- 1Museu Nacional, Departamento de Geologia e Paleontologia, Federal University of Rio de Janeiro, Rio de Janeiro, RJ, Brazil
- 2ICHNOS Research Group, Geology Graduate Program, Unisinos University, São Leopoldo, RS, Brazil
- 3Programa de Pós-Graduação em Ecologia e Recursos Naturais (PPGERN), Universidade Federal de São Carlos (UFSCar—São Carlos Campus), São Carlos, SP, Brazil
- 4Laboratório de Paleobiologia e Astrobiologia (LPA), Departamento de Biologia (DBio), Universidade Federal de São Carlos (UFSCar—Sorocaba Campus), Sorocaba, SP, Brazil
- 5Faculdade de Ciências de Bauru, Universidade Estadual Paulista Júlio de Mesquita Filho, Bauru, SP, Brazil
- 6Centro de Tecnologia e Geociências, Universidade Federal de Pernambuco, Recife, PE, Brazil
- 7Laboratório de Geologia Sedimentar, Departamento de Geologia, Universidade Federal do Rio de Janeiro, Rio de Janeiro, RJ, Brazil
Trilobites inhabited all environments of Paleozoic seas, ranging from estuaries to continental slopes, and were globally distributed. Although their functional morphology and phylogenetic relations are established by well-preserved body fossils, the behavior of trilobites has received less attention. Three well-known trace fossils are interpreted to be produced by trilobitomorphs when preserved in Paleozoic rocks, Rusophycus (a resting trace), Cruziana (a furrowing trace), and Diplichnites (a locomotion trace). Those trace fossils unveil some aspects of trilobite behavior, but they were not investigated to test paleoecologic strategies based on morphometric parameters. This study uses Rusophycus to access the paleoecologic strategies of trilobites in storm-dominated shallow marine deposits of the Pimenteira and Cabeças formations (Middle to Upper Devonian, Parnaíba Basin, Brazil). It was conducted a detailed analysis of the Rusophycus specimens in a section that represents the transition between the Pimenteira and Cabeças formations (Parnaíba Basin). The width and length of the Rusophycus were measured, and statistical analyses were performed to understand the population characteristics. Relatively small-sized Rusophycus are dominant in such deposits, suggesting the dominance of young tracemakers and inferred r-strategist populations. The here reported multiple-Rusophycus assemblage reveals paleoecologic strategies of the population, and tiers relationship (cross-cutting epistratal and shallow-tier trace fossils such as Bergaueria, Palaeophycus, and Protopaleodictyon) indicate deep Rusophycus. The main reason for those burrowing activities deep in the substrate might be protection during ecdysis. Thus, the random distribution, lack of hunting evidence, and depth of Rusophycus suggest molting activity as the trigger for their production in storm-influenced beds of the Pimenteira Formation, probably by calmoniids or homalonotids.
1. Introduction
Trilobites are among the most prominent Paleozoic tracemakers that inhabited worldwide almost all marine settings, from estuaries to the continental slope (Fortey, 2014; Mángano et al., 2021). Their well-preserved fossils have helped to establish their functional morphology and phylogenetic relations. However, ethological studies based on trilobite trace fossils have received little attention (Seilacher, 2007), although trilobites are among the taxa with the greatest behavior representation in the fossil record (Hsieh and Plotnick, 2020). Three classical ichnogenera are assumed to be produced by trilobites when preserved in lower Paleozoic rocks, Rusophycus (a resting trace), Cruziana (a furrowing trace), and Diplichnites (a locomotion trace). The latter is rarely preserved because depositional processes, such as waves and currents, easily obliterate or remove the trackways. Cruziana and Rusophycus, otherwise, are preserved as they represent burrows produced in response to a combination of feeding, locomotion, and resting habits (Crimes and Herdman, 1970; Goldring, 1985; Brandt, 2007; Seilacher, 2007). In some cases, Cruziana and Rusophycus may occur as connected or representing a continuous, making it challenging to distinct between the ichnogenera (Keighley and Pickerill, 1996). Pickerill (1995) suggested that a length/width ratio lower than 2:1 could be applied to identify Rusophycus and a ratio over 2:1 to identify Cruziana.
Rusophycus specimens can occur isolated or form multiple-Rusophycus assemblages (Brandt, 2007). In multiple-Rusophycus assemblages, Rusophycus may intergrade with Cruziana, suggesting a feeding strategy of a single individual that possibly alternates detritus-feeding (Cruziana) with forays (Rusophycus) for prey (Brandt, 2007). However, multiple-Rusophycus assemblages might be produced by different individuals randomly or nonrandomly distributed. Brandt (2007) stated that random distribution occurs in resource-rich, low-energy environments. Non-randomly distributed multiple traces were interpreted as reflecting a rheotactic behavior, in which trilobites oriented themselves against the current to passively capture organic matter (Crimes and Herdman, 1970; Pickerill, 1995), or a circling behavior reflecting a mode of sediment feeding (Neto de Carvalho, 2006).
Trilobites have been reported in Devonian strata of the Parnaíba Basin (Kegel, 1953; Castro, 1968; Carvalho et al., 1997; Meira et al., 2016); however, there has been a lack of ethological studies based on their trace fossils. Considering that Rusophycus dimensions might evidence ontogenetic phases and paleoecologic strategies of trilobites (Levi-Setti, 1995), this study aims to (i) discuss the preservational bias represented by multiple-Rusophycus assemblages in a storm-dominated setting, and (ii) infer the paleoecological strategies of trilobites in this context.
2. Geological setting
The study area is located on the eastern border of the Parnaíba Basin, in Picos Municipality (Figure 1). The intracratonic Parnaíba Basin covers an area of approximately 600,000 km2, reaching ~3,500 m thick in the depocenter (Góes and Feijó, 1994; Milani and Zal’An, 1999). Vaz et al. (2007) divided the stratigraphic record of the basin into five supersequences. The deposits studied herein belong to the Devonian–Mississippian Supersequence, comprising siliciclastic deposits of the lithostratigraphic Canindé Group. This unit is represented by storm-influenced shelf, deltaic, and glacial deposits, recording deposition in shallow marine environments (Barbosa et al., 2015).
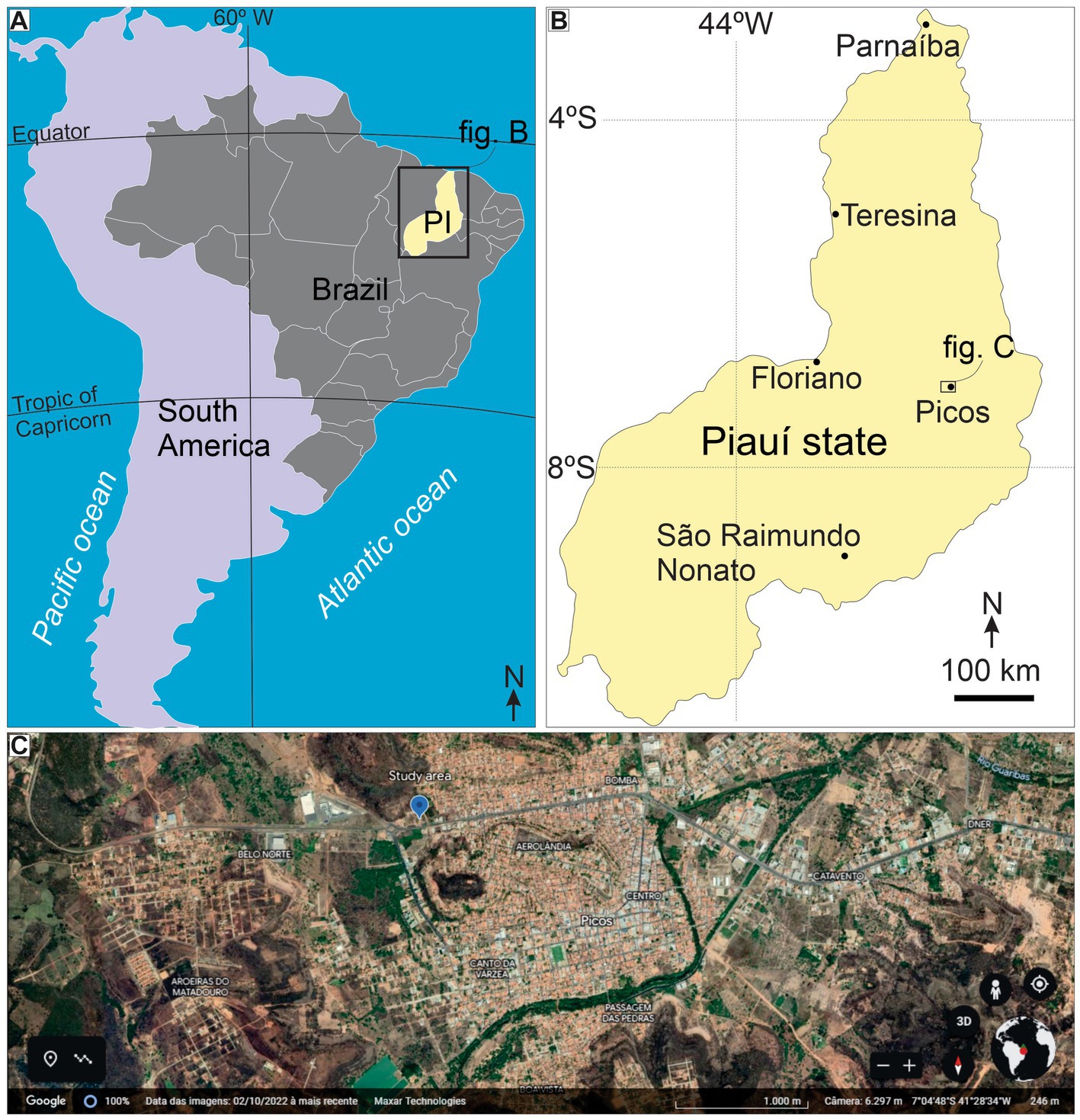
Figure 1. Location of the study area. (A) South America Overview. (B) Position of Picos at Piauí state. (C) Location of study area at Picos City (adapted from Google Earth).
