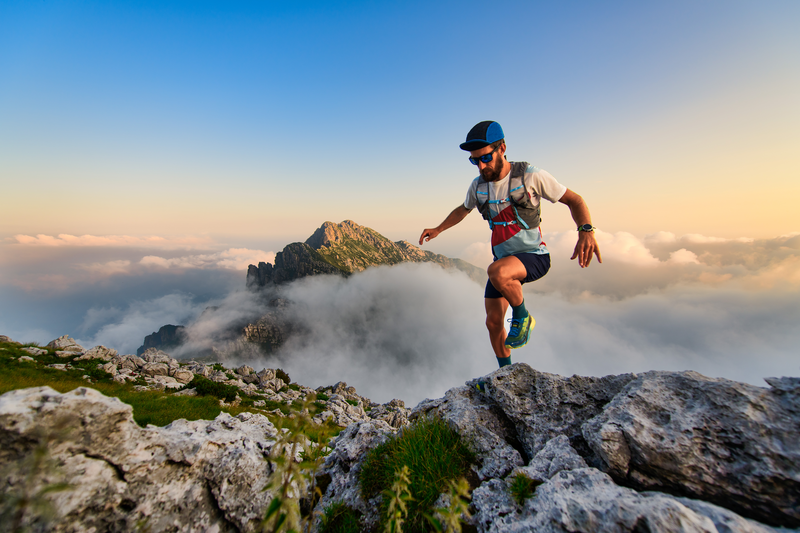
95% of researchers rate our articles as excellent or good
Learn more about the work of our research integrity team to safeguard the quality of each article we publish.
Find out more
ORIGINAL RESEARCH article
Front. Ecol. Evol. , 02 May 2023
Sec. Chemical Ecology
Volume 11 - 2023 | https://doi.org/10.3389/fevo.2023.1114636
This article is part of the Research Topic Women in Chemical Ecology 2022 View all 8 articles
Many herbivorous Lepidoptera accumulate plant toxins within their own tissues as a defensive strategy. Pioneering research in this area was conducted by Miriam Rothschild and Deane Bowers, who showed that the cycad-feeding butterfly Eumaeus atala sequester the toxic plant compound cycasin and thereby deter vertebrate and invertebrate predators. The current study focuses on another cycad compound, β-methylamino-L-alanine (BMAA), that is known to accumulate in the tissues of insects and other herbivores, and which has been shown to have neurotoxic effects in humans. Chemical analyses revealed that BMAA accumulates in both immature and adult tissues of E. atala, as well as adult tissues of another cycad-feeding lepidopteran, Seirarctia echo. However, the distribution of BMAA across life stages and tissues did not conform to patterns predicted for defensive sequestration, and subsequent behavioral experiments with ants showed that these invertebrate predators were not deterred by BMAA. Our results suggest that high levels of BMAA in the tissues of cycad-feeding insects likely reflect passive bioaccumulation rather than defensive sequestration. Combined with the previous work by Rothschild and Bowers, these results provide an example in which two different plant toxins accumulate within the tissues of a single herbivore species via different mechanisms and with different implications for ecology and evolution. They thereby lay the groundwork for further investigation into the processes underlying active sequestration and non-adaptive bioaccumulation.
The study of sequestration of plant defensive compounds by herbivorous insects is a cornerstone of chemical ecology. Sequestration is the ability to selectively accumulate plant compounds, often into specialized tissues, to gain a fitness advantage (Opitz and Müller, 2009; Beran and Petschenka, 2022), and defensive sequestration is a special case in which the fitness advantages of accumulating compounds are realized through protection from natural enemies. Historically, defensive sequestration among moths and butterflies (Lepidoptera) has provided particularly useful study systems for elucidating such important concepts as co-evolution, tri-trophic interactions, mimicry, and natural selection (Nishida, 2002). Knowledge of defensive sequestration among Lepidoptera has grown considerably in the past decades, though the field still suffers from a few widespread limitations. Many putative cases of defensive sequestration lack experimental evidence of deterrence among ecologically relevant predators, such that defensive sequestration often is not sufficiently distinguished from other adaptive functions or even non-adaptive bioaccumulation. Furthermore, there is significant taxonomic and chemical bias in defensive sequestration studies, with most studies focused on a handful of well-studied lepidopteran groups (e.g., danaids) and plant compound classes (e.g., cardenolides), leaving a huge portion of lepidopteran diversity and plant chemistry unexplored. Finally, sequestration is typically studied in terms of single compounds or compound classes, despite the fact that most plants produce multiple defensive compounds that could be co-sequestered. The present study addresses these limitations by investigating the accumulation and defensive potential of an unusual plant compound by a butterfly from a large family that is underrepresented in defensive sequestration studies.
Our study was motivated by the research of two pioneering chemical ecologists, Miriam Rothschild and Deane Bowers. These influential scientists investigated the chemical ecology of diverse lepidopteran groups (as well as other organisms), but in the late 1980's their scientific interests converged on a somewhat unusual butterfly, Eumaeus atala Poey 1968 (Lycaenidae). Eumaeus atala is a Caribbean species whose larvae feed solely on cycads, a group of highly toxic gymnosperms. The larval, pupal, and adult stages of E. atala all have bright warning coloration (Koi and Daniels, 2015), and even the eggs are conspicuous due to the bright butterfly scales deposited on them during oviposition (Rothschild, 1992). Warning coloration, in addition to the gregarious larvae and slow-flying adults, indicate that E. atala is aposematic, a somewhat rare characteristic among lycaenid butterflies.
