- 1State Key Laboratory of Urban and Regional Ecology, Research Center for Eco-Environmental Sciences, Chinese Academy of Sciences, Beijing, China
- 2University of Chinese Academy of Sciences, Beijing, China
- 3School of Geosciences & Surveying Engineering, China University of Mining & Technology, Beijing, China
- 4Inner Mongolia Academy of Agricultural and Animal Husbandry Sciences, Hohhot, China
- 5Baotou Teachers’ College, Baotou, China
In the context of global climate change, temperate forests in climate-sensitive areas are inevitably affected. To deepen the understanding of the impact on precipitation changes into the relationship between key ecosystem services (ESs), this study selected net primary productivity (NPP), soil conservation (SC) and water yield (WY) of temperate forest in northern China as objects, and the Spearman correlation test and redundancy analysis were applied to analyze the response of ESs relationship to precipitation gradient. The results show that precipitation is the meteorological factor with the greatest impact (contribution 21.2%, p<0.01) on ESs and their relationships in temperate forests. The 600-700 mm precipitation gradient is the key turning point in the change of ESs relationship of WY with NPP and SC. This indicates that attention should be paid to the spatial variation of the 600-700 mm precipitation region in the future warm-wet in northern China, which should be used as a dividing line of forest management and policy development. Based on the results, future restoration projects in northern temperate forest should focus on (1) in areas with less than 600-700 mm of precipitation, attention should be paid to the selection of tree species for afforestation to maintain regional water balance; (2) in areas with more than 700 mm of precipitation, soil and water conservation projects need to be planned, especially in mountainous area. The research can not only support the management of temperate forest ecosystems in northern China, but also provide reference to other forest ecosystems to cope with climate change.
1. Introduction
Forest ecosystems cover 30% of Earth’s land surface and are the primary ecosystems supporting terrestrial life (FAO, 2020). As the source of essential ecosystem services (ESs) such as timber supply, soil conservation, carbon sequestration, and climate regulation (Gamfeldt et al., 2013; Brockerhoff et al., 2017; Ding et al., 2022), forest ecosystems are susceptible and vulnerable to global climate change (Seidl et al., 2017; Wan et al., 2018; Anderegg et al., 2022). Changes in precipitation are an important indicator of global climate change, which can directly or indirectly affect the growth and distribution of forests, altering their structure and function, and thus affecting ESs and their interrelationships (Weltzin et al., 2003; Xu et al., 2020; Chen J. et al., 2021; Liu et al., 2022). From the perspective of the impact path on ESs on forest ecosystem, the intensity and duration of precipitation can affect the flow production mechanism and water yield, along with soil properties (Balasubramanian, 2017; Li M. et al., 2021; Wu et al., 2022); Spatial and temporal variations of precipitation can affect vegetation growth, change vegetation productivity and biomass (Wang et al., 2020), and then affect the carbon sequestration of forest. Forest canopy density and fine root distribution, which are affected by precipitation, can also impact on water yield and soil conservation (Lozano-Parra et al., 2015; Jiang et al., 2016; Thompson et al., 2016). In addition, temperate forest growth and ESs are more sensitive to water restriction (Gilliam, 2016; Seidl et al., 2020; Zhang et al., 2020). Therefore, exploring the response of temperate forest ESs and their inter-relationships to precipitation can not only support local forest management, but also provide basis for forest ecosystems to cope with global climate change.
Forest ecosystems provide a variety of ESs to human beings. Till now, most research on forest ESs focuses on the spatio-temporal changes and the cold and hot spots identification (Ditt et al., 2010; Mamat et al., 2018; Vatandaslar, 2022). Also, there are studies on forest ESs under different water conditions. In water-scarce temperate drylands, more attention has been paid to the dynamic interactions between rainfall and vegetation coverage and the ESs they provide (Wang et al., 2011; Rohatyn et al., 2018; Wang H. et al., 2022). In areas with abundant hydrothermal conditions, studies have revealed the effects of forest supply and support services on precipitation gradients (Alamgir et al., 2016; Thomas et al., 2021). In addition, the influence of temporal variation in precipitation on forest ESs is also one of the hotspots, e.g., the effects of inter-annual and intra-annual temporal dynamics of precipitation on forest area and regulation services (soil erosion, nutrient retention and etc.) in eco-sensitive zones (Suescún et al., 2017; Nelson et al., 2018; Rodrigues et al., 2020).
However, tradeoffs and synergies of ESs exist extensively in forest ecosystem (Liu et al., 2019; Wang et al., 2021; Feng et al., 2022; Jia et al., 2022), the dynamic of which can significantly affect the forest growth and management (Biber et al., 2020). Compared with the impact of precipitation on forest ESs (Hely et al., 2006; Lu et al., 2014), we still have relatively little understanding of the effects of precipitation on the interrelationships of forest ESs, especially the interconversion between tradeoffs and synergies. Due to the various response of different ESs and precipitation, the interrelationships among ESs with precipitation should be diverse. Therefore, it is of necessity to clarify the response of forest ES relationships to precipitation variation, especially in the temperate zone which is sensitive to climate change (Galicia et al., 2015; Wang et al., 2019).
The temperate forests of northern China are selected as the study area, of which is important national ecological security barriers. Forests in this area play the important role in regulating the local and regional carbon and water cycles and mitigating climate change (Thom et al., 2017; Jiao et al., 2021). In addition, the temperate zone is climate sensitive (Wan et al., 2017, 2018), and water conditions are the primary limiting factor for forest growth and ESs supply (Fang et al., 2005; Galicia et al., 2015). Therefore, in this study, three ESs, e.g., Net Primary Product (NPP), soil conservation (SC) and water yield (WY), which closely related to water resources in temperate forests were selected. The specific objectives are (1) to identify the relationship between the three ESs and precipitation; (2) quantify the interrelationships of ESs in response to precipitation gradients; and (3) assess the contribution of precipitation to interrelationship of ESs. The results can provide insight into the impact of precipitation changes on ES relationships and the information for coping with possible damage to forest due to climate change.
2. Materials and methods
2.1. Study area
To cover the overall temperature zone, the study area covers the Songliao River Basin and Haihe River Basin in the north of China (Figure 1). The area has warm-rainy summer and cold-dry winter with 445–973 mm in annual average rainfall and −7 to 13°C in annual average air temperature. Based on the statistical results of national weather stations in the study area, there was a significant downward trend in precipitation in the region, with a precipitation change rate of −7.35 mm/10a during the period 1960–20181. However, existing models predict that the precipitation trend in the region will reverse in the future (Jiang et al., 2020; Jiang R. et al., 2021). The model simulations combined with IPCC SRES A2 scenario also indicate an increasing precipitation trend of 0.08-2 mm/d in the future (2035–2044) (Tang et al., 2009).
