- 1State Key Laboratory of Loess and Quaternary Geology, Institute of Earth Environment, Chinese Academy of Sciences, Xi’an, China
- 2School of Geography and Information Engineering, China University of Geosciences, Wuhan, China
- 3State Key Laboratory of Biogeology and Environmental Geology, China University of Geosciences, Wuhan, China
- 4Department of Marine Science and Engineering, Southern University of Science and Technology, Shenzhen, China
- 5University of Chinese Academy of Sciences, Beijing, China
- 6Institute of Global Environmental Change, Xi’an Jiaotong University, Xi’an, China
Glycerol dialkyl glycerol tetraethers (GDGTs), which are derived from microbial membranes, occur widely in soils, peats, lake sediments, marine sediments, hot springs, and other environments. GDGTs respond sensitively to environmental changes and have become an important tool in paleoenvironmental reconstruction. However, their application in speleothems, one of the most important archives of the study of past climates, has been quite limited. In this paper, we review the recent progress in the study of GDGTs in speleothems, from their sources to their distribution characteristics and paleoenvironmental applications. GDGTs in speleothems have been shown to come from dripping water, and cave systems. The distribution of GDGTs in speleothems shows that archaeal isoprenoid GDGTs (isoGDGTs) dominate over bacterial branched GDGTs (brGDGTs). The proxies based on isoGDGTs (TEX86) and brGDGTs (MBT/CBT) are correlated with temperature, which may offer promising potential methods for reconstructing terrestrial palaeotemperature. Ultimately, we discuss the difficulties and problems to be solved in studying speleothem GDGTs and the possible future directions for this field.
1. Introduction
At present, the issue of global change is attracting increasing attention. The frequent occurrence of extreme climate events has jeopardized people’s lives and productivity and caused huge social and economic losses. Therefore, it is essential to study the principles of historical climate change to predict future climate change. The wide distribution of stalagmites and their utility in accurate high-resolution dating have made them an important archive in the field of paleoenvironment research. A series of advances have been made in stalagmite analysis (Wang et al., 2001; Hu et al., 2008; Cai et al., 2010; Cheng et al., 2016; Zhu et al., 2017; Tan et al., 2018; Zhang et al., 2018; Zhang et al., 2021), and environmental proxies based on stalagmites such as carbon and oxygen isotopes (Dorale et al., 1998; Wang et al., 2001), trace elements (Roberts et al., 1998; Tan et al., 2014; Zhang et al., 2018), and magnetism (Zhu et al., 2017) have been used extensively in the reconstruction of paleoenvironments. However, most of these studies have focused on inorganic proxies, which are often affected by many factors (McDermott, 2004). Carbonate δ13C is affected by temperature, soil moisture, cave system openness, and other factors (Hendy, 1971; Genty et al., 2003). As one of the most important proxies of stalagmites, the interpretation of δ18O remains controversial. Some studies indicate that δ18O reflects the monsoon intensity or monsoon rainfall amount (Wang et al., 2001; Cheng et al., 2016), whereas other studies suggest that δ18O indicates the changes in the relative contributions of different water vapor sources (Maher, 2008; Maher and Thompson, 2012). Therefore, a comprehensive comparison of multiple proxies is important to fully understand their real climatic significance.
In addition to inorganic proxies, the organic matter in stalagmites also records environmental information, and the rich variety of lipid biomarkers, a class of organic matter preserved in stalagmites can provide more evidence for paleoenvironment research. Lipid biomarkers are derived from specific organisms. During the degradation of organic matter, the carbon skeleton is retained, and the information on the biological precursors and the environment where the source organism inhabits is recorded; this information can be used in paleoenvironmental reconstructions (Meyers and Ishiwatari, 1993; Castañeda and Schouten, 2011). Until recently, the most well-studied biomarkers include long-chain n-alkanes of leaf wax (Castañeda et al., 2016), fatty acids (Wang et al., 2018), long-chain alkenones (Uk’37) (Abrantes et al., 2005), glycerol dialkyl glycerol tetraethers (GDGTs) (Weijers et al., 2007a), which have been extensively used in paleo-reconstruction in a variety of archives.
Among lipids, GDGTs derived from archaea and some bacteria are relatively stable. They are resistant to degradation in sediments, and have been detected as far back as Jurassic (Kuypers et al., 2001; Schouten et al., 2003; Jenkyns et al., 2012). GDGTs are found ubiquitously in soils, peatlands, lake sediments, marine sediments, hot springs, and speleothems (Weijers et al., 2006a,b, 2007b; Yang et al., 2011, 2014; Schouten et al., 2013; Zhang et al., 2013; De Jonge et al., 2014; Sinninghe Damsté et al., 2014; Dang et al., 2016). Over the last decade, GDGTs have become a hotspot of bio-organic geochemical research. Researchers have analyzed GDGTs in marine sediments (Wuchter et al., 2004; Zhang et al., 2011), lake sediments (Powers et al., 2010; Sinninghe Damsté et al., 2012a; Hu et al., 2015), peatlands (Zhou et al., 2011; Wang et al., 2017), and loess (Gao et al., 2012; Jia et al., 2013; Tang et al., 2017; Wang et al., 2020) around the world, and successfully reconstructed temperature changes in different regions, such as tropical Africa (Weijers et al., 2007a), Amazon basin in South America (Bendle et al., 2010), Antarctic coast (Pross et al., 2012), and China (Yang et al., 2014; Dong et al., 2015; Tang et al., 2017; Lu et al., 2019). The results of these studies provide important support for understanding climate change across the globe.
The use of proxies in the study of cave paleoenvironment is an important research area and the GDGTs preserved in stalagmites have the potential to provide a terrestrial organic paleothermometer independent of isotopic records (Yang et al., 2011; Blyth and Schouten, 2013). However, there have been relatively few studies of stalagmite GDGTs, and most of these studies have focused on the provenance of GDGTs in stalagmites, as well as the relationship between GDGTs and environmental variables (Yang et al., 2011; Blyth and Schouten, 2013; Blyth et al., 2014, 2016; Baker et al., 2016, 2019; Zhang et al., 2020). Paleoenvironmental reconstructions have only been determined in Mawmluh Cave (India) and Jiangjun Cave (China) (Figure 1; Huguet et al., 2018; Wassenburg et al., 2021). Besides, 5-methylated and 6-methylated bacterial branched GDGT (brGDGTs) isomers have been rarely reported (Zhang et al., 2020). In this paper, we review the sources and distribution characteristics of stalagmite GDGTs based on recent studies and the use of GDGT-based proxies in stalagmites to provide references for future paleoenvironmental reconstructions based on stalagmite GDGTs.
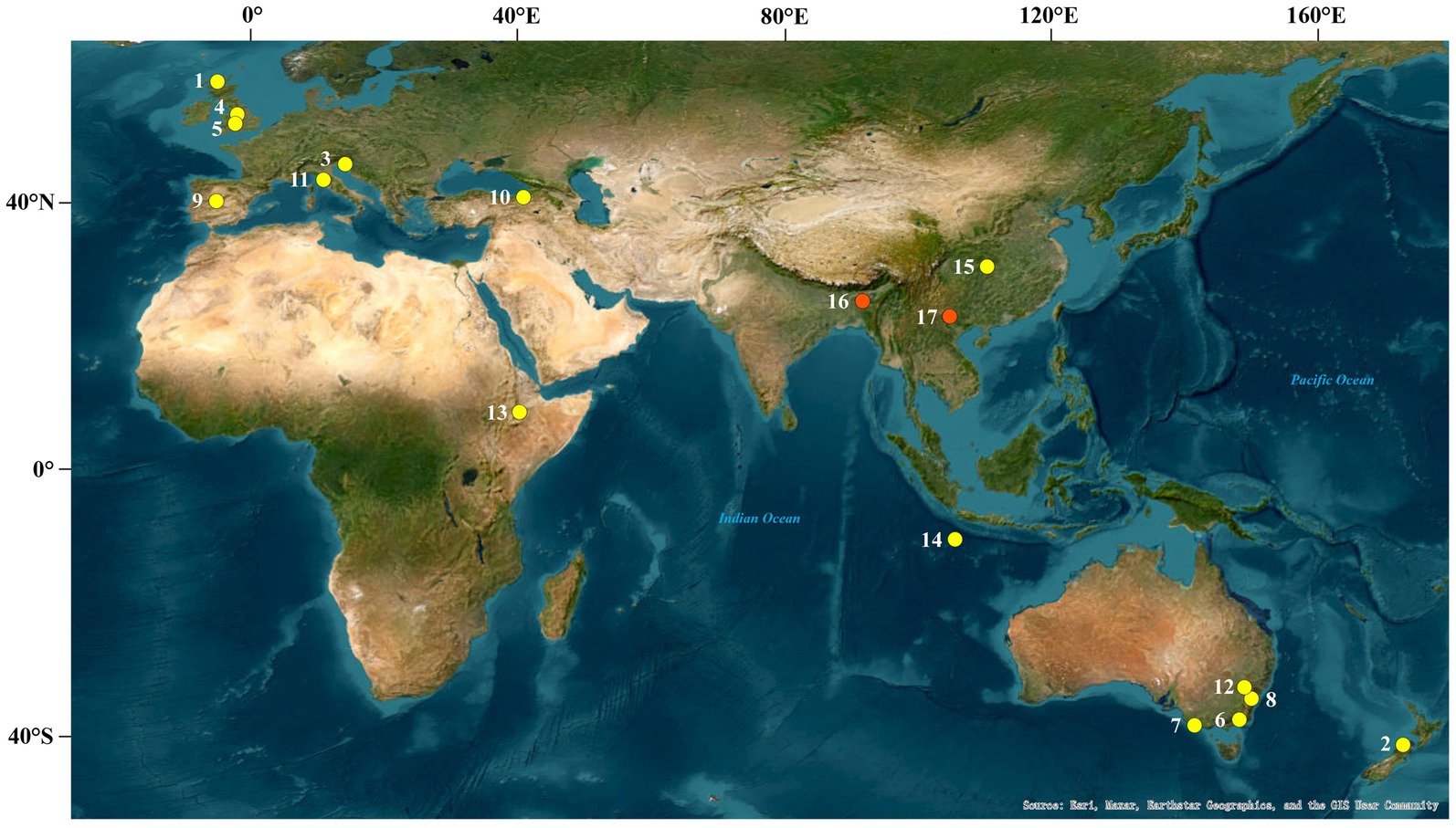
Figure 1. Global cave locations of published GDGT-based studies (1, Uamh am Tartair, Scotland; 2, Nettlebed Cave; 3, Postojna Cave; 4, Pooles Cavern; 5, Lower Balls Mine; 6, Buchan Caves; 7, Bats Ridge Cave System; 8, Wombeyan Caves; 9, Eagle Cave; 10, Turkey; 11, Tuscany, Italy; 12, Cathedral Cave and Gaydon Cave; 13, Achere Cave and Rukessia Cave; 14, Christmas Island; 15, Heshang Cave; 16, Mawmluh Cave; 17, Jiangjun Cave. The yellow dots 1–15 are sites of modern-process studies: Yang et al., 2011; Blyth and Schouten, 2013; Baker et al. 2019; and the red dots 16–17 are sites of paleoclimatic studies: Huguet et al., 2018; Wassenburg et al., 2021).
2. Structures and biological sources of GDGTs
GDGTs are the core membrane lipids of archaea and some bacteria (Schouten et al., 2013) and can be divided into two groups: archaeal isoprenoid GDGTs (isoGDGTs) and bacterial branched GDGTs (brGDGTs) (Figure 2). The configuration of the glycerol backbone in archaeal isoGDGTs is 2,3-di-O-alkyl-sn-glycerol, and its alkyl chain contains 0–8 cyclopentane moieties. Crenarchaeol and crenarchaeol regioisomer (denoted by crenarchaeol′) contain a unique cyclohexane moiety and were thought to be specifically produced by Thaumarchaeota (Pitcher et al., 2011a; Sinninghe Dasmté et al., 2012b; Elling et al., 2017). The brGDGTs have 1,2-di-O-alkyl-sn-glycerol configuration, and its alkyl chain contains various numbers of methyl groups and cyclopentane moieties (Weijers et al., 2006b). BrGDGTs are biosynthesized by some bacteria, among which one strain of Acidobacteria, Solibacter usitatus, was capable of producing 5-methylated and tetramethylated brGDGTs (Sinninghe Damsté et al., 2018; Chen et al., 2022; Halamka et al., 2022). The presence of ether bonds confers good thermal stability on GDGTs. In general, “GDGTs” refers to core lipid GDGTs (CL-GDGTs), whose structures include glycerol backbones and alkyl chains. However, in living cells, GDGTs exist in the form of intact polar lipid GDGTs (IPL-GDGTs), which have glycerol backbones at both ends and polar head groups composed of phosphoric acid and sugar moieties (Sturt et al., 2004; Lipp et al., 2008; Peterse et al., 2011). The polar head groups are rapidly lost after cell death to form CL-GDGTs, so IPL-GDGTs indicate living biomass (Lipp et al., 2008; Tierney et al., 2012).