Through a series of experiments performed in the late 1980's, Rothschild, Bowers, and their colleagues were able to elucidate the chemical basis of aposemetism in E. atala. First, Rothschild and colleagues showed that E. atala sequester cycasin, a glycosylated form of the cycad toxin, methylazoxymethanol (MAM), and that cycasin accumulates in the insect's tissues throughout its lifespan (Rothschild et al., 1986). Bowers and colleagues subsequently demonstrated that E. atala adults are distasteful to birds (Bowers and Farley, 1990) and that both the butterflies and pure cycasin are avoided by ants (Bowers and Larin, 1989), suggesting that cycasin sequestration confers protection against both vertebrate and invertebrate predators. Based on their combined work, Eumaeus butterflies are the only lycaenids that are included in Nishida's (2002) seminal review of sequestration by Lepidoptera, despite the Lycaenidae being one of the largest and most trophically diverse butterfly families.
Recent years have seen a renewed interest in the chemical ecology of cycad-insect interactions, including in E. atala and its congeners. All six Eumaeus species are obligate cycad specialists and genomic evidence suggests that toxin tolerance is likely a key innovation underlying the evolution of the genus (Robbins et al., 2021), possibly through an “escape and radiate” process (Ehrlich and Raven, 1964; Thompson, 1989) in which the insects diversified after evolving tolerance to cycad toxins facilitated their shift to these chemically distinctive host plants. However, the importance of individual cycad toxins in this process is unknown. In addition to cycasin and other MAM-glycosides, cycads produce sterol glycosides, the non-protein amino acid, β-N-methylamino-L-alanine (BMAA), and likely other toxic compounds (Bell, 2009; Liu et al., 2022). BMAA is a particularly interesting compound due to its toxicity to diverse organisms and its role in human neurodegenerative disease (Cox et al., 2003). Recent work suggests that BMAA may not be an effective anti-herbivore compound for cycads (Whitaker et al., 2022), but its potential as a defensive compound for cycad-feeding insects has never been explored. Eumaeus butterflies and other cycad herbivores encounter BMAA in their larval host plants, which is produced by endophytic cyanobacteria in specialized roots (Banack and Cox, 2003; Cox et al., 2003), but aside from evidence that BMAA is present in the larvae and pupal casings of the cycad-pollinating weevil, Rhopalotria mollis (Vovides et al., 1993), nothing is known about BMAA sequestration by insects.
It is therefore unclear whether cycad-feeding insects sequester BMAA, in addition to cycasin, for defense against natural enemies. Although many insects sequester multiple defensive compounds from their host plants, these compounds tend to be structurally similar and belong to the same broad compound classes (e.g., cardenolides, pyrolyzidine alkaloids). In contrast, BMAA and cycasin are chemically dissimilar and likely require distinct adaptations for sequestration. Although mixed or dual-sequestration defense strategies appear to be relatively uncommon, they have been reported among insects—for example, Platyphora leaf beetles that sequester both amyrins and pyrolyzidine alkaloids from their hosts (Termonia et al., 2002). The circumstances under which such dual defense strategies are adaptive are unclear, though Termonia et al. (2002) argue that dual chemical defense increases the chances of host transitions among chemically specialized insects. If E. atala and other cycad herbivores sequester multiple cycad compounds, the selective pressures giving rise to sequestration among cycad-feeding Lepidoptera may be more complex than previous research suggests.
Our study aimed to investigate the role of BMAA in interactions between cycads and their specialized lepidopteran herbivores by addressing the following questions: (1) Do cycad-feeding Lepidoptera sequester BMAA from cycads, and if so, in which life stages and body parts? (2) Is sequestered BMAA likely to confer protection from predators? and (3) How does BMAA sequestration compare to cycasin sequestration? If BMAA is sequestered as a defensive compound, we predict that (1) it will be distributed across the insects' body parts in a way that would maximize a predator's exposure to BMAA while minimizing fatal attacks and (2) both pure BMAA and butterfly extracts containing BMAA will be deterrent to ants, a common predator of lepidopterans. By testing these predictions, we build on Miriam Rothschild's and Deane Bowers' prior research on cycasin in Eumaeus atala to better understand the chemical ecology of cycad-herbivore interactions.
Eumaeus atala specimens were collected from the Montgomery Botanical Center in Coral Gables, Florida, a research and conservation garden cultivating more than 200 species of native and exotic cycads that support a robust E. atala population (Whitaker et al., 2021). Third-5th instar larvae were collected from accessioned Zamia integrifolia (Zamiaceae) and Z. aff. lucayana plants and starved for 24 h before storage at –80°C. Pupae were collected from accessioned Z. sp. Andros and Z. aff. lucayana plants; a subset of pupae were immediately frozen, and the remaining pupae were kept under controlled conditions (27°C, 60% RH, 16:8 L:D cycle) until eclosion, after which the freshly emerged adults were sexed and immediately frozen. Cycad leaflets from which larvae and pupae were collected were also frozen.
For comparison to E. atala, which is an obligate cycad specialist, we also analyzed specimens of Seirarctia echo, the only known lepidopteran herbivore that feeds facultatively on cycad leaves (Whitaker and Salzman, 2020). Final instar S. echo larvae were collected from Z. integrifolia cycads in Naples, Florida, and reared in captivity under controlled conditions (27°C, 60% RH, 16:8 L:D cycle) through pupation and eclosion. Adults were housed in mesh cages and allowed to mate and oviposit, and adults found dead in the cages were frozen. Eggs were collected daily and F1 larvae were reared on a combination of artificial diet (Frontier Insect Diets, product F9772) and store-bought lettuce (following Packard, 1890). F1 adults were sexed and preserved immediately after eclosion. All cycad and insect samples were stored at –80°C and lyophilized for 24 h prior to blinded analysis.
Six E. atala adult males and 6 adult females were dissected into wings, heads (including antennae), thoraxes (including legs), and abdomens. Due to the butterflies' small size, the dissected tissues did not meet minimum starting weights (10 mg) for the analysis and were therefore pooled by sex and tissue. Twelve S. echo adult males and 12 females (half of which were reared on cycads and half on lettuce/artificial diet) were also dissected into separate tissues as above, each of which were massive enough be analyzed separately with the exception of wings, which were pooled by sex and larval diet. Fifteen E. atala larvae and 5 pupae were left whole and were large enough to be processed individually. For both species, pooled wing samples were not massive enough to be weighed using the analytical balance available to us, so wing BMAA was quantified per individual (2 wings) rather than per gram.
