diacamma indicum. R. Soc Open Sci. 4160476160476, 1–12. doi: 10.1098/rsos.160476
Kaur R., Sumana A. (2014). Coupled adult-brood transport augments relocation in the Indian queenless ant diacamma indicum. Insectes Soc. 61, 141–143. doi: 10.1073/pnas.0900042106
Kummer H., Goodall J. (1985). Conditions of innovative behaviour in primates. Philos. Trans. R. Soc Lond. B. 308, 203–214. doi: 10.1098/rstb.1985.0020
Lihoreau M., Dubois T., Gomez-Moracho T., Kraus S., Monchanin C., Pasquaretta C. (2019). Putting the ecology back into insect cognition research. Adv. Insect Physiol. (Amsterdam), 1–25.
Lihoreau M., Latty T., Chittka L. (2012). An exploration of the social brain hypothesis in insects. Front. Physiol. 3. doi: 10.3389/fphys.2012.00442
Liker A., Bókony V. (2009). Larger groups are more successful in innovative problem solving in house sparrows. Proc. Natl. Acad. Sci. U.S.A. 106, 7893–7898. doi: 10.1073/pnas.0900042106
Loukola O. J., Solvi C., Coscos L., Chittka L. (2017). Bumblebees show cognitive flexibility by improving on an observed complex behaviour. Sci. (1979) 355, 833–836. doi: 10.1126/science.aag2360
Maák I., Tóth E., Lenda M., Lőrinczi G., Kiss A., Juhász O., et al. (2020). Behaviours indicating cannibalistic necrophagy in ants are modulated by the perception of pathogen infection level. Sci. Rep. 10, 17906. doi: 10.1038/s41598-020-74870-8
Perry C. J., Barron A. B. (2013). Honey bees selectively avoid difficult choices. Proc. Natl. Acad. Sci. 110, 19155–19159. doi: 10.1073/pnas.1314571110
Marler P. (1997). Three models of song learning: evidence from behaviour. J. Neurobiol. 33, 501–516. doi: 10.1002/(SICI)1097-4695(19971105)33:5<501::AID-NEU2>3.0.CO;2-8
McGlynn T. P. (2012). The ecology of nest movement in social insects. Ann. Rev. Entomol. 57, 291–308. doi: 10.1146/annurev-ento-120710-100708
Mukhopadhyay S., Pathak M. K., Annagiri S. (2019). Path minimization in a tandem running Indian ant in the context of colony relocation. J. Exp. Biol. 222, jeb206490. doi: 10.1242/jeb.206490
Papaj D. R., Lewis A. C. (1992). Insect learning: ecology and evolutinary perspectives (New York, NY: Springer).
Paul B., Annagiri S. (2019). Caught red-handed: behaviour of brood thieves in an Indian ant. J. Exp. Biol. 222, jeb193755. doi: 10.1242/jeb.193755
Perry C. J., Barron A. B., Chittka L. (2017). The frontiers of insect cognition. Curr. Opin. Behav. Sci. 16, 111–118. doi: 10.1016/j.cobeha.2017.05.011
Preiszner B., Papp S., Pipoly I., Seress G., Vincze E., Liker A., et al. (2017). Problem-solving performance and reproductive success of great tits in urban and forest habitats. Anim. Cognit. 20, 53–63. doi: 10.1007/s10071-016-1008-z
Pritchard D. J., Hurly T. A., Tello-Ramos M. C., Healy S. D. (2016). Why study cognition in the wild (and how to test it)? J. Exp. Anal. Behav. 105, 41–55. doi: 10.1002/jeab.195
Reader S. M., Laland K. N. (2003). Animal innovation (Oxford: Oxford University). doi: 10.1093/acprof:oso/9780198526223.001.0001
Reader S. M., Morand-Ferron J., Flynn E. (2016). Animal and human innovation: novel problems and novel solutions. Philos. Trans. R. Soc. B: Biol. Sci. 371, 20150182. doi: 10.1098/rstb.2015.0182
Reznikova Z. (2018). Ants. individual and social cognition. field and laboratory methods in animal cognition: a comparative guide (Cambridge, UK: Cambridge University Press).
Santana L. H., Garcia-Mijares M. (2022). Animal creativity as a function of behavioural innovation and behaviour flexibility in problem-solving situations. Integr. Psychol. Behav. Sci. 56, 218–233. doi: 10.1016/j.anbehav.2012.03.018
Sasaki T., Granovskiy B., Mann R. P., Sumpter D. J. T., Pratt S. C. (2013). Ant colonies outperform individuals when a sensory discrimination task is difficult but not when it is easy. Proc. Natl. Acad. Sci. U.S.A. 110, 13769–13773. doi: 10.1073/pnas.1304917110
Sol D., Duncan R. P., Blackburn T. M., Cassey P., Lefebvre L. (2005). Big brains, enhanced cognition, and response of birds to novel environments. Proc. Natl. Acad. Sci. U.S.A. 102, 5460–5465. doi: 10.1073/pnas.0408145102
Sol D., Timmermans S., Lefebvre L. (2002). Behavioural flexibility and invasion success in birds. Anim. Behav. 63, 495–502. doi: 10.1006/anbe.2001.1953
Thornton A., Samson J. (2012). Innovative problem solving in wild meerkats. Anim. Behav. 83, 1459–1468. doi: 10.1016/j.anbehav.2012.03.018
Wilson E. (1959). Communication by tandem running in the ant genus cardiocondyla. Psyche 66, 29–34. doi: 10.1155/1959/29093
Keywords: Diacamma indicum, brood transport, colony relocation, behavioral plasticity, innovation, learning
Citation: Pathak MK, Halder S, Mukhopadhyay S and Annagiri S (2023) Two is better than one: innovative ants overcome the nature-inspired cognitive challenge of pupal insertion. Front. Ecol. Evol. 11:1135068. doi: 10.3389/fevo.2023.1135068
Received: 31 December 2022; Accepted: 28 June 2023;
Published: 28 July 2023.
Edited by:
Anindya Sinha, National Institute of Advanced Studies, IndiaReviewed by:
Jean-Baptiste Leca, University of Lethbridge, CanadaDivya Uma, Azim Premji University, India
Copyright © 2023 Pathak, Halder, Mukhopadhyay and Annagiri. This is an open-access article distributed under the terms of the Creative Commons Attribution License (CC BY). The use, distribution or reproduction in other forums is permitted, provided the original author(s) and the copyright owner(s) are credited and that the original publication in this journal is cited, in accordance with accepted academic practice. No use, distribution or reproduction is permitted which does not comply with these terms.
*Correspondence: Sumana Annagiri, c3VtYW5hQGlpc2Vya29sLmFjLmlu