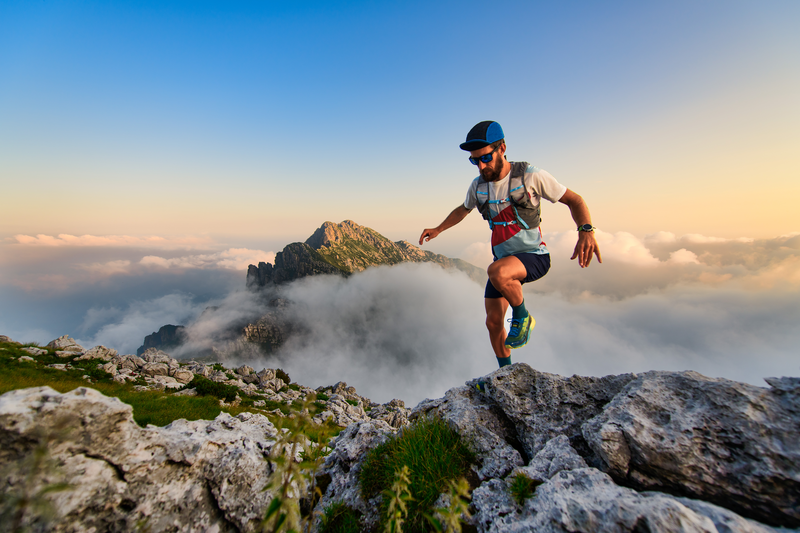
95% of researchers rate our articles as excellent or good
Learn more about the work of our research integrity team to safeguard the quality of each article we publish.
Find out more
ORIGINAL RESEARCH article
Front. Ecol. Evol. , 27 October 2023
Sec. Behavioral and Evolutionary Ecology
Volume 11 - 2023 | https://doi.org/10.3389/fevo.2023.1112045
This article is part of the Research Topic The Evolutionary Roots of Reproductive Ageing and Reproductive Health Across the Tree of Life View all 17 articles
Flourishing recent comparative studies on senescence have revealed an uncovered diversity across the tree of life of the shapes of the age trajectories of mortality (actuarial senescence) and to a lesser extent of reproduction (reproductive senescence). Evolutionary theories have been called up to explain why some species suffer from positive senescence while others benefit from negligible or even negative senescence. We still know little about how, within a species, the shapes of the age trajectories of different traits are linked to each other and how they vary or covary depending on the genetic background and environmental conditions. We report here the results of an experimental study whose aim was to describe the actuarial and reproductive senescence in various genetically distinct lineages of a Collembola, a hexapod with indeterminate growth. We compared the age trajectories of individuals raised under two food regimes to study if and how the shapes of these age trajectories are plastically modified by environmental conditions. We found clear evidence of actuarial and reproductive senescence, especially when the springtails were fully fed. Clutch size increased as female become older and then declined progressively after reproduction reached a maximum. This age decline in fertility went along with a progressive slowing down of the pace of the egg-laying, a reduction of egg quality (more sterile eggs), while egg size undergoes little change with age. We found that the onset of reproductive decline occurred before the beginning of actuarial senescence, and show that escaping senescence is physiologically possible for certain lineages under dietary restricted conditions.
While ageing affects every organism - because all become older with time - only some of them senesce. Indeed, following several authors (Medawar, 1952; Baudisch, 2011), we use the word ageing to describe the effect of time on adult’s phenotypes without its familiar connotation of decline at advanced ages while senescence refers to a progressing age-related decline of several functional traits.
Empirical studies on senescence in the wild, and on experimental populations, have focused on two major fitness components: survival (actuarial senescence) and reproduction (reproductive senescence). While it has long been thought that senescence was somehow inevitable – apart from a few well-known special cases (Martínez, 1998; Schaible et al., 2015) – survival and reproduction have been found to decrease at various rates, depending on species, populations or on some environmental factors. Several comparative studies have demonstrated the existence of a great diversity in the patterns of ageing, the shape of the reproductive and survival age trajectories ranging from senescence (decline of reproduction or survival with age) to negative senescence (increase of reproduction or survival with age) through negligible senescence (negligible effect of age) (Baudisch, 2011; Baudisch et al., 2013; Jones et al., 2014; Baudisch and Stott, 2019; Colchero et al., 2019; Lemaître et al., 2020; Reinke et al., 2022).
Similarly, some studies have revealed a remarkable qualitative intraspecific variation in the age pattern of reproduction and mortality in species like dogs (Kraus et al., 2013), social insects (Münch et al., 2008), reptiles and amphibians (Cayuela et al., 2020a; Tully et al., 2020; Cayuela et al., 2021) or hexapods (Mallard et al., 2015; Li and Zhang, 2021), while other studies have found similar age-specific mortality patterns across populations of the same species (Reichard, 2016).
Although many traits can be simultaneously affected by senescence, most studies have focused on describing the effects of age on a single trait in isolation, usually survival rate (Hoekstra et al., 2020). Few longitudinal studies have jointly studied for instance the shape of the age trajectories of mortality and reproduction (Roach and Carey, 2014; Mallard et al., 2015; Tully et al., 2020; Li and Zhang, 2021). A multi-trait approach is, however, required to describe and compare how the effects of age manifest themselves on the different components of fitness. This can help address the question of the timing of senescence and more particularly whether it is a synchronized process. Indeed, in theory, the onsets of actuarial and of the different components of reproductive senescence should be close to each other, senescence being expected to begin at the time of reproductive maturation (Williams, 1957). This prediction has been challenged (Gaillard and Lemaître, 2017). Several empirical studies have for instance reported pronounced levels of asynchrony between actuarial and several components of reproductive senescence. The death rate of green whip snakes has been shown to increase after about six years while their fecundity continued to increase with age (Cayuela et al., 2020b). Similarly, in a population of meadow vipers, the mortality was found to increase markedly with age after about 4–5 years while no sign of reproductive senescence could be observed on several reproductive traits (Tully et al., 2020). In the laboratory, a cohort follow-up of the Collembola Folsomia candida showed that mortality started to increase progressively long before reproduction began to decline (Mallard et al., 2015). These observations are still too few in number to know to what extent this asynchrony is general and whether reproductive decline is always subsequent to the onset of actuarial senescence. On the other hand, the synchrony of the different components of reproduction remains very little studied (Tully et al., 2020).
