- 1Department of Biological Sciences, Macquarie University, Sydney, NSW, Australia
- 2Evolutionary Biomechanics, Zoological Institute and Museum, Greifswald, Germany
- 3School of Molecular Science, Arizona State University, Tempe, AZ, United States
- 4Mark Wainwright Analytical Centre, University of New South Wales, Sydney, NSW, Australia
- 5School of Chemistry, University of New South Wales, Sydney, NSW, Australia
- 6School of Biological, Earth and Environmental Science, University of New South Wales, Sydney, NSW, Australia
- 7School of Mechanical and Mechatronic Engineering, University of Technology, Sydney NSW, Australia
Natural glues offer great potential as bio-inspired solutions to problems associated with the performance of synthetic adhesives. Spider viscous glues are elastic pressure sensitive adhesives (PSAs) that physically adhere to surfaces on contact across a range of environmental conditions. Extracting useful components from these secretions remains a challenge that can be met by the comparative analyses of functional analogues. Here we used 1H NMR spectroscopy and mass spectrometry to ascertain the organic salt compositions of the PSAs of four different species of Australian spiders belonging to two lineages that independently acquired aqueous gluey secretions: the St Andrew’s cross (Argiope keyserlingi), the redback (Latrodectus hasselti), the false widow (Steatoda grossa), and the daddy long-legs spider (Pholcus phalangiodes). The PSAs from each of these spiders contained similar organic salts, albeit in variable concentrations. The adhesives of the false widow and daddy long-legs spider had mixtures of only a few components, of which betaine predominated, while the PSAs of the other spiders predominantly contained small organic acids such as GABA/GABA-amide, isethionate, and choline salts. Our results suggest that the PSA composition of spiders is likely to be influenced more by environmental factors than evolutionary history and are guided by common principles. Our findings could be valuable for facilitating the design of more sustainable synthetic glues.
Introduction
Loss of adhesion as temperature or humidity fluctuates is one of many drawbacks of modern synthetic adhesives (Kinloch, 1987). The adhesives used by animals, on the other hand retain their adhesive function across most environmental conditions and are often tailored to perform best at conditions that are challenging for artificial adhesives (Smith and Callow, 2006; Hennebert et al., 2015; Jain et al., 2015; Singla et al., 2018). For this reason, there is immense interest among researchers and industries in investigating the chemical and physical properties of different animal adhesives (Gorb, 2008; von Byern and Grunwald, 2010; Santos et al., 2013). Earmarked applications for bioinspired glues include in situ tissue adhesives, toxin free food packaging adhesives, wet and/or underwater adhesives, and reusable adhesives for walking and climbing robots (Liu and Jiang, 2011; Hanks and Sweigers, 2012). Despite such interest our understanding of the chemical functionality of most natural glues and adhesives is rudimentary at best.
As animal adhesives are usually a diverse and variable mix of components (Wolff et al., 2021), identifying the core functional units leading to the impressive performance represents a major milestone toward a successful biomimetic implementation. One way to achieve this is through the comparative study of analogous systems (Wolff et al., 2017). Most research so far has focused on specific aspects of a few well-known systems, such as gecko toe pads and mussel byssus threads (Gorb, 2008; Liu and Jiang, 2011; Bré et al., 2013; Wolff et al., 2017). It is thus evident that a more thorough examination of the chemistry and functionality of a wider range of different animal glues and adhesives is needed. We accordingly took a bioprospecting approach herein to identify whether common chemistries could have repeatedly evolved among some phylogenetically distinct spider prey capture adhesives.
Natural and synthetic adhesive substances that require some degree of pressure to activate their adhesion without creating durable chemical bonds with the substrate are called pressure sensitive adhesives (PSAs) (Vendamme et al., 2014). Spiders produce a variety of sticky secretions (Sahni et al., 2011; Wolff and Gorb, 2016). These includes: (1) glue-like substances that dry within seconds and form strong and durable bonds between the silks, environmental substrates and anchor lines (Wolff et al., 2015; Wolff and Gorb, 2016; Wirth et al., 2019), (2) PSAs such as the so-called viscid silk of orb webs and cobwebs, that give spider webs their stickiness and permit silk lines to reversibly adhere to prey (Sahni et al., 2010; Townley and Tillinghast, 2013; Jain et al., 2016; Kelly et al., 2019), and (3) dry adhesives based on nanofibers which are utilized for prey capture by some spiders instead of viscid silk (Hawthorn and Opell, 2003; Bott et al., 2017; Piorkowski et al., 2020).
Viscid or viscid-like silk is found among distantly related lineages of spiders, such as orb web spiders (Araneoidea: Araneidae), cobweb spiders (Araneoidea: Theridiidae) and the daddy long-legs spider (Synspermiata: Pholcidae) (Briceño, 1985; Japyassú and Macagnan, 2004; Wolff and Gorb, 2016). These gluey secretions appear as droplets on the sticky capture spirals of orb webs (Figure 1A), or at the base of the capture gumfoot threads of cobwebs (Figure 1B; Blamires, 2022). The aqueous glues are secreted from the so-called aggregate glands possessed by most araneoid spiders. Pholcids on the other hand do not possess aggregate glands, rather a set of modified pyriform glands produce gluey secretions that superficially resembles aggregate glue (Japyassú and Macagnan, 2004; Vasanthavada et al., 2012; Wolff and Gorb, 2016). In all instances the glues are applied onto silk threads whereupon they form droplets that look somewhat like beads-along-a-string (Blamires, 2022). Although they lack the aggregate gland, the gluey secretion of the daddy long-legs spider seem to function similarly to aggregate glues despite being evolutionarily distant (Japyassú and Macagnan, 2004). Hence, it is reasonable to infer that PSAs could have repeatedly evolved among multiple lineages of spider (Vasanthavada et al., 2012).
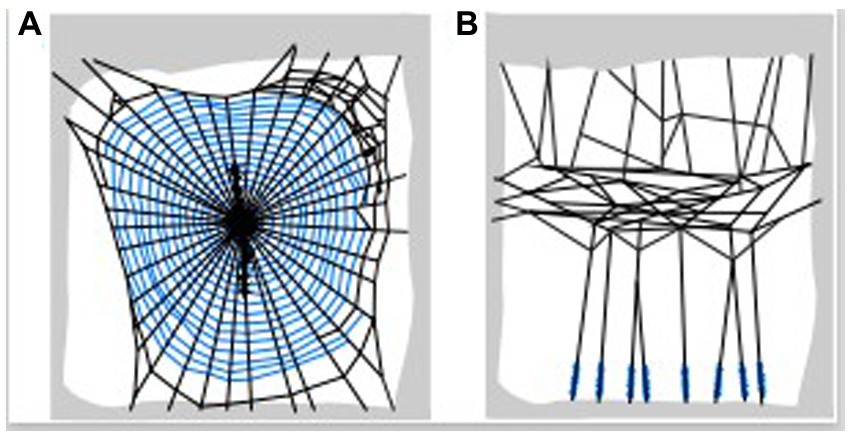
Figure 1. Location of the different gluey secretions in spider webs. Panel (A) shows the sticky capture spirals in spider orb webs, and (B) the gumfoot capture threads of cobwebs.
