- 1Degree Programs in Life and Earth Sciences, University of Tsukuba, Tsukuba, Japan
- 2Faculty of Life and Environmental Sciences, University of Tsukuba, Tsukuba, Japan
Herbivore-induced plant volatiles (HIPVs) from infested plants are exploited by parasitoid wasps as cues to search for host habitat. However, uninfested (intact) and infested plants are often intermingled in nature, and how volatiles released by intact plants affect host-searching behavior in natural habitats remains unknown. In a previous study, using a wind tunnel, we found that intact maize plants negatively influenced landing behavior of a parasitoid wasp, Cotesia kariyai (Hymenoptera: Braconidae), when they were placed downwind of infested plants. To determine whether intact plant volatiles affect host searching by C. kariyai, we collected volatiles from intact plants using a headspace volatile collection technique. In a wind tunnel, wasps landed less frequently on a green paper model treated with intact plant volatiles placed in front of an infested plant, than on a green paper model treated with the solvent, hexane. In a 4-arm olfactometer, wasps spent significantly less time in an arena containing mixed volatiles from intact and infested plants, compared to the same arena treated only with volatiles from infested plants. Both results indicate that intact plant volatiles re-direct host-searching behavior in C. kariyai females. Chemical analyses suggest differences in volatiles between intact and infested plants. In terms of parasitoid adaptations for effective searching for hosts in complex environments, aversive effects of intact plant volatiles may help C. kariyai to locate patches of infested plants.
Introduction
Parasitoid insects exploit volatiles produced by host-infested plants to locate habitat of suitable herbivorous hosts (Turlings and Wäckers, 2004; Afsheen et al., 2008; Erb, 2018; Turlings and Erb, 2018; Takabayashi, 2022). In chemically complex environments, parasitoid wasps adopt different foraging strategies, such as ignoring complexity, avoiding complexity, or seeking complexity so as to locate their hosts. In spatial responses to this complexity, they are influenced by the composition and concentrations of plant compounds from neighboring plants (Schröder and Hilker, 2008; Wäschke et al., 2013). Such neighboring plant odors may render resource-indicating odor less detectable, or may enhance detection (Schröder and Hilker, 2008).
To seek host habitat in a diverse environment, parasitoids can follow multiple plant odor plumes and may leave the track of one plume to follow another, if it gives a more reliable signal about their host (Beyaert and Hilker, 2014). To locate host-infested plant patches, natural enemies are generally thought to detect modifications in plant volatile blends (Meiners, 2015). As most vascular plants constitutively release volatile organic compounds (VOCs) (Holopainen and Gershenzon, 2010), intact plants also emit volatiles in trace amounts or in amounts that are qualitatively and/or quantitatively different from those emitted by infested conspecific plants (Takabayashi and Dicke, 1996; Arimura et al., 2009; Mumm and Dicke, 2010; Turlings and Erb, 2018; Takabayashi and Shiojiri, 2019; Takabayashi, 2022). In this paper, volatiles from intact plants (constitutive odor) and infested plants overlap and may result in a blend that allows parasitoid wasps to search for patches of host-infested plants in the field more efficiently.
Parasitoid females are attracted more to volatiles emitted by host-infested plants than to those by intact, i.e., uninfested plants (McCormick et al., 2012; Wäschke et al., 2013; Turlings and Erb, 2018). Several studies have found that volatiles from neighboring plants affect olfactory responses of predators and parasitoids to induced volatiles (Schröder and Hilker, 2008; Liu et al., 2019; Vosteen et al., 2020; Takabayashi, 2022). Some authors have tested discrimination ability of parasitoid wasps in searching for host-infested plants in the presence of heterospecific neighboring plants (Wäschke et al., 2013; De Rijk et al., 2016). Deterrent effects of plant volatiles have also been reported when non-host infested plants are provided (Schröder and Hilker, 2008; Wäschke et al., 2013). The very first case demonstrating that intact plants, a potential food source for host herbivores of the parasitoid insects, negatively influence host-searching parasitoids, was reported by Isono et al. (2019). They conducted a series of wind tunnel experiments on deterrent effects of intact plants on host-searching behavior of Cotesia kariyai (Hymenoptera: Braconidae), a parasitoid wasp of Mythimna separata larvae (Lepidoptera: Noctuidae). Cotesia kariyai females use induced volatiles from plant leaves infested with M. separata caterpillars to locate their hosts (Takabayashi et al., 1991, 1995; Fujiwara et al., 2000). According to Isono et al. (2019), when intact plants were placed downwind of host-infested plants, the landing rate of C. kariyai females on intact plants was significantly lower than when intact plants were replaced by green paper models. Those results suggested that intact plants deter host-searching parasitoids. However, attributes of intact plants that discourage host-searching C. kariyai have not been identified. We hypothesized that volatiles are the responsible agents. In order to test this hypothesis, in the absence of visual cues, we demonstrated effects of intact plant volatiles on host-searching C. kariyai in bioassays using a wind tunnel (Fukushima et al., 2001; Isono et al., 2019) and a 4-arm olfactometer (Thanikkul et al., 2017; Mizuno et al., 2022). In addition, we also compared volatile profiles of both intact and infested plants.