Whole pupae, larvae, and adult abdomens were homogenized in 600 μL cold 20% w/v TCA in Eppendorf tubes with a sonicator probe on ice. Samples were then transferred to glass hydrolysis vials by washing each Eppendorf tube with an equal volume of 12M HCl. Leaf, head, thorax and wing samples did not require sonification; instead, heads and thoraxes were manually crushed with forceps, wings were left whole, and cycad leaves were homogenized in 50 mL Falcon tubes containing stainless steel beads in a Genogrinder. These pre-weighed tissues were then transferred directly to individual glass hydrolysis vials, to which 600-3000 μL (depending on sample volume, see Supplementary Table S1) of 6M HCl was added. All samples were heated to 110°C for 16 h, after which time 100 μL of the hydrolysate was transferred to a 0.22 μm centrifugal filter (Ultrafree-MC GV PVDF, Sigma-Aldrich #UFC30GV) and centrifuged at 14,000 x g for 3 min.
BMAA was quantified following validated methods (Banack, 2021) that allow for discrimination between BMAA and its isomers, N-(2 aminoethyl)glycine (AEG), 2,4-diaminobutyric acid (DAB), and β-amino-L-methylalanine. Briefly, filtered sample extracts were diluted 1:50 with ultrapure water and derivitized using the AccQ•Tag Ultra Derivitization Kit (Waters WAT052880) for amino acids according to the manufacturer's instructions, with the addition of an internal standard (D3|15N2-BMAA) to the kit's buffer solution. Derivitized samples were injected in triplicate on a Thermo TSQ Quantiva triple quadrupole mass spectrometer run in positive mode (settings available in Banack, 2021) with water blanks between samples, and the three measurements per sample were averaged for final values. BMAA concentrations (μg/g) were calculated based on tissue starting weights and the reagent volumes used in each step of the preparation workflow (Supplementary Table S1).
BMAA can occur in biological samples in different forms: freely circulating BMAA, BMAA that is associated with small peptides, and protein-bound BMAA. Free BMAA is immediately toxic to cells, while protein-bound BMAA can be detrimental to the organism by interfering with protein folding and function (Dunlop et al., 2013). To quantify these BMAA fractions separately, we processed 8 E. atala specimens (6 larvae and 2 pupae) using a modified version of the above protocol. Samples were sonicated in 600 μL cold 20% w/v TCA and allowed to sit for 2 h at room temperature. Extracts were then centrifuged at 14,000 x g for 3 min (0.22 μm) to pellet out the proteins. The supernatants were decanted into fresh Eppendorf tubes, and an additional 600 μL cold 20% w/v TCA was added to each protein pellet. The pellets were re-sonicated and allowed to sit for 1 h at room temperature, then re-centrifuged. The supernatants were again removed and pooled, for a total of 1200 μL per sample. 600 μL of each pooled supernatant was added to 600 μL chloroform in new Eppendorf tubes, then vortexed and allowed to separate. 100 μL of the TCA layer was centrifuge-filtered at 14,000 x g for 3 min, diluted 1:100 in ultrapure water, then derivitized and analyzed as above. 200 μL of the remaining supernatant was transferred to glass hydrolysis vials, to which 200 μL 12M HCl was added. Protein pellets were transferred to separate glass hydrolysis vials by rinsing the sample tubes twice with 200 μL 6M HCl, for a total volume of 400 μL. Samples were hydrolyzed at 110°C for 16 h, and 200 μL of the hydrolysate was filtered, diluted, derivitized, and analyzed as above. Based on initial derivitization efficiency, some samples needed to be diluted with ultrapure water then re-derivitized and re-analyzed to optimize quantification efficiency. Because the hydrolyzed supernatant contained both free and small peptide-associated BMAA, free BMAA values were subtracted from hydrolyzed supernatant values to avoid double counting free BMAA. Samples subjected to fraction-specific analysis are not included in measurements of “Total BMAA.”
Behavioral experiments followed the methods of Bowers and Larin (1989) with some modifications. We used 4 captive colonies of Lasius niger, which, like the Camponotus abdominalis used by Bowers & Larin, are generalist foragers that feed on scavenged insects, plant nectar, and insect honeydew. Colonies were individually housed in 32.5 x 17.6 x 15 cm plastic containers with plaster floors and Fluon®-coated walls, such that ants could forage freely without escaping. Glass test tubes wrapped in aluminum foil were provided as nesting spaces, and colonies were maintained on a diet of dried mealworms and 10% honey water. Colonies were starved for 48 h prior to testing.
Three solutions were tested simultaneously: a control solution of 25% sucrose, an experimental solution of 150 μg L-BMAA hydrochloride (Sigma-Aldrich) dissolved in 1 mL 25% sucrose solution, and an experimental solution containing a single dried, ground female E. atala adult (8.5 mg) suspended in 1 mL 25% sucrose solution, such that the BMAA- and butterfly-spiked solutions contained roughly equal concentrations of BMAA. A single droplet of each solution was placed on a glass microscope slide inside each colony box, equidistant to the nesting chambers. Ants were allowed to forage freely on the droplets for 1 h, over the course of which the number of ants feeding on each droplet was counted at 1-min intervals.
To account for correlations among time points, raw data were de-correlated by partitioning them into four 15-min time chunks (partitions), and averaging each partition into a single observation. We fit a linear mixed-effects model with the log-transformed means as the response variable, experimental solution as a fixed effect, and partition and colony as random effects. We confirmed that the model residuals were normally distributed, though partitioning the raw data in this way likely renders our model somewhat underpowered due to the reduction in observations. After finding that overall preference for the butterfly-spiked solution differed significantly from control and BMAA-spiked solutions, we performed post-hoc tests for pairwise differences between solutions by comparing estimated marginal means (using the emmeans package in R). We used a multivariate time series regression on the raw (un-partitioned) data to test whether ant preferences changed over the course of the experiment. R code for the statistical analyses is provided in the Supplementary material.