2.1. isoGDGTs
Many archaea can synthesize isoGDGTs observed in the environment (Schouten et al., 2007a). Archaea were initially believed to synthesize isoGDGTs to resist the stresses of extreme environments, such as high temperatures, high salinity, and extreme acidity (Schouten et al., 2013). However, numerous recent studies show that isoGDGTs were found not only in extreme environments, but also widely distributed in normal environments such as oceans, soils, and lakes (Schouten et al., 2013). GDGT-0 is the compound most commonly found in cultured archaea other than halophiles, and therefore GDGT-0 may be prevalent in uncultured archaea living in the environment (Blaga et al., 2009; Weijers et al., 2009). GDGT-1–4 commonly occur in Euryarchaeota, Thaumarchaeota, and Crenarchaeota, and at especially high abundances in anaerobic methanotrophic archaea group 1 (ANME-1; Blumenberg et al., 2004). GDGT-5–8 usually occur in high-temperature environments, such as hot springs, and some cultures of thermophilic Crenarchaeota and Euryarchaeota (Pearson et al., 2004; Pearson et al., 2008; Zhang et al., 2008; Burgess et al., 2012). GDGT-5–8 have only been detected in peat environments and acid mine drainage and may be contributed by the acidophilic archaea in these environments (Naafs et al., 2018; Pei et al., 2019; Yang et al., 2019).
Crenarchaeol was thought to be a biomarker for ammonia-oxidizing Thaumarchaeota (Pitcher et al., 2011b; Sinninghe Dasmté et al., 2012b; Elling et al., 2017) and was initially thought to be derived from marine Group I archaea (Hoefs et al., 1997; Delong et al., 1998). Subsequent studies show that crenarchaeol was abundant in diverse settings and can be produced by pure cultures of several strains of Thaumarchaeota. Sinninghe Damsté et al. (2012b) considered that crenarchaeol specifically containing a cyclohexane moiety is formed by microorganisms to adapt to low-temperature environments because it changes the structure of the cell membrane to increase its fluidity. However, archaea with high crenarchaeol content have since been detected in hot springs (Schouten et al., 2007b). The isoGDGTs detected by de la Torre et al. (2008) and Pitcher et al. (2010) in thermophilic Thaumarchaeota were mainly crenarchaeol, confirming that crenarchaeol with a cyclohexane moiety is not restricted to low-temperature environments. The formation mechanism of the cyclohexane moiety remains to be determined. Crenarchaeol in the subsurface waters of oceans and lakes may be derived from Thaumarchaeota Group I.1a, which prefers micro-anaerobic environments (Pitcher et al., 2011b; Schouten et al., 2012, 2013).
As well as the marine water column, the most likely biological sources of crenarchaeol in soils (Timonen and Bomberg, 2009) and lake sediments (Buckles et al., 2013) are Thaumarchaeota Group I.1b (Xie et al., 2015) because Group I.1b is most widespread in these environments. Crenarchaeol′ (a regioisomer of crenarchaeol) is also thought to be derived from Thaumarchaeota and has been detected in high proportions in soil group I.1b Thaumarchaeota (Sinninghe Damsté et al., 2012b).
2.2. brGDGTs
The alkyl chain of brGDGTs has a methyl branched-chain structure rather than the isoprenoid structure of the isoGDGT alkyl chain, and the glycerol skeleton configuration also differs from that of isoGDGTs. Therefore, these compounds are called “branched” GDGTs. BrGDGTs are derived from some Acidobacteria and possibly by other unknown bacteria (Sinninghe Damsté et al., 2011; Chen et al., 2022). BrGDGTs have been found in soils (Weijers et al., 2007c), lakes (Loomis et al., 2011; Tierney et al., 2012), rivers (Hopmans et al., 2004), and marine sediments (Zhu et al., 2011). To date, more than 15 brGDGT compounds have been detected in different environments around the world, and Acidobacteria may be a biological source of at least the 5-methyl brGDGTs (Chen et al., 2022).
The analysis of peat profiles in Sweden showed that the high content of brGDGTs was probably produced by the major subdivisions of Acidobacteria (Weijers et al., 2009). As peat becomes more anaerobic, the content of IPL-brGDGT increases significantly, indicating that the bacteria producing brGDGTs are probably anaerobic or facultatively anaerobic (Liu et al., 2010; Peterse et al., 2011). However, brGDGTs have also been detected in aerobic environments, such as surface peat and surface soil (Weijers et al., 2006a; Liu et al., 2010), indicating that the bacteria producing brGDGTs in the environment may be able to adapt to a wide range of oxygen concentrations. The content of brGDGTs in soils decreases as pH increases (Peterse et al., 2010) and is relatively high in acidic soils, whereas the contents of some groups of Acidobacteria, such as subdivision 1, decrease significantly at high pH (Jones et al., 2009). The contents of IPL-GDGTs in soils do not differ significantly across the seasons (Weijers et al., 2011), which is consistent with the slow growth of Acidobacteria. BrGDGTs are also relatively stable and not easily degraded, and the high contents of brGDGTs in soil and peat may be the result of their long-term accumulation (Yang et al., 2014).
2.3. OH-GDGTs
In addition to the conventional GDGTs discussed above, GDGTs with 1–2 hydroxyl groups in the alkyl chain, hydroxyl GDGTs (OH-GDGTs), occur in marine sediments (Huguet et al., 2013), lake sediments (Wang et al., 2017), and acid soils (Man et al., 2023). They are designated OH-GDGT-0, OH-GDGT-1, and OH-GDGT-2 according to the number of cyclopentane moieties. It has been demonstrated that pure cultured Thaumarchaeota Group I.1a can produce OH-GDGTs, whereas Group I.1b cannot (Sinninghe Damsté et al., 2012b). Some methanogenic archaea, such as the thermophilic methanogenic archaea Methanococcus thermolithotrophicus, can produce OH-GDGT-0, and the anaerobic methane-oxidizing archaea ANME-2d can synthesize OH-GDGT-2–3 (Kurth et al., 2019).
Huguet et al. (2013) investigated lake and marine sediments worldwide and found that OH-GDGTs occur predominantly in low-temperature regions and that their relative content correlates well with temperature. A subsequent study of OH-GDGTs showed that the number of cyclopentane moieties in OH-GDGTs (the index RI-OH) correlates positively with temperature (Lü et al., 2015). In the sediments of Qinghai Lake, the relative content of OH-GDGTs was consistent with relative changes in the abundance of crenarchaeol and amoA (a functional gene of ammonia oxidation in Archaea and bacteria), suggesting that OH-GDGTs are derived mainly from Thaumarchaeota (Wang et al., 2017). The composition of OH-GDGTs in peat may be affected by methanogenic archaea. The RI-OH index of OH-GDGTs correlates significantly with the ratio of GDGT-0/crenarchaeol, and the relative abundance of OH-GDGT-0 increases gradually with the increasing depth of peat (Yang et al., 2019).
3. Extraction and analytical methods for stalagmite GDGTs
3.1. Pretreatment of samples
The organic carbon content preserved in stalagmites accounts for about 0.01–0.3% of the total carbon (Blyth et al., 2013; Li et al., 2014; Quiers et al., 2015), and the content of lipids in stalagmites is relatively low (Huang et al., 2007). Therefore, the extraction of GDGTs requires large samples, and external pollution must be reduced as much as possible during their extraction. All glassware should be cleaned using ultrapure water. After drying, the glassware should be heated in a muffle furnace at 500°C for 6 h to remove all organic matter from the vessel. Other materials used in the experiment, such as filter film and hydrochloric acid, should be treated with dichloromethane (DCM). The stalagmite samples are cut along the growth axis and then divided into small pieces according to the growth level (to ensure high-accuracy dating). The thin surface layer of the massive stalagmite sample is removed, washed using ultrapure water, and dried. The residual organic pollutants on the surface are removed using ultrasound in DCM before analysis (Blyth et al., 2006; Blyth and Schouten, 2013; Zhang et al., 2020).
3.2. Extraction of GDGTs from stalagmites
The early experimental extraction techniques required about 100 g of powdered speleothem sample to extract the lipids (Xie et al., 2003). With the application of acid digestion technology, more organic matter can be released when only limited stalagmite samples are available (Blyth et al., 2006; Huang et al., 2008). In general, GDGTs can be extracted from approximately 5–20 g of stalagmite samples (Blyth and Schouten, 2013). Wang et al. (2012) tested different acid digestion conditions and found that a 5 g stalagmite sample was sufficient for lipid analysis using 3 mol/l hydrochloric acid and heating at 130°C for 3 h. After the stalagmite sample is digested, liquid–liquid extraction is performed using DCM–MeOH (9:1, v/v) reagent (30 ml × 6). The collected total lipid extracts are concentrated to 1–2 ml, filtered through 0.45 μm polytetrafluoroethylene (PTFE) membrane, and dried under nitrogen before their preparation for analysis (Yang et al., 2011; Wang et al., 2018; Zhang et al., 2020).
3.3. Analytical method for stalagmite GDGTs
In recent years, many studies have shown that some brGDGT may contain one or more isomers, that coelute in the chromatogram obtained by the traditional liquid chromatography method. These isomers are often treated as one compound because they cannot be easily separated. These isomers can interfere with the study of brGDGT distributions, proxies, and their paleoenvironmental applications (Zech et al., 2012). With improvements in HPLC methods, De Jonge et al. (2014) successfully used four silica gel columns to separate all 5-methyl and 6-methyl isomers of GDGT-III and GDGT-II. Hopmans et al. (2016) optimized the liquid chromatography method and separated 15 brGDGT compounds using only two silica gel columns in series. Further study showed the separation effect of the method is better than the previous studies. Isomers have even been found for Ia and Ib (Yang et al., 2015).
Yang et al. (2011) first analyzed the contents of isoGDGTs and brGDGTs in stalagmites following Hopmans et al. (2000). The method used does not separate 5-methyl from 6-methyl brGDGTs. The specific analytical method followed here is described by Zhang et al. (2020). The GDGTs are analyzed using high-performance liquid chromatography–mass spectrometry (HPLC–MS). The dried extracts were resolved in n-hexane: isopropanol (9:1, v/v) and a known amount of C46 GTGT (glycerol trialkyl glycerol tetraether; Huguet et al., 2006) internal standard was added. The injection volume of each sample is 10–30 μl (selected according to the concentration of the sample).
Separation of 5- and 6-methylated brGDGTs was performed by using two silica columns in tandem (BEH HILIC column, 150 mm × 2.1 mm, 1.7 μm, Waters or Thermo Finnigan silica column, 150 mm × 2.1 mm, 1.9 μm) (Yang et al., 2015; Hopmans et al., 2016). The liquid chromatography methodologies proposed by Yang et al. (2015) and Hopmans et al. (2016), respectively, differ in the solvent and elution gradient. The former used ethyl acetate and n-hexane while the latter used isopropanol and n-hexane. The APCI and MS conditions vary among laboratories, depending on the instrument brand used. GDGTs were generally detected with single ion monitoring (SIM) at m/z 1302, 1300, 1298, 1296, 1292, 1050, 1048, 1046, 1036, 1034, 1032, 1022, 1020, 1018, and 744 (Yang et al., 2015).
4. Distribution and sources of stalagmite GDGTs
4.1. Distribution of GDGTs in stalagmites
Stalagmites are connected to the surrounding environment by dripping water and receive organic matter from the overlying soil, vegetation, percolation system, and the interior of the cave, so a variety of chemical signals can be preserved within them. GDGT compounds are widely distributed in stalagmites, and the content of GDGTs varies clearly across different periods (Yang et al., 2011). Previous studies have shown that GDGTs can generally be extracted from 5–20 g stalagmite samples, but the content of GDGTs in some stalagmites is below the limit of detection.
The content of archaeal isoGDGTs in stalagmites is generally higher than that of bacterial brGDGTs (Figure 3). The data from 33 stalagmite samples collected from 16 sites in Europe, Africa, and Oceania showed that the speleothem GDGTs were dominated by archaeal isoGDGTs. The content of crenarchaeol was high, whereas the contents of crenarchaeol′ and crenarchaeol, brGDGT-Ic, -IIc, -IIIc, and -IIIb were relatively low (Blyth and Schouten, 2013). The distribution of specific compounds also varied greatly among stalagmites from different regions. The contents of GDGTs in stalagmites from Heshang Cave ranged from 0.15 to 4.19 ng/g dry weight of stalagmite. The isoGDGTs were dominated by crenarchaeol, which reached 0.07–0.94 ng/g dry weight of stalagmite, accounting for 48% of the total weight of the isoGDGTs. However, the contents of GDGT-0–3 and crenarchaeol′ were relatively low. The contents of bacterial brGDGTs ranged from 0 to 0.67 ng/g stalagmite dry weight (Yang et al., 2011), and they were dominated by IIa′ (Figure 3C). The content of brGDGTs without cyclopentane rings was higher than the content of brGDGTs containing cyclopentane rings (Yang et al., 2011). Similarly, the main isoGDGT of stalagmites in Australian caves was also crenarchaeol, and the content of GDGT-3 was higher than that of GDGT-1 or GDGT-2. The main brGDGTs were Ib and IIb, but the content of brGDGTs containing cyclopentane rings was higher than the content of those without cyclopentane rings (Baker et al., 2016).