Materials and methods
Insect colonies and rearing procedures
A colony of C. kariyai was reared on M. separata larvae. Adult parasitoids were fed with honey in a container (17 × 24 cm, 15 cm high) and cotton soaked with water. The M. separata colony was reared on an artificial diet (Silkmate® 2M, Nosan Corporation, Kanagawa, Japan) under laboratory conditions (16 h light: 8 h dark) at 25 ± 1°C, 60 ± 20% r. h. following the method of Fukushima et al. (2001). Both species were obtained from stock cultures at the Laboratory of Applied Entomology and Zoology, University of Tsukuba, Japan. Cotesia kariyai used in bioassays were 2- to 4-day-old mated, naïve females (without oviposition experience).
Plant materials
Maize (“Honey-Bantam Peter 610”: Sakata Seed Co., Kanagawa, Japan) was used as the host plant for M. separata in all experiments. Maize seeds were planted individually in semi-transparent plastic pots (5.5 cm in diam., 7.0 cm in height) and kept in an incubator (25 ± 1°C, photoperiod: 16L-8D) for 4–5 days, before being transferred to larger plastic flowerpots (11.0 cm in diameter, 9.0 cm in height) in an air-conditioned greenhouse (25 ± 1°C). Plants were watered daily. Two- to three-week-old maize plants (ca. 30 cm high) were used in experiments.
To obtain host-infested maize plants, 10 third-instar larvae of M. separata were introduced onto maize plants and were allowed to feed for 12 h in a cage (50 × 50 cm, 90 cm high, under natural light conditions). All larvae and their feces were removed from plants before experiments. Aluminum foil was used to cover the pot and soil.
Volatile collection
An intact maize plant, ~2 weeks old, was utilized for headspace collection. An intact maize plant that was previously used for headspace collection was subsequently infested with 10 third-instar M. separata larvae for 12 h. Then it was subjected to another headspace collection the following day. The volatile collection method employed was modified from Kobayashi et al. (2012) and Liu et al. (2019). Volatiles were collected from the whole plant, so aluminum foil was used to wrap the pot and soil to eliminate background odor. For infested maize, all larvae and their feces were removed from the plant with a brush and forceps before headspace collection. During the collection process, an intact or an infested maize plant was placed in a separable glass flask (i.d. 120 mm, o.d. 130 mm, height 300 mm; AS ONE Corporation, Osaka, Japan) with a three-necked cover having the middle neck closed. One side-neck was connected to an Erlenmeyer flask (500 mL) containing activated charcoal (granules) to filter the air passed over potted plants. The other inlet neck was connected to a vacuum pump (KNF Neuberger, Freiburg, Germany) via a glass tube containing adsorbent. The adsorbent used in volatile collection was “HayeSep Q” (ca. 100 mg, 60–80 mesh, Restek Corporation, USA), sandwiched between quartz wool (fine grade; Daico Mfg, Kyoto, Japan) in a glass tube cartridge (i.d. 6.0 mm, o.d. 7.0 mm, 30 mm long). For conditioning, the cartridge was washed with hexane before heating at 150°C for 2 h under nitrogen flow (30 mL/min). Headspace volatiles were collected for 3 h between 1,200 and 1,500 at 25 ± 1°C, 60 ± 20% r.h., with light intensity at ca. 2,950 lx and airflow at 1 L min−1. After collection, 1 mL of distilled hexane was used to elute volatiles from the adsorbent into a vial and the eluate was stored in a freezer at −20°C. An aliquot that provided the amount of volatiles equivalent to a 5-min collection per plant (PE: plant equivalent) was applied to a filter paper strip for use in wind tunnel bioassays and volatile extracts were subsequently used for chemical analysis (Supplementary Figure S1).
Experiment 1: effect of intact plant volatiles on host-searching behavior of C. kariyai in a wind tunnel
To determine whether intact plant volatiles affect host-searching behavior of C. kariyai, crude extracts of intact plant volatiles were tested in a wind tunnel. Volatiles used in these bioassays were collected from 6 individual intact plants separately. Five individual wasps were tested per plant extract sample. An infested plant was placed upwind behind a black mesh screen and a green paper model was used as visual stimulus in front of the black mesh screen (Figure 1). A filter paper strip (4 × 40 mm, No. 2, Advantec Toyo Kaisha, Ltd., Japan) treated with volatile extract was attached to the green paper model with double-sided tape. The green paper model was more attractive to C. kariyai females than other tested colors following the method of Isono et al. (2019). Paper models were made of fine green papers (Muse Co., Ltd., Tokyo, Japan), a bamboo stick (4 mm in diam., 10 cm in length) and a rubber stopper (2 cm in diam., 2.3 cm in length). The reflectance of the green paper was highest between 520 and 580 nm (Isono et al., 2019). A green paper model (Figure 1) consisted of four leaves and those leaves were attached to the bamboo stick with cellophane tape and the base of the stick was inserted into a rubber stopper. The bioassay was conducted in a wind tunnel following the methods of Fukushima et al. (2001). The wind tunnel consisted of an acrylic rectangular box 30 cm in height, 30 cm in depth, 150 cm in length at a wind speed of 25–30 cm/s, 25 ± 1°C, 60 ± 20% r. h., and light intensity 900 lx. A metal platform (Labo Jack, AS ONE Corporation, top plate: 8 × 8 cm, 15 cm in height) was used as a release stage for parasitoid females and placed 25 cm downwind from the green paper model. Infested plants were placed 10 cm upwind from the green paper model and 35 cm upwind from the release point. In order to prevent the infested plant from providing visual cues, a black mesh screen (nylon, 90#) was inserted between the green paper model and the host-infested plant. Also, we tested a lower concentration (0.1 PE) in addition to a natural one (1.0 PE) to examine effects of intact plant volatiles at a different concentration.
