- 1Faculty of Biology, University of Belgrade, Belgrade, Serbia
- 2Faculty of Chemistry, University of Belgrade, Belgrade, Serbia
- 3Faculty of Medical Sciences, University of Kragujevac, Kragujevac, Serbia
- 4Geographical Institute “Jovan Cvijić”, Serbian Academy of Sciences and Arts, Belgrade, Serbia
- 5Đerdap National Park, Working Unit in Tekija, Tekija, Serbia
- 6Faculty of Geography, University of Belgrade, Belgrade, Serbia
- 7Faculty of Sciences and Mathematics, University of Priština in Kosovska Mitrovica, Kosovska Mitrovica, Serbia
The pygidial glands of carabids produce strong-smelling vapours. In this study, we examined the chemical composition of the gland secretions and the structure of the glands in five species of Carabini ground beetles (one species from the subtribe Calosomatina and four species from the subtribe Carabina): Calosoma (Calosoma) maderae (Fabricius, 1775), Carabus (Carabus) granulatus Linnaeus, 1758, C. (Limnocarabus) clathratus Linnaeus, 1760, C. (Carabus) ulrichii Germar, 1823, and C. (Procerus) gigas Creutzer, 1799. Additionally, we tested the antibacterial potential of the pygidial gland secretions of the two latter species against 11 bacterial strains. In order to detect the chemical content of the secretions, we used gas chromatography–mass spectrometry (GC–MS). The secretion extracts were applied against selected strains of medically important bacteria. We used bright-field microscopy to examine the morphology of the glands. We discovered a total of 11 chemical compounds in the pygidial gland extracts of the ground beetles we analysed. Ten of these compounds were identified as seven carboxylic acids, two hydrocarbons, and one aromatic aldehyde, while one chemical remained unidentified. Most of the components were isolated from the secretion of C. (L.) clathratus (nine), while the lowest number of compounds was found in C. (P.) gigas (two). Methacrylic acid was the most dominant compound by percentage in all five species, while angelic acid was also detected in all samples. As expected, salicylaldehyde was exclusively found in the species of the genus Calosoma Weber, 1801. The secretion of C. (P.) gigas was shown to achieve the highest level of antibacterial activity against Pseudomonas aeruginosa, Salmonella enterica, and S. typhimurium (even the same level as the positive control streptomycin), while the secretion of C. (C.) ulrichii achieved the highest antimicrobial potential against Staphylococcus epidermidis, S. aureus, Listeria monocytogenes, and Bacillus cereus. The most noticeable difference in the structure of the glands between the two genera is that the reservoir in Calosoma is more significantly narrowed as it leads into the efferent duct, compared to that of Carabus.
1. Introduction
Multiple aspects of chemical interactions exist between insects and their predators, but defence certainly takes a prominent place among those factors which have contributed to their widespread success in ecosystems (Blum, 1981; Pasteels et al., 1983). Ground beetles (Carabidae) are capable of producing exocrine compounds, whose outstanding diversity has attracted attention for many years, leading to multiple investigations of their chemical ecology (Moore and Wallbank, 1968; Will et al., 2000; Rork and Renner, 2018). There is no doubt that releasing those chemicals is one of the strategies for predator avoidance. However, not enough is known about the additional biological functions of the defensive compounds of carabids. Considering other groups of insects, it is known that the discharge of defensive compounds leads to an overall more successful response to predatory attack (alarm pheromone function) among conspecifics (Pasteels et al., 1983; Blum, 1996). Although several studies on the dual function (intraspecific and interspecific chemical communication) of ground beetles’ defensive substances have been carried out (Attygalle et al., 1991; Bonacci et al., 2011; Holliday et al., 2016), no generalised conclusions have been drawn. The occurrence of multi-compound defensive secretions creates the possibility of variations. To date, a limited number of studies indicate the existence of alterations to defensive secretions caused by various ecological factors (Wheeler et al., 1970; Holliday et al., 2012, 2016; Vesović et al., 2022).
One of the diagnostic features of beetles belonging to the suborder Adephaga is the presence of paired defensive glands in their abdomen (Forsyth, 1968, 1970, 1972; Vesović, 2019). These exocrine glands, called anal or pygidial, play a primary role in their chemical defence against predators (Schildknecht et al., 1964; Will et al., 2000), while additional roles remain poorly understood (Rork and Renner, 2018). The role of pygidial glands in survival is certainly significant (Blum, 1996), which is one of the reasons for the rather conservative morphology of these organs in the representatives of various subfamilies of ground beetles (Vesović, 2019). Each pygidial gland consists of secretory lobes, a main collection canal (which transports the secretion to the storage site), and a glandular reservoir, from where the secretion is released into the external environment, usually by oozing or spraying (Moore, 1979). The third type of secretion discharge is a mechanism called crepitation, which is found in bombardier beetles and a few other related tribes, in which a reaction chamber occurs next to the glandular reservoir (Eisner et al., 1977; Dean et al., 1990). In the exergonic chemical reaction, during which catalase releases oxygen from hydrogen, while peroxidases oxidize hydroquinones into 1,4-quinones, accompanied by sound and a temperature rise up to 100°C, bombardier beetles release their toxic chemicals towards the attacker (Aneshansley et al., 1969; Di Giulio et al., 2015).
The chemical composition of pygidial gland secretions has been determined in slightly more than 500 species of ground beetles, which is still a small number of species in relation to the total species diversity of the entire family. The chemicals released by the pygidial glands can be classified into several groups of compounds: quinones, aldehydes, alcohols, esters, phenols, carboxylic acids, ketones, monoterpenes, nitriles and hydrocarbons (Moore, 1979; Vesović, 2019). Recently, a sulphur-bearing isoprene derivate has been recorded for the first time in ground beetles and arthropods overall (Xu et al., 2019).
In recent years, the biological activity of secretions in Coleoptera has been increasingly investigated (Barnes et al., 2010; Nenadić et al., 2016a,b, 2017a,b, 2018; Nenadić, 2019; Dimkić et al., 2020; Vesović et al., 2022). This is mainly due to the fact that compounds with antimicrobial properties could occur in their secretions, which probably contributes to the ecological role of these natural products (Nenadić et al., 2017a; Dimkić et al., 2020; Pu et al., 2020). Accordingly, the antibacterial and antifungal properties of secondary metabolites in species of the family Silphidae and the pygidial gland secretions of species of the family Carabidae are apparently provided by the presence of isobutyric acid within their secretion mixtures (Schildknecht and Weis, 1962; Degenkolb et al., 2011; Lečić et al., 2014). In addition, benzoic acid, phenylacetic acid, p-cresol, m-cresol, 1,4-benzoquinone and formic acid represent the typical constituents of the pygidial gland secretions of numerous beetles (Francke and Dettner, 2005; Bonacci et al., 2008; Degenkolb et al., 2011), with previously established bactericidal and bacteriostatic properties (Huang et al., 2011; Carcamo et al., 2019). The production of compounds with antimicrobial properties within their secretions is most likely a strategy used by those insects in order to protect their individuals (primarily in the pupal stage) from possible pathogenic microorganisms within their environment. In this sense, certain taxa of ground beetles [e.g., Carabus (Chaetocarabus) intricatus lefebvrei Dejean, 1826] even produce terpenes in the pupal stage, which were previously found to be responsible for the antibacterial properties of plant extracts (Giglio et al., 2009, 2011).
In addition to their possible ecological role, certain ground beetles’ secretions could presumably also be a source of antimicrobial agents due to the low level of cytotoxicity in minimal concentrations (Nenadić et al., 2018). Therefore, such investigations may be promising in order to improve the effectiveness of existing antibiotics in the future (Nenadić, 2019).
The structure of the pygidial glands in Calosoma (Calosoma) maderae (Fabricius, 1775), Carabus (Carabus) granulatus Linnaeus, 1758, C. (C.) ulrichii Germar, 1823, C. (Limnocarabus) clathratus Linnaeus, 1760, and C. (Procerus) gigas Creutzer, 1799 has not been analysed to date. Of all the aforementioned ground beetle species, only the antimicrobial activity of the pygidial gland secretion of C. (C.) ulrichii [but of another subspecies of C. (C.) ulrichii and against different microorganisms] has been analysed so far (Nenadić et al., 2016a). When it comes to the chemical composition of the secretions of the listed species, this was identified a long time ago only in C. (C.) granulatus (Schildknecht et al., 1968) and C. (C.) ulrichii [in its subspecies C. (C.) ulrichii rhilensis Kraatz, 1876] (Lečić et al., 2014).
The aims of the current study were to: (i) identify the chemical composition of the pygidial gland secretions of five ground beetle taxa belonging to the tribe Carabini (one belonging to the subtribe Calosomatina and four belonging to the subtribe Carabina): C. (C.) maderae, C. (C.) granulatus, C. (L.) clathratus, C. (C.) ulrichii [subspecies C. (C.) ulrichii arrogans Schaum, 1859], and C. (P.) gigas; (ii) present the antibacterial action of the pygidial gland secretions of the two latter taxa; and (iii) describe the morphology of the pygidial gland components of the five mentioned taxa.
2. Materials and methods
2.1. Insect material
Adult specimens (five males and five females) of C. (C.) maderae (Figure 1A) were collected using pitfall traps with no preservative fluid on a field of oilseed rape on 15th June 2021 in the village of Crepaja (near the town of Kovačica), northern Serbia, 45°03′28.2″N, 20°43′24.5″E. The subspecies analysed in this paper is C. (C.) maderae maderae (Fabricius, 1775).
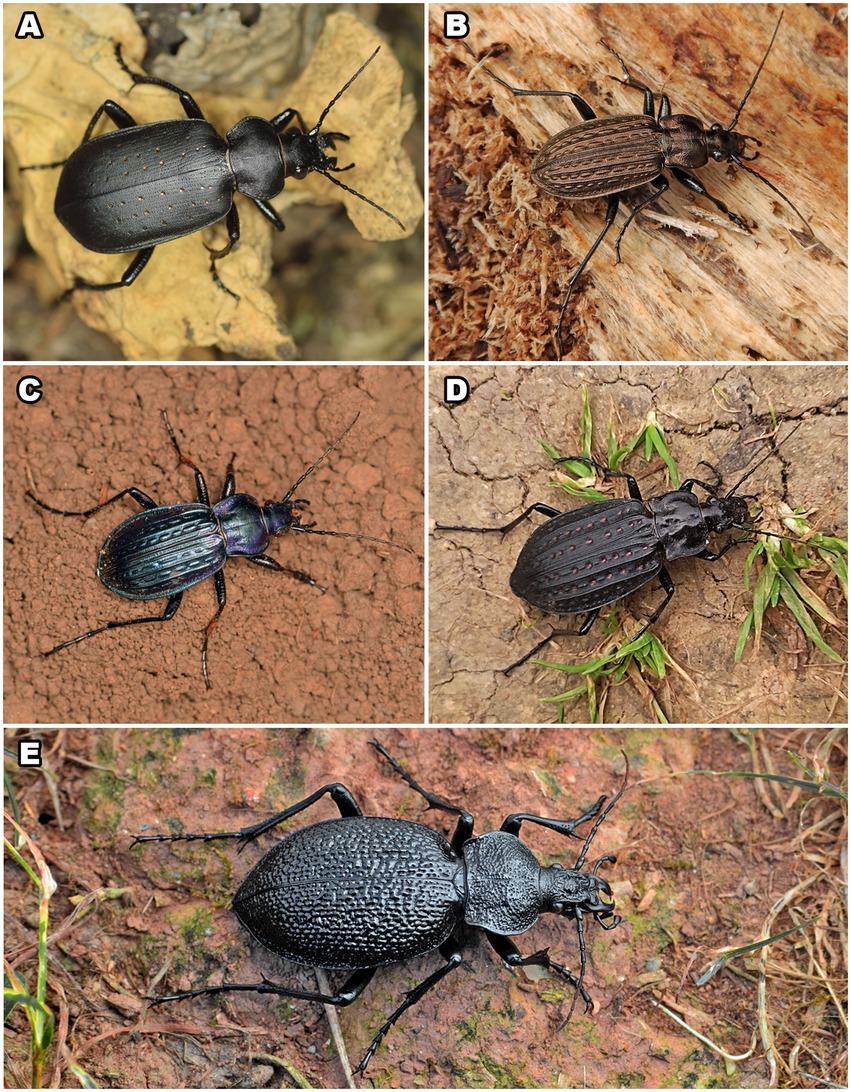
Figure 1. Five species of Carabini whose pygidial glands were analysed: Calosoma (Calosoma) maderae (A), Carabus (Carabus) granulatus (B), C. (Carabus) ulrichii (C), C. (Limnocarabus) clathratus (D), and C. (Procerus) gigas (E). Photos: N. Vesović.