BMAA was detected in all plant and insect samples, with the exception of F1 S. echo adults that had been reared on lettuce and artificial diet, and the head of one S. echo adult that has been reared on cycads. BMAA concentrations in the leaves of three species of Zamia cycads ranged from 8.8-77 μg/g, within the range of previously published values from other cycad species (Figure 1). Total BMAA concentrations differed across E. atala life stages: larvae contained the highest concentration of BMAA (comparable to concentrations found in larval foodplants), followed by adults then pupae. This pattern is quite different from what Rothschild et al. (1986) reported for cycasin in E. atala (Figure 2). The majority of BMAA in E. atala larvae and pupae was present in its free form, with protein-bound BMAA making up the smallest fraction of total BMAA in these samples (Figure 3). In both E. atala and cycad-reared S. echo adults, BMAA was least concentrated in the insects' wings relative to their heads, thoraxes, and abdomens (Figure 4), and although BMAA was detected in the wings of both male and female E. atala butterflies, it was present in amounts below the lower limit of quantification [LOQ = 0.037 ng/mL; limit of detection (LOD) = 0.01 ng/mL].
Figure 1. Foliar BMAA concentrations across cycad species, based on data from the present study as well as three previously published studies. Both Duncan et al. (1990) and Charlton et al. (1992) detected BMAA in much higher amounts (>1,300 μg/g) in a species they call Cycas circinalis, but due to taxonomic uncertainty associated with this species epithet, those data are excluded here. Measurements from the present study fall within the range of previously reported values.
Figure 2. Concentrations of total BMAA (left) in Eumaeus atala, compared to cycasin concentrations (right) reported by Rothschild et al. (1986) (right), show different patterns of toxin accumulation across development. Note the different y-axis scales. All larvae and pupae samples were whole and unpooled, such that each point represents one individual. Adults subjected to BMAA analysis were dissected and pooled by sex and tissue, and BMAA quantities of all tissues were summed to calculate BMAA quantities in whole adults, such that each point represents 6 pooled individuals of the same sex. Adults subjected to cycasin analysis were dissected but not pooled, such that each point represents the summed tissues of one individual.
Figure 3. Separate BMAA fractions were extracted from whole, unpooled Eumaeus atala larvae and pupae in stages to compare quantities of free BMAA, small peptide-associated BMAA, and protein-bound BMAA within the insect tissues. Larvae contained considerably more free BMAA than protein-bound BMAA, and BMAA associated with small peptides was not detected in larvae (ND, not detected). All three forms of BMAA were found in low quantities in pupae.
Figure 4. Total BMAA quantified in the tissues of cycad-reared male and female Seirarctia echo (left) and Eumaeus atala (right) adults. Colored data points represent 6 pooled individuals, whereas boxplots include 6 males and 6 females (unpooled). Even after pooling, wing mass was too low to be measured so plotted values represent per-individual BMAA quantities. ND, not detected; NQ, below the lower limit of quantification.
Ants fed on all three experimental solutions (Figure 5) and exhibited no obvious aversive behavior toward droplets containing BMAA or butterfly extracts. However, the overall preference for the butterfly-spiked solution differed significantly from control and BMAA-spiked solutions (t = -2.321, p = 0.025), and post-hoc tests showed an overall aversion to the sucrose solution containing whole E. atala extracts: ant feeding was significantly greater on BMAA-spiked solution than butterfly-spiked solution (t = 3.71, p = 0.0018), slightly greater on control solution over butterfly-spiked solution (t = 2.32, p = 0.0645), and no different between control and BMAA-spiked solutions (t = -1.393, p = 0.3544). Over the course of the 60-min experiment, the number of ants feeding on the butterfly-spiked solution declined significantly (slope = -0.1041, t = -7.15, p < 0.001) whereas the number of ants feeding on control and BMAA-spiked diets did not significantly change (slope = -0.0159, t = -1.09, p = 0.279; and slope = 0.0282, t = 1.40, p = 0.166, respectively), indicating that ants' attraction to the butterfly-spiked solution declined as they gained experience feeding on the three solutions.
Figure 5. The number of ants (left) and the proportion of total ants (right) feeding on three experimental solutions for 60 min. At the beginning of the experiment, the numbers of ants feeding on each solution were roughy equal, whereas the number of ants feeding on the butterfly-spiked solution decreased significantly over the course of the trial period.
Our results show that BMAA accumulates in cycad-feeding insects' tissues, but we did not find evidence of dual defensive sequestration. We detected BMAA in all insects that were fed on cycads, though quantities varied across life stages and tissues. We did not detect BMAA in S. echo adults that had been reared on a larval diet without cycads, indicating that BMAA is sequestered from cycads and is not synthesized de novo by the insect, at least in this species.
Specific patterns of toxin localization—i.e., the life stages, tissues, and body parts in which insects store defensive compounds—influence the costs and benefits of sequestration, yet surprisingly few studies have compared the concentrations of sequestered plant toxins across different insect tissues. In one study, Mason and Deane Bowers (2017) investigated the distribution of sequestered iridoid glycosides in two Euphydryas butterfly species and framed their results in the context of three hypotheses regarding localization of sequestered compounds. According to their “differential risk hypothesis,” insects' defensive compounds should be most concentrated in tissues that can be easily and non-lethally sampled by birds and other pursuing predators, such as wings. In a classic example, Brower and Glazier (1975) found that monarch butterflies (Danaus plexippus) sequester very high concentrations of cardiac glycosides in their wings relative to other adult body parts, which the authors interpreted as evidence of an adaptive anti-predatory strategy. The results of the present study, however, do not match the predictions of this hypothesis: although we detected BMAA in all adult body parts of cycad-fed S. echo and E. atala, quantities were very low in the wings of both species—too low, even, to be quantified in the wings of E. atala (Figure 4). This may be due to the low starting mass of wings, but even so, birds or other predators that ingest the wings of these insects will therefore not immediately encounter appreciable quantities of BMAA and are unlikely to be deterred.