Lithostratigraphically, the Canindé Group is divided into four units, from base to top: Itaim, Pimenteira, Cabeças, and Longá formations (Góes and Feijó, 1994; Vaz et al., 2007). Grahn et al. (2008) dated the transition between the Pimenteira and Cabeças formations, where the Rusophycus specimens studied here are preserved, as Givetian and lower Fransnian. The Pimenteira Formation comprises dark gray to black shales interbedded with thin beds of very fine-grained sandstone (Góes and Feijó, 1994; Young, 2003). In contrast, the Cabeças Formation is dominated by sandstones representing storm-influenced and glacial beds (Vaz et al., 2007; Vettorazzi, 2012; Barbosa et al., 2015).
In the studied section it was recognized eight sedimentary facies (Table 1; Figure 2), from proximal (ME1) to distal (ME6) marine environments: (M1) Facies Sh and Sl are represented by stratified (horizontal stratification or low angle cross-stratification), very fine- to medium-grained sandstone, generally low bioturbated, deposited in shoreface settings; (ME2) facies Sw, represented by very fine- to fine-grained sandstone with wave cross-lamination locally with asymmetric ripples, rarely bioturbated, deposited in shoreface settings; (ME3) facies St and Sp characterized by fine- to medium-grained sandstone bearing trough or planar cross-stratification, with low bioturbation, representing deposition in shoreface settings; (ME4) facies Shcs characterized by interbedded, very fine-grained sandstone with hummocky cross-stratification and siltstone, locally highly bioturbated, reflecting a mix of suspension and tractive processes and indicating transitional offshore settings; (ME5) facies F characterized by moderately bioturbated heterolithic deposits alternating siltstone and fine-grained sandstone showing parallel lamination and locally lenticular to wavy bedding, rich in plant debris, reflecting deposition in upper offshore settings close to storm-wave base; and (ME6) facies M represented by parallel-laminated siltstone with low to locally high bioturbation, locally with plant debris, reflecting deposition in relatively quiet environments in offshore settings.
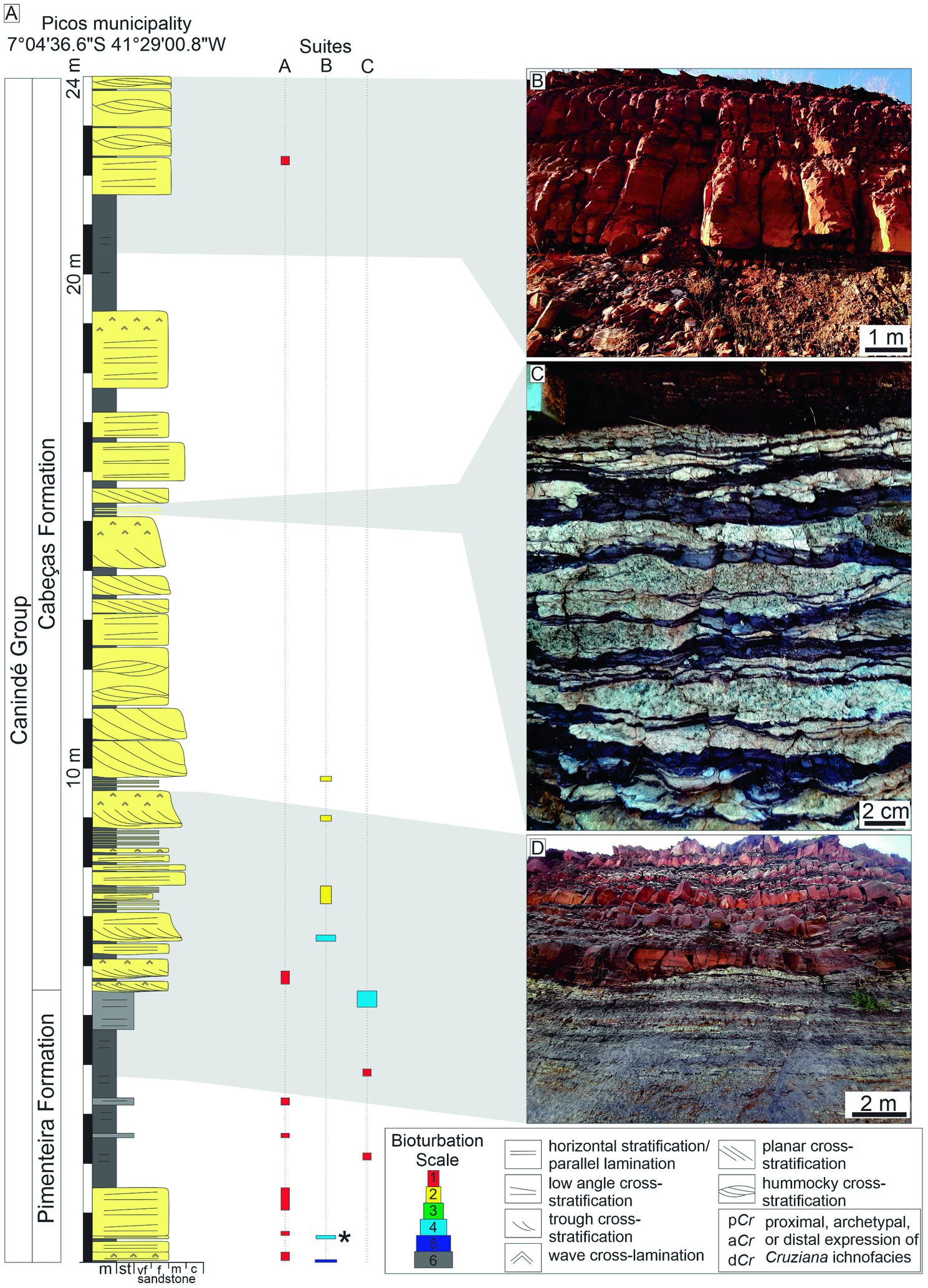
Figure 2. Sedimentary facies and geologic context of studies section. (A) Geologic section and ichnofacies distribution of studied section. (B) General view of fine-grained sandstone with hummocky cross-stratification from the topmost section. (C) Detail of interbedded siltstones and fine-grained sandstone in wavy bedding. (D) Erosive contact between fine-grained sandstone and siltstones. *Level with multiple-Rusophycus.
3. Materials and methods
The studied section is in Picos Municipality, Piauí State, Brazil (7°04′36.3″ S, 41°29′00.9″ W; Figure 1) and represents the transition between the Pimenteira and Cabeças formations (see Figure 2A). Trace fossil characterization used the ichnotaxobases approach (Bromley, 1996). We measured the length and width of all Rusophycus specimens preserved in very fine- to fine-grained sandstone available in the study area (n = 70; see Supplementary Table S1). All Rusophycus specimens used for statistical analysis came from the same bed. For facies analysis, we considered texture, general composition, primary sedimentary structures, trace fossils, geometry, and fossils.
In order to investigate the trends of colonization, five different tests were performed on the data: (i) modality, to verify the existence of more than one mode; (ii) normality, to observe whether the inferred population follows a normal Gaussian distribution; (iii) a skewness test, to verify trends toward a larger number of individuals in a certain size; (iv) density estimates of multi-distribution of length using Gaussian mixture models, and (v) linear regression to observe the trend between lengths vs. width in the ichnospecimens. The Gaussian mixture model was adapted based on the method developed for trilobite (Triarthrus eatoni) analysis by Pauly and Holmes (2022).