Adult individuals (three males and five females) of C. (C.) granulatus (Figure 1B) were collected manually from the inside of rotten willow logs alongside the Tamiš River on 27th March 2021 in the village of Jabuka (near the town of Pančevo), northern Serbia, 44°56′21.3″N, 20°34′37.3″E. We analysed the subspecies C. (C.) granulatus granulatus Linnaeus, 1758.
Adult individuals of C. (C.) ulrichii arrogans (Figure 1C) were collected manually on 1st May 2022 in the village of Tekija (near the town of Kladovo), eastern Serbia, 44°40′52.6″N, 22°24′42.9″E (four males and five females), as well as on 3rd May 2021 from Mali Štrbac peak, Mt. Miroč, eastern Serbia, 44°38′11.0″N, 22°18′43.3″E (one male and one female).
Adult individuals (six males and seven females) of C. (L.) clathratus (Figure 1D) were collected manually from the inside of rotten willow and poplar logs alongside the Tamiš River on 27th March 2021 in the village of Jabuka (near the town of Pančevo), northern Serbia, 44°56′19.1″N, 20°35′10.2″E. The subspecies analysed in this paper is C. (L.) clathratus auraniensis Müller, 1903.
Adult individuals of C. (P.) gigas (Figure 1E) were collected manually under the bark of old tree trunks, in leaf litter, or on roads alongside rivers and streams, mainly near or in deciduous forests at several sites in Serbia: Košutnjak Forest, Belgrade, central Serbia, 44°45′30.4″N, 20°26′14.4″E (two females collected on 8th June 2021); the village of Jelovica, Stara Planina Mts., southeastern Serbia, 43°12′01.7″N, 22°49′56.0″E (one male and one female collected on 30th June 2021); Stepin Lug Forest, Belgrade, central Serbia, 44°44′56.0″N, 20°31′49.9″E (one female collected on 30th April 2022); and the village of Dugo Polje, Mt. Devica, southeastern Serbia, 43°35′53.6″N, 21°56′50.1″E (one male collected on 25th June 2022). We analysed the subspecies C. (P.) gigas gigas Creutzer, 1799.
The beetles were transferred from the field to the laboratory, where they were subjected to constant temperature (15°C) and high humidity (maintained by water spraying). The specimens were fed on snails and earthworms and were placed in the laboratory for several weeks in order to complete all the planned experiments (chemical, antimicrobial, and morphological analyses).
After completing the analyses, the beetles used in this study were pinned and deposited in the collection of the Institute of Zoology, University of Belgrade - Faculty of Biology, Belgrade, Serbia. Macro photographs of the studied Carabini ground beetles were taken by a Nikon D5300 DSLR digital camera equipped with a Tamron SP Di AF 90 mm F/2.8 macro lens and a Sigma EM-140 DG flash ring.
2.2. Chemical extraction and chemical analyses of the secretions
We used gas chromatography–mass spectrometry (GC–MS) for the separation and identification of the chemical constituents of the secretions. The pygidial gland secretions of five species of Carabini were dissolved in dichloromethane (DCM) obtained from Merck (Darmstadt, Germany). The adult specimens of each tested ground beetle species [five males and five females of C. (C.) maderae, three males and five females of C. (C.) granulatus, four males and five females of C. (C.) ulrichii, six males and seven females of C. (L.) clathratus, and two males and four females of C. (P.) gigas] were induced (by pressing and squeezing their abdominal tips and pinching their legs using tweezers) to release their pygidial gland secretions into a 5-ml glass vial with 0.5 ml of DCM for 5 min. Extracts of individuals of the same sex of each species were pooled and analysed together [except in the case of C. (P.) gigas, where two females were analysed separately]. We also pooled together the samples of different sexes for C. (C.) granulatus and C. (L.) clathratus, as preliminary analyses failed to show any differences between the sexes. Just in case, we retained the analyses separated by sex for the remaining two species of carabids [C. (C.) ulrichii and C. (P.) gigas], since we did not have any previous data on the chemistry of their pygidial gland secretions. In order to eliminate the effects of composition-altering oxidation and the degradation of compounds, a portion of each secretion extract was subjected to immediate GC–MS analysis after collecting the secretion.
We used an Agilent 7890A GC system equipped with a 5975C inert XL EI/CI mass selective detector (MSD) and a flame ionization detector (FID), connected by capillary flow technology through a two-way splitter, and a polar HP-INNOWax capillary column [30 m length, 0.32 mm i.d., 0.25 μm film thickness (Agilent Technologies, Santa Clara, United States)] for the chemical analyses. The GC and GC–MS analyses were carried out in splitless mode. For all the analyses, the injection volume was 1 μl and the injector temperature was 240°C, while the transfer line temperature was 280°C. The carrier gas (He) flow rate was 2.0 ml min−1 at 40°C (constant pressure mode). The oven temperature was set at 40°C, then increased at a rate of 10°C min−1, with a final 10-min holding time. The FID temperature was 300°C. EI mass spectra (70 eV) were acquired in the range of 35–550 m/z. For all the analyses, the ion source temperature was 230°C and the quadrupole temperature was 150°C (Vesović et al., 2022).
Mass spectral deconvolution and extraction, as well as a library search were performed using NIST Automated Mass Spectral Deconvolution and Identification System (AMDIS) ver. 2.70 software and the commercially available NIST17 and Wiley07 libraries. Additionally, all the identified chemical compounds were compared with authentic standards [salicylaldehyde, n-tricosane, (Z)-9-tricosene, isobutyric, butyric, 2-methylbutyric, methacrylic, angelic, benzoic, and tiglic acids] (Tokyo Chemical Industry Co., Ltd., Tokyo, Japan).
The relative percentages of the identified compounds were computed from the corresponding GC–FID peak areas using MSD ChemStation ver. E02.02 software (Agilent Technologies, Santa Clara, United States).
2.3. In vitro antimicrobial assay
2.3.1. Commercial chemicals
Methacrylic acid >99% (Tokyo Chemical Industry Co., Ltd., Tokyo, Japan) [10 mg of active substance dissolved in 1 ml of 0.01 M phosphate-buffered saline (PBS)] and angelic acid >98% (Tokyo Chemical Industry Co., Ltd., Tokyo, Japan) (10 mg of active substance dissolved in 1 ml of 0.01 M PBS) were applied as the commercial standards in this study.
2.3.2. Commercial antibiotics
Streptomycin (1 mg of active substance dissolved in 1 ml of physiological solution) (Sigma-Aldrich, Munich, Germany) and gentamicin (500 mg of amoxicillin trihydrate dissolved in 500 ml of physiological solution) (Panfarma, Belgrade, Serbia) were used as positive controls.
2.3.3. Tested bacterial strains
In total 11 laboratory strains of bacterial clinical pathogens were tested in this study, of which five were gram-positive [Bacillus cereus (ATCC 11778), MRSA (ATCC 43300), Staphylococcus epidermidis (ATCC 12228), S. aureus (ATCC 29213), and Listeria monocytogenes (ATCC 13932)] and six were gram-negative [Enterobacter cloacae (ATCC 13047), Klebsiella pneumoniae (ATCC 70603), Escherichia coli (ATCC 35218), Salmonella typhimurium (ATCC 14028), S. enterica (ATCC 13076), and Pseudomonas aeruginosa (ATCC 10145)].
All the bacterial strains were deposited in the laboratory of the Institute of Physiology and Biochemistry “Ivan Đaja”, University of Belgrade - Faculty of Biology, Belgrade, Serbia.
2.3.4. Quantitative analysis of the secretions and the preparation of the samples for antimicrobial study
The total amounts of the pygidial gland secretions of the ground beetle species C. (P.) gigas (one adult female) and C. (C.) ulrichii (four males and five females) were quantified. The amounts of the discharged pygidial gland secretions were measured on a Sartorius Model 2405 analytical balance for weighing up to 30 g maximum capacity, with 0.001 mg resolution (Sartorius Group, Göttingen, Germany) at the University of Belgrade - Faculty of Chemistry, Belgrade, Serbia. Adult individuals of each species were milked into a 2-ml empty vial in the laboratory to measure the amount of secretion. The weight of the vials was measured on the analytical balance both prior to (an empty vial) and after the pygidial gland secretion was discharged. The differences between these weights represented the total amounts of the released pygidial gland secretions.
Both the biological samples and commercial standards were prepared and dissolved in 0.01 M PBS (Sigma-Aldrich, Munich, Germany) at room temperature and then stored in a refrigerator at 4°C in the laboratory. An antimicrobial assay was performed immediately after sampling to eliminate the impacts of composition-altering oxidation and component degradation.
2.3.5. Preparing overnight bacterial cultures
A total of 11 bacterial strains, previously stocked in glycerol, were seeded on Trypticase Soy Agar (TSA) medium [15 g of agar dissolved in 1 l of Tryptic Soy Broth (TSB) medium] (Lab M Ltd., Heywood, United Kingdom) and incubated in a thermostat for 24 h at 30°C. After incubation, each bacterial strain was inoculated in 5 ml of 0.01 M PBS and stored in a refrigerator at 4°C in the laboratory. The final tested concentration of the bacterial suspensions was 1 × 108 CFU/ml.
2.3.6. In vitro broth microdilution method
Minimum inhibitory concentrations (MICs) are defined as the lowest concentrations of antimicrobial agents which inhibit the visible growth of a microorganism after incubation, while minimum bactericidal concentrations (MBCs) represent the lowest concentrations of antimicrobials which prevent bacterial growth after subculture on to antibiotic-free media (Andrews, 2001). The MICs and MBCs of the tested agents were determined in vitro using the broth microdilution method according to Ristivojević et al. (2016) and Dimkić et al. (2020). Two-fold serial dilutions of the tested secretions and commercial standards dissolved in 0.01 M PBS were performed with TSB medium (Lab M Ltd., Heywood, UK) in 96-well microtiter plates (F-microplate with a cover) (Thermo Scientific™ BioLite Multidishes and Microwell Plates, Thermo Fisher Scientific, Waltham, United States). Each well, except in the case of the sterility control, was inoculated with 60 μl of the tested agents and 10 μl of bacterial culture (1 × 108 CFU/ml). The suspensions were adjusted to reach a final volume of 100 μl.
The final tested concentrations of the analysed agents for 11 selected bacterial strains were in the following range: C. (P.) gigas – 0.3–0.6 mg/ml, C. (C.) ulrichii – 0.403–0.806 mg/ml, methacrylic acid – 0.375–1.5 mg/ml, and angelic acid – 0.375–3.0 mg/ml. In addition to a negative control (bacterial growth control) and a sterility control, the antibiotics streptomycin (in a concentration range of 0.019–0.6 mg/ml) and gentamicin (in a concentration range of 0.009–0.3 mg/ml) were used as positive controls. Finally, the plates were incubated for 24 h at 30°C.
2.3.7. Determination of the MICs and MBCs
In order to evaluate the MICs and MBCs, 22 μl of resazurin [resazurin sodium salt (C12H6NNaO4) (TCI, Zwijndrecht, The Netherlands)], at a final concentration of 0.675 mg/ml, was added to each well, following which the microtiter plates were additionally incubated for 24 h at 30°C. After incubation, the resazurin colour change reaction has been observed. The MIC values were determined as indicated without any colour change, while the MBCs were obtained by subculturing the test dilutions from each well without any colour change on agar plates and incubating them for 24 h. The lowest concentration showing no bacterial growth was defined as the MBC value. The results were expressed in mg/ml.
2.3.8. Statistical processing of the results
One-way analysis of variance (ANOVA) was used to examine the results presented as mean values and standard errors. Tukey’s HSD test, with a significance level of 0.05, was then conducted. Statgraphics Centurion XVI ver. 16.2 software was used to evaluate the results of this study.
2.4. General morphology of pygidial glands
All the morpho-functional compartments of the paired pygidial glands of the adults of five Carabini species [C. (C.) maderae, C. (C.) granulatus, C. (C.) ulrichii, C. (L.) clathratus, and C. (P.) gigas] were isolated, described, measured and photographed.
