- 1Centro Austral de Investigaciones Científicas—Consejo Nacional de Investigaciones Científicas y Técnicas (CADIC—CONICET), Ushuaia, Argentina
- 2Forest Ecology Lab, Northern Arizona University, Flagstaff, AZ, United States
Introduction: Large wildfires were historically absent in the forests of Tierra del Fuego, southern Argentina. This has changed in recent decades as humans have increased ignition sources, and warmer, drier periods have fueled large, severe wildfires. As a result of its location at an extreme southern latitude, Tierra del Fuego has very low tree species diversity. One of the region's dominant tree species, Nothofagus antarctica, is believed to have traits that may make it resilient to wildfire (e.g., the ability to resprout prolifically).
Methods: This study examined post-fire N. antarctica regeneration across an environmental and time-since-fire gradient. Plots were established in burned areas (n = 160) and unburned controls (n = 32) in and around two wildfires that occurred circa 1940 and in 2019. Seedling/resprout and sapling regeneration densities, as well as site characteristics (e.g., slope) and fire-impacted variables (e.g., distance to mature live trees), were measured.
Results: Seedling and resprout densities were lower in burned plots than in controls, with this trend being exacerbated in the 2019 fire with increasing distance from mature live trees. Regeneration generally occurs in clumps and principally through sprouting from live and top-killed trees, with not all top-killed trees having resprouted. Seedling and resprout densities were most strongly impacted by time since fire, distance to mature live trees and post-fire canopy cover. Sapling densities were modulated by slope, time since fire, and distance to mature live trees.
Discussion: Despite lower regeneration densities in recently burned plots and less live basal area and canopy cover in older burned plots compared to unburned controls, burned stands may be on a trajectory to recover pre-fire characteristics, although this recovery is spatially variable. However, full recovery has not occurred 80 years after the 1940's fire. Currently, these burned areas resemble grasslands or savannas. They do not provide the habitat or ecosystem services that denser forests do and may require active restoration to fully recover their pre-fire characteristics.
1. Introduction
The frequency, severity, and size of forest fires in regions that historically experienced little to no wildfires have increased in recent decades (e.g., Parks et al., 2018; Busby et al., 2020; Tyukavina et al., 2022). While these changes seriously threaten the survival of some tree species, others are adapted and resilient to change (e.g., McCord et al., 2019; Rossman et al., 2020). Globally, many of the site characteristics and fire-impacted variables that determine which trees regenerate post-fire and at what densities are the same (e.g., aspect, distance to seed source, and fire severity).
Site characteristic variables are those that are largely unaffected by fire and include elevation, aspect, slope, herbivore use, and time since fire, among others (Johnstone et al., 2020). Fire-impacted variables, conversely, are those that are directly altered by fire and include post-fire basal area, distance to seed source, and remnant canopy cover (Dawe et al., 2020). Variables from both categories often influence post-fire forest recovery (Johnstone et al., 2020). Time since fire, for example, is a site characteristic often strongly linked to regeneration. For many sprouting species, for example, resprouting is triggered by the death of their above ground biomass and tends to be strongest in the years immediately following a fire (e.g., Lee et al., 2019). Distance to seed source and canopy cover tend to be universally important factors that impact post-fire tree regeneration as well (e.g., Clark et al., 1999; Busby et al., 2020; Peeler and Smithwick, 2020). For resprouting species, recruits tend to cluster closely around top-killed individuals post-fire (e.g., Hammett et al., 2017). Meanwhile, greater canopy cover can have either a positive (e.g., Boag et al., 2020) or negative (e.g., Kemp et al., 2019) impact on regeneration depending on the silvics of individual species and the moisture availability in a given environment. Reduced canopy cover tends to increase xeric conditions and favor species that are adapted to post-disturbance and early-seral environments (Kemp et al., 2019; Johnstone et al., 2020). Influencing all fire-impacted variables is fire severity, which describes the damage produced by a fire, and is informed by plant mortality and forest floor changes (Keeley, 2009). High-severity fires result in post-fire conditions where few live trees remain and ground cover is dominated by bare-mineral soil. These conditions can strongly deter the regeneration of certain tree species that are ill-adapted to burned landscapes (e.g., Holz et al., 2015).
The silvics of species present pre-fire are generally a significant driver of post-fire forest dynamics as well: wildfire promotes species with adaptations such as resprouting or serotiny (e.g., McKenzie and Tinker, 2012; Lamont et al., 2020). Prolific resprouting post-fire is frequently associated with hardwoods like many poplar (Populus) and oak (Quercus) species, and several conifer species, notably species in the genus Juniperus (Donato et al., 2009; Errington and Pinno, 2021). Resprouting is an ancient adaptation; it develops not only in response to fire but more generally in response to the loss of above-ground biomass (Keeley et al., 2011). In general, resprouting species have a higher chance of surviving or returning after fire than species that do not resprout (Lloret et al., 1999) and they tend to dominate a diverse array of post-fire landscapes globally (e.g., Steinke et al., 2008; Catry et al., 2013; Torres et al., 2014).
In southern Patagonia, thunderstorms, and thus lightning strikes, are extremely rare, and wildfire is not considered to have been a historical driver of ecological communities (Heusser, 1994). The historic fire regime in the forests on the island of Tierra del Fuego at the tip of South America has not been studied or described in detail. Still, the island's native peoples used fire continuously before and after their first sustained contact with Europeans in the mid to late 1800's. Their survival in the cold, wet climate of Tierra del Fuego depended heavily on constantly maintaining fires (Lothrop, 1928; Bridges, 1951). Though there are no written accounts of fire being used intentionally to promote specific plants or to clear areas for hunting, as was the case elsewhere in the Americas (e.g., Vale, 2002), it is known that the native Selk'nam people would abandon campfires and that these fires may have occasionally escaped and burned more extensive areas (Bridges, 1951).
As the human population of Tierra del Fuego and production activities such as logging, peat mining, and cattle ranching have increased on the island over the last century, wildfire ignition sources have also increased. The forests in the center and north of the island, where wildfire is most frequent, are dominated by Nothofagus antarctica, which generally forms monospecific stands. Though N. antarctica can produce mass quantities of lightweight seed, it is considered to be the species of Nothofagus that most heavily depends on resprouting to reproduce (Premoli and Steinke, 2008); it can resprout from its root system, branches, or from its base (root collar or crown) in relatively undisturbed forests, or in response to the loss of above ground biomass. The species has shown resilience to repeated and accumulated disturbances in Tierra del Fuego, as the ability of N. antarctica to resprout and persevere after silvicultural management and grazing has been previously documented in the region (Bahamonde et al., 2013, 2018; Soler et al., 2013, 2018). Although the selective pressure that resulted in its ability to resprout has not been confirmed with absolute certainty, it likely developed in response to grazing from the native herbivore Lama guanicoe, or in response to wildfires further north in N. antarctica's range (Veblen et al., 1999). As N. antarctica is commonly found in the ecotone between forest and grassland, it has been subject historically to more consistent and intense grazing than other Nothofagus species from herbivores that move between grasslands and adjacent forests (Veblen and Lorenz, 1988; Iranzo et al., 2022). Despite adapting to this selective pressure, constant trampling and browsing by livestock (cattle, sheep, and horses) and native herbivores over many years can still deform or impede N. antarctica regeneration (Raffaele et al., 2011; Echevarría et al., 2014).
The response of Nothofagus species in Tierra del Fuego to grazing has been well-documented but their responses to fire have not been. N. pumilio in Tierra del Fuego has been shown to be highly sensitive to wildfire and struggles to successfully recolonize burned areas (Ruggirello et al., 2023b). Post-fire regeneration of N. antarctica after a 2008 wildfire was also not as abundant as expected (Ruggirello et al., 2023a). Further study is still needed to more fully describe the post-fire regeneration trends of Nothofagus species in Tierra del Fuego. This work focuses specifically on N. antarctica. Given that N. antarctica reproduces through vigorous asexual sprouting, we hypothesized that it would successfully recover after a forest fire and we sought to answer the following questions relating to its post-fire regeneration dynamics:
• Which site characteristics (elevation, aspect, slope, herbivore use, and time since fire) most strongly impact regeneration density and spatial distribution?
• Which fire-impacted variables (distance to mature live trees, total basal area, canopy cover, and seed abundance) most strongly impact regeneration density and spatial distribution?