The statistical analysis was produced in the R (R Core Team, 2013; v. 4.2.0) programming language, using the integrated development environment RStudio (RStudio Team, 2022; v. 2022.02.2). The “readxl” (Wickham and Bryan, 2017) package was used to support the import of Excel files (“.xlsx”) into R. Graphics were produced using the “ggplot2” (Wickham, 2016) and “ggpubr” (Kassambara, 2020) packages, while data organization and manipulation were performed with the “dplyr” (Wickham et al., 2022) package. The “multimode” (Ameijeiras-Alonso et al., 2021) package was used for the HH (Hartigan and Hartigan) test to determine the number of modes, “stats” (R Core Team, 2013) to do the Shapiro–Wilks and fitting of linear models, and “moments” (Komsta and Novomestky, 2022) package was employed for the skewness test. Finally, “mclust” (Scrucca et al., 2016) package was used to fit Gaussian mixture models to investigate multi-distribution patterns. R Script can be found in Supplementary data 2.
4. Results
The trace fossil content of the section can be subdivided into three suites. Suite A is characterized by low bioturbated beds with Arenicolites, Asterosoma, Palaeophycus, Rosselia, Rhizocorallium, Skolithos, and Thalassinoides (Figures 3A–D). Suite B, the focus of this study, is represented by Arenicolites, Bergaueria, Cruziana, Diplichnites, Diplocraterion, Helminthopsis, Lockeia, Palaeophycus, Protopaleodyction, Rusophycus, and Skolithos (Figures 3E–H, 4), showing moderate to high bioturbation in the bedding plane. Rusophycus and Protopaleodyction dominate suite B. Suite C comprise Chondrites, Phycosiphon, and Planolites (Figure 3I), with low to locally moderate bioturbation.
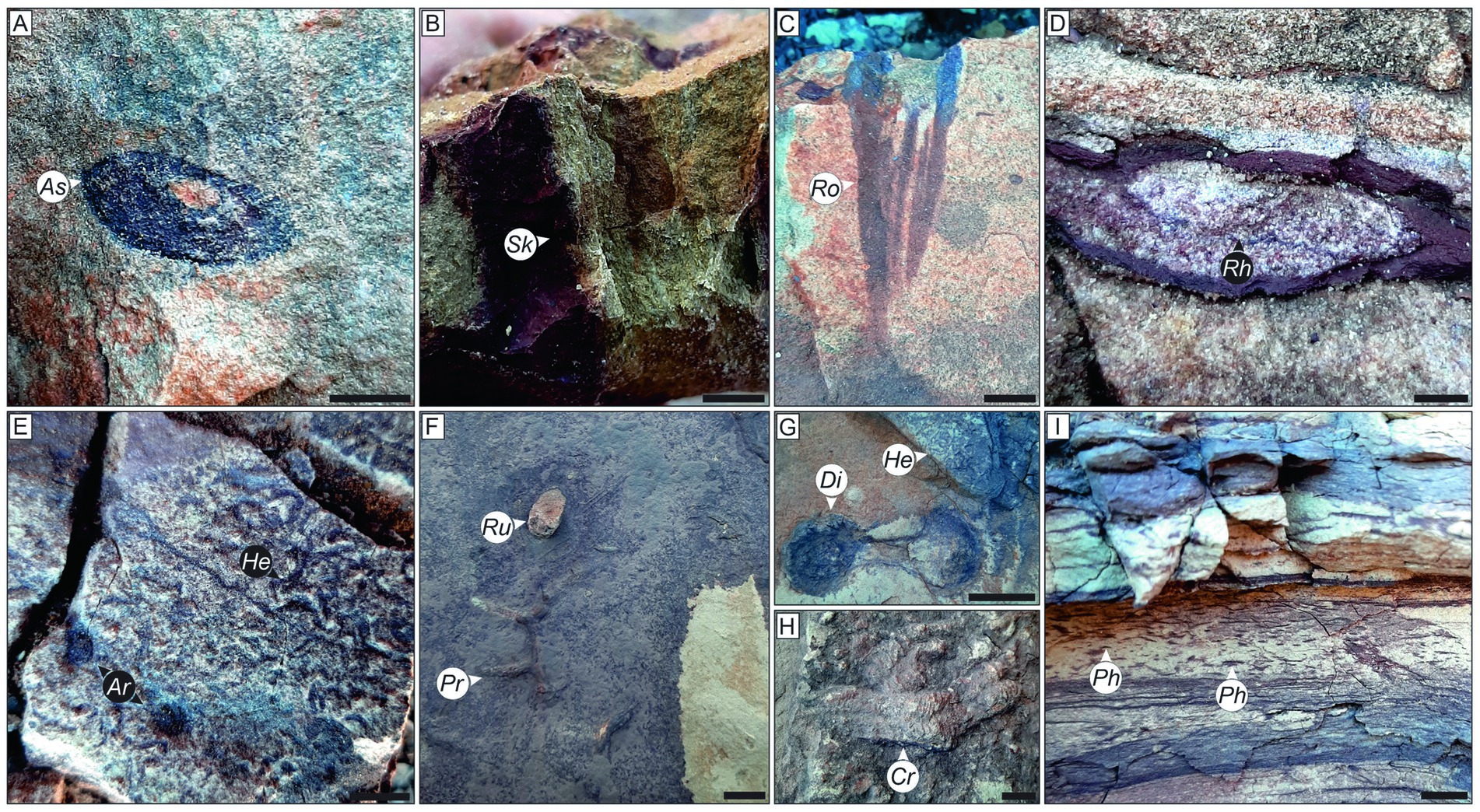
Figure 3. Trace fossil suites from studied section. (A–D) Suite A with Asterosoma (As), Skolithos (Sk), Rosselia (Ro), and Rhizocorallium (Rh). (E–H) Trace fossils from suite B, focus of this study, with Arenicolites (Ar), Helminthopsis (He), Rusophycus (Ru), Protopaleodyction (Pr), Diplocraterion (Di), and Cruziana (Cr). (I) Suite C represented by Phycosiphon (Ph). Scale bar = 1 cm.
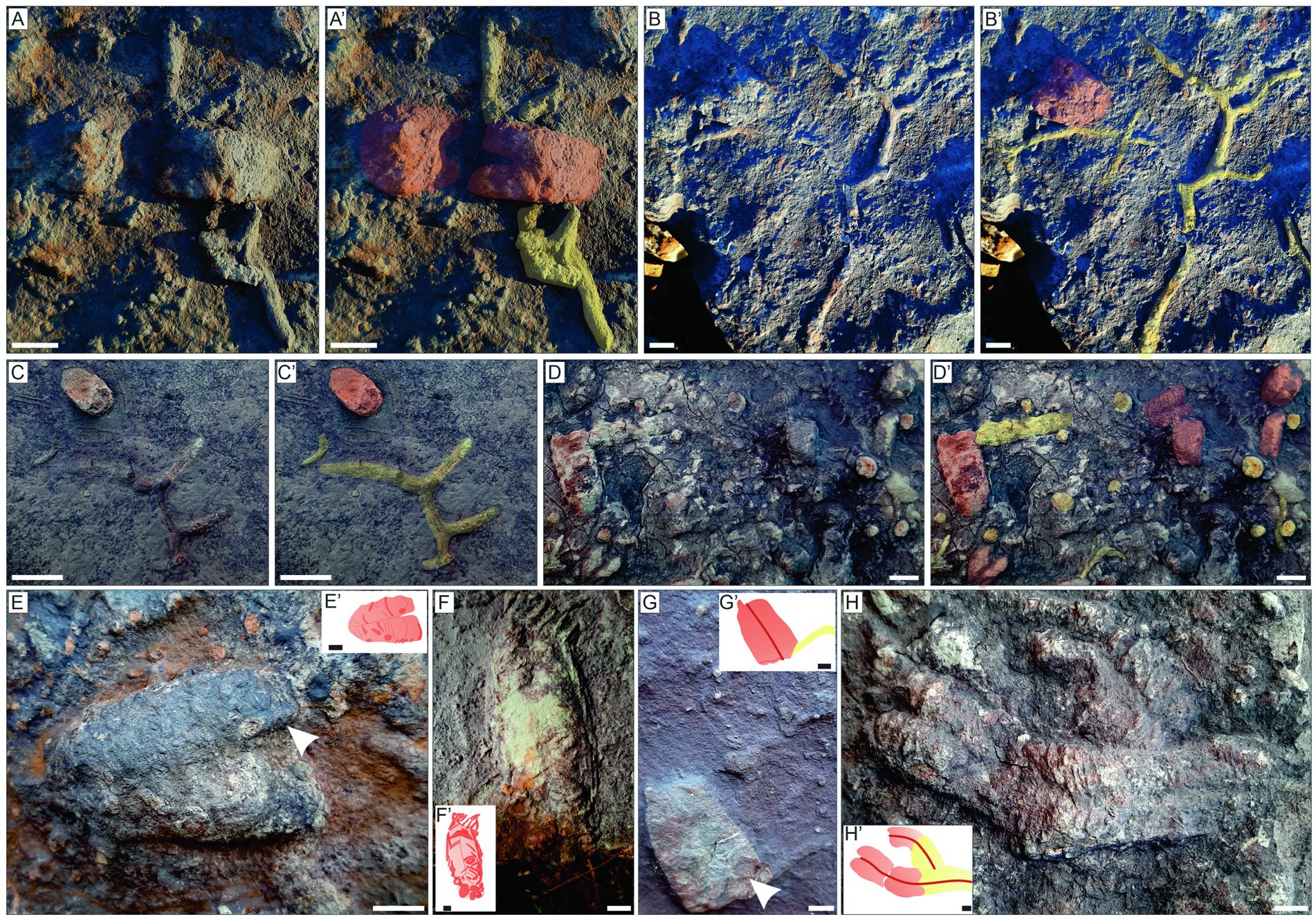
Figure 4. Details of Rusophycus from the studied section. (A–C,A′–C′) Rusophycus (highlighted in red) in association with Protopaleodyction (highlighted in yellow). (D,D′) Rusophycus (highlighted in red) in association with Bergaueria and Palaeophycus (highlighted in yellow). (E–G,E′–G′) Detail of the morphology of Rusophycus. Rusophycus also overlaps Palaeophycus (white arrow) in (E,G). (H,H′) Rusophycus (highlighted in red) in association with Cruziana (highlighted in yellow). Scale bars = 2 cm.