One male and one female of each of the five species were subjected to abdominal dissections with the aim of isolating and characterising their pygidial gland components morphologically. The ground beetles were anaesthetised with CO2 prior to euthanasia. The abdomens were carefully dissected in a physiological saline solution (0.90% NaCl). After removing the elytra and abdominal tergites, precision tweezers were used to remove the non-target tissues around the pygidial glands. For the purpose of imaging, the pygidial glands were transferred to glycerol because it prevents the delicate structures from moving during photographing. The pygidial glands were dissected and imaged using a Nikon SMZ800N stereomicroscope (Nikon, Tokyo, Japan) with a Nikon DS-Fi2 digital camera (Nikon, Tokyo, Japan) attached. A Nikon DS-L3 control unit (Nikon, Tokyo, Japan) was used for scaling.
3. Results
3.1. Chemical composition of the pygidial gland secretions
A total of 11 chemicals were detected in the pygidial gland secretions of five Carabini species, of which 10 were identified [seven carboxylic acids, one aromatic aldehyde, and two hydrocarbons (one alkane and one alkene)] and one was unidentified (Table 1). The most complex chemical mixture was found in the pygidial gland secretion of C. (L.) clathratus, where we detected nine components (methacrylic acid was the most dominant chemical, followed by tiglic, angelic and benzoic acids, while the remaining five chemicals were represented by less than 1% each). The secretions of C. (C.) maderae and C. (C.) granulatus also contained a significant number of chemicals (six in the former species – with methacrylic acid and salicylaldehyde as the major compounds, followed by tiglic, benzoic and angelic acids, as well as by the hydrocarbon (Z)-9-tricosene found in traces vs. four carboxylic acids in the latter species – methacrylic, tiglic, 2-methylbutyric, and angelic). The pygidial gland secretion of C. (C.) ulrichii [subspecies C. (C.) ulrichii arrogans] contained three carboxylic acids (methacrylic was predominant, followed by tiglic and angelic). The simplest chemical mixture was detected in the secretion of C. (P.) gigas, where we found only two components in the adult specimens of both the analysed populations (methacrylic acid was the dominant compound, while angelic acid was present in a smaller percentage). We did not observe any qualitative differences in the chemical composition of the secretions in different sexes and in individuals from different populations, while the quantitative differences were insignificant.
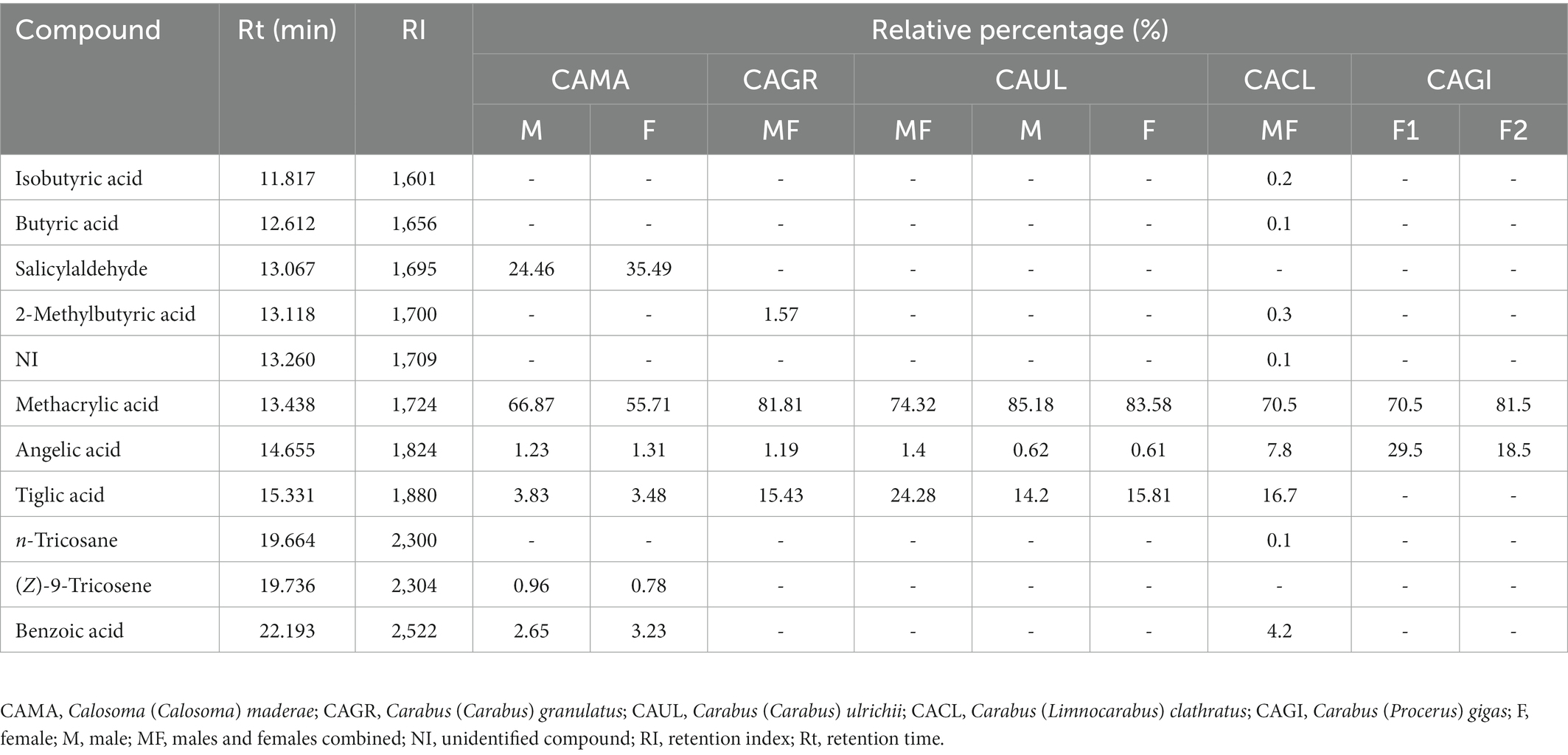
Table 1. The chemical composition of the pygidial gland secretions of five species of Carabini analysed by GC–FID and GC–MS.
3.2. Total amount of discharged pygidial gland secretions
The total amount of discharged pygidial gland secretion measured from one adult specimen of C. (P.) gigas was 2.494 ± 0.001 mg, while the total amount of the secretion of C. (C.) ulrichii obtained from nine adult individuals was 3.222 ± 0.001 mg (the average amount per specimen was 0.358 ± 0.001 mg).
The initial concentrations of the secretions dissolved in 0.01 M PBS used in the antimicrobial assay were as follows: 1 mg/ml [in C. (P.) gigas] and 1.343 mg/ml [in C. (C.) ulrichii].
All the measurements were repeated three times in order to confirm the validity of the results.
3.3. Antibacterial activity of the pygidial gland secretions of C. (P.) gigas, C. (C.) ulrichii and its most dominant chemicals
According to the obtained results, the tested pygidial gland secretions of the species C. (P.) gigas and C. (C.) ulrichii [subspecies C. (C.) ulrichii arrogans] showed a certain level of antimicrobial potential against most of the tested bacterial strains. Eleven tested bacterial strains showed different levels of susceptibility towards all the observed agents, whereby E. coli and MRSA proved to be the most resistant strains.
As determined in this study, the most dominant chemical component of the pygidial gland secretions of C. (P.) gigas and C. (C.) ulrichii was methacrylic acid, while angelic acid was found in significantly lower percentages in these two species (Table 1). Each of these two acids achieved a certain level of antimicrobial activity, with methacrylic acid showing a twice as strong effect in relation to all the observed bacteria. Tiglic acid, the second most dominant compound in the secretion of C. (C.) ulrichii, did not show any antimicrobial effect, so these results were not included in the study.
In relation to the secretion of C. (P.) gigas, P. aeruginosa, S. enterica, and S. typhimurium (MIC 0.3 ± 0.001 mg/ml) proved to be the most sensitive compared to the other tested strains (Table 2). Similarly, S. enterica and P. aeruginosa showed a certain level of susceptibility to methacrylic acid (MIC 0.375 ± 0.001 mg/ml), while the secretion of C. (C.) ulrichii achieved better antimicrobial potential against S. epidermidis, S. aureus, L. monocytogenes, and B. cereus (MBC 0.806 ± 0.001 mg/ml, MIC 0.403 ± 0.001 mg/ml) compared to all the tested bacteria (Table 2). In relation to angelic acid, K. pneumoniae proved to be the most sensitive strain (MIC 0.75 ± 0.001 mg/ml), while the remaining bacteria showed similar levels of susceptibility.
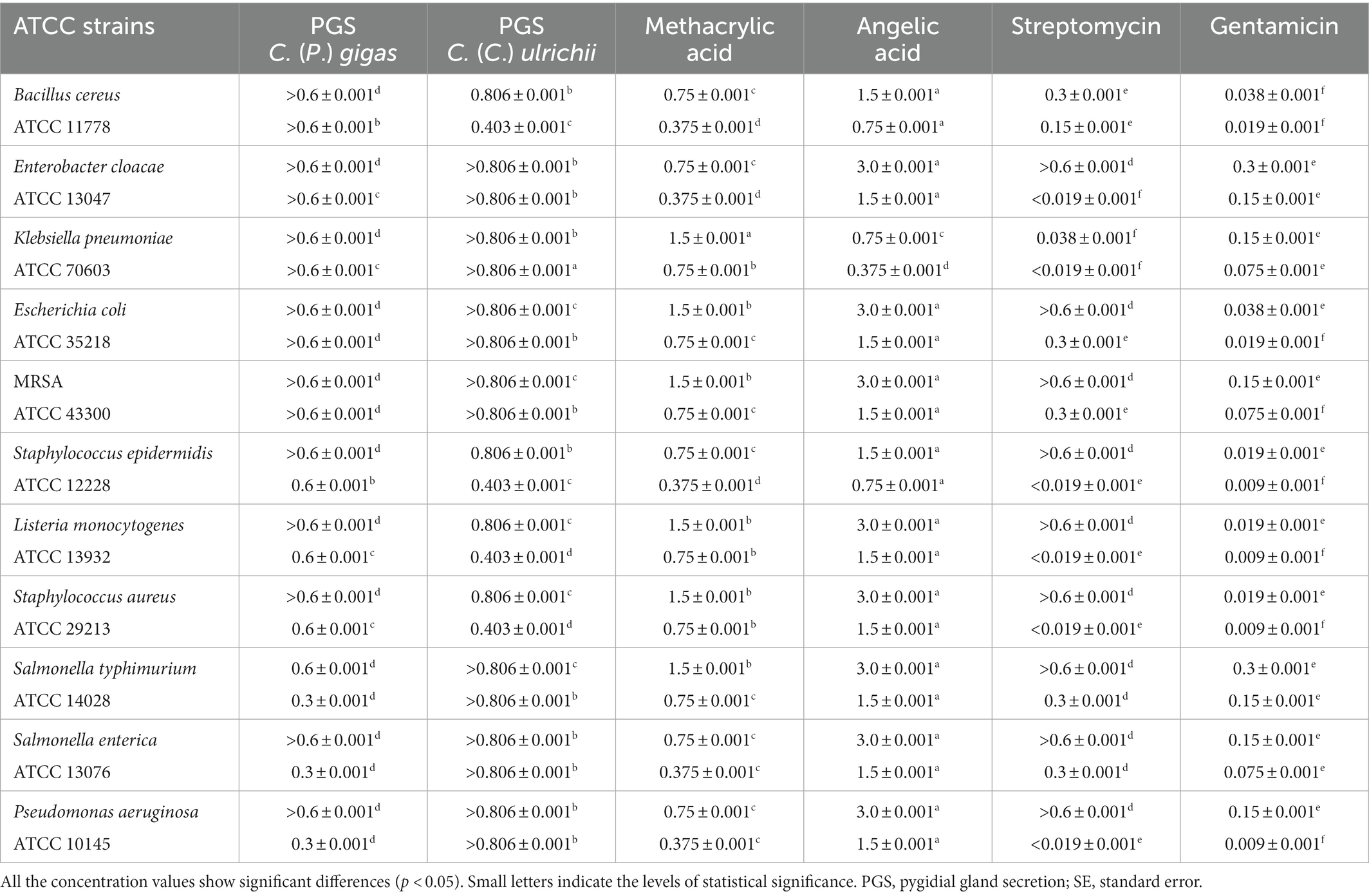
Table 2. The mean values of the minimum bactericidal concentrations (MBCs) and the minimum inhibitory concentrations (MICs) (mg/ml) of the pygidial gland secretions of C. (P.) gigas and C. (C.) ulrichii and of the standards of methacrylic and angelic acids compared to the positive controls (antibiotics streptomycin and gentamicin).