The timing and rate of senescence have also been found to vary between groups of individuals such as sexes, populations, lineages, and according to environmental factor such as temperature, competition, food provisioning for instance (Massot et al., 2011; Kelly et al., 2013; Hayward et al., 2015; Hayward et al., 2015; Mallard et al., 2015; Stroustrup et al., 2016; Gribble et al., 2018; Cayuela et al., 2020b; Tully et al., 2020; Cayuela et al., 2021).
Food provisioning is probably the most studied environmental factors known to modulate the timing and intensity of senescence: a moderate reduction of food intake through caloric or dietary restriction increases the longevity in a large range of taxa by delaying the onset and slowing down the rate of actuarial senescence (Mair and Dillin, 2008). Quite logically, low food availability is also generally associated with a reduced reproduction given that reproductive output is constrained by the availability of nutrients. The effect of a food scarcity on reproductive senescence has been less well studied (Auld and Henkel, 2014). In a freshwater snail, a change in diet (Lettuce compared to Spirulina) associated with a longer somatic lifespan was tied with a prolonged reproductive lifespan (Auld, 2018) and the negative effect of parental age on offspring survival was stronger in individuals fed with richer food (Spirulina), thus suggesting that, as for mortality, a poor diet (Lettuce) may result in slowing down reproductive senescence. Similarly, in the Collembola Folsomia candida, compared to a rich diet (yeast), a poor diet (leaf diet) decreased the oviposition rate and also delayed and slowed down the loss of mean fertility as groups of individuals become old (Van Amelsvoort and Usher, 1989). In a selection experiment, the shape of the age-specific fecundity of Drosophila melanogaster changed depending on the food selection regime (Dasgupta et al., 2022). Dietary restriction has also been found to slow down the rate of germline stem cells loss with age in Drosophila melanogaster (Mair et al., 2010).
These studies suggest that reproductive senescence may itself be modulated by food provisioning but the specific analysis of how a change in food provisioning modifies the shape of the reproductive age-trajectories remains to be clarified. Can dietary restriction modify the mean level of reproductive output and also, on the long term, the onset and rate of reproductive senescence? Are the age trajectories of different components of reproduction equally sensitive to a change in food provisioning? And finally, can an environmental factor like food modulate the asynchrony between different components of ageing?
Our aim here is to use a laboratory longitudinal study of the ametabolous hexapod Folsomia candida Willem, 1902 (Collembola: Isotomidae (Willem, 1902)) to address these various questions. This species has already proven to be an interesting model organism in ageing research (Van Amelsvoort and Usher, 1989; Tully and Ferrière, 2008; Tully and Lambert, 2011; Mallard et al., 2015). Being a parthenogenetic species, individuals - all females - can be isolated, raised and followed up from birth to death, which usually occurs after several months. Moreover, Collembola are in a way comparable to non-avian reptiles and amphibians that have been shown to be fascinating groups to study ageing (Reinke et al., 2022), in that, unlike most hexapods, they are iteroparous indeterminate growers: they continue to mould and grow up to an asymptotic size after reproductive maturity and they reproduce multiple times during their lifespan (Briti, 1951). Moreover, their fecundity usually increases with size. In F. candida, the size of the first clutch increases linearly with the body weight at maturity (Stam et al., 1996). In several species of Collembola, including F. candida, fecundity increases right after maturation as the adults become old and continue to grow but not after their asymptotic size is reached (Gregoire-Wibo, 1974; Grimnes and Snider, 1981; Booth, 1983; Snider, 1983). We report in Figure S1, the detailed relationship between clutch size and adult body length which shows that body size determines the clutch size in F. candida, at least during their lives as young adults. F. candida is thus a good candidate species for expressing slow or negligible senescence given that it is known that negligible or negative senescence can evolve in species that continue to grow after maturity and that gain reproductive capacity as they become older and thus also larger (Vaupel et al., 2004; Baudisch, 2008).
Below, we list the questions that we will address in this work. First, does this species suffer from actuarial and reproductive senescence or can it benefit from negligible senescence as one might predict? We will describe the shape of the long-term age trajectories of mortality and several components of reproduction, namely clutch size, inter-clutch intervals, egg size and proportion of sterile eggs. Second, to what extent is the senescence of the different components of fitness synchronized? To address this question, we will compare the timing of actuarial and reproductive senescence and the timing of senescence of the different traits that make up reproduction. Third, what is the degree of plasticity of these age trajectories? By following the life history trajectory of isolated springtails either fed without limitation or dietary restricted, we intend to describe and compare the environmentally induced malleability of the age trajectories of mortality and reproduction. More specifically we wish to verify the expected protective effect of dietary restriction on longevity and mortality and explore the potential effects of food deprivation on how various components of reproduction may change with age. Finally, how genetically variable are these age trajectories? We will compare several genetically distinct lineages of Collembola to explore if and how the age trajectories of mortality and reproduction and the aforementioned issues can genetically vary within a species.