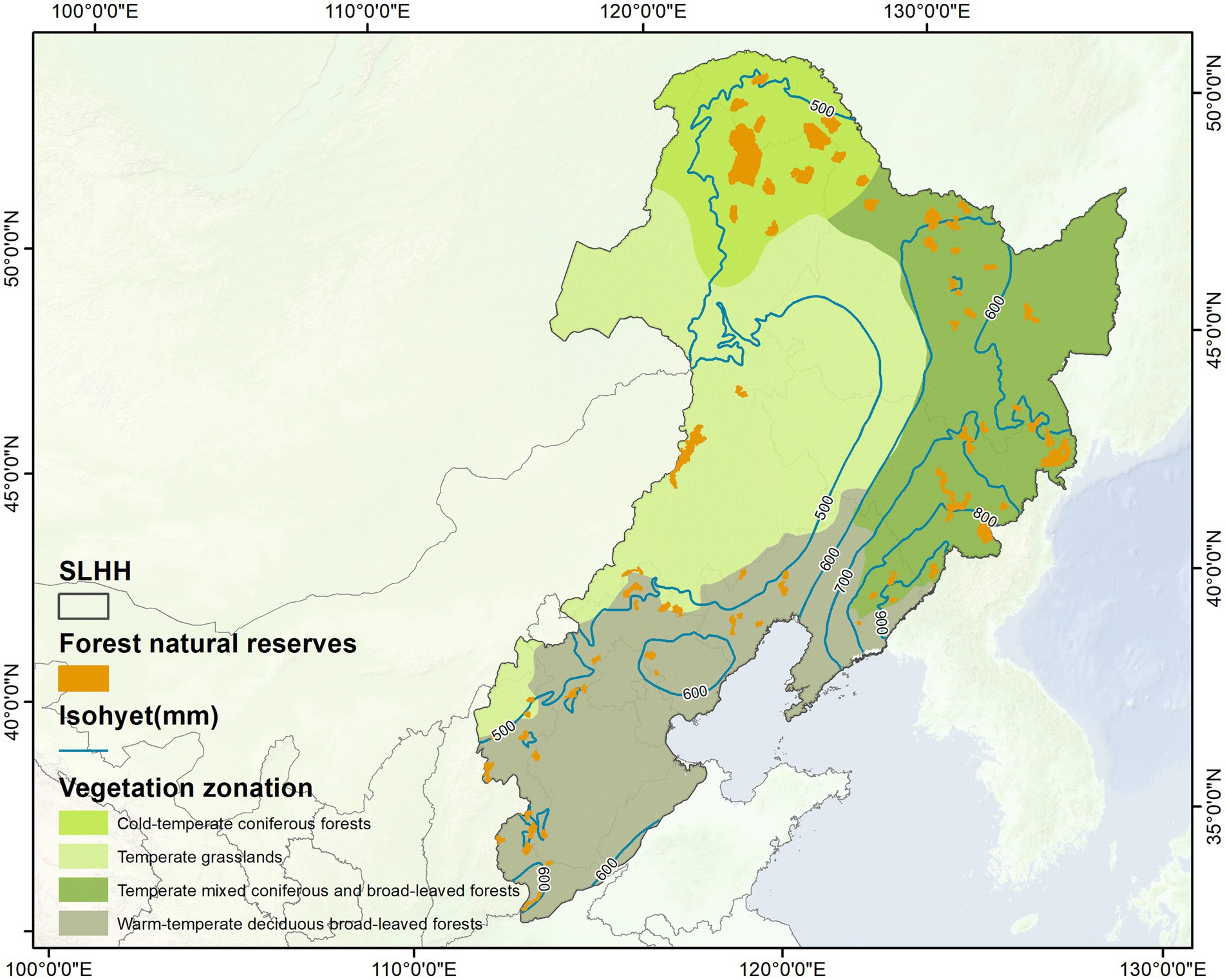
Figure 1. Distribution of precipitation gradients and forest natural reserves in the Songliao River Basin and Haihe River Basin, Northern China.
The forests of this region are not only capable of providing high-quality supplies and support services, but are also essential for local and regional regulation services (Mao et al., 2019). Climate fluctuations at large spatial and temporal scales will have irreversible effects on the distribution and function of forest ecosystems. In order to remove the influence of human disturbance, forest in 77 nature reserves were selected as typical objects (Xu et al., 2017), and the forest types are mainly coniferous broad-leaved mixed forest and deciduous broad-leaved forest. Based on dry-wet boundary, precipitation is divided into five gradients every 100 mm (i.e., <500, 500–600, 600–700, 700–800, and >800 mm).
2.2. Quantification of ecosystem services
Based on the previous studies on temperate forest ESs, three major ESs were selected, i.e., net primary product (NPP), soil conservation (SC), and water yield (WY) (Li et al., 2020; Li X. et al., 2021; Wang R. et al., 2022).
2.2.1. Net primary productivity
Net primary productivity (NPP) refers to the total amount of dry organic matter accumulated by vegetation per unit of time and area. It measures the net amount of carbon fixed in vegetation by photosynthesis of (Field et al., 1998). NPP is an important component of the terrestrial carbon cycle, not only directly reflecting the production capacity of vegetation communities under natural environmental conditions, representing the quality of the land ecosystem, but also determining the main factors of the carbon source and sink and regulating the ecological process, playing an important role in global change and carbon balance (Zhao and Running, 2010; Ji et al., 2020).
2.2.2. Soil conservation
Soil conservation service is the regulatory capacity of an ecosystem to prevent soil erosion. In this study, soil conservation is calculated based on the Revised Universal Soil Loss Equation (RUSLE), using the difference between actual and potential soil erosion (Ouyang et al., 2016). The calculation method is as follows:
2.2.3. Water yield
In this study, the water yield module of the InVEST model was used for the simulation estimation of water yield (Sharp et al., 2018). The module is based on the Budyko curve and the average annual rainfall (Hamel and Guswa, 2015). The principle is briefly defined as the amount of precipitation per calculation unit minus the amount of water lost to storage and evapotranspiration, and the difference is the water yield (Ouyang et al., 2016), as follows:
2.3. Data sources
Based on regional precipitation frequency analysis, the year 2015, which is closest to the precipitation frequency p = 50% (around 617.8 mm), is selected as the reference year. The datasets involved used for assessing ESs based on the ArcGIS platform were listed in Table 1. ANUSPLIN interpolation was used for station data, and the spatial scale of raster data was finally unified to 1 km.
2.4. Analysis of trade-offs and synergies of ecosystem services
To derive trade-off/synergistic relationships between multiple ecosystem services, we analyzed the correlation between the two ecosystem services using the Spearman correlation test using the R language. A negative correlation coefficient indicates a trade-off between the two ESs, which means that as one ES increases, the other decreases. In contrast, a positive value indicates a synergistic relationship that grows or synergizes. The larger the absolute value of the correlation coefficient, the stronger the trade-off/synergy between the ESs and vice versa.
where, and represent the rank of two ESs, is the correlation coefficient between and ; n is the rainfall gradient; is the correlation coefficient between and at rainfall gradient n. >0 indicates a synergistic relationship between and ; = 0 indicates no correlation between and ; < 0 indicates a trade-off relationship between and ; a more significant of indicates a stronger correlation between and .
2.5. Redundancy analysis
Redundancy analysis (RDA) is a direct gradient analysis extension of principal components analysis (Šmilauer and Lepš, 2014). It can identify trends in the scatter of data points that are maximally and linearly related to a set of constraining (explanatory) variables (Makarenkov and Legendre, 2002). Mostly used by researchers to test hypotheses relating response (e.g., species data) to explanatory (e.g., environmental) variables (Legendre and Anderson, 1999; Dai et al., 2020). In this study, ES were clustered to better understand the analysis of the relationships between ES under different rainfall gradients (trade-offs and synergies) and their relations to other environmental factors.
3. Results
3.1. Correlations between ESs and precipitation
Throughout the study area (Figure 2), there was a significantly synergistic correlation with ecosystem services, with NPP having the strongest correlation, followed by WY and SC. Between ESs, WY had a significant synergy relationship with NPP and SC, respectively, and there was no significant correlation between NPP and SC.