The adhesive functionality of viscid gluey silks may be associated with specific ecological processes. In contrast to artificial PSAs, the viscid silk of araneoids remains tacky under high humidity because it contains hygroscopic salts which remove water from the substrate surface (Singla et al., 2018). The stickiness of these viscid silks is conferred by the presence of phosphorylated glycoproteins, while the salts are thought to keep these glycoproteins hydrated (Vollrath et al., 1990; Sahni et al., 2010, 2014a,b; Opell et al., 2014; Ayoub et al., 2021). Consequently, the material is kept very soft, permitting the adhesive functionality (Torres et al., 2014). In orb web and cobweb spiders the salts comprise a combination of low molecular weight organic (e.g., GABamide, choline, isethionic acid) and inorganic (e.g., HPO4, and KNO3) compounds (Vollrath et al., 1990; Opell et al., 2018). Nevertheless, very little is known about the salts present in the glues of other spiders.
It remains unclear if the modulation of adhesion by the organic salts represents a common principal in spider PSAs, as previous research has been limited to just a small number of orb web and cobweb spiders. Ascertaining any common chemical properties in spider glues across a broad lineage of spiders would thus be useful for designing biomimetic glues to push the limits of adhesives applications (Patek, 2014; Wolff et al., 2017). To this end, we ascertained the organic salt composition of the viscid gluey silks of four phylogenetically dissimilar spiders found along the East Coast of Australia: (i) an orb web building spider, Argiope keyserlingi Karsch 1878, and two cobweb spiders, (ii) Latrodectus hasselti Thorell 1870 and (iii) Steatoda grossa Koch 1838 (all Superfamily Araneoidae), and (iv) the daddy long-legs spider, Pholcus phalangioides Fuesslin 1775 (Pholcidae). These species represent two distinct evolutionary origins of PSAs in spiders, thus comparisons among them allow us to seek a possible common chemical principal underlying the functionality of different spider PSAs.
Materials and methods
Sample collections
Ten adult female orb web building spiders, Argiope keyserlingi, were collected from urban locations in Eastern Sydney, Australia. The spiders were weighed on an electronic balance (Ohaus Corp., Pine Brook, NY, USA) upon capture to ensure the individuals used in our experiments were of approximately equal mass before taking them to the University of New South Wales where they housed in 30 (length) × 30 (height) × 55 (width) cm perspex enclosures and fed house flies to promote web building (Blamires et al., 2017). We then monitored the enclosures for webs. Once they had built their webs we removed each spider using forceps and wound heir webs onto 200 μl plastic pipette tips to be subjected to analysis using 1H Nuclear Magnetic Resonance (NMR) and Mass Spectroscopy (MS).
Approximately 20 Latrodectus hasselti and Steatoda grossa were collected from the grounds of the University of New South Wales, Sydney, Australia. These were placed within either 24.5 × 18 × 13 cm wooden frames within 27.5 × 18 × 16 cm Perspex enclosures (L. hasselti) or 19 × 16.5 × 13 cm wooden frames within 20.5 × 14.5 × 13 cm Perspex enclosures (S. grossa) at the University of New South Wales. The spiders were left unfed, to avoid contamination of the glues with insect secretions, in the enclosures for 2 weeks. The enclosures were thereupon checked for capture threads (so-called gumfooted threads), which were individually wound onto sterile 200 μl plastic pipette tips by carefully making contact with the glue and causing the line to break just above the ground. The upper end of the line was then cut with a hot soldering iron. We prodded the spider with forceps so that it moved into a purposely built retreat to ensured it was not interacting with any of the lines being collected. We continued to collect threads until each pipette tip (n = 3 per species) contained approximately 200 threads from between five and ten individual webs.
Daddy long-legs spiders (Pholcus phalangioides) were collected in and around buildings at Macquarie University, Sydney, Australia. Spiders were housed at Macquarie University within cardboard frames (20 × 10 × 15 cm) with the front and hind wall being open and covered with plastic wrap to prevent the spiders from escaping and to allow visual inspection of the webs. Spiders were left unfed in the boxes for 2 weeks, upon which the plastic wrapping was removed, and the spider carefully taken out of the container without touching the threads. The sticky threads were then collected on 200 μl plastic pipette tips as described above for cobweb spiders. In total ~ 1,500 threads were collected from 40 daddy long-legs spiders, which were stored in sterile plastic jars before being transported to the University of New South Wales for NMR and MS analyses. The glue covered pipette tips were fixed with plasticine to the jar lid to prevent contact to the jar walls during storage and transportation of the specimens.
Sample preparations
All of the spider glue samples were washed off the sampling tips with a 150 mM potassium phosphate buffer, pH 6.95, containing an internal reference (deuterated trimethylsilyl propanoate, TMSP), a pH indicator (difluorotrimethylsilanylphosphonic acid, DFTMP) and 99.96% D2O (Cambridge Isotope Laboratories). Single samples were washed with multiple aliquots of buffer adding up to a total volume of 180 μl. Combined samples were prepared from a single aliquot and added sequentially to 3 mm NMR tubes (Norell).
Nuclear magnetic resonance spectroscopy
Proton (1H) NMR spectroscopy was performed using a Bruker Avance III HD 600 MHz spectrometer (600.13 MHz, 1H; 150.9 MHz 13C) fitted with a 5 mm cryoprobe. Samples were stored in a refrigerated Sample Jet autosampler on the magnet. NMR spectra were acquired using the program TOPSPIN 3.6.0 (Bruker, Melbourne, Australia). 1H solvent suppression was performed using 1D NOESY pre-saturation (noesy1dpr) at the HOD solvent residual chemical shift. 1H-13C HSQC spectra were acquired using an optimized pulse program in the Bruker library (hsqcedetgpsisp2.4) (Palmer et al., 1991; Kay et al., 1992; Willker et al., 1993; Schleucher et al., 1994; Zwahlen et al., 1997). A sweep width (time domain) of 12 ppm (2 k data points) in the 1H and 240 ppm (512 data points) in the 13C dimension was used over 16 scans. 1H-13C HMBC spectra were acquired using the Bruker pulse program hmbcgplpndqf (Cicero et al., 2001). A sweep width (time domain) of 12 ppm (2 k) in the 1H and 195 ppm (512 data points) in the 13C dimension was used with a collection of 16 scans. Fourier transformation, phasing, solvent filtering, chemical shift referencing, baseline correction and reference line shape convolution were performed using program Bayesil (Ravanbakhsh et al., 2015). We then compared the relative peak positions of our deconvoluted spectra to a published reference spectra (Vollrath et al., 1990) to identify the individual organic and inorganic hygroscopic salts, and any other small and large molecular weight compounds, within each species’ glues. The relative concentrations of each of the compounds identified was calculated upon baseline correction and integration of the peaks using Bayesil’s metabolomics calculator.
Mass spectrometry
We used tandem mass spectrometry (MS/MS) to verify the presence of compounds identified by 1H NMR as follows.
Individual pipette tips containing either gumfoot threads or threads from orb weaver spiders were washed with 300 uL methanol (HPC grade, Merk, USA) into a 1.5 ml Eppendorf tube. A 7 uL aliquot of each sample was taken for analysis on an Orbitrap LTQ XL (Thermo Fisher Scientific, San Jose Ca, USA) ion trap mass spectrometer using a nanospray (nano-electrospray) ionization source to generate ions from the analytes in solution.
The instrument was calibrated with a standard calibration solution (as outlined in the instrument manual) on each of the analyses. All analyses were carried out in positive ion mode using the orbitrap FTMS analyser at a resolution of 100,000. Sample aliquots were injected into a glass needle and inserted onto the nanospray source. Ions generated were measured over the molecular mass range 150 to 2000. Data was acquired in full scan mode over 60 s. The data generated were analyzed using the Qual Browser feature in Xcaliber 2.1 (Thermo Fisher Scientific, San Jose, CA, USA).