We used, as a model organism, the Collembola Folsomia candida, which is a ~ 2mm long ametabolous unpigmented hexapod inhabiting in the wild microhabitats like moist layers of soil, decaying wood, leaf litter, edges of streams, and underground environments such as caves or mines (Fountain and Hopkin, 2005). The asymptotic body length (2 to ~2.5mm) can be reached in about two months at 15-16°C (Booth, 1983; Mallard et al., 2015; Mallard et al., 2019). Populations are composed of females who reproduce parthenogenetically and can form aggregations in the soil and in cultures (Usher and Hider, 1975). Population density can vary a lot in the wild between sites and over the seasons (Krivtsov et al., 2006). Also often absent or elusive, this species is sometime relatively abundant as in some caves (Alonso et al., 2019) and can even become dominant, reaching average densities of more than 500 individuals per 100g of dry forest litter (Krivtsov et al., 2003; Krivtsov et al., 2006). Being parthenogenetic, isolated individuals produce clonal populations that can be maintained relatively easily in the laboratory. The clonal lineages collected and kept in our laboratory come from two genetically distinct clades (Tully et al., 2006; Tully and Ferrière, 2008; Tully and Potapov, 2015) with contrasting life histories. We have reported large difference on several life-history traits among the two clades (called clade A and B). Collembola from clade B have on average a higher fecundity than those from clade A but also a higher mortality and shorter lifespan (Tully and Ferrière, 2008; Tully and Lambert, 2011). Collembola of clade B generally reach an asymptotic size smaller than those of clade A (Mallard et al., 2015; Mallard et al., 2019) which suggests the existence of genetic compensation between reproduction, growth and maintenance. Within each clade, the different lineages are more similar in terms of the studied life history traits, although some diversity still exists (Tully and Ferrière, 2008; Tully and Lambert, 2011). This springtail can reach a long lifespan (up to more than one year) in the protected laboratory conditions where mortality is low (Tully and Lambert, 2011; Mallard et al., 2015). We found that two strains from the two clades suffer from actuarial and reproductive senescence, but with especially contrasted patterns of age-dependent survival (Mallard et al., 2015).
We studied and compared eleven isofemale clonal lineages of F. candida issued from the two above-mentioned phylogenetic distinct clades, labelled ‘clade A’ and ‘clade B’. Clade A is composed of five lineages called “AP”, “BR”, “BV”, “GB”, “HA”, and clade B of six, called “DK”, “GM”, “PB”, “TO”, “US” and “WI”. The letters that designate these strains come from the initial sites of origin of these strains or from the people who collected them (Tully et al., 2006). These lineages come from eleven distinct geographical origins in Europe (France, Germany, The Netherland, Great Britan) and North America (Michigan, Wisconsin), with no clear phylogeographical pattern (Tully et al., 2006). Most of the reported genetic, life-history and morphological diversity was between the two clades (Tully et al., 2006; Tully and Ferrière, 2008; Tully and Potapov, 2015). For each of the eleven strains, we isolated 20 individuals right after birth. To avoid confounding “strains” effect with “culture population” or “clutch” effects, these individuals originated from at least four clutches coming from different replicates of stock populations that have been maintained in incubators at 21°C and 100% relative humidity for several months. The 220 individuals were kept isolated in rearing containers (52 mm diameter and 65 mm high polyethylene vials filled with a 30 mm layer of plaster of Paris prepared with water mixed with 600 mL of Pébéo graphic Indian ink to darken it) and raised and followed up until death. They were kept in similar conditions during their whole lifespan (alone, 21°C, 100% relative humidity) except for food provisioning: for each strain, 10 individuals were maintained in high food conditions (food was provided ad libitum) and the 10 others in low food conditions. Food was provided as a small pellet (5uL) of a mixture of agar-agar and dried baker’s yeast in a standardized concentration and volume (5000 mL water+80 mg agar+800 mg dried yeast, to produce pellets of 2 mL). In the low food condition, we applied an intermittent fasting regime by providing food one day per week, followed by six days of fasting. This was done for practical reasons because, given the Collembola size, it was not possible to control the amount of food eaten by varying the pellet size or concentration of yeast. Intermittent fasting was also chosen for biological reasons given that regular periods of starvation are normal for many invertebrates (Fontana and Partridge, 2015).
Rearing containers were visually inspected regularly for clutches. This monitoring allowed us to know when each female laid a new clutch, and thus to know the number of clutches laid by each individual, and to measure the interval between successive clutches.
For each of the 1347 clutches laid during this experiment, clutch size was measured by counting the eggs by eye under a dissecting microscope. We photographed about one third of the clutches (randomly selected) to measure the size of the eggs using a digital camera fixed on a dissecting microscope. More precisely we measured the diameter of 9834 eggs from 583 clutches, a couple of days after oviposition, before their chorion ruptured (Marshall and Kevan, 1962). We counted the number of sterile eggs (eggs that fail to hatch and whose chorion has not ruptured) in 404 randomly chosen clutches. Clutches were removed from the containers before egg hatching to keep females isolated during their whole lifespan. Digital pictures were taken weekly or so to measure the female length and track their growth trajectory until death. Body length was measured from the front of the head to the rear of the abdomen. Repeatability of the egg size and body length was measured in an independent experiment and reached 79% for egg size and 96% for body length (Tully and Ferrière, 2008).
During the 571 days of the experiment - the time it took for all the individuals to die - 8 individuals were lost or died accidentally due to improper handling, while the 212 others died ‘naturally’.
We used the data collected to study the effect of age, food regime (ad libitum versus dietary restriction) and to search for genetic and environmentally induced variability on the following life-history traits: lifespan and survival rates, clutch size, inter-clutch intervals, egg size and proportion of sterile eggs.
In the analysis, we systematically compared the four groups of Collembola made by the two clades raised under the two food regimes (clade A & B * ad libitum versus dietary restriction) and we used the same four colours to identify these groups in the different figures. Additionally, we report, for some traits, the variability among individuals and among clones within each clade. These graphs underline not only the mean age trajectories estimated at the level of a group of individuals but also the age trajectories measured at the level of each individual.
The analyses have been made with R (The R Development Core Team, 2021) and all the figures have been produced with the tools from the library ggplot2 (Wickham, 2009) and visreg (Breheny and Burchett, 2017).