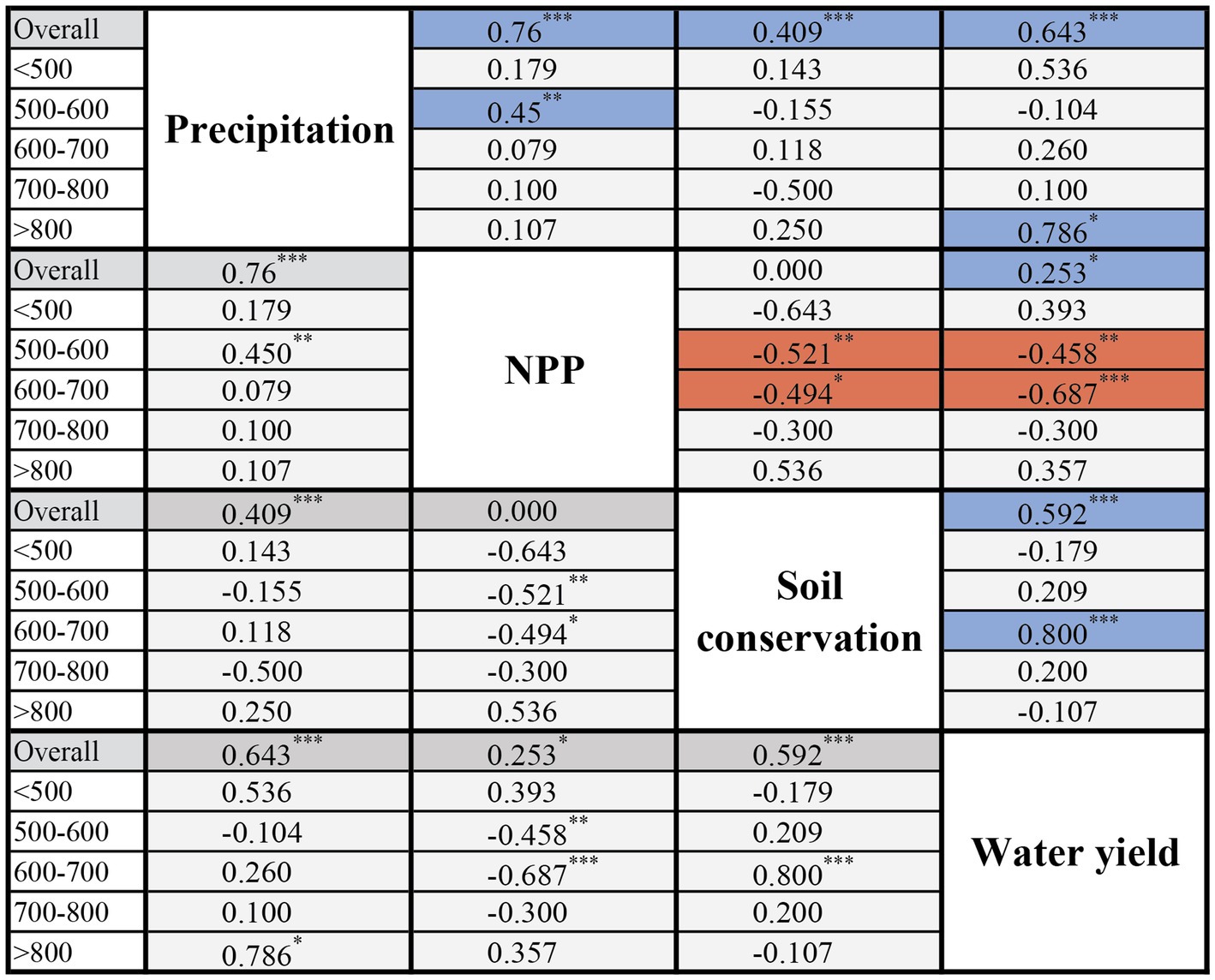
Figure 2. Relationship between ESs and precipitation. ***p < 0.001; **p < 0.01; *p < 0.05. Blue and red represent significant positive and negative, respectively. Gray represents all the study areas, and successive ones represent different precipitation gradients.
Under different precipitation gradients, the heterogeneity of the relationships among ESs and precipitation was obvious. Precipitation has a synergistic correlation with NPP only at 500-600 mm gradient, and with water yield at >800 mm gradient. From the perspective of internal relations between ESs, synergistic relationship was found only between soil conservation and water yield at 600–700 mm gradient. Besides, NPP exhibited trade-off relationship with the other ESs at 500–600 and 600–700 mm gradients.
3.2. Characteristics of ESs along the precipitation gradients
As shown in Figure 3, all three ESs upraised with the increase of precipitation, but there was significant variability in the growth trend. By the analysis of variance between groups, it was also found that there was considerable variability in the service values between different precipitation gradients. The mean values of NPP under different rainfall gradients were 481.4 g C/m2, 546.1 g C/m2, 657.2 g C/m2, 694.5 g C/m2, and 710.7 g C/m2, respectively, with the growth rate decreased significantly over 600–700 mm of gradient. The mean values of soil conservation under different rainfall gradients were 77.7 t/km2, 139.8 t/km2, 259.5 t/km2, 441.9 t/km2, and 535.4 t/km2, respectively, with the growth rate increased significantly over 600–700 mm of precipitation. The mean values of WY under five rainfall gradients were 8.4, 30.8, 50.9, 67.4, and 118.2 mm, respectively, with a significantly increased growth rate over the 600–700 mm of precipitation.
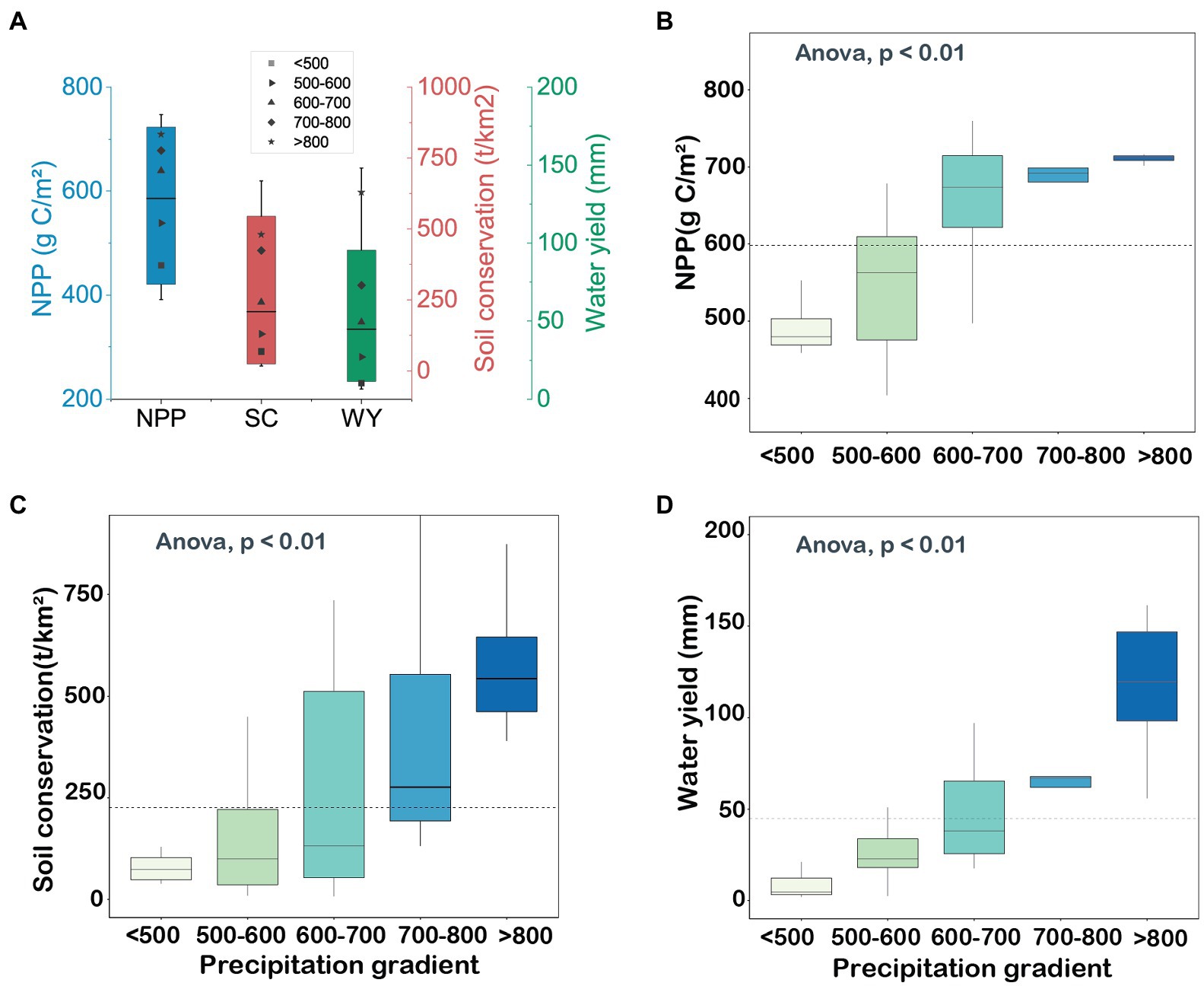
Figure 3. Three ESs in the forest natural reserves and the average of ESs under each precipitation gradient (A); Variation of ESs along the precipitation gradients (B: NPP; C: Soil conservation; D: Water yield).