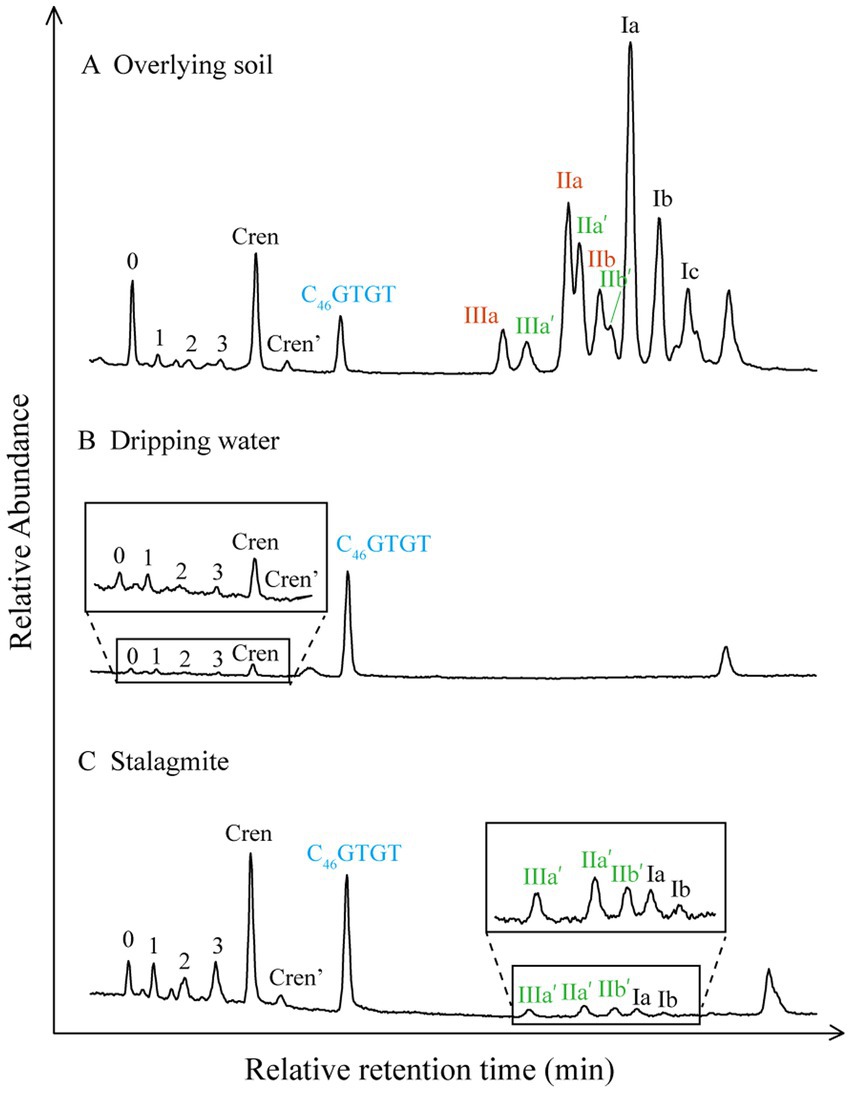
Figure 3. Chromatograms of the base peaks showing the distribution of GDGTs in overlying soil (LBT-1), dripping water (HS4D-4), and stalagmite (HS4-2) (data from Heshang Cave, Zhang et al., 2020).
4.2. Sources of GDGTs in stalagmites
The two main sources of the organic matter preserved in caves are the roof environment (e.g., vegetation and soil) and inside the cave (dripping water and cave microorganisms) (Xie et al., 2003; Huang et al., 2007; Yang et al., 2011; Blyth et al., 2014; Wang et al., 2018). To examine the sources of stalagmite GDGTs, the stalagmites, dripping water, and overlying soils have been analyzed for GDGTs and GDGT distribution among them differ significantly.
4.2.1. Archaeal isoGDGTs
Yang et al. (2011) analyzed the contents of GDGTs in stalagmites, the surface of weathered dolomite, dripping water, and overlying soil samples from Heshang Cave in China. The distribution of stalagmite isoGDGTs in Heshang Cave was similar to those of the dripping water, both of which were dominated by crenarchaeol, whereas the overlying soil isoGDGTs were dominated by crenarchaeol and GDGT-0. No OH-GDGTs were detected in the cave or overlying soil. Neither the dripping water nor stalagmites inherited the distribution pattern of the soil archaeal isoGDGTs. Furthermore, the distribution of archaeal isoGDGTs in the surrounding rocks was similar to that in the stalagmite, indicating that archaeal isoGDGTs can grow on the surfaces of stalagmites in situ. Therefore, the archaeal isoGDGTs of the stalagmites in Heshang Cave were thought to be mainly contributed by microorganisms growing on the surfaces of the stalagmites and in dripping water (Yang et al., 2011). Ding et al. (2018) first reported GDGTs in a groundwater system, and the CL-GDGTs correlated strongly with the IPL-GDGTs, indicating the presence of a living archaeal community in the groundwater system.
Crenarchaeol and GDGT-0 in soil samples are mainly from Thaumarchaeota Group I.1, and the archaea in underground environments are usually phylogenetically closer to those in soil, belonging to Thaumarchaeota Group I.1a and I.1b (Weidler et al., 2007; Zhao et al., 2017). Therefore, Thaumarchaeota may be the main source of stalagmite isoGDGTs. Because the microbial abundance in the dripping water in caves is low, it is impossible to use the Bligh-Dyer extraction method to extract IPL-GDGTs, which represent the biomass of living archaea. Therefore, it can only be inferred that the crenarchaeol in dripping water is derived from the living archaea in groundwater (Yang et al., 2011).
A comparison of stalagmites and soils in five caves in the UK and Australia showed significant differences in the distribution of GDGTs, and therefore, the soil was not the main source of the stalagmite isoGDGTs either (Blyth et al., 2014). A recent irrigation experiment showed that the GDGT components in dripping water included a high proportion of GDGT-0, which differed significantly from the GDGT compositions in soil and stalagmites. This indicated that soil and dripping water were not the main sources of the isoGDGTs in the stalagmites (Baker et al., 2016). There are rich species of archaea in caves (Wu et al., 2015), and the presence of Thaumarchaeota in caves has been detected in DNA studies (Gonzalez et al., 2006; Zhao et al., 2017). This provides further evidence for the in situ growth of stalagmite isoGDGTs.
4.2.2. Bacterial brGDGTs
High abundances of bacterial brGDGTs are generally present in soils. If the brGDGTs preserved in stalagmites are derived from soil, their distributions should be consistent with their characteristic distribution in soils. Studies have shown that the concentrations of bacterial brGDGTs in soils are generally higher than the concentrations of archaeal isoGDGTs (Figure 3). However, this is not the case in stalagmites (Yang et al., 2011; Zhang et al., 2020). The distribution of bacterial brGDGTs in stalagmites from Heshang Cave was similar to that in weathered rock samples, which are usually dominated by brGDGT-IIb. However, the soil was dominated by brGDGTs IIa, IIb, and Ib, indicating that soil was not the main source of bacterial brGDGTs in the stalagmites (Yang et al., 2011). Huguet et al. (2018) analyzed the stalagmite GDGTs in Mawmluh Cave and showed that the lower abundances of bacterial brGDGTs in the stalagmites were probably produced in the cave because the thin layer of overlying soil was poorly developed, and the content of brGDGTs in the soil was higher than the content of isoGDGTs. Therefore, the soil is unlikely to be the main source of stalagmite bacterial brGDGTs.
Other studies have shown that bacterial brGDGTs can be produced in situ in aquatic environments (Sinninghe Damsté et al., 2009). Given the significant differences between stalagmites and soils, we can speculate that the bacterial brGDGTs in stalagmites are derived mainly from dripping water or the microorganisms growing on the surfaces of the stalagmites. A 5-year program that monitored the dripping-water bacterial community in Heshang Cave revealed that the bacteria showed ecological adaptability in the oligotrophic environment of dripping water, surviving on the poor nutrients in the water or obtaining essential nutrients from minerals (Yun et al., 2015). Barton et al. (2014) also found that the dripping water in the cave provided the carbon and energy required for the survival and growth of the microbial communities in the cave, which supports the possibility that bacterial brGDGTs are produced in dripping water or inside the cave.
5. Application of GDGT-based proxies in stalagmites
5.1. TEX86
As the temperature increases, the number of cyclopentane rings in isoGDGTs also increases (Uda et al., 2001). Therefore, Schouten et al. (2002) established the tetraether index of 86 carbon atoms (TEX86) proxy (Table 1) to characterize the relative numbers of cyclopentane moieties in isoGDGTs. TEX86 has a good linear relationship with sea surface temperature. Subsequent pure culture experiments have confirmed that the number of cyclopentane moieties in isoGDGTs produced by Thaumarchaeota changes with temperature (Wuchter et al., 2004). The TEX86 proxy is basically unaffected by salinity, and the upper limit of its response to temperature is 38.6°C. The large temperature response range of the TEX86 proxy also makes it better than Uk’37 for quantitative paleotemperature reconstruction (especially in high-temperature equatorial seas). When the temperature exceeds 30°C, the response of Uk’37 becomes insensitive to temperature (Pelejero and Calvo, 2003). Therefore, TEX86 is used as a thermometer for aquatic settings and has been widely used in marine and terrestrial paleoenvironmental reconstruction.
Blyth and Schouten (2013) investigated the GDGTs of several cave stalagmites around the world and found that TEX86 correlated significantly with the measured temperature. They established calibration equations, one for the surface mean annual air temperature (MAAT; R2 = 0.78, p < 0.001, n = 30) and one for cave MAAT (R2 = 0.68, p < 0.001, n = 19; Figures 4A, 4B, Table 2). The standard errors were 2.3 and 2.0°C, respectively. The TEX86 values for stalagmite samples from Heshang Cave were 0.60–0.68, which was lower than that of soil. There is a good negative correlation between TEX86 and δ18O in stalagmites and the value of TEX86 increases as δ18O decreases. Because the air temperature increases with the strengthening of monsoon, TEX86 can record the temperature changes in a cave (Yang et al., 2011). Blyth et al. (2014) found that the TEX86 values for most stalagmites in their study area were lower than the soil TEX86 and had a good linear relationship with the surface MAAT (R2 = 0.93, p < 0.001). Baker et al. (2019) updated the calibration equations for TEX86 and MAAT based on the data of Blyth and Schouten (2013) combined with data from Yang et al. (2011) and two unpublished datasets (R2 = 0.84, p < 0.001; R2 = 0.82, p < 0.001; Figures 4C, 4D, Table 2). The calibration equations were successfully applied to the cave air temperature reconstruction for Jiangjun Cave (Wassenburg et al., 2021).
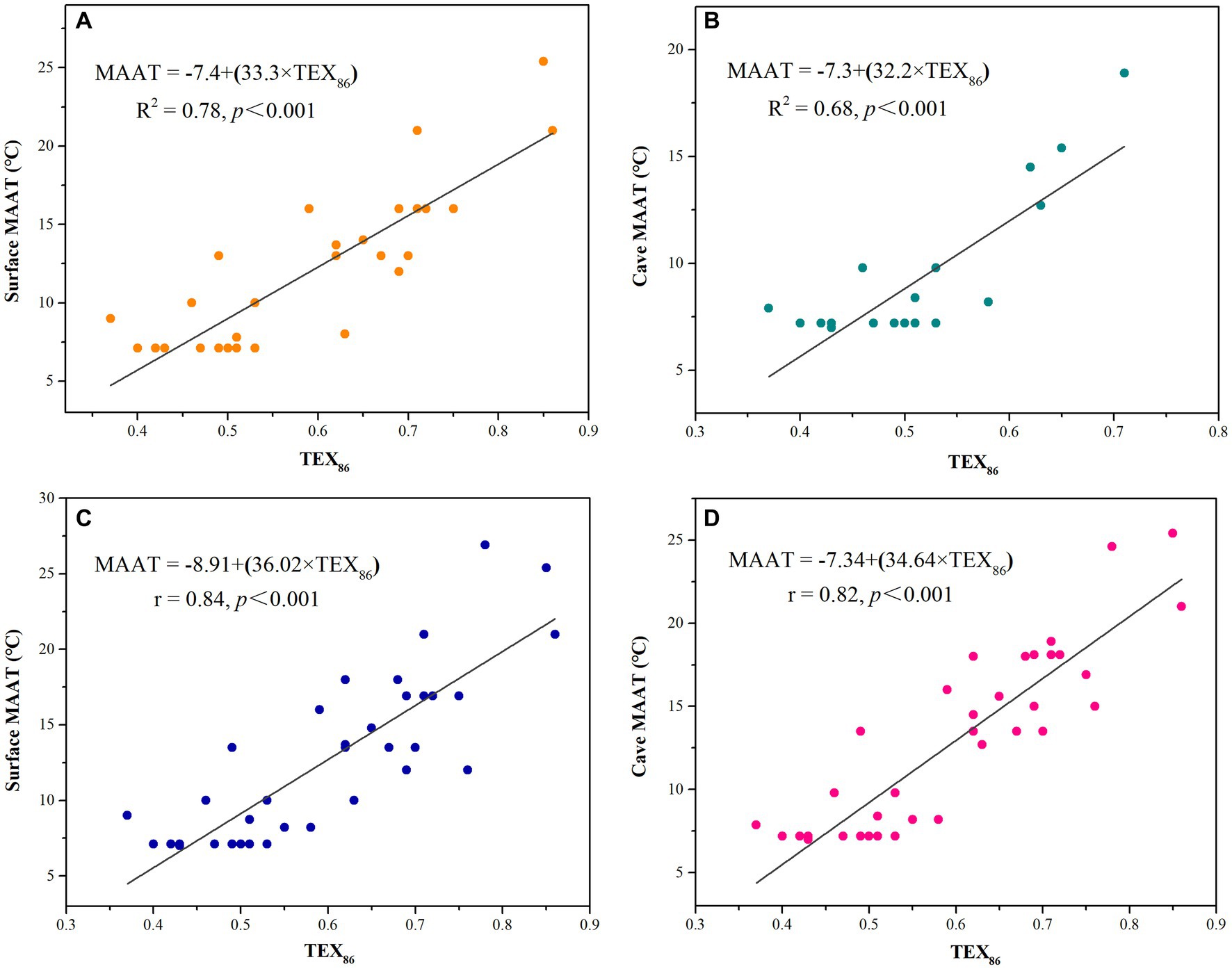
Figure 4. Relationships between TEX86, surface MAAT, and cave MAAT (data A and B from Blyth and Schouten, 2013; data C and D from Yang et al., 2011; Blyth and Schouten, 2013; Baker et al., 2019).
