- 1Department for Continuing Education, University of Oxford, Oxford, United Kingdom
- 2Department of Biology, University of Oxford, Oxford, United Kingdom
Subterranean habitats can be considered harsh conditions with lack of light, low nutrients levels and constant high humidity. To thrive under such conditions, cave-adapted species have evolved a range of novel morphological, physiological and behavioural adaptations. These adaptations might have significant biomimetic potential. Biomimetics or biologically inspired design is a relatively new interdisciplinary field that aims to harness the processes and mechanisms in nature that have been optimised over millions of years’ evolution to improve our own technology. There are two main approaches to biologically inspired design—the problem-driven approach starting with an engineering problem and searching through biological equivalents and the solution-driven approach, which starts with a biological example or solution followed by the identification of a suitable engineering application. While the former approach is the most popular and is favoured by engineers, the latter remains the most successful and is typically driven by fundamental biological research. However, few biomimetic solutions or concepts have so far been described from subterranean habitats despite the rich potential. In this review paper, I first outline the theory behind biologically inspired design before I review the few biomimetic related studies of cave adapted organisms mainly based on the exceptional lateral line systems in blind cave fish. However, the main body of the review focuses on identifying and discussing subterranean adaptations with a particular strong potential including biomimetics sensors, adhesion in high humidity and wet conditions and biomaterials
Introduction
The dark subterranean environment has long been recognised as a natural laboratory for studies on evolutionary and ecological adaptations (Mammola, 2019). Caves and other subterranean habitats are characterised by constant darkness, constant temperatures and high humidity often resulting in standing pools, dripping water and water film on the cave walls. The lack of light means that no primary production takes place in the majority of caves with only a few sulphide enriched and oxygen deprived caves hosting significant amounts of chemoautotrophs (Culver and Pipan, 2019). This again generally results in nutrient limited conditions where organic material only enters the subterranean system through wind blowing material into the cave, underground streams and percolated water bringing in material from the outside, surface animals accidentally entering the cave, or occasional cave dwellers, such as bats and crickets, leaving and entering the cave on a regular basis (Reichle et al., 1965).
The environmental and ecological constraints in subterranean habitats, especially the constant darkness and lack of nutrients mentioned above, combined with stable temperatures and, usually, very high humidity give rise to a very specialised fauna (Mammola, 2019). Traditionally, although this is not universally agreed on (see Trajano and de Carvalho, 2017), cave inhabiting organisms have been classified into three groups (Culver and Pipan, 2019). Trogloxenes are animals that are occasional or accidental visitors to the cave, but do not rely on subterranean habitats to complete their life cycle and include animals such as moths, flies and small mammals. Trogloxenes are most often found in the entrance zone, the area near the cave mouth, which is characterised by some light and climatic variables fluctuating with the outside climate (Howarth, 1983). They do not show any adaptations to subterranean habitats and will therefore not be discussed further in this paper. Troglophiles are animals that spend at least part of their life inside subterranean habitats and include bats, crickets and spiders. They usually reside in the twilight zone, which is characterised by very reduced light and limited daily and seasonal fluctuations in climatic variables compared to the outside (Mammola and Isaia, 2018). Troglophiles usually do not show any morphological adaptations, but they can show physiological and behavioural adaptations (Howarth, 1983). The troglophile cave spider Meta menardi, for example, builds highly modified orb webs lacking a frame structure (Simonsen and Hesselberg, 2021), while another troglophile spider Metellina merianae, which also occurs outside of caves, do not show any modifications to their webs (Hesselberg and Simonsen, 2019). Finally, troglobites (or troglobionts) are animals that spend their entire life inside caves and includes most of the well-known cave animals such as the olm (Proteus anguinus), the Mexican cave fish (Astyanax mexicanus) and many arthropod species (Howarth and Moldovan, 2018). Almost all troglobites inhabit the dark (or deep) zone of caves, which is characterised by a complete lack of light and a stable temperature and a high humidity. Many, but far from all, troglobites show examples of convergent evolution with morphological adaptations (in which case they are often referred to as troglomorphs, although it is worth noting here that for most species, function and fitness benefits of these putative adaptations have not been explicitly studied) including eye loss, depigmentation and elongation of limbs (Howarth, 1983; Pipan and Culver, 2012). Physiological adaptations include enhanced non-visual sensory systems, erratic or a complete lack of circadian rhythms and lower metabolic rates (Hervant, 2012), while behavioural adaptations, which are the least studied of all, include a broadening of diet, reduced social or aggregation behaviours and a shift of reproductive behaviour to producing fewer, but larger offspring (Howarth and Moldovan, 2018; McGaugh et al., 2020).
The insular nature of caves has also resulted in a high proportion of endemic species, sometimes with species known from just one specific cave (Mammola, 2019). Examples include 23% of amphipods and 31% of beetles in known from single subterranean sites in the Western Balkans (Bregović et al., 2019), the guano moth (Kangerosithyris kotumarensis) found only in Kotumsar Cave in India (Biswas, 2010), the centipede (Cryptops speleorex) found only in Movile Cave in Romania (Vahtera et al., 2020) and the West Virginia Spring Salamander (Gyrinophilus subterraneus) known only from General Davis Cave in the US (Grant et al., 2022). However, it is worth noting that caves may be less insular than we think, as many individual caves are linked together through a vast network of small fissures in the karst (Culver and Pipan, 2019; Mammola et al., 2021). Consequently, while most of our knowledge on the hypogean fauna is based on studies from caves, many subterranean organisms may actually be adapted to live in the narrow fissures rather than the open caves, which we are more familiar with (Mammola, 2019).
These convergent adaptations and the large number of endemic species make the subterranean habitat a potential goldmine for developing novel biomimetic technologies. Biomimetics (also referred to as biologically inspired design) is a relatively new interdisciplinary field that aims to extract inspiration for technological breakthroughs from biologically processes and organism traits, refined over millions of years of trial and error through natural selection (Vincent et al., 2006; Lenau et al., 2018). While the examples of commercially successful biomimetic products are still far and few between, the focus on harsh ecosystems is already proving successful. Deserts organisms, for example have generated a number of biomimetics ideas, including algorithms for effective visual vector based navigation in the desert ant Cataglyphis (Dupeyroux et al., 2019), anti-erosion properties from the exoskeleton of the sand scorpion Leiurus quinquestriatus (Han et al., 2010) and passive water collection technology inspired by desert organisms (Gurere and Bhushan, 2020) such as the tenebrionid beetle Stenocara gracilipes from the Namib desert (Parker and Lawrence, 2001) and the Argentinian cactus Gymnocalycium baldianum (Liu et al., 2015). However, despite the unique subterranean fauna, very few biomimetic related studies have focused on cave-adapted animals.
The aim of this paper is to review the very few existing studies on cave biomimetics and to suggest adaptations in cave-dwelling species with significant biomimetic potential. I focus on putative adaptations in three areas relating to the environmental characteristics of the habitat; morphological adaptations for adhesion and locomotion on smooth cave rocks, the evolution of alternative sensory systems in response to the lack of vision in the constant darkness, and finally on adaptive changes to biomaterials, especially spider silk, in response to the high humidity and unique environmental and ecological conditions. My hope is to encourage cave biologists to look again at the species they encounter in light of potentially novel functions, so that they, together with engineers, can explore their biomimetics potential.
What is biomimetics and biologically inspired design?
Biomimetics can be defined as the study of biological functions, processes or morphological traits with the aim of applying them for generating inspiration to develop novel technological solutions. The term biomimetics was coined in 1969 by Otto Schmitt, but it first became recognised as an independent discipline (typically nested within mechanical or materials engineering) in the 1990s (Vincent et al., 2006). However, long before the term was coined, humans looked to nature for inspiration with classical examples ranging from early human tools mimicking the function of sharp canines in mammal predators to the unsuccessful attempts by Leonardi da Vinci to develop flying machines based on the wing design of birds and bats (Hesselberg, 2007). The first successful example of biomimetics dates from before the term was coined and is that of Velcro, which was discovered in 1955 by a Swiss engineer when walking his dog. He noticed that burdock seed attached strongly to the fur of his dog, and decided to investigate the reason for that, which led him to discover the many tiny hooks on the burdock seed. He designed Velcro in a similar way with one side consisting of tiny hooks and the other side of furlike hairs (Hesselberg, 2007). Since the 1990s, the field of biomimetics has attracted a lot of attention, and both the number of papers and the number of patents grew exponentially in the early parts of this millennium (Bonser, 2006; Hesselberg, 2007; Lenau et al., 2018). The proportion of papers published in the general area of biomimetics continues to rise to the present day (Figure 1).