3.3. Ecosystem services relationships under different precipitation gradients
The relationships between NPP, SC, and WY showed different trends under precipitation gradients (Figures 4A–C). The relation between NPP and SC showed a shift from tradeoff to synergy with rainfall increase. The relations of NPP with soil conservation and water yield showed an upward parabolic and downward parabolic trend, respectively, along the rainfall gradient. The peak and valley values of the relationship both appear in the precipitation gradient of 600-700 mm.
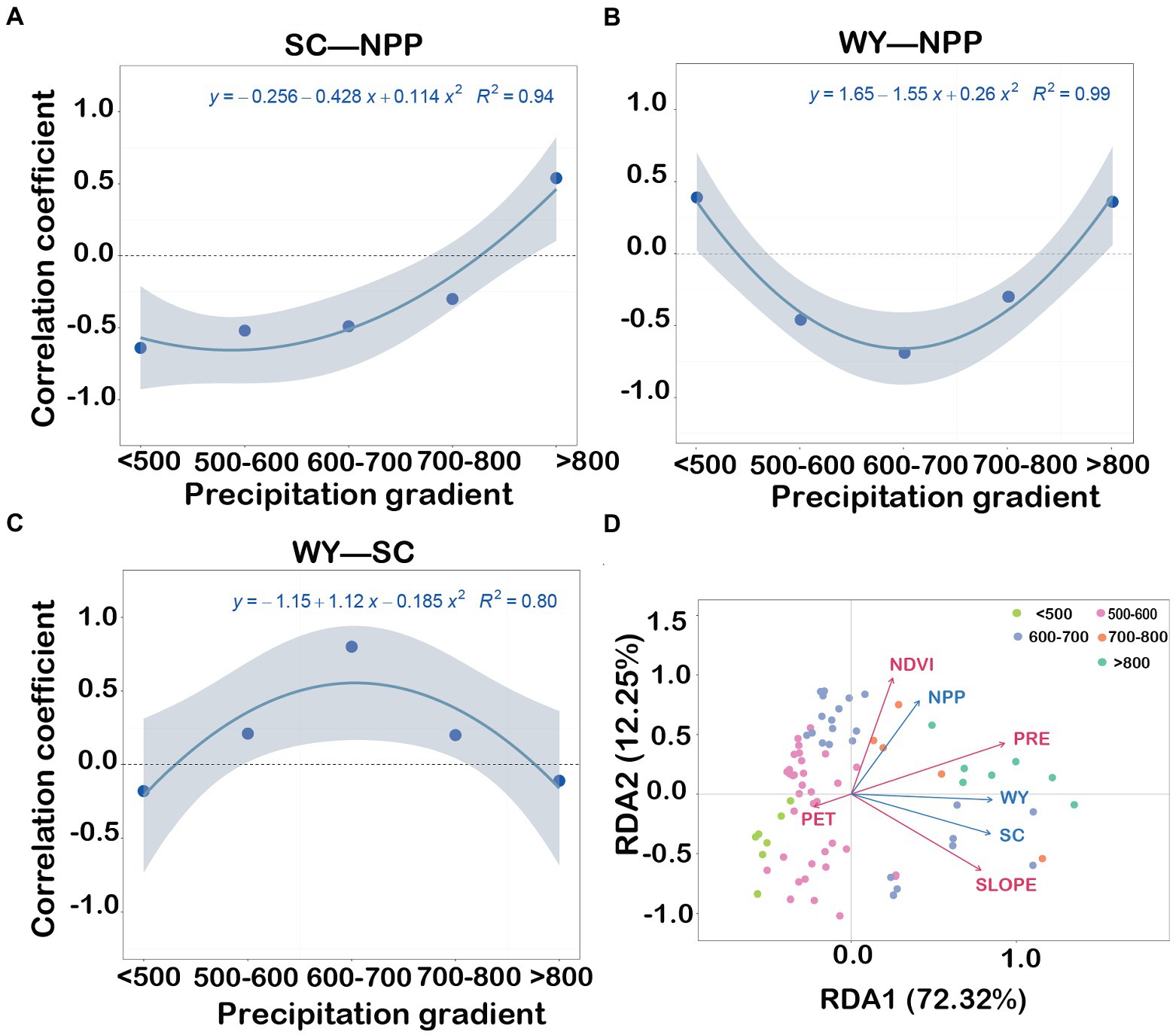
Figure 4. Changes in ES relationships between precipitation gradients [(A) soil conservation and NPP; (B) water yield and NPP; (C) water yield and soil conservation and RDA analysis (D)]. SC, soil conservation; WC, water yield; and PET, potential evapotranspiration. Explanation of ESs by influences in RDA and the permutation test result is 0.002, the explanatory variables account for 85.1% (adjusted explained variation is 83.3%).
RDA results (Figure 4D) showed that precipitation was the most contributing meteorological factor (with contribution of 21.2%), after the slope (with contribution of 68.5%), followed by NDVI (4.5%) and Potential evapotranspiration (3.2%). Precipitation showed a positive correlation with all three ESs, while WY and SC showed more distinct clustering than NPP at different precipitation gradients. ESs under 600 mm precipitation are more distributed in the third quadrant, which also indicated that ESs are relatively small in these gradients. Under a 600–700 mm gradient, ESs are distributed in the second or fourth quadrant, meaning that there is a clear trade-off between NPP, WY, and SC. Meanwhile, in areas with precipitation less than 700, WY and SC are significantly influenced by slope, temperature and potential evapotranspiration. In areas with precipitation greater than 700, ESs are all in the first quadrant, which indicates that the ESs are relatively high, and the increasing trend is reflected in SC and WY driven by NDVI and slope.
4. Discussion
4.1. Response of key ESs and their relations to precipitation gradient
Our results quantify NPP, SC, and WY in temperate forest ecosystem, which are consistent with previous studies in northern China (Wang C. et al., 2020; Qi et al., 2021; Yan et al., 2021). Although the three ESs showed an increasing trend with precipitation, there was a clear difference in the rate of increase with the 600–700 mm gradient as the dividing line (Figure 3). As precipitation increased, NPP tended to increase faster and then slower, on the contrary, WY and SC showed a increase of slow first and then fast. The main reason for this difference is that the water limitation threshold in the climax community of temperate forest is about 700 mm (Chi et al., 2018). In the area where the rainfall is less than 700 mm, the forest community gradually succeed from shrubbery to arboreal forest, which leads to rapid increase in vegetation productivity and NPP. However, the vegetation cover is not yet high, and the water surplus is low after meeting the needs of vegetation growth, the growth of SC and WY is slow. In areas where rainfall is greater than 700 mm, the vegetation community structure is stable and the growth of NPP slows down. Moisture conditions in this area are already adequate for forest growth, so WY has increased significantly. Although increased precipitation also intensified the risk of soil erosion, these negative effects were offset by changes in forest structure and improvements in the water-holding capacity of the litter and soil water storage capacity, minimizing actual soil erosion (Hart and Frasier, 2003; Wu et al., 2020).
In addition, the relationship between the three ESs showed different trends with the precipitation gradient (Figure 4). As precipitation increased, the relationship between NPP and SC changed from a trade-off to synergy, while the synergy and trade-off between WY with SC and NPP reached the maximum on the 600–700 mm precipitation gradient. For NPP and SC, increased precipitation improved vegetation cover, while increased temperature and vegetation uptake accelerated soil water evaporation and soil erosion (Lu et al., 2014; Wang H. et al., 2020), resulting in the relationship between NPP and SC changing from a trade-off to a synergy (Figure 4D). For NPP and WY, NPP increased significantly with precipitation, and the succession of shrubbery to arboreal forest led to a relative decrease in WY, which intensifies the trade-off; after the community structure stabilized (above the 600–700 mm precipitation gradient), NPP and WY gradually reverted to synergy. For WY and SC, in areas with less precipitation, where shrubs still account for a certain proportion, and the combined effects of vegetation structure succession, temperature and other environmental factors keep SC and WY at a low level, showing a synergy between them; in areas with high precipitation (>700 mm), the growth of forest has been less restricted by water conditions (Chi et al., 2018), and excessive precipitation will cause soil erosion, making the synergistic relationship between SC and WY gradually weakened (Dai et al., 2022; Li et al., 2022).