In general, the positive controls showed a better antibacterial effect compared to the tested agents except against S. typhimurium. In fact, streptomycin and the secretion of C. (P.) gigas achieved the same level of antibacterial potential against this bacterial strain (MIC 0.3 ± 0.001 mg/ml) (Table 2).
The secretion of C. (P.) gigas was shown to achieve the highest level of antibacterial activity against P. aeruginosa, S. enterica, and S. typhimurium compared to all the tested agents, including methacrylic acid.
3.4. Morphology of the pygidial glands
We analysed the pygidial gland morphology in five species of Carabidae: C. (C.) maderae, C. (C.) granulatus, C. (L.) clathratus, C. (C.) ulrichii, and C. (P.) gigas. No sexual dimorphism related to the size or structure of the pygidial glands was observed in the mentioned ground beetle species. As all five species belong to the same tribe (Carabini), their glandular components were relatively similar. The clusters of secretory lobes are tightly associated with numerous respiratory tracheal tubules (tracheoles). Secretion is produced in each secretory lobe, which consists of secretory cells radially arranged around duct-carrying cells, which surround a centrally positioned collecting lumen. Each reservoir is coated with a strong muscular exterior composed of well-developed muscle layers of different orientations and thicknesses. The listed features are common for all five species. However, there are some qualitative differences between the glands of the studied species, as well as certain differences in numeric and morphometric measurements.
The secretory part of each pygidial gland of C. (C.) maderae comprises a cluster or aggregation of 130–150 whitish spheroidal to ellipsoidal secretory lobes (Figure 2A). The diameter of each lobe is 100–130 μm in the minor axis and 120–160 μm in the major axis. Two or more adjacent secretory lobes often coalesce (Figure 2A). The collecting lumens of all the secretory lobes are connected via radial collecting canals (25–45 μm in diameter), which eventually merge into one main collecting canal. The width of the main collecting canal is up to 90 μm (its length was not measured since the canal was incomplete). It guides glandular products towards a reservoir. The reservoirs of the pygidial glands are elongated, wider in their anterior part, somewhat narrower in their posterior part. The maximum length of the reservoir is 3.09 mm, while its maximum width is 1.65 mm. Each reservoir is coated with a strong muscular exterior composed of well-developed muscle layers. Posteriorly, the reservoir continues into an efferent duct (Figure 2B). The efferent duct is 1.87 mm long and 0.13 mm wide. It ends with an opening valve.
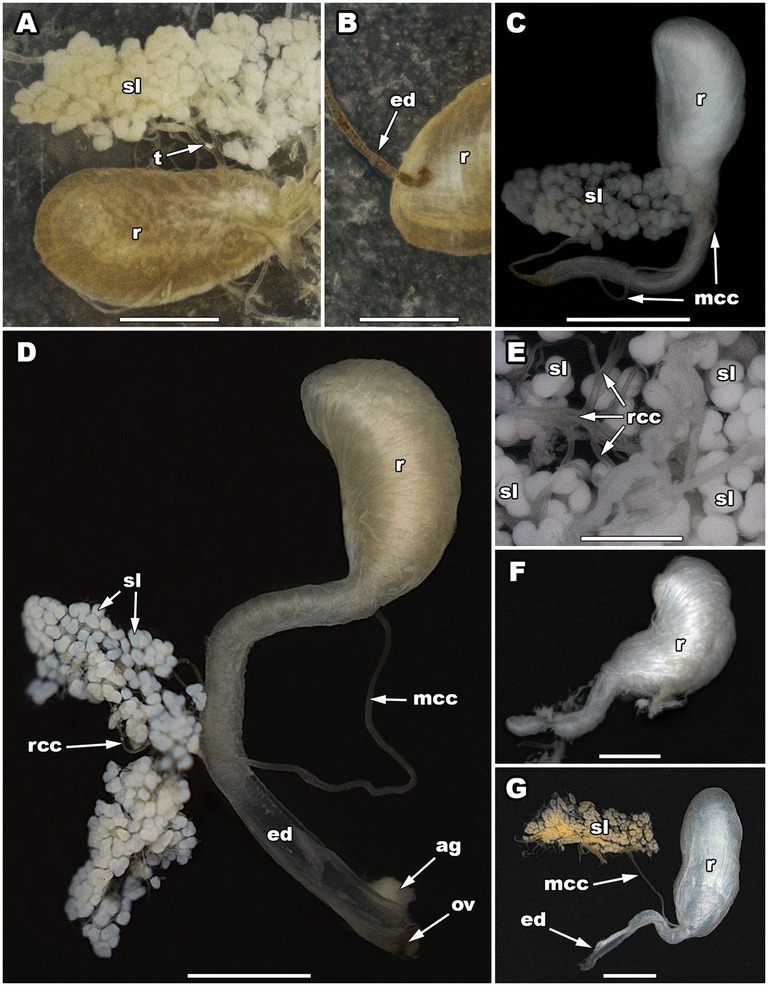
Figure 2. The pygidial gland morphology of five Carabini ground beetle taxa. The secretory lobes and reservoir of Calosoma (Calosoma) maderae (A), the connection of a main collecting canal with a reservoir in C. (C.) maderae (B), a pygidial gland of Carabus (Carabus) granulatus (C), a pygidial gland of Carabus (Carabus) ulrichii (D), a close-up of the secretory lobes of Carabus (Limnocarabus) clathratus (E), a reservoir of C. (L.) clathratus (F), a pygidial gland of Carabus (Procerus) gigas (G). ag, accessory gland; ed, efferent duct; mcc, main collecting canal; ov, opening valve; r, reservoir; rcc, radial collecting canal; sl, secretory lobe(s); t, tracheole. Scale bars: 0.5 mm (E), 1 mm (A–D,F), and 2 mm (G). Photos: N. Vesović.
The secretory part of each pygidial gland of C. (C.) granulatus consists of about 90 whitish spheroidal or ellipsoidal secretory lobes (Figure 2C). The diameter of each lobe is 80–100 μm in the minor axis and 90–120 μm in the major axis. Two or more adjacent secretory lobes frequently coalesce (Figure 2C). The collecting lumens of all the secretory lobes are connected via radial collecting canals (up to 40 μm in diameter), which eventually merge into one main collecting canal (Figure 2C). The main collecting canal is 4.13 mm long and 50 μm wide (the diameter of its lumen is 32 μm). It guides glandular products towards a reservoir. The main collecting canal enters the reservoir in the basal part of its concave region, near its posterior narrowed part. The reservoirs of the pygidial glands are bean-shaped in their anterior wider part, while cylindrical and narrowed in their posterior part (Figure 2C). The maximum length of the anterior part of the reservoir is 1.52 mm, while its maximum width is 0.72 mm. Posteriorly, the reservoir gradually narrows (its narrow section is 2.07 mm long and 0.19–0.27 mm wide) and continues into an efferent duct. The efferent duct is 1.65 mm long and 0.19–0.24 mm wide. It ends with an opening valve.
The secretory part of each pygidial gland of C. (C.) ulrichii consists of about 180–200 whitish spheroidal or ellipsoidal secretory lobes (Figure 2D). The diameter of each lobe is 80–90 μm in the minor axis and 100–140 μm in the major axis. Two or more adjacent secretory lobes often coalesce (Figure 2D). The collecting lumens of all the secretory lobes are connected via radial collecting canals (20–30 μm in diameter), which eventually merge into one main collecting canal (Figure 2D). The main collecting canal is 4.55–4.77 mm long and 70 μm wide (the diameter of its lumen is 40 μm). It guides glandular products towards a reservoir. The main collecting canal enters the reservoir in the basal part of its concave region, near its posterior narrowed part. The reservoirs of the pygidial glands are bean-shaped in their anterior wider part, while cylindrical and narrowed in their posterior part (Figure 2D). The maximum length of the anterior part of the reservoir is 1.91–2.03 mm, while its maximum width is 0.74–1.01 mm. Posteriorly, the reservoir gradually narrows (its narrow section is 2.24 mm long and 0.33–0.37 mm wide) and continues into an efferent duct. The efferent duct is 1.93–2.16 mm long and 0.33–0.37 mm wide (the diameter of its lumen is 0.18–0.22 mm). It ends with an opening valve. The opening valve is joined at its base by an accessory gland, which is 0.40 mm long and 0.28 mm wide. The gland assists secretion discharge.
The secretory part of each pygidial gland of C. (L.) clathratus consists of about 230 whitish spheroidal or ellipsoidal secretory lobes (Figure 2E). The diameter of each lobe is 100–140 μm in the minor axis and 110–160 μm in the major axis. Two or more adjacent secretory lobes frequently coalesce (Figure 2E). The collecting lumens of all the secretory lobes are connected via radial collecting canals (20–40 μm in diameter), which eventually merge into one main collecting canal. The main collecting canal is 4.88 mm long and 70 μm wide (the diameter of its lumen is 46 μm). It guides glandular products towards a reservoir. The main collecting canal enters the reservoir in the basal part of its concave region, near its posterior narrowed part. The reservoirs of the pygidial glands are bean-shaped in their anterior wider part, while cylindrical and narrowed in their posterior part (Figure 2F). The maximum length of the anterior part of the reservoir is 2.54 mm, while its maximum width is 1.38 mm. Posteriorly, the reservoir gradually narrows (its narrow section is 2.10 mm long and 0.52–0.74 mm wide) and continues into an efferent duct. The efferent duct is 2.20 mm long and 0.35 mm wide (the diameter of its lumen is 0.25 mm). It ends with an opening valve.
The secretory part of each pygidial gland of C. (P.) gigas consists of about 250 whitish spheroidal or ellipsoidal secretory lobes (Figure 2G). The diameter of each lobe is 140–230 μm in the minor axis and 170–270 μm in the major axis. Two or more adjacent secretory lobes often coalesce (Figure 2G). The collecting lumens of all the secretory lobes are connected via radial collecting canals (on average 30 μm in diameter), which eventually merge into one main collecting canal. The main collecting canal is 5.07–5.97 mm long and 80–90 μm wide (the diameter of its lumen is 52 μm). It guides glandular products towards a reservoir. The main collecting canal enters the reservoir in the basal part of its concave region, near its posterior narrowed part. The reservoirs of the pygidial glands are bean-shaped in their anterior wider part, while cylindrical and narrowed in their posterior part (Figure 2G). The maximum length of the anterior part of the reservoir is 3.66–5.57 mm, while its maximum width is 0.39–0.62 mm. Posteriorly, the reservoir gradually narrows (its narrow section is 2.06–2.57 mm long and 0.39–0.62 mm wide) and continues into an efferent duct. The efferent duct is 2.29–2.59 mm long and 0.31–0.52 mm wide (the diameter of its lumen is 0.20–0.28 mm). It ends with an opening valve, which is 0.60 mm wide.