• Does N. antarctica's ability to resprout from top-killed individuals drive its establishment in burned areas?
• Are N. antarctica stands that burned 3 years ago and over 80 years ago on trajectories to recover pre-fire characteristics?
2. Materials and methods
2.1. Study area
This study was conducted in central Tierra del Fuego, near the town of Tolhuin (54°51′09′′ S, 67°19′30′′ W), in southern Argentina. Temperatures, precipitation, and winds are strongly influenced by the Andes mountains to the south, which produce drier conditions, stronger winds, and more extreme temperatures in the central and northern parts of the island. The average precipitation in the center of the island is 400–500 mm yr−1 and the average annual temperature is between 5 and 6°C (Goya et al., 2005). In general, the largest and most severe fires in Tierra del Fuego occur in this part of the island, as there is substantial commercial (e.g., logging, peat mining, and cattle ranching) and outdoor recreation activity in the area. Vegetation responds to the strong climatic gradient produced by the Fuegian Andes. To the south of the mountains, forests are dominated by pure N. pumilio and mixed N. pumilio-N. betuloides stands, but the study area in the center of the island is a mosaic of alternating pure N. pumilio and N. antarctica stands, grasslands, and peatlands.
This study was carried out in N. antarctica forests that burned circa 1940 in the Pirinaica Ranch Fire (hereafter called “old fire”) and in 2019 in the Lenga Patagonia Sawmill Fire (hereafter called “recent fire;” Figure 1, Table 1). The old fire was intentionally set by early homesteaders to destroy mature forests to clear land for sheep grazing, which it largely succeeded in doing. The site has since been regularly grazed by sheep and cattle. The recent fire was started in November by a forestry company that was burning residue piles behind their sawmill when strong winds carried the fire into the adjacent forest. The site also burned in the 1950's. There is light grazing at the site by the native ungulate the guanaco (L. guanicoe) and wild horses. In terms of climate and site quality, the two burned areas are not entirely analogous. The old fire occurred ~5 km from the Atlantic Ocean where temperatures are moderated by the sea and precipitation tends to be higher, whereas the recent fire occurred in the center of the island where temperatures are more extreme and precipitation is lower (Allué et al., 2010).

Table 1. Fire details and mean elevation, slope, and predominant aspect by fire with minimum and maximum values in parenthesis.
Due to the age of one of the two fires and the lack of region-specific burn-class ranges for Normalized Burn Ratio (NBR) calculations, specific estimates of burn severity in these fires were not included in this work. However, given the general absence of trees that survived fire, both wildfires are considered to have burned as stand-replacing, high-severity fires, resulting in deep ground layer consumption and high tree mortality. These results are supported by field estimates of fire severity in the recent fire (Ruggirello et al., 2023c).
2.2. Species description
In mature, undegraded N. antarctica forests, seedlings and resprouts are present at densities between 100,000 and 200,000 individuals ha−1 (Peri and Collado, 2009). N. antarctica has intermediate shade tolerance and is generally less tolerant of shade than other Nothofagus species in the region. However, it regenerates at high densities under semi-closed canopies, where it can persist for several years until canopy openings form or widen (Peri et al., 2016). Under the natural disturbance regime of blowdowns and stand decadence, N. antarctica forests are often semi-open, rather than completely closed, and provide frequent opportunities for regeneration to take advantage of constantly forming gaps (Peri et al., 2016). Given the lack of even moderate shade provided 3 years post-fire, it can be expected that individuals will likely not regenerate at similar densities as in undegraded forests.
2.3. Study design and regeneration measurements
The methods used in this study were largely the same as those used in Ruggirello et al. (2023b). Two research sites were established in each fire for a total of four sites. Each site consisted of four, 300 m long transects (n = 16). Transects were located starting at a random point along the unburned forest edge, and set toward the interior of the burned area, with a minimum distance of 50 m between transects. This distance often ended up being well over 150 m to capture the intra-fire variability at each site. Each transect contained five plot locations. Plot locations were established at 60 m intervals starting 30 m from the unburned forest edge (Coop et al., 2010; Figure 2). For each transect, a control plot location was established at random at least 50 m into the adjacent, unburned forest. Each plot location contained two rectangular plots: one 10 m by 2 m (20 m2) running north-south in which saplings were tallied, and another 5 m by 20 cm (1 m2) running west-east starting from the 5 m mark of the sapling plot (hereafter referred to as plot center) in which seedlings and resprouts were counted (Figure 2). These methods have been shown to be adequate for surveying regeneration in unburned Nothofagus forests in Tierra del Fuego (Soler et al., 2013). However, given the scarcity of regeneration in parts of the burned areas, if a seedling/resprout or sapling was not present in its respective plot, that plot was expanded until finding at least one individual. The new plot size (either a rectangle or square) was then calculated, resulting in regeneration plots of differing areas. Regeneration was later standardized for each plot to seedlings/resprouts and saplings per hectare. Given the variable distance between opposite, unburned forest edges and the presence of tree refugia within the fire perimeters, average distance to live mature trees was used for analyses rather than the expected distance of a plot to the unburned forest edge.
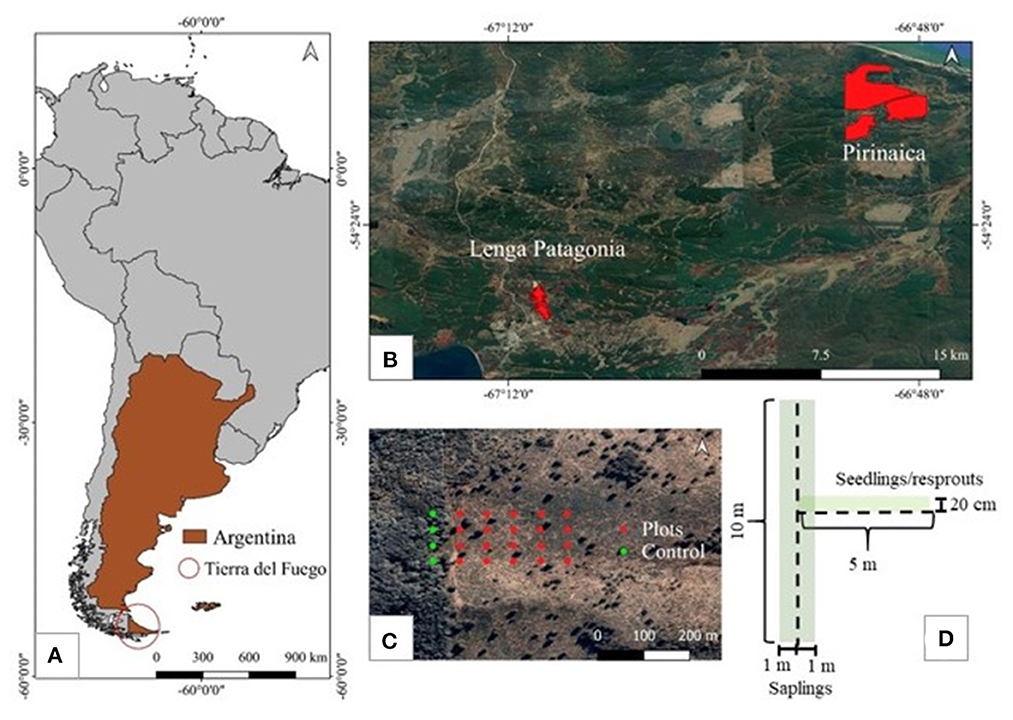
Figure 2. Maps of the general study area (A) and fire boundaries (B), as well as diagrams of the transect design (C) and plot layout (D).
Seedlings and resprouts were defined as individuals with a height ≤30 cm and saplings as having a height >30 cm, but ≤130 cm (Soler et al., 2013, 2018). As one research site contains four transects, and a transect contains five seedling/resprout and five sapling plots and one seedling/resprout control plot and one sapling control plot, this resulted in a total of 192 plots, 160 burned and 32 unburned, across the four sites.