4.2. Response of ESs and their relations to other environment factors
Slope (contribution = 68.5%; p < 0.01) was the most important factor influencing the relationship between ESs and ESs, and had a strong impact on ESs in areas with rainfall greater than 600–700 mm. Under this gradient, water demand of forest has stabilized, and slope can directly influence runoff velocity, alter infiltration, and have a positive correlation with erosion rates (Sun et al., 2018; Vanacker et al., 2019). Larger slope enhance the possibility for runoff generation (Hümann et al., 2011; Balasubramanian, 2017), increase the scouring force on the soil surface (Emmett, 1968), and change the relationship between WY and SC from synergy to trade-off. Also, slope is an important factor influencing local meteorological factor, which is closely related to precipitation (contribution = 1.0%; p < 0.05).
NDVI can reflect the state of vegetation coverage and biomass, and is influenced by environmental conditions such as precipitation and temperature (Defries and Townshend, 1994; Seidl et al., 2020). In the RDA results, NDVI (contribution = 4.5%; p < 0.01) was the important vegetation factor affecting NPP and WY, and was correlated with precipitation significantly and positively. Under the gradient of less than 600–700 mm, vegetation growth and carbon sequestration in temperate forest are limited by water (Mao et al., 2014; Higgins et al., 2023); when rainfall is greater than 700 mm, community succession and vegetation cover in temperate forests stabilize, and ESs become progressively less influenced by NDVI.
Potential evapotranspiration (PET) is comprehensively affected by meteorological factors, higher PET means less water resources available (Donohue et al., 2010; Tu and Yang, 2022). In the RDA results, PET (contribution = 3.2%; p < 0.01) had a significant negative effect on ESs in areas with less than 600 precipitation, indicating that in areas with less precipitation, precipitation has difficulty in meeting the water requirements of vegetation growth, which limit the provision of temperate forest ESs (Rohatyn et al., 2018), and will also exacerbates trade-off between ESs.
Except for the four environmental factors SLOPE, PRE, NDVI, and PET that have outstanding contributions, the influence of other environmental factors is not significant: temperature (contribution = 1.0%; p = 0.042), vegetation type (contribution = 0.2%; p = 0.8) and soil type (contribution = 0.7%; p = 0.1). At the same time, based on the multi-year average temperature gradient, and it was found that there was no inter-group difference in WY under different temperature gradients (ANOVA; p = 0.085) (Supplementary Figure S1). It can be inferred when precipitation increases and the vegetation community tends to be stable, the sensitivity of forest water yield changes to temperature will be weakened (Zhou et al., 2015; Chen X. et al., 2021).
4.3. Implications of ESs interrelations for forest restoration and managements
Since 1960, both Northeast China and North China have experienced a shift from wet to dry climates (Fu et al., 2009; Shi et al., 2014), however, in the context of global climate change, it has been predicted that the future climate change in northern China will tend to be more wetter than warmer (Wu et al., 2010; Liu et al., 2017; Hui et al., 2018).
The increase in rainfall and temperature will favor the growth of promote the increase in the extent of coniferous and broad-leaf forests and broadleaf forests (Zhao et al., 2013; Zhang et al., 2022). The future increase of precipitation will bring westward shift of precipitation contour in northern China. For the new area with rainfall from 400 mm to 700 mm, it is vital to select vegetation types based on matching environmental elements such as temperature and topography with plant characteristics. Besides, Higher soil erosion rate may also be closely related to local topography, slope, temperature and other environmental factors (Fang and Guo, 2015). In areas with a future rainfall gradient of below 600 mm, the scrub-dominated vegetation types require less water for growth and are less capable of soil conservation, promoting a shift to a trade-off between the two ESs, and therefore further soil conservation measures are needed in this part of the area. Moreover, in the area where rainfall may increase to more than 700 mm, there is still a need to focus on the construction of soil and water conservation forests to cope with the potential for increased trade-offs. In addition, to better capture the inflection points and thresholds of ESs trade-offs, it is suggested that additional ground-based data monitoring sites should be added in the future, to avoid possible errors in ESs assessment of this study. For other regions that are significantly affected by global climate change, e.g., the forest area of the southeast Tibetan Plateau, attention should also be paid to the changes in ES relationships. Studies predict that the alpine meadows in the hinterland of the plateau will be converted to shrub areas on a large scale (Korcz et al., 2021). Our results reveal that in the region dominated by shrubs, particular attention should be paid to the trade-offs between vegetation growth and water related ESs (Jiang C. et al., 2021; Jia et al., 2022). Therefore, in the restoration and management of vegetation in these areas, it is necessary to comprehensively consider the vegetation selection, the trade-off between vegetation water use and other water uses such as grain production, in order to reduce the trade-off between ESs.
5. Conclusion
This study revealed the response of major ESs interrelationships to precipitation gradient in temperate forest of northern China. The results show that 600–700 mm is a clear inflection point of the ESs and their correlations. The tradeoff between the regulation services (SC and NPP) gradually turned into synergy with the increase in precipitation, while the relationship between supply (WY) and regulation services (SC and NPP) presents a parabolic relationship.
The results indicated that afforestation and forest management should pay attention to the selection of tree species and the density of planting in areas where precipitation is less than 700 mm; while in high precipitation area, especially in mountainous areas, soil and water conservation projects should be paid focused. This study improve the current understanding of the impact of rainfall on ESs and relationships in temperate forests and provide predictive information to proactively address the potential damage to ESs in temperate forests from climate change.
Data availability statement
The raw data supporting the conclusions of this article will be made available by the authors, without undue reservation.
Author contributions
ML and RL: conceptualization. ML, JM, and RL: methodology. ML, TH, RL, XQ, HS, and YY: investigation and data. ML, RL, and HZ: writing – original draft preparation. ML, TH, JM, RL, YY, XQ, HS, and HZ: writing—review and editing. All authors have read and agreed to the published version of the manuscript.
Funding
This work was supported by the National Natural Science Foundation of China (42171099 and 41871218).
Conflict of interest
The authors declare that the research was conducted in the absence of any commercial or financial relationships that could be construed as a potential conflict of interest.
Publisher’s note
All claims expressed in this article are solely those of the authors and do not necessarily represent those of their affiliated organizations, or those of the publisher, the editors and the reviewers. Any product that may be evaluated in this article, or claim that may be made by its manufacturer, is not guaranteed or endorsed by the publisher.
Supplementary material
The Supplementary material for this article can be found online at: https://www.frontiersin.org/articles/10.3389/fevo.2023.1132396/full#supplementary-material
Footnotes
References
Alamgir, M., Turton, S. M., Macgregor, C. J., and Pert, P. L. (2016). Assessing regulating and provisioning ecosystem services in a contrasting tropical forest landscape. Ecol. Indic. 64, 319–334. doi: 10.1016/j.ecolind.2016.01.016
Anderegg, W. R. L., Wu, C., Acil, N., Carvalhais, N., Pugh, T. A. M., Sadler, J. P., et al. (2022). A climate risk analysis of Earth’s forests in the 21st century. Science 377, 1099–1103. doi: 10.1126/science.abp9723
Balasubramanian, A., (2017). Surface water runoff. India: Centre for Advanced Studies in Earth Science, University of Mysore.