Alternatively, rather than storing compounds in the body parts that are most vulnerable to predator attack, Mason & Bowers' “differential costs hypothesis” posits that insects should concentrate toxins away from tissues and organ systems that could be damaged by exposure to sequestered compounds. Under this hypothesis, the costs of sequestration, rather than its benefits, drive the adaptive localization of toxins. Because many important organ systems are located in the insect abdomen, Mason and Deane Bowers (2017) predicted that iridoid glycosides should be least concentrated in the abdomens of Euphydryas butterflies relative to other body parts, for which the authors found mixed evidence. Of course, one might expect different localization patterns for compounds with differing modes of toxicity, and in the case of a neurotoxin like BMAA, nervous tissues might warrant the most protection. However, BMAA concentrations were moderate to high in the heads and thoraxes of S. echo and E. atala adults (though with considerable individual variation), suggesting that these insects do not sequester BMAA in a way that would prevent exposure to their cerebral or thoracic ganglia.
Finally, in contrast to the differential risk and differential costs hypotheses, both of which treat toxin localization as an adaptive process, the “tissue bias hypothesis” predicts that localization is driven by a sequestered compound's physiochemical properties that influence its affinity for different insect tissues. While some degree of tissue bias is likely always present, it will be most evident in the absence of significant adaptive drivers of toxin localization. Hay-Roe and Nation (2007) measured concentrations of cyanide released from adult tissues of Heliconius butterflies fed on various Passiflora hosts and found evidence that cyanogenic compounds were least concentrated in the butterflies' wings relative to other tissues, which they suggest is a result of low levels of β-glucosidase in the wings. In our case, the physiochemical properties of BMAA or of butterfly wings (or both) may similarly preclude storage of BMAA in these tissues.
In addition to evaluating BMAA localization in adult tissues, we also investigated BMAA sequestration in juvenile stages of E. atala and compared our findings to patterns of cycasin sequestration in different life stages. Cycasin has been found in all lifestages of E. atala (Rothschild et al., 1986), as well as S. echo larvae (Teas et al., 1966; Teas, 1967) and the adults of several other cycad-feeding Lepidoptera (Nash et al., 1992). In E. atala, cycasin accumulates over the course of development, with the lowest concentrations in larvae and the highest concentrations in adults (Rothschild et al., 1986). We observed a very different pattern for BMAA, which was most concentrated in larvae and least concentrated in pupae, with low to intermediate concentrations in adults. A large portion of sequestered BMAA appears to be lost, excreted, or chemically altered between the larval and pupal stages. Adult butterflies do not feed on cycad tissues, so it is somewhat peculiar that concentrations of both BMAA and cycasin increase from the pupal to adult stage.
The biochemical fates of sequestered toxins during insect metamorphosis are often unknown, and because BMAA can incorporate into proteins and interfere with protein folding and function (Dunlop et al., 2013), we were interested in the different fractions of BMAA present in larval and pupal stages. We quantified each fraction of BMAA—protein-bound, small peptide-associated, and freely circulating—in E. atala larvae and pupae, and found that the majority of BMAA in larvae occurs in its free form, with comparatively little BMAA incorporated into proteins and no detectable BMAA interacting with small peptides. Pupae sample sizes were too small to draw general conclusions, especially since quantities of all BMAA fractions in pupae were low, but relative differences between BMAA fractions were smaller in these samples. While we did not measure individual fractions in adult samples, these results suggest that E. atala may avoid some degree of toxicity by preventing the misincorporation of BMAA into proteins.
After establishing that two cycad-feeding insects accumulate BMAA in their tissues, we tested whether BMAA might confer a protective advantage for these insects. Lepidoptera have many natural enemies, including vertebrate and invertebrate predators, microbial pathogens, and parasitoids, and it is often not feasible to test the effects of a given compound on the preferences and performance of all potential enemies. Ants have been successfully used as a representative higher trophic level in butterfly palatability experiments, as the foraging preferences of ants are sensitive to prey palatability and nutritional value (Molleman et al., 2010). Bowers and Larin (1989) demonstrated that both cycasin and the extracts of whole E. atala adults were deterrent to ants, and concluded that the accumulation of cycasin by E. atala butterflies is therefore a case of defensive sequestration. We also found that ants visited butterfly-spiked food the least out of the 3 experimental solutions, and that their attraction to butterfly-spiked food declined over the course of the experiment (Figure 5). We suspect that this aversion is driven by the presence of cycasin (and potentially other defensive compounds) within the adult tissues. However, we observed no significant differences in preference for control vs. BMAA-spiked solutions, nor did ants' preferences for either solution change significantly over the trial period, suggesting that BMAA was not deterrent to ants under these conditions. Though BMAA is commonly considered a defensive compound for cycads, the only experimental test of this hypothesis found that concentrations of BMAA comparable to amounts naturally occurring in cycad leaves are insufficient to deter the generalist herbivore, Spodoptera littoralis (Whitaker et al., 2022). That BMAA is more concentrated in E. atala larvae than adults may indicate that the compound deters predators that specifically target the larval stages (e.g., parasitoids), though our data provide further evidence that BMAA may not be a feeding deterrent to invertebrates, in this case to a different trophic level.