Per transect, ~12 seedling-sized individuals were chosen at random from within established seedling/resprout regeneration plots when possible, or the nearest seedling-sized individuals to a plot not containing regeneration were selected, and their regeneration source (sexual or asexual) was determined. For this, the relationship between an individual's age and height and its age and DAC (diameter at root collar) was examined, as well as its proximity to nearby individuals from which it could have sprouted (Soler et al., 2018). Age is estimated in N. antarctica by counting nodes along a plant's stem; the amount of stem between nodes corresponds to 1 year's growth. If an individual exhibited exceptional growth relative to its age (>2.5 cm year−1 according to Soler et al., 2018), broadened significantly at its root collar, and was within 2 m of a live or dead N. antarctica tree, it was designated to be of asexual origin. To confirm our designations, the root systems of individuals whose origins were not obviously apparent upon inspection were excavated until finding a union with the tree from which they sprouted, or until finding the end of their root system, indicating germination from seed.
2.4. Collecting site characteristics and fire-impacted variables
Field sampling was conducted in the southern hemisphere summer of 2021–2022 (December-February). At plot center, elevation, aspect, and slope were recorded using a GPS, compass, and laser rangefinder (TruPulse/Laser Technology, Centennial, CO, USA; Busby et al., 2020). One hemispheric photograph was also taken from plot center with a camera with a fisheye lens and canopy cover was later extracted from each photograph using the software program Gap Light Analyzer (Frazer et al., 1999). Horizontal distance from plot center to the three nearest live, mature trees was also recorded using a laser rangefinder (TruPulse/Laser Technology, Centennial Colorado, USA; Busby et al., 2020). At each plot location, forest structure was measured from plot center using the Bitterlich method of angular counting (Pulido et al., 2000), resulting in variable-radius plots whose sizes depended on the diameters of trees at breast height (DBH) and the basal area factor used. For trees with diameters at breast height ≥5 cm and a height >130 cm included within variable-area plots, DBH, total height, and tree condition (live/dead) were recorded. From these measurements, dead basal area, live basal area, and total basal area (dead + live basal area) could be calculated. To estimate herbivore activity, in each 20 m2 plot, all herbivore feces present from cattle, horses, and guanaco were tallied. Seedfall was estimated in the autumn of 2022 (April), by counting all N. antarctica seeds present on the forest floor in five, 20 cm2 subplots per plot (Chianucci et al., 2021; Tattoni et al., 2021) in all control, 30 m, and 270 m plots (n = 48).
2.5. Quantifying forest recovery
Specific metrics for analyzing forest recovery in degraded (by logging and cattle grazing) N. antarctica stands are offered by Peri et al. (2021) and set seedling/resprout regeneration thresholds as: (1) none/scarce: ≤50 individuals ha−1; (2) good: <300 individuals ha−1, but >50 individuals ha−1; (3) very good: ≥300 individuals ha−1. Forest recovery after the recent fire was thus analyzed by comparing seedling/resprout regeneration in the recent fire to thresholds for both undegraded (for reference only, values listed in Section 2.2) and degraded forests, knowing that study sites more closely resemble degraded than undegraded forest. In our study, sapling regeneration was not considered for recovery analysis at the recent fire as not enough time had passed for many seedling-size individuals to grow into saplings and therefore serve as an indicator of potential forest recovery in the recently burned area.
Regeneration thresholds outlined by Peri et al. (2021) were not used to assess forest recovery at the older fire. Because of the amount of time that had passed since that fire, current seedling/resprout densities do not fully capture forest recovery that may have occurred over the last eight decades. We, therefore, used an estimated stand age ratio between burned and unburned forests to assess forest recovery at the older burned sites. Forests that burned over 80 years ago in the old fire are approximately a third as old as their control plots, which were estimated at ~240 years old, given the diameter to age relationship established in the region for N. antarctica (Ivancich, 2011). Assuming that forest recovery began immediately after the 1940's fire, burned-area stands are approximately a third as old as the adjacent unburned forest. Therefore, minimum recovery thresholds of 33% of unburned average canopy cover and total basal area were used to assess forest recovery in the old fire.
2.6. Data analysis
Seedling/resprout and sapling densities were standardized to stems ha-1 for each control and burned plot. These values could then be summed and averaged by site and by the recent fire vs. the older one. Regeneration differences were expressed as a percentage and calculated at the site and fire level as:
After averaging regeneration densities at the plot level, the effects of site characteristics and fire-impacted variables on regeneration density could also be analyzed by applying generalized linear mixed models (GLMMs). The average seedling/resprout and sapling densities from all sites combined were dependent variables. Although sapling regeneration was scarce in the 2019 fire, likely due to the fire having taken place recently, there were still some saplings present, given that resprouting N. antarctica trees often exhibit rapid height growth (Soler et al., 2018). We thought it important to capture the relative importance of drivers of this sapling regeneration in the recent fire as well as sapling regeneration present in the old fire. Site characteristics (elevation, aspect, slope, herbivore use, and time since fire) and fire-impacted variables (distance to mature live trees, canopy cover, total remnant basal area, and seed abundance) were used as fixed factors of analysis, while transects nested in sites were considered as a random factor. When considering the impact of distance to mature live trees, in particular, it was possible that adjacent plots referenced the same groups of trees and were therefore not strictly independent replicates. For all analyses, aspect was categorized numerically with north-facing plots given a value of one, northeast-facing plots a value of two, and so on, with south-facing plots being given the highest values. The negative binomial distribution using the log function yielded the lowest deviance and best model fit (AIC) for all response variables. Time since fire was 3 (years) for the recent burn plots, 82 (years) for the older fire burn plots, and left blank for control plots. A natural log transformation was used for seedling/resprout density to analyze the effect of distance to mature live trees and to calculate the intercept for fire-impacted variables and a natural log transformation was used for sapling density to analyze the effect of herbivore use. Significant predictors of regeneration identified in the GLMM analyses were then re-run with fires separated to detect whether effects differed in significance between fires. This was not done for time since fire as a single fire had the same time since fire for all burned plots. Generalized linear mixed models were adjusted using the nlme and lme4 packages of the R language through the statistical software interface to run R “InfoStat” (di Rienzo et al., 2015).
Seedling/resprout densities per plot in the recent fire were linearly correlated to the average distance to a mature live tree using Spearman's correlation (ρ). To determine which combinations of variables characterized burned and unburned plots, a Principal Component Analysis (PCA) was used based on a correlation matrix and included all site characteristic and fire-impacted variables except for total basal area and seed abundance, which were excluded because of their correlation with distance to mature live trees, and time since fire because it contains only two values that by nature divide old burned plots from recently burned plots. These analyses were completed with a Monte Carlo permutation test (n = 999) to assess the significance of each axis (p < 0.05). PCA analyses were carried out in PC-Ord 5.0 (McCune and Mefford, 2011).
3. Results
3.1. Regeneration patterns
All sites, across all fires, had less average per hectare regeneration in burned plots than unburned plots, except for the old fire site 1, where burned plots had 32% more regeneration on average than unburned controls (Table 2). Conversely, the old fire site 2 burned plots had the least regeneration relative to unburned plots, with 80% less regeneration than their respective control plots. However, this site had the highest overall average number of seedlings/resprouts and saplings per hectare (20,363 individuals ha−1) of any burned site. Overall, sites from the recent and old fire combined had only 32 seedlings/resprouts and saplings in burned plots for every 100 seedlings/resprouts and saplings in unburned plots, a difference of −68%. For the recent fire, burned plots had only 25 seedlings/resprouts and saplings per hectare for every 100 in the unburned plots. Regeneration in the older fire was 33% of control plots, a reduction in regeneration of −67%.
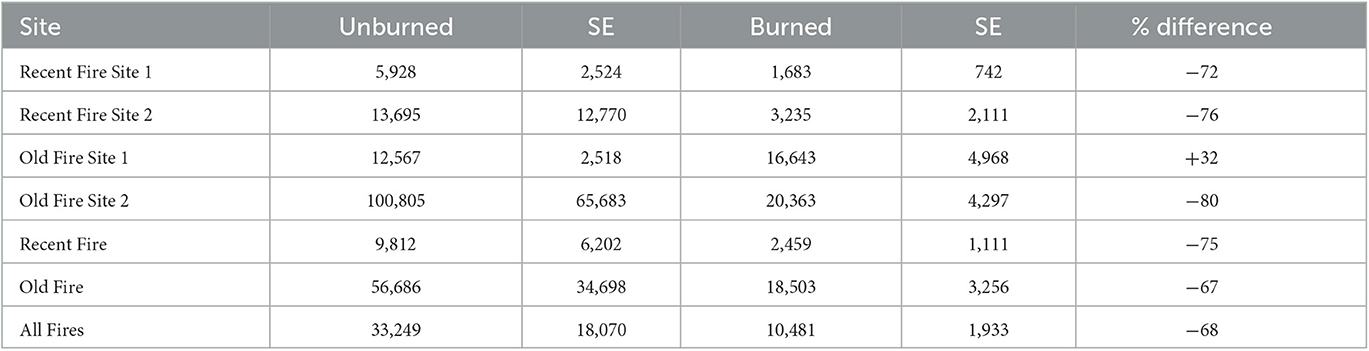
Table 2. Mean values (stems ha−1) of regeneration (seedlings/resprouts and saplings combined) at the site and fire level (n = 48 per site, 96 per fire).