Biber, P., Felton, A., Nieuwenhuis, M., Lindbladh, M., Black, K., Bahýl', J., et al. (2020). Forest biodiversity, carbon sequestration, and Wood production: modeling synergies and trade-offs for ten Forest landscapes across Europe. Front. Ecol. Evol. 8:547696. doi: 10.3389/fevo.2020.547696
Brockerhoff, E. G., Barbaro, L., Castagneyrol, B., Forrester, D. I., Gardiner, B., González-Olabarria, J. R., et al. (2017). Forest biodiversity, ecosystem functioning and the provision of ecosystem services. Biodivers. Conserv. 26, 3005–3035. doi: 10.1007/s10531-017-1453-2
Chen, X., Liu, L., Su, Y., Yuan, W., Liu, X., Liu, Z., et al. (2021). Quantitative association between the water yield impacts of forest cover changes and the biophysical effects of forest cover on temperatures. J. Hydrol. 600:126529. doi: 10.1016/j.jhydrol.2021.126529
Chen, J., Wang, Y., Sun, J., Liang, E., Shen, M., Yang, B., et al. (2021). Precipitation dominants synergies and trade-offs among ecosystem services across the Qinghai-Tibet plateau. Glob. Ecol. Conserv. 32:e01886. doi: 10.1016/j.gecco.2021.e01886
Chi, D., Wang, H., Li, X., Liu, H., and Li, X. (2018). Estimation of the ecological water requirement for natural vegetation in the Ergune River basin in northeastern China from 2001 to 2014. Ecol. Indic. 92, 141–150. doi: 10.1016/j.ecolind.2017.04.014
Dai, X., Wang, L., Huang, C., Fang, L., Wang, S., and Wang, L. (2020). Spatio-temporal variations of ecosystem services in the urban agglomerations in the middle reaches of the Yangtze River, China. Ecol. Indic. 115:106394. doi: 10.1016/j.ecolind.2020.106394
Dai, E., Wang, X., Zhu, J., and Tian, Q. (2022). Quantifying co-benefits and trade-offs between Forest ecosystem Services in the Gan River Basin of South China. Sustainability 14:8271. doi: 10.3390/su14148271
Defries, R. S., and Townshend, J. R. G. (1994). NDVI-derived land cover classifications at a global scale. Int. J. Remote Sens. 15, 3567–3586. doi: 10.1080/01431169408954345
Ding, Z., Zheng, H., Wang, J., O’Connor, P., Li, C., Chen, X., et al. (2022). Integrating top-down and bottom-up approaches improves practicality and efficiency of large-scale ecological restoration planning: insights from a social-ecological system. Engineering. doi: 10.1016/j.eng.2022.08.008
Ditt, E. H., Mourato, S., Ghazoul, J., and Knight, J. (2010). Forest conversion and provision of ecosystem services in the Brazilian Atlantic Forest. Land Degrad. Dev. 21, 591–603. doi: 10.1002/ldr.1010
Donohue, R. J., McVicar, T. R., and Roderick, M. L. (2010). Assessing the ability of potential evaporation formulations to capture the dynamics in evaporative demand within a changing climate. J. Hydrol. 386, 186–197. doi: 10.1016/j.jhydrol.2010.03.020
Emmett, W. W. (1968). “The hydraulics of overland flow on hillslopes” in Dynamic and Descriptive Studies of Hillslopes (Geological Survey Professional Paper 662-A). U.S. Govt. Print. Off. Available at: https://doi.org/10.3133/pp662A
Fang, H., and Guo, M. (2015). Aspect-induced differences in soil erosion intensity in a gullied hilly region on the Chinese loess plateau. Environ. Earth Sci. 74, 5677–5685. doi: 10.1007/s12665-015-4648-4
Fang, J., Piao, S., Zhou, L., He, J., Wei, F., Myneni, R. B., et al. (2005). Precipitation patterns alter growth of temperate vegetation. Geophys. Res. Lett. 32:L21411. doi: 10.1029/2005GL024231
FAO. (2020). Global Forest Resources Assessment 2020. Food and Agriculture Organization of the United Nations (FAO). Available at: https://doi.org/10.4060/ca8753en
Feng, J., Chen, F., Tang, F., Wang, F., Liang, K., He, L., et al. (2022). The trade-offs and synergies of ecosystem Services in Jiulianshan National Nature Reserve in Jiangxi Province, China. Forests 13:416. doi: 10.3390/f13030416
Field, C. B., Behrenfeld, M. J., Randerson, J. T., and Falkowski, P. (1998). Primary production of the biosphere: integrating terrestrial and oceanic components. Science 281, 237–240. doi: 10.1126/science.281.5374.237
Fu, G., Charles, S. P., Yu, J., and Liu, C. (2009). Decadal climatic variability, trends, and future scenarios for the North China plain. J. Clim. 22, 2111–2123. doi: 10.1175/2008JCLI2605.1
Galicia, L., Gómez-Mendoza, L., and Magaña, V. (2015). Climate change impacts and adaptation strategies in temperate forests in Central Mexico: a participatory approach. Mitig. Adapt. Strateg. Glob. Change 20, 21–42. doi: 10.1007/s11027-013-9477-8
Gamfeldt, L., Snäll, T., Bagchi, R., Jonsson, M., Gustafsson, L., Kjellander, P., et al. (2013). Higher levels of multiple ecosystem services are found in forests with more tree species. Nat. Commun. 4:1340. doi: 10.1038/ncomms2328
Gilliam, F. S. (2016). Forest ecosystems of temperate climatic regions: from ancient use to climate change. New Phytol. 212, 871–887. doi: 10.1111/nph.14255
Hamel, P., and Guswa, A. J. (2015). Uncertainty analysis of a spatially explicit annual water-balance model: case study of the cape fear basin, North Carolina. Hydrol. Earth Syst. Sci. Discuss. 19, 839–853. doi: 10.5194/hess-19-839-2015
Hart, R. H., and Frasier, G. W. (2003). Bare ground and litter as estimators of runoff on short- and mixed-grass prairie. Arid Land Res. Manage. 17, 485–490. doi: 10.1080/713936119
Hely, C., Bremond, L., Alleaume, S., Smith, B., Sykes, M. T., and Guiot, J. (2006). Sensitivity of African biomes to changes in the precipitation regime. Glob. Ecol. Biogeogr. 15, 258–270. doi: 10.1111/j.1466-822X.2006.00235.x
Higgins, S. I., Conradi, T., and Muhoko, E. (2023). Shifts in vegetation activity of terrestrial ecosystems attributable to climate trends. Nat. Geosci. 16, 147–153. doi: 10.1038/s41561-022-01114-x
Hui, P., Tang, J., Wang, S., Niu, X., Zong, P., and Dong, X. (2018). Climate change projections over China using regional climate models forced by two CMIP5 global models. Part II: projections of future climate: Projections of climate over China with multi-RCM driven by CMIP5 GCM. Int. J. Climatol. 38, e78–e94. doi: 10.1002/joc.5409
Hümann, M., Schüler, G., Müller, C., Schneider, R., Johst, M., and Caspari, T. (2011). Identification of runoff processes – the impact of different forest types and soil properties on runoff formation and floods. J. Hydrol. 409, 637–649. doi: 10.1016/j.jhydrol.2011.08.067
Ji, Y., Zhou, G., Luo, T., Dan, Y., Zhou, L., and Lv, X. (2020). Variation of net primary productivity and its drivers in China’s forests during 2000–2018. For. Ecosyst. 7:15. doi: 10.1186/s40663-020-00229-0
Jia, G., Dong, Y., Zhang, S., He, X., Zheng, H., Guo, Y., et al. (2022). Spatiotemporal changes of ecosystem service trade-offs under the influence of forest conservation project in Northeast China. Front. Ecol. Evol. 10:978145. doi: 10.3389/fevo.2022.978145
Jiang, C., Guo, H., Wei, Y., Yang, Z., Wang, X., Wen, M., et al. (2021). Ecological restoration is not sufficient for reconciling the trade-off between soil retention and water yield: a contrasting study from catchment governance perspective. Sci. Total Environ. 754:142139. doi: 10.1016/j.scitotenv.2020.142139
Jiang, C., Li, D., Wang, D., and Zhang, L. (2016). Quantification and assessment of changes in ecosystem service in the Three-River headwaters region, China as a result of climate variability and land cover change. Ecol. Indic. 66, 199–211. doi: 10.1016/j.ecolind.2016.01.051