Based on this combined evidence, the presence of BMAA in the tissues of cycad-feeding insects may be more accurately considered a case of bioaccumulation than defensive sequestration. While it remains possible that BMAA may protect insects from other natural enemies such as parasitoids or microbial pathogens, or that it confers some non-defensive (e.g. nutritional or reproductive) advantage, our results support a scenario of non-adaptive BMAA accumulation in cycadivorous insects, as has been observed in diverse animals from both terrestrial and marine environments. For example, there is evidence that flying foxes (Pteropus mariannus) biomagnify BMAA from the cycad seeds on which they feed (Banack and Cox, 2003). BMAA has been found in the brains of experimental primates whose diets contained BMAA (Cox et al., 2016a), as well as humans with certain neurodegenerative diseases (Murch et al., 2004; Pablo et al., 2009). Marine BMAA—likely of microbial origin—bioaccumulates in the muscles and fins of sharks (Hammerschlag et al., 2016), the brains of dolphins (Davis et al., 2019), and the brains and other tissues of fish and shellfish (Jonasson et al., 2010). Similarly, BMAA has been detected in the tissues of Drosophila melanogaster adults fed on BMAA-spiked diet (Zhou et al., 2009). In none of these cases is BMAA thought to confer a fitness advantage, and for many animals BMAA accumulation is associated with neurodegeneration and cognitive impairment.
Chronic dietary exposure to BMAA has been found to reduce lifespan, fertility, and motor function in Drosophila in a dose-dependent manner (Zhou et al., 2009, 2010; Nguyen et al., 2020; Cabrita et al., 2022). Chronic dietary exposure to BMAA has also been found to trigger neuropathology in non-human primates, including neurofibrillary tangles and sparse amyloid plaques in the brain, as well as TDP-43 +, FUS, and Ubiquitin proteinopathies in the spine (Cox et al., 2016a; Davis et al., 2020), whereas acute exposures do not produce such neuropathologies (Cox et al., 2016b). Since chronic exposure to BMAA is deleterious to vertebrates, further research is needed to determine if foraging on cycad-feeding insects reduces the fitness of birds and other insectivorous vertebrates.
It is possible that BMAA accumulation comes at a similar cost to cycad-feeding insects: even in established cases of adaptive sequestration, accumulating and storing defensive compounds is costly to specialized insects. But perhaps more interesting than the costs or benefits of BMAA accumulation is how cycad-feeding insects such as E. atala and S. echo tolerate their BMAA-rich diets. Our results show that these insects do not merely excrete BMAA, isolate it in non-vulnerable tissues, or detoxify it in the gut. Insects' ability to accumulate intact BMAA in their tissues while avoiding BMAA toxicity may therefore shed light on mechanisms of BMAA toxicity and resistance in other organisms. As we did not find evidence of a dual sequestration strategy among cycad-feeding Lepidoptera, we instead present a case in which defensive sequestration of one compound (cycasin) and likely bioaccumulation of another (BMAA) occur simultaneously within an individual. Given that most plants make multiple defensive compounds, there are likely many similar cases in which herbivorous insects accumulate multiple plant toxins, each with different adaptive (or maladaptive) consequences. Understanding the selective significance of accumulating multiple compounds should therefore be a focus of future studies of defensive sequestration among Lepidoptera and other insects.
The raw data supporting the conclusions of this article will be made available by the authors, without undue reservation.
MW and SB collected and analyzed the data. MW performed the statistical analyses and wrote the first draft of the manuscript with substantive input from all co-authors. All authors contributed to conception and design of the study and approved the final version of the manuscript.
The William Stamps Farish Fund provided funding to support this work. Open access funding was provided by ETH Zürich.
We thank William Tang for collecting the Seirarctia echo specimens used in this analysis; Diego Salazar for providing access to a lyophilizer; the ETH Seminar for Statistics for assistance with analyzing ant preference data; the Montgomery Botanical Center for their continued support; and the entomologists, chemists, evolutionary biologists, and ecologists of all genders whose work advances gender equality in the field of chemical ecology. This manuscript was much improved by the thoughtful suggestions of two reviewers.
The authors declare that the research was conducted in the absence of any commercial or financial relationships that could be construed as a potential conflict of interest.
All claims expressed in this article are solely those of the authors and do not necessarily represent those of their affiliated organizations, or those of the publisher, the editors and the reviewers. Any product that may be evaluated in this article, or claim that may be made by its manufacturer, is not guaranteed or endorsed by the publisher.