The relationship between TEX86 and temperature could be biased by the following factors. The first is the input signal from the overlying soil. The soil is leached by rain, and a small amount of archaeal isoGDGTs is brought into the stalagmites through the seepage system, which could affect the TEX86 value directly. Because the input from the external soil (indicated by a high branched and isoprenoidal tetraether [BIT] index, see below) causes TEX86 to deviate (Weijers et al., 2006a), Blyth and Schouten et al. (2013) tried to remove samples with BIT >0.5 to improve the accuracy of the calibration equation. However, this did not significantly improve the relationship between TEX86 and surface MAAT (R2 = 0.77, p < 0.001, n = 27) or cave MAAT (R2 = 0.70, p < 0.001, n = 16; Table 2). The standard errors were 2.2 and 1.9°C, respectively, and had decreased by only 0.1°C. Therefore, more samples are required to confirm this calibration method.
The second factor biasing TEX86 is the differences in archaeal communities. The archaea in caves may differ from those in soil or marine environments and may respond differently to temperature. Therefore, further microbial research is required. The third factor is the differences in regional microclimates. The measured surface temperatures used in previous studies are generally obtained from the nearest meteorological station. Because there is a certain distance between the sampling point and the meteorological station, the temperature in the area directly above the cave may be somewhat different from the meteorological data (Yang et al., 2011; Blyth et al., 2016). Besides, due to the low growth rate of stalagmites, TEX86 from stalagmites may record an average of temperature for multiple years.
Finally, TEX86 from stalagmites may record temperature signals biased towards the growing season. Herfort et al. (2006) found that TEX86 in surface sediments from the North Sea was influenced by isoGDGT production in a specific season of the year (Herfort et al., 2006). The TEX86-derived temperatures were strongly biased towards the summer temperature in Tibetan lakes (Günther et al., 2014). Leider et al. (2010) also found that TEX86 in the surface sediments was best correlated with summer sea surface temperatures of the Mediterranean Sea. Zhang et al. (2022) observed the seasonal bias of TEX86-derived temperatures in high latitudes and high elevations where freezing occurred. Owing to varying water inputs across different seasons, the growth of stalagmites may have hiatus in dry seasons and the temperature signal recorded is expected to be primarily derived from the growing season. Therefore, TEX86 proxy in stalagmites may also be affected by seasonal factors, despite that there is no conclusive evidence yet.
5.2. BIT
According to early studies, brGDGTs are derived mainly from terrestrial soil and peat, whereas crenarchaeol occurs predominantly in marine environments. Hopmans et al. (2004) proposed the branched and isoprenoid tetraether (BIT) index (Table 1) to measure the relative contributions of terrestrial organic matter in marine environments. The principle underlying the BIT proxy is that crenarchaeol represents the contribution of the marine environment and brGDGTs represent the contribution of terrestrial soil, so a BIT value is close to 0 in an open marine environment and close to 1 in terrestrial soil and peat (Schouten et al., 2013). The BIT proxy has been increasingly used to trace the migration of terrestrial organic carbon.
According to the stalagmite data published to date, the BIT value of stalagmites is generally lower than that of soil (Figure 5B). The BIT values of the stalagmites in Heshang Cave are 0.21–0.58, and the value of the overlying soils is >0.9 (Yang et al., 2011). The BIT value of Mawmluh Cave is <0.35 (Huguet et al., 2018), and the BIT values of stalagmites in Australia are <0.1 (Blyth and Schouten, 2013). These data indicate that there is no significant soil input signal in stalagmite GDGTs and that they are more likely to be generated in situ than to be derived from the soil.
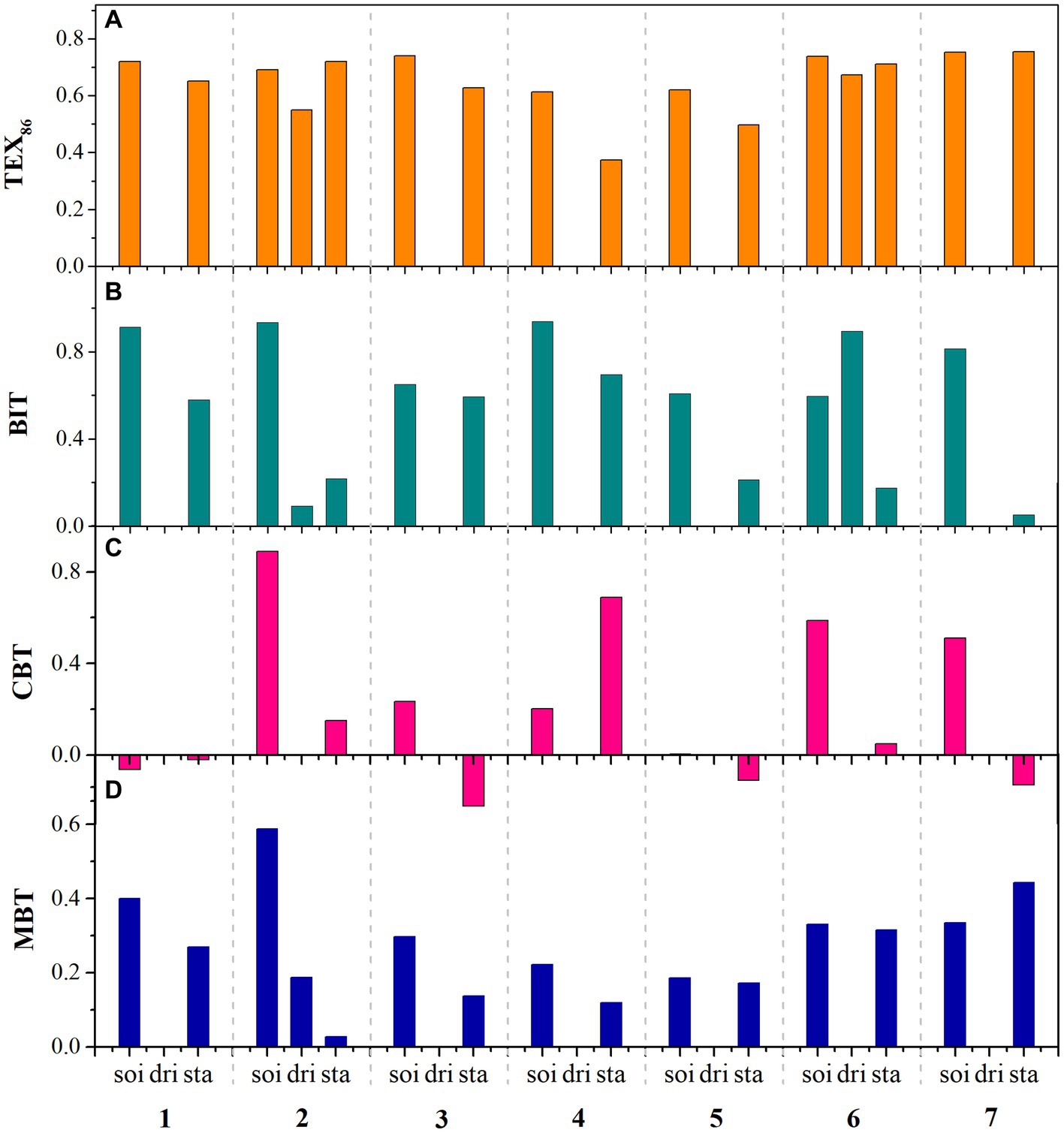
Figure 5. Comparison of GDGT proxies for soils (soi), dripping water (dri), and speleothems (spe) (1, Heshang Cave, Yang et al., 2011; 2, Heshang Cave, Zhang et al., 2020; 3, Wombeyan Caves; 4, Pooles Cavern; 5, Lower Balls Mine; 6, Cathedral Cave; 7, Gaden Cave; data 3–7 from Blyth et al., 2014).
Huguet et al. (2018) showed that the BIT values of the stalagmites in Mawmluh Cave were relatively low (BIT <0.35). The BIT value decreased to ~0.1 during the Holocene, indicating the high contribution of archaea in the cave. During the weak monsoon period, the retention time of dripping water in the surface soil and seepage system increases, and the relative contributions of the microorganisms in the soil or seepage system to GDGT production increase. In contrast, the higher rate of dripping water during the period of strong monsoon reduces the GDGTs produced in situ by microorganisms in caves, so the BIT correlates positively with δ18O (R2 = 0.49, p < 0.005) (Huguet et al., 2018).
5.3. MBT/CBT
Weijers et al. (2007c) proposed the methylation index of branched tetraethers (MBT; Table 1) and the cyclization ratio of branched tetraethers (CBT; Table 1) to describe the relative changes in the number of methyl groups and the number of cyclopentane rings in bacterial brGDGTs, respectively. A negative correlation was detected between MBT and MAAT, which was also affected by soil pH. The CBT proxy, which represents the relative number of cyclopentane rings, is related to the soil pH. Because the pH can be calculated from CBT, the combination of MBT and CBT (MBT/CBT) can jointly reconstruct MAAT, or CBT can be used for pH reconstruction alone (Weijers et al., 2007c).
The MBT and CBT values for stalagmites are generally lower than those for the overlying soil (Figures 5C, 5D). However, pH data were not available at all sampling sites in the published data, which limited the application of the CBT proxy in stalagmites. Nevertheless, the pH of Buchan soil was 6.8–7.5, whereas the pH of the dripping cave water was 6.9–8.0, which is slightly alkaline. The CBT-derived pH of stalagmite was 8.0, suggesting that CBT values reflect pH changes (Blyth and Schouten, 2013). Other studies have found that the CBT proxy in caves overestimates pH (Blyth et al., 2014; Zhang et al., 2020). Because the equation is based on soil and the instantaneous pH of sampling cannot represent the long-term changes in dripping water, it will be necessary to develop a CBT–pH calibration equation for the cave environment to improve its application in the future.
Based on the MBT/CBT calibration equation of Weijers et al. (2007b), Yang et al. (2011) calculated that the temperature range under which the stalagmites in Heshang Cave formed was 1.6–7.5°C, which is significantly different from the temperature in the study area and much lower than the temperature calculated with TEX86. These results indicate that brGDGT-producing bacteria in caves respond differently to temperature than those in soils. There was a significant correlation between stalagmite MBT and MAAT outside the cave (R2 = 0.62) but a weak correlation between stalagmite MBT and MAAT inside the cave (R2 = 0.38). Blyth and Schouten et al. (2013) conducted a multiple regression analysis of stalagmite CBT, MBT, and measured MAAT. Two sets of calibration equations were obtained (surface MAAT, R2 = 0.73, p < 0.001, standard error = 2.7°C; cave MAAT, R2 = 0.56, p = 0.003, standard error = 2.5°C; Table 2). In a multiple regression analysis, the relationship between stalagmite CBT, MBT, and measured MAAT was significantly improved, and the calibration standard error was smaller than that reported in soil (R2 = 0.77, standard error = 5°C) (Weijers et al., 2007b) or a lake (R2 = 0.62, standard error = 2.5°C) (Sun et al., 2011).