4. Discussion
4.1. Chemical survey
Methacrylic acid was the most dominant compound in all the analysed secretion samples. The highest concentration of this acid was found in the pygidial gland secretion of C. (C.) ulrichii (83.58 and 85.18% in the females and males, respectively), while the lowest concentration was recorded in C. (C.) maderae (55.71 and 66.87% in the females and males, respectively). The recorded percentage variation of methacrylic acid is considered usual in the defensive secretions of ground beetles. Within Carabini, methacrylic acid is by far the most abundant compound (Schildknecht et al., 1964, 1968; Lečić et al., 2014; Vesović et al., 2017, 2020, 2022). Tiglic acid is typically present along with methacrylic acid in the pygidial gland secretions of ground beetles (Giglio et al., 2021). In the current study, tiglic acid was found in all the samples with the exception of the secretion of C. (P.) gigas. The absence of tiglic acid is occasionally detected among Carabini, but more commonly among various species of the subtribe Calosomatina (McCullough, 1969, 1972). Within the subtribe Carabina, only two species [Carabus (Tanaocarabus) taedatus Fabricius, 1787 and C. (Hemicarabus) tuberculosus Dejean, 1829] lacked tiglic acid in their defensive secretions (Benn et al., 1973; Kanehisa and Murase, 1977). A significantly lower percentage of this compound was detected in C. (C.) maderae (3.48 and 3.83% in the females and males, respectively). The remaining secretion samples showed similar concentrations of tiglic acid. On the other hand, angelic acid was detected in all the analysed samples, with the highest prevalence in C. (P.) gigas (18.5–29.5%). A relatively high percentage of this compound was also recorded in C. (L.) clathratus (7.8%). The remaining samples, however, showed a very low relative percentage of this carboxylic acid. It is fairly common for tiglic acid to be more abundant than angelic acid. The opposite case has been found to occur only in three taxa of Carabini [Carabus (Chaetocarabus) intricatus Linnaeus, 1760, C. (C.) ulrichii rhilensis and C. (Megodontus) violaceus Linnaeus, 1758] (Lečić et al., 2014; Vesović et al., 2020, 2022).
Benzoic acid was detected in the secretions of C. (C.) maderae (3.23 and 2.65% in the females and males, respectively) and C. (L.) clathratus (4.2%). This acid was previously recorded in Carabini. Namely, it was detected in the defensive secretions of Calosoma (Calosoma) sycophanta (Linnaeus, 1758) and a few Carabus taxa. This chemical is considered a minor constituent of the secretions of Carabus spp., in contrast with the secretions of Calosoma spp., where a slightly higher percentage was recorded (Lečić et al., 2014; Vesović et al., 2017, 2020). The relative percentage of benzoic acid in C. (C.) maderae is in line with the previous statement, whilst its abundance in C. (L.) clathratus was greater than expected. Benzoic acid was recently recorded in the pygidial gland secretions of three species of the genus Ceroglossus Solier, 1848 (Xu et al., 2019). 2-Methylbutyric acid was found in the secretions of C. (C.) granulatus and C. (L.) clathratus (with 1.57 and 0.3%, respectively). Isobutyric and butyric acids were both present only in the defensive secretion of C. (L.) clathratus as its minor constituents (with 0.2 and 0.1%, respectively). The combined presence of these three acids is not rare within Carabini, or within ground beetles in general (Giglio et al., 2021). However, these acids do not seem to be widely distributed within Carabini. To date, their presence has only been reported in several Carabini taxa (Kanehisa and Kawazu, 1982; Lečić et al., 2014; Vesović et al., 2020, 2022). In addition, when present, the acids were most often detected in small or even minor amounts. Most of those records are reported in relatively recent studies (Lečić et al., 2014; Vesović et al., 2020, 2022). Furthermore, although several species of Carabini [C. (C.) sycophanta, C. (C.) intricatus, C. (C.) ulrichii, and C. (M.) violaceus] were previously analysed in terms of chemical ecology, none of these three acids was detected (Schildknecht and Weis, 1962; Schildknecht et al., 1964; Casnati et al., 1965). The same can be noted for benzoic acid, which was not detected in initial investigations, but was found in more recent studies (Schildknecht et al., 1964; Casnati et al., 1965; Lečić et al., 2014; Vesović et al., 2017, 2020). In that regard, it may be possible that a significantly wider presence of the aforementioned compounds in the defensive secretions of Carabini was left undetected due to the limitations of the instruments used in the past. Carboxylic acids represent allomonically active compounds in the secretions of Carabini, as in other arthropods. Their sharp odour, along with high volatility, make them efficient deterrents of various predators (Blum, 1981).
Within Carabini, the presence of salicylaldehyde is characteristic of the defensive secretions of the members of the subtribe Calosomatina, clearly distinguishing that group from the representatives of another subtribe – Carabina (Eisner et al., 1963; Kanehisa and Murase, 1977; Lečić et al., 2014). The defensive properties of salicylaldehyde were tested in detail by Eisner et al. (1963), who showed that its producers exhibit great effectiveness in predator avoidance. The compound was found in the secretion extracts of C. (C.) maderae (35.49 and 24.46% in the females and males, respectively). Salicylaldehyde always constitutes a significant part of the pygidial gland secretions of Calosomatina. In our study, it was the second most dominant compound (methacrylic acid was the most dominant) in the secretion of C. (C.) maderae. Nevertheless, in other studies, its dominance over methacrylic acid was also reported (Moore and Wallbank, 1968; Kanehisa and Murase, 1977). Interestingly, according to the study carried out by McCullough and Weinheimer (1966), the defensive secretion of Calosoma (Calodrepa) scrutator (Fabricius, 1781) lacked salicylaldehyde. Up to this point, it has been the only exception to the rule. In the defensive secretion of C. (C.) maderae the alkene (Z)-9-tricosene was found as its minor constituent (with 0.78 and 0.96% in the females and males, respectively). The only other hydrocarbon detected in our study was n-tricosane, which was present in the defensive secretion of C. (L.) clathratus in minor amounts (0.1%). Both hydrocarbons are very rare in the defensive secretions of ground beetles (Attygalle et al., 1992; Bonacci et al., 2011; Vranić et al., 2020). Hydrocarbons have not been previously recorded in the pygidial gland secretions of representatives of the tribe Carabini. Within the whole subfamily Carabinae, another alkene, 11-tricosene, was documented in the defensive secretions of Ceroglossus buqueti (Laporte de Castelnau, 1834) and C. chilensis (Eschscholtz, 1829) (Xu et al., 2019). Hydrocarbons, as non-polar compounds, are generally thought to act as penetration facilitators of active compounds through the lipophilic cuticle of predators (Blum, 1981).
In this paper, we provide first data on the chemistry of the pygidial gland secretions of four ground beetle taxa: C. (C.) maderae, C. (C.) ulrichii arrogans, C. (L.) clathratus, and C. (P.) gigas. Schildknecht et al. (1964) first examined the defensive secretion of C. (C.) ulrichii and reported only the presence of methacrylic and tiglic acids. Later, Lečić et al. (2014) investigated the defensive secretion of the subspecies C. (C.) ulrichii rhilensis, reporting the presence of seven carboxylic acids: 2-methylbutyric, angelic, benzoic, butyric, isobutyric, methacrylic and tiglic. Interestingly, in the study of Lečić et al. (2014), angelic acid was the second most dominant compound, which is generally rare among Carabini ground beetles. Angelic and tiglic acids are a pair of cis-trans isomers. It is known that their abundance can vary, with tiglic acid typically being more prevalent. However, in the defensive secretion of C. (C.) ulrichii rhilensis, the opposite was observed. Our study on the related C. (C.) ulrichii arrogans showed a typical arrangement, with tiglic acid prevalent over angelic acid. This may either be as a result of subspecific differences or even of differences in food sources, which might have had an impact on the physiological pathways of certain individuals. Furthermore, C. (C.) ulrichii arrogans had a much simpler secretion mixture, lacking most of the acids present in C. (C.) ulrichii rhilensis.
Schildknecht et al. (1964) previously examined the defensive secretion of C. (C.) granulatus. The authors detected only the presence of methacrylic and tiglic acids. In our study, we found two additional acids: 2-methylbutyric and angelic. Also, the amounts of methacrylic and tiglic acids differed slightly than those in the former study (81.81 and 15.43%, respectively vs. 93.7 and 6.3%, respectively).
We did not detect any significant differences in the defensive secretions between genders in C. (C.) ulrichii and C. (C.) maderae. To be more precise, minor quantitative differences were observed, probably due to the different metabolic status of the tested individuals. If we compare our findings on the defensive secretion of C. (C.) maderae with the recently studied C. (C.) sycophanta, the former species had a much simpler secretion mixture, with a smaller number of carboxylic acids (angelic, benzoic, methacrylic, and tiglic) compared to the latter species, which contained six additional acids (2-methylbutyric, butyric, crotonic, isobutyric, propionic, and senecioic), but lacked angelic acid (Lečić et al., 2014). Salicylaldehyde was present in both species, but in slightly lower amounts in C. (C.) maderae compared to C. (C.) sycophanta (24.46–35.49% vs. 42.70%). Finally, the secretion of C. (C.) maderae included (Z)-9-tricosene, a previously unregistered compound within the genus, as well as within the whole subfamily Carabinae. A comparison between the pygidial gland secretions of C. (C.) maderae and other chemoecologically tested species of the same subtribe might not be appropriate at the moment. As mentioned before, investigations of the defensive secretions of the aforementioned taxa were carried out a long time ago and the lower sensitivity of the former techniques is cause for doubt in terms of data completeness. However, it is interesting to note that two species inhabiting Australia and New Caledonia, namely Calosoma (Australodrepa) oceanicum Perroud & Montrouzier, 1864 and C. (A.) schayeri Erichson, 1842, both produce hexanoic acid, a compound that has not been detected in the defensive secretions of other representatives of the subfamily Carabinae thus far (Moore and Wallbank, 1968). Since both species belong to the subgenus Australodrepa Lapouge, 1929, the distribution of the mentioned acid might be limited to the representatives of this subgenus. Many species of the genus Calosoma [C. (Callitropa) externum Say, 1823, C. (C.) macrum LeConte, 1853, C. (Camedula) parvicolle Fall, 1910, C. (C.) peregrinator Guérin-Méneville, 1844, C. (C.) prominens LeConte, 1853, C. (Castrida) alternans Fabricius, 1792, and C. (Chrysostigma) affine Chaudoir, 1843] seem to produce salicylaldehyde as the sole product of their defensive glands (Eisner et al., 1963; McCullough, 1966, 1969; McCullough and Weinheimer, 1966). Calosoma (C.) scrutator is thought to produce only methacrylic acid. In addition to salicylaldehyde, Calosoma (Calosoma) chinense Kirby, 1819 also had a mixture of methacrylic and tiglic acids, which is characteristic of Carabinae (Kanehisa and Murase, 1977). The only chemoecologically analysed species of the genus Callisthenes Fabricius, 1775 [C. luxatus (Say, 1823)] and Calosoma (Camegonia) marginale Casey, 1897 both produced methacrylic acid in addition to salicylaldehyde (McCullough and Weinheimer, 1966; McCullough, 1972). Tiglic acid appears to be rare in the defensive secretions of Calosomatina. To date, it has been found in the secretions of C. (C.) chinense, C. (C.) maderae and C. (C.) sycophanta (Kanehisa and Murase, 1977; Lečić et al., 2014). It is important to note that minor and trace compounds in the secretion of the majority of the analysed Calosomatina may still remain undetected. Further analyses and re-analyses of the previously investigated taxa are necessary in order to gain insight into the defensive secretion complexity of Calosomatina.
Out of all the studied taxa, C. (P.) gigas had the simplest defensive secretion mixture, composed only of two carboxylic acids. Its adult specimens were sampled at two locations. The secretions of individuals from both populations were qualitatively identical. In contrast, specimens of distant populations of species within the genus Carabus Linnaeus, 1758 are known to exhibit certain qualitative variations in their defensive secretions (Vesović et al., 2022). The relative percentages of compounds in the two samples of C. (P.) gigas appear to be marginally different. However, we tested only female individuals. The combination of methacrylic and angelic acids alone has not been previously recorded in ground beetles. Moreover, when present, angelic acid is frequently found along with tiglic acid (Giglio et al., 2021). The exact distribution patterns and the alternation in the prevalence of angelic and tiglic acids are yet to be examined. Although some studies of Cychrini ground beetles suggest that the otherwise absent tiglic acid can be produced under specific circumstances (Wheeler et al., 1970), a larger-scale study is necessary to clarify the factors involved in the qualitative and quantitative fluctuations of the pygidial gland secretions of ground beetles.
In our study, the most complex chemical mixture was detected in the pygidial gland secretion of C. (L.) clathratus. Apart from methacrylic, tiglic and angelic acids, which were the most dominant compounds, a relatively high relative percentage (4.2%) of benzoic acid was also detected. Out of all the Carabus species chemically tested to date, C. (L.) clathratus had the highest relative percentage of benzoic acid (Lečić et al., 2014; Vesović et al., 2017, 2020). Aside from that, three carboxylic acids (2-methylbutyric, isobutyric, and butyric) and one alkane (n-tricosane) were present as the minor constituents of the secretion mixture. These three carboxylic acids are commonly found in lesser amounts in ground beetles, so our findings are consistent with the literature (Schildknecht et al., 1968; Kanehisa and Kawazu, 1982; Vesović et al., 2020). Interestingly, alkanes were not previously found in the defensive secretions of any species of the genus Carabus.