Jiang, R., Sun, L., Sun, C., and Liang, X.-Z. (2021). CWRF downscaling and understanding of China precipitation projections. Clim. Dyn. 57, 1079–1096. doi: 10.1007/s00382-021-05759-z
Jiang, Z., Tian, Z., Dong, G., Sun, L., Zhang, P., Buonomo, E., et al. (2020). High-resolution projections of mean and extreme precipitation over China by two regional climate models. J. Meteorol. Res. 34, 965–985. doi: 10.1007/s13351-020-9208-5
Jiao, Y., Bu, K., Yang, J., Li, G., Shen, L., Liu, T., et al. (2021). Biophysical effects of temperate forests in regulating regional temperature and precipitation pattern across Northeast China. Remote Sens. 13:4767. doi: 10.3390/rs13234767
Korcz, N., Koba, J., Kobyłka, A., Janeczko, E., and Gmitrowicz-Iwan, J. (2021). Climate change and informal education in the opinion of Forest users in Poland. Sustainability 13:7892. doi: 10.3390/su13147892
Legendre, P., and Anderson, M. J. (1999). Distance-based redundancy analysis: testing multispecies responses in multifactorial ecological experiments. Ecol. Monogr. 69, 1–24. doi: 10.1890/0012-9615(1999)069[0001:DBRATM]2.0.CO;2
Li, M., Liang, D., Xia, J., Song, J., Cheng, D., Wu, J., et al. (2021). Evaluation of water conservation function of Danjiang River basin in Qinling Mountains, China based on InVEST model. J. Environ. Manage. 286:112212. doi: 10.1016/j.jenvman.2021.112212
Li, Z., Lu, Y., Wang, Y., and Liu, J. (2022). The Spatio-temporal evolution of the soil conservation function of ecosystems in the north–south transition zone in China: a case study of the Qinling-Daba Mountains. Sustainability 14:5829. doi: 10.3390/su14105829
Li, J., Wang, Y., and Liu, L. (2020). Responses of the terrestrial ecosystem productivity to droughts in China. Front. Earth Sci. 8:59. doi: 10.3389/feart.2020.00059
Li, X., Zhang, C., Zhang, B., Wu, D., Zhu, D., Zhang, W., et al. (2021). Nitrogen deposition and increased precipitation interact to affect fine root production and biomass in a temperate forest: implications for carbon cycling. Sci. Total Environ. 765:144497. doi: 10.1016/j.scitotenv.2020.144497
Liu, J., Du, H., Wu, Z., He, H. S., Wang, L., and Zong, S. (2017). Recent and future changes in THE combination of annual temperature and precipitation throughout China: changes in the combination of temperature and precipitation over China. Int. J. Climatol. 37, 821–833. doi: 10.1002/joc.4742
Liu, L., Wang, Z., Wang, Y., Zhang, Y., Shen, J., Qin, D., et al. (2019). Trade-off analyses of multiple mountain ecosystem services along elevation, vegetation cover and precipitation gradients: a case study in the Taihang Mountains. Ecol. Indic. 103, 94–104. doi: 10.1016/j.ecolind.2019.03.034
Liu, Z., Ye, L., Wei, Z., Jiang, J., Zhang, Q., and Lv, X. (2022). Water use by trees is linked to precipitation: a case study of a mixed forest in a hilly area in southern China. Ecol. Indic. 143:109343. doi: 10.1016/j.ecolind.2022.109343
Lozano-Parra, J., Schnabel, S., and Ceballos-Barbancho, A. (2015). The role of vegetation covers on soil wetting processes at rainfall event scale in scattered tree woodland of Mediterranean climate. J. Hydrol. 529, 951–961. doi: 10.1016/j.jhydrol.2015.09.018
Lu, N., Fu, B., Jin, T., and Chang, R. (2014). Trade-off analyses of multiple ecosystem services by plantations along a precipitation gradient across loess plateau landscapes. Landsc. Ecol. 29, 1697–1708. doi: 10.1007/s10980-014-0101-4
Makarenkov, V., and Legendre, P. (2002). Nonlinear redundancy analysis and canonical correspondence analysis based on polynomial regression. Ecology 83, 1146–1161. doi: 10.1890/0012-9658(2002)083[1146:NRAACC]2.0.CO;2
Mamat, Z., Halik, Ü., Keyimu, M., Keram, A., and Nurmamat, K. (2018). Variation of the floodplain forest ecosystem service value in the lower reaches of Tarim River, China. Land Degrad. Rehab. 29, 47–57. doi: 10.1002/ldr.2835
Mao, D., He, X., Wang, Z., Tian, Y., Xiang, H., Yu, H., et al. (2019). Diverse policies leading to contrasting impacts on land cover and ecosystem services in Northeast China. J. Clean. Prod. 240:117961. doi: 10.1016/j.jclepro.2019.117961
Mao, D., Wang, Z., Wu, C., Song, K., and Ren, C. (2014). Examining forest net primary productivity dynamics and driving forces in northeastern China during 1982–2010. Chin. Geogr. Sci. 24, 631–646. doi: 10.1007/s11769-014-0662-9
Nelson, H. P., Devenish-Nelson, E. S., Rusk, B. L., Geary, M., and Lawrence, A. J. (2018). A call to action for climate change research on Caribbean dry forests. Reg. Environ. Chang. 18, 1337–1342. doi: 10.1007/s10113-018-1334-6
Ouyang, Z., Zheng, H., Xiao, Y., Polasky, S., Liu, J., Xu, W., et al. (2016). Improvements in ecosystem services from investments in natural capital. Science 352, 1455–1459. doi: 10.1126/science.aaf2295
Qi, L., Zhang, Y., Xu, D., Zhu, Q., Zhou, W., Zhou, L., et al. (2021). Trade-offs and synergies of ecosystem services in forest barrier belt of Northeast China. Chin. J. Ecol. 40, 3401–3411. doi: 10.13292/j.1000-4890.202111.020
Rodrigues, A. R., Botequim, B., Tavares, C., Pécurto, P., and Borges, J. G. (2020). Addressing soil protection concerns in forest ecosystem management under climate change. For. Ecosyst. 7:34. doi: 10.1186/s40663-020-00247-y
Rohatyn, S., Rotenberg, E., Ramati, E., Tatarinov, F., Tas, E., and Yakir, D. (2018). Differential impacts of land use and precipitation on “ecosystem water yield.”. Water Resour. Res. 54, 5457–5470. doi: 10.1029/2017WR022267
Seidl, R., Honkaniemi, J., Aakala, T., Aleinikov, A., Angelstam, P., Bouchard, M., et al. (2020). Globally consistent climate sensitivity of natural disturbances across boreal and temperate forest ecosystems. Ecography 43, 967–978. doi: 10.1111/ecog.04995
Seidl, R., Thom, D., Kautz, M., Martin-Benito, D., Peltoniemi, M., Vacchiano, G., et al. (2017). Forest disturbances under climate change. Nat. Clim. Chang. 7, 395–402. doi: 10.1038/nclimate3303
Sharp, R., Chaplin-Kramer, R., Wood, S., Guerry, A., Tallis, H., Ricketts, T., et al. (2018). InVEST3.2.0: User’s Guide. The Natural Capital Project, Stanford University, University of Minnesota, The Nature Conservancy, and World Wildlife Fund. Available at: https://doi.org/10.13140/RG.2.2.32693.78567
Shi, P., Sun, S., Wang, M., Li, N., Wang, J., Jin, Y., et al. (2014). Climate change regionalization in China (1961–2010). Sci. China Earth Sci. 57, 2676–2689. doi: 10.1007/s11430-014-4889-1
Šmilauer, P., and Lepš, J. (2014). Multivariate analysis of ecological data using CANOCO 5. 2nd Edn Cambridge University Press.
Suescún, D., Villegas, J. C., León, J. D., Flórez, C. P., García-Leoz, V., and Correa-Londoño, G. A. (2017). Vegetation cover and rainfall seasonality impact nutrient loss via runoff and erosion in the Colombian Andes. Reg. Environ. Chang. 17, 827–839. doi: 10.1007/s10113-016-1071-7
Sun, J., Fan, D., Yu, X., and Li, H. (2018). Hydraulic characteristics of varying slope gradients, rainfall intensities and litter cover on vegetated slopes. Hydrol. Res. 49, 506–516. doi: 10.2166/nh.2017.097
Tang, J., Chen, X., Zhao, M., and Su, B. (2009). Numerical simulation of regional climate change under IPCC A2 scenario in China. J. Meteorol. Res. 23, 29–42.