Since the GDGTs in stalagmites are dominantly derived from dripping water and cave systems, it is more logical to see that GDGT-derived proxies show a better correlation with the cave MAAT. The cave MAAT is usually close to the surface MAAT. However, published work indicated both TEX86 and MBT/CBT from caves are more closely related to the surface MAAT. This may be ascribed to limited stalagmite GDGTs data and less cave MAAT data (compared to more surface MAAT data). Blyth and Schouten et al. (2013) found both TEX86 and MBT/CBT proxy are better correlated with cave MAAT, if the cave and surface MAAT data were both observed (Table 2). Therefore, more stalagmite GDGTs data and cave monitoring data around the world are needed to improve the accuracy of calibration in the future.
In terms of correlation and standard error, MBT/CBT is not as applicable as the TEX86 proxy, which may be because more-complex factors influence the brGDGTs in stalagmites. In fact, the MBT and CBT proxies for brGDGTs in alkaline environments, such as soil, are influenced by factors other than temperature and pH (Yang et al., 2014; Dang et al., 2016).
5.4. 5-methylated and 6-methylated brGDGT isomer ratio (IR) proxies
De Jonge et al. (2014) found that the presence of 6-methyl brGDGTs was one of the reasons for the bias in the MBT(′)/CBT proxy, and that the higher levels of IIa′ and IIIa′ in arid areas led to lower MBT′. The relative abundance of 6-methyl brGDGTs is mainly controlled by pH, and after 6-methyl brGDGTs were excluded from the MBT′ proxy, MBT′5ME correlated more closely with temperature. The four proxies MBT′5ME, MBT′6ME, CBT5ME, and CBT6ME were then derived (Table 1). It has also been proposed CBT′, thereafter the accuracy of brGDGT-based proxies in temperature and pH reconstructions was improved to a greater extent (De Jonge et al., 2014; Yang et al., 2015).
De Jonge et al. (2014) proposed the use of an isomer ratio (IR; Table 1) representing the relative amounts of methyl isomers in brGDGTs. After separating the 5-methyl and 6-methyl isomers of bacterial brGDGTs. Yang et al. (2015) showed that the 6-methyl brGDGTs in soils from an altitude transect in Shennongjia correlated with the soil pH, and therefore proposed that IRIIIa′ and IRIIa′ be used to reconstruct pH. Dang et al. (2016) investigated the brGDGTs in soils with a gradient of different water contents (0–61%) along the coast of Qinghai Lake, and they found that the soil water content strongly affected 6-methyl brGDGTs, whereas 5-methyl brGDGTs were controlled by temperature. This causes a deviation in the temperatures reconstructed using the MBT′/CBT proxy in arid and semi-arid areas. Therefore, IR6ME can be used to specify the use of proxies: when IR6ME < 0.5, MBT′/CBT can be used as a proxy for temperature, and when IR6ME > 0.5, MBT′ correlates well with the hydrological conditions in the region (Dang et al., 2016). However, the number “0.5” is just an empirical or statistical value. There might be a range within which the MBT is both controlled by temperature and precipitation.
Zhang et al. (2020) tested 5-methyl and 6-methyl brGDGTs from stalagmites using the new method and found that 6-methyl brGDGTs were the main isomer type in stalagmites, whereas 5-methyl brGDGTs were largely below the limit of detection. However, 5-methyl brGDGTs were dominant in the surface soil and the soil profile (Figure 3). The MBT′5ME and MBT′6ME proxies of soil and stalagmites were significantly different, and the pH of the stalagmite reconstructed with CBT5ME was significantly higher than that of the soil, confirming again that the stalagmite GDGTs were not inherited from the overlying soil. Furthermore, the IR value of stalagmites is stable at about 1, which is also quite different from that of soil. The environmental information recorded by 5-methyl and 6-methyl bacterial brGDGTs in stalagmites remains unclear, and the application of proxies established using the new method in reconstructing the paleoclimates of stalagmites has not yet been reported. Moreover, a study of 102 surface sediments from 37 lakes in China found that they contained 7-methyl isomers as well as 5-methyl and 6-methyl isomers of brGDGT compounds (Ding et al., 2016). These results suggest there may be many unidentified compounds in GDGTs, with great potential utility in paleoenvironmental reconstruction.
5.5. Applications of stalagmite GDGTs in the paleoenvironment
So far, stalagmite GDGTs have been used for paleoenvironmental reconstructions in limited works by using. Huguet et al. (2018) first applied GDGTs to the paleoenvironmental reconstruction from stalagmites in Mawmluh cave and found that it could provide both temperature and moisture information. Over the 22 to 6 ka period, the gradual increase in monsoon strength was related to the higher TEX86 temperatures. The highest TEX86-derived temperatures (19–20°C, standard error = 1.9°C) in KM-1 were observed at ~6 ka, which was consistent with the surface and cave MAAT, whereas the temperature of the last glacial maximum (LGM) was about 4°C lower. The GDGT proxies supported the hypothesis of a shift in paleohydrologic conditions at the end of the LGM and before the onset of the Holocene (Huguet et al., 2018). Wassenburg et al. (2021) used TEX86-derived temperatures to deconvolve the effects of temperature in stalagmite δ18OCc. The structure of the TEX86-derived temperatures was similar to δ18OCc record in Jiangjun Cave. Thus, GDGTs can provide a quantitative estimate of temperature and is an important addition to stable isotope records in stalagmites (Huguet et al., 2018).
6. Summary and prospects
(1) Identifying the biological sources of stalagmite GDGTs. At present, the GDGTs in stalagmites have been shown to come from dripping water, in situ production within a cave, and their proportions in stalagmites remain unclear. In cave systems, both archaeal isoGDGTs and bacterial brGDGTs may originate from in situ production in the cave, but the contributions from dripping water cannot be excluded. The biological sources of GDGTs in stalagmites remain unclear, and their clarification requires the use of microbiological and molecular biological techniques. Resolving this issue will extend our understanding of the environmental information recorded by stalagmite GDGTs.
(2) Establishing regional calibration equations. Both TEX86 and MBT/CBT have potential utility as proxies for temperature in stalagmites, and the calculated temperature correlates strongly with the measured temperature. However, the input signal of the overlying soil, the differences in the archaeal communities in caves, and the microclimate at the top of the cave may affect the accuracy of reconstructions. More modern regional monitoring efforts in the future could significantly reduce these uncertainties and improve the accuracy of temperature reconstructions.
(3) Improving extraction and analytical methods. Stalagmite samples are relatively precious, and the content of organic matter is less than 0.3% of the total carbon. Therefore, the lipid content in stalagmites is usually low, and a large number of samples is required to ensure their instrumental detection. Consequently, the high-resolution detection of GDGTs in these samples is difficult. More-efficient methods for GDGT analysis must be established to reduce the amount of samples required, while still obtaining high-resolution paleoclimatic records.
(4) Focusing on new compounds in stalagmite GDGTs. The distributions of 5-methyl and 6-methyl brGDGT compounds separated using the new method in dark caves and oligotrophic environments differ from those in soil, and the environmental factors operating may also be different. However, 5-methyl and 6-methyl bacterial brGDGTs and their related proxies have only been reported in Heshang Cave.
(5) More-extensive paleoenvironment research on stalagmite GDGTs. Stalagmite GDGTs have great potential utility in the quantitative reconstruction of paleoclimates, especially paleotemperatures. However, recent studies have focused mainly on modern processes (paleoclimate reconstruction has only been undertaken in two caves, Mawmluh Cave and Jiangjun Cave). In the future, more-extensive studies of the paleoclimates represented by GDGTs in caves are required to provide supplementary information on the relationships between temperature, pH, and humidity and other proxies, such as isotopes, to better understand and interpret monsoonal changes.
Author contributions
All authors listed have made a substantial and direct contribution to the work and approved it for publication.
Funding
This work was supported by the Strategic Priority Research Program of Chinese Academy of Sciences (XDB40000000), the second Tibetan Plateau Scientific Expedition and Research (2019QZKK0101), and the Fund of Shandong Province (No. LSKJ202203300).
Acknowledgments
We thank editors KB and Xianyu Huang and reviewers ZR and YX for their constructive suggestions. We also thank professor Ashish Sinha for improving the manuscript.
Conflict of interest
The authors declare that the research was conducted in the absence of any commercial or financial relationships that could be construed as a potential conflict of interest.
Publisher’s note
All claims expressed in this article are solely those of the authors and do not necessarily represent those of their affiliated organizations, or those of the publisher, the editors and the reviewers. Any product that may be evaluated in this article, or claim that may be made by its manufacturer, is not guaranteed or endorsed by the publisher.
Supplementary material
The Supplementary material for this article can be found online at: https://www.frontiersin.org/articles/10.3389/fevo.2023.1117599/full#supplementary-material
References
Abrantes, F., Lebreiro, S., Rodrigues, T., Gil, I., Bartels-Jónsdóttir, H., Oliveira, P., et al. (2005). Shallow-marine sediment cores record climate variability and earthquake activity off Lisbon (Portugal) for the last 2000 years. Quat. Sci. Rev. 24, 2477–2494. doi: 10.1016/j.quascirev.2004.04.009
Baker, A., Jex, C. N., Rutlidge, H., Woltering, M., Blyth, A. J., Andersen, M. S., et al. (2016). An irrigation experiment to compare soil, water and speleothem tetraether membrane lipid distributions. Org. Geochem. 94, 12–20. doi: 10.1016/j.orggeochem.2016.01.005
Baker, A., Blyth, A. J., Jex, C. N., Mcdonald, J. A., Woltering, M., and Khan, S. J. (2019). Glycerol dialkyl glycerol tetraethers (GDGT) distributions from soil to cave: refining the speleothem paleothermometer. Org. Geochem. 136:103890. doi: 10.1016/j.orggeochem.2019.06.011
Barton, H. A., Giarrizzo, J. G., Suarez, P., Robertson, C. E., Broering, M. J., Banks, E. D., et al. (2014). Microbial diversity in a Venezuelan orthoquartzite cave is dominated by the Chloroflexi (class Ktedonobacterales) and Thaumarchaeota group I.1c. Front. Microbiol. 5:615. doi: 10.3389/fmicb.2014.00615
Bendle, J. A., Weijers, J. W. H., Maslin, M. A., Sinninghe Damsté, J. S., Schouten, S., Hopmans, E. C., et al. (2010). Major changes in glacial and Holocene terrestrial temperatures and sources of organic carbon recorded in the Amazon fan by tetraether lipids. Geochem. Geophys. Geosyst. 11:Q12007. doi: 10.1029/2010GC003308
Blaga, C. I., Reichart, G. J., Heiri, O., and Sinninghe Damsté, J. S. (2009). Tetraether membrane lipid distributions in water-column particulate matter and sediments: a study of 47 European lakes along a north-south transect. J. Paleolimnol. 41, 523–540. doi: 10.1007/s10933-008-9242-2
Blumenberg, M., Seifert, R., Reitner, J., Pape, T., and Michaelis, W. (2004). Membrane lipid patterns typify distinct anaerobic methanotrophic consortia. Proc. Natl. Acad. Sci. U. S. A. 101, 11111–11116. doi: 10.1073/pnas.0401188101
Blyth, A. J., Farrimond, P., and Jones, M. (2006). An optimised method for the extraction and analysis of lipid biomarkers from stalagmites. Org. Geochem. 37, 882–890. doi: 10.1016/j.orggeochem.2006.05.003