The representatives of the tribe Carabini mainly produce carboxylic acids in their pygidial glands (Lečić et al., 2014; Vesović et al., 2017, 2020, 2022; Giglio et al., 2021). Considering the subtribe Carabina, n-tricosane (detected in the present study) and n-butanol (Vesović et al., 2022) are the only exceptions to the rule. Apart from that, all of the investigated species are characterised by the presence of various mixtures of carboxylic acids. To date, 11 different carboxylic acids have been isolated from the pygidial gland secretion extracts of Carabina: 2-methylbutyric, 2-hexenoic, acetic, angelic, benzoic, butyric, ethacrylic, isobutyric, methacrylic, senecioic, and tiglic (Vesović et al., 2020, 2022). With the exception of 2-hexenoic acid, which was detected only in Carabus (Megodontus) caelatus Fabricius, 1801, the other acids have a wider distribution within the group (Vesović et al., 2020). Ethacrylic acid seems to be characteristic of the North American and Japanese taxa of Carabini (Benn et al., 1973; Kanehisa and Murase, 1977). The representatives of Calosomatina are primarily distinguished by the presence of salicylaldehyde, but also crotonic, hexanoic, and propionic acids. Nonetheless, since only a limited number of species of Carabini have been chemoecologically tested to date, it would be inappropriate to draw any conclusions on the absence of the acids from the secretions of either groups.
Despite indications that some substances from the pygidial gland secretions of ground beetles can be used in taxonomy, the lack of information in that field currently makes the presence of allomones and the usage of other semiochemical characters in chemotaxonomy unreliable. Within Carabini, it is well known that salicylaldehyde is exclusively produced by the representatives of the subtribe Calosomatina (Vesović et al., 2020), so its presence might serve as a chemotaxonomic character. On the other hand, the same compound was detected in the species of the subfamilies Oodinae, Pterostichinae and Trechinae of Carabidae, which means that its importance in chemotaxonomy is limited to the subfamily level (Moore and Wallbank, 1968; Schildknecht et al., 1968; Moore, 1979; Will et al., 2000). At the same time, the presence/absence of certain pygidial gland secretion chemicals is unlikely to have any value as a character for distinguishing lower taxa because the active chemicals of the secretions are almost always registered in a large number of related species and even in unrelated ones. Future investigations focused on establishing the definitive distribution of defensive compounds may provide greater insight into the chemotaxonomic value of the mentioned characters.
The groups of compounds from the pygidial gland secretions of ground beetles which have confirmed defensive, repellent and antiseptic features are carboxylic acids, phenols, alcohols, quinones and aldehydes (Kanehisa and Murase, 1977; Blum, 1981). On the other hand, some other groups of neutral compounds, such as hydrocarbons and esters, are also usually found in the secretions in minor concentrations and are considered to have the roles of solvents and carriers of the active components of the secretions (Kanehisa and Murase, 1977). It has also been shown that some compounds from these classes may synergistically participate in the defensive and/or repellent properties of the secretions (Kanehisa and Kawazu, 1985). Based on the above, it is our assumption that the carboxylic acids (isobutyric, butyric, 2-methylbutyric, methacrylic, angelic, tiglic, and benzoic) and aldehydes (salicylaldehyde) registered in the species of Carabini analysed in this study play the role of allomones, while the detected hydrocarbons [n-tricosane and (Z)-9-tricosene] take on the presumed role of solvents and carriers of carboxylic acids as active components of the secretions. Thus, the carboxylic acids and aldehydes found in the studied Carabini species could have a presumed role in the defence against predators and microbes in their environment, so they can be said to represent antipredator and/or antimicrobial agents. Salicylaldehyde has been shown to play a role in the defence of Calosomatina against predators (Eisner et al., 1963) and it possesses pronounced antifungal potential (Pelttari et al., 2011; Nenadić et al., 2017b). On the other hand, the antibacterial and antifungal properties of the secretions of the species of the subtribe Carabina, which mainly contain carboxylic acids, have been proven (Nenadić et al., 2016a). In other groups of insects, it was noted that the secretions with a dominant allomonic role often have the simultaneous function of an intraspecific signal – an alarm pheromone (Vesović, 2019). Future studies on the components of the pygidial gland secretions of Carabini will reveal their role and clarify whether they are allomonic compounds, solvents/carriers, with a role in sexual communications (Holliday et al., 2016) or perhaps it is a combination of these functions.
4.2. Antimicrobial survey
Recently, several studies have reported the existence of antimicrobial activity in the pygidial gland secretions of some ground beetle species, indicating that different organic compounds, such as short-chain fatty acids and salicylaldehyde, could be responsible for the antimicrobial potential of these gland products (Nenadić et al., 2016a,b, 2017a,b; Nenadić, 2019; Dimkić et al., 2020; Vesović et al., 2022).
In this study, we showed that the pygidial gland secretions of C. (P.) gigas and C. (C.) ulrichii arrogans possess a certain level of antibacterial potential against the majority of the tested laboratory strains of clinical bacterial pathogens (MIC in the range of 0.3–0.403 mg/ml). Although the antimicrobial properties of the pygidial gland secretions of some species of the genus Carabus have already been investigated (Nenadić et al., 2016a; Nenadić, 2019; Vesović et al., 2022), we provided data on the antimicrobial activity of the secretions for an additional two taxa.
Out of the taxa of the genus Carabus whose secretions were analysed for antimicrobial potential, C. (C.) ulrichii rhilensis, C. (C.) intricatus and C. (P.) gigas contain methacrylic and angelic acids in their defensive pygidial gland secretions in quite similar relative percentages, with the dominance of methacrylic acid. In C. (P.) coriaceus Linnaeus, 1758, no angelic acid was detected (Nenadić et al., 2016a; Vesović et al., 2022). Similar to most species analysed so far, the secretion of C. (C.) ulrichii arrogans possesses only 0.87% of angelic acid in its mixture, as determined in this study. Interestingly, regardless of the similar relative percentages of the aforementioned chemical constituents of the secretions, three gram-positive (B. cereus, S. aureus, and L. monocytogenes) and three gram-negative bacterial strains (E. coli, S. typhimurium, and P. aeruginosa) varied in their susceptibility to all the tested pygidial gland secretions (Nenadić et al., 2016a; Vesović et al., 2022), but also to methacrylic and angelic acids (Vesović et al., 2022). Although it has been previously revealed that organic acids, such as formic, acetic, and other short-chain fatty acids, could be associated with antimicrobial activity (Glassman, 1948; Kabara et al., 1972; Desbois and Smith, 2010; Raftari et al., 2012; Nenadić et al., 2016a,b; Nenadić, 2019), there is a particular lack of data on the antimicrobial properties of methacrylic and angelic acids. It has been determined that angelic acid is a potent antioxidant (Varsha et al., 2015; VasudhaUdupa et al., 2021) with assumed antimicrobial potential (Vesović et al., 2022).
On the other hand, Tao et al. (2012) revealed that methacrylic acid does not possess antibacterial properties unless it is coupled with TiO(2)/PMMA denture base resin. However, both in our study and that carried out by Vesović et al. (2022) methacrylic acid was shown to achieve a certain level of antimicrobial activity against different gram-negative and gram-positive bacterial strains. The MIC values of methacrylic acid we obtained were twice as low as the MIC values of angelic acid, which represents a difference in relation to the results of Vesović et al. (2022), where the MBC and MIC values were up to 10 times lower. Although methacrylic acid was reported as the dominant chemical of the secretion of C. (P.) coriaceus (with 79.2%), with P. aeruginosa (ATCC 27853) proving to be the most resistant to this acid (MIC 25.5 mg/ml) among all the tested bacterial strains (Nenadić et al., 2016a), the secretion of C. (P.) gigas with a similar percentage share of this acid (76%) showed almost 100 times higher antimicrobial potential against the same bacterium, but a different laboratory strain (ATCC 10145) (MIC 0.3 mg/ml). In general, P. aeruginosa is recognised as one of the most resistant bacteria towards various antibiotics, including aminoglycosides, quinolones and β-lactams (Hancock and Speert, 2000; Pang et al., 2019). In this study, this bacterium proved to be resistant to streptomycin (MBC > 0.6 mg/ml), but it is interesting to mention that methacrylic acid also achieved a bactericidal effect (MBC 0.75 mg/ml). Similarly, S. enterica also showed a certain level of susceptibility to methacrylic acid (MIC 0.375 mg/ml, MBC 0.75 mg/ml).
We showed that the secretion of C. (P.) gigas achieved the highest level of antibacterial activity against S. enterica, S. typhimurium, and P. aeruginosa compared to all the tested agents, including methacrylic acid. Comparing our results with previous reports (Nenadić et al., 2016a; Vesović et al., 2022), it seems that C. (P.) gigas accomplished the highest level of antimicrobial activity against the abovementioned bacteria in relation to all other congeners and tested agents.
It can be noted from the comparison of the antimicrobial effect of the pygidial gland secretion of C. (P.) gigas with that of the secretions of previously examined Carabus species that the secretion of C. (P.) gigas (MIC in the range of 0.3–0.6 mg/ml) has the strongest antibacterial potential, achieving almost the same level of antibacterial activity as the secretion of C. (C.) intricatus (MIC in the range of 0.301–0.604 mg/ml) (Nenadić, 2019; Vesović et al., 2022). In relation to the secretion of the subspecies C. (C.) ulrichii rhilensis (Nenadić et al., 2016a), the secretion of C. (C.) ulrichii arrogans showed better antimicrobial properties, achieving MIC values in the range of 0.403–0.806 mg/ml. According to Nenadić et al. (2016a), S. aureus, L. monocytogenes, and S. typhimurium showed higher levels of susceptibility to the secretion of C. (C.) ulrichii rhilensis (MIC 51.986 mg/ml) compared to the other bacterial strains tested in this study (B. cereus, Micrococcus flavus, P. aeruginosa, E. coli, and E. cloacae), while the positive controls ampicillin and streptomycin proved to have stronger antibacterial potential. Similarly, in the present study, all the tested bacteria showed higher susceptibility to the positive controls streptomycin (MIC in the range of 0.019–0.3 mg/ml) and gentamicin (MIC in the range of 0.009–0.15 mg/ml), with the exception of S. enterica, which proved to be equally sensitive to the secretion of C. (P.) gigas and streptomycin (MIC 0.3 mg/ml).
In general, the variation in the levels of the antimicrobial potential of the observed secretions, despite their similar chemical composition (Nenadić et al., 2016a; Nenadić, 2019; Vesović et al., 2022), probably stems from the different percentage share of their constituents and the synergistic action of some or all of the organic components occurring in the secretions.
Studies dealing with the antimicrobial potential of the pygidial gland secretions of ground beetles could be promising due to the low level of cytotoxicity of these chemical mixtures originating from different ground beetle species. The pygidial gland secretions of C. (C.) ulrichii and C. (P.) coriaceus inhibited porcine liver primary cell proliferation in quite low percentages (Nenadić et al., 2018).
According to the results obtained in this study, it may be stated that the pygidial gland secretions of C. (P.) gigas and C. (C.) ulrichii arrogans exhibit previously assumed antimicrobial activity against certain bacterial strains in addition to their defensive role against predators, which indicates their possible application in biomedical and pharmacological research.
4.3. Morphological survey
A comparison of the morphological elements of the pygidial glands between the genera Calosoma Weber, 1801 and Carabus shows that despite there being a common general structure (the reservoirs are of similar dimensions relative to the body size, their lumen and structure are similar, as well as the thickness of the muscle coat), it is still possible to point out some differences. The epicuticular spines directed towards the lumen of the reservoir are significantly more strongly developed in the genus Calosoma than in Carabus (Vesović, 2019). The reservoirs of the species of the genus Calosoma are more curved and reniform. A sharp narrowing transition of the reservoir into the efferent duct can be clearly seen in Calosoma, in contrast to the species of the genus Carabus, where this transition is gradual (Vesović, 2019). Moreover, the secretory lobes of Calosoma spp. show a greater tendency to fuse with each other than in Carabus spp.