Analysing the complex non-linear age trajectories is not straightforward. We choose to fit models with non-linear smoothers, such as generalized additive models (gam), to describe the shapes of the life history age trajectories because these models do not need to specify a priori a specific shape that would rely on a linear effect of age on the studied traits. Comparing statistically the life-history age trajectories among the different groups is tricky given the potential complexity of the shapes of these trajectories and given the structure of the measurements (eggs nested within clutches, clutches within individuals, individuals within clones, clones within clades and clades within food regime…). Unfortunately using a single generalized additive mixed model (gamm4) to model our data, and thus statistically test for differences among clades or clones and treatments while taking into account different smoothed effects of age for each group of individuals and the random effects (multiple measurements per individual) was not possible due to convergence problems. Thus, for most traits, generalized additive models (gam from mgcv library) were used to fit a smooth trajectory on each group of individuals (clades*food regimes) independently and the predicted mean trajectories were plotted to reveal the mean shapes of the various age trajectories with minimal constraints (Wood, 2017). Their associated confidence bands can be used to determine by eye whether the trajectories differ, and if so, over which age range the divergences are most marked (Cumming, 2009). For some traits, we grouped the data collected by periods of 14 days (fortnights) or of longer periods and used generalized linear mixed models (lmer from lme4 library) to estimate the mean and 95% confidence intervals of each of the four groups of individuals (clades*food) within each fortnight period. These models take into account, in their random parts, the structure of the data (eggs nested within clutches, clutches within individuals, individuals within clonal lineages) and thus provide reliable unbiased predictors. As for gams, the confidence intervals associated with the fortnights’ means can be used to visually compare and discuss the differences between groups of these age trajectories. The results of the main statistical models selected to support our analyses are presented in detail the tables and in the Supplementary Materials.
We used Kaplan-Meyer survival curves – computed and plotted with the R survival (Therneau and Grambsch, 2000) and ggsurvplot libraries (Kassambara et al., 2021) – to compare visually and statistically (with log-rank tests) the lifespan distribution among food regimes for each clone (Figure 1) or among the four groups of clade and food regimes (Figure 2). We also used Cox proportional hazard models (coxph function form survival library) to estimate and compare the median lifespans and hazard ratios (Table 1). The hazard functions h(t), which describe the risk of mortality per unit of time, have been estimated for each clade and food regime with the bshazard library (Rebora et al., 2018) which enables to produce nonparametric smoothed estimates of the effect of age on the instantaneous mortality rates (Figures 3, S4). We were constrained to group together the clones within each clade to have a sufficiently large number of longevities to estimate the hazard functions, after verifying that the survival curves of each clone did not vary much within each clade and food regime (Figure S2).
Figure 1 Survival curves and their associated 95% confidence intervals are plotted for each clone in the two food regimes grouped by clades (first two rows for clade A, rows 3 and 4 for clade B, 10 individuals per group of clone and food regime). For each clone, differences between food regimes are assessed with log-rank tests (printed p-values). While every clone from clade A significantly extend their lifespan when dietary restricted, the difference between the two food regimes was less pronounced or even not present in the clones from clade B.
Figure 2 Survival curves and their associated 95% confidence intervals are plotted for the two clades and two food regimes with the p-value from the global log-rank test. Specific contrasts show that the survival curves significantly vary between the two food regimes for clade A (χ21 = 90, p<0.001) and also, to a lesser extent, for clade B (χ21 = 17, p<0.001). 100 individuals have been followed-up for each food regime for clade A and 120 collembola for clade B.
Figure 3 The estimated age trajectories and their associated 95% confidence intervals of daily death rate (solid lines) and daily risk of starting a reproductive decline (clutch size, dotted lines) for the four groups of Collembola (two clades under the two food regimes).
For reproduction, we measured and analysed the following traits: clutch size (number of eggs per clutch), inter-clutch interval (number of days between two successive clutches), egg size (diameter) and proportion of sterile eggs for the clutches for which these two later traits have been measured.
The individual age trajectories of cumulative fecundity and clutch size are plotted in Figure 4. The mean clutch size trajectories of the four groups of Collembola (two clades * two food treatments) have been estimated using generalized additive models (gam) fitted for each of these four groups while imposing the same dimension of the basis used to represent smooth terms (k=5, Figure 5). The same value of k was used in the different models to ensure that the predicted smoothed effects of age were comparable among groups of individuals. A value of 5 was found to be sufficiently high to capture long-term non-linear effects of age of the trait’s trajectories. In a second analysis, we studied the individual clutch size age trajectories by fitting a gam (with a k=5 as well) for each individual that died from a natural cause and that laid five clutches or more. From these fits we estimated the onset of reproductive senescence as the age at which the predictions from the gam reached its maximum value (Figure S5). We considered these ages as estimates of ‘onset of reproductive senescence’ (Figure S6), and used them to build up some survival-like curves for the initiation of reproductive senescence (Figure S7) and, as for the survival analysis detailed above, we estimated hazard functions for the four groups of Collembola to illustrate how the risk of starting clutch size decline changes with increasing age. These ‘reproductive hazard functions’ have been plotted alongside those of death rates to facilitate visual comparison of the timing and rate of the actuarial and reproductive senescence (Figure 3, dotted lines). We also used the individual fecundity trajectories to estimate the ‘rate of reproductive senescence’ as the mean slope of the decline in clutch size after the onset of reproductive senescence. We then used these estimates to look for differences in the rate of reproductive senescence among clones, clades and treatments (Figure S8). Note that what we call here for simplicity ‘reproductive senescence’ is in fact a fecundity senescence since it ignores the other dimensions of reproduction analysed below.
Figure 4 Clutch size age trajectories and cumulative fecundity. The panel (A) displays the individual clutch size age trajectories for each clone and food regimes together with smoothed curves to underline the mean effects of age on clutch size. The panel (B) represents the individual trajectories of cumulative fecundity. Each circle on the figure represents an egg-laying event, except for the last circles at the end of each trajectory, which represents the death of the individuals. In each panel the clones from the first two rows belong to clade A and the clones from the rows 3 and 4 belong to clade B.
Figure 5 Mean clutch size age trajectories for each clade in the two food regimes. The small pale circles represent the raw data. The large circles and associated 95% confidence intervals are the fortnight’s means estimated from a linear mixed model with individuals nested within clones as random factors. Smoothed trajectories and their 95% confidence bands estimated from four independent generalized additive models have been added to underline the non-linear shapes of the mean fecundity trajectories.
Reproductive senescence can affect not only the fecundity (clutch size) but also the rate at which a female reproduces. To study this aspect, we analysed if and how the time between two consecutive clutches varies with age (Figure 6). As for clutch size, we unveil the effects of age on inter-clutch intervals using generalised additive model (Table 2, Model 4). We also used a generalized linear mixed model with clone and individuals as random factor to estimate fortnights and monthly estimates and their associated 95% confidence intervals (Figure 7).