The Rusophycus specimens are preserved in positive hyporelief (sensu Seilacher, 1970) in the sandy beds interbedded to the fine-grained facies (mudstones to siltstones). They are bilobed ovate traces presenting a central groove that separates two symmetrical lobes ornamented by scratches (Figures 4B–E). Their widths range from 14 to 87 mm, and lengths from 21 to 155 mm (Supplementary Table S1; Figure 5). Considering that most of the investigated specimens were found in moved blocks (ex situ), their azimuthal orientation was not measured. However, no preferential orientation is evident in blocks showing more than 10 specimens. Multiple-Rusophycus assemblages occur with several specimens preserved. Rusophycus can locally intersect or overlap Protopaleodyction and Palaeophycus (Figure 4) or be associated with Bergaueria (Figures 4D,D′), all in the same stratigraphic level. It also occurs forming continuous traces with Cruziana (Figures 4H,H′).
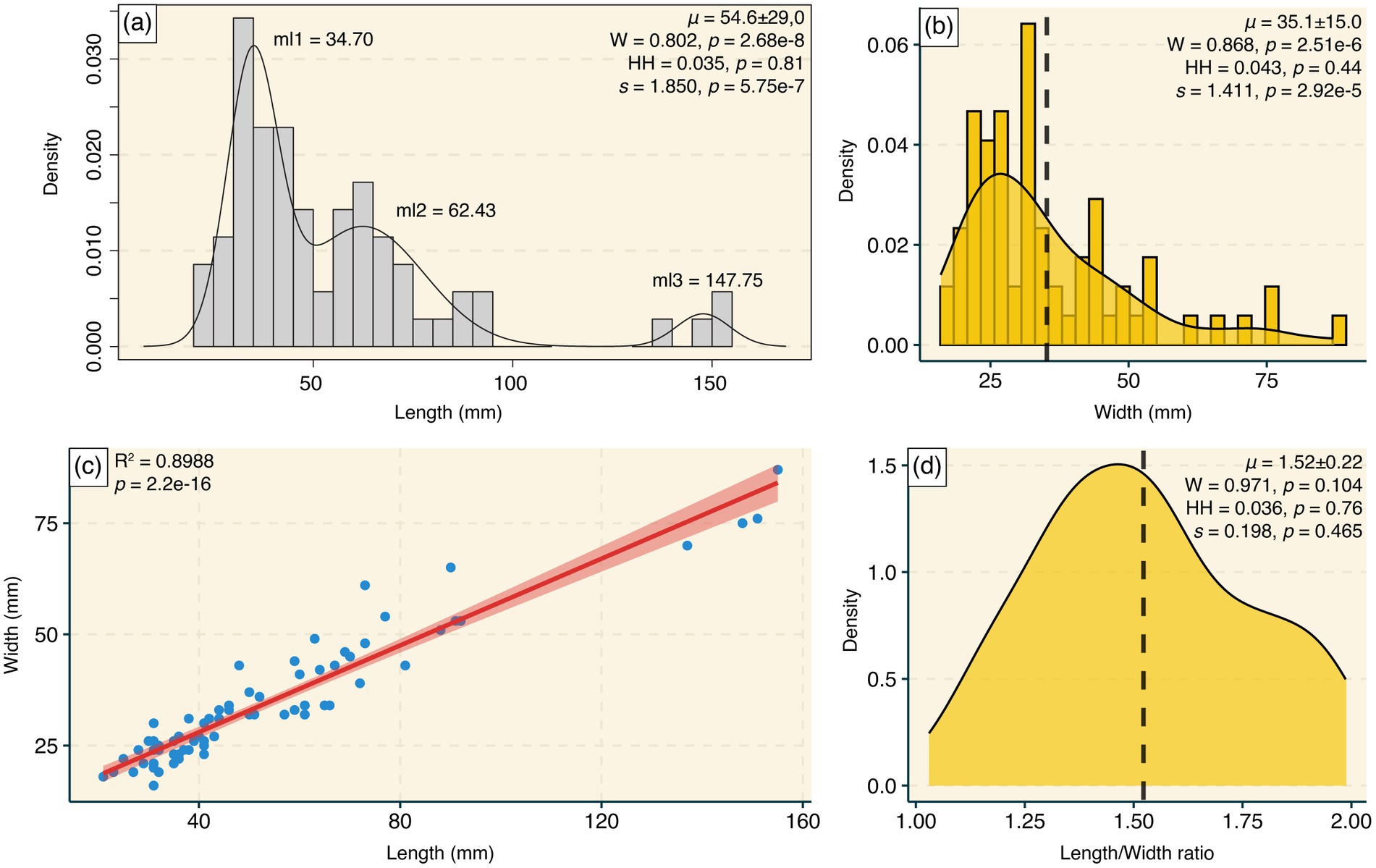
Figure 5. (A) Histogram of the length of the Rusophycus ichnospecimens, with the inclusion of the Gaussian fit model (density line), with three groupings of components (ml1–3). (B) Histogram and density plot of the width (mm) of Rusophycus specimens. (C) Scatterplot with linear regression (red line), showing a high correlation between length and width of the ichnospecimens. (D) Density plot of the length/width ratio. It is possible to observe a population trend of smaller organisms in (A) and (B), and the accumulation of smaller specimens in the scatterplot. The positive skewness visually corroborates the a and b density plots. Vertical dashed line, mean; ml1–3, mean length of the Gaussian components; μ, population mean; W, normality test; HH, multimodality test; s, skewness; R2, coefficient of determination; p, value of p.
The width and length of the specimens (Figures 5A,B) do not follow a normal distribution (Shapiro–Wilk; W = 0.868, p = 2.51e−6 for width, W = 0.802, p = 2.68e−8 for length), indicating only one mode (Hartigan and Hartigan; Dip = 0.043, p = 0.44 for width, Dip = 0.035, p = 0.81 for length). These data have a positive skewness (D’Agostino; skew = 1.411, p = 2.92e−5 for width, skew = 1.850, p = 5.75e−7 for length), showing a trend toward a population with more individuals of small width and a predominance of short lengths. This trend toward a population with more small individuals is also evident in the multi-distribution analysis and the scatter-plot regression (Figures 5A,D). We used a density estimate of the Gaussian mixture model to observe the multi-distribution in the length of the ichnospecimens, and the analysis suggested three main components (ml1−3) with means of 34.70 mm (ml1), 62.43 mm (ml2), and 147.75 mm (ml3).
The length/width ratio was also examined to determine if it could be used to distinguish between different animal groups producing the same ichnogenus (Figure 5D). According to the results, the data follow a normal distribution (W = 0.971, p = 0.104). There is a small peak related to specimens with a greater length/width ratio (Figure 5D), but there is no statistical basis for attesting that there is more than one mode in the data (Dip = 0.036, p = 0.76), and no skewness was observed (skew = 0.198, p = 0.465). The normality, unimodality, lack of skewness, and the prevalence of a 1.5:1 length/width ratio (μ = 1.52 ± 0.22) in the majority of Rusophycus specimens suggest that these traces were most likely produced by the same trilobite species, representing a population. A strong linear correlation was found between the length and width of the specimens (R2 = 0.8988, p = 2.2e−16).