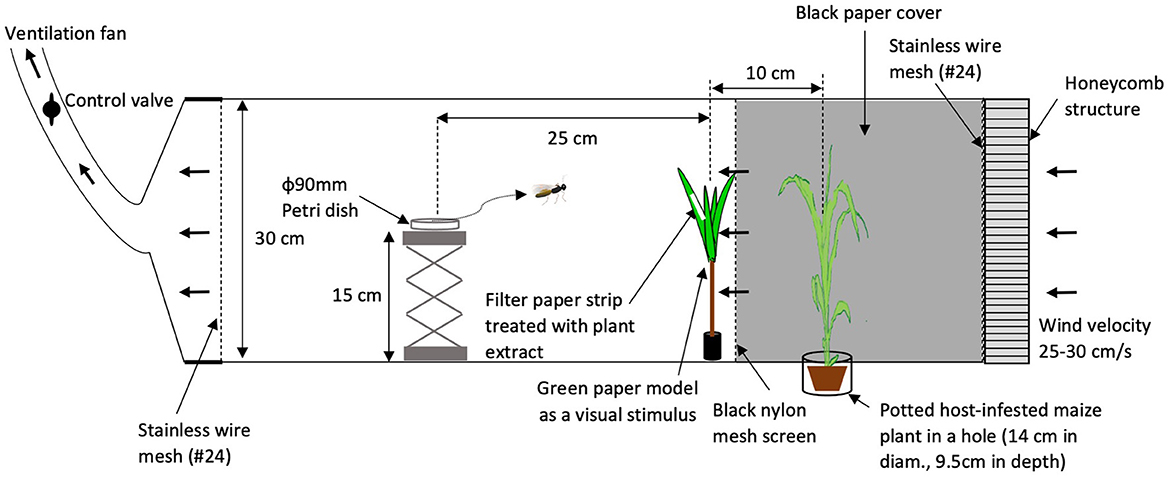
Figure 1. Setup of the wind tunnel for bioassays. A potted, host-infested maize plant was placed in a cylindrical container, recessed in the wind tunnel floor. A honeycombed diffuser panel was fixed at the windward end of the tunnel to facilitate laminar airflow. Stainless wire mesh screens at both end sides of the wind tunnel were equipped to avoid escapes of wasps. Arrows indicate the direction of airflow. In Experiment 1, the filter paper strip was attached directly to the green paper model.
Female wasps were randomly selected from the rearing cage, and each individual was placed in a plastic Petri dish (5.3 cm in diameter, 1.5 cm in height), in which a piece of wet cotton wick was provided. For acclimatization, we placed parasitoid females for 1 h in a wind tunnel room before experiments. A plastic Petri dish containing a parasitoid was placed on the release stage and the cover was opened gently to release it. We observed each wasp for 5 min and noted whether it landed on the target paper model.
Experiment 2: effect of intact plant volatiles on host-searching behavior of C. kariyai in a 4-arm olfactometer
Behavioral responses of C. kariyai females to various odor sources were tested in a 4-arm olfactometer (24 × 24 cm, 2 cm high) (Fujinuma et al., 2010; Nurkomar et al., 2017) in a dual-choice setup. Intact and infested maize were tested individually and in combination. When intact or infested maize plants were provided as odor sources, each intact or infested maize plant, or both intact and infested plants were placed in a 3-L flask as described above in “Volatile collection”. An individual female wasp was prepared as described in “Experiment 1”, without previous exposure to plants or hosts, and was transferred to the center of the olfactometer arena with an insect aspirator. In a two-choice situation using a 4-arm olfactometer at an airflow of 1 L/min, two opposing arms were provided with the same odor source. Time that wasps spent in each olfactometer arena was monitored for 5 min (Mizuno et al., 2022) with a digital camera (Action Cam, AS100V, Sony, Japan) installed 37 cm above the arena, which was connected to an iPad (Apple Inc., Cupertino, CA) through Wi-Fi. The time that wasps spent actively moving (walking, antennating, hereafter referred to as “residence time”) in each treated arm of the 4-arm olfactometer was recorded using The Observer XT ver. 9.0 (Noldus Information Technology, Wageningen, The Netherlands). A test using an individual wasp was counted as one replicate as for all bioassays. Experiments were replicated at least 15 times. Intact or infested maize was replaced every five replicates, while filter paper strips were replaced for each replicate.
Statistical analysis
In Experiment 1, we compared landing rates and response rates of female wasps in each treatment using Ryan's multiple range test after a Chi-square test. In Experiment 2, residence times of wasps in treated and control areas of the olfactometer were compared using paired t-tests with Holm's p adjustment method (Holm, 1979). Normality of paired data was tested and confirmed with a Shapiro–Wilk test (P > 0.05). Wasps that stayed in the central arena were regarded as showing no response, and their data were not included in the analysis (Fujinuma et al., 2010; Nurkomar et al., 2017; Thanikkul et al., 2017; Liu et al., 2019). All analyses were performed in R v. 4.1.2 software (R Core Team., 2016) and Ryan's multiple range test was performed using an open source package (http://aoki2.si.gunma-u.ac.jp/R/m_multi_comp.html).