Figure 6 The age trajectories of the inter-clutch intervals (number of days between two consecutive events of egg laying) are plotted for each individual (thin lines, individual trajectories). The thick curves represent the mean smoothed trajectories for each clone and food regime. Note that, to better reveal the details, the data have been plotted on logarithmic scales both for the x- and y-axis. This graph reveals that on average the beginning of reproduction is delayed under dietary restriction and the pace of reproduction is also reduced when food is scarce. This figure also shows the progressive increase in the inter-clutch intervals as the springtails become older.
Figure 7 This graph displays the mean smoothed age trajectories (estimated with gams) for each combination of clade and food regimes over the raw measurements (pale circles). We also added the means and 95%CI of the inter-clutch intervals over fortnights (between 0 and 100 days), and over one month (between 100 and 300 days) or two months for measurements made after 300 days. We used a generalized mixed model with individuals and clones as random effects to estimate these means (large circle). Note that to increase the readability, we have used logarithmic scales for the x- and y-axis.
The smoothed effects of age on egg size (diameter) were plotted for each clone (Figure 8) and compared among clades and food regimes using gam fits (Figure 9) and linear mixed models (Table 3). As for interclutch intervals, we used a generalized mixed linear model (Gaussian) that took into account the structure of the dataset (eggs nested with clutches, nested within individuals) to estimate the mean egg diameter (and 95% confidence intervals) by fortnight periods in the four groups of clade*food regime (Figure 9). Similarly, we used generalized linear mixed models (binomial) to estimate the mean proportions of sterile eggs by fortnight periods in the four groups of individuals. For both traits, these models were fitted to the four groups of individuals only, because these traits were measured solely on a subset of the clutches which was not large enough to enable relevant comparison among clones (Figure 10).
Figure 8 Egg size (diameter, mm) as a function of springtail’s age. This figure displays the row measurements made on various clutches produced by different Collembola raised under the two food regimes (pale circles). The data are sorted by clade and clones within each clade. A smoothed curve is adjusted to each combination of clone and food regime.
Figure 9 Egg size (diameter, mm) as a function of age. This figure represents the trend estimated for the two clades in the two food regimes and the mean (and 95% confidence interval) egg size estimated per fortnight for each of the four groups of individuals. These estimates come from a linear mixed model with clutches, individuals and clones as nested random factors.
Figure 10 The proportion of sterile eggs has been measured for 404 clutches (small circles). The mean and 95% CI of the proportion of sterile eggs has been estimated every fortnight for the four groups of Collembola (large filled circles and error bars) with a binomial linear mixed model with clutches, individuals and clones as randoms effects.
Lifespan ranged from two weeks (17 days) to a maximum of one and a half year (571 days, Figure 1). We observed a high genetic variability of lifespan among the clones (Figure 1) and of its plastic response to food provisioning (statistically significant clone*food regime interaction, χ210 = 43.4, p<0.001, Table 1, Model 1): when grown under ad libitum food conditions, median lifespan varied from 42 days (GM, clade B) to 115 days (GB, clade A) and under caloric restriction, from 67 (DK, B) to 341 days (BR, A). For clade A, the five clones responded similarly to caloric restriction by a remarkable increase of the mean lifespan (x 2.82 on average among the clones, Figure 1 and Table 1). For clade B, the effect of caloric restriction was less pronounced (x 1.96 on average, nonetheless, for the clones GM, PB, WI) or even negligible for three clones (DK, TO, US, Figure 1 and Table 1).
By grouping the clones within each clade, we found that (1) in both environments, the clade A had on average a lower mortality and thus a higher longevity than clade B (Figures 2, 3 and Table 1) and (2) that on average, the magnitude of the positive effect of food deprivation on lifespan varied dramatically among the two clades: the amplitude of the right shift of the survival curves under dietary restriction is remarkably singular for clade A whose mean longevity almost tripled under dietary restriction (Figure 2). This genetic*environment interaction (Table 1, Model 2, χ21 = 25.6, p<0.001) is also reflected in the shapes of the mortality trajectories (Figure 3, solid lines): based on the visual comparison of these trajectories and of the overlap of their 95% confidence bands (see Figure S4), under each food regime, individuals from clade B suffered on average from a higher initial basal mortality rate (before ~50 days), an earlier onset of senescence and a tend to suffer from a steeper increase of mortality with advancing age. Clade A is also remarkable by its low mortality and almost negligible actuarial senescence under dietary restriction. Under ad libitum food regime, the onset of senescence – which is not easy to quantitatively determine - seems also delayed compared to clade B and the increase in mortality rate was not only slow, but also visually seemed to reach a mortality plateau (green trajectory, Figures 3, S4).
Clutch size ranged from a minimum of one egg to a maximum of 178 eggs per clutch with a mean of 39 and a median of 26 eggs (Figure 4A) and the maximum number of eggs laid during a whole lifespan reached 1298 (Figure 4B). Not surprisingly, we found a strong effect of food regime on clutch size in every clone (Figure 4A) and clade (Figure 5). Over the whole lifespan, the mean clutch size under ad libitum food regime was 73 eggs, and 72% of the laid clutches were larger than 50 eggs. As expected, under dietary restricted conditions, the clutches remained much smaller (20 eggs on average, 95% of the clutches having fewer than 50 eggs). Both age and food regime influenced the clutch size: the first clutch laid under dietary restriction was on average half the size (11 eggs) of the ones laid in ad libitum food conditions (22 eggs). This strong effect of food regime on fecundity amplified as the Collembola became older (Figure 5) and larger (Figure S1).
Under ad libitum food regime, the mean fecundity progressively increased up to reaching a maximum after about 40 or 50 days (Figure 5). Following this productive period, the fecundity of the Collembola still alive begins to decline then eventually becomes null, a certain number of springtails managing to survive long after laying their last clutch (horizontal ends of cumulative fecundity trajectories visible on Figure 4B and analysed in detail in a previous paper (Tully and Lambert, 2011)). It a nutshell, we found that most clutch size age trajectories were bell-shaped: an initial increase, followed by a narrow maximum and a progressive decline. The mean trajectories adjusted for the four groups of Collembola (two clades * two food regimes, Figure 5) brings out the higher fecundity (and higher lifetime fecundity) of clade B compared to clade A, especially under the ad libitum food regime (Table 2, Model 3). Figure 5 shows not only the positive effect of ad libitum food on fecundity in both clades but also the delayed and slower fecundity decline under dietary restricted conditions. One can also remark that, as for mortality, the negative effect of age was barely noticeable under dietary restricted regime for clade A (negligible senescence).