5. Discussion
The three trace fossil suites registered in the study area, A, B, and C, represent different expressions of the Cruziana ichnofacies. Suite A is considered a proximal expression of Cruziana ichnofacies because it presents a mix of shallow-tier, suspension-feeding, or domicile trace fossils (e.g., Arenicolites, Palaeophycus, Skolithos, and Thalassinoides) and shallow- to middle-tiers, detritus-feeding trace fossils (e.g., Asterosoma, Rosselia; MacEachern et al., 2007; Sedorko et al., 2018c), suggesting colonization dominantly in lower shoreface settings. Suite B is interpreted as archetypal Cruziana ichnofacies due to the higher ichnodiversity and variation on preserved behaviors, such as resting traces (Bergaueria, Lockeia, and Rusophycus), locomotion (Cruziana and Diplichnites), grazing activity (Helminthopsis and Protopaleodyction), dwelling or suspension-feeding (Arenicolites, Palaeophycus, and Skolithos), and equilibrium (Diplocraterion); they present moderate to high bioturbation degree and were produced in fine-grained substrates, but often cast by overlying sandy strata, suggesting colonization in a transitional offshore zone (MacEachern et al., 2007; Sedorko et al., 2018a). Lastly, Suite C represents low-energy settings, with deposit-feeding habits (e.g., Chondrites, Phycosiphon, and Planolites) in offshore settings (MacEachern et al., 2007; Sedorko et al., 2018b). Thus, in the transition between the Pimenteira and Cabeças formations, the Cruziana ichnofacies dominate in a lower shoreface to offshore setting, and the trilobites had their trace fossils preferentially preserved in an offshore transition zone.
Rusophycus has been recorded in the Lower Paleozoic beds of the Parnaíba Basin, particularly in the Pimenteira Formation. However, none of the known ichnospecies shows the same morphological features that characterize the analyzed specimens. Rusophycus polonica Seilacher (1970) shows a deep oval central area and thicker ridges (Correa et al., 2004). Rusophycus dispar Linnarsson, 1869 is characterized by a heart shape and scratches in several directions (Gracioso, 2011). Rusophycus biloba Vanuxem, 1842 presents thick bifid transverse scratches and united posterior and anterior margins (Silva et al., 2012). Rusophycus piauiensis Agostinho et al. (2004) described in the Longá Formation, shows a smaller length/width ratio and thicker striae when present (Muniz, 1982). Considering that not all observed Rusophycus in this study bear a clear pattern of scratches, we treat them at the ichnogeneric level.
The statistical analysis of the studied specimens revealed three components (grouping) of sizes (Figure 5A), indicating different ontogenetic stages of burrowing trilobites. In the early ontogenetic phases (protaspis and meraspid), trilobites were extremely small (less than 1 or 2 mm), hampering a prominent ichnologic record. This situation changes when they reach the holaspis phase and assume a burrowing behavior (Seilacher, 2007). Smaller tracemakers dominate the analyzed suite (Suite B), suggesting a typical form for r-strategists’ populations (Bromley, 1996; Ruppert et al., 2005). The dominance of small organisms in storm-influenced strata points to the prevalence of opportunistic strategies in a stressful substrate, possibly due to high hydrodynamic levels and short colonization windows (sensu Pollard et al., 1993). The high depositional frequency is also corroborated by the occurrence of Diplocraterion, both protrusive and retrusive in the same bed, suggesting frequent vertical replacement of the infauna (Buatois and Mángano, 2011).
The growth pattern observed in trilobite species in the fossil record is relatively continuous (e.g., Kim et al., 2002; Crônier, 2003; Park and Choi, 2009; Shen et al., 2014; Hou et al., 2017; Pauly and Holmes, 2022; Dai et al., 2023), and this trend is also evident in the size patterns of Rusophycus from the studied section (see Figure 5C, R2 = 0.8988). The Rusophycus ichnospecimens show a pattern of greater prolongation in length than the width (Figure 5C), possibly due to the accumulation of new segments in post-protaspid trilobites (Hughes, 2003; Hughes et al., 2006), leading to slightly greater growth in length than in width.
The population trend of smaller organisms is a common phenomenon in trilobite species, as observed in previous studies (Sheldon, 1988; Kim et al., 2002; Crônier, 2003; Park and Choi, 2009; Shen et al., 2014; Hou et al., 2017; Pauly and Holmes, 2022; Dai et al., 2023). This trend is characterized by a higher number of smaller individuals, and as the size increases, the number of individuals decreases continuously, which is a typical trait of r-strategist species (Pianka, 1970) and type III survivorship curves (Demestrius, 1978).
In this work, the analysis of Rusophycus reveals a similar pattern of size abundance as that observed in trilobite species (see Figure 5 and positive skewness). This is consistent with the survival pattern of trilobites, where many die in the process of protaspis and small meraspids stages, with few reaching the larger holaspid stage (Cisne, 1973; Brezinski, 1986; Sheldon, 1988; Shen et al., 2014; Hou et al., 2017; Pauly and Holmes, 2022; Dai et al., 2023). This is similar to a type III survivorship curve (Demestrius, 1978). Our analysis also revealed three different groups of components in the length of the Rusophycus specimens (Figure 5A). This pattern of “pulses” of size has been observed in trilobite species (see Cisne, 1973), and reevaluation of the data by Brezinski (1986) and Pauly and Holmes (2022) could be related to different instars in trilobite tracemakers (Hunt and Chapman, 2001) resulting in Rusophycus of different sizes.
Trilobites are generally considered marine organisms, although some trace fossils attributed to trilobites were found in estuarine settings (Mángano et al., 2021). In the studied area, the Rusophycus occur at the bottom of a storm-influenced bed but were generated in the fine-grained deposits of facies F and M, characterizing pre-depositional colonization.
The dominance of Rusophycus in an ichnoassemblage, as observed in Suite B, has been interpreted as a hunting strategy, in which trilobites hide in shallow burrows to catch detritus or hunt for soft-bodied prey, as reported in other Paleozoic units (e.g., Whittington, 1980; Seilacher, 1985; Jensen, 1990; Brandt et al., 1995; Pickerill and Blissett, 1999; Rydell et al., 2001; Brandt, 2007). However, the close association of Rusophycus with Protopaleodyction in these beds and their overlapping by Palaeophycus (Figures 4A,B,G) indicate that they were relatively deep burrows. Although a carnivorous habit cannot be discharged, in study area there is no record of Rusophycus overlapping a trace fossil that would be produced by some worm-like organism. In addition, there is no record of carnivorous trilobites in the Brazilian sedimentary basins (Carvalho and Ponciano, 2015; Carbonaro et al., 2018).
The depth of the Rusophycus specimens, the random distribution against the currents, the concentration in a single bed, and the presence of different component groups of length (Figure 5A) with positive skewness, allow interpreting the studied Rusophycus as result of a resting behavior related to molting burrow for ecdysis. The trilobites probably excavated deeper in a previously bioturbated substrate for protection during the molting process, as proposed for some burrows attributed to trilobites (Seilacher, 2007). The preservation of Rusophycus in palimpsest preservation with other burrows (as represented in Figure 6) further supports this interpretation.