Breaking regeneration down by class (seedlings/resprouts vs. saplings) revealed similar patterns as those described above (Figure 3). However, the difference in regeneration totals between unburned plots and burned plots was much larger for seedlings/resprouts than for saplings. Though control plots did have more sapling regeneration than burned plots, the difference was far less pronounced than for seedlings/resprouts, for which controls had thousands (recent fire) and tens of thousands (old fire) more seedlings/resprouts per hectare than burned plots.
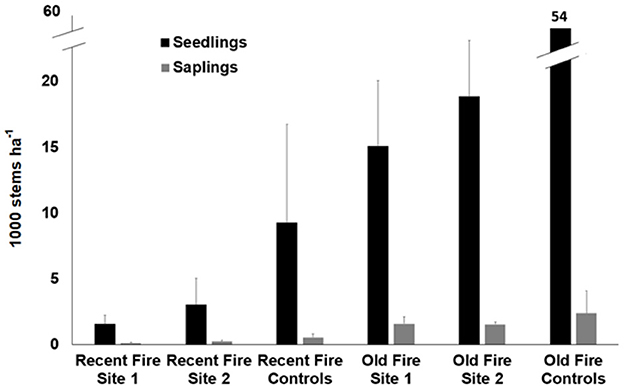
Figure 3. Mean regeneration broken down by site and divided into seedlings/resprouts (≤30 cm tall) (n = 96) and saplings (>30 cm, but <130 cm tall) (n = 96) in burned plots and unburned controls. Bars represent standard error; not shown for all controls to maintain scale.
Seedling-sized regeneration examined by origin (sexual vs. asexual) revealed that regeneration was heavily dominated by resprouts (Table 3). In total, 93% of the subset of 199 individuals examined were of asexual origin. This did not vary significantly based on time since fire: in the recent fire, 94% of seedling-sized plants examined originated from resprouting, whereas 92% originated from asexual reproduction in the older fire.

Table 3. Subsample of Nothofagus antarctica regeneration checked for reproductive origin (sexual vs. asexual) broken down by fire and number of individuals and percent of individuals originating from seed vs. sprouting.
3.2. Impact of key variables on regeneration
A greater number of fire-impacted variables, rather than site characteristics, explained seedling/resprout regeneration densities (Table 4). Time since fire was the only site characteristic that significantly explained seedling/resprout density, with seedling regeneration increasing linearly with greater time since fire. Of the fire-impacted variables, distance to mature live trees (negative) and canopy cover (positive) most clearly explained seedling/resprout density. Both variables had a linear relationship with seedling/resprout densities, with distance to mature live trees being the strongest. Canopy cover was significant in the recent fire (z = 2.39, p = 0.017), but not the older one (z = 0.42, p = 0.67). Distance to mature live trees was significant in recent fire sites (z = −3.89, p < 0.001) but not in older fire sites (z = −0.67, p > 0.5).
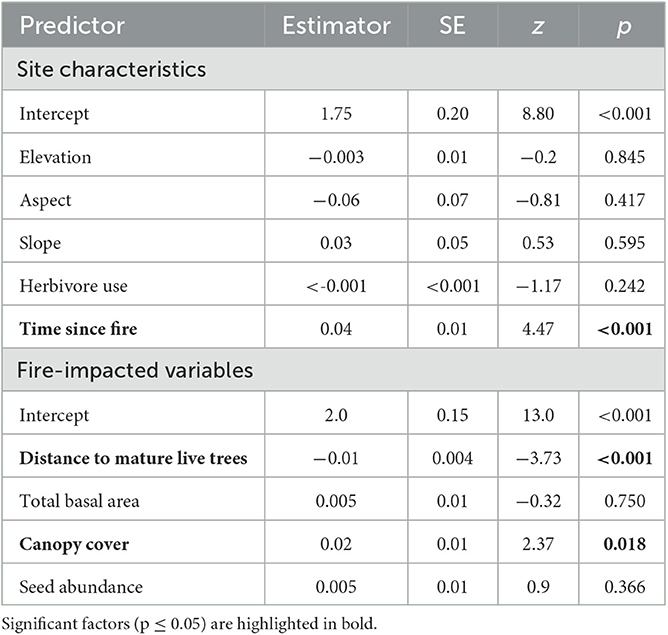
Table 4. GLMM analyses of factors impacting seedling/resprout regeneration grouped by site characteristics and fire-impacted variables (n = 96).
Sapling densities, conversely, were explained by more site characteristics than fire-impacted variables (Table 5). For sapling density, significant site characteristics were slope (positive) and time since fire (positive). Both time since fire vs. sapling density and slope vs. sapling density were linear relationships, with time since fire having a stronger correlation. Although slope was significant when both fires were considered together, it was not significant at the older fire (z = 0.33, p = 0.74) and only marginally significant at the recent fire (z = 1.74, p = 0.081). Distance to mature live trees (negative) was the only fire-impacted variable that explained sapling regeneration. This relationship was linear and significant in the recent fire sites (z = −4.18, p < 0.001), but not the older sites (z = 0.16, p = 0.87).
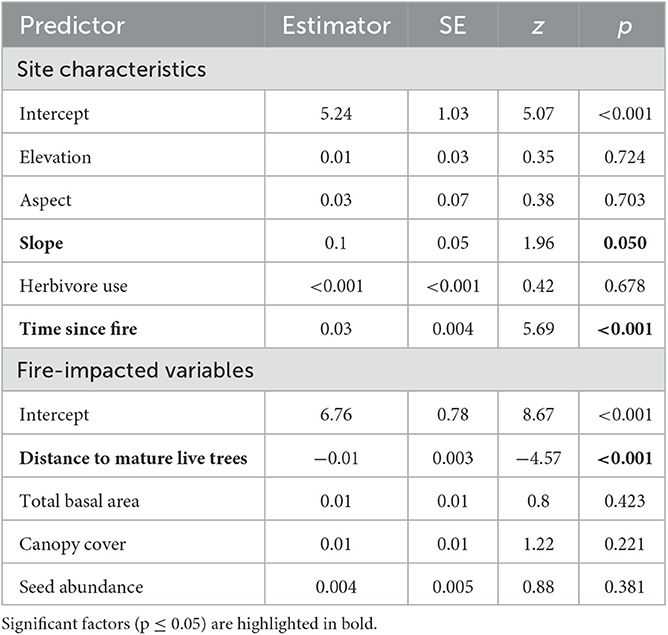
Table 5. GLMM analyses of factors impacting sapling regeneration grouped by site characteristics and fire-impacted variables (n = 96).
3.3. Forest recovery
At no point in the burned or unburned plots of the recent fire did average seedling-sized regeneration per plot approach 100,000 stems ha−1 (Table 2): recent fire site 1 had an average of 1,552 seedlings/resprouts per hectare and site 2 averaged 2,998 seedlings/resprouts per hectare. These values are 1.6 and 3%, respectively, of the low range value of seedlings/resprouts known to establish in the gaps of mature, undegraded N. antarctica forests (100,000 seedlings ha−1). However, recovery, or lack thereof, was not spatially uniform across the recently burned area. Seedling-sized regeneration densities per plot generally declined with increasing distance from mature live trees (ρ = −0.5, p < 0.001; Figure 4). When comparing regeneration recovery at the recent fire to thresholds for degraded N. antarctica forests, 35% of plots (n = 14) fell in the very good category (≥300 seedlings/resprouts ha−1), 35% of plots (n = 14) fell in the good category (>50, but <300 seedlings/resprouts ha−1), and 30% of plots (n = 12) fell in the scarce category (≤50 seedlings/resprouts ha−1).