Results
Experiment 1: effects of intact plant volatiles on host-searching behavior of C. kariyai in a wind tunnel
In a wind tunnel, wasps landed less frequently on a green paper model treated with intact plant extract placed downwind of an infested plant (16.0%) than on a green paper model treated with hexane placed downwind of an infested plant (control) (69.0%) (χ2 = 14.81, df = 3, P > 0.05; Figure 2). At a 10-fold lower concentration of intact plant volatiles, there was no significant difference between the landing rate of parasitoid females on the control treatment (69.0%) and on a green paper model treated with diluted intact plant extract placed downwind of an infested plant (59.0%). No wasps landed on a green paper model treated with hexane without an upwind host-infested plant (0%). Seventy-nine percent of wasps (114/145) took flight in the wind tunnel and response rate did not differ among the treatments.
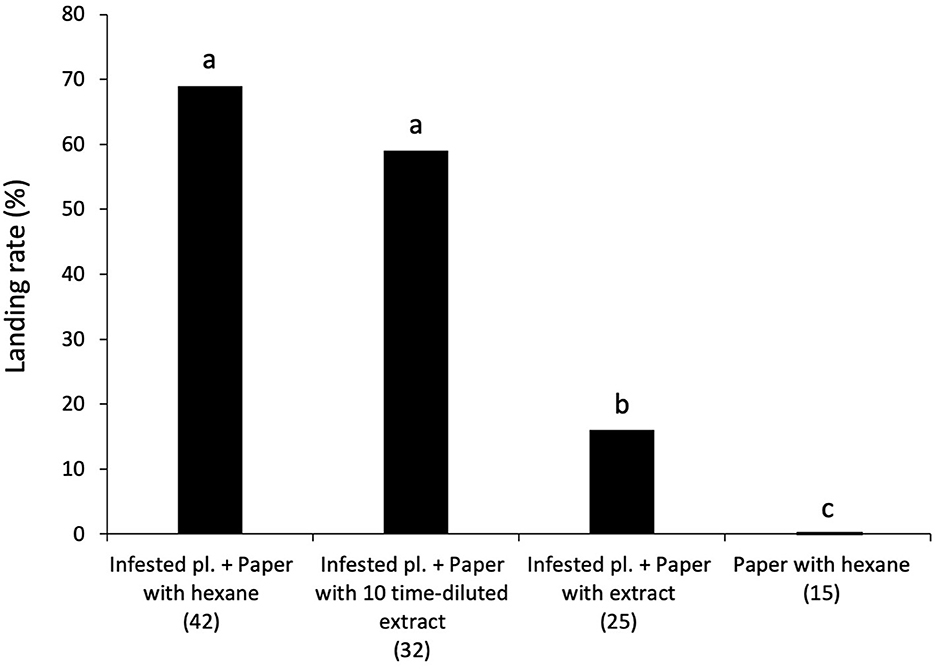
Figure 2. Flight responses of naïve female C. kariyai in a wind tunnel to a green paper model with different upwind plant settings. A green paper model treated with hexane with an upwind infested plant (control), a green paper model treated with diluted (×0.1) intact plant extract with an upwind infested plant, a green paper model treated with intact plant extract with an upwind infested plant, and a green paper model treated with hexane without an upwind infested plant. The percentage of parasitoid wasps landing on each target was recorded. Different letters above bars show significant differences (Ryan's multiple range test for proportion after χ2-test, P < 0.05). “n” indicates the number of wasps. “pl.” refers plant.
Experiment 2: effects of intact plant volatiles on host-searching behavior of C. kariyai in a 4-arm olfactometer
Cotesia kariyai females spent significantly more time in an olfactometer arena containing volatiles from an infested plant than in those with clean air (t = −9.22, df = 14; Figure 3) or an intact plant (t = −5.66, df = 14). There was no significant difference between time spent in the olfactometer arena containing odor from an intact plant and those from clean air. When we tested a combination of intact and infested plants vs. infested plants, wasps spent significantly less time in the arena containing mixed volatiles from intact and infested plants than in those from infested plants alone (t = −4.08, df = 26). However, wasps spent significantly more time in the olfactometer arena containing odor from an intact plant and an infested plant than in the arena with an intact plant alone (t = 3.79, df = 26). Ninety-four percent of wasps (99/105) responded in the olfactometer and the response rate did not differ among the treatments.