Results
The deconvoluted 1H NMR spectra for the four species of spiders are shown in Figures 2A–D. The concentrations of the substances that we identified in the gluey secretions for each species’ are summarised in Table 1, with comparisons to the concentrations found elsewhere for similar species (Vollrath et al., 1990; Jain et al., 2018; Opell et al., 2018). The mass spectrometry spectra for the four species’ gluey secretions are shown in Figures 3A–D.
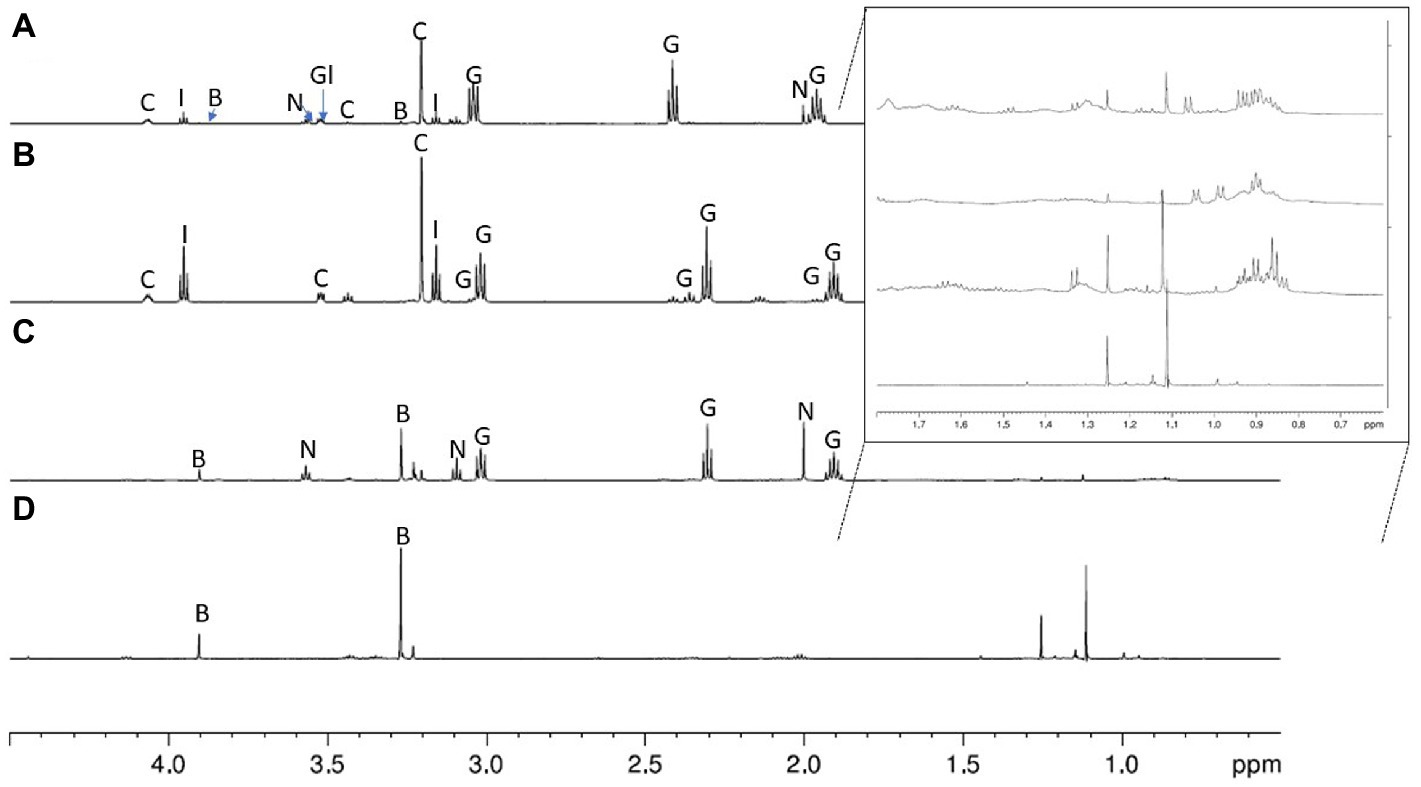
Figure 2. 600 MHz 1H NMR spectra of glues from (from top to bottom): Argiope keyserlingi (A), Latrodectus hasselti (B), Steatoda grossa (C), and Pholcus phalangioides (D) across the spectral range 0 to 5 ppm, with the 0.5 to 2.0 ppm spectral range enhanced as an inset. See the ‘Materials and Methods’ section for details about NMR preparation and data acquisition. Annotations on the spectra are: C, choline; B, betaine; I, isethionic acid; G, GABA/GABAmide; N, N-acetyltaurine; Gl, glycine.
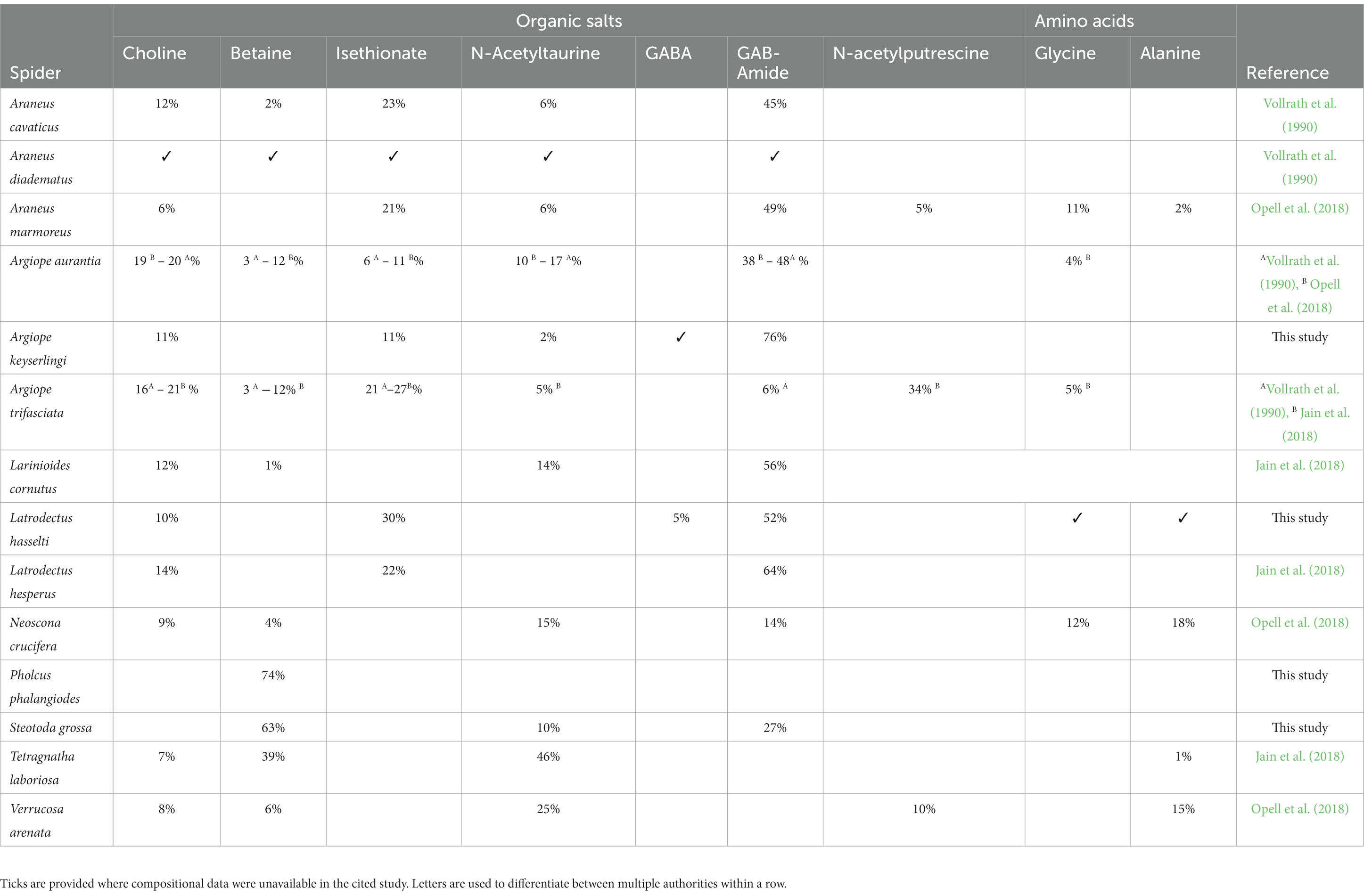
Table 1. Comparisons of the compositions of organic salts identified within the glues for a range of spider species (see the ‘Reference’ column for the authority in each instance).