Considering the pygidial gland morphology of the genus Calosoma, only three species have been analysed in greater detail to date. These are previously examined C. (C.) inquisitor and C. (C.) sycophanta (Casnati et al., 1965; Nenadić et al., 2017a,b; Vesović, 2019), as well as C. (C.) maderae analysed in this study, all three species native to the Palaearctic. In addition to these three species, the African species Calosoma (Ctenosta) senegalense Dejean, 1831 was also mentioned, but with very limited information (Forsyth, 1972). The pygidial gland structure of C. (C.) maderae is similar to the morphology of the other two congeneric species [C. (C.) inquisitor and C. (C.) sycophanta], but with one significant difference – the reservoir narrows even more abruptly into the efferent duct (this observation may be to some extent affected by the fact that the pygidial glands were kept in 70% ethanol before examination, which could lead to shrinkage due to dehydration). Although Forsyth (1972) reported that the number of secretory lobes in Calosoma was significantly greater than that of Carabus, we did not confirm this statement in our study (out of four Carabus species, three had more secretory lobes in the cluster than Calosoma species have).
The pygidial gland morphology of representatives of the subgenera Limnocarabus Géhin, 1876 and Procerus Dejean, 1821 of the genus Carabus were previously unexplored. The morphological features of the glandular components (the presence of the elongated, unilobal reservoir with a slight median constriction, the shape and structure of the secretory lobes, the appearance of the main collecting canal and the efferent duct, as well as their attachments to the reservoir) of Carabus taxa experimentally obtained in this study were similar to those of the closely related congeners belonging to different subgenera previously investigated (Forsyth, 1972; Kanehisa and Shiraga, 1978; Nenadić et al., 2016a; Vesović et al., 2017, 2020, 2022).
Given the small number of species of the genera Calosoma and Carabus whose pygidial glands have been analysed morphologically to date (Casnati et al., 1965; Forsyth, 1972; Vesović, 2019; Vesović et al., 2020, 2022) and the considerable morphological variability of the glands, it seems that at the moment no significant taxonomic conclusions can be drawn based on the structure of these glands (Forsyth, 1972; Vesović et al., 2017, 2020). Considering the phylogenetic relationships within the tribe Carabini, the general morphology of pygidial glands at the subtribe level does not provide a great deal of information (Forsyth, 1972).
Data availability statement
The original contributions presented in the study are included in the article/supplementary material, further inquiries can be directed to the corresponding author/s.
Author contributions
NV: conceptualization. NV, MN, LV, MT, ID, and TJ: methodology. NV and MN: software. SĆ and LV: validation. NV and NS: fieldwork investigation. NV: writing – original draft preparation and visualization. SĆ, LV, MN, and SV: writing – review and editing. SĆ: supervision. SĆ, NĆ, DV, and LM: project administration. KM: funding acquisition. All authors contributed to the article and approved the submitted version.
Funding
This study was supported by the Serbian Ministry of Science, Technological Development and Innovation (contracts nos. 451-03-47/2023-01/200178, 451-03-47/2023-01/200168, and 451-03-47/2023-01/200172).
Acknowledgments
We thank Dalibor Stojanović (University of Belgrade - Faculty of Biology, Belgrade, Serbia) and Marija Vasović (Belgrade, Serbia) for collecting some of the insect samples.
Conflict of interest
The authors declare that the research was conducted in the absence of any commercial or financial relationships that could be construed as a potential conflict of interest.
Publisher’s note
All claims expressed in this article are solely those of the authors and do not necessarily represent those of their affiliated organizations, or those of the publisher, the editors and the reviewers. Any product that may be evaluated in this article, or claim that may be made by its manufacturer, is not guaranteed or endorsed by the publisher.
References
Andrews, J. M. (2001). Determination of minimum inhibitory concentrations. J. Antimicrob. Chemother. 48, 5–16. doi: 10.1093/jac/48.suppl_1.5
Aneshansley, D. J., Eisner, T., Widom, J. M., and Widom, B. (1969). Biochemistry at 100°C: explosive secretory discharge of bombardier beetles (Brachinus). Science 165, 61–63. doi: 10.1126/science.165.3888.61
Attygalle, A. B., Meinwald, J., and Eisner, T. (1992). Defensive secretion of a carabid beetle, Helluomorphoides clairvillei. J. Chem. Ecol. 18, 489–498. doi: 10.1007/BF00994247
Attygalle, A. B., Meinwald, J., Liebherr, J. K., and Eisner, T. (1991). Sexual dimorphism in the defensive secretion of a carabid beetle. Experientia 47, 296–299. doi: 10.1007/BF01958165
Barnes, K. M., Gennard, D. E., and Dixon, R. A. (2010). An assessment of the antibacterial activity in larval excretion/secretion of four species of insects recorded in association with corpses, using Lucilia sericata Meigen as the marker species. Bull. Entomol. Res. 100, 635–640. doi: 10.1017/S000748530999071X
Benn, M. H., Lencucha, A., Maxie, S., and Telang, S. A. (1973). The pygidial defensive secretion of Carabus taedatus. J. Insect Physiol. 19, 2173–2176. doi: 10.1016/0022-1910(73)90132-7
Blum, M. S. (1996). Semiochemical parsimony in the Arthropoda. Annu. Rev. Entomol. 41, 353–374. doi: 10.1146/annurev.en.41.010196.002033
Bonacci, T., Brandmayr, P., Dalpozzo, R., De Nino, A., Massolo, A., Tagarelli, A., et al. (2008). Odour and colour similarity in two species of gregarious carabid beetles (Coleoptera) from the Crati Valley, southern Italy: a case of Müllerian mimicry? Entomol.News 119, 325–337. doi: 10.3157/0013-872X-119.4.325
Bonacci, T., Brandmayr, P., Zetto, T., Perrotta, I. D., Guarino, S., Peri, E., et al. (2011). Volatile compounds released by disturbed and undisturbed adults of Anchomenus dorsalis (Coleoptera, Carabidae, Platynini) and structure of the pygidial gland. ZooKeys 81, 13–25. doi: 10.3897/zookeys.81.1122
Carcamo, E., Shyam, S., Banerjee, S., Gnanamani, E., Mendoza-Trujillo, M., Mata-Espinosa, D., et al. (2019). 1,4-Benzoquinone antimicrobial agents against Staphylococcus aureus and Mycobacterium tuberculosis derived from scorpion venom. Proc. Natl. Acad. Sci. U. S. A. 116, 12642–12647. doi: 10.1073/pnas.1812334116
Casnati, G., Pavan, M., and Ricca, A. (1965). Sulla costituzione del veleno dell’insetto Calosoma sycophanta L. (Coleoptera Carabidae). Ann. Soc. Entomol. Fr. (N. S.) 1, 705–710.
Dean, J., Aneshansley, D. J., Edgerton, H. E., and Eisner, T. (1990). Defensive spray of the bombardier beetle: a biological pulse jet. Science 248, 1219–1221. doi: 10.1126/science.2349480
Degenkolb, T., Düring, R. A., and Vilcinskas, A. (2011). Secondary metabolites released by the burying beetle Nicrophorus vespilloides: chemical analyses and possible ecological functions. J. Chem. Ecol. 37, 724–735. doi: 10.1007/s10886-011-9978-4
Desbois, A. P., and Smith, V. J. (2010). Antibacterial free fatty acids: activities, mechanisms of action and biotechnological potential. Appl. Microbiol. Biotechnol. 85, 1629–1642. doi: 10.1007/s00253-009-2355-3
Di Giulio, A., Muzzi, M., and Romani, R. (2015). Functional anatomy of the explosive defensive system of bombardier beetles (Coleoptera, Carabidae, Brachininae). Arthropod Struct. Dev. 44, 468–490. doi: 10.1016/j.asd.2015.08.013
Dimkić, I., Stanković, S., Kabić, J., Stupar, M., Nenadić, M., Ljaljević-Grbić, M., et al. (2020). Bat guano-dwelling microbes and antimicrobial properties of the pygidial gland secretion of a troglophilic ground beetle against them. Appl. Microbiol. Biotechnol. 104, 4109–4126. doi: 10.1007/s00253-020-10498-y
Eisner, T., Jones, T. H., Aneshansley, D. J., Tschinkel, W. R., Silberglied, R. E., and Meinwald, J. (1977). Chemistry of defensive secretions of bombardier beetles (Brachinini, Metriini, Ozaenini, Paussini). J. Insect Physiol. 23, 1383–1386. doi: 10.1016/0022-1910(77)90162-7
Eisner, T., Swithenbank, C., and Meinwald, J. (1963). Defense mechanisms of arthropods. VIII. Secretion of salicylaldehyde by a carabid beetle. Ann. Entomol. Soc. Am. 56, 37–41. doi: 10.1093/aesa/56.1.37
Forsyth, D. J. (1968). The structure of the defence glands in the Dytiscidae, Noteridae, Haliplidae and Gyrinidae (Coleoptera). Trans. Zool. Soc. London 120, 159–181. doi: 10.1111/j.1365-2311.1968.tb00352.x
Forsyth, D. J. (1970). The structure of the defence glands of the Cicindelidae, Amphizoidae, and Hygrobiidae (Insecta: Coleoptera). J. Zool. 160, 51–69. doi: 10.1111/j.1469-7998.1970.tb02897.x
Forsyth, D. J. (1972). The structure od pygidial defence glands of Carabidae (Coleoptera). Trans. Zool. Soc. London 32, 249–309. doi: 10.1111/j.1096-3642.1972.tb00029.x
Francke, W., and Dettner, K. (2005). Chemical signalling in beetles. Top. Curr. Chem. 240, 85–166. doi: 10.1007/b98316
Giglio, A., Brandmayr, P., Dalpozzo, R., Sindona, G., Tagarelli, A., Talarico, F., et al. (2009). The defensive secretion of Carabus lefebvrei Dejean, 1826 pupa (Coleoptera, Carabidae): gland ultrastructure and chemical identification. Microsc. Res. Tech. 72, 351–361. doi: 10.1002/jemt.20660
Giglio, A., Brandmayr, P., Talarico, F., and Brandmayr, T. Z. (2011). Current knowledge on exocrine glands in carabid beetles: structure, function and chemical compounds. ZooKeys 100, 193–201. doi: 10.3897/zookeys.100.1527
Giglio, A., Vommaro, M. L., Brandmayr, P., and Talarico, F. (2021). Pygidial glands in Carabidae, an overview of morphology and chemical secretion. Life 11:562. doi: 10.3390/life11060562
Glassman, H. N. (1948). Surface active agents and their application in bacteriology. Bacteriol. Rev. 12, 105–148. doi: 10.1128/br.12.2.105-148.1948
Hancock, R. E., and Speert, D. P. (2000). Antibiotic resistance in Pseudomonas aeruginosa: mechanisms and impact to treatment. Drug Resist. Updat. 3, 247–255. doi: 10.1054/drup.2000.0152
Holliday, A. E., Holliday, N. J., Mattingly, T. M., and Naccarato, K. M. (2012). Defensive secretions of the carabid beetle Chlaenius cordicollis: chemical components and their geographic patterns of variation. J. Chem. Ecol. 38, 278–286. doi: 10.1007/s10886-012-0078-x
Holliday, A. E., Mattingly, T. M., Toro, A. A., Donald, L. J., and Holliday, N. J. (2016). Age- and sex-related variation in defensive secretions of adult Chlaenius cordicollis and evidence for their role in sexual communication. Chemoecology 26, 107–119. doi: 10.1007/s00049-016-0210-4
Huang, C. B., Alimova, Y., Myers, T. M., and Ebersole, J. L. (2011). Short- and medium-chain fatty acids exhibit antimicrobial activity for oral microorganisms. Arch. Oral Biol. 56, 650–654. doi: 10.1016/j.archoralbio.2011.01.011
Kabara, J. J., Swieczkowski, D. M., Conley, A. J., and Truant, J. P. (1972). Fatty acids and derivatives as antimicrobial agents. Antimicrob. Agents Chemother. 2, 23–28. doi: 10.1128/AAC.2.1.23
Kanehisa, K., and Kawazu, K. (1982). Fatty acid components of the defensive substances in acid-secreting carabid beetles. Appl. Entomol. Zool. 17, 460–466. doi: 10.1303/aez.17.460
Kanehisa, K., and Kawazu, K. (1985). Differences in neutral components of the defensive secretion in formic acid-secreting carabid beetles. Appl. Entomol. Zool. 20, 299–304. doi: 10.1303/aez.20.299
Kanehisa, K., and Murase, M. (1977). Comparative study of the pygidial defensive systems of carabid beetles. Appl. Entomol. Zool. 12, 225–235. doi: 10.1303/aez.12.225
Kanehisa, K., and Shiraga, T. (1978). Morphological study of the defensive systems of carabid beetles. Ber. Ohara Inst. Landwirtsch. Biol. Okayama Univ. 17, 83–94.