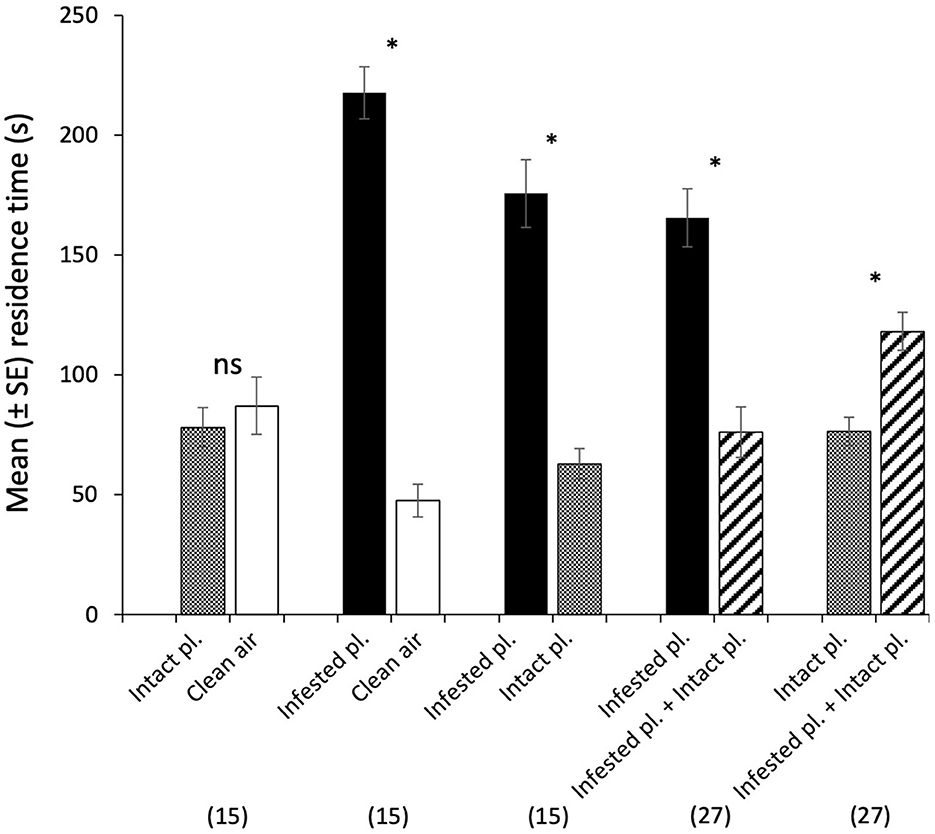
Figure 3. Behavioral responses of naïve female C. kariyai in a 4-arm olfactometer to various two-choice comparisons. An intact plant vs. clean air, an infested plant vs. clean air, an infested plant vs. an intact plant, an infested plant vs. infested + intact plants, an intact plant vs. infested + intact plants. Bars represent mean residence time of female wasps in each arena. Error bars are SEM and asterisks indicate a significant difference in paired t-tests with the Holm p-adjustment (The asterisk indicates a significant P-value based on Holm's adjusted significance level; ns, not significant). “n” indicates the number of wasps. “pl.” refers to plant.
Discussion
Deterrent effects of plant volatiles have been reported when non-host infested plants are provided (Schröder and Hilker, 2008; Wäschke et al., 2013). The very first proof that an intact plant that could be a potential host for host herbivores of parasitoids had negative effects on host-searching parasitoids was reported by Isono et al. (2019); however, the cause of aversion was not determined. Results obtained from a wind tunnel and a 4-arm olfactometer indicate that parasitoid females are less attracted to host-infested plant volatiles when intact plant volatiles are present (Figures 2, 3). In a 4-arm olfactometer, wasps spent significantly more time in an olfactometer arena containing odor from intact plants and infested plants than that containing odor from intact plants alone. This indicates that mixed blend is less attractive than the pure HIPV blend or that background odor partially masks HIPVs (Schröder and Hilker, 2008). Since insects can follow several plant odor plumes to their target source, they may leave the odor plume of one plant and switch to another one if it represents a signal with higher reliability or indicates a more suitable resource (Schröder and Hilker, 2008; Beyaert and Hilker, 2014). Vosteen et al. (2019) also demonstrated that parasitoids briefly inspect uninfested plants that are placed in front of host-infested plants; thus, volatiles from intact plants may assist parasitoids in determining that emitting plants do not carry any hosts. Therefore, using intact plant volatiles may increase host-searching efficiency in complex natural habitats. In our experiments, parasitoids recognized blends of intact and infested plants and showed decreased responses when volatiles from infested plants were mixed with volatiles from intact plants. This observation suggests that intact plant volatiles may help C. kariyai to efficiently find infested plants in the field by moving out of areas or wind plumes that smell of intact plants.
In wind tunnel tests, at a lower concentration of intact plant extract, landing rates of C. kariyai females on a green paper model treated with hexane downwind of an infested plant, vs. on a green paper model treated with diluted intact plant extract (0.1 PE) in front of an infested plant were not significantly different (Figure 2). This indicates a concentration threshold of intact plant volatiles to affect host-searching behavior of C. kariyai. Both the quality and quantity of plant compounds present as background odor, as well as their spatial presentation, greatly influence orientation of foraging parasitoids (Schröder and Hilker, 2008; Wäschke et al., 2013). So far, the detectability of resource-indicating odor has been discussed with respect to amounts of volatiles emitted by a resource (Vet and Dicke, 1992; Dicke, 1999; Turlings et al., 2002; Steidle and van Loon, 2003), but our study suggests that odor concentration also matters for avoidance of non-resources. A high concentration of volatiles from intact plants indicates either close proximity of the intact plant or a large plant patch. Experiments at a larger spatial scale such as field or semi-field are required to further investigate these effects.
To cope with chemical complexity, parasitoids ignore, avoid, and seek odors. In spatial responses to complexity, parasitoids adjust their movements to locate host-infested plants (Wäschke et al., 2013). By moving out of areas or wind plumes carrying high odor diversity (quality and quantity of plant compounds present as background odor), some parasitoids have a better chance of perceiving odors of their host's food plants against a less complex background. Behaviors such as short flights or other movements can change the angle at which two odor sources are presented. When odor sources are widely separated, parasitoids, may be able to respond accordingly (Schröder and Hilker, 2008; Wäschke et al., 2013). As our experiments were performed in a limited space (wind tunnel and olfactometer), different behaviors of C. kariyai females responding to intact and infested plant volatiles may occur in a larger space or in an open field.