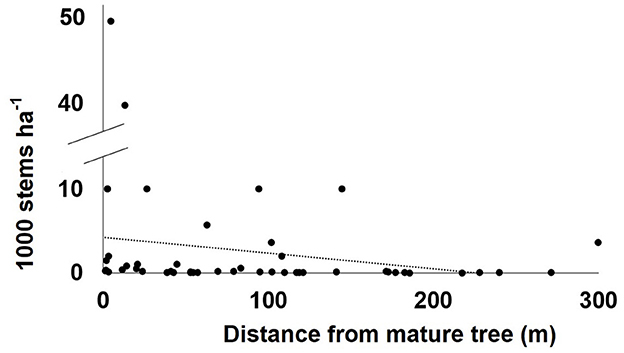
Figure 4. Seedling/resprout density (stems ha−1) of burned and control plots from the recent fire (n = 48) as a function of average distance from the closest mature live trees, with a line of best fit for the data shown.
Examining forest recovery in the older fire indicated that canopy cover reached thresholds for recovery (33% of unburned values) at both sites; however, live basal area only reached the recovery threshold at site 2. Canopy cover was highest in the old fire site 1, where it averaged 38.6%, which was 67% of its respective control plots; canopy cover also recovered to 60% of unburned values at the old fire site 2 (Table 6). Live basal area only reached 18% recovery at site 1 but did reach 36% at site 2. Recovery of both canopy cover and live basal area varied by plot and with distance from live mature trees, generally decreasing with increased distance from live mature trees (data not shown). Averaged across both old fire sites, beyond 10 m average distance from a mature live tree, live basal area was generally below the recovery threshold. Canopy cover, however, largely stayed above the recovery threshold even as distances to mature live trees increased beyond 10 m.

Table 6. Comparison of live basal area (BA) and canopy cover (CC) between burned and unburned plots 80 years after the old fire (n = 48).
The PCA displayed the combination of different site characteristics and fire-impacted variables (with the exception of total basal area, seed abundance, and time since fire) explaining the ordination of burned and unburned plots in all fires and controls (Figure 5). Two axes were retained for analysis. Axis one was significant (p < 0.05) and explained 32.9% of the variance and axis two was non-significant (p > 0.1) and explained 20.4% of the variance. Burned plots from the recent fire separated clearly from all other plot types along the horizontal axis. Old burned plots overlapped in some instances with recent and old unburned plots but also separated along the vertical axis in certain cases. Axis one, which captured more of the variability in recently burned plots, correlated most closely with distance to mature live trees (0.83), canopy cover (−0.73), and slope (−0.65). Axis two, encapsulating more of the variability in older burned plots and all unburned plots, was most strongly correlated with aspect (0.84) and elevation (0.71). A complete description of these results is provided in the Supplementary material.
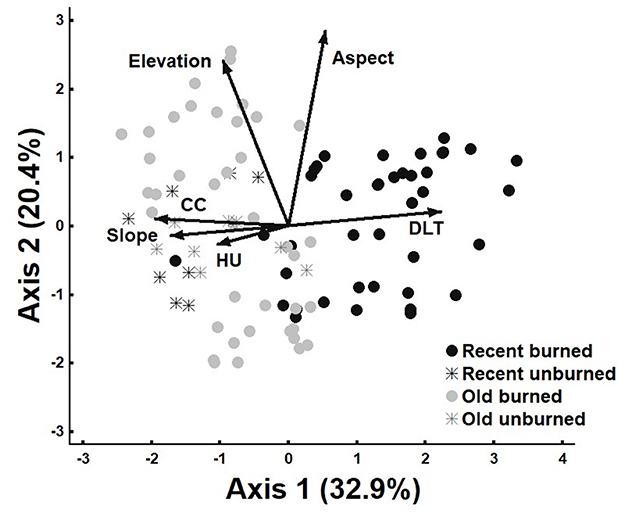
Figure 5. Principal Component Analysis (PCA) of explanatory variables in burned and unburned plots of the recent and old fire (n = 96); DLT, Distance to mature live trees; CC, Canopy cover; HU, Herbivore use.
4. Discussion
4.1. Site characteristics: seedlings and resprouts grow into saplings, despite herbivory
In absolute terms, there were, on average, many more thousands of seedlings/resprouts and saplings per hectare in the old fire than in the recent fire. This may be attributed to the fact that not enough time has passed since the recent fire occurred for seedlings/resprouts to establish in greater numbers and grow into saplings. There is also likely a site quality difference between the two sites that impacts regeneration height growth rates (Peri and Collado, 2009): the average height of trees in the older fire control plots was 9.3 vs. 7.3 m in the recent fire control plots. A number of factors other than site quality could explain this height difference, though, including differing histories of land use and disturbance. Trees in the control plots associated with the older fire are also likely at least 100 years older than those in the controls for the recent fire. However, given the higher rates of precipitation and warmer temperatures in the older burned area, it is reasonable to assume that the old fire sites have higher site qualities than the recent fire sites. Increased site quality is known to impact seed production, viability, and germination positively (e.g., Moya et al., 2007), and may have resulted in higher seedling/resprout and sapling densities in the older fire.
Both recent and old fire sites have been exposed to post-fire grazing, which could theoretically lead to reduced regeneration densities in heavily-used areas and widespread herbivore damage to plants. Herbivore use, however, was not statistically significant (Tables 4, 5) for seedlings/resprouts or saplings, and regeneration was rarely observed as being stunted, as is often the case for post-fire, grazed Nothofagus species (e.g., Ruggirello et al., 2023b). The high abundance of unpalatable grasses in burned areas and the presence of phenolic compounds in N. antarctica's leaves (González et al., 2018) may deter grazing. Downed logs can also provide protection to regeneration from herbivory (e.g., Fairweather et al., 2014), and the high number of large downed logs in both burned areas may deter cattle grazing in particular, allowing seedlings and resprouts to more frequently grow into saplings and, eventually, into sexually mature trees that can serve to further recolonize burned areas.
Slope was the only significant site characteristic other than time since fire, and was only significant for sapling density (Table 5). As is the case for all non-experimental wildfire research, sites are not selected at random, as they are defined by areas that were not experimentally burned. In the case of our two studied fires, the ranges of elevations, slopes, and aspects present were relatively narrow. Both fires took place on relatively gentle, north-facing terrain at similar elevations. Still, subtle differences in elevation, slope, and aspect between and within sites generally did not produce significant variation in regeneration densities: when gradients are mild, site characteristics do not appear to impact regeneration.
4.2. Fire-impacted variables: resprouts concentrate around legacies
Seedling/resprout density was positively correlated to canopy cover in both the recent and old fire (Table 2) but was not significant for saplings. This may best be explained by the silvics of N. antarctica and intermediate shade tolerant species in general: regeneration that has intermediate shade tolerance benefits from some shade under which they germinate and grow in the 1st years of life, but ultimately need the formation of gaps to thrive (Wagner et al., 2011; Zhu et al., 2014). Such a situation appears to be playing out at our study sites, as seedlings and resprouts are more likely to benefit from greater canopy cover than saplings.
Distance to mature live trees was significant for both seedlings/resprouts and saplings, but this relationship was only significant in the recent fire. In both the recent and old fire, seedlings/resprouts and saplings were defined using the same height criteria. This means that regeneration from the same classes in the two different fires established at very different moments in time after fire. As more than 80 years have passed since the old fire occurred and none of our predictive variables other than time since fire explained regeneration at the older burned sites, regeneration patterns there are responding to factors not analyzed in this study. Suitable microsite availability, for example, may be driving regeneration patterns in the older fire, where large swaths of the burned area have converted to savannahs dominated by grasses and dotted with occasional, scattered trees. In these conditions, dense mats of grasses, composed mainly of introduced forage species that established over time post-fire, likely limit tree regeneration (Ruggirello et al., 2022). Seedlings, resprouts, and saplings are thus often limited to areas along fences and roadsides, where disturbance creates opportunities for N. antarctica to establish (Soler et al., 2018). In the recent fire, conversely, grasses and forbs have not yet widely established and the burned area is still largely dominated by bare-mineral soil. Seedlings and resprouts in the recent fire, therefore, do not experience the same intense competition and absence of suitable microsites that they do in the older fire.