Thom, D., Rammer, W., and Seidl, R. (2017). The impact of future forest dynamics on climate: interactive effects of changing vegetation and disturbance regimes. Ecol. Monogr. 87, 665–684. doi: 10.1002/ecm.1272
Thomas, E., Jansen, M., Chiriboga-Arroyo, F., Wadt, L. H. O., Corvera-Gomringer, R., Atkinson, R. J., et al. (2021). Habitat quality differentiation and consequences for ecosystem service provision of an Amazonian Hyperdominant tree species. Front. Plant Sci. 12:621064. doi: 10.3389/fpls.2021.621064
Thompson, A., Davis, J. D., and Oliphant, A. J. (2016). Surface runoff and soil erosion under eucalyptus and oak canopy: surface runoff and soil erosion under eucalyptus and oak canopy. Earth Surf. Process. Landf. 41, 1018–1026. doi: 10.1002/esp.3881
Tu, Z., and Yang, Y. (2022). On the estimation of potential evaporation under wet and dry conditions. Water Resour. Res. 58. doi: 10.1029/2021WR031486
Vanacker, V., Ameijeiras-Mariño, Y., Schoonejans, J., Cornélis, J.-T., Minella, J. P. G., Lamouline, F., et al. (2019). Land use impacts on soil erosion and rejuvenation in southern Brazil. Catena 178, 256–266. doi: 10.1016/j.catena.2019.03.024
Vatandaslar, C. (2022). Spatial assessment of ecosystem services provisioning changes in a forest-dominated protected area in NE Turkey. Environ. Monit. Assess. 194:539. doi: 10.1007/s10661-022-10212-7
Wan, J.-Z., Wang, C.-J., Qu, H., Liu, R., and Zhang, Z.-X. (2018). Vulnerability of forest vegetation to anthropogenic climate change in China. Sci. Total Environ. 621, 1633–1641. doi: 10.1016/j.scitotenv.2017.10.065
Wan, J.-Z., Wang, C.-J., and Yu, F.-H. (2017). Spatial conservation prioritization for dominant tree species of Chinese forest communities under climate change. Clim. Chang. 144, 303–316. doi: 10.1007/s10584-017-2044-7
Wang, Y., Dai, E., Ge, Q., Zhang, X., and Yu, C. (2021). Spatial heterogeneity of ecosystem services and their trade-offs in the Hengduan Mountain region, Southwest China. Catena (Amst) 207:105632. doi: 10.1016/j.catena.2021.105632
Wang, S., Fu, B.-J., He, C.-S., Sun, G., and Gao, G.-Y. (2011). A comparative analysis of forest cover and catchment water yield relationships in northern China. For. Ecol. Manage. 262, 1189–1198. doi: 10.1016/j.foreco.2011.06.013
Wang, C., Jiang, Q., Deng, X., Lv, K., and Zhang, Z. (2020). Spatio-temporal evolution, future trend and phenology regularity of net primary productivity of forests in Northeast China. Remote Sens. 12:3670. doi: 10.3390/rs12213670
Wang, R., Peng, Q., Zhang, W., Zhao, W., Liu, C., and Zhou, L. (2022). Ecohydrological service characteristics of Qilian Mountain ecosystem in the next 30 years based on scenario simulation. Sustainability 14:1819. doi: 10.3390/su14031819
Wang, H., Sun, B., Yu, X., Xin, Z., and Jia, G. (2020). The driver-pattern-effect connection of vegetation dynamics in the transition area between semi-arid and semi-humid northern China. Catena 194:104713. doi: 10.1016/j.catena.2020.104713
Wang, H., Wang, W. J., Liu, Z., Wang, L., Zhang, W., Zou, Y., et al. (2022). Combined effects of multi-land use decisions and climate change on water-related ecosystem services in Northeast China. J. Environ. Manage. 315:115131. doi: 10.1016/j.jenvman.2022.115131
Wang, C.-J., Zhang, Z.-X., and Wan, J.-Z. (2019). Vulnerability of global forest ecoregions to future climate change. Glob. Ecol. Conserv. 20:e00760. doi: 10.1016/j.gecco.2019.e00760
Weltzin, J. F., Loik, M. E., Schwinning, S., Williams, D. G., Fay, P. A., Haddad, B. M., et al. (2003). Assessing the response of terrestrial ecosystems to potential changes in precipitation. Bioscience 53:941. doi: 10.1641/0006-3568(2003)053[0941:ATROTE]2.0.CO;2
Wu, C., Qiu, D., Gao, P., Mu, X., and Zhao, G. (2022). Application of the InVEST model for assessing water yield and its response to precipitation and land use in the Weihe River basin, China. J. Arid. Land 14, 426–440. doi: 10.1007/s40333-022-0013-0
Wu, X., Shi, W., Guo, B., and Tao, F. (2020). Large spatial variations in the distributions of and factors affecting forest water retention capacity in China. Ecol. Indic. 113:106152. doi: 10.1016/j.ecolind.2020.106152
Wu, S., Zheng, D., Yin, Y., Lin, E., and Xu, Y. (2010). Northward-shift of temperature zones in China’s eco-geographical study under future climate scenario. J. Geogr. Sci. 20, 643–651. doi: 10.1007/s11442-010-0801-x
Xu, W., Xiao, Y., Zhang, J., Yang, W., Zhang, L., Hull, V., et al. (2017). Strengthening protected areas for biodiversity and ecosystem services in China. Proc. Natl. Acad. Sci. 114, 1601–1606. doi: 10.1073/pnas.1620503114
Xu, H., Zhao, C., and Wang, X. (2020). Elevational differences in the net primary productivity response to climate constraints in a dryland mountain ecosystem of northwestern China. Land Degrad. Dev. 31, 2087–2103. doi: 10.1002/ldr.3587
Yan, M., Xue, M., Zhang, L., Tian, X., Chen, B., and Dong, Y. (2021). A Decade’s change in vegetation productivity and its response to climate change over Northeast China. Plan. Theory 10:821. doi: 10.3390/plants10050821
Zhang, L., Sun, P., Huettmann, F., and Liu, S. (2022). Where should China practice forestry in a warming world? Glob. Change Biol. Bioenergy. 28, 2461–2475. doi: 10.1111/gcb.16065
Zhang, X., Xing, Y., Wang, Q., Yan, G., Wang, M., Liu, G., et al. (2020). Effects of long-term nitrogen addition and decreased precipitation on the fine root morphology and anatomy of the main tree species in a temperate forest. For. Ecol. Manag. 455:117664. doi: 10.1016/j.foreco.2019.117664
Zhao, M., and Running, S. W. (2010). Drought-induced reduction in global terrestrial net primary production from 2000 through 2009. Science 329, 940–943. doi: 10.1126/science.1192666
Zhao, D., Wu, S., and Yin, Y. (2013). Responses of terrestrial ecosystems’ net primary productivity to future regional climate change in China. PLoS One 8:e60849. doi: 10.1371/journal.pone.0060849
Keywords: temperate forest, forest ecosystem services, trade-offs and synergies, precipitation gradients, northern China, climate change
Citation: Liang M, Han T, Ma J, Li R, Yang Y, Qiu X, Sun H and Zheng H (2023) Response of temperate forest ecosystem services to rainfall: A case study in the forest nature reserves of northern China. Front. Ecol. Evol. 11:1132396. doi: 10.3389/fevo.2023.1132396
Edited by:
Guoqi Wen, Laval University, CanadaReviewed by:
Zi-jian Xie, Chinese Research Academy of Environmental Sciences, ChinaSerge Rambal, UMR5175 Centre d'Ecologie Fonctionnelle et Evolutive (CEFE), France
Copyright © 2023 Liang, Han, Ma, Li, Yang, Qiu, Sun and Zheng. This is an open-access article distributed under the terms of the Creative Commons Attribution License (CC BY). The use, distribution or reproduction in other forums is permitted, provided the original author(s) and the copyright owner(s) are credited and that the original publication in this journal is cited, in accordance with accepted academic practice. No use, distribution or reproduction is permitted which does not comply with these terms.
*Correspondence: Ruonan Li, rnli@rcees.ac.cn