Chemical analyses of volatiles suggested that differences existed in both components and ratios between intact and infested plants (Supplementary Figure S1). Takabayashi et al. (1995) reported that intact maize plants emit aldehydes, alcohols, ketones, acetates, terpenoids and other compounds. In addition, intact maize plants release unique compounds that are not found in infested plants, or emit larger amounts of certain compounds (l-butyl acetate, (Z)-3-hexen-l-yl acetate, α-pinene, myrcene, limonene, and methyl salicylate) than infested plants. Several studies demonstrated that a single component of background odor can affect olfactory responses of insects to induced volatiles by making the blend less attractive (Schröder and Hilker, 2008; Takabayashi, 2022). Visser and Avé (1978) found that the Colorado potato beetle (Leptinotarsa decemlineata) was no longer attracted to undamaged potato plants after addition of a ubiquitous green leaf volatile compound (E)-3-hexen-1-ol, which doesn't elicit a behavioral response of the beetle when it is presented alone. Similarly, alate virginoparae of the black bean aphid, Aphis fabae, were no longer attracted to the odor blend of their host-plant leaves when 3-butenyl or 4-pentenyl isothiocyanate was added to the blend (Nottingham et al., 1991). The host plant itself may release a component in admixture with other components and addition of the same component may disturb the ratio of host-plant volatiles crucial for attraction (Schröder and Hilker, 2008). In the context of intact plant volatiles, the presence of a single component and its amount may influence host-searching behavior of C. kariyai. Detailed bioassays with synthetic compounds may reveal how the negative effect of intact plant volatiles works on the behavior of C. kariyai, which remains a subject for future research.
Laboratory experiments (Isono et al., 2019; this study) indicate that intact plants direct host-searching behavior of C. kariyai elsewhere. Our present results showed that volatiles emitted by intact plants are the responsible agent for this effect. These results suggest that by using plant volatiles, parasitoids detect not only the presence of their hosts, but also their absence on intact plants. Using volatiles from intact plants may increase host-searching efficiency in complex natural habitats. Interestingly, some studies have shown that neighboring intact plants can also detect volatiles of infested plants and prime themselves for subsequent attack by herbivores by attracting herbivore natural enemies, providing evidence of plant-plant communications (Dicke and Baldwin, 2010; Ninkovic et al., 2016, 2019; Heil and Karban, 2020; Loreto and D'Auria, 2021; Takabayashi, 2022). This study highlights the importance of considering effects of volatile emissions from surrounding plants on parasitoid foraging behavior and calls for behavioral experiments in more complex foraging situations in laboratory and field set-ups (see for example Vosteen et al., 2020; de Bruijn et al., 2021).
Data availability statement
The raw data supporting the conclusions of this article will be made available by the authors, without undue reservation.
Author contributions
SR, KK, and YK designed the experiments. SR and SM performed the experiments. SR wrote the first draft. SM, SF, KK, and YK reviewed the paper and checked all the details. All authors read and approved the final manuscript.
Acknowledgments
We appreciate the kind help of Dr. DeMar Taylor for his helpful comments on an early draft. We are grateful to Dr. Yukie Sato for her helpful advice in statistical analysis and Dr. Steven D. Aird for reviewing the manuscript.
Conflict of interest
The authors declare that the research was conducted in the absence of any commercial or financial relationships that could be construed as a potential conflict of interest.
Publisher's note
All claims expressed in this article are solely those of the authors and do not necessarily represent those of their affiliated organizations, or those of the publisher, the editors and the reviewers. Any product that may be evaluated in this article, or claim that may be made by its manufacturer, is not guaranteed or endorsed by the publisher.
Supplementary material
The Supplementary Material for this article can be found online at: https://www.frontiersin.org/articles/10.3389/fevo.2023.1118905/full#supplementary-material
References
Afsheen, S., Wang, X., Li, R., Zhu, C., and Lou, Y. (2008). Differential attraction of parasitoids in relation to specificity of kairomones from herbivores and their by-products. Insect. Sci. 15, 381–397. doi: 10.1111/j.1744-7917.2008.00225.x
Arimura, G., Matsui, K., and Takabayashi, J. (2009). Chemical and molecular ecology of herbivore-induced plant volatiles: proximate factors and their ultimate functions. Plant Cell Physiol. 50, 911–923. doi: 10.1093/pcp/pcp030
Beyaert, I., and Hilker, M. (2014). Plant odour plumes as mediators of plant-insect interactions. Biol. Rev. 89, 68–81. doi: 10.1111/brv.12043
de Bruijn, J. A. C., Vosteen, I., Vet, L. E. M., Smid, H. M., and de Boer, J. G. (2021). Multi-camera field monitoring reveals costs of learning for parasitoid foraging behaviour. J. Anim. Ecol. 90, 1635–1646. doi: 10.1111/1365-2656.13479
De Rijk, M., Yang, D., Engel, B., Dicke, M., and Poelman, E. H. (2016). Feeding guild of non-host community members affects host-foraging efficiency of a parasitic wasp. Behav. Ecol. 97, 1388–1399. doi: 10.1890/15-1300.1
Dicke, M. (1999). Are herbivore-induced plant volatiles reliable indicators of herbivore identity to foraging carnivorous arthropods? Entomol. Exp. Appl. 91, 131–142.