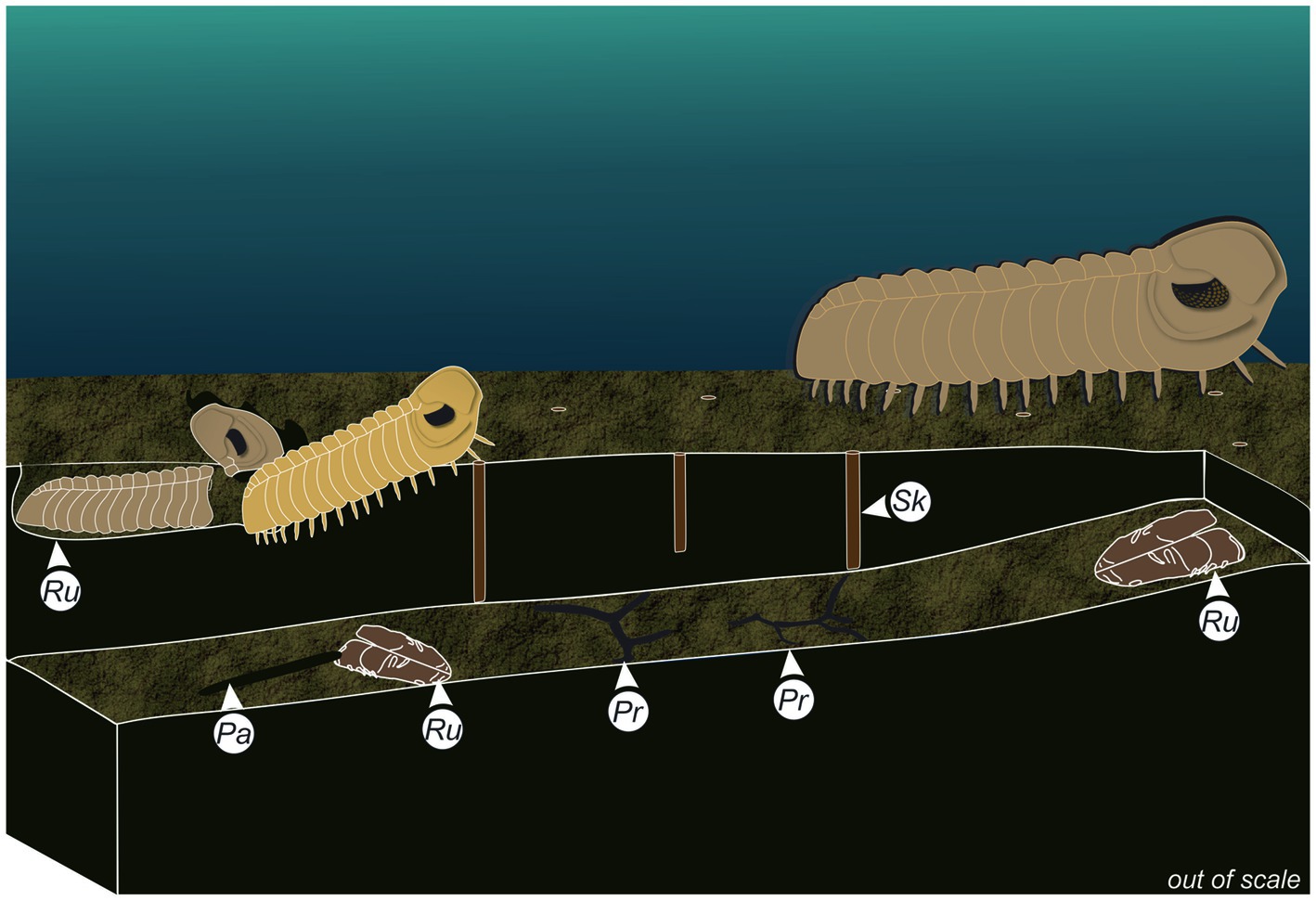
Figure 6. Reconstitution of the molting strategy of trilobites while producing Rusophycus, and tier relation of commonly associated trace fossils. Pr, Protopaleodyction; Ru, Rusophycus; Sk, Skolithos.
The dominance of traces produced by smaller organisms in the Suite B assemblage suggests that trilobites had an opportunistic behavior in offshore transition settings. The prevalence of an r-strategist population in stable environments such as the offshore transition zone indicates punctual ecologically stressing conditions, probably due to salinity fluctuations resulting from episodic continental inputs, as suggested by the presence of combined flow ripples and abundant phytodebris (Ponciano and Della Fávera, 2009). However, the spawning behavior of trilobites naturally implies a high number of small and fewer mature adults in the population, since the option of a reproductive strategy with simultaneous spawning reduces the possibility of individuals within the same cluster being consumed by predators (Hegna et al., 2017). According to Cisne (1973) and the reevaluation of Pauly and Holmes (2022), the spawning of trilobites was seasonal (once a year), resulting in populations of high rates of small organisms compared to holaspis individuals. Although the spawning behavior of trilobites could explain the record of a population with a higher number of young adults compared with the mature ones, there is no evidence of seasonal events in the studied deposits. The erosive nature of the sandy beds that preserved the trilobite burrows suggests episodic storm events. The presence of sedimentary structures that suggest continental input, allied with plant debris, reinforces the hypothesis of sporadic salinity stress as the most parsimonious cause of stress for the studied section.
During molting, arthropods are more vulnerable to potential predators, so burrowing becomes a useful protection strategy for the tracemaker (e.g., Bromley, 1996; Seilacher, 2007). The presence of three different groups of sizes (Figure 5A) reinforces the interpretation of this strategy of burrow during molting (Hunt and Chapman, 2001). Body fossils of homalonotid and calmoniid trilobites have been recorded in the Brazilian Devonian beds (e.g., Simões et al., 2009; Carvalho and Ponciano, 2015), and in some cases there is a record of trilobites (Burmeisteria notica and Metacryphaeus cf. australis = M. meloi) with Rusophycus (Leme et al., 2013). According to Simões et al. (2009), homalonotids occur associated with shallow, sandy subaquatic deposits accumulated just in and/or above the fair-weather wave base zone and calmoniids are more common in muddy facies (flooding surfaces) generated below the storm wave base zone. Therefore, considering this paleoenvironmental distribution, calmoniids might be considered as the main potential tracemaker of the studied Rusophycus specimens, mostly during the molting phase.
However, the Parnaíba Basin has a limited record of trilobites, with only few species from the Calmonidae and Homalonotidae families (Ghilardi and Simões, 2007; Meira, 2014; Carbonaro et al., 2018). The most common genus within Calmonidae is Metacryphaeus, with three species recorded: M. tuberculatus, M. meloi, and M. kegeli (Carvalho et al., 1997; Leme et al., 2013; Meira, 2014; Meira et al., 2016; Carbonaro et al., 2018), along with a record of Eldredgeia cf. venusta (Carvalho and Ponciano, 2015). In the Homalonotidae family, only Burmeisteria notica has been identified (Leme et al., 2013; Meira, 2014), with a width range of 22–40 mm (Leme et al., 2013), similar to the mean width of the Rusophycus specimens studied here (~35 mm). The sizes of the Metacryphaeus species differ, with M. kegeli being the smallest, ranging widthwise from ~7 to 23 mm (Carvalho et al., 1997), M. meloi ranging in width from ~17 to 31 mm (Carvalho et al., 1997; Leme et al., 2013), and M. tuberculatus ranging in width from approximately from 30 to 108 mm, with some complete specimens ranging lengthwise from 75 to 176 mm (Carvalho et al., 1997; Meira et al., 2016). Therefore, it is possible that both calmoniids and homalonotids have produced the Rusophycus traces reported in this study.
6. Final remarks
The trace fossil suites in the studied section are an expression of proximal, archetypal, and distal Cruziana ichnofacies in lower shoreface to offshore settings. The suite with multiple Rusophycus can be interpreted as a pre-depositional suite, generated in the underlying muddy beds, and cast by the sandy sediments carried by storm-generated and combined current flows in transitional offshore to offshore settings. Most Rusophycus can be attributed to a tracemaker in meraspis and few holaspids stages. This distribution suggests an r-strategist population. The random distribution in low energy depositional setting, absence of hunt evidence, and high deep of Rusophycus allowed the assumption that a molting activity is the triggered behavior to the production of Rusophycus in those storm-influenced beds from Pimenteira Formation, while Cruziana represents detritus-feeding strategy.
Data availability statement
The original contributions presented in the study are included in the article/Supplementary material, further inquiries can be directed to the corresponding author.
Author contributions
DS: conceptualization, methodology, formal analysis, investigation, validation, visualization, data curation, writing—original draft, review and editing, and project administration. GB: methodology, software, formal analysis, data curation, writing—original draft, review and editing, and visualization. RN: conceptualization, formal analysis, investigation, validation, writing—original draft, and review and editing. RG: formal analysis, validation, writing—original draft, review and editing, and project administration. SA: methodology, validation, and review and editing. KR: methodology, formal analysis, validation, visualization, and writing—original draft. EFN: methodology, visualization, and writing—original draft. CM: visualization and writing—original draft. LB: methodology, validation, writing—original draft, and review and editing. All authors contributed to the article and approved the submitted version.
Funding
This work was supported by Brazilian Scientific and Technological Research Council—CNPq (159548/2018-7, 153661/2021-6, and 306493/2022-5), Coordination of Superior Level Staff Improvement—CAPES (master’s grant Finance Code 1, 88887.569703/2020-00 and doctorate grant 88887.799772/2022-00), and FAPESP (2020/12409-4).
Acknowledgments
The authors thank Mirian L. A. F. Pacheco, Marcelo Guimarães Simões, and the reviewers for valuable discussions which greatly improved the paper. The authors would like to express their gratitude to Fernanda Quaglio and editorial board for the assistance with the entire editing process. We would also like to thank the financial support of the Brazilian Scientific and Technological Research Council—CNPq (159548/2018-7, 153661/2021-6, and 306493/2022-5), the Coordination of Superior Level Staff Improvement—CAPES (master's grant Finance Code 1, 88887.569703/2020-00, and doctorate grant 88887.799772/2022-00), and FAPESP (2020/12409-4).
Conflict of interest
The authors declare that the research was conducted in the absence of any commercial or financial relationships that could be construed as a potential conflict of interest.
Publisher’s note
All claims expressed in this article are solely those of the authors and do not necessarily represent those of their affiliated organizations, or those of the publisher, the editors and the reviewers. Any product that may be evaluated in this article, or claim that may be made by its manufacturer, is not guaranteed or endorsed by the publisher.