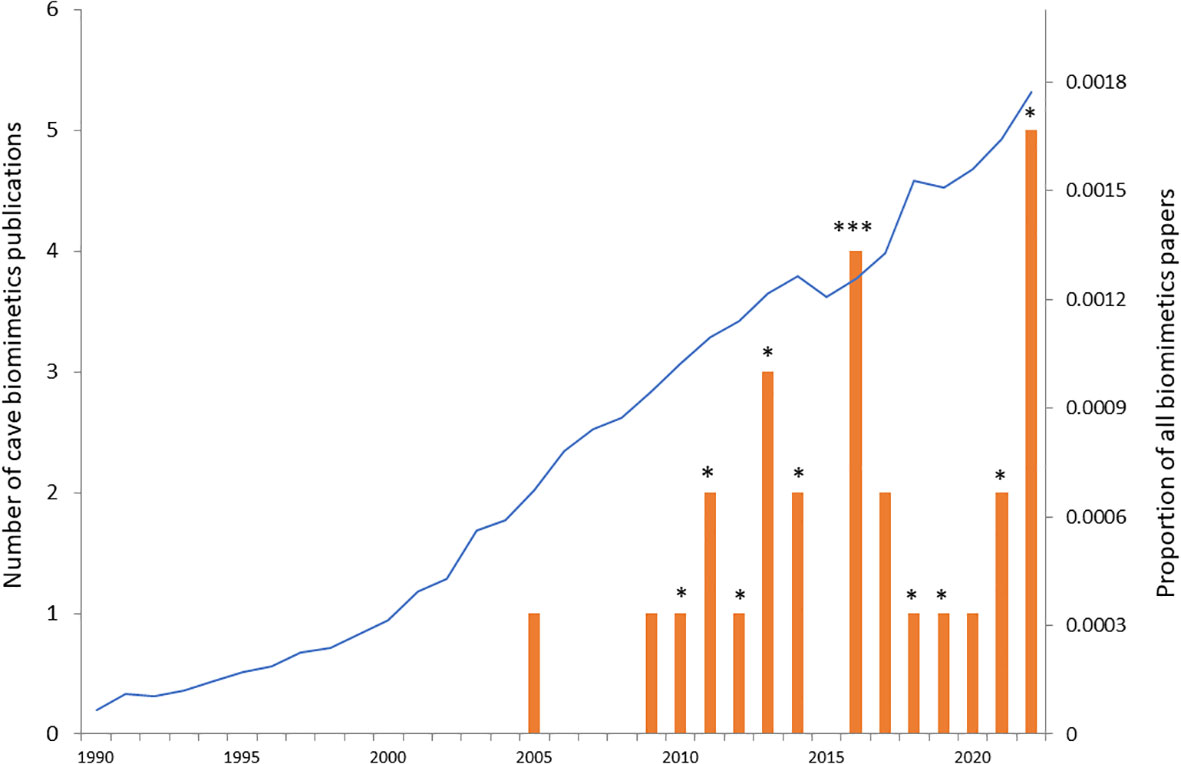
Figure 1 Cave biomimetics papers in relation to all biomimetics papers over the past 33 years (from 1990 to 2022). Red bars show the number of published papers in the Web of Science catalogue on cave biomimetics on the 7th of August 2023 {search phrase: TS=[cave AND (biomim* OR “biologically inspir*” OR bioinsp*)]}. Each star (*) indicates one of the 12 papers that were of direct relevance to cave biomimetics (the search phrase returned a number of papers not directly related to cave biomimetics). The proportion of all published papers that include biomimetics is shown by the blue curve. For each year the number of published papers on biomimetics [search phrase: TS = (biomim* OR “biologically inspir*” OR bioinsp*)] was divided by the total number of published papers in that year.
Recently, there has been a large push to try to develop a more systematic approach to biomimetics often referred to as the biologically inspired design process, which consists of a number of steps ranging from the initial idea to the finalised commercial product (Lenau et al., 2018; Speck and Speck, 2019). One of the earliest systematic methods is the Biomimicry Innovation Method, developed by the Biomimicry Guild in the early 2000s, which identified 4 steps: Identify function, biologize (translate into biology) the question, find nature’s best practice and generate product ideas (Gebeshuber et al., 2009). However, the most well-known and most adopted method is the Georgia Tech model of biologically inspired design, which consists of the following 6 steps: problem definition, reframing the problem, biological solution search, defining the biological solution, principle extraction and principle application (Helms et al., 2009). In general, we can divide the biologically inspired methodology into two; the problem-driven and the solution-driven approach (Figure 2). The problem-driven approach (also known as top down or technology-pull) starts with a technical problem, which is followed by a search for potential biological solutions, before principles are extracted and applied (Speck and Harder, 2006). The solution-driven approach, on the other hand, is usually initiated by biologists, who have discovered a novel function in a biological organism, which is followed by a search for potential technological problems or gaps which the biologically function could solve, before principles are extracted and applied (Speck and Harder, 2006). The later steps in the solution-driven approach require heavy involvement of designers and engineers.
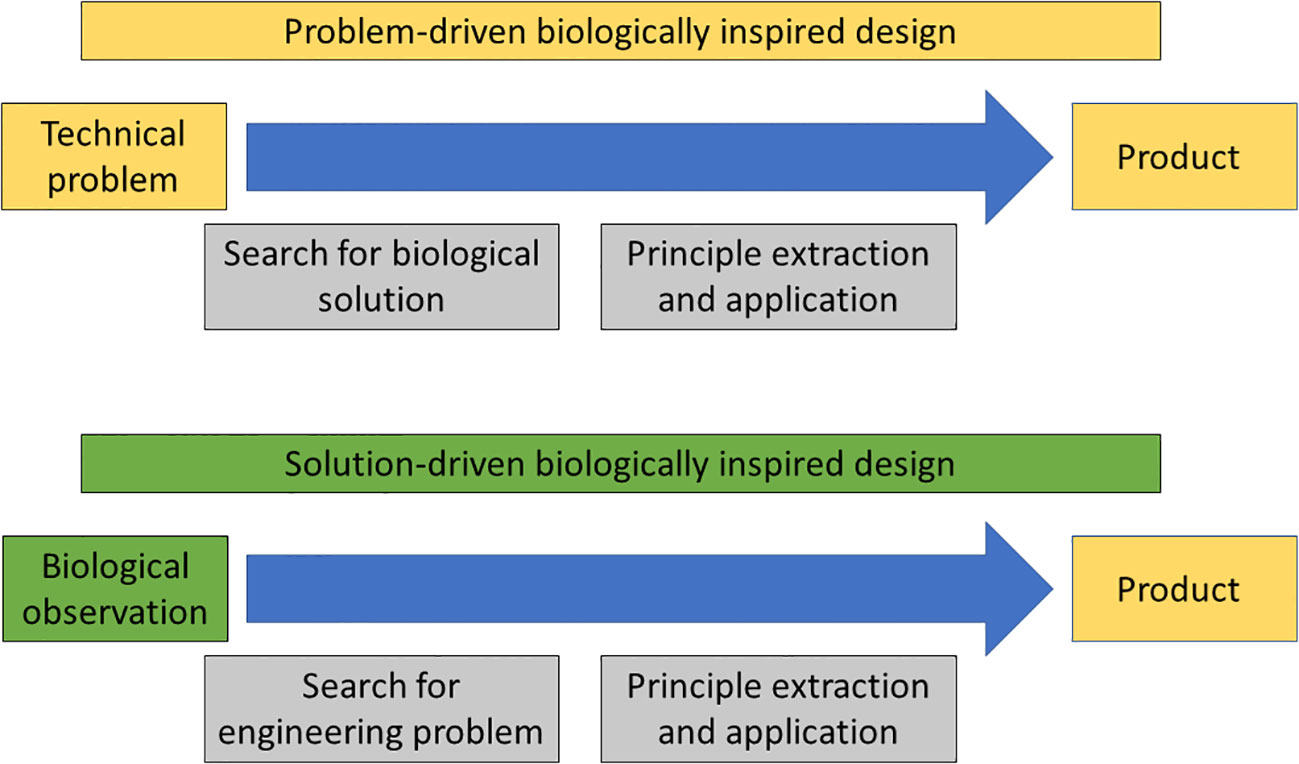
Figure 2 Problem-driven biologically inspired design (above) starts with an engineering problem followed by a search for potential biological solutions before principle extraction and application. Solution-driven biologically inspired design starts with a biological observation (or solution) followed by a search for an engineering (or technical) problem before principle extraction and application. Inspiration for this figure comes from Lenau et al. (2018) and Speck and Speck (2019).
Much effort has gone into developing the problem-driven approach in recent years, especially trying to overcome the obstacles of translating biological concepts into a language that designers and engineers can understand (Vincent et al., 2006). A number of different tools have been developed including BioTRIZ, a biomimetics ontology and AskNature (Vincent et al., 2006; Deldin and Schuknecht, 2014; Vincent and Cavallucci, 2018). The latter is the most immediately accessible tool, allowing anybody to search for potential biological solutions to technological problems on the internet (asknature.org), although it still struggles, in some cases, with limited explanations and questionable references. Still, the most effective route for the problem-driven approach to lead to a successful outcome is to include biologists into the project team (Snell-Rood, 2016; Graeff et al., 2019). The problem-driven approach has to date delivered few success stories with the most promising ones probably being in the area of medical technology including using biomaterials, such as silk, as scaffolds for tissue regeneration and in surgery (Holland et al., 2019; Song et al., 2020), and developing painless microneedles based on the mouth parts and biting behaviour of mosquitoes (Lenau et al., 2017; Ma et al., 2022). Nonetheless, the most successful methodology remains the solution-driven one to which the above mentioned Velcro belongs. Further promising research with large potential commercial applications include the self-cleaning Lotusan paint based on the Lotus effect in water lily leaves (Neinhuis and Barthlott, 1997; Antony et al., 2016) and strong adhesives based on the intricate morphology of gecko feet, which allows them to cling on to, and move on, smooth vertical surfaces (Autumn et al., 2000; Geim et al., 2003). However, very few out of the estimated 8 million species of living organisms on Earth have been the focus of biomimetics studies suggesting that there remains a large scope for further success stories arising from for the solution-driven approach.