Dicke, M., and Baldwin, I. T. (2010). The evolution context for herbivore-induced plant volatiles: beyond the ‘cry for help'. Trends Plant Sci. 15, 167–175. doi: 10.1016/j.tplants.2009.12.002
Erb, M. (2018). Volatiles as inducers and suppressors of plant defense and immunity-origins, specificity, perception and signaling. Curr. Opin. Plant Biol. 44, 117–121. doi: 10.1016/j.pbi.2018.03.008
Fujinuma, M., Kainoh, Y., and Nemoto, H. (2010). Barago officinalis attracts the aphid parasitoid Aphidius colemani (Hymenoptera: Braconidae). Appl. Entomol. Zool. 45, 615–620. doi: 10.1303/aez.2010.615
Fujiwara, C., Takabayashi, J., and Yano, S. (2000). Effects of host-food plant species on parasitization rates of Mythimna separata (Lepidoptera: Noctuidae) by a parasitoid, Cotesia kariyai (Hymenoptera: Braconidae). Appl. Entomol. Zool. 35, 131–136. doi: 10.1303/aez.2000.131
Fukushima, J., Kainoh, Y., Honda, H., and Takabayashi, J. (2001). Learning of host-infested plant volatiles in the larval parasitoid Cotesia kariyai. Entomol. Exp. Appl. 99, 341–346. doi: 10.1046/j.1570-7458.2001.00833.x
Heil, M., and Karban, R. (2020). Explaining evolution of plant communication by airborne signals. Trends Ecol. Evol. 25, 137–144. doi: 10.1016/j.tree.2009.09.010
Holm, S. (1979). A simple sequentially rejective multiple test procedure. Scand. J. Statist. 6, 65–70.
Holopainen, J. K., and Gershenzon, J. (2010). Multiple stress factors and the emission of plant VOCs. Trends Plant Sci. 15, 177–184. doi: 10.1016/j.tplants.2010.01.006
Isono, K., Kuramitsu, K., and Kainoh, Y. (2019). Deterrent effects of intact plants on host-searching behavior of parasitoid wasps. Appl. Entomol. Zool. 55, 199–204. doi: 10.1007/s13355-020-00669-8
Kobayashi, K., Arai, M., Tanaka, A., Matsuyama, S., Honda, H., and Ohsawa, R. (2012). Variation in floral scent compounds recognized by honeybees in Brassicaceae crop species. Breed Sci. 62, 293–302. doi: 10.1270/jsbbs.62.293
Liu, C. M., Matsuyama, S., and Kainoh, Y. (2019). Synergistic effects of volatiles from host-infested plants on host-searching behavior in the parasitoid wasp Lytopylus rufipes (Hymenoptera: Braconidae). J. Chem. Ecol. 45, 684–692. doi: 10.1007/s10886-019-01088-y
Loreto, F., and D'Auria, S. (2021). How do plants sense volatiles sent by other plants? Trends Plant Sci. 27, 29–38. doi: 10.1016/j.tplants.2021.08.009
McCormick, A. C., Unsicker, S. B., and Gershenzon, J. (2012). The specificity of herbivore-induced plant volatiles in attracting herbivore enemies. Trends Plant Sci. 17, 303–310. doi: 10.1016/j.tplants.2012.03.012
Meiners, T. (2015). Chemical ecology and evolution of plant–insect interactions: a multitrophic perspective. Curr. Opin. Insect Sci. 8, 22–28. doi: 10.1016/j.cois.2015.02.003
Mizuno, Y., Kuramitsu, K., and Kainoh, Y. (2022). Determining suitable observation times for testing odor preferences of a parasitoid wasp, Cotesia kariyai, using a 4-arm olfactometer. Entomol. Exp. Appl. 170, 843–849. doi: 10.1111/eea.13208
Mumm, R., and Dicke, M. (2010). Variation in natural plant products and the attraction of bodyguards involved in indirect plant defense. Can. J. Zool. 88, 628–667. doi: 10.1139/Z10-032
Ninkovic, V., Markovic, D., and Dahlin, I. (2016). Decoding neighbour volatiles in preparation for future competition and implications for tri-trophic interactions. Perspect. Plant Ecol. Evol. Syst. 23, 11–17. doi: 10.1016/j.ppees.2016.09.005
Ninkovic, V., Rensing, M., Dahlin, I., and Markovic, D. (2019). Who is my neighbor? Volatile cues in plant interactions. Plant Signal. Behav. 14, e1634993. doi: 10.1080/15592324.2019.1634993
Nottingham, S. F., Hardie, J., Dawson, G. W., Hick, A. J., Pickett, J. A., Wadhams, L. J., et al. (1991). Behavioral and electrophysiological responses of aphids to host and nonhost plant volatiles. J. Chem. Ecol. 17, 1231–1242. doi: 10.1007/BF01402946
Nurkomar, I., Pudjianto, M. S., Buchori, D., Matsuyama, S., Taylor, D. M., and Kainoh, Y. (2017). (E,E)-α-Farnesene as a host-induced plant volatile that attracts Apanteles taragamae (Hymenoptera: Braconidae) to host-infested cucumber plants. Biocontrol Sci. Tech. 28, 34–48. doi: 10.1080/09583157.2017.1413340
R Core Team. (2016). R: A Language and Environment for Statistical Computing. Vienna: R Foundation for Statistical Computing. Available online at: https://www.R-project.org/ (accessed February 25, 2023).