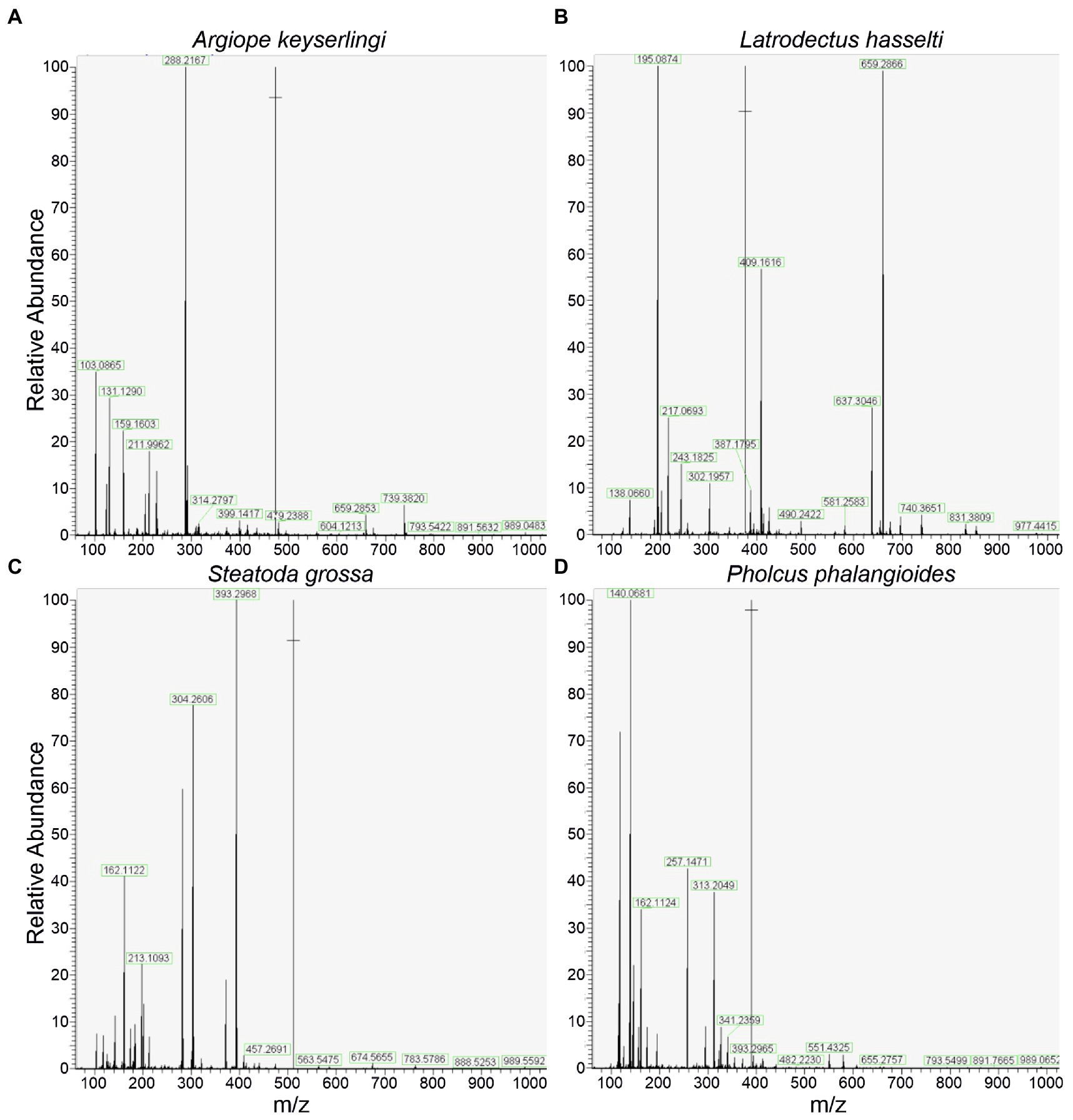
Figure 3. Spectral outputs for the tandem mass spectrometry (MS/MS) of the glues of Argiope keyserlingi (A), Latrodectus hasselti (B), Steatoda grossa (C), and Pholcus phalangiodes (D).
Argiope keyserlingi
The 1H NMR spectra of the glue of the orb web spider A. keyserlingi had a prominent triplet at ~δH 2.41 ppm, coupled with multiplets at 3.04 and 1.96 ppm. According to a previously published spectra (Vollrath et al., 1990), this feature is indicative of the presence of the compounds γ-Aminobutyric acid (GABA) and/or GABA-amide. Our compositional calculations of the integrated peak areas found GABA/GABA-amide to be far and away the most predominant compound (~76%) in the glue of Argiope keyserlingi. Examination of the MS/MS spectra revealed a prominent single peak at 104.07 m/z (Supplementary Figure S1), which we attributed to a GABA/GABA-amide adduct (since GABA/GABA-amide has a corresponding molecular mass of ~103.12 g/mol).
Other compounds identified within the glues of A. keyserlingi in significant quantities were choline (11%), isethionic acid (~11%) and N-acetyltaurine (~2%). Choline was identified by the 1H NMR spectrum from the co-existing multiplets at δH 4.1 and 3.65 ppm and the singlet at δH 3.2 ppm. Isethionic acid was identified by the paired triplets at δH 3.95 ppm and 3.25 ppm, while N-acetyltaurine identified by triplets at ~δH 3.56–3.10 ppm and a singlet for the acetyl group at δH 2.0 ppm. We confirmed the presence of these compounds by reference to the MS/MS spectra by single peaks at 104.10 m/z (attributed to choline), 125.55 (Isethionic acid), and 168.03 (N-acetyltaurine) (Supplementary Figures S2, S3). The concentrations of these compounds were reasonably similar (i.e., within 10%) to those derived for other Argiope sp. glues using 1H NMR (Vollrath et al., 1990; Jain et al., 2018; Table 1). We were thus convinced that our 1H NMR spectra were of comparable resolution to those derived elsewhere for other orb web spider glues (e.g., Vollrath et al., 1990; Sahni et al., 2014b; Jain et al., 2016, 2018).