It is here that cave biologists can potentially make a large contribution as this field of biology has until now largely been overlooked in biologically inspired design. Despite the sustained high output of biomimetics papers during the past decades, only 27 hits came up on Web of Science relating to cave biomimetics (Figure 1). A closer scrutiny of the titles and abstracts of these 27 hits, revealed that only 12 of them were specifically about biomimetic inspiration gained from cave adapted organisms (* in Figure 1; Table 1). The remaining hits mainly relate to using bioinspired robotics to explore cave systems. Nine out of the 12 papers focus on developing biomimetic hydrodynamic flow sensors based on the lateral line system of various species of cave fishes (predominantly, but not exclusively the blind Mexican cavefish Astyanax mexicanus). Thus there is a large untapped potential for using the solution-driven biologically inspired design approach to develop novel biomimetic technologies based on a broader range of cave-dwelling species. I discuss a few promising cave adapted animals where the solution-driven approach might be particularly fruitful in the section “Promising cave adaptation examples” below.
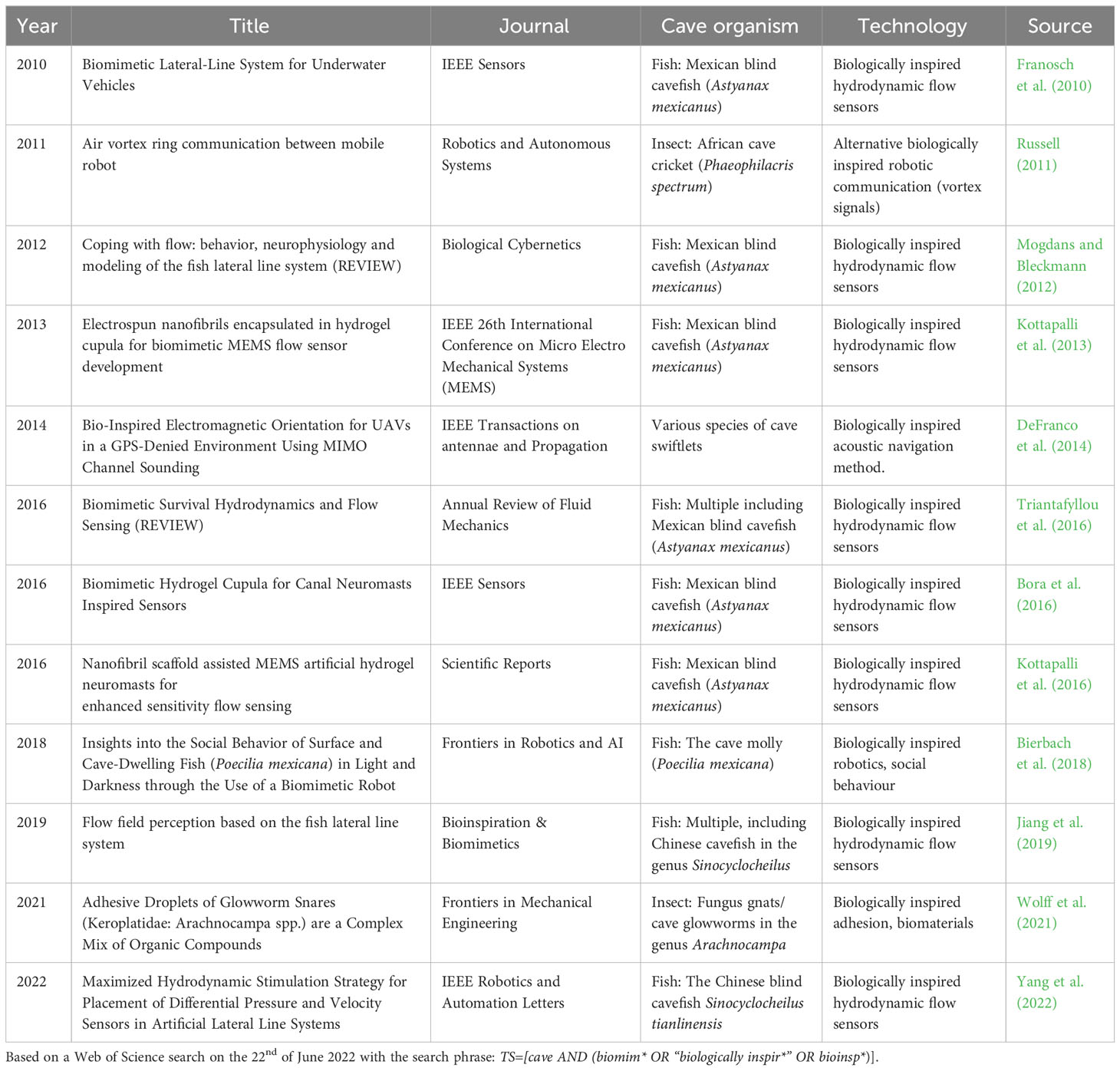
Table 1 Biomimetics cave papers directly relating to gaining inspiration from cave-adapted organisms.
Cave biomimetics
We saw in the section above, how few biomimetics related studies have so far been carried out on the subterranean fauna (Figure 1; Table 1). In this section, we cover three of the most interesting and promising examples; two relating to the field of biomimetics sensors and one relating to the field of novel biomaterials.
The best studied example is that of the enhanced lateral line system in blind cave fish compared to epigean fish (Figure 3). The lateral line system (also referred to as the lateral line organ) consists of a series of flow sensors, called neuromasts that are found either on the surface (superficial neuromasts) or embedded in fluid filled canals just below the skin (canal neuromasts) (Figure 3). The two types of neuromasts have complementary functions with the superficial neuromasts being directly exposed to the flow and able to directly respond to different flow regimes, while the canal neuromasts, which are exposed to the water via pores are able to detect differences in pressure and inertial forces (McHenry and van Netten, 2007). The neuromasts are made up of sensory hairs enclosed in a gelatinous dome-like cupula and innervated by afferent nerves (Bleckmann and Zelick, 2009). While all fish possess a lateral line system, it is particularly well developed in blind cave fish (Yoshizawa et al., 2010) suggesting that there will be particular technological benefits by focusing on cave fish over their surface counterparts. The Mexican blind cave fish (Astyanax mexicanus) is, for example, able to detect minute changes down to 0.4 cm/s in oscillatory fluid flow (Montgomery et al., 1997). It is therefore unsurprising that cave fish in general, and the Mexican cave fish in particular, have been used as a model for developing biomimetic flow sensors in a relatively large number of studies (Table 1). Examples range from the development of individual flow sensors to whole artificial lateral line systems to be used on swimming robots (Liu et al., 2016). In the former category, we find a range of inexpensive and low-powered micro-electromechanical systems (MEMS) sensors inspired by the superficial neuromasts of the Mexican blind cave fish (Triantafyllou et al., 2016). Interestingly, it has been shown that embedding these MEMS sensors in a hydrogel structure inspired by the cupula increases the sensitivity of the flow sensors 3.5–5 times compared to a naked sensor (Kottapalli et al., 2016). A similar functional MEMS based sensor with a hydrogel based capula inspired by canal neuromasts was developed with material and mechanical properties similar to the canal neuromasts in A. fasciatus (Bora et al., 2016). Complete artificial lateral line systems consisting of a series of flow sensors have also been developed and implemented on robotic fish (Liu et al., 2016). However, one of the main challenges with implementing effective artificial lateral line systems is finding the optimal location for placing them on the robotic fish (Yang et al., 2022). To address this issue, Yang et al. (2022) developed a hydrodynamic model based on the geometry of the Chinese blind cavefish (Sinocyclocheilus tianlinensis), which has a characteristic dorsal hump and horn. They compared results from their hydrodynamic model with flow sensors on the robot and found that the model was able to correctly predict where the superficial and canal neuromasts were placed on the real fish. This suggests that their biomimetic hydrodynamic simulation model can be used to predict optimal placement of flow sensors on robots and other small underwater vehicles (Yang et al., 2022).