Lečić, S., Ćurčić, S., Vujisić, L., Ćurčić, B., Ćurčić, N., Nikolić, Z., et al. (2014). Defensive secretion in three ground-beetle species (Insecta: Coleoptera: Carabidae). Ann. Zool. Fenn. 51, 285–300. doi: 10.5735/086.051.0301
McCullough, B. T. (1966). Quantitative determination of salicylaldehyde in the scent fluid of Calosoma macrum, C. alternans sayi, C. affine, and C. parvicollis (Coleoptera: Carabidae). Ann. Entomol. Soc. Am. 59, 1018. doi: 10.1093/aesa/59.5.1018
McCullough, B. T. (1969). Chemical analysis of the defensive scent fluid produced by the ground beetle Calosoma peregrinator. Ann. Entomol. Soc. Am. 62, 1498–1499. doi: 10.1093/aesa/62.6.1498
McCullough, B. T. (1972). Defensive chemistry of the ground beetle Callisthenes luxatus (Coleoptera: Carabidae). Ann. Entomol. Soc. Am. 65, 275. doi: 10.1093/aesa/65.1.275
McCullough, B. T., and Weinheimer, A. J. (1966). Compounds found in the defensive scent fluids of Calosoma marginalis, C. externum, and C. scrutator (Coleoptera: Carabidae). Ann. Entomol. Soc. Am. 59, 410–411. doi: 10.1093/aesa/59.2.410a
Moore, B. P. (1979). “Carabid beetles: their evolution, natural history and classification” in Chemical Defence in Carabids and its Bearing on Phylogeny. eds. T. L. Erwin, G. E. Ball, and D. R. Whitehead (London: Dr. W. Junk), 193–203.
Moore, B. P., and Wallbank, B. E. (1968). Chemical composition of the defensive secretion in carabid beetles and its importance as a taxonomic character. Proc. R. Entomol. Soc. B 37, 62–72. doi: 10.1111/j.1365-3113.1968.tb00199.x
Nenadić, M. (2019). Antimicrobial and Antitumor Potential of the Secretions and Morphological Characterization of the Glandular Structures of the Pygidial Glands of Selected Species of Ground Beetles (Coleoptera: Carabidae). [Dissertation]. [Belgrade (Serbia)]: University of Belgrade - Faculty of Biology [in Serbian]
Nenadić, M., Ljaljević-Grbić, M., Stupar, M., Vukojević, J., Ćirić, A., Tešević, V., et al. (2017a). Antifungal activity of the pygidial gland secretion of Laemostenus punctatus (Coleoptera: Carabidae) against cave-dwelling micromycetes. Sci. Nat. 104:52. doi: 10.1007/s00114-017-1474-4
Nenadić, M., Soković, M., Calhelha, R. C., Ferreira, I. C. F. R., Ćirić, A., Vesović, N., et al. (2018). Inhibition of tumour and non-tumour cell proliferation by pygidial gland secretions of four ground beetle species (Coleoptera: Carabidae). Biologia 73, 787–792. doi: 10.2478/s11756-018-0082-x
Nenadić, M., Soković, M., Glamočlija, J., Ćirić, A., Perić-Mataruga, V., Ilijin, L., et al. (2016a). Antimicrobial activity of the pygidial gland secretion of three ground beetle species (Insecta: Coleoptera: Carabidae). Sci. Nat. 103:34. doi: 10.1007/s00114-016-1358-z
Nenadić, M., Soković, M., Glamočlija, J., Ćirić, A., Perić-Mataruga, V., Ilijin, L., et al. (2017b). The pygidial gland secretion of the forest caterpillar hunter, Calosoma (Calosoma) sycophanta: the antimicrobial properties against human pathogens. Appl. Microbiol. Biotechnol. 101, 977–985. doi: 10.1007/s00253-016-8082-7
Nenadić, M., Soković, M., Glamočlija, J., Ćirić, A., Perić-Mataruga, V., Tešević, V., et al. (2016b). Antimicrobial activity of the pygidial gland secretion of the troglophilic ground beetle Laemostenus (Pristonychus) punctatus (Dejean, 1828) (Insecta: Coleoptera: Carabidae). Bull. Entomol. Res. 106, 474–480. doi: 10.1017/S0007485316000109
Pang, Z., Raudonis, R., Glick, B. R., Lin, T.-J., and Cheng, Z. (2019). Antibiotic resistance in Pseudomonas aeruginosa: mechanisms and alternative therapeutic strategies. Biotechnol. Adv. 37, 177–192. doi: 10.1016/j.biotechadv.2018.11.013
Pasteels, J. M., Grégoire, J.-C., and Rowell-Rahier, M. (1983). The chemical ecology of defense in arthropods. Annu. Rev. Entomol. 28, 263–289. doi: 10.1146/annurev.en.28.010183.001403
Pelttari, E., Lehtinen, M., and Elo, H. (2011). Substituted salicylaldehydes as potential antimicrobial drugs: minimal inhibitory and microbicidal concentrations. Z. Naturforsch. C 66, 571–580. doi: 10.1515/znc-2007-7-806
Pu, Y.-C., Xiang, H.-J., Liang, X.-Y., Wang, Y., Hou, Y.-M., Fu, L., et al. (2020). External immune inhibitory efficiency of external secretions and their metabolic profiling in red palm weevil, Rhynchophorus ferrugineus (Coleoptera: Curculionidae). Front. Physiol. 10:1624. doi: 10.3389/fphys.2019.01624
Raftari, M., Jalilian, F. A., Abdulamir, A. S., Ghafurian, S., Sekawi, Z., Son, R., et al. (2012). Antibacterial activity of organic acids on Salmonella and Listeria. Asia Life Sci. 21, 13–30.
Ristivojević, P., Dimkić, I., Trifković, J., Berić, T., Vovk, I., Milojković-Opsenica, D., et al. (2016). Antimicrobial activity of Serbian propolis evaluated by means of MIC, HPTLC, bioautography and chemometrics. PLoS One 11:e0157097. doi: 10.1371/journal.pone.0157097
Rork, A. M., and Renner, T. (2018). Carabidae semiochemistry: current and future directions. J. Chem. Ecol. 44, 1069–1083. doi: 10.1007/s10886-018-1011-8
Schildknecht, H., Holoubek, K., Weis, K. H., and Krämer, H. (1964). Defensive substances of the arthropods, their isolation and identification. Angew. Chem. Int. Ed. 3, 73–82. doi: 10.1002/anie.196400731
Schildknecht, H., Maschwitz, U., and Winkler, H. (1968). Zur Evolution der Carabiden-Wehrdrüsensekrete. Über Arthropoden-Abwehrstoffe XXXII. Naturwissenschaften 55, 112–117. doi: 10.1007/BF00624238
Schildknecht, H., and Weis, K. H. (1962). Die Abwehrstoffe einiger Carabiden, insbesondere von Abax ater. XII. Mitteilung über Insektenabwehrstoffe. Z. Naturforsch. B 17, 439–447. doi: 10.1515/znb-1962-0706
Tao, J. X., Song, X. L., Yao, Y. Y., Chen, F. S., and Su, J. S. (2012). Self-cleaning and antimicrobial properties of methacrylic acid coupled TiO(2)/PMMA denture base resin. Shanghai J. Stomatol. 21, 130–133.
Varsha, K. K., Devendra, L., Shilpa, G., Priya, S., Pandey, A., and Nampoothiri, K. M. (2015). 2,4-Di-tert-butyl phenol as the antifungal, antioxidant bioactive purifed from a newly isolated Lactococcus sp. Int. J. Food Microbiol. 211, 44–50. doi: 10.1016/j.ijfoodmicro.2015.06.025
VasudhaUdupa, A., Gowda, B., Kumarswammy, B. E., and Shivanna, M. B. (2021). The antimicrobial and antioxidant property, GC-MS analysis of non-edible oil-seed cakes of neem, madhuca, and simarouba. Bull. Natl. Res. Cent. 45:41. doi: 10.1186/s42269-021-00498-x
Vesović, N. (2019). Morphological Study of the Pygidial Glands and Analysis of the Chemical Composition of the Secretions of Selected Ground Beetle Species (Insecta: Coleoptera: Carabidae). [Dissertation]. [Belgrade (Serbia)]: University of Belgrade - Faculty of Biology [in Serbian]
Vesović, N., Ćurčić, S., Todosijević, M., Nenadić, M., Zhang, W., and Vujisić, L. (2020). Pygidial gland secretions of Carabus Linnaeus, 1758 (Coleoptera: Carabidae): chemicals released by three species. Chemoecology 30, 59–68. doi: 10.1007/s00049-019-00298-w
Vesović, N., Nenadić, M., Soković, M., Ćirić, A., Vujisić, L., Todosijević, M., et al. (2022). Pygidial glands of the blue ground beetle Carabus intricatus: chemical composition of the secretion and its antimicrobial activity. Sci. Nat. 109:19. doi: 10.1007/s00114-022-01790-0
Vesović, N., Vujisić, L., Perić-Mataruga, V., Krstić, G., Nenadić, M., Cvetković, M., et al. (2017). Chemical secretion and morpho-histology of the pygidial glands in two Palaearctic predatory ground beetle species: Carabus (Tomocarabus) convexus and C. (Procrustes) coriaceus (Coleoptera: Carabidae). J. Nat. Hist. 51, 545–560. doi: 10.1080/00222933.2017.1293183
Vranić, S., Ćurčić, S., Vesović, N., Mandić, B., Pantelić, D., Vasović, M., et al. (2020). Chemistry and morphology of the pygidial glands in four Pterostichini ground beetle taxa (Coleoptera: Carabidae: Pterostichinae). Zoology 142:125772. doi: 10.1016/j.zool.2020.125772
Wheeler, J. W., Chung, R. H., Oh, S. K., Benfield, E. F., and Neff, S. E. (1970). Defensive secretions of cychrine beetles (Coleoptera: Carabidae). Ann. Entomol. Soc. Am. 63, 469–471. doi: 10.1093/aesa/63.2.469
Will, K. W., Attygalle, A. B., and Herath, K. (2000). New defensive chemical data for ground beetles (Coleoptera: Carabidae): interpretations in a phylogenetic framework. Biol. J. Linn. Soc. 71, 459–481. doi: 10.1006/bijl.2000.0456
Keywords: carabids, GC–MS, exocrine glands, antibacterial activity, broth microdilution, allomones, chemical defence, semiochemistry
Citation: Vesović N, Nenadić M, Vranić S, Vujisić L, Milinčić KM, Todosijević M, Dimkić I, Janakiev T, Ćurčić NB, Stevanović N, Mihajlović L, Vukoičić DŽ and Ćurčić S (2023) The chemical composition of the secretions, their antibacterial activity, and the pygidial gland morphology of selected European Carabini ground beetles (Coleoptera: Carabidae). Front. Ecol. Evol. 11:1120006. doi: 10.3389/fevo.2023.1120006
Edited by:
Nurhayat Tabanca, Agricultural Research Service (USDA), United StatesReviewed by:
Irena Valterova, Institute of Organic Chemistry and Biochemistry (ASCR), CzechiaSalvatore Guarino, Institute of Biosciences and Bioresources (CNR), Italy
Copyright © 2023 Vesović, Nenadić, Vranić, Vujisić, Milinčić, Todosijević, Dimkić, Janakiev, Ćurčić, Stevanović, Mihajlović, Vukoičić and Ćurčić. This is an open-access article distributed under the terms of the Creative Commons Attribution License (CC BY). The use, distribution or reproduction in other forums is permitted, provided the original author(s) and the copyright owner(s) are credited and that the original publication in this journal is cited, in accordance with accepted academic practice. No use, distribution or reproduction is permitted which does not comply with these terms.
*Correspondence: Nikola Vesović, nikola.vesovic@bio.bg.ac.rs