Blyth, A. J., and Schouten, S. (2013). Calibrating the glycerol dialkyl glycerol tetraether temperature signal in speleothems. Geochim. Cosmochim. Acta 109, 312–328. doi: 10.1016/j.gca.2013.02.009
Blyth, A. J., Shutova, Y., and Smith, C. (2013). δ13C analysis of bulk organic matter in speleothems using liquid chromatography-isotope ratio mass spectrometry. Org. Geochem. 55, 22–25. doi: 10.1016/j.orggeochem.2012.11.003
Blyth, A. J., Jex, C. N., Baker, A., Khan, S. J., and Schouten, S. (2014). Contrasting distributions of glycerol dialkyl glycerol tetraethers (GDGTs) in speleothems and associated soils. Org. Geochem. 69, 1–10. doi: 10.1016/j.orggeochem.2014.01.013
Blyth, A. J., Hartland, A., and Baker, A. (2016). Organic proxies in speleothems-new developments, advantages and limitations. Quat. Sci. Rev. 149, 1–17. doi: 10.1016/j.quascirev.2016.07.001
Buckles, L. K., Villanueva, L., Weijers, J. W. H., Verschuren, D., and Sinninghe Damsté, J. S. (2013). Linking isoprenoidal GDGT membrane lipid distributions with gene abundances of ammonia-oxidizing Thaumarchaeota and uncultured crenarchaeotal groups in the water column of a tropical Lake (lake Challa, East Africa). Environ. Microbiol. 15, 2445–2462. doi: 10.1111/1462-2920.12118
Burgess, E. A., Unrine, J. M., Mills, G. L., Romanek, C. S., and Wiegel, J. (2012). Comparative geochemical and microbiological characterization of two thermal pools in the Uzon caldera, Kamchatka, Russia. Microb. Ecol. 63, 471–489. doi: 10.1007/s00248-011-9979-4
Cai, Y. J., Tan, L. C., Cheng, H., An, Z. S., Edwards, R. L., Kelly, M. J., et al. (2010). The variation of summer monsoon precipitation in Central China since the last deglaciation. Earth Planet. Sci. Lett. 291, 21–31. doi: 10.1016/j.epsl.2009.12.039
Castañeda, I. S., and Schouten, S. (2011). A review of molecular organic proxies for examining modern and ancient lacustrine environments. Quat. Sci. Rev. 30, 2851–2891. doi: 10.1016/j.quascirev.2011.07.009
Castañeda, I. S., Caley, T., Dupont, L., Kim, J., Malaizé, B., and Schouten, S. (2016). Middle to late Pleistocene vegetation and climate change in subtropical southern East Africa. Earth Planet. Sci. Lett. 450, 306–316. doi: 10.1016/j.epsl.2016.06.049
Chen, Y. F., Zheng, F. F., Yang, H., Yang, W., Wu, R. J., Liu, X. Y., et al. (2022). The production of diverse brGDGTs by an Acidobacterium allows a direct test of temperature and pH controls on their distribution. Geochim. Cosmochim. Acta 337, 155–165. doi: 10.1016/j.gca.2022.08.033
Cheng, H., Edwards, R. L., Sinha, A., Sptl, C., Yi, L., Chen, S. T., et al. (2016). The Asian monsoon over the past 640,000 years and ice age terminations. Nature 534, 640–646. doi: 10.1038/nature18591
Dang, X. Y., Yang, H., Naafs, B. D. A., Pancost, R. D., and Xie, S. C. (2016). Evidence of moisture control on the methylation of branched glycerol dialkyl glycerol tetraethers in semi-arid and arid soils. Geochim. Cosmochim. Acta 189, 24–36. doi: 10.1016/j.gca.2016.06.004
De Jonge, C., Hopmans, E. C., Zell, C. I., Kim, J.-H., Schouten, S., and Sinninghe Damsté, J. S. (2014). Occurrence and abundance of 6-methyl branched glycerol dialkyl glycerol tetraethers in soils: implications for palaeoclimate reconstruction. Geochim. Cosmochim. Acta 141, 97–112. doi: 10.1016/j.gca.2014.06.013
de la Torre, J., Walker, C., Ingalls, A. E., Knneke, M., and Stahl, D. A. (2008). Cultivation of a thermophilic ammonia oxidizing archaeon synthesizing crenarchaeol. Environ. Microbiol. 10, 810–818. doi: 10.1111/j.1462-2920.2007.01506.x
Delong, E. F., King, L. L., Massana, R., Cittone, H., Murray, A., Schleper, C., et al. (1998). Dibiphytanyl ether lipids in nonthermophilic crenarchaeotes. Appl. Environ. Microbiol. 64, 1133–1138. doi: 10.1128/AEM.64.3.1133-1138.1998
Ding, S., Schwab, V. F., Ueberschaar, N., Roth, V. N., and Pohnert, G. (2016). Identification of novel 7-methyl and cyclopentanyl branched glycerol dialkyl glycerol tetraethers in lake sediments. Org.Geochem 102, 52–58. doi: 10.1016/j.orggeochem.2016.09.009
Ding, S., Kohlhepp, B., Trumbore, S., Küsel, K., Totsche, K.-U., Pohnert, G., et al. (2018). In situ production of core and intact bacterial and archaeal tetraether lipids in groundwater. Org. Geochem. 126, 1–12. doi: 10.1016/j.orggeochem.2018.10.005
Dong, L., Li, Q. Y., Li, L., and Zhang, C. L. (2015). Glacial-interglacial contrast in MBT/CBT proxies in the South China Sea: implications for marine production of branched GDGTs and continental teleconnection. Org. Geochem. 79, 74–82. doi: 10.1016/j.orggeochem.2014.12.008
Dorale, J. A., Edwards, R. L., Ito, E., and Conzalez, L. A. (1998). Climate and vegetation history of the midcontinent from 75 to 25 ka: a speleothem record from crevice cave, Missouri, USA. Science 282, 1871–1874. doi: 10.1126/science.282.5395.1871
Elling, F. J., Könneke, M., Nicol, G. W., Stieglmeier, M., Bayer, B., Spieck, E., et al. (2017). Chemotaxonomic characterisation of the thaumarchaeal lipidome. Environ. Microbiol. 18, 2681–2700.doi: 10.1111/1462-2920.13759
Gao, L., Nie, J. S., Clemens, S., Liu, W. G., Sun, J. M., Zech, R., et al. (2012). The importance of solar insolation on the temperature variations for the past 110 kyr on the Chinese loess plateau. Palaeogeogr. Palaeoclimatol. Palaeoecol. 317-318, 128–133. doi: 10.1016/j.palaeo.2011.12.021
Genty, D., Blamart, D., Ouahdi, R., Baker, A., Jouzel, J., and Van-Exter, S. (2003). Precise dating of Dansgaard-Oeschger climate oscillations in western Europe from stalagmite data. Nature 421, 833–837. doi: 10.1038/nature01391
Gonzalez, J. M., Portillo, M. C., and Saiz-Jimenez, C. (2006). Metabolically active Crenarchaeota in Altamira cave. Sci. Nat. 93, 42–45. doi: 10.1007/s00114-005-0060-3
Günther, F., Thiele, A., Gleixner, G., Xu, B. Q., Yao, T. D., and Schouten, S. (2014). Distribution of bacterial and archaeal ether lipids in soils and surface sediments of Tibetan lakes: implications for GDGT-based proxies in saline high mountain lakes. Org. Geochem. 67, 19–30. doi: 10.1016/j.orggeochem.2013.11.014
Halamka, T. A., Raberg, J. H., McFarlin, J. M., Younkin, A. D., Mulligan, C., Liu, X.-L., et al. (2022). Production of diverse brGDGTs by Acidobacterium Solibacter usitatus in response to temperature, pH, and O2 provides a culturing perspective on brGDGT proxies and biosynthesis. Geobiology 00, 1–17. doi: 10.1111/gbi.12525
Hendy, C. H. (1971). The isotopic geochemistry of speleothems: I. the calculation of the effects of different modes of formation on the isotopic composition of speleothems and their applicability as paleoclimatic indicators[J]. Geochim. Cosmochim. Acta 35, 801–824. doi: 10.1016/0016-7037(71)90127-X
Herfort, L., Schouten, S., Boon, J. P., and Sinninghe Damsté, J. S. (2006). Application of the TEX86 temperature proxy to the southern North Sea. Org. Geochem. 37, 1715–1726. doi: 10.1016/j.orggeochem.2006.07.021
Hoefs, M. J. L., Schouten, S., De Leeuw, J., King, L. L., Wakeham, S., and Sinninghe Damsté, J. S. (1997). Ether lipids of planktonic archaea in the marine water column. Appl. Environ. Microbiol. 63, 3090–3095. doi: 10.1128/aem.63.8.3090-3095.1997
Hopmans, E. C., Schouten, S., Pancost, R. D., van der Meer, M. T., and Sinninghe Damsté, J. S. (2000). Analysis of intact tetraether lipids in archaeal cell material and sediments by high performance liquid chromatography/atmospheric pressure chemical ionization mass spectrometry. Rapid Commun. Mass Spectrom. 14, 585–589. doi: 10.1002/(SICI)1097-0231(20000415)14:7<585::AID-RCM913>3.0.CO;2-N
Hopmans, E. C., Weijers, J. W. H., Schefuß, E., Herfort, L., Sinninghe Damsté, J. S., and Schouten, S. (2004). A novel proxy for terrestrial organic matter in sediments based on branched and isoprenoid tetraether lipids. Earth Planet. Sci. Lett. 224, 107–116. doi: 10.1016/j.epsl.2004.05.012
Hopmans, E. C., Schouten, S., and Sinninghe Damsté, J. S. (2016). The effect of improved chromatography on GDGT-based palaeoproxies. Org. Geochem. 93, 1–6. doi: 10.1016/j.orggeochem.2015.12.006
Hu, C. Y., Henderson, G. M., Huang, J. H., Xie, S. C., Sun, Y., and Johnson, K. R. (2008). Quantification of Holocene Asian monsoon rainfall from spatially separated cave records. Earth Planet. Sci. Lett. 266, 221–232. doi: 10.1016/j.epsl.2007.10.015
Hu, J. F., Zhou, H. D., Peng, P. A., Yang, X. Q., and Spiro, B. (2015). Reconstruction of a paleotemperature record from 0.3–3.7 ka for subtropical South China using lacustrine branched GDGTs from Huguangyan maar. Palaeogeogr. Palaeoclimatol. Palaeoecol. 435, 167–176. doi: 10.1016/j.palaeo.2015.06.014
Huang, X. Y., Pu, Y., Cui, J. W., Wu, Y. M., Li, X. L., Huang, J. H., et al. (2007). Distributions of fatty acids in the dripping water at Heshang cave, Hubei Province and the ecological implications. Quat. Sci. 27, 401–407.
Huang, X. Y., Cui, J. W., Pu, Y., Huang, J. H., and Blyth, A. J. (2008). Identifying "free" and "bound" lipid fractions in stalagmite samples: An example from Heshang cave, southern China. Appl. Geochem. 23, 2589–2595. doi: 10.1016/j.apgeochem.2008.05.008
Huguet, C., Hopmans, E. C., Febo-Ayala, W., Thompson, D. H., and Schouten, S. (2006). An improved method to determine the absolute abundance of glycerol dibiphytanyl glycerol tetraether lipids. Org. Geochem. 37, 1036–1041. doi: 10.1016/j.orggeochem.2006.05.008
Huguet, A., Fosse, C., Laggoun-Défarge, F., Delarue, F., and Derenne, S. (2013). Effects of a short-term experimental microclimate warming on the abundance and distribution of branched GDGTs in a French peatland. Geochim. Cosmochim. Acta 105, 294–315. doi: 10.1016/j.gca.2012.11.037
Huguet, C., Routh, J., Fietz, S., Lone, M. A., Kalpana, M. S., Ghosh, P., et al. (2018). Temperature and monsoon tango in a tropical stalagmite: last glacial-interglacial climate dynamics. Sci. Rep. 8:5386. doi: 10.1038/s41598-018-23606-w
Jenkyns, H. C., Schouten-Huibers, L., Schouten, S., and Sinninghe Damsté, J. S. (2012). Warm middle Jurassic-early cretaceous high-latitude sea-surface temperatures from the Southern Ocean. Clim. Past 8, 215–226. doi: 10.5194/cp-8-215-2012
Jia, G. D., Rao, Z. G., Zhang, J., Li, Z. Y., and Chen, F. H. (2013). Tetraether biomarker records from a loess-paleosol sequence in the western Chinese loess plateau. Front. Microbiol. 4:199. doi: 10.3389/fmicb.2013.00199
Jones, R. T., Robeson, M. S., Lauber, C. L., Hamady, M., and Knight, R. (2009). A comprehensive survey of soil acidobacterial diversity using pyrosequencing and clone library analyses. ISME J. 3, 442–453. doi: 10.1038/ismej.2008.127
Kurth, J. M., Smit, N. T., Berger, S., Schouten, S., Jetten, M. S. M., and Welte, C. U. (2019). ANME-2d anaerobic methanotrophic archaea differ from other ANME archaea in lipid composition and carbon source. FEMS Microbiol. Ecol. 95:fiz082. doi: 10.1093/femsec/fiz082
Kuypers, M. M., Blokker, P., Erbacher, J., Kinkel, H., Pancost, R. D., Schouten, S., et al. (2001). Massive expansion of marine archaea during a mid-cretaceous oceanic anoxic event. Science 293, 92–95. doi: 10.1126/science.1058424
Leider, A., Hinrichs, K.-U., Mollenhauer, G., and Versteegh, G. J. M. (2010). Core-top calibration of the lipid-based UK′37and TEX86 temperature proxies on the southern Italian shelf (SW Adriatic Sea, Gulf of Taranto). Earth Planet. Sci. Lett. 300, 112–124. doi: 10.1016/j.epsl.2010.09.042
Li, X. L., Hu, C. Y., Huang, J. H., Xie, S. C., and Baker, A. (2014). A 9000-year carbon isotopic record of acid-soluble organic matter in a stalagmite from Heshang cave, Central China: paleoclimate implications. Chem. Geol. 388, 71–77. doi: 10.1016/j.chemgeo.2014.08.029
Lipp, J. S., Morono, Y., Inagaki, F., and Hinrichs, K. U. (2008). Significant contribution of archaea to extant biomass in marine subsurface sediments. Nature 454, 991–994. doi: 10.1038/nature07174