Latrodectus hasselti
The compounds found in the glues from Latrodectus hasselti were remarkably similar to those found for the congeneric cobweb spider Latrodectus hesperus, as reported by Jain et al. (2018); with a combination of peaks indicating the presence of choline (as described above for A. keyserlingi), along with paired triplets attributable to Isethionic acid, and the group of three multiplets attributable to GABA/GABA-amide (with an estimated composition of 52%). These compounds were also verified by peaks in our MS spectra (Figure 2B, Supplementary Figures S4–S6). Our 1H NMR spectra for the glues of L. hasselti’s differed from those for L. hesperus (Jain et al., 2016, 2018), however, by having an additional quintet at ~δH 2.15 ppm. We ascribed these peaks, with reference to the Human Metabalome Database (Wishart et al., 2009) and our MS spectra, as representing the presence of GABA. Accordingly, we concluded that the glue produced by L. hasselti must have contained both GABA-amide and GABA in quantities shown in Table 1.
An additional feature we detected in the PSAs of L. hasselti that was not detected in those of L. hesperus’ (Jain et al., 2018) was a small triplet peak at around ~ δH 3.4 ppm. Without a reference spectrum of the pure component, nevertheless, the identity of the compound remains uncertain. However, we know it is a three-carbon chain coupled to a triplet at δH 2.346 and a quintet at δH 2.138. One possibility supported by a peak in the MS spectra at 104.07 m/z (see Supplementary Figure S6) is that it may indicate phosphorylated GABA (Wishart et al., 2009). Upon inspection of the 1H NMR spectrum beyond the 5 ppm chemical shift window (Supplementary Figure S7) we noticed several additional peaks that may be indicative of aromatic amino acids, which may form components of the spider coating proteins (SCPs), as observed by Hu et al. (2007). Examination of the spectra to include the 0–1.5 ppm chemical shift window (see inset of Figure 2) revealed further peaks that may be attributable to yet-to-be-identified small chain fatty acids.
Steatoda grossa
Although Steatoda grossa and L. hassleti both belong to the Family Theridiidae, the chemical profile of the glues of the two species differed markedly in several respects (Figures 2B,C). For instance, the singlet at ~δH 3.27 ppm in the spectra derived for the glues of S. grossa was notably absent in that for L. hasselti. According to previous research, this feature, along with the singlet at ~δH 3.90 ppm can be assigned to betaine (Jain et al., 2018). This assignment is further supported by a peak in our MS/MS spectra at 118.08 m/z, which is consistent with the presence of betaine (Supplementary Figures S8, S9). The relative concentration of betaine in the PSA of S. grossa was estimated to be around 63%.
Betaine seems to be absent in the glue of L. hasselti and present in only trace amounts within A. keyserlingi’s glue. It is nonetheless the primary constituent of P. phalangioides’ gluey secretions (see below for details). Its composition detected within the PSAs of other spiders is highly variable, ranging from absent to >40%. The other major compound found in the glues of S. grossa was GABA-amide (~27%), which we determined from the prominent triplet of peaks at δH 2.30 ppm and the MS/MS spectra peak at 104.07 m/z (Supplementary Figures S8, S10). N-acetyltaurine (~10%) was also identified by the triplets at δH 3.56 and δH 3.01 ppm and a singlet at δH 2.00 ppm for the acetyl group. The latter compound was absent altogether from the glue of L. hasselti.
Pholcus phalangioides
Pholcid spiders belong to the spider group called the Synspermiata, which branched out early in the evolution of spiders, thus they are only distantly related to orb web and cobweb building spiders (Wheeler et al., 2017). They also lack aggregate glands so have evolved to use a PSA independent to the three aforementioned areneoids. This explains why the composition of the PSAs of P. phalangioides differed rather substantially from those of the other spider species. The dominating presence of betaine was deduced from two singlets in the NMR spectra at ~δH 3.90 ppm and δH 3.25 ppm, and the peak at 118.086 m/z within the MS/MS spectra (Supplementary Figures S11, S12). Our subsequent compositional analyses found these glues to comprise of >70% betaine, thus resembling S. grossa glues in this regard. Nevertheless, none of the other compounds found in the glue produced by S. grossa (i.e., GABA-amide and N-acetyltaurine) were found within those of P. phalangioides’. Some extremely small peaks, at ~ δH 2.0–2.6 ppm amd ~ δH 3.40 ppm, indicate there were trace amounts of other, unidentified, aliphatic compounds in the glue of P. phalangioides.
Other compounds that we, and others (Jain et al., 2016, 2018; Opell et al., 2018), have detected in spider PSAs include choline and isethionic acid, but these compounds were notably absent from those of P. phalangioides. Instead, the 1H NMR spectra for the PSAs of P. phalangioides had at least two multiplets not found in the spectra of any other spider’s PSAs. One was found at ~δH 3.2 ppm, and another at ~ δH 3.9 ppm. These peaks might represent the methyl resonances of certain amino acids (Mendz et al., 1989). However, amino acid methyl groups should not appear at these chemical shift values if not closely associated with oxygen species (Mendz et al., 1989). These peaks are also at chemical shifts similar to what we might expect for betaine. Notwithstanding, the peaks found in the MS spectra at around 116 m/z (see Supplementary Figures S11, S13) provide evidence enough to suggest that the PSA of P. phalangioides certainly must contain a detectable amount of L-proline.
Discussion
We herein elucidated the compositional profiles of organic salts in the pressure sensitive adhesives of four phylogenetically disparate species of spider (Argiope keyserlingi, Latrodectus hasselti, Steatoda grossa, and Pholcus phalangioides) using 1H Nuclear Magnetic Resonance spectroscopy and mass spectrometry. Previously 1H NMR analyses had been performed for congeners of A. keyserlingi and L. hasselti, albeit using lower field (300 MHz rather than 600 MHz) instrumentation (Vollrath et al., 1990; Jain et al., 2015, 2016, 2018), and yielded reasonably similar results. This study represents the first analysis of organic salt composition within the glues of Steatoda and Pholcus species. Since the members of the genus Pholcus appear to have evolved their gluey secretion independently from those of orb-web and cobweb spiders (i.e., the areneoids), our study stands to significantly expand knowledge about spider glue diversity and evolution, and our understanding of the chemical diversity of natural PSAs. Remarkably, while the composition of the PSAs of P. phalangioides is comparably simple compared to those of the distantly related araneoids, all of the spider’s PSAs appear to be based on a common principle, i.e., that of enabling the softening of a proteinaceous material through the addition of organic salts. While the relative concentrations of the salts differed, the recruitment of the same ubiquitous compounds, such as GABA, choline, isethionic acid, or betaine, recurs remarkably frequently.
Other 1H NMR studies of spider PSAs have found relatively high compositions of GABA/GABA-amide (i.e., >40%) in other orb web spider glues, including those of Aranues diadematus, Araneus cavaticus, Araneus marmoreus, Neoscona crucifera, and Argiope aurantia (Vollrath et al., 1990; Opell et al., 2018). The former-most is a congener of A. keyserlingi so we speculate that similar compounds might be expect in the PSAs of related spiders. Intriguingly, however, these other studies (Vollrath et al., 1990; Jain et al., 2018) have found that yet another species of spider from the genus Argiope, i.e., A. trifasciata, has a low GABA/GABA-amide composition within its gluey secretions (~6% or less). We are unsure as to why the GABA/GABA-amide component from that particular species’ PSA is so different from those of most other species of Argiope. Diet, habitat and geography have been proposed as factors affecting the composition of compounds within the PSAs of different species of Argiope (Blamires et al., 2014, 2017; Henneken et al., 2017; Opell et al., 2018; Henneken et al., 2022). However, considering the general homogeneity in habitat use and diet within the genus Argiope, we have no reason to suspect that the diets, habitats or geographies of the A. trifasciata spiders sampled in the aforementioned studies differed substantially from those of A. keyserlingi.