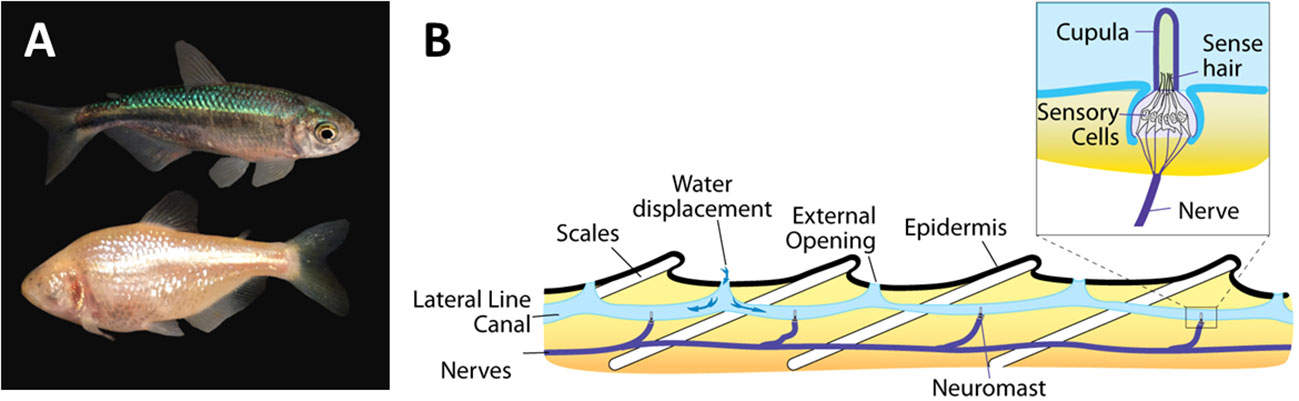
Figure 3 The lateral line system in blind cave fish. (A) A surface (above) and a cave (below) morph of the Mexican blind cave fish Astyanax mexicanus. Photo by Daniel Castranova, National Institute of Health, courtesy of FlickR (in public domain). (B) A schematic overview of the lateral line system showing the canal neuromasts consisting of nerves, sense hairs and the cupula. Created by Thomas Haslwanter and made available courtesy of Wikimedia Commons (CC BY-SA 3.0).
Another interesting, albeit far less studied, example of a potential biomimetics sensor inspired by cave-dwelling species is that of the use of air vortex rings as a form of short distance communication in the African cave cricket, Phaeophilacris spectrum (Heinzel and Dambach, 1987). P. spectrum is found in East Africa and shows an affinity for caves for at least part of their life cycle (a likely troglophile), but it can be easily maintained in the laboratory (Heidelbach and Dambach, 1997). It is not troglomorphic, as it does not have any apparent reduction in pigmentation or eyes, although it does have relatively long antennae (Saussure, 1878). Unlike epigean crickets, P. spectrum males do not rely on stridulation (the sound produced when crickets rub their wings against each other) or other auditory signals to signal to females, possibly due to the lack of control of direction of acoustic signals due to echo in caves (Heinzel and Dambach, 1987). Instead they communicate over short distances of up to 15 cm by rapidly flicking their wings to generate travelling air vortices (Heinzel and Dambach, 1987). It looks likely that two different messages can be communicated; a threat display of a single vortex to nearby conspecifics and a courting display to nearby wingless females consisting of a rapid sequence of vortices (Heinzel and Dambach, 1987; Russell, 2011). This novel method of communication was implemented on a mobile robotic system carrying 8 air disturbance sensors that were found to be able to successfully respond to directional cues from a stationary vortex generator (Russell, 2011). Robotic communication by air vortices thus could be an additional form of inter-robot communication in close quarters, where traditional forms of communication such as wireless, acoustic or optical signals may not work or be undesired including underground, in high turbulence and turbidity water, or if part of secret military operations (Russell, 2011).
The final example relates to biologically inspired material properties in the form of adhesion under the high humidity conditions seen in caves based on the silk threads of cave glowworms. There are 9 described species of cave glowworms in the genus Arachnocampa, all of which are endemic to New Zealand and Australia (Baker, 2010). The short lived adult fungus gnats are capable of flight, but typically stay close to where they emerged, while the bioluminescent larvae can live up to 12 months with most populations inhabiting caves or cave-like habitats, although a few can also be found in other dark habitats such as sheltered tropical forests where they, interestingly, are more pigmented than the populations found in caves (Meyer-Rowchow, 2007; Baker, 2010). The larvae spin hanging silk snares that contain mucous adhesive droplets that are regularly arranged liked beads on a string (Meyer-Rowchow, 2007). The bioluminescence produced by the larvae lures flying insects such as moths, gnats and mosquitoes into the snares (von Byern et al., 2019). Both larval survival and the adhesiveness of the snares require the high humidity found in caves and rain forests (von Byern et al., 2019). The ability of the snares to function effectively at high humidity, was recently investigated by Wolff et al. (2021), who found that polar substances and hygroscopic compounds in the glue droplets, especially urea and a range of amino acids, were responsible for their function, although interestingly, variation was found both between species and between forest and cave populations (Wolff et al., 2021). Thus, glowworm glue droplets may serve as inspiration for developing moisture resistant and moisture activated adhesives that are required to function in environments with a constant high humidity, which would, for example, be useful as tissue adhesives in surgery (Wolff et al., 2021).
Promising cave adaptation examples
We have seen in the previous sections that while biomimetics studies on cave-adapted species should in theory provide a wealth of inspiration for novel technology, very few studies have in practice been carried out. In this section, I provide a flavour of the vast potential by highlighting a number of unusual adaptations in cave species, that I believe have significant biomimetics potential. Although again we should note that function, fitness benefit and heritability of the putative adaptation have not, in most cases, been tested explicitly. The examples I provide here roughly belong to one of three categories: adhesion and locomotion to smooth and wet surfaces, non-visual biomimetic sensors and wet resistant biomaterials and structures.
Adhesion and locomotion
Caves are characterised by often having very high humidity often with dripping water and water films covering the cave walls (Mammola, 2019). This makes it potentially challenging for cave-dwelling species to adhere to, and effectively move on, the smooth rock surface. One group of cave-adapted species that has been particularly well studied in this regard are the cave springtails, which, due to their size, in effect need to attach to, and move on, vertical water surfaces on the cave wall (Christiansen, 1961; Christiansen, 1965). They achieve this primarily by having claws with a longer, and to some extent more serrated, tooth (the unguis), which allows for a much better grip by anchoring the claw into the water film (Christiansen, 1961; Christiansen, 1965). Springtails are very common in caves with more than 300 described troglobiotic species from European caves alone (Fiera et al., 2021). Undoubtedly, more species awaits discovery, some of which may show additional variation and novel adaptations of their claws (Fiera et al., 2021). A case in point is the recent description of the troglomorphic springtail Troglaphorura gladiator from a cave in the Caucasus (Vargovitsh, 2019). This species has extremely long and thin claws, which are either completely submerged in or entirely rising above the water. This alternating sequence makes it in effect walk on water, while simultaneously ensuring a firm grip on the surface preventing the springtails from being blown away by air currents (Vargovitsh, 2019). These adaptations in springtail claws are reminiscent of the intricate setal morphology present in marine polychaete worms required to crawl on muddy and slippery substrate (Hesselberg and Vincent, 2006). The number, morphology and placement of these setae were studied from a biomimetic point of view and implemented on a simple crawling robot (La Spina et al., 2005) with the aim of using them for inspiration for developing novel self-moving colonoscopes (Hesselberg, 2007). The cave springtails may provide a similar inspiration for medical robots or devices required to move on watery and slippery surfaces.
Another potential and larger-sized group of organisms to study in this respect are the millipedes. Detritivorous millipedes are often found in caves, primarily in Europe, but also in relatively large numbers in tropical countries (Liu et al., 2017). Very little is known about the locomotion of cave millipedes, but they do show a number of convergent evolutionary traits including larger size and longer limb length and frequently elongated primary tarsal claws, although surprisingly not elongated antennae (Liu et al., 2017). Three recently described cave-adapted species from continental Portugal and Madeira show an interesting unique adaptation of their tarsal pads with very short accessory claws thought to be an adaptation to climb on the hard, smooth cave walls (Reboleira and Enghoff, 2014; Reboleira and Enghoff, 2018). We need more biomechanical studies on locomotion and foot morphology in troglomorph millipedes, since they might be useful for the ongoing development of biorobots inspired by the morphology and locomotion of aboveground millipedes (Lu et al., 2018; Garcia et al., 2021). A final intriguing example comes from the cave angel fish, Cryptotora thamicola, which is endemic to caves in Northern Thailand (Figure 4A), where it is found living in fast flowing streams (Brooke et al., 2016). It has a unique ability to climb hard, smooth rock surfaces in waterfalls using a tetrapod-like walking gait enabled by a robust pelvic girdle and other morphological adaptations otherwise only seen in terrestrial vertebrates (Brooke et al., 2016). We do not yet know how this ability might be facilitated by specialised adaptations of their fins to allow better grip with the smooth surface, but the combination of tetrapod-like crawling with normal fish swimming may in itself have potential for the development of amphibious biorobots (Ijspeert, 2020).