Supplementary material
The Supplementary material for this article can be found online at: https://www.frontiersin.org/articles/10.3389/fevo.2023.1117947/full#supplementary-material
References
Agostinho, S., Viana, M. S. S., and Fernandes, A. C. S. (2004). Duas novas icnoespécies de Bifungites Desio, 1940 na Formação Pimenteira, Devoniano da Bacia do Parnaíba, Brasil. Arq. do Mus. Nac. 62, 519–530.
Ameijeiras-Alonso, J., Crujeiras, R. M., and Rodriguez-Casal, A. (2021). multimode: An R Package for Mode Assessment. J. Stat. Softw. 97, 1–32. doi: 10.18637/jss.v097.i09
Barbosa, R. C. M., Nogueira, A. C. R., and Domingos, F. H. G. (2015, 2015). Famennian glaciation in the eastern side of Parnaíba Basin, Brazil: evidence of advance and retreat of glacier in Cabeças Formation. Brazil J Geol 45:147. doi: 10.1590/2317-4889201530147
Brandt, D. S. (2007). Multiple-Rusophycus (arthropod ichnofossil) assemblages and their significance. Ichnos 15, 28–43. doi: 10.1080/10420940701192898
Brandt, D. S., Meyer, D. L., and Lask, P. B. (1995). Isotelus (trilobita) “hunting burrow” from Upper Ordovician strata. J. Paleo. 69, 1079–1083. doi: 10.1017/S0022336000038063
Brezinski, D. K. (1986). An opportunistic Upper Ordovician trilobite assemblage from Missouri. Lethaia 19, 315–325. doi: 10.1111/j.1502-3931.1986.tb00746.x
Bromley, R. G. (1996). Trace fossils: Biology, taphonomy and applications. New York, USA: Springer-Science+Business Media, B.V.
Buatois, L. A., and Mángano, M. G. (2011). Ichnology: Organism-substrate interactions in space and time. Cambridge University Press, Cambridge, UK.
Carbonaro, F. A., Langer, M. C., Nihei, S. S., Ferreira, G. S., and Ghilardi, R. P. (2018). Inferring ancestral range reconstruction based on trilobite records: a study-case on Metacryphaeus (Phacopida, Calmoniidae). Sci. Rep. 8:15179. doi: 10.1038/s41598-018-33517-5
Carvalho, M. P., Edgecombe, G. D., and Lieberman, B. S. (1997). Devonian Calmoniid Trilobites from the Parnaiba Basin, Piaui State, Brazil. Am. Mus. Novit. 3192, 1–12.
Carvalho, M. G. P., and Ponciano, L. C. M. O. (2015). The Devonian trilobites of Brazil: A summary. J. South Am. Earth Sci. 64, 217–228. doi: 10.1016/j.jsames.2015.10.010
Castro, J. D. S. (1968). Trilobitas da Formação Pimenteiras, Devoniano do estado do Piauí. An. Acad. Bras. Cienc. 40, 481–489.
Cisne, J. L. (1973). Life History of an Ordovican Trilobite Triarthrus eatoni. Ecology 54, 135–142. doi: 10.2307/1934382
Correa, L. M. S. A., Agostinho, S., Fernandes, A. C. S., and Vieira, P. M. (2004). Icnofósseis da Formação Pimenteira (Devoniano da Bacia do Parnaíba), município de Miranorte, Estado do Tocantins, Brasil. Arq. do Mus. Nac. 62, 283–291.
Crimes, T. P., and Herdman, J. (1970). Trilobite tracks and other trace fossils from the Upper Cambrian of North Wales. Geol. J. 7, 47–68.
Crônier, C. (2003). Systematic relationships of the blind phacopine trilobite Trimerocephalus, with a new species from Causses-et-Veyran, Montagne Noire. Acta Palaeontol. Pol. 48, 55–70.
Dai, T., Zhang, X., and Peng, S. (2023). Developmental traits and life strategy of redlichiid trilobites. Biol. Rev. 98, 63–80. doi: 10.1111/brv.12895
Demestrius, L. (1978). Adaptive value, entropy and survivorship curves. Nature 275, 213–214. doi: 10.1038/275213a0
Fortey, R. A. (2014). The palaeoecology of trilobites. J. Zool. 292, 250–259. doi: 10.1111/jzo.12108
Ghilardi, R. P., and Simões, M. G. (2007). “History and Development of Trilobite Research in Brazil Paleontologists” in Fabulous Fossils—300 Years of Worldwide Research on Trilobites. ed. D. G. Mikulic (New York, USA: Illinois State Geological Survey), 97–104.
Góes, A. M. O., and Feijó, F. J. (1994). Bacia do Parnaíba. Boletim de Geociências da Petrobras 8, 57–67.
Goldring, R. (1985). The formation of the trace fossil Cruziana. Geol. Mag. 122, 65–72. doi: 10.1017/S0016756800034099
Gracioso, D. E. (2011). Icnofósseis de invertebrados da Formação Pimenteira (Devoniano) na borda leste da Bacia do Parnaíba. Rio de Janeiro, Brazil: Universidade Federal do Rio de Janeiro.
Grahn, Y., Young, C., and Borghi, L. (2008). Middle Devonian chitinozoan biostratigraphy and sedimentology in the eastern outcrop belt of the Parnaíba Basin, Northeastern Brazil. Revista Brasileira de Paleontologia 11, 137–146. doi: 10.4072/rbp.2008.3.01
Hegna, T. A., Martin, M. J., and Darroch, S. A. F. (2017). Pyritized in situ trilobite eggs from the Ordovician of New York (Lorraine Group): Implications for trilobite reproductive biology. Geology 45, 199–202. doi: 10.1130/G38773.1
Hou, J., Hughes, N. C., Yang, J., Lan, T., Zhang, X., and Dominguez, C. (2017). Ontogeny of the articulated yiliangellinine trilobite Zhangshania typica from the lower Cambrian (Series 2, Stage 3) of southern China. J. Paleo. 91, 86–99. doi: 10.1017/jpa.2016.118
Hsieh, S., and Plotnick, R. E. (2020). The representation of animal behaviour in the fossil record. Anim. Behav. 169, 65–80. doi: 10.1016/j.anbehav.2020.09.010
Hughes, N. C. (2003). Trilobite body patterning and the evolution of arthropod tagmosis. Bioessays 25, 386–395. doi: 10.1002/bies.10270
Hughes, N. C., Minelli, A., and Fusco, G. (2006). The ontogeny of trilobite segmentation: a comparative approach. Paleobiology 32, 602–627. doi: 10.1666/06017.1
Hunt, G., and Chapman, R. E. (2001). Evaluating hypotheses of instar-grouping in arthropods: a maximum likelihood approach. Paleobiology 27, 466–484. doi: 10.1666/0094-8373(2001)027<0466:EHOIGI>2.0.CO;2
Jensen, S. (1990). Predation by early Cambrian trilobites on infaunal worms-evidence from the Swedish Mickwitzia Sandstone. Lethaia 23, 29–42. doi: 10.1111/j.1502-3931.1990.tb01779.x
Kassambara, A. (2020). ggpubr: 'ggplot2' Based Publication Ready Plots. R package version 0.4.0. Available at: https://CRAN.R-project.org/package=ggpubr
Kegel, W. (1953). Contribuição para o estudo do Devoniano da Bacia do Parnaíba. Bol. da Div. Geol. e Mineral. do Dep. Nac. da Produção Miner., 1–48.
Keighley, D. G., and Pickerill, R. K. (1996). Small Cruziana, Rusophycus, and related ichnotaxa from eastern Canada: The nomenclatural debate and systematic ichnology. Ichnos 4, 261–285. doi: 10.1080/10420949609380136
Kim, K., Sheets, H. D., Haney, R. A., and Mitchell, C. E. (2002). Morphometric analysis of ontogeny and allometry of the Middle Ordovician trilobite Triarthrus becki. Paleobiology 28, 364–377. doi: 10.1666/0094-8373(2002)028<0364:MAOOAA>2.0.CO;2
Komsta, L., and Novomestky, F. (2022). moments: Moments, Cumulants, Skewness, Kurtosis and Related Tests. R package version 0.14.1. Available at: https://CRAN.R-project.org/package=moments
Leme, J. M., Meira, F. E., Stasi, A. M., and Soares, S. P. (2013). The occurrence of Phacopida trilobites from Pimenteira Formation at João Costa, Piauí, Brazil. Geologia USP Série Científica 13, 17–22. doi: 10.5327/Z1519-874X201300030003
MacEachern, J. A., Bann, K. L., Pemberton, S. G., and Gingras, M. K. (2007). “The Ichnofacies paradigm: High-resolution paleoenvironmental interpretation of the rock record” in Applied Ichnology, Society for Sedimentary Geology Short Course Notes. eds. J. A. MacEachern, K. L. Bann, M. K. Gingras, and S. G. Pemberton (Oklahoma, USA: SEPM (Society for Sedimentary Geology)) 52, 27–64.