Our findings, along with those of Vollrath et al. (1990), point to there being variation in the organic salt composition within the PSAs of related species (e.g., between species of Argiope). This may suggest that the composition of any given spider’s PSA is more of a consequence of its environment, or other immediately imminent circumstance, than evolutionary history. We note that two Latrodectus species from very different locations (i.e., L. hasselti from eastern Australia and L. hesperus from North America) had relatively similar PSA components (c.f. this study and Jain et al., 2018), suggesting phylogeny may well be a factor in determining each of the spider’s PSA compositions. Nevertheless, a comparison of the organic salt compositions of the Latrodectus species PSAs with those of Steatoda grossa (which also belongs to the family Theridiidae) suggests that phylogenetic effects are not the primary determinant of the observed variability.
It has been hypothesized that the salt composition of a particular spider’s PSAs correlates with the humidity of its habitat (Opell et al., 2018). The premise here is that spider webs placed in dry environments, for instance those found in open fields and forests during the day experience a greater threat of dehydration than those placed in humid environments, such as closed forests at night, so need to explicitly invest in hygroscopic salts to augment atmospheric water uptake into the PSAs. As stated, comparisons of our findings with those of Jain et al. (2016, 2018) shows that the two species of Latrodectus found on different continents have remarkably similar organic salt compositions within their PSA profiles. This finding could, however, be explained by both species being found in dry, sheltered, urban environments. The organic salt compositions in the PSAs of three species of Argiope (A. aurantia, A. trifasciata, and A. keyserlingi), all of which likely inhabit relatively similar dry temperate grassland habitats, differ substantially from each other (i.e., GABA is abundant in the PSAs of A. keyserlingi and A. auranta, but is virtually absent in those of A. trifasciata).
Another hypothesis posits that the spider’s diet can influence the composition of its PSAs, either by directly affecting the nutrients available for investment in organic salts or because the spiders are induced to alter their investment into organic salts and other PSA compounds in order to tune the adhesive properties of their web’s capture threads (Blamires et al., 2014, 2015, 2017). Without corresponding stickiness testing we were unable to specifically address this hypothesis. Other hypotheses suggest that the organic components in spider PSAs vary across and within species because of the need to invest in pheromones, allomones, and other signalling compounds (Maji et al., 2014; Henneken et al., 2017). Among the organic compounds found in spider PSAs that may vary for this reason are GABA-amide and N-acetyltaurine, and N-acetylputricine (Anderson and Tillinghast, 1980; Henneken et al., 2017). N-acetylputricine, when present, is found in relatively low concentrations (i.e., <10%) (as found here and elsewhere; Opell et al., 2018), so we cannot speculate much about the causes of its variation across species. N-acetyltaurine on the other hand is found in concentrations ranging from 2 to 25% across spider species, including A. keyserlingi (2%) and S. grossa (10%). It has been ascribed as one of the salts responsible for facilitating the uptake of atmospheric water by the gluey droplets (Townley et al., 1991; Edmonds and Vollrath, 1992; Sahni et al., 2014a). Since N-acetyltaurine appears in higher concentrations in the PSAs of nocturnal forest-dwelling spiders than diurnal grassland spiders (Opell et al., 2018), we concur with speculation that its concentration in spider PSAs is likely driven by habitat and/or humidity.
GABA-amide and/or GABA was found in highly variable amounts across all of the spider’s PSAs with the exception of P. phalangioides, where it was detected in trace amounts, if at all. It is also found in large quantities in the PSAs of the orb web spiders Argiope aurantia, Araneus marmoreus, Aranues cavaticus, and Neoscona crucifera, as well as the cobweb spider Latrodectus hesperus (Jain et al., 2015, 2018). Nevertheless, the compounds are found in relatively low concentrations in Argiope trifasciata (Vollrath et al., 1990; Jain et al., 2018), and are absent in Verrecosa arenata (Opell et al., 2018). Different assaying methods across the different studies might explain some of the variation. However, it cannot explain shifts from its predominance (>70%) in some instances, to virtual absence in others. There seems to be factors other than humidity, diet, or inter−/intra-specific signalling, driving investment in the different GABA-derived compounds in the PSAs of spiders. Another possibility is that, since they have the same function (i.e., mobilizing the glycoproteins to keep them hydrated and tacky), many of the organic salts are replaceable and different spiders have recruited one or another salt depending on diet or some other circumstances.
Conclusion
We herein determined the composition of the water-soluble fraction in the PSAs from four different Australian spiders, each of which have, to date, not been previously investigated. Our results demonstrate that analogous spider PSAs are comprised of concentrations of organic salts that are both similar and ubiquitous, but their relative abundances will often vary considerably between species. Comparisons across species within our study, and those examined in other studies (e.g., Vollrath et al., 1990; Jain et al., 2018), suggest that, while guided by common underlying principles, there is not any discernible evolutionary trend in the organic salt composition in the PSAs of different spiders. More likely, factors such as habitat, humidity, diet, and within/between species chemical signalling needs, are most likely driving the individual chemical compositions.
Given the comparatively low diversity of compounds residing in the glues of the species examined herein, it seems that adhesion is attained by a similar biochemical means across species. By examining the chemistries of a wider range of spider glues, as well as the glues of other animals that produce glues with similar functionalities, e.g., comb jellies, mussels, velvet worms, and Arachnocampa glow worms (von Byern et al., 2017; Blamires, 2019; Wolff et al., 2021), common features underlying the functionality of all animal PSAs could be revealed, demonstrating a promising bioprospecting approach for the design of novel, sustainable, adhesives with extended functions.
Data availability statement
The original contributions presented in the study are included in the article/Supplementary material, further inquiries can be directed to the corresponding author.
Author contributions
JW and SB conceived the study, collected spiders, and prepared samples. BC, JY, LA, DT, and JH performed experiments. JW, LA, DT, JH, and SB contributed to the analysis of the MS and NMR experiments. JW and SB wrote the manuscript. All authors have read, commented on, and contributed and approved to the final version of the manuscript.
Funding
Funding was provided by a US National Science Foundation (NSF DMR BMAT # 2105312) grant to JY, a Hermon Slade Foundation grant to SB (HSF 17/6), a Plus Alliance Seed Grant (PA21089) to SB and JY, and an Australian Research Council grants to SB (DE140101281) and JW (DE190101338).
Acknowledgments
Braxton Jones, Hamish Craig, and Madeleine Blamires assisted with the collection of the spiders and glues.
Conflict of interest
The authors declare that the research was conducted in the absence of any commercial or financial relationships that could be construed as a potential conflict of interest.
Publisher’s note
All claims expressed in this article are solely those of the authors and do not necessarily represent those of their affiliated organizations, or those of the publisher, the editors and the reviewers. Any product that may be evaluated in this article, or claim that may be made by its manufacturer, is not guaranteed or endorsed by the publisher.