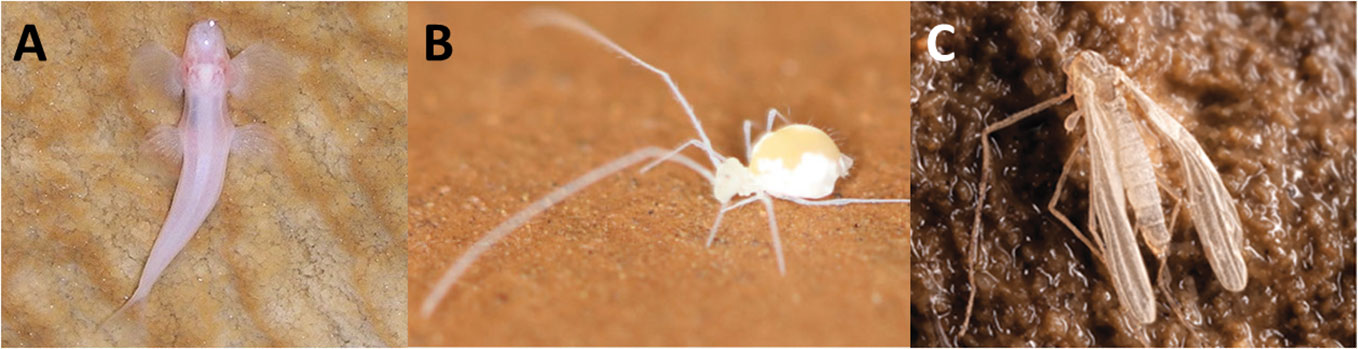
Figure 4 Some cave adapted species with biomimetic potential. (A) The cave angel fish (Cryptotora thamicola) is adapted to climb slippery rocks in fast flowing streams. Photo: Chulabush Khatancharoen via FlickR (CC BY 2.5). (B) The springtail (Troglobentosminthurus luridus) has extremely long antennae, which likely function as effective direct mechanosensors. Photo modified from de Souza et al., 2022 (CC BY 4.0). (C) The cave adapted midge (Troglocladius hajdi) is capable of flying in total darkness, likely using the its long forelegs as feelers. Photo taken by J. Bedek and modified from Anderson et al., 2016 (CC BY 4.0).
Biomimetic sensors
In the previous section, we discussed the relatively large effort that has gone into developing biomimetic flow sensors based on the lateral line system of blind cave fish. Interestingly, one cave-adapted fish, the catfish Astroblepus pholeter, has relatively few neuromasts and instead seems to rely on innervated dorsal skin teeth (denticles) to detect hydrodynamic forces (Haspel et al., 2012). The catfish is endemic to Jumandi Cave in Ecuador, where it lives in a very fast flowing and turbulent stream suggesting that these denticles could provide inspiration for a different type of biomimetic flow sensor. In addition to fish, many other cave adapted species are likely to have evolved heightened non-visual sensory systems to compensate for the lack of light suggesting a rich source for inspiration in developing novel mechanosensory systems. Many invertebrates, especially arthropods, have convergently evolved elongated limbs and sensory structures, presumably in order to increase the range of their contact mechano-sensory system (Howarth, 1983; Christiansen, 2012), although more function based studies are needed to confirm that these are in fact adaptations. The recently described cave springtail, Troglobentosminthurus luridus, from the Água Clara cave system in Brazil (Figure 4B), shows, among other troglomorphic traits, extremely elongated antennae (de Souza et al., 2022). The antennae are more than twice the length of the body and consist of 44 subsegments. While the behaviour of this new species is virtually unknown, gut content analyses suggest that they, unusually for a springtail, may act as predators (de Souza et al., 2022). Future studies on this, and other troglobites with very long antennae, should provide interesting data on how they move efficiently with such long antennae, and how they manage to sweep them effectively to detect obstacles, prey and predators, which might have significant biomimetic potential for developing subterranean search and rescue robots (Rouček et al., 2020).
Most cave-dwelling insects, except for the species found in the entrance and twilight zones, have lost or reduced wings and hence do not fly (Wagner and Liebherr, 1992), which make sense given that insect flight is heavily dependent on vision for optomotor flight control (Fry et al., 2009; Lecoeur et al., 2019). Nonetheless, a few troglomorph species of flying insects have been discovered including the pale midge, Troglocladius hajdi, with has reduced eyes, long legs and normal wings (Andersen et al., 2016) (Figure 4C). It is known only from one chamber 800–1000 meters down the vertical Lukina jama–Trojama cave system in Croatia. It has so far never been observed in flight, but its morphology suggests that it could engage in slow flight with its long legs stretched ahead of it acting as feelers (Andersen et al., 2016), which could provide alternative inspiration for non-visual sensory structures in micro-air vehicles required to operate in dark and spatially complex environments.
Biomaterials and structures
In the previous section, we saw how the silk used in glowworm capture threads may have biomimetic potential due to their ability to operate effectively at a very high relative humidity. However, glowworms are not the only animals that rely on silk in subterranean habitats. Spiders are relatively common in caves with more than 1000 troglobiotic species worldwide (Mammola and Isaia, 2017). No studies on the mechanical properties of the silk of spiders inhabiting the deep zone have yet been conducted, but one study on the Tasmanian cave spider, Hickmania troglodytes, which build large horizontal sheet webs within the twilight zone, found that the structural silk of these webs are as tough as that in vertical orb webs and that they show a unique ontogenetic shift with larger spiders constructing webs using tougher silk (Piorkowski et al., 2017). More than 80% of spiders found in caves build webs (Mammola and Isaia, 2017; Mammola et al., 2022). This combined with the unique environment, especially in relation to the occasionally strong and turbulent airflows near the mouth of many caves (Tuttle and Stevenson, 1977; Gomell and Pflitsch, 2022), suggests that it might be worth paying closer attention to these silk structures. Meta cave spiders, for example, construct their orb webs in the twilight zones of many temperate caves (Hesselberg et al., 2019). Their webs differ from epigean orb webs in that they are smaller, less dense, lack frame threads and have many more attachment points to the cave walls (Simonsen and Hesselberg, 2021). While these modifications are primarily thought to be adaptations to capture non-flying prey on the cave walls, they could also reduce web damage caused by wind, as reduced size and less dense spiral turns are generally seen in orb webs built in windy conditions (Vollrath et al., 1997; Wu et al., 2013). Thus, more biomechanical studies on the silk and geometry of these webs might yield insights that could be beneficial in the development of strong lightweight biomimetic structures (Leach et al., 2014; Vollrath and Krink, 2020). This potential was highlighted in a study of the tensile strength of the silk stalks of the eggsac of Meta menardi, which are often built close to the cave mouth (Lepore et al., 2012). The study found that these eggsacs have the stretchiest stalks ever measured allowing them to regularly extend up to 300% before breaking (Lepore et al., 2012).
Conclusion
In this review, I have shown that the unusual environmental and ecological factors that drives evolution in subterranean habitats have resulted in highly unique adaptations with significant biomimetic potential, especially within the areas of biomimetics sensors, biomaterials, adhesion and biologically inspired robotic locomotion. Our discussion has mainly focussed on cave-adapted species found in terrestrial or aquatic habitats in the deep zones of large caves, where convergent evolution has often resulted in depigmentation and eye loss enabling the development of effective non-visual sensory systems, and in limb elongation increasing the effective mechanosensory range (Culver and Pipan, 2019; Mammola, 2019). Undoubtedly, many more discoveries on adaptations with biomimetics potential awaits discovery in these zones, but it is worth noting that troglobiotic species are also found in other subterranean habitats such as lava tubes, mines and under stones and boulders in scree habitats (Růžička et al., 2013; Culver and Pipan, 2019). It has also been argued that many cave-adapted species actually spend most of their time in the small fissures and cracks adjacent to the large chambers in which we observe them (Howarth, 1983; Mammola et al., 2021). Adaptations to live in such small spaces may offer inspiration for search-and-rescue robotics, similar to how the amazing compressibility of aboveground cockroaches have been used to develop robust and versatile biorobots (Jayaram and Full, 2016). Additionally, species found in other types of caves may offer significant biomimetic potential. Remipedes, for example, are eyeless crustaceans found in marine caves, where they swim in an unusual upside-down way, while continuously beating their trunk limbs in a metachronal manner (Koenemann et al., 2007). Their flexible and elongated trunks give them excellent steerability, but they are also capable of sudden jumps through the water while beating their limbs simultaneously suggesting overall high biomimetic potential for the development of versatile autonomous underwater robots (Kwak and Bae, 2018).
The present study highlights that more research effort needs to go into discovering and describing new cave-adapted species, which allows us to identify suitable candidates species for further biomimetic studies. However, equally importantly is more in depth functional studies on physiological and behavioural processes of already discovered species given that the most promising biomimetic potential relate to the biomechanical function of morphological structures in terms of locomotion, adhesion and mechanosensory sensitivity. Traditionally, such studies have been difficult to carry out in caves due the large number of impediments facing cave researchers including difficult working conditions, low abundance of target species and difficulties in studying cave-dwelling species in ex-situ laboratories (Mammola et al., 2021). However, recent technological advances in computer simulation and remote sensing/bioinspired robotics as well as more appreciation of the value of having laboratories in caves should allow us to overcome many of these impediments (Mammola et al., 2021). Studies on adaptations have also recently been identifies as one of the most important areas of research for subterranean biologists (Mammola et al., 2020), so it is likely that we will see a huge growth of biomimetics studies on cave-dwelling species in the near future.
It is also my hope that this paper will provide an additional case for the importance of conserving subterranean habitats. While caves are to some extent buffered from many of the main threats facing aboveground ecosystems including climate change, pollution, habitat fragmentation and invasive species (Brook et al., 2008), troglobites, evolved to survive in stable conditions nonetheless face serious threats from even minor disruptions to environmental conditions caused by climate change or human visitors and tourisms, as well as large scale destruction of habitats caused by mining or excessive groundwater extraction (Mammola et al., 2019). The present study gives an overview of some of the many unique adaptations found in cave-adapted species, but also suggests that there is a vast reservoir of species and adaptations that we have not yet discovered. We must therefore preserve as much of the cave biodiversity as possible since it is very likely that future technologies including biorobotics, internal surgery and novel adhesive structures depend on the inspiration we can get from studying subterranean species.