To better compare the age distribution of the onset of clutch size senescence with the shape of the mortality age trajectories, we produced some “mortality like trajectories” of the daily risk of starting a reproductive decline using the individual’s estimates of the onset of clutch size senescence (Figures S5–S7). We found that the shape of the “age trajectories of the risk of starting a reproductive decline” varied among clades and food regimes in a manner that echoed the observed variations among the mortality trajectories (Figure 3, dotted versus solid lines). In clade A, under dietary restricted conditions, as for mortality, the risk of starting a reproductive decline remained low with a negligible effect of age. For the three other groups of Collembola, the hazard rate for clutch size senescence started to increase earlier and at a higher rate than for mortality. Interestingly, the relative order between the different trajectories were maintained: in both clades, the hazard rates started to increase with age earlier and faster under ad libitum conditions for both mortality and fecundity.
Once reproductive senescence had started, the clutch sizes declined on average at a higher rate in fully fed springtails than in dietary restricted ones (Figure S8).
The intervals between successive clutches ranged from at least half a week (3.5 days) to over five months (172 days for an old GB female). Well-fed females had on average a higher spawning frequency than females on diet, with a median value of about a week in high food conditions and 12 days in low food conditions. This effect of food regime is visible for each clone especially during the first two months (Figure 6). The gam revealed that, for each lineage and each food regime, the egg-laying frequency tended to slow down with age (Figure 7 and Table 2, Model 4). The pace of this slowdown appears to be somehow irregular: during the first part of their reproductive life, most adults managed to maintain a more or less regular or barely slowing spawning rate, but after about 75 days for well-fed individuals the mean interval between successive clutches started to increase markedly (breaks in slope visible in the gam of Figure 7). Such discontinuity was less visible for dietary restricted individuals whose spawning rate decreases more gradually with age.
As time goes on, the speed of this increase became typically higher in well-fed individuals such that for several clones and for the two clades on average, after a certain age (~80-100 days), the rate of clutch production of the well-fed individuals became lower than that of their same-age dietary restricted congenerics (cf. intersecting smoothed curves in Figures 6, 7). The mean effect of age on inter-clutch intervals appeared to be roughly similar between the two clades (Figure 7) despite some marked differences between some clonal lineages (Figure 6).
Egg diameter was on average 146 μm and ranged between 2 and 189 μm. Despite some marked variation in egg size between and within clutches (Figure 8), and contrary to the other studied traits, the visual inspection of the smoothed age trajectories among clades (Figure 9) indicates that Collembola managed to maintain a relatively constant egg size during their whole lifespan even if a closer look suggests that Collembola age can have some marginal effects on egg size under some conditions.
Given the structure of the data (eggs nested within clutches, clutches within individuals and individuals within clones) and the relatively flat or linear effect of age revealed by the gam models, we used linear mixed models to study if age had indeed a detectable negative effect on egg size. We found evidence of interactions between age and clade (p=0.026), and between age and food regimes (p=0.004, see Table 3, Model 5 & Model 6). The study of sub-models (Table 3, Models 7-11) revealed that the mean size of eggs laid by well-fed springtails was slightly larger in clade B (p<0.001) but did not vary with increasing age (p=0.13, negligible senescence, Model 8). It is only in the long-lived dietary restricted clade A that we found a decline of egg size with increasing age (p<0.001, Model 10 & Figure 9). This effect, especially due to some very old individuals, was, however, quantitatively modest: the mean diameter of the eggs produced by Collembola older than 180 days was indeed only 6% smaller than those laid by adults younger than 80 days old (Figure 9).
On average, over the whole lifespan, about 6% of the eggs were sterile. This proportion varied among clutches and ranged between 0% (for 40% of the clutches) and up to 100% for a few of them. Clade B produced on average 2.5% of sterile eggs while this proportion was seven times higher in clade A on average (17.5%). The proportion of sterile eggs changed with age but differently depending on the clade and food regime (significant age*food*clade interaction, p=0.002, Table S1). For clade A, the proportion of sterile eggs increased with age but at a slower rate in the dietary restriction regime. For Clade B, the effect of age also varied among treatments: old Collembola produced on average more sterile eggs when fed ad libitum while the proportion of sterile eggs dropped to almost zero in old dietary restricted springtails (Figure 10, Table S2).
Using our unique longitudinal dataset, we were first able to show that different strains of F. candida can have contrasting lifespans, as has been shown in wild populations of various species like the fruit fly Drosophila melanogaster (Grandison et al., 2009), the rotifer Brachionus (Gribble et al., 2018), or the nematod Caenorhabditis elegans (Chen et al., 2001). We also found that Collembola can suffer from actuarial senescence and that the onset and the rate of senescence were both, at the same time, phenotypically plastic and genetically variable. This echoes previous research where we found that, in protected laboratory conditions (16°C, constant density), mortality rate of F. candida can increase exponentially with age and that different components of the shape of the mortality trajectories can vary among genetically distinct lineages (Mallard et al., 2015). Even if the conditions varied between the two experiments, it is interesting to notice that the clonal lineage that was able to delay remarkably the onset of actuarial senescence in this former publication belongs to clade A which is the one that also had here a delayed onset and slower ageing rate. Thus, this shows that the qualitative difference observed between the two clades can remain in different environmental and demographic contexts.
As expected from a very large number of empirical studies (Kirkwood and Shanley, 2005; Piper et al., 2011), including this Collembola (Tully and Lambert, 2011), we found that in dietary restricted conditions, our springtails managed to extend their longevity. This can be achieved either by reducing the short-term mortality rate (Mair et al., 2003; Mair et al., 2005), by delaying the onset of ageing or of associated health disorders (Mattison et al., 2012) or by slowing the rate of ageing (Merry, 2005).