The Supplementary Material for this article can be found online at: https://www.frontiersin.org/articles/10.3389/fevo.2023.1114636/full#supplementary-material
Banack, S. A. (2021). Second laboratory validation of β-N-methylamino-L-alanine, N-(2aminoethyl) glycine, and 2,4-diaminobuytric acid by ultra-performance liquid chromatography and tandem mass spectrometry. Neurotox Res. 39, 107–116. doi: 10.1007/s12640-020-00208-x
Banack, S. A., and Cox, P. A. (2003). Biomagnification of cycad neurotoxins in flying foxes: implications for ALS-PDC in Guam. Neurology 61, 387–389. doi: 10.1212/01.WNL.0000078320.18564.9F
Bell, E. A. (2009). The discovery of bmaa, and examples of biomagnification and protein incorporation involving other non-protein amino acids. Amyotrophic Lateral Sclerosis 10(Supl. 2), 21–25. doi: 10.3109/17482960903268700
Beran, F., and Petschenka, G. (2022). Sequestration of plant defense compounds by insects: From mechanisms to insect-plant coevolution. Annu. Rev. Entomol. 67, 163–180. doi: 10.1146/annurev-ento-062821-062319
Bowers, M. D., and Farley, S. (1990). The behaviour of grey jays, Perisoreus canadensis, towards palatable and unpalatable Lepidoptera. Anim. Behav. 39, 699–705. doi: 10.1016/S0003-3472(05)80381-1
Bowers, M. D., and Larin, Z. (1989). Acquired chemical defense in the lycaenid butterfly, Eumaeus atala. J. Chem. Ecol. 15, 1133–1146. doi: 10.1007/BF01014817
Brower, L. P., and Glazier, S. C. (1975). Localization of heart poisons in the monarch butterfly: Sequestered cardenolides are concentrated to different degrees in various body parts as an antipredator strategy. Science 188, 19–25. doi: 10.1126/science.188.4183.19
Cabrita, A., Medeiros, A. M., Pereira, T., Rodrigues, A. S., Kranendonk, M., and Mendes, C. S. (2022). Motor dysfunction in Drosophila melanogaster as a biomarker for developmental neurotoxicity. iScience 2022, 104541. doi: 10.1016/j.isci.2022.104541
Charlton, T. S., Marini, A. M., Markey, S. P., Norstog, K., and Duncan, M. W. (1992). Quantification of the neurotoxin 2-amino-3-(methylamino)-propanoic acid (BMAA) in Cycadales. Phytochemistry 31, 3429–3432. doi: 10.1016/0031-9422(92)83700-9
Cox, P. A., Banack, S. A., and Murch, S. J. (2003). Biomagnification of cyanobacterial neurotoxins and neurodegenerative disease among the Chamorro people of Guam. Proc. Natl. Acad. Sci. U.S.A. 100, 13380–13383. doi: 10.1073/pnas.2235808100
Cox, P. A., Davis, D. A., Mash, D. C., Metcalf, J. S., and Banack, S. A. (2016a). Dietary exposure to an environmental toxin triggers neurofibrillary tangles and amyloid deposits in the brain. Proc. R Soc. B Biol. Sci. 283, 20152397. doi: 10.1098/rspb.2015.2397
Cox, P. A., Davis, D. A., Mash, D. C., Metcalf, J. S., and Banack, S. A. (2016b). Do vervets and macaques respond differently to BMAA? Neurotoxicology 57, 310–311. doi: 10.1016/j.neuro.2016.04.017
Davis, D. A., Cox, P. A., Banack, S. A., Lecusay, P. D., Garamszegi, S. P., Hagan, M. J., et al. (2020). L-serine reduces spinal cord pathology in a vervet model of preclinical ALS/MND. J. Neuropathol. Exp. Neurol. 79, 393–406. doi: 10.1093/jnen/nlaa002
Davis, D. A., Mondo, K., Stern, E., Annor, A. K., Murch, S. J., Coyne, T. M., et al. (2019). Cyanobacterial neurotoxin BMAA and brain pathology in stranded dolphins. PLoS ONE 14, e0213346. doi: 10.1371/journal.pone.0213346
Duncan, M. W., Steele, J. C., Kopin, I. J., and Markey, S. P. (1990). 2-amino-3-(methylamino)-propanoic acid (BMAA) in cycad flour: An unlikely cause of amyotrophic lateral sclerosis and parkinsonism-dementia of guam. Neurology 40, 767–767. doi: 10.1212/WNL.40.5.767
Dunlop, R. A., Cox, P. A., Banack, S. A., and Rodgers, K. J. (2013). The non-protein amino acid bmaa is misincorporated into human proteins in place of l-serine causing protein misfolding and aggregation. PLoS ONE 8, e75376. doi: 10.1371/journal.pone.0075376
Ehrlich, P. R., and Raven, P. (1964). Butterflies and plants: a study in coevolution. Evolution 18, 586–608. doi: 10.2307/2406212
Hammerschlag, N., Davis, D. A., Mondo, K., Seely, M. S., Murch, S. J., Glover, W. B., et al. (2016). Cyanobacterial neurotoxin BMAA and mercury in sharks. Toxins 8, 238. doi: 10.3390/toxins8080238
Hay-Roe, M. M., and Nation, J. (2007). Spectrum of cyanide toxicity and allocation in Heliconius erato and Passiflora host plants. J. Chem. Ecol. 33, 319–329. doi: 10.1007/s10886-006-9234-5
Jonasson, S., Eriksson, J., Berntzon, L., Spáčil, Z., Ilag, L. L., Ronnevi, L.-O., et al. (2010). Transfer of a cyanobacterial neurotoxin within a temperate aquatic ecosystem suggests pathways for human exposure. Proc. Natl. Acad. Sci. U.S.A. 107, 9252–9257. doi: 10.1073/pnas.0914417107
Koi, S., and Daniels, J. (2015). New and revised life history of the Florida hairstreak Eumaeus atala (Lepidoptera: Lycaenidae) with notes on its current conservation status. Florida Entomol. 98, 1134–1148. doi: 10.1653/024.098.0418
Liu, Y., Wang, S., Li, L., Yang, T., Dong, S., Wei, T., et al. (2022). The Cycas genome and the early evolution of seed plants. Nat. Plants 8, 389–401. doi: 10.1038/s41477-022-01129-7
Mason, P. A., and Deane Bowers, M. (2017). Localization of defensive chemicals in two congeneric butterflies (Euphydryas, Nymphalidae). J. Chem. Ecol. 43, 480–486. doi: 10.1007/s10886-017-0841-0
Molleman, F., Whitaker, M. R. L., and Carey, J. R. (2010). Rating palatability of butterflies by measuring ant feeding behaviour. Entomol. Bericht 70, 52–62.