Supplementary material
The Supplementary material for this article can be found online at: https://www.frontiersin.org/articles/10.3389/fevo.2023.1123614/full#supplementary-material
References
Anderson, C. M., and Tillinghast, E. K. (1980). GABA and taurine derivatives on the adhesive spiral of the orb web of Argiope spiders, and their possible behavioural significance. Physiol. Entomol. 5, 101–106. doi: 10.1111/j.1365-3032.1980.tb00216.x
Ayoub, N. A., Friend, K., Clarke, T., Baker, R. H., Correa-Garhwal, S. M., Crean, A., et al. (2021). Protein composition and associated material properties of cobweb spiders’ gumfoot glue droplets. Integr. Comp. Biol. 61, 1459–1460. doi: 10.1093/icb/icab086
Blamires, S. J. (2019). Biomechanical costs and benefits of sit-and-wait foraging traps. Israel J. Ecol. Evol. 66, 1–10. doi: 10.1163/22244662-20191056
Blamires, S. J., Hasemore, M., Martens, P. J., and Kasumovic, M. M. (2017). Diet-induced covariation between architectural and physicochemical plasticity in an extended phenotype. J. Exp. Biol. 220, 876–884. doi: 10.1242/jeb.150029
Blamires, S. J., Piorkowski, D., Chuang, A., Tseng, Y. H., Toft, S., and Tso, I. M. (2015). Can differential nutrient extraction explain property variations in a predatory trap? Roy. Soc. Open Sci. 2:140479. doi: 10.1098/rsos.140479
Blamires, S. J., Sahni, V., Dhinojwala, A., Blackledge, T. A., and Tso, I. M. (2014). Nutrient deprivation induces property variations in spider gluey silk. PLoS One 9:e88487. doi: 10.1371/journal.pone.0088487
Bott, R. A., Baumgartner, W., Bräunig, P., Menzel, F., and Joel, A.-C. (2017). Adhesion enhancement of cribellate capture threads by epicuticular waxes of the insect prey sheds new light on spider web evolution. Proc. R. Soc. B 284:20170363. doi: 10.1098/rspb.2017.0363
Bré, L. P., Zheng, Y., Pêgo, A. P., and Wang, W. (2013). Taking tissue adhesives to the future: from traditional synthetic to new biomimetic approaches. Biomater. Sci. 1, 239–253. doi: 10.1039/C2BM00121G
Briceño, R. D. (1985). Sticky balls in webs of the spider Modisimus sp. (Araneae, Pholcidae). J. Arachnol. 13, 267–269.
Cicero, D. O., Barbato, G., and Bazzo, R. (2001). Sensitivity enhancement of a two-dimensional experiment for the measurement of heteronuclear long-range coupling constants, by a new scheme of coherence selection by gradients. J. Magn. Reson. 148, 209–213. doi: 10.1006/jmre.2000.2234
Edmonds, D. T., and Vollrath, F. (1992). The contribution of atmospheric water vapour to the formation and efficiency of a spider's capture web. Proc. Roy. Soc. B 248, 145–148. doi: 10.1098/rspb.1992.0055
Gorb, S. N. (2008). Biological attachment devices: exploring nature’s diversity for biomimetics. Phil. Trans. R. Soc. A 366, 1557–1574. doi: 10.1098/rsta.2007.2172
Hanks, T. W., and Sweigers, G. F. (2012). “Introduction: the concept of biomimicry and bioinspiration in chemistry” in Bioinspiration and biomimicry in chemistry. ed. G. F. Sweigers (Hoboken, N.J: Wiley), 1–15.
Hawthorn, A. C., and Opell, B. D. (2003). Van der Waals and hygroscopic forces of adhesion generated by spider capture threads. J. Exp. Biol. 206, 3905–3911. doi: 10.1242/jeb.00618
Hennebert, E., Maldonado, B., Ladurner, P., Flammang, P., and Santos, R. (2015). Experimental strategies for the identification and characterization of adhesive proteins in animals: a review. Interf. Focus 5:20140064. doi: 10.1098/rsfs.2014.0064
Henneken, J., Blamires, S. J., Goodger, J. Q. D., Jones, T. M., and Elgar, M. A. (2022). Population level variation in silk chemistry but not web architecture in a widely distributed orb web spider. Biol. J. Linn. Soc. 137, 350–358. doi: 10.1093/biolinnean/blac089
Henneken, J., Goodger, J. Q. D., Jones, T. M., and Elgar, M. A. (2017). Variation in the web-based chemical cues of Argiope keyserlingi. J. Insect Physiol. 101, 15–21. doi: 10.1016/j.jinsphys.2017.06.005
Hu, X., Yuan, J., Wang, X., Vasanthavada, K., Falick, A. M., Jones, P. R., et al. (2007). Analysis of aqueous glue coating proteins on the silk fibers of the cob weaver, Latrodectus hesperus. Biochemistry 46, 3294–3303. doi: 10.1021/bi602507e
Jain, D., Amarpuri, G., Fitch, J., Blackledge, T. A., and Dhinojwala, A. (2018). Role of hygroscopic low molecular mass compounds in humidity responsive adhesion of spider’s capture silk. Biomacromolecules 19, 3048–3057. doi: 10.1021/acs.biomac.8b00602
Jain, D., Blackledge, T. A., Miyoshi, T., and Dhinojwala, A. (2016). “Unraveling the design principles of black widow’s gumfoot glue” in Biological adhesives. ed. A. M. Smith Second ed (Gewerbestrasse: Springer), 303–319. doi: 10.1007/978-3-319-46082-6_13
Jain, D., Zhang, C., Cool, L. R., Blackledge, T. A., Wesdemiotis, C., Miyoshi, T., et al. (2015). Composition and function of spider glues maintained during the evolution of cobwebs. Biomacromolecules 16, 3373–3380. doi: 10.1021/acs.biomac.5b01040
Japyassú, H. F., and Macagnan, C. R. (2004). Fishing for prey: the evolution of a new predatory tactic among spiders (Araneae, Pholcidae). Revista de Etologia 6, 79–94.
Kay, L., Keifer, P., and Saarinen, T. (1992). Pure absorption gradient enhanced heteronuclear single quantum correlation spectroscopy with improved sensitivity. J. Amer. Chem. Soc. 114, 10663–10665. doi: 10.1021/ja00052a088
Kelly, S. D., Opell, B. D., and Owens, L. L. (2019). Orb weaver glycoprotein is a smart biological material, capable of repeated adhesion cycles. Sci. Nat. 106:10. doi: 10.1007/s00114-019-1607-z
Liu, K., and Jiang, L. (2011). Bio-inspired design of multiscale structures for function integration. Nano Today 6, 155–175. doi: 10.1016/j.nantod.2011.02.002
Maji, K., Sarkar, R., Bera, S., and Haldar, D. (2014). A small molecule peptidomimetic of spider silk and webs. Chem. Comm. 50, 12749–12752. doi: 10.1039/C4CC04475D
Mendz, G. L., McCall, M. N., and Kuchel, P. W. (1989). Identification of methyl resonances in the 1H spectrum of incubated blood cell lysates. J. Biol. Chem. 264, 2100–2107. doi: 10.1016/S0021-9258(18)94147-5
Opell, B. D., Andrews, S. F., Karinshak, S. E., and Sigler, M. A. (2014). The stability of hygroscopic compounds in orb-web spider viscous thread. J. Arachnol. 43, 152–157. doi: 10.1636/M14-62
Opell, B. D., Jain, D., Dhinojwala, A., and Blackledge, T. A. (2018). Tuning orb spider glycoprotein glue performance to habitat humidity. J. Exp. Biol. 221:jeb161539. doi: 10.1242/jeb.161539
Palmer, A. G., Cavanagh, J., Wright, P. E., and Rance, M. (1991). Sensitivity improvement in proton-detected two-dimensional heteronuclear correlation NMR spectroscopy. J. Magnet. Res. 93, 151–170. doi: 10.1016/0022-2364(91)90036-S
Patek, S. N. (2014). Biomimetics and evolution. Science 345, 1448–1449. doi: 10.1126/science.1256617
Piorkowski, D., Liao, C. P., Joel, A. C., Wu, C. L., Doran, N., Blamires, S. J., et al. (2020). Adhesion of cribellate spider silk enhanced in high humidity by mechanical plasticization of the underlying fiber. J. Mech. Behav. Biomed. Mater. 114:104200. doi: 10.1016/j.jmbbm.2020.104200
Ravanbakhsh, S., Liu, P., Bjorndahl, T. C., Mandal, R., Grant, J. R., Wilson, M., et al. (2015). Accurate, fully-automated NMR spectral profiling for metabolomics. PLoS One 10:e0124219. doi: 10.1371/journal.pone.0124219
Sahni, V., Blackledge, T. A., and Dhinojwala, A. (2010). Viscoelastic solids explain spider web stickiness. Nature Comm. 1:19. doi: 10.1038/ncomms1019
Sahni, V., Blackledge, T. A., and Dhinojwala, A. (2011). A review on spider silk adhesion. J. Adhes. 87, 595–614. doi: 10.1080/00218464.2011.583588
Sahni, V., Dhinojwala, A., Opell, D. B., and Blackledge, T. A. (2014a). “Prey capture adhesives produced by orb-weaving spiders” in Biotechnology of silk. eds. T. Asakura and T. Miller (Dordrecht: Springer), 203–217.