Still, if regeneration in the most recent fire were being driven principally by resprouting from top-killed individuals, distance to mature live trees would not have been strongly tied to seedling/resprout and sapling densities. When regeneration is tied exclusively to resprouting from top-killed individuals, there is no clear link between regeneration and distance to mature live trees or the unburned forest edge (e.g., Owen et al., 2017). The fact that N. antarctica regeneration is tied significantly to mature live trees and not to total basal area, which captures top-killed individuals capable of resprouting, suggests that resprouting from top-killed trees may not be the dominant form of widespread burned-area colonization, but rather a dynamic playing out sporadically immediately after a fire (Burns, 1993; Steinke et al., 2008). Still, the subset of seedlings examined in this study for reproductive origin revealed a pattern already observed in N. antarctica (Steinke et al., 2008), that seedling/resprout regeneration is largely driven by asexual reproduction and not by seeds (Table 3). Here, we see a distinction between resprouting from top-killed individuals and sprouting from mature live trees that survived the fire (also called legacies). Given the strong link between regeneration and mature live trees (and not to total basal area encapsulating top-killed individuals), N. antarctica may depend more strongly on sprouting, asexual reproduction, from legacies that survive fire or trees that are unaffected by fire (those outside the fire boundary) than on resprouting from top-killed individuals. Although some top-killed individuals do resprout post-fire, many do not. In the recent fire, in particular, larger top-killed individuals (average DBH > 20 cm) almost never resprouted, while smaller mature individuals (average DBH < 20 cm) were frequently observed resprouting. Older, larger, and reburned individuals may not be able to resprout, as is the case for other tree species (Golden, 1999; Belz, 2003; Bock et al., 2007). For now, we can only circumstantially conclude that increases in an N. antarctica tree's size, age, and fire exposure may inhibit its resprouting ability after being top-killed.
4.3. Forests will likely take over a century to recover
Although N. antarctica may not rely as strongly on top-killed resprouting as on sprouting from legacies, its prolific asexual reproduction does result in some degree of forest recovery. Canopy cover reached recovery thresholds in both old fire sites and basal area reached set thresholds at one of the two sites. The fact that canopy cover recovery was stronger than basal area recovery may be attributed to the fact that N. antarctica emphasizes height growth when young before adding diameter and thus basal area with age. Ultimately, basal area also appears to be recovering, but more slowly than canopy cover.
Seedling/resprout regeneration was good or very good in 70% of the plots recently burned by fire. Recovery within the fires varied considerably by location, however, as N. antarctica expanded outward from surviving legacies and certain top-killed individuals. Though N. antarctica may be able to resprout and expand around surviving and some top-killed trees and therefore begin to re-establish in burned areas, this process has not resulted in complete forest recovery ~80 years after the old fire. Wildfires produce severe impacts in Tierra del Fuego (Ruggirello et al., 2023c), top-killing or completely killing most trees within a burned area. Although there are likely many variables at play that result in slow post-fire forest recovery, the absence of sufficient individuals capable of resprouting post-fire due to high fire severity is likely a significant factor that slows forest recovery. For example, in the recent fire, only two of 40 plots contained living mature trees that survived the fire. With top-killed individuals resprouting sporadically at this fire, asexual reproduction from live trees along the fire boundary or legacies that survived fire will likely take decades or centuries to return the area to pre-fire conditions. As a result, tree cover in the short to mid-term is fragmented and patchy.
N. antarctica's ability to recover post-fire, albeit slowly, does nevertheless provide some hope that closed-canopy forests may not be permanently lost after wildfire, which could be significant considering that many N. pumilio stands in Tierra del Fuego transition to grasslands or shrublands after fire and show few signs of ever returning to pre-fire conditions (Ruggirello et al., 2023b). N. antarctica forests that reform post-fire, however, will not resemble pre-fire forests for well over a century until trees fill in the area between legacies and expand out from fire boundaries mainly through asexual reproduction. Until this process unfolds completely, savannah-like conditions will dominate the post-fire landscape. These conditions generally favor grassland fauna and understory plant species and provide different ecosystem services than denser-canopy forests (Ruggirello et al., 2023a).
5. Restoration recommendations
In burned areas with relatively uniform site conditions, variations in aspect, elevation, and slope do not generally impact N. antarctica regeneration. Recolonization of burned areas is driven by sprouting from live individuals and supplemented by sporadic resprouting from top-killed individuals. As not all top-killed individuals resprout post-fire, when conducting forest restoration, it is important to identify resprouting patterns for a particular burned area by determining which size and age classes of top-killed trees tend to resprout and where individuals are more or less likely to sprout (e.g., hilltops, roadsides, and low-severity burn patches). Planting (active restoration) will be most necessary where live trees are absent and top-killed individuals are unlikely to resprout.
Data availability statement
The raw data supporting the conclusions of this article will be made available by the authors, without undue reservation.
Author contributions
MR: investigation, methodology, data curation, formal analysis, visualization, writing—original draft, and writing—review and editing. RS: investigation, methodology, formal analysis, supervision, and writing—review and editing. GB: investigation and writing—review and editing. PF: supervision and writing—review and editing. All authors contributed to the article and approved the submitted version.
Funding
Funding for this work was provided by the Argentine National Scientific and Technical Research Council (CONICET) and the National Agency for Scientific Promotion through project PICT 2019 675. Additional funding for fieldwork was provided by The Rufford Foundation (grant number 35530-1/2) and the International Association of Wildland Fire (IAWF).
Acknowledgments
We would like to thank Santiago Favoretti of the Lenga Patagonia forestry company, Jorge and Silvia Sevillano of Estancia Pirinaica, and Martin Parodi and Seba Farina of the Tierra del Fuego Provincial Department of Forestry for their collaboration and willingness to support research sites. We would also like to thank Francisco Mattenet of the locally-sourced products company Neurona and Ana Josefina Tibaudin for help with field sampling.
Conflict of interest
The authors declare that the research was conducted in the absence of any commercial or financial relationships that could be construed as a potential conflict of interest.
Publisher's note
All claims expressed in this article are solely those of the authors and do not necessarily represent those of their affiliated organizations, or those of the publisher, the editors and the reviewers. Any product that may be evaluated in this article, or claim that may be made by its manufacturer, is not guaranteed or endorsed by the publisher.