Murch, S., Cox, P., Banack, S., Steele, J., and Sacks, O. (2004). Occurrence of β-methylamino-L-alanine (BMAA) in ALS/PDC patients from Guam. Acta Neurol Scand. 110, 267–269. doi: 10.1111/j.1600-0404.2004.00320.x
Nash, R. J., Bell, E. A., and Ackery, P. R. (1992). The protective role of cycasin in cycad-feeding Lepidoptera. Phytochemistry 31, 1955–1957. doi: 10.1016/0031-9422(92)80340-K
Nguyen, Q. T., Rivera, L. V., and Goto, J. J. (2020). A locomotor activity study of the effects of L-serine to neurodegeneration impacts of β-Methylamino-L-alanine in Drosophila melanogaster. FASEB J. 34, 1. doi: 10.1096/fasebj.2020.34.s1.00641
Nishida, R. (2002). Sequestration of defensive substances from plants by Lepidoptera. Annu. Rev. Entomol. 47, 57–92. doi: 10.1146/annurev.ento.47.091201.145121
Opitz, S. E., and Müller, C. (2009). Plant chemistry and insect sequestration. Chemoecology 19, 117–154. doi: 10.1007/s00049-009-0018-6
Pablo, J., Banack, S., Cox, P., Johnson, T., Papapetropoulos, S., Bradley, W., et al. (2009). Cyanobacterial neurotoxin BMAA in ALS and Alzheimer's disease. Acta Neurol Scand. 120, 216–225. doi: 10.1111/j.1600-0404.2008.01150.x
Packard, A. S. (1890). The life-history of Seirarctia echo. Psyche J. Entomol. 5, 351–353. doi: 10.1155/1890/17952
Robbins, R. K., Cong, Q., Zhang, J., Shen, J., Riera, J. Q., Murray, D., et al. (2021). A switch to feeding on cycads generates parallel accelerated evolution of toxin tolerance in two clades of Eumaeus caterpillars (Lepidoptera: Lycaenidae). Proc. Natl. Acad. Sci. U.S.A. 118, 5118. doi: 10.1073/pnas.2018965118
Rothschild, M. (1992). Egg protection by the Atala hairstreak butterfly (Eumaeus atala florida. Phytochemistry 31, 1959–1960. doi: 10.1016/0031-9422(92)80341-B
Rothschild, M., Nash, R. J., and Bell, E. A. (1986). Cycasin in the endangered butterfly Eumaeus atala florida. Phytochemistry 25, 1853–1854. doi: 10.1016/S0031-9422(00)81161-9
Teas, H. J. (1967). Cycasin synthesis in Seirarctia echo (Lepidoptera) larvae fed methylazoxymethanol. Biochem. Biophys. Res. Commun. 26, 686–690. doi: 10.1016/S0006-291X(67)80127-X
Teas, H. J., Dyson, J. G., and Whisenant, B. R. (1966). Cycasin metabolism in Seirarctia echo Abbot and Smith (Lepidoptera: Arctiidae). J. Georgia Entomol. Soc. 1, 21–22.
Termonia, A., Pasteels, J. M., Windsor, D. M., and Milinkovitch, M. C. (2002). Dual chemical sequestration: a key mechanism in transitions among ecological specialization. Proc. R. Soc. Londo. B Biol. Sci. 269, 1–6. doi: 10.1098/rspb.2001.1859
Thompson, J. N. (1989). Concepts of coevolution. Trends Ecol. Evolut. 4, 179–183. doi: 10.1016/0169-5347(89)90125-0
Vovides, A. P., Norstog, K. J., Fawcett, P. K. S., Duncan, M. W., Nash, R. J., and Molsen, D. V. (1993). Histological changes during maturation in male and female cones of the cycad Zamia furfuracea and their significance in relation to pollination biology. Bot. J. Linnean Soc. 111, 241–252. doi: 10.1111/j.1095-8339.1993.tb01901.x
Whitaker, M. R., Gilliéron, F., Skirgaila, C., Mescher, M. C., and De Moraes, C. M. (2022). Experimental evidence challenges the presumed defensive function of a “low toxin” in cycads. Sci. Rep. 12, 1–6. doi: 10.1038/s41598-022-09298-3
Whitaker, M. R., and Salzman, S. (2020). Ecology and evolution of cycad-feeding Lepidoptera. Ecol. Lett. 23, 1862–1877. doi: 10.1111/ele.13581
Whitaker, M. R. L., Salzman, S., Gratacos, X., and Tucker Lima, J. (2021). Localized overabundance of an otherwise rare butterfly threatens endangered cycads. Florida Entomol. 103, 519–522. doi: 10.1653/024.103.00416
Zhou, X., Escala, W., Papapetropoulos, S., Bradley, W. G., and Zhai, R. G. (2009). BMAA neurotoxicity in Drosophila. Amyotrophic Lateral Sclerosis 10, 61–66. doi: 10.3109/17482960903273445
Keywords: Miriam Rothschild, Deane Bowers, β-methylamino-L-alanine (BMAA), chemical defense, non-protein amino acid, Eumaeus atala, Seirarctia echo, aposemetism
Citation: Whitaker MRL, Banack SA, Mescher MC, Cox PA and De Moraes CM (2023) BMAA in cycad-feeding Lepidoptera: defensive sequestration or bioaccumulation? Front. Ecol. Evol. 11:1114636. doi: 10.3389/fevo.2023.1114636
Received: 02 December 2022; Accepted: 03 April 2023;
Published: 02 May 2023.
Edited by:
Anne Marie Cortesero, University of Rennes 1, FranceReviewed by:
Adriana Moriguchi Jeckel, University of Bern, SwitzerlandCopyright © 2023 Whitaker, Banack, Mescher, Cox and De Moraes. This is an open-access article distributed under the terms of the Creative Commons Attribution License (CC BY). The use, distribution or reproduction in other forums is permitted, provided the original author(s) and the copyright owner(s) are credited and that the original publication in this journal is cited, in accordance with accepted academic practice. No use, distribution or reproduction is permitted which does not comply with these terms.
*Correspondence: Consuelo M. De Moraes, Y29uc3VlbG8uZGVtb3JhZXNAdXN5cy5ldGh6LmNo
Disclaimer: All claims expressed in this article are solely those of the authors and do not necessarily represent those of their affiliated organizations, or those of the publisher, the editors and the reviewers. Any product that may be evaluated in this article or claim that may be made by its manufacturer is not guaranteed or endorsed by the publisher.
Research integrity at Frontiers
Learn more about the work of our research integrity team to safeguard the quality of each article we publish.