Sahni, V., Miyoshi, T., Chen, K., Jain, D., Blamires, S. J., Blackledge, T. A., et al. (2014b). Direct solvation of glycoproteins by salts in spider silk glues enhances adhesion and helps to explain the evolution of modern spider orb webs. Biomacromolecules 15, 1225–1232. doi: 10.1021/bm401800y
Santos, R., Aldred, N., Gorb, S. N., and Flammang, P. (2013). Biological and biomimetic adhesives: Challenges and opportunities (RSC Publishing: Cambridge, UK.
Schleucher, J., Schwendinger, M., Sattler, M., Schmidt, P., Schedletzky, O., Glaser, S. J., et al. (1994). A general enhancement scheme in heteronuclear multidimensional NMR employing pulsed field gradients. J. Biomol. NMR 4, 301–306. doi: 10.1007/BF00175254
Singla, S., Amarpuri, G., Dhopatkar, N., Blackledge, T. A., and Dhinojwala, A. (2018). Hygroscopic compounds in spider aggregate glue remove interfacial water to maintain adhesion in humid conditions. Nat. Comm. 9:1890. doi: 10.1038/s41467-018-04263-z
Torres, F. G., Troncoso, O. P., and Cavalie, F. (2014). Physical characterization of the liquid adhesive from orb-weaving spiders. Mater. Sci. Eng. C Mater. Biol. Appl. 34, 341–344. doi: 10.1016/j.msec.2013.09.030
Townley, M. A., Berbstein, D. T., Gallagher, K. S., and Tillinghast, E. K. (1991). Comparative study of orb web hygroscopicity and adhesive spiral composition in three araneid spiders. J. Exp. Zool. 259, 154–165. doi: 10.1002/jez.1402590203
Townley, M. A., and Tillinghast, E. K. (2013). “Aggregate silk gland secretions of Araneoid spiders” in Spider Ecophysiology. ed. W. Nentwig (Berlin: Springer), 283–302.
Vasanthavada, K., Hu, X., Tuton-Blasingame, T., Hsia, Y., Sampath, S., Pacheco, R., et al. (2012). Spider glue proteins have distinct architectures compared with traditional spidroin family members. J. Biol. Chem. 287, 35986–35999. doi: 10.1074/jbc.M112.399816
Vendamme, R., Schüwer, N., and Evans, W. (2014). Recent synthetic approaches and emerging bio-inspired strategies for the development of sustainable pressure-sensitive adhesives derived from renewable building blocks. J. Appl. Polym. Sci. 131:40669. doi: 10.1002/app.40669
Vollrath, F., Fairbrother, W. J., Williams, R. J. P., Tillinghast, E. K., Bernstein, D. T., Gallagher, K. S., et al. (1990). Compounds in the droplets of the orb spider's viscid spiral. Nature 345, 526–528. doi: 10.1038/345526a0
von Byern, J., and Grunwald, I. (2010). Biological Adhesive Systems: From Nature to Technical and Medical Application, Springer, Wien.
von Byern, J., Muller, C., Voigtländer, K., Dorrer, V., Marchetti-Deschmann, M., Flamming, P., et al. (2017). “Examples of bioadhesives for defense and predation” in Functional surfaces in biology III. eds. S. N. Gorb and E. V. Gorb (Cham, Switzerland: Springer), 141–191.
Wheeler, W. C., Coddington, J. A., Crowley, L. M., Dimitrov, D., Goloboff, P. A., Griswald, C. E., et al. (2017). The spider tree of life: phylogeny of Araneae based on target gene analysis from an extensive taxon sampling. Cladistics 33, 574–616. doi: 10.1111/cla.12182
Willker, W., Leibfritz, D., Kerssebaum, R., and Bermel, W. (1993). Gradient selection in inverse heteronuclear correlation spectroscopy. Magnet. Res. Chem. 31, 287–292. doi: 10.1002/mrc.1260310315
Wirth, M., Wolff, J. O., Appel, E., and Gorb, S. N. (2019). Ultrastructure of spider thread anchorages. J. Morphol. 280, 534–543. doi: 10.1002/jmor.20962
Wishart, D. S., Knox, C., Guo, A. C., Eisner, R., Young, N., Gautam, B., et al. (2009). HMDB: a knowledgebase for the human metabolome. Nucleic Acids Res. 37, D603–D610. doi: 10.1093/nar/gkn810
Wolff, J. O., and Gorb, S. N. (2016). Attachment structures and adhesive secretions in arachnids. (Springer: Cham, Switzerland
Wolff, J. O., Grawe, I., Writh, M., Karstedt, A., and Gorb, S. N. (2015). Spider's super-glue: thread anchors are composite adhesives with synergistic hierarchical organization. Soft Matt. 11, 2394–2403. doi: 10.1039/C4SM02130D
Wolff, J. O., von Byern, J., Piorkowski, D., Fang, J., Wang, X., Adler, L., et al. (2021). Adhesive compounds in the capture threads of glow worms. Front. Mech. Eng. 7:661422. doi: 10.3389/fmech.2021.661422
Wolff, J. O., Wells, D., Reid, C. R., and Blamires, S. J. (2017). Clarity of objectives and working principles enhances the success of biomimetic programs. Bioinspir. Biomim. 12:051001. doi: 10.1088/1748-3190/aa86ff
Keywords: 1H-nuclear magnetic resonance spectroscopy, mass spectrometry, natural adhesives, spider silk, Araneoidea
Citation: Wolff JO, Cherry BR, Yarger JL, Adler L, Thomas DS, Hook JM and Blamires SJ (2023) Organic salt composition of pressure sensitive adhesives produced by spiders. Front. Ecol. Evol. 11:1123614. doi: 10.3389/fevo.2023.1123614
Edited by:
David Guez, Hydrogen Technologies, AustraliaReviewed by:
Brent Opell, Virginia Tech, United StatesNadia A. Ayoub, Washington and Lee University, United States
Copyright © 2023 Wolff, Cherry, Yarger, Adler, Thomas, Hook and Blamires. This is an open-access article distributed under the terms of the Creative Commons Attribution License (CC BY). The use, distribution or reproduction in other forums is permitted, provided the original author(s) and the copyright owner(s) are credited and that the original publication in this journal is cited, in accordance with accepted academic practice. No use, distribution or reproduction is permitted which does not comply with these terms.
*Correspondence: Sean J. Blamires, c2Vhbi5ibGFtaXJlc0B1bnN3LmVkdS5hdQ==; c2Vhbi5ibGFtaXJlc0B1dHMuZWR1LmF1