Finally, the present study also highlights the importance of convergent evolution in identifying biomimetics potential. Arguably, convergent adaptations, i.e. the independent evolution of similar traits in response to similar environmental drivers, suggest a more optimal solution to a given problem than non-convergent traits that may be more evolutionarily constrained (Graeff et al., 2020). However, to make full biomimetics use of convergence, we need many more studies on the adaptive value of traits (i.e. function, fitness benefits and heritability) from not only subterranean environments, but also from other harsh ecosystems such as deserts, polar regions and hydrothermal vents.
Author contributions
TH developed the idea and wrote up the manuscript.
Acknowledgments
The author would like to thank the Oxford University Department for Continuing Education for financial support to attend the 25th International Conference for Subterranean Biology in Cluj, Romania, where the ideas behind this paper were first presented as well as conference attendees for stimulating discussions on the topic. The author also thanks three reviewers for their very useful suggestions to a previous version of this manuscript.
Conflict of interest
The author declares that the research was conducted in the absence of any commercial or financial relationships that could be construed as a potential conflict of interest.
Publisher’s note
All claims expressed in this article are solely those of the authors and do not necessarily represent those of their affiliated organizations, or those of the publisher, the editors and the reviewers. Any product that may be evaluated in this article, or claim that may be made by its manufacturer, is not guaranteed or endorsed by the publisher.
References
Andersen T., Baranov V., Hagenlund L. K., Ivković M., Kvifte G. M., Pavlek M. (2016). Blind flight? A new troglobiotic orthoclad (Diptera, chironomidae) from the Lukina Jama–Trojama cave in Croatia. PloS One 11, e0152884. doi: 10.1371/journal.pone.0152884
Antony F., Griesshammer R., Speck T., Speck O. (2016). The cleaner, the greener? Product sustainability assessment of the biomimetic facade paint Lotusan(R) in comparison to the conventional facade paint Jumbosil(R). Beilstein J. Nanotechnol. 7, 2100–2115. doi: 10.3762/bjnano.7.200
Autumn K., Liang Y. A., Hsieh S. T., Zesch W., Chan W. P., Kenny T. W., et al. (2000). Adhesive force of a single gecko foot-hair. Nature 405, 681–685. doi: 10.1038/35015073
Baker C. H. (2010). A new subgenus and five new species of Australian glow-worms (Diptera : Keroplatidae: Arachnocampa). Memoirs Queensland Museum—Nat. 55, 11–43.
Bierbach D., Lukas J., Bergmann A., Elsner K., Hohne L., Weber C., et al. (2018). Insights into the social behavior of surface and cave-dwelling fish (Poecilia mexicana) in light and darkness through the use of a biomimetic robot. Front. Robot. AI. 5, 3. doi: 10.3389/frobt.2018.00003
Biswas J. (2010). Kotumsar Cave biodiversity: a review of cavernicoles and their troglobiotic traits. Biodivers. Conserv. 19, 275–289. doi: 10.1007/s10531-009-9710-7
Bleckmann H., Zelick R. (2009). Lateral line system of fish. Integr. Zool. 4, 13–25. doi: 10.1111/j.1749-4877.2008.00131.x
Bonser R. H. (2006). Patented biologically-inspired technological innovations: a twenty year view. J. Bionic Eng. 3, 39–41. doi: 10.1016/S1672-6529(06)60005-X
Bora M., Kottapalli A. G. P., Miao J. M., Asadnia M., Triantafyllou M. S. (2016). “Biomimetic hydrogel cupula for canal neuromasts inspired sensors,” in Proceedings of the 2016 IEEE SENSORS, Orlando, FL, USA, 9 January 2017. 1–3. Available at: https://ieeexplore.ieee.org/document/7808842.
Bregović P., Fišer C., Zagmajster M. (2019). Contribution of rare and common species to subterranean species richness patterns. Ecol. Evol. 9, 11606–11618. doi: 10.1002/ece3.5604
Brook B. W., Sodhi N. S., Bradshaw C. J. A. (2008). Synergies among extinction drivers under global change. Trends Ecol. Evol. 23, 453–460. doi: 10.1016/j.tree.2008.03.011
Brooke E. F., Suvarnaraksha A., Markiewicz J., Soares D. (2016). Tetrapod-like pelvic girdle in a walking cavefish. Sci. Rep. 6, 23711. doi: 10.1038/srep23711
Christiansen K. (1961). Convergence and parallelism in cave Entomobryinae. Evolution 15, 288–301. doi: 10.2307/2406229
Christiansen K. (1965). Behaviour and form in the evolution of cave collembola. Evolution 19, 529–537. doi: 10.2307/2406249
Christiansen K. (2012). “Morphological adaptations,” in Encyclopedia of Caves. Eds. White W. B., Culver D. C. (Amsterdam: Elsevier), 517–528.
Culver D. C., Pipan T. (2019). Biology of Caves and Other Subterranean Habitat. 2nd edition (Oxford, UK: Oxford University Press).
DeFranco P., Mackie J. D., Morin M., Warnick K. F. (2014). Bio-inspired electromagnetic orientation for UAVs in a GPS-denied environment using MIMO channel sounding. IEEE Trans. Antennas Propagation 62, 5250–5259. doi: 10.1109/TAP.2014.2341300
Deldin J. M., Schuknecht M. (2014). “The AskNature Database: Enabling Solutions in Biomimetic Design,” in Biologically Inspired Design. Eds. Goel A., McAdams D., Stone R. (London: Springer).
de Souza P. G. C., Medeiros G., Ferreira R. L., Souza-Silva M. (2022). A highly troglomorphic new genus of sminthuridae (Collembola, Symphypleona) from the Brazilian semiarid region. Insects 13, 650. doi: 10.3390/insects13070650
Dupeyroux J., Serres J. R., Viollet S. (2019). AntBot: A six-legged walking robot able to home like desert ants in outdoor environments. Sci. Robot. 4 (27). doi: 10.1126/scirobotics.aau030
Fiera C., Arbea J. I., Vargovitsh R. S., Barjadze S. (2021). A synthesis on troglobitic springtails in Europe. J. Zool. System. Evolution. Res. 59, 1874–1890. doi: 10.1111/jzs.12560
Franosch J. M. P., Sosnowski S., Chami N. K., Kuhnlenz K., Hirche S., van Hemmen J. L. (2010). Biomimetic lateral-line system for underwater vehicles. Sensors 2010 IEEE., 2212–2217. doi: 10.1109/ICSENS.2010.5690626
Fry S., Rohrseitz N., Straw A. D., Dickonson M. H. (2009). Visual control of flight speed in Drosophila melanogaster. J. Exp. Biol. 212, 1120–1130. doi: 10.1242/jeb.020768
Garcia A., Krummel G., Priya S. (2021). Fundamental understanding of millipede morphology and locomotion dynamics. Bioinspiration Biomimetics 16, 026003. doi: 10.1088/1748-3190/abbdcc
Gebeshuber I. G., Majlis B. Y., StachelBerger H. (2009). Tribology in bioloogy: Biomimetic studies across dimensions and across fields. Int. J. Mech. Mater. Eng. 4, 321–327. Available at: https://www2.iap.tuwien.ac.at/~gebeshuber/P15_IJMME.pdf.
Geim A. K., Dubonos S. V., Grigorieva I. V., Novoselov K. S., Zhukov A. A., Shapoval S. Y. (2003). Microfabricated adhesive mimicking gecko foot-hair. Nat. Mater. 2, 461–463. doi: 10.1038/nmat917
Gomell A. K., Pflitsch A. (2022). Airflow dynamics in Wind Cave and Jewel Cave: How do barometric caves breathe? Int. J. Speleol. 51, 163–179. doi: 10.5038/1827-806X.51.3.2437
Graeff E., Maranzana N., Aoussat A. (2019). Biomimetics, where are the biologists? J. Eng. Design 30, 289–310. doi: 10.1080/09544828.2019.1642462
Graeff E., Maranzana N., Aoussat A. (2020). Biological practices and fields, missing pieces of the biomimetics’ Methodological puzzle. Biomimetics 5, 62. doi: 10.3390/biomimetics5040062
Grant E. H. C., Mulder K. P., Brand A. B., Chambers D. B., Wynn A. H., Capshow G., et al. (2022). Speciation with gene flow in a narrow endemic West Virginia cave salamander (Gyrinophilus subterraneus). Conserv. Genet. 23, 727–744. doi: 10.1007/s10592-022-01445-7
Gurere D., Bhushan B. (2020). Passive water harvesting by desert plants and animals: lessons from nature. Philos. Trans. R. Soc. A. 378 (2167). doi: 10.1098/rsta.2019.0444
Han Z., Zhang J., Ge C., Lu Y., Jiang J., Liu Q., et al. (2010). Anti-erosion function in animals and its biomimetic application. J. Bionic Eng. 7, S50–S55. doi: 10.1016/S1672-6529(09)60217-1
Haspel G., Schwartz A., Streets A., Camacho D. E., Soares D. (2012). By the teeth of their skin, cavefish find their way. Curr. Biol. 22, R629–R630. doi: 10.1016/j.cub.2012.06.035
Heidelbach J., Dambach M. (1997). Wing-flick signals in the courtship of the African cave cricket, Phaeophilacris spectrum. Ethology 103, 827–843. doi: 10.1111/j.1439-0310.1997.tb00124.x
Heinzel H.-G., Dambach M. (1987). Travelling air vortex rings as potential communication signals in a cricket. J. Comp. Physiol. 160, 79–88. doi: 10.1007/BF00613443
Helms M., Vattam S. S., Goel A. K. (2009). Biologically inspired design: process and products. Design Stud. 30, 606–622. doi: 10.1016/j.destud.2009.04.003
Hervant F. (2012). “Starvation in subterranean species versus surface-dwelling species: crustaceans, fish and salamanders,” in Comparative physiology of fasting, starvation and food limitation. Ed. McCue M. D. (Berlin—Heidelberg: Springer), 91–102.