Liu, X.-L., Leider, A., Gillespie, A., Gröger, J., Versteegh, G. J. M., and Hinrichs, K.-U. (2010). Identification of polar lipid precursors of the ubiquitous branched GDGT orphan lipids in a peat bog in northern Germany. Org. Geochem. 41, 653–660. doi: 10.1016/j.orggeochem.2010.04.004
Loomis, S. E., Russell, J. M., and Sinninghe Damsté, J. S. (2011). Distributions of branched GDGTs in soils and lake sediments from western Uganda: implications for a lacustrine paleothermometer. Org. Geochem. 42, 739–751. doi: 10.1016/j.orggeochem.2011.06.004
Lu, H. X., Liu, W. G., Yang, H., Wang, H. Y., Liu, Z. H., Leng, Q., et al. (2019). 800-kyr land temperature variations modulated by vegetation changes on Chinese loess plateau. Nat. Commun. 10:1958. doi: 10.1038/s41467-019-09978-1
Lü, X. X., Liu, X.-L., Elling, F. J., Yang, H., and Xie, S. C. (2015). Hydroxylated isoprenoid GDGTs in Chinese coastal seas and their potential as a paleotemperature proxy for mid-to-low latitude marginal seas - ScienceDirect. Org. Geochem. 89-90, 31–43. doi: 10.1016/j.orggeochem.2015.10.004
Maher, B. A. (2008). Holocene variability of the east Asian summer monsoon from Chinese cave records: a re-assessment. The Holocene 18, 861–866. doi: 10.1177/0959683608095569
Maher, B. A., and Thompson, R. (2012). Oxygen isotopes from Chinese caves: records not of monsoon rainfall but of circulation regime. J. Quat. Sci. 27, 615–624. doi: 10.1002/jqs.2553
Man, Y., Fan, J. C., Huang, M., and Yang, H. (2023). An unusual occurrence of hydroxylated isoprenoid GDGTs in forest soils. Org. Geochem. 177:104540. doi: 10.1016/j.orggeochem.2022.104540
McDermott, F. (2004). Palaeo-climate reconstruction from stable isotope variations in speleothems: a review. Quat. Sci. Rev. 23, 901–918. doi: 10.1016/j.quascirev.2003.06.021
Meyers, P. A., and Ishiwatari, R. (1993). Lacustrine organic geochemistry-an overview of indicators of organic matter sources and diagenesis in lake sediments. Org. Geochem. 20, 867–900. doi: 10.1016/0146-6380(93)90100-P
Naafs, B. D. A., Rohrssen, M., Inglis, G. N., Lähteenoja, O., Feakins, S. J., Collinson, M. E., et al. (2018). High temperatures in the terrestrial mid-latitudes during the early Palaeogene. Nat. Geosci. 11, 766–771. doi: 10.1038/s41561-018-0199-0
Pearson, A., Huang, Z., Ingalls, A. E., Romanek, C. S., Wiegel, J., Freeman, K. H., et al. (2004). Nonmarine crenarchaeol in Nevada hot springs. Appl. Environ. Microbiol. 70, 5229–5237. doi: 10.1128/AEM.70.9.5229-5237.2004
Pearson, A., Pi, Y. D., Zhao, W. D., Li, W. J., Li, Y. L., Inskeep, W., et al. (2008). Factors controlling the distribution of archaeal Tetraethers in terrestrial Hot Springs. Appl. Environ. Microbiol. 74, 3523–3532. doi: 10.1128/AEM.02450-07
Pei, H. Y., Wang, C. F., Wang, Y. B., Yang, H., and Xie, S. C. (2019). Distribution of microbial lipids at an acid mine drainage site in China: insights into microbial adaptation to extremely low pH conditions. Org. Geochem. 134, 77–91. doi: 10.1016/j.orggeochem.2019.05.008
Pelejero, C., and Calvo, E. (2003). The upper end of the UK’37 temperature calibration revisited. Geochem. Geophys. Geosyst. 4:1014. doi: 10.1029/2002GC000431
Peterse, F., Nicol, G. W., Schouten, S., and Sinninghe Damsté, J. S. (2010). Influence of soil pH on the abundance and distribution of core and intact polar lipid-derived branched GDGTs in soil. Org. Geochem. 41, 1171–1175. doi: 10.1016/j.orggeochem.2010.07.004
Peterse, F., Hopmans, E. C., Schouten, S., Mets, A., Rijpstra, W. I. C., and Sinninghe Damsté, J. S. (2011). Identification and distribution of intact polar branched tetraether lipids in peat and soil. Org. Geochem. 42, 1007–1015. doi: 10.1016/j.orggeochem.2011.07.006
Peterse, F., van der Meer, J., Schouten, S., Weijers, J. W. H., Fierer, N., Jackson, R. B., et al. (2012). Revised calibration of the MBT-CBT paleotemperature proxy based on branched tetraether membrane lipids in surface soil. Geochim. Cosmochim. Acta 96, 215–229. doi: 10.1016/j.gca.2012.08.011
Pitcher, A., Rychlik, N., Hopmans, E. C., Spieck, E., Rijpstra, W. I. C., Ossebaar, J., et al. (2010). Crenarchaeol dominates the membrane lipids of Candidatus Nitrososphaera gargensis, a thermophilic group I.1b archaeon. ISME J. 4, 542–552. doi: 10.1038/ismej.2009.138
Pitcher, A., Hopmans, E. C., Mosier, A. C., Park, S.-J., Rhee, S.-K., Francis, C. A., et al. (2011a). Core and intact polar glycerol dibiphytanyl glycerol tetraether lipids of ammonia-oxidizing archaea enriched from marine and estuarine sediments. Appl. Environ. Microbiol. 77, 3468–3477. doi: 10.1128/AEM.02758-10
Pitcher, A., Villanueva, L., Hopmans, E. C., Schouten, S., Reichart, G. J., and Sinninghe Damsté, J. S. (2011b). Niche segregation of ammonia-oxidizing archaea and anammox bacteria in the Arabian Sea oxygen minimum zone. ISME J. 5, 1896–1904. doi: 10.1038/ismej.2011.60
Powers, L., Werne, J. P., and Vanderwoude, A. J. (2010). Applicability and calibration of the TEX86 paleothermometer in lakes. Org. Geochem. 41, 404–413. doi: 10.1016/j.orggeochem.2009.11.009
Pross, J., Contreras, L., Bijl, P. K., Greenwood, D. R., Bohaty, S. M., Schouten, S., et al. (2012). Persistent near-tropical warmth on the Antarctic continent during the early Eocene epoch. Nature 488, 73–77. doi: 10.1038/nature11300
Quiers, M., Perrette, Y., Chalmin, E., Fanget, B., and Poulenard, J. M. (2015). Geochemical mapping of organic carbon in stalagmites using liquid-phase and solid-phase fluorescence. Chem. Geol. 411, 240–247. doi: 10.1016/j.chemgeo.2015.07.012
Roberts, M. S., Smart, P. L., and Baker, A. (1998). Annual trace element variations in a Holocene speleothem. Earth Planet. Sci. Lett. 154, 237–246. doi: 10.1016/S0012-821X(97)00116-7
Schouten, S., Hopmans, E. C., Schefuß, E., and Sinninghe Damsté, J. S. (2002). Distributional variations in marine crenarchaeotal membrane lipids: a new tool for reconstructing ancient sea water temperatures? Earth Planet. Sci. Lett. 204, 265–274. doi: 10.1016/S0012-821X(02)00979-2
Schouten, S., Hopmans, E. C., Forster, A., van Breugel, Y., Kuypers, M. M. M., and Sinninghe Damsté, J. S. (2003). Extremely high sea-surface temperatures at low latitudes during the middle cretaceous as revealed by archaeal membrane lipids. Geology 31, 1069–1072. doi: 10.1130/G19876.1
Schouten, S., Ossebaar, J., Brummer, G. J., Elderfield, H., and Sinninghe Damsté, J. S. (2007a). Transport of terrestrial organic matter to the deep North Atlantic Ocean by ice rafting. Org. Geochem. 38, 1161–1168. doi: 10.1016/j.orggeochem.2007.02.012
Schouten, S., van der Meer, M. V. D., Hopmans, E. C., Rijpstra, W. I. C., Reysenbach, A. L., Ward, D. M., et al. (2007b). Archaeal and bacterial glycerol Dialkyl glycerol Tetraether lipids in Hot Springs of Yellowstone National Park. Appl. Environ. Microbiol. 73, 6181–6191. doi: 10.1128/AEM.00630-07
Schouten, S., Rijpstra, W., Durisch-Kaiser, E., Schubert, C. J., and Sinninghe Damsté, J. S. (2012). Distribution of glycerol dialkyl glycerol tetraether lipids in the water column of Lake Tanganyika. Org. Geochem. 53, 34–37. doi: 10.1016/j.orggeochem.2012.01.009
Schouten, S., Hopmans, E. C., and Sinninghe Damsté, J. S. (2013). The organic geochemistry of glycerol dialkyl glycerol tetraether lipids: a review. Org. Geochem. 54, 19–61. doi: 10.1016/j.orggeochem.2012.09.006
Sinninghe Damsté, J. S., Ossebaar, J., Abbas, B., Schouten, S., and Verschuren, D. (2009). Fluxes and distribution of tetraether lipids in an equatorial African lake: constraints on the application of the TEX86 palaeothermometer and BIT index in lacustrine settings. Geochim. Cosmochim. Acta 73, 4232–4249. doi: 10.1016/j.gca.2009.04.022
Sinninghe Damsté, J. S., Rijpstra, W. I., Hopmans, E. C., Weijers, J. W., Foesel, B. U., Overmann, J., et al. (2011). 13,16-dimethyl octacosanedioic acid (iso-diabolic acid), a common membrane-spanning lipid of Acidobacteria subdivisions 1 and 3. Appl. Environ. Microbiol. 77, 4147–4154. doi: 10.1128/AEM.00466-11
Sinninghe Damsté, J. S., Ossebaar, J., Schouten, S., and Verschuren, D. (2012a). Distribution of tetraether lipids in the 25-ka sedimentary record of Lake Challa: extracting reliable TEX86 and MBT/CBT palaeotemperatures from an equatorial African lake. Quat. Sci. Rev. 50, 43–54. doi: 10.1016/j.quascirev.2012.07.001
Sinninghe Damsté, J. S., Rijpstra, W. I. C., Hopmans, E. C., Jung, M.-Y., Kim, J.-G., Rhee, S.-K., et al. (2012b). Intact polar and core glycerol Dibiphytanyl glycerol Tetraether lipids of group I.1a and I.1b Thaumarchaeota in soil. Appl. Environ. Microbiol. 78, 6866–6874. doi: 10.1128/AEM.01681-12
Sinninghe Damsté, J. S., Rijpstra, W. I. C., and Hopmans, E. C. (2014). Ether- and Ester-Boundiso-diabolic acid and other lipids in members of Acidobacteria subdivision 4. Appl. Environ. Microbiol. 80, 5207–5218. doi: 10.1128/AEM.01066-14
Sinninghe Damsté, J. S., Rijpstra, W. I. C., Foesel, B. U., Huber, K. J., Overmann, J., Nakagawa, S., et al. (2018). An overview of the occurrence of ether- and ester-linked iso-diabolic acid membrane lipids in microbial cultures of the Acidobacteria: implications for brGDGT paleoproxies for temperature and pH. Org. Geochem. 124, 63–76. doi: 10.1016/j.orggeochem.2018.07.006
Sturt, H. F., Summons, R. E., Smith, K., Elvert, M., and Hinrichs, K. U. (2004). Intact polar membrane lipids in prokaryotes and sediments deciphered by high-performance liquid chromatography/electrospray ionization multistage mass spectrometry - new biomarkers for biogeochemistry and microbial ecology. Rapid Commun. Mass Spectrom. 18, 617–628. doi: 10.1002/rcm.1378
Sun, Q., Chu, G. Q., Liu, M. M., Xie, M. M., and Li, S. Q. (2011). Distributions and temperature dependence of branched glycerol dialkyl glycerol tetraethers in recent lacustrine sediments from China and Nepal. J. Geophys. Res. 116:G01008. doi: 10.1029/2010JG001365
Tan, L. C., Shen, C.-C., Cai, Y. J., Lo, L., Cheng, H., and An, Z. S. (2014). Trace-element variations in an annually layered stalagmite as recorders of climatic changes and anthropogenic pollution in Central China. Quat. Res. 81, 181–188. doi: 10.1016/j.yqres.2013.12.001
Tan, L. C., Cai, Y. J., Cheng, H., Edwards, L. R., Gao, Y. L., Xu, H., et al. (2018). Centennial- to decadal-scale monsoon precipitation variations in the upper Hanjiang River region, China over the past 6650 years. Earth Planet. Sci. Lett. 482, 580–590. doi: 10.1016/j.epsl.2017.11.044
Tang, C. Y., Yang, H., Pancost, R. D., Griffiths, M. L., Xiao, G. Q., Dang, X. Y., et al. (2017). Tropical and high latitude forcing of enhanced megadroughts in northern China during the last four terminations. Earth Planet. Sci. Lett. 479, 98–107. doi: 10.1016/j.epsl.2017.09.012
Tierney, J. E., Schouten, S., Pitcher, A., Hopmans, E. C., and Sinninghe Damsté, J. S. (2012). Core and intact polar glycerol dialkyl glycerol tetraethers (GDGTs) in sand pond, Warwick, Rhode Island (USA): insights into the origin of lacustrine GDGTs. Geochim. Cosmochim. Acta 77, 561–581. doi: 10.1016/j.gca.2011.10.018
Timonen, S., and Bomberg, M. (2009). Archaea in dry soil environments. Phytochem. Rev. 8, 505–518. doi: 10.1007/s11101-009-9137-5
Uda, I., Sugai, A., Itoh, Y. H., and Itoh, T. (2001). Variation in molecular species of polar lipids from Thermoplasma acidophilum depends on growth temperature. Lipids 36, 103–105. doi: 10.1007/s11745-001-0914-2
Wang, C. F., Zhang, H. B., Huang, X. Y., Huang, J. H., and Xie, S. C. (2012). Optimization of acid digestion conditions on the extraction of fatty acids from stalagmites. Front. Earth Sci. 6, 109–114. doi: 10.1007/s11707-012-0311-5
Wang, C. F., Bendle, J. A., Zhang, H. B., Yang, Y., Liu, D., Huang, J. H., et al. (2018). Holocene temperature and hydrological changes reconstructed by bacterial 3-hydroxy fatty acids in a stalagmite from Central China. Quat. Sci. Rev. 192, 97–105. doi: 10.1016/j.quascirev.2018.05.030
Wang, H. Y., Liu, W. G., Zhang, C. L., Dong, H. L., Jiang, H. C., and Lu, H. X. (2017). Hydroxylated glycerol dialkyl glycerol tetraethers in Lake Qinghai sediments and their paleoclimate implications. Quat. Sci. 37, 1151–1160.