Mángano, M. G., Buatois, L. A., Waisfeld, B. G., Muñoz, D. F., Vaccari, N. E., and Astini, R. A. (2021). Were all trilobites fully marine? Trilobite expansion into brackish water during the early Palaeozoic. Proc. R. Soc. B Biol. Sci. 288, 1–10. doi: 10.1098/rspb.2020.2263
Meira, F. V. E. (2014). Revisão Sistemática e Paleobiogeográfica de Trilobitas Phacopida (Homalonotidae e Calmoniidae) do Devoniano das Bacias do Parnaíba e Amazonas, Brasil. PhD Thesis, University of São Paulo, 184 pp.
Meira, F. V. E., Carbonaro, F. A., Ghilardi, R. P., and Leme, J. D. M. (2016). The “Metacryphaeus tuberculatus Group” (Trilobita, Calmoniidae) from the Devonian of the Parnaíba Basin, Brazil. Am. Demogr. 53, 463–472. doi: 10.5710/AMGH.03.12.2015.2924
Milani, E. J., and Zal’An, P. V. (1999). An outline of the geology and petroleum systems of the Paleozoic interior basins of South America. Episodes 22, 199–205. doi: 10.18814/epiiugs/1999/v22i3/007
Muniz, G. C. B. (1982). “Ichnofósseis Devonianos da Formação Longá, no Estado do Piauí” in Congresso Brasileiro de Geologia (Salvador Brazil: Sociedade Brasileira de Geologia), 1305–1316.
Neto de Carvalho, C. (2006). Roller coaster behavior in the Cruziana rugosa group from Penha Garcia (Portugal): Implications for the feeding program of trilobites. Ichnos 13, 255–265. doi: 10.1080/10420940600843740
Park, T., and Choi, D. K. (2009). Post-embryonic development of the Furongian (late Cambrian) trilobite Tsinania canens: implications for life mode and phylogeny. Evol. Dev. 11, 441–455. doi: 10.1111/j.1525-142X.2009.00350.x
Pauly, D., and Holmes, J. D. (2022). Reassessing growth and mortality estimates for the Ordovician trilobite Triarthrus eatoni. Paleobiology, 1–11. doi: 10.1017/pab.2022.22
Pickerill, R. K. (1995). Deep-water marine Rusophycus and Cruziana from the Ordovician Lotbiniere Formation of Quebec. Atl. Geol. 31, 103–108. doi: 10.4138/2102
Pickerill, R. K., and Blissett, D. (1999). A predatory Rusophycus burrow from the Cambrian of southern New Brunswick, eastern Canada. Atl. Geol. 35, 179–185.
Pollard, J. E., Goldring, R. J. E., and Buck, S. G. (1993). Ichnofabrics containing Ophiomorpha: their significance in shallow-water facies interpretation. J. Geol. Soc. London 150, 149–164. doi: 10.1144/gsjgs.150.1.0149
Ponciano, L. C. M. O., and Della Fávera, J. C. (2009). Flood-dominated fluvio-deltaic system: a new depositional model to Cabeças Formation, Parnaíba Basin, Piauí, Brazil. An. Acad. Bras. Cienc. 81, 769–780. doi: 10.1590/S0001-37652009000400014
R Core Team (2013). R: a language and environment for statistical computing. R Foundation for Statistical Computing. Available at: https://www.R-project.org
RStudio Team (2022). RStudio: Integrated Development Environment for R. RStudio, PBC, Boston, MA. Available at: http://www.rstudio.com/.
Ruppert, E. E., Fox, R. S., and Barnes, R. D. (2005). Zoologia dos Invertebrados. 7th Edn., Ed. Roca, São Paulo, 1145.
Rydell, J., Hammarlund, J., and Seilacher, A. (2001). Trace fossil associations in the Swedish Mickwitzia sandstone (Lower Cambrian): did trilobites really hunt for worms? GFF 123, 247–250. doi: 10.1080/11035890101234247
Scrucca, L., Fop, M., Murphy, T., and Raftery, A. E. (2016). Mclust 5: clustering, classification and density estimation using Gaussian finite mixture models. R J 8, 289–317. doi: 10.32614/RJ-2016-021
Sedorko, D., Bosetti, E. P., and Netto, R. G. (2018a). An integrative ichnological and taphonomic approach in a transgressive-regressive cycle: a case study from Devonian of Paraná Basin, Brazil. Lethaia 51, 15–34. doi: 10.1111/let.12219
Sedorko, D., Netto, R. G., and Horodyski, R. S. (2018b). A Zoophycos carnival in Devonian beds: paleoecological, paleobiological, sedimentological, and paleobiogeographic insights. Palaeogeogr. Palaeoclimatol. Palaeoecol. 507, 188–200. doi: 10.1016/j.palaeo.2018.07.016
Sedorko, D., Netto, R. G., and Savrda, C. E. (2018c). Ichnology applied to sequence stratigraphic analysis of Siluro-Devonian mud-dominated shelf deposits, Paraná Basin, Brazil. J. South Am. Earth Sci. 83, 81–95. doi: 10.1016/j.jsames.2018.02.008
Seilacher, A. (1970). “Cruziana stratigraphy of "nonfossiliferous" Palaeozoic sandstones” in Trace Fossils. eds. T. P. Crimes and J. C. Harper (New York, USA: John Wiley & Sons.) vol. 3, 447–476.
Seilacher, A. (1985). Trilobite palaeobiology and substrate relationships. Earth Environ. Sci. Trans. R. Soc. 76, 231–237.
Sheldon, P. R. (1988). Trilobite size-frequency distributions, recognition of instars, and phyletic size changes. Lethaia 21, 293–306. doi: 10.1111/j.1502-3931.1988.tb01759.x
Shen, C., Clarkson, E. N. K., Yang, J., Lan, T., Hou, J. B., and Zhang, X. G. (2014). Development and trunk segmentation of early instars of a ptychopariid trilobite from Cambrian Stage 5 of China. Sci. Rep. 4, 1–11. doi: 10.1038/srep06970
Silva, R. C., Dominato, V. H., and Fernandes, A. C. S. (2012). Novos registros e aspectos paleoambientais dos icnofósseis da Formação Pimenteira, Devoniano da Bacia do Parnaíba, Piauí. Brasil. Gaea J. Geosci. 8, 33–41. doi: 10.4013/gaea.2012.81.05
Simões, M. G., Leme, J. M., and Soares, S. P. (2009). Systematics, taphonomy and paleoecology of Homalonotid trilobites (Phacopida) from the Ponta Grossa Formation (Devonian), Paraná Basin, Brazil. Revista Brasileira de Paleontologia 12, 27–42. doi: 10.4072/rbp.2009.1.03
Vaz, P. T., Resende, N. G. A. M., Wanderley Filho, J. R., and Travassos, W. A. (2007). Bacia do Parnaíba. Boletim de Geociências da Petrobras 15, 253–263.
Vettorazzi, A. L. S. (2012). Caracterização sedimentológica dos arenitos da Formação Cabeças (Devoniano) na borda leste da Bacia do Parnaíba. MsC Thesis, Universidade Federal do Rio de Janeiro.
Whittington, H. B. (1980). Exoskeleton, moult stage, appendage morphology, and habits of the Middle Cambrian trilobite Olenoides serratus. Palaeontology 23, 171–204.
Wickham, H., François, R., Henry, L., and Müller, K. (2022). dplyr: A Grammar of Data Manipulation. R package version 1.0.10, Available at: https://CRAN.R-project.org/package=dplyr.
Keywords: pimenteira formation, cabeças formation, canindé group, molting, trilobites
Citation: Sedorko D, de Barros GEB, Netto RG, Ghilardi RP, Agostinho S, Ramos KS, Franco Neto E, Moreira Junior CA and Borghi L (2023) Multiple-Rusophycus assemblage from the Parnaíba Basin (NE Brazil) reflects trilobites as tracemakers and molting behavior. Front. Ecol. Evol. 11:1117947. doi: 10.3389/fevo.2023.1117947
Edited by:
Fernanda Quaglio, Federal University of São Paulo, BrazilReviewed by:
Andrej Spiridonov, Vilnius University, LithuaniaOm Narain Bhargava, Geological Survey of India, India
Copyright © 2023 Sedorko, de Barros, Netto, Ghilardi, Agostinho, Ramos, Neto, Moreira Junior and Borghi. This is an open-access article distributed under the terms of the Creative Commons Attribution License (CC BY). The use, distribution or reproduction in other forums is permitted, provided the original author(s) and the copyright owner(s) are credited and that the original publication in this journal is cited, in accordance with accepted academic practice. No use, distribution or reproduction is permitted which does not comply with these terms.
*Correspondence: Daniel Sedorko, c2Vkb3Jrb0Btbi51ZnJqLmJy