Hesselberg T. (2007). Biomimetics and the case of the remarkable ragworms. Naturwissenschaften 94, 613–621. doi: 10.1007/s00114-006-0212-0
Hesselberg T., Simonsen D. (2019). A comparison of morphology and web geometry between two hypogean and epigean species of Metellina orb spiders (family Tetragnathidae). Subterranean Biol. 32, 1–13. doi: 10.3897/subtbiol.32.36222
Hesselberg T., Simonsen D., Juan C. (2019). Do cave orb spiders show unique behavioural adaptations to subterranean life? A Rev. Evidence Behav. 156, 969–996. doi: 10.1163/1568539X-00003564
Hesselberg T., Vincent J. F. V. (2006). A comparative study of functional morphology of parapodia and setae in nereids (Polychaeta: Nereididae). Anim. Biol. 56, 103–120. doi: 10.1163/157075606775904704
Holland C., Numata K., Rnjak-Kovacina J., Seib F. P. (2019). The biomedical use of silk: past, present, future. Adv. Healthcare Mater. 8, 1800465. doi: 10.1002/adhm.201800465
Howarth F. G. (1983). Ecology of cave arthropods. Annu. Rev. Entomol. 28, 365–389. doi: 10.1146/annurev.en.28.010183.002053
Howarth F. G., Moldovan O. T. (2018). “The ecological classification of cave animals and their adaptations,” in Cave Ecology, vol. 235. Eds. Moldovan O. T., Kováč L., Halse S. (Cham: Springer), 41–67. Available at: https://link.springer.com/chapter/10.1007/978-3-319-98852-8_4#citeas.
Ijspeert A. J. (2020). Amphibious and sprawling locomotion: from biology to robotics and back. Annu. Rev. Of Control Robot. And Autonomous Syst. 3, 173–193. doi: 10.1146/annurev-control-091919-095731
Jayaram K., Full R. J. (2016). Cockroaches traverse crevices, crawl rapidly in confined spaces, and inspire a soft, legged robot. Proc. Natl. Acad. Sci. U.S.A. 113, E950–E957. doi: 10.1073/pnas.1514591113
Jiang Y. G., Ma Z. Q., Zhang D. Y. (2019). Flow field perception based on the fish lateral line system. Bioinspiration Biomimetics. 14, 041001. doi: 10.1088/1748-3190/ab1a8d
Koenemann S., Schram F. R., Iliffe T. M., Hinderstein L. M., Bloechl A. (2007). Behavior of Remipedia in the laboratory, with supporting field observations. J. Crustacean Biol. 27, 534–542. doi: 10.1651/S-2809A.1
Kottapalli A. G. P., Asadnia M., Miao J. M., Triantafyllou M. S. (2013). “Electrospun nanofibrils encapsulated in hydrogel cupula for biomimetic MEMS flow senseor development,” in Proceedings IEEE Micro Electro Mechanical Systems. 26th IEEE International Conference on Micro Electro Mechanical Systems (MEMS), Taipei, TAIWAN. 25–28.
Kottapalli A. G. P., Bora M., Asadnia M., Miao J., Venkatraman S. S., Triantafyllou M. (2016). Nanofibril scaffold assisted MEMS artificial hydrogel neuromasts for enhanced sensitivity flow sensing. Sci. Rep. 6, 19336. doi: 10.1038/srep19336
Kwak B., Bae J. (2018). Locomotion of arthropods in aquatic environment and their applications in robotics. Bioinspiration Biomimetics 13, 041002. doi: 10.1088/1748-3190/aab460
La Spina G., Hesselberg T., Williams J., Vincent J. F. V. (2005). A biomimetic approach to robot locomotion in unstructured and slippery environments. J. Bionics Eng. 2, 1–14. doi: 10.1007/BF03399476
Leach D., Wang L., Reusser D., Lida F. (2014). Automatic building of web-like structure based on thermoplastic adhesive. Bioinspiration Biomimetics 9, 036014. doi: 10.1088/1748-3182/9/3/036014
Lecoeur J., Dacke M., Floreano D., Baird E. (2019). The role of optic flow pooling in insect flight control in cluttered environments. Sci. Rep. 9, 7707. doi: 10.1038/s41598-019-44187-2
Lenau T., Hesselberg T., Drakidis A., Silva P., Gomes S. (2017). Mosquito inspired medical needles, (2017). Proc. SPIE Bioinspiration Biomimetics Bioreplication 10162, 1016208. doi: 10.1117/12.2261399
Lenau T., Metze A. L., Hesselberg T. (2018). Paradigms for biologically inspired design. Proc. SPIR Bioinspiration Biomimetics Bioreplication 10593, 1059302. doi: 10.1117/12.2296560
Lepore E., Marchioro A., Isaia M., Buehler M. J., Pugno N. M. (2012). Evidence of the most stretchable egg sac silk stalk, of the European spider of the year Meta menardi. PloS One 7, e30500. doi: 10.1371/journal.pone.0030500
Liu W., Golavatch S., Wesener T., Tian M. (2017). Convergent evolution of unique morphological adaptations to a subterranean environment in cave millipedes (Diplopoda). PloS One 12, e0170717. doi: 10.1371/journal.pone.0170717
Liu G., Wang A., Wang X., Liu P. (2016). A review of artificial lateral line in sensor fabrication and bionic applications for robot fish. Appl. Bionics Biomech. 2016, 4732703. doi: 10.1155/2016/4732703
Liu C., Xue Y., Chen Y., Zheng Y. (2015). Effective directional self-gathering of drops on spine of cactus with splayed capillary arrays. Sci. Rep. 5. 17757. doi: 10.1038/srep17757
Lu H., Zhang M., Yang Y., Huang Q., Fukada T., Wang Z., et al. (2018). A bioinspired multilegged soft millirobot that functions in both dry and wet conditions. Nat. Commun. 9, 3944. doi: 10.1038/s41467-018-06491-9
Ma Y., Xiao X., Ren H., Meng M. Q.-H. (2022). A review of bio-inspired needle for percutaneous interventions. Biomimetic Intell. Robot. 2, 4. doi: 10.1016/j.birob.2022.100064
Mammola S. (2019). Finding answers in the dark: caves as models in ecology fifty years after Poulson and White. Ecography 42, 1331–1351. doi: 10.1111/ecog.03905
Mammola S., Amorim I. R., Bichuette M. E., Borges P. A. V., Cheeptham N., Cooper S. J. B., et al. (2020). Fundamental research questions in subterranean biology. Biol. Rev. 95, 1855–1872. doi: 10.1111/brv.12642
Mammola S., Cardoso P., Culver D. C., Deharveng L., Ferreira R. L., Fišer C., et al. (2019). Scientists’ Warning on the conservation of subterranean ecosystems. BioScience 69, 641–650. doi: 10.1093/biosci/biz064
Mammola S., Isaia M. (2017). Spiders in caves. Proceed. R. Soc. London. Ser. B. 284, 20170193. doi: 10.1098/rspb.2017.0193
Mammola S., Isaia M. (2018). Day-night and seasonal variations of a subterranean invertebrate community in the twilight zone. Subterranean Biol. 27, 31–51. doi: 10.3897/subtbiol.27.28909
Mammola S., Lunghi E., Bilandžija H., Cardoso P., Grimm V., Schmidt S. I., et al. (2021). Collecting eco-evolutionary data in the dark: Impediments to subterranean research and how to overcome them. Ecol. Evol. 11, 5911–5926. doi: 10.1002/ece3.7556
Mammola S., Pavlek M., Huber B. A., Isaia M., Ballarin F., Tolve M., et al. (2022). A trait database and updated checklist for European subterranean spiders. Sci. Data 9, 236. doi: 10.1038/s41597-022-01316-3
McGaugh S. E., Weaver S., Gilbertson E. N., Garrett B., Rudeen M. L., Grieb S., et al. (2020). Evidence for rapid phenotypic and behavioural shifts in a recently established cavefish population. Biol. J. Linn. Soc. 129, 143–161. doi: 10.1093/biolinnean/blz162
McHenry M. J., van Netten S. M. (2007). The flexural stiffness of superficial neuromasts in the zebra fish (Dania rerio) lateral line. J. Exp. Biol. 210, 4244–4253. doi: 10.1242/jeb.009290
Meyer-Rowchow V. B. (2007). Glowworms: a review of Arachnocampa spp. and kin. Arachnocampa 22, 251–265. doi: 10.1002/bio.955
Mogdans J., Bleckmann H. (2012). Coping with flow: behavior, neurophysiology and modeling of the fish lateral line system. Biol. Cybernetics 106, 627–642. doi: 10.1007/s00422-012-0525-3
Montgomery J. C., Baker C. F., Carton A. G. (1997). The lateral line can mediate rheotaxis in fish. Nature 389, 960–963. doi: 10.1038/40135
Neinhuis C., Barthlott W. (1997). Characterization and distribution of water-repellent, self-cleaning plant surfaces. Ann. Bot. 79, 667–677. doi: 10.1006/anbo.1997.0400
Parker A., Lawrence C. R. (2001). Water capture by a desert beetle. Nature 414, 33–34. doi: 10.1038/35102108
Piorkowski D., Blamires S. J., Doran N. E., Liao C.-P., Wu C.-L., Tso I.-M. (2017). Ontogenetic shift toward stronger tougher silk of a web-building, cave-dwelling spider. J. Zool. 304, 81–89. doi: 10.1111/jzo.12507
Pipan T., Culver D. C. (2012). Convergence and divergence in the subterranean realm: a reassessment. Biol. J. Linn. Soc. 107, 1–14. doi: 10.1111/j.1095-8312.2012.01964.x
Reboleira A. S. P. S., Enghoff H. (2014). Insular species swarm goes underground: two new troglobiont Cylindroiulus millipedes from Madeira (Diplopoda: Julidae). Zootaxa 3785, 481–489. doi: 10.11646/zootaxa.3785.3.9
Reboleira A. S. P. S., Enghoff H. (2018). First continental troglobiont Cylindroiulus millipede (Diplopoda, Julida, Julidae). Zookeys 795, 93–103. doi: 10.3897/zookeys.795.27619
Reichle D. E., Palmer J. D., Park O. (1965). Persistent rhythmic locomotor activity in the cave cricket, Hadenoecus subterraneus, and its ecological significance. Am. Midland Nat. 74, 57–66. doi: 10.2307/2423119
Rouček T., Pecka M., Čížek P., Petříček T., Bayer J., Šalanský V., et al. (2020). “DARPA subterranean challenge: multi-robotic exploration of underground environments,” in Modelling and Simulation for Autonomous Systems. MESAS 2019. Lecture Notes in Computer Science, vol. 11995 . Eds. Mazal J., Fagiolini A. (Cham: Springer).