Supplementary material
The Supplementary Material for this article can be found online at: https://www.frontiersin.org/articles/10.3389/fevo.2023.1113970/full#supplementary-material
References
Allué, C., Arranz, J. A., Bava, J., Beneitez, J. M., Collado, L., and García-López, J. M. (2010). Phytoclimatic characterization and cartography of subantarctic native forests in Isla Grande de Tierra del Fuego (Patagonia, Argentina). For. Syst. 19, 189–207. doi: 10.5424/fs/2010192-01314
Bahamonde, H., Martínez Pastur, G., Soler, R., and Monelos, L. (2018). Ten years of seed production and establishment of regeneration measurements in Nothofagus antarctica forests under different crown cover and quality sites, in Southern Patagonia. Agroforest. Syst. 92, 623–635. doi: 10.1007/s10457-016-9999-7
Bahamonde, H., Peri, P., Monelos, L., and Martínez Pastur, G. (2013). Regeneración por semillas en bosques nativos de Nothofagus antarctica bajo uso silvopastoril en Patagonia Sur, Argentina. Bosque 34, 89–101. doi: 10.4067/S0717-92002013000100011
Belz, D. (2003). Severing red alder: timing the cut to achieve the best mortality. West. J. Appl. For. 18, 199–201. doi: 10.1093/wjaf/18.3.199
Boag, A. E., Ducey, M. J., Palace, M. W., and Hartter, J. (2020). Topography and fire legacies drive variable post-fire juvenile conifer regeneration in eastern Oregon, USA. For. Ecol. Manag. 474, 118312. doi: 10.1016/j.foreco.2020.118312
Bock, C. E., Kennedy, L., Bock, J. H., and Jones, Z. F. (2007). Effects of fire frequency and intensity on velvet mesquite in an Arizona grassland. Rangel. Ecol. Manag. 60, 508–514. doi: 10.2111/1551-5028(2007)60508:EOFFAI2.0.CO
Burns, B. R. (1993). Fire-induced dynamics of Araucaria araucana-Nothofagus antarctica forest in the southern Andes. J. Biogeogr. 20, 669–685. doi: 10.2307/2845522
Busby, S. U., Moffett, K. B., and Holz, A. (2020). High-severity and short-interval wildfires limit forest recovery in the Central Cascade Range. Ecosphere 11, e03247. doi: 10.1002/ecs2.3247
Catry, F. X., Moreira, F., Tujeira, R., and Silva, J. S. (2013). Post-fire survival and regeneration of Eucalyptus globulus in forest plantations in Portugal. For. Ecol. Manag. 310, 194–203. doi: 10.1016/j.foreco.2013.08.036
Chianucci, F., Bajocco, S., and Ferrara, C. (2021). Continuous observations of forest canopy structure using low-cost digital camera traps. Agr. For. Meteorol. 307, 108516. doi: 10.1016/j.agrformet.2021.108516
Clark, J. S., Beckage, B., Camill, P., Cleveland, B., Hille Ris Labers, J., Lichter, J., et al. (1999). Interpreting recruitment limitation in forests. Am. J. Bot. 86, 1–16. doi: 10.2307/2656950
Coop, J., Massatti, R., and Schoettle, A. (2010). Subalpine vegetation pattern three decades after stand-replacing fire: effects of landscape context and topography on plant community composition, tree regeneration, and diversity. J. Veg. Sci. 21, 472–487. doi: 10.1111/j.1654-1103.2009.01154.x
Dawe, D. A., Peters, V. S., and Flannigan, M. D. (2020). Post-fire regeneration of endangered limber pine (Pinus flexilis) at the northern extent of its range. For. Ecol. Manag. 457, 1127. doi: 10.1016/j.foreco.2019.117725
di Rienzo, J. A., Casanoves, F., Balzarini, M. G., and Gonzalez, L. (2015). InfoStat. Available online at: http://www.infostat.com.ar (accessed April 2023).
Donato, D., Fontaine, J., Robinson, W., Kauffman, J., and Law, B. (2009). Vegetation response to a short interval between high-severity wildfires in a mixed-evergreen forest. J. Ecol. 97, 142–154. doi: 10.1111/j.1365-2745.2008.01456.x
Echevarría, D., von Müller, A., Hansen, N., and Bava, J. (2014). Efecto del ramoneo bovino en renovales de Nothofagus antarctica en Chubut, Argentina, en relación con la carga ganadera y la altura de las plantas. Bosque 35, 353–368. doi: 10.4067/S0717-92002014000300010
Errington, R. C., and Pinno, B. D. (2021). Relationships between overstory and understory components of young natural and reconstructed boreal aspen stands. Ecol. Restor. 39, 182–193. doi: 10.3368/er.39.3.182
Fairweather, M. L., Rokala, E. A., and Mock, K. E. (2014). Aspen seedling establishment and growth after wildfire in central Arizona: an instructive case history. For. Sci. 60, 703–712. doi: 10.5849/forsci.13-048
Frazer, G. W., Canham, C. D., and Lertzman, K. P. (1999). Gap Light Analyzer (GLA), Version 2.0: Imaging Software to Extract Canopy Structure and Gap Light Transmission Indices From True-Colour Fisheye Photographs, Users Manual and Program Documentation. Burnaby, BC: Copyright © 1999: Simon Fraser University; Millbrook, NY: Institute of Ecosystem Studies.
Golden, M. S. (1999). “Factors affecting sprouting success in a bottomland mixed hardwood forest,” in Proceedings of the Tenth Biennial Southern Silvicultural Research Conference. USDA For. Gen. Tech. Rep. SRS-30 (Shreveport, LA), 157–163.
González, S., Gastaldi, B., Mattenet, F., Peri, P. L., van Baren, C., Di Leo, L. P., et al. (2018). Análisis fitoquímicos comparativos de Nothofagus antarctica proveniente de dos sitios con ambientes diferentes en la provincia de Santa Cruz. Dominguezia 34, 41–42.
Goya, J. F., Frangi, J. L., Barrera, M. D., and Puigdefábregas, J. (2005). Ecología de los bosques de Tierra del Fuego. Buenos Aires: Editorial de la Universidad Nacional de La Plata (EDULP).
Hammett, E. J., Ritchie, M. W., and Berrill, J. P. (2017). Resilience of California black oak experiencing frequent fire: regeneration following two large wildfires 12 years apart. Fire Ecol. 13, 91–103. doi: 10.4996/fireecology.1301091
Heusser, C. J. (1994). Paleoindians and fire during the late Quaternary in southern South America. Rev. Chil. Hist. Nat. 67, 435–443.
Holz, A., Wood, S. W., Veblen, T. T., and Bowman, D. M. (2015). Effects of high-severity fire drove the population collapse of the subalpine Tasmanian endemic conifer Athrotaxis cupressoides. Glob. Change Biol. 21, 445–458. doi: 10.1111/gcb.12674
Iranzo, E. C., Smith, C., Moraga, C. A., Radic-Schilling, S., and Corti, P. (2022). Patterns of guanaco distribution and microhabitat use in Tierra del Fuego: from protected to sheep ranching areas. Acta Oecol. 116, 103853. doi: 10.1016/j.actao.2022.103853
Ivancich, H. (2011). Relaciones entre la estructura forestal y el crecimiento del bosque de Nothofagus antarctica en gradientes de edad y calidad de sitio. (dissertation/doctoral thesis), National University of La Plata, La Plata, Argentina.
Johnstone, J. F., Celis, G., Chapin, F. S., Hollingsworth, T. N., Jean, M., and Mack, M. C. (2020). Factors shaping alternate successional trajectories in burned black spruce forests of Alaska. Ecosphere 11, e03129. doi: 10.1002/ecs2.3129
Keeley, J. (2009). Fire intensity, fire severity and burn severity: a brief review and suggested usage. Int. J. Wildland Fire 18, 116–126. doi: 10.1071/WF07049
Keeley, J., Pausas, J., Rundel, P., Bond, W., and Bradstock, R. (2011). Fire as an evolutionary pressure shaping plant traits. Trends Plant Sci. 16, 406–411. doi: 10.1016/j.tplants.2011.04.002
Kemp, K., Higuera, P., Morgan, P., and Abatzoglou, J. (2019). Climate will increasingly determine post-fire tree regeneration success in low-elevation forests, Northern Rockies, USA. Ecosphere 10, e02568. doi: 10.1002/ecs2.2568
Lamont, B. B., Pausas, J. G., He, T., Witkowski, E. T., and Hanley, M. E. (2020). Fire as a selective agent for both serotiny and nonserotiny over space and time. Crit. Rev. Plant Sci. 39, 140–172. doi: 10.1080/07352689.2020.1768465
Lee, C. S., Robinson, G. R., and Robinson, I. P. (2019). Regeneration of pitch pine (Pinus rigida) stands inhibited by fire suppression in Albany Pine Bush Preserve, New York. J. For. Res. 30, 233–242. doi: 10.1007/s11676-018-0644-3
Lloret, F., Casanovas, C., and Penuelas, J. (1999). Seedling survival of Mediterranean shrubland species in relation to root: shoot ratio, seed size and water and nitrogen use. Funct. Ecol. 13, 210–216. doi: 10.1046/j.1365-2435.1999.00309.x
Lothrop, S. K. (1928). The Indians of Tierra del Fuego. New York. NY: Museum of the American Indian, Heye Foundation.
McCord, M., Reilly, M., Jules, E., and Butz, R. (2019). Early seral pathways of vegetation change following repeated, short interval high-severity wildfire in a low elevation mixed-conifer-hardwood forest landscape of the Klamath Mountains, California. Can. J. For. Res. 50, 13–23. doi: 10.1139/cjfr-2019-0161
McCune, B., and Mefford, M. (2011). PC-ORD: Multivariate Analysis of Ecological Data. Version 4. Gleneden Beach, OR: MjM Software.