Contrary to what has been observed in Drosophila melanogaster (Partridge et al., 2005) or spider mites for instance (Li and Zhang, 2021), where dietary restriction lowers the risk of death but affects only marginally the rate of ageing itself, dietary restricted Collembola achieved lifespan extension by maintaining a low basal adult mortality rate and by setting back the onset of actuarial senescence and slowing its rate. This plasticity, especially remarkable in one clade (A), enabled the springtails not only to double or almost triple their life expectancy but also to change the qualitative pattern of senescence: a change in diet can trigger a shift from strong negative actuarial senescence to negligible senescence. Such dramatic plastic changes resemble the ones that we described in the meadow vipers where, depending on the microhabitats, ageing vipers could either suffer from severe decline of survival or benefit from negligible senescence (Tully et al., 2020). These similarities among such distant species imply that this level of age-trajectory plasticity may be more common than one could imagine. More empirical studies in the wild and in the laboratory are required to assess how common are these plastic changes in the shape of the mortality age trajectory. If found to be widespread, this plasticity may call into question the generality of conclusions drawn from comparative studies that do not consider the possibility of a crucial role of environmental conditions on the shape of the trajectories studied.
This plasticity could also be linked to two common properties of these species, namely continuous growth and a fertility that increases with adult size, given that these features are linked to the evolution of negligible senescence (Vaupel et al., 2004; Baudisch, 2008; Baudisch and Vaupel, 2010). In other words, it can be especially advantageous to be able to survive long periods of fasting if you are able, as an adult, to resume growth then benefit from an especially high fecundity once better days have come. It would be worth exploring the Collembola’s responses after a return to an ad libitum diet succeeding a period of fasting to better assess if dietary restricted induced lifespan extension coupled with slackening reproduction is indeed an adaptive response (Sultanova et al., 2021).
Regarding reproduction, nothing unexpected in the fact that undernourished Collembola lay smaller clutches and less often. The novelty of our work is rather to quantify effect of dietary restriction on the timing and pace of reproductive decline on several traits simultaneously that we discuss in more details below.
Our study offers a unique opportunity to describe and compare how chronological age alters various dimensions of reproduction. Apart from egg size, all reproductive traits declined markedly with increasing age: ageing springtails on the wane lay smaller clutches, less often, and with on average a larger proportion of sterile eggs. This shows that senescence can touch nearly all the dimensions of reproduction, with non-linear effects and some abrupt accelerations.
We also found notable differences between clones and clades for all the traits analysed, which shows that several parameters of reproductive ageing still possess the potential to evolve. The comparison of the two clades also discloses a potential link between reproduction and survival: the clade that suffers from an earlier and more intense actuarial senescence is also the one with the highest reproductive potential (as also discussed in (Tully and Ferrière, 2008)) which supports the idea that a trade-off between somatic maintenance and reproduction may be at the heart of the ageing process, following the logic of the disposable soma theory (Kirkwood and Austad, 2000; Kirkwood, 2002).
We also found an odd difference between the clades, namely in the proportion of sterile eggs. The more fertile clade was the one with the lowest level of sterile eggs even at old ages. Given the implication of the endosymbiont Wolbachia in this species’ reproduction and parthenogenesis (Frati et al., 2004), one could speculate that part of the difference observed between the clones could be linked to differences in Wolbachia’s loads, given that it has been shown that Wolbachia are essential for embryonic development and egg hatching in this species (Pike and Kingcombe, 2009; Timmermans and Ellers, 2009; Hafer and Pike, 2010). Our results also encourage exploring the potential effects of ageing on Wolbachia’s load and, in turn, the potential effects of Wolbachia’s load on the age trajectories, as is the case in Drosophila melanogaster, where the presence of this endosymbiont increases lifespan by delaying the onset of actuarial senescence (Alexandrov et al., 2007).
As expected, we found that dietary restriction reduced the reproductive output: poorly fed Collembola lay smaller clutches and at a slower pace. We also found that dietary restriction had, as for survival, a protective effect on the reproductive ageing trajectory: the onset of reproductive senescence was delayed and the reproductive decline when it has started was more gradual. Such protective effect has been observed in a mite (Li and Zhang, 2021) where dietary restriction delayed the onset of reproductive decline. As for survival, this protective effect was large enough in one clade - clade A - to get rid of almost any negative effect of age on fecundity. The slow rate of decline in fertility in starving Collembola (Figure S8) could also be determined by a protective effect of dietary restriction, but one cannot rule out the fact that this could also be a simple statistical effect: the fall being all the faster the higher you start.
The joint study of reproduction and survival enabled us to compare the timing of senescence of various traits. We provide another empirical evidence of the asynchrony of senescence (Cayuela et al., 2020b): the onset of senescence varied between traits and among genetic lineages and environmental backgrounds.
Behind this diversity, we found some regularity in the timing of senescence among different traits. Negligible mortality senescence was associated with a marginal decline in fecundity, a low and roughly constant risk of starting a reproductive decline and a slow and progressive slowdown of the rhythm of reproduction, with no jolt. In this case the pace of ageing was faint or barely noticeable for every trait analysed. We did not observe a combination of marked actuarial senescence with negligible reproductive senescence as has been observed in snakes for instance (Cayuela et al., 2020b; Tully et al., 2020). Whenever senescence was tangible, we found that clutch size senescence was followed closely by a marked increase in the inter-clutch intervals and the senescence of these two components of reproduction precede the observed rises in mortality rates. It is as if, after reaching a reproductive peak, the Collembola became reproductively exhausted but were still able to postpone their death of old age until they could capitalize on their whole reproductive lifespan. This is indeed coherent with our previous finding of a remarkably long post-reproductive lifespan (Tully and Lambert, 2011), and the selective forces that may have acted to select for such a gap between the end of reproductive life and the end of somatic life can be called upon to explain the systematic shift observed between reproductive and actuarial senescence. This may also explain similar observations in other species (Reznick et al., 2006; Auld, 2018; Nielsen et al., 2021).