Schröder, R., and Hilker, M. (2008). The relevance of background odor in resource location by insect: a behavioral approach. BioScience 58, 308–316. doi: 10.1641/B580406
Steidle, J. L. M., and van Loon, J. J. A. (2003). Dietary specialization and infochemical use in carnivorous arthropods: testing a concept. Entomol. Exp. Appl. 108, 133–148. doi: 10.1046/j.1570-7458.2003.00080.x
Takabayashi, J. (2022). Herbivory-induced plant volatiles mediate multitrophic relationships in ecosystems. Plant Cell Physiol. 63:1344–1355. doi: 10.1093/pcp/pcac107
Takabayashi, J., and Dicke, M. (1996). Plant–carnivore mutualism through herbivore-induced carnivore attractants. Trends Plant Sci. 1, 109–113. doi: 10.1016/S1360-1385(96)90004-7
Takabayashi, J., Noda, T., and Takahashi, S. (1991). Plants produce attractants for Apanteles kariyai, a parasitoid of Pseudaletia separata; cases of “communication” and “misunderstanding” in parasitoid-plant interactions. Appl. Entomol. Zool. 26, 237–243. doi: 10.1303/aez.26.237
Takabayashi, J., and Shiojiri, K. (2019). Multifunctionality of herbivory-induced plant volatiles in chemical communication in tritrophic interactions. Curr. Opin. Insect Sci. 32, 110–117. doi: 10.1016/j.cois.2019.01.003
Takabayashi, J., Takahashi, S., Dicke, M., and Posthumus, M. A. (1995). Developmental stage of herbivore Pseudaletia separata affects production of herbivore-induced synomone by corn plants. J. Chem. Ecol. 21, 273–287. doi: 10.1007/BF02036717
Thanikkul, P., Piyasaengthong, N., Menezes-Netto, A. C., Taylor, D., and Kainoh, Y. (2017). Effects of quantitative and qualitative differences in volatiles from host- and non-host-infested maize on the attraction of the larval parasitoid Cotesia kariyai. Entomol. Exp. Appl. 163, 60–69. doi: 10.1111/eea.12555
Turlings, T. C. J., and Erb, M. (2018). Tritrophic interactions mediated by herbivore-induced plant volatiles: mechanisms, ecological relevance, and application potential. Annu. Rev. Entomol. 63, 433–452. doi: 10.1146/annurev-ento-020117-043507
Turlings, T. C. J., Gouinguené, S., Degen, T., and Fritzsche-Hoballah, M. E. (2002). “The chemical ecology of plant-caterpillar-parasitoid interactions,” in Multitrophic Level Interactions, eds T. Tscharntke, and B. Hawkins (Cambridge: Cambridge University Press), 148–173.
Turlings, T. C. J., and Wäckers, F. (2004). “Recruitment of predators and parasitoids by herbivore-injured plants,” in Advances in Insect Chemical Ecology, eds R. T. Cardé (Cambridge: Cambridge University Press), 21–75. doi: 10.1017/CBO9780511542664.003
Vet, L., and Dicke, M. (1992). Ecology of infochemical use by natural enemies in a tritrophic context. Annu. Rev. Entomol. 37, 141–172. doi: 10.1146/annurev.en.37.010192.001041
Visser, J. H., and Avé, D. A. (1978). General green leaf volatiles in the olfactory orientation of the Colorado beetle, Leptinotarsa decemlineata. Entomol. Exp. Appl. 24, 243–247. doi: 10.1111/j.1570-7458.1978.tb02838.x
Vosteen, I., van den Meiracker, N., and Poelman, E. H. (2019). Getting confused: learning reduces parasitoid foraging efficiency in some environments with non-host-infested plants. Behav. Ecol. 189, 919–930. doi: 10.1007/s00442-019-04384-2
Vosteen, I., van den Meiracker, N., and Poelman, E. H. (2020). Gone with the wind: low availability of volatile information limits foraging efficiency in downwind-flying parasitoids. Anim. Behav. 165, 59–70. doi: 10.1016/j.anbehav.2020.04.025
Keywords: Braconidae, intact plant volatiles, HIPVs, tri-trophic interactions, wind tunnel, 4-arm olfactometer
Citation: Ross S, Matsuyama S, Furukawa S, Kuramitsu K and Kainoh Y (2023) Aversive effects of volatiles from intact plants help to fine tune host-searching behavior of the parasitoid wasp, Cotesia kariyai. Front. Ecol. Evol. 11:1118905. doi: 10.3389/fevo.2023.1118905
Received: 08 December 2022; Accepted: 17 April 2023;
Published: 17 May 2023.
Edited by:
Joachim Ruther, University of Regensburg, GermanyReviewed by:
James D. Blande, University of Eastern Finland, FinlandIlka Vosteen, Georg-August University Göttingen, Germany
Copyright © 2023 Ross, Matsuyama, Furukawa, Kuramitsu and Kainoh. This is an open-access article distributed under the terms of the Creative Commons Attribution License (CC BY). The use, distribution or reproduction in other forums is permitted, provided the original author(s) and the copyright owner(s) are credited and that the original publication in this journal is cited, in accordance with accepted academic practice. No use, distribution or reproduction is permitted which does not comply with these terms.
*Correspondence: Yooichi Kainoh, a2Fpbm9oLnlvb2ljaGkuZ2ZAdS50c3VrdWJhLmFjLmpw