Wang, H. Y., An, Z. S., Lu, H. X., Zhao, Z. H., and Liu, W. G. (2020). Calibrating bacterial tetraether distributions towards in situ soil temperature and application to a loess-paleosol sequence. Quat. Sci. Rev. 231:106172. doi: 10.1016/j.quascirev.2020.106172
Wang, M. Y., Zheng, Z., Man, M., Hu, J., and Gao, Q. (2017). Branched GDGT-based paleotemperature reconstruction of the last 30,000 years in humid monsoon region of Southeast China. Chem. Geol. 463, 94–102. doi: 10.1016/j.chemgeo.2017.05.014
Wang, Y. J., Cheng, H., Edwards, R. L., An, Z. S., Wu, J. Y., Shen, C.-C., et al. (2001). A high-resolution absolute-dated late Pleistocene monsoon record from Hulu cave, China. Science 294, 2345–2345, 2348. doi: 10.1126/science.1064618
Wassenburg, J. A., Vonhof, H. B., Cheng, H., Martinez-Garcia, A., Ebner, P. R., Li, X. L., et al. (2021). Penultimate deglaciation Asian monsoon response to North Atlantic circulation collapse. Nat. Geosci. 14, 937–941. doi: 10.1038/s41561-021-00851-9
Weidler, G. W., Dornmayr-Pfaffenhuemer, M., Gerbl, F. W., Heinen, W., and Stan-Lotter, H. (2007). Communities of archaea and bacteria in a subsurface radioactive thermal spring in the Austrian Central Alps, and evidence of ammonia-oxidizing Crenarchaeota. Appl. Environ. Microbiol. 73, 259–270. doi: 10.1128/AEM.01570-06
Weijers, J. W. H., Schouten, S., Spaargaren, O. C., and Sinninghe Damsté, J. S. (2006a). Occurrence and distribution of tetraether membrane lipids in soils: implications for the use of the TEX86 proxy and the BIT index. Org. Geochem. 37, 1680–1693. doi: 10.1016/j.orggeochem.2006.07.018
Weijers, J. W. H., Schouten, S., Hopmans, E. C., Geenevasen, J. A. J., David, O. R. P., Coleman, J. M., et al. (2006b). Membrane lipids of mesophilic anaerobic bacteria thriving in peats have typical archaeal traits. Environ. Microbiol. 8, 648–657. doi: 10.1111/j.1462-2920.2005.00941.x
Weijers, J. W. H., Schefuß, E., Schouten, S., and Sinninghe Damsté, J. S. (2007a). Coupled thermal and hydrological evolution of tropical Africa over the last deglaciation. Science 315, 1701–1704. doi: 10.1126/science.113813
Weijers, J. W. H., Schouten, S., Sluijs, A., Brinkhuis, H., and Sinninghe Damsté, J. S. (2007b). Warm arctic continents during the Palaeocene-Eocene thermal maximum. Earth Planet. Sci. Lett. 261, 230–238. doi: 10.1016/j.epsl.2007.06.033
Weijers, J. W. H., Schouten, S., van den Donker, J., Hopmans, E. C., and Sinninghe Damsté, J. S. (2007c). Environmental controls on bacterial tetraether membrane lipid distribution in soils. Geochim. Cosmochim. Acta 71, 703–713. doi: 10.1016/j.gca.2006.10.003
Weijers, J. W. H., Panoto, E., van Bleijswijk, J., Schouten, S., Rijpstra, W. I. C., Balk, M., et al. (2009). Constraints on the biological source(s) of the orphan branched tetraether membrane lipids. Geomicrobiol J. 26, 402–414. doi: 10.1080/01490450902937293
Weijers, J. W. H., Bernhardt, B., Peterse, F., Werne, J. P., Dungait, J. A. J., Schouten, S., et al. (2011). Absence of seasonal patterns in MBT-CBT indices in mid-latitude soils. Geochim. Cosmochim. Ac. 75, 3179–3190. doi: 10.1016/j.gca.2011.03.015
Wu, Y. C., Tan, L. C., Liu, W. X., Wang, B. Z., Wang, J. J., and Cai, Y. J. (2015). Profiling bacterial diversity in a limestone cave of the western loess plateau of China. Front. Microbiol. 6:244. doi: 10.3389/fmicb.2015.00244
Wuchter, C., Schouten, S., Coolen, M. J. L., and Sinninghe Damsté, J. S. (2004). Temperature-dependent variation in the distribution of tetraether membrane lipids of marine Crenarchaeota: implications for TEX86 paleothermometry. Paleoceanography 19:PA4028. doi: 10.1029/2004PA001041
Xie, W., Zhang, C. L., and Ma, C. L. (2015). Temporal variation in community structure and lipid composition of Thaumarchaeota from subtropical soil: insight into proposing a new soil pH proxy. Org. Geochem. 83-84, 54–64. doi: 10.1016/j.orggeochem.2015.02.009
Xie, S. C., Yi, Y., Huang, J. H., Hu, C. Y., Cai, Y. J., Collins, M., et al. (2003). Lipid distribution in a subtropical southern China stalagmite as a record of soil ecosystem response to paleoclimate change. Quat. Res. 60, 340–347. doi: 10.1016/j.yqres.2003.07.010
Yang, H., Ding, W. H., Zhang, C. L., Xia, W., and Xie, S. C. (2011). Occurrence of tetraether lipids in stalagmites: implications for sources and GDGT-based proxies. Org. Geochem. 42, 108–115. doi: 10.1016/j.orggeochem.2010.11.006
Yang, H., Pancost, R. D., Dang, X. Y., Zhou, X., Evershed, R. P., Xiao, G. Q., et al. (2014). Correlations between microbial tetraether lipids and environmental variables in Chinese soils: optimizing the paleo-reconstructions in semi-arid and arid regions. Geochim. Cosmochim. Acta 126, 49–69. doi: 10.1016/j.gca.2013.10.041
Yang, H., Lü, X. X., Ding, W. H., Lei, Y. Y., Dang, X. Y., and Xie, S. C. (2015). The 6-methyl branched tetraethers significantly affect the performance of the methylation index (MBT') in soils from an altitudinal transect at Mount Shennongjia. Org. Geochem. 82, 42–53. doi: 10.1016/j.orggeochem.2015.02.003
Yang, H., Xiao, W. J., Słowakiewicz, M., Ding, W. H., Ayari, A., Dang, X. Y., et al. (2019). Depth-dependent variation of archaeal ether lipids along soil and peat profiles from southern China: implications for the use of isoprenoidal GDGTs as environmental tracers. Org. Geochem. 128, 42–56. doi: 10.1016/j.orggeochem.2018.12.009
Yun, Y., Xiang, X., Wang, H., Man, B., Gong, L., Liu, Q., et al. (2015). Five-year monitoring of bacterial communities in dripping water from the Heshang cave in Central China: implication for paleoclimate reconstruction and ecological functions. Geomicrobiol J. 33, 1–11. doi: 10.1080/01490451.2015.1062062
Zech, R., Gao, L., Tarozo, R., and Huang, Y. (2012). Branched glycerol dialkyl glycerol tetraethers in Pleistocene loess-paleosol sequences: three case studies. Org. Geochem. 53, 38–44. doi: 10.1016/j.orggeochem.2012.09.005
Zhang, C., Zhao, C., Yu, S.-Y., Yang, X. D., Cheng, J., Zhang, X. J., et al. (2022). Seasonal imprint of Holocene temperature reconstruction on the Tibetan plateau. Earth Sci. Rev. 226:103927. doi: 10.1016/j.earscirev.2022.103927
Zhang, C. L., Ye, Q., Huang, Z. Y., Li, W. J., Chen, J. Q., Song, Z. Q., et al. (2008). Global occurrence of archaeal amoA genes in terrestrial hot springs. Appl. Environ. Microbiol. 74, 6417–6426. doi: 10.1128/AEM.00843-08
Zhang, C. L., Wang, J. X., Dodsworth, J. A., Williams, A. J., Zhu, C., Hinrichs, K. U., et al. (2013). In situ production of branched glycerol dialkyl glycerol tetraethers in a great basin hot spring (USA). Front. Microbiol. 4:181. doi: 10.3389/fmicb.2013.00181
Zhang, H. B., Griffiths, M. L., Chiang, J. C. H., Kong, W. W., Wu, S. T., Atwood, A., et al. (2018). East Asian hydroclimate modulated by the position of the westerlies during termination I. Science 362, 580–583. doi: 10.1126/science.aat9393
Zhang, H. W., Cheng, H., Sinha, A., Sptl, C., Cai, Y. J., Liu, B., et al. (2021). Collapse of the Liangzhu and other Neolithic cultures in the lower Yangtze region in response to climate change. Sci. Adv. 7:eabi9275. doi: 10.1126/sciadv.abi9275
Zhang, J. H., Huang, Y. Y., Wang, C. F., and Yang, H. (2020). Sources of GDGTs in stalagmites from Heshang cave, Hubei Province in Central China: new evidence from 5, 6-methyl brGDGTs and brGMGTs. Quat. Sci. 40, 992–1007.
Zhang, Y. G., Zhang, C. L., Liu, X.-L., Li, L., Hinrichs, K.-U., and Noakes, J. E. (2011). Methane index: a tetraether archaeal lipid biomarker indicator for detecting the instability of marine gas hydrates. Earth Planet. Sci. Lett. 307, 525–534. doi: 10.1016/j.epsl.2011.05.031
Zhao, R., Wang, H. M., Yang, H., and Yun, Y. (2017). Ammonia-oxidizing archaea dominate ammonia-oxidizing communities within alkaline cave sediments. Geomicrobiol J. 34, 511–523. doi: 10.1080/01490451.2016.1225861
Zhou, H. D., Hu, J. F., Ming, L. L., Peng, P. A., and Zhang, G. (2011). Branched glycerol dialkyl glycerol tetraethers and paleoenvironmental reconstruction in Zoigê peat sediments during the last 150 years. Chin. Sci. Bull. 56, 2456–2463. doi: 10.1007/s11434-011-4594-9
Zhu, C., Weijers, J. W. H., Wagner, T., Pan, J.-M., Chen, J.-F., and Pancost, R. D. (2011). Sources and distributions of tetraether lipids in surface sediments across a large river-dominated continental margin. Org. Geochem. 42, 376–386. doi: 10.1016/j.orggeochem.2011.02.002
Keywords: speleothems, GDGTs, Paleoenvironmental reconstruction, TEX86, MBT/CBT
Citation: Zang J, Yang H, Zhang J, Tang H and Tan L (2023) Application of microbial membrane tetraether lipids in speleothems. Front. Ecol. Evol. 11:1117599. doi: 10.3389/fevo.2023.1117599
Edited by:
Kunshan Bao, South China Normal University, ChinaReviewed by:
Zhiguo Rao, Hunan Normal University, ChinaYunping Xu, Shanghai Ocean University, China
Copyright © 2023 Zang, Yang, Zhang, Tang and Tan. This is an open-access article distributed under the terms of the Creative Commons Attribution License (CC BY). The use, distribution or reproduction in other forums is permitted, provided the original author(s) and the copyright owner(s) are credited and that the original publication in this journal is cited, in accordance with accepted academic practice. No use, distribution or reproduction is permitted which does not comply with these terms.
*Correspondence: Liangcheng Tan, dGFubGNoQGllZWNhcy5jbg==