Russell R. A. (2011). Air vortex ring communication between mobile robots. Robot. Autonomous Syst. 59, 65–73. doi: 10.1016/j.robot.2010.11.002
Růžička V., Ŝmilauer P., Mlejnek R. (2013). Colonization of subterranean habitats by spiders in Central Europe. Int. J. Speleol. 42, 133–140. doi: 10.5038/1827-806X.42.2.5
Saussure H. D. (1878). Mélanges orthoptérologiques. Memoires la Société physique d’histoire naturelle Genève 25, 446.
Simonsen D., Hesselberg T. (2021). Unique behavioural modifications in the web structure of the cave orb spider Meta menardi (Araneae, Tetragnathidae). Sci. Rep. 11, 1–10. doi: 10.1038/s41598-020-79868-w
Snell-Rood E. (2016). Interdisciplinarity: Bring biologists into biomimetics. Nature 529, 277–278. doi: 10.1038/529277a
Song Y., Wang H., Yue F., Lv Q., Cai B., Dong N., et al. (2020). Silk-based biomaterials for cardiac tissue engineering. Adv. Healthcare Mater. 9, 2000735. doi: 10.1002/adhm.202000735
Speck T., Harder D. D. (2006). “Bionics or Biomimetics: Taking a leaf out of nature’s book,” in BIOKON BionikKompetenz-Netz–Creative transfer of biological principles into engineering, vol. 4–6 . Ed. Harder D. (Berlin, Germany: BIOKON e.V. Bionics Competence Network).
Speck O., Speck T. (2019). An overview of bioinspired and biomimetic self-repairing materials. Biomimetics 4, 26. doi: 10.3390/biomimetics4010026
Trajano E., de Carvalho M. R. (2017). Towards a biologically meaningful classification of subterranean organisms: a critical analysis of the Schiner-Racovitza system from a historical perspective, difficulties of its application and implications for conservation. Subterranean Biol. 22, 1–26. doi: 10.3897/subtbiol.22.9759
Triantafyllou M. S., Weymouth G. D., Miao J. (2016). Biomimetic survival hydrodynamics and flow sensing. Annu. Rev. Fluid Mechanics 48, 1–24. doi: 10.1146/annurev-fluid-122414-034329
Tuttle M. D., Stevenson D. E. (1977). “Variation in the cave environemnt and its biological implications,” in Proceedings of the National Cave Management Symposium. Eds. Zuber R., Chester J., Gilbert S., Rhodes D. (New Mexicon: Adope Press), 108–121.
Vahtera V., Stoev P., Akkari N. (2020). Five million years in the darkness: A new troglomorphic species of cryptops leach 1814 (Chilopoda, scolopendromorpha) from movile cave, Romania. ZooKeys 1004, 1–26. doi: 10.3897/zookeys.1004.58537
Vargovitsh R. S. (2019). Cave water walker: An extremely troglomorphic Troglaphorura gladiator gen. et sp. nov. (Collembola, Onychiuridae) from Snezhnaya Cave in the Caucasus. Zootaxa 4619, 267–284. doi: 10.11646/zootaxa.4619.2.4
Vincent J. F. V., Bogatyreva O. A., Bogatyreva N. R., Bowyer A., Pahl A.-K. (2006). Biomimetics—its practice and theory. J. R. Soc. Interface 3, 471–482. doi: 10.1098/rsif.2006.0127
Vincent J., Cavallucci D. (2018). “Development of an ontology of biomimetics based on Altshuller’s Matrix,” in Automated invention for smart industries. TFC 2018. Eds. Cavallucci D., De Guio R., Koziołek S.. IFIP Adv. Inf. Commun. Technol. (Springer, Cham), vol. 541. doi: 10.1007/978-3-030-02456-7_2
Vollrath F., Downes M., Krackow S. (1997). Design variability in web geometry of an orb-weaving spider. Physiol. Behav. 62, 735–743. doi: 10.1016/S0031-9384(97)00186-8
Vollrath F., Krink T. (2020). Spider webs inspiring soft robotics. J. R. Soc. Interface 17, 20200569. doi: 10.1098/rsif.2020.0569
von Byern J., Chandler P., Merritt D., Adlassnig W., Stringer I., Meyer-Rochow V. B., et al. (2019). Biomechanical properties of fishing lines of the glowworm Arachnocampa luminosa (Diptera; Keroplatidae). Sci. Rep. 9, 3082. doi: 10.1038/s41598-019-39098-1
Wagner D. L., Liebherr J. K. (1992). Flightlessness in insects. Trends Ecol. Evol. 7, 216–220. doi: 10.1016/0169-5347(92)90047-F
Wolff J. O., von Byern J., Piorkowski D., Fang J., Wang X., Adler L., et al. (2021). Adhesive droplets of glowworm snares (Keroplatidae: arachnocampa spp.) are a complex mix of organic compounds. Front. Mechanical Eng. 6, 661422. doi: 10.3389/fmech.2021.661422
Wu C.-C., Blamires S. J., Wu C.-L., Tso I.-M. (2013). Wind induces variations in spider web geometry and sticky spiral droplet volume. J. Exp. Biol. 216, 3342–3349. doi: 10.1242/jeb.083618
Yang Z., Gong Z., Jiang Y., Cai Y., Ma Z., Na X., et al. (2022). Maximized hydrodynamic stimulation strategy for placement of differential pressure and velocity sensors in artificial lateral line systems. IEEE Robot. Automation Lett. 7, 2170–2177. doi: 10.1109/LRA.2022.3143203
Keywords: cave-dwelling animals, cave biomimetics, biologically inspired design, troglomorphs, cave adaptations
Citation: Hesselberg T (2023) The biomimetic potential of novel adaptations in subterranean animals. Front. Ecol. Evol. 11:1127728. doi: 10.3389/fevo.2023.1127728
Received: 19 December 2022; Accepted: 28 September 2023;
Published: 13 October 2023.
Edited by:
Matthew Niemiller, University of Alabama in Huntsville, United StatesReviewed by:
Cene Fišer, University of Ljubljana, SloveniaIlle C. Gebeshuber, Faculty of Physics, Vienna University of Technology, Austria
Copyright © 2023 Hesselberg. This is an open-access article distributed under the terms of the Creative Commons Attribution License (CC BY). The use, distribution or reproduction in other forums is permitted, provided the original author(s) and the copyright owner(s) are credited and that the original publication in this journal is cited, in accordance with accepted academic practice. No use, distribution or reproduction is permitted which does not comply with these terms.
*Correspondence: Thomas Hesselberg, dGhvbWFzLmhlc3NlbGJlcmdAYmlvbG9neS5veC5hYy51aw==