McKenzie, D. A., and Tinker, D. B. (2012). Fire-induced shifts in overstory tree species composition and associated understory plant composition in Glacier National Park, Montana. Plant Ecol. 213, 207–224. doi: 10.1007/s11258-011-0017-x
Moya, D., Espelta, J. M., Verkaik, I., López-Serrano, F., and De Las Heras, J. (2007). Tree density and site quality influence on Pinus halepensis Mill. reproductive characteristics after large fires. Ann. For. Sci. 64, 649–656. doi: 10.1051/forest:2007043
Owen, S. M., Sieg, C. H., Meador, A. J. S., Fulé, P. Z., Iniguez, J. M., Baggett, L. S., et al. (2017). Spatial patterns of ponderosa pine regeneration in high-severity burn patches. For. Ecol. Manag. 405, 134–149. doi: 10.1016/j.foreco.2017.09.005
Parks, S. A., Holsinger, L. M., Miller, C., and Parisien, M. (2018). Analog-based fire regime and vegetation shifts in mountainous regions of the western US. Ecography 41, 910–921. doi: 10.1111/ecog.03378
Peeler, J., and Smithwick, E. (2020). Seed source pattern and terrain have scale-dependent effects on post-fire tree recovery. Landscape Ecol. 35, 1071. doi: 10.1007/s10980-020-01071-z
Peri, P. L., Bahamonde, H. A., Lencinas, M. V., Gargaglione, V., Soler, R., Ormaechea, S., et al. (2016). A review of silvopastoral systems in native forests of Nothofagus antarctica in southern Patagonia, Argentina. Agroforest. Syst. 90, 933–960. doi: 10.1007/s10457-016-9890-6
Peri, P. L., and Collado, L. (2009). Relevamiento de los bosques nativos de ñire (Nothofagus antartica) de Tierra del Fuego (Argentina) como herramienta para el manejo sustentable. Buenos Aires: Instituto Nacional de Tecnología Agropecuaria.
Peri, P. L., Rusch, V. E., Von Müller, A., Varela, S. A., Quinteros, C. P., and Martinez Pastur, G. J. (2021). Manual de Indicadores para Monitoreo de Planes Prediales de Manejo de Bosque con Ganadería Integrada–MBGI Región Patagónica. Buenos Aires: Instituto Nacional de Tecnología Agropecuaria.
Premoli, A. C., and Steinke, L. (2008). Genetics of sprouting: effects of long-term persistance in fireprone ecosystems. Mol. Ecol. 17, 3827–3827. doi: 10.1111/j.1365-294X.2008.03889.x
Pulido, F. J., Díaz, B., and Martinez-Pastur, G. J. (2000). Incidencia Del Ramoneo Del Guanaco (Lama Guanicoe Müller) Sobre La Regeneración Temprana En Bosques De Lenga [Nothofagus Pumilio (Poepp Et Endl) Krasser] De Tierra Del Fuego, Argentina. Invest. Agr. Sist. Recur. For. 9, 381–394.
Raffaele, E., Veblen, T., Blackhall, M., and Tercero-Bucardo, N. (2011). Synergistic influences of introduced herbivores and fire on vegetation change in northern Patagonia, Argentina. J. Veg. Sci. 22, 59–71. doi: 10.1111/j.1654-1103.2010.01233.x
Rossman, A., Bakker, J., Peterson, D., and Halpern, C. (2020). Long-term effects of fuels treatments, overstory structure, and wildfire on tree regeneration in dry forests of central Washington. Forests 11, 888. doi: 10.3390/f11080888
Ruggirello, M. J., Soler, R., and Bustamante, G. (2023b). Nothofagus pumilio regeneration failure following wildfire threatens sub-antarctic forests of Tierra del Fuego, Argentina. Forestry 2023, cpad028. doi: 10.1093/forestry/cpad028
Ruggirello, M. J., Soler, R., Bustamante, G., and Fulé, P. (2023c). “Determining burn severity and its impact on post-fire regeneration in the Nothofagus forests of Tierra del Fuego, Argentina,” in Poster Presented at the 8TH International Wildland Fire Conference, Porto, Portugal, May 16-19, 2023.
Ruggirello, M. J., Soler, R., Bustamante, G., and Lencinas, M. V. (2023a). Understory plant dynamics following a wildfire in southern Patagonia. For. Ecol. Manag. 527, 120606. doi: 10.1016/j.foreco.2022.120606
Ruggirello, M. J., Soler, R. M., Bustamante, G. N., and Lencinas, M. V. (2022). “Setting restoration priorities for burned Nothofagus forests: impacts of wildfire on microsite abundance,” in Poster Presented at: The International Association for Ecology 2022 Hybrid Conference. Available online at: https://www.researchgate.net/publication/363066637_Setting_Restoration_Priorities_For_Burned_Nothofagus_Forests_Impacts_Of_Wildfire_On_Microsite_Abundance (accessed April 2023).
Soler, R., Lencinas, M. V., Bustamante, G., and Martínez Pastur, G. M. (2018). Atributos de la regeneración natural de ñire (Nothofagus antarctica) en Tierra del Fuego: beneficios y perjuicios que genera el uso silvopastoril. Ecosist 27, 41–47. doi: 10.7818/ECOS.1505
Soler, R., Martínez Pastur, G., Peri, P., and Lencinas, M. V. (2013). Are silvopastoral systems compatible with forest regeneration? An integrative approach in southern Patagonia. Agroforest. Syst. 87, 1213–1227. doi: 10.1007/s10457-013-9631-z
Steinke, L. R., Premoli, A. C., Souto, C. P., and Hedrén, M. (2008). Adaptive and neutral variation of the resprouter Nothofagus antarctica growing in distinct habitats in north-western Patagonia. Silva Fenn. 42, 177. doi: 10.14214/sf.251
Tattoni, C., Chianucci, F., and Ciolli, M. (2021). A comparison of ground-based count methods for quantifying seed production in temperate broadleaved tree species. Ann. For. Sci. 78, 11. doi: 10.1007/s13595-020-01018-z
Torres, R. C., Giorgis, M. A., Trillo, C., Volkmann, L., Demaio, P., Heredia, J., et al. (2014). Post-fire recovery occurs overwhelmingly by resprouting in the Chaco Serrano forest of Central Argentina. Austral Ecol. 39, 346–354. doi: 10.1111/aec.12084
Tyukavina, A., Potapov, P., Hansen, M. C., Pickens, A. H., Stehman, S. V., Turubanova, S., et al. (2022). Global trends of forest loss due to fire from 2001 to 2019. Front. Rem. Sens. 3, 825190. doi: 10.3389/frsen.2022.825190
Veblen, T. T., Kitzberger, T., Villalba, R., and Donnegan, J. (1999). Fire history in northern Patagonia: the roles of humans and climatic variation. Ecol. Monogr. 69, 47–67. doi: 10.1890/0012-9615(1999)0690047:FHINPT2.0.CO
Veblen, T. T., and Lorenz, D. C. (1988). Recent vegetation changes along the forest/steppe ecotone of northern Patagonia. Ann. Am. Assoc. Geogr. 78, 93–111. doi: 10.1111/j.1467-8306.1988.tb00193.x
Wagner, S., Fischer, H., and Huth, F. (2011). Canopy effects on vegetation caused by harvesting and regeneration treatments. Eur. J. For. Res. 130, 17–40. doi: 10.1007/s10342-010-0378-z
Keywords: wildfire, ñire, Patagonia, regeneration, restoration, resprout, resilience, time since fire
Citation: Ruggirello MJ, Bustamante G, Fulé PZ and Soler R (2023) Drivers of post-fire Nothofagus antarctica forest recovery in Tierra del Fuego, Argentina. Front. Ecol. Evol. 11:1113970. doi: 10.3389/fevo.2023.1113970
Received: 01 December 2022; Accepted: 19 May 2023;
Published: 22 June 2023.
Edited by:
Anthony Lehmann, University of Geneva, SwitzerlandReviewed by:
Andrew Marder Barton, University of Maine at Farmington, United StatesJennifer Schafer, Winthrop University, United States
Copyright © 2023 Ruggirello, Bustamante, Fulé and Soler. This is an open-access article distributed under the terms of the Creative Commons Attribution License (CC BY). The use, distribution or reproduction in other forums is permitted, provided the original author(s) and the copyright owner(s) are credited and that the original publication in this journal is cited, in accordance with accepted academic practice. No use, distribution or reproduction is permitted which does not comply with these terms.
*Correspondence: Matthew Joseph Ruggirello, matthew.ruggirello@conicet.gov.ar