Contrary to all the other traits examined, we found negligible senescence on egg size in the two clades and two food regimes. Similarly, no negative effect of old age was found on mean egg size in quails (Vedder et al., 2022), Turtles (Congdon et al., 2003), bugs (Kasule, 1991) or on neonate size in the meadow viper (Tully et al., 2020) while a negative effect of age on egg volume has been reported in a long-lived sea bird (Beamonte-Barrientos et al., 2010). This later effect was, however, quantitatively marginal and inconsequential on hatching success. A recent study on polar bears also found no senescence of the maternal effects: on the contrary, old females produced cubs whose survival tended to be higher from that of congeners born form younger and fitter - but probably also less experienced – females (Naciri et al., 2022).
These various evidences support the idea that some components of reproduction are less susceptible to decline with age than others. Egg size is highly variable in this species (Stam et al., 1996) and we have shown that juveniles hatched from large eggs survive better than those from small eggs in conditions where food is scarce (Tully and Ferrière, 2008). Given its fundamental role in determining the offspring chance to survive to adulthood, egg size - or size at birth - is under severe selective pressure, especially if there is a certain threshold size below which development or survival becomes abruptly impossible. Such strong selection could explain why egg size, in our Collembola and in the above-mentioned empirical examples, appears to be so canalized, i.e. phenotypically consistent, in face of increasing mothers’ age, despite some potential plasticity or flexibility in egg size (Liefting and Ellers, 2008; Tully and Ferrière, 2008; Liefting et al., 2010). Natural selection has likely favoured individuals who manage to maintain egg size above a critical lethal threshold even at advanced ages, when other components of reproduction whose lessening is not as critical for female fitness have already progressively declined with age. Such difference in the shape of the functions linking a trait’s value to an individual’s fitness could help understand the evolutionary origin of the observed diversity and asynchrony in senescence’s pathways.
We still have to reconcile our present finding with the previous observation that the Collembola reproductive decline was subsequent to the onset of actuarial senescence (Mallard et al., 2015). In the latter case, individuals were kept and maintained at a colder temperature and by groups of 10, rather than isolated. Low temperature and intraspecific competition may have created conditions favourable for delaying the onset of reproductive ageing. Following groups of individuals prevented a close follow-up and quantification of the onset of reproductive ageing based on individual trajectories as we have done here. Conversely, Mallard et al. used many more Collembola that in this study which let them describe with a fine resolution the shape of the mortality trajectory which probably helps detect earlier onsets. This also underlines that determining the onset of senescence can be methodologically challenging, and may be biased by the quality of the data collected. More experimental and theoretical works are also required to help identify if some environmental conditions could lead to reversals in the relative order of ageing of different traits.
We would finally like to pay particular attention to the method that we used to compare the senescence of mortality and reproduction. Describing and comparing the shapes of the age trajectories of reproduction is always informative, but deriving from these trajectories an estimation of the hazard rate of becoming reproductively senescent uncovers a new telltale signature of the process of reproductive senescence. The advantage of this method is that reproductive hazard rate trajectories can be directly compared to the death rate trajectories, and they even share the same units. It could be interesting to test this novel approach on other empirical or theoretical examples to assess its usefulness in ageing research.
A strong point of this study is undoubtedly the fact that we carried out individual monitoring, which eliminates all the problems of density that can covary with age during cohort monitoring. Our individual monitoring also enabled us to detect the effects of age on the various reproductive traits in a robust and unbiased manner. However, this is at the expense of the number of individuals monitored. For logistical reasons, we were only able to monitor 10 individuals per clone and treatment, which is low, particularly for estimating survival curves. These small numbers may be at the origin of the stochasticity observed in the patterns of survival curves in Figure 1. Inter-clone comparisons, particularly for survival, are therefore undoubtedly fragile because of this limitation. On the other hand, inter-clade comparisons, which allow several clones to be grouped together, are based on a hundred or so individuals, which makes it possible to obtain more robust results.
To stay as close as possible to the traits analysed and avoid making the analyses too complex, we ignored here the covarying change of springtail body length with age. Collembola, like snakes, start to reproduce largely before reaching their asymptotic size, and they continue to mould and grow during their whole life. Ignoring the confounding effects of body length with age, in such indeterminate growers whose fecundity is proportional to size (as can be seen in Figure S1 for our springtail), can mask some potential negative effects of age, and this could lead to the questionable conclusion that there is no senescence (Sparkman et al., 2007; Tully et al., 2020). In the present case, we found marked signs of senescence despite this potential bias, and negligible senescence when observed, was revealed largely over a period during which the Collembola stopped growing. This supports the idea that we provide conservative results, although considering the effect of size could lead to the detection of slightly earlier onsets of reproductive senescence.
We have explored the shapes of the age trajectories by grouping together clonal lineages belonging to the two phylogenetically distinct clades. This does not mean that within each clade the clonal lineages are perfectly similar. Indeed, although reduced, there is a certain level of genetic diversity within each clade. Although the number of replicates per clone is low to quantify precisely potential differences among clades, one cannot rule out the fact that part of the senescence phenotypes that we describe here could result from among-clones differences. For instance, the observed increase over time of the proportion of sterile eggs in some conditions can result either from a phenotypic change that impacts potentially all the individuals, or from an effect of selective mortality - or of reduction in fecundity - that could affect predominantly the lineages that have intrinsically a lower level of sterile eggs. More experiments, with a higher number of replicates per clonal lineages, could help ascertain what is due to genetic or to non-genetic variance.
A fourth limitation is that we did not explore the potential links and correlations between the different traits examined here. Yet, the fact the different traits have been measured on isolated individuals make it feasible to search for genetic, plastic, or physiological compensations between pairs of traits like clutch size and inter-clutch intervals, clutch size and egg size. One could also study if and how such potential physiological trade-off vary while the springtails become older. Other classical trade-offs between longevity (lifespan, onset or rate of senescence) and reproduction (reproductive output, or early reproduction) could have been studied. Finally, it could also be worth exploring if the onset and the rate of reproductive senescence are linked to each other or if these two later traits are linked to longevity for instance.
Senescence can also manifest itself through long-term transgenerational effects. The offspring produced by ageing individuals can have a lower fitness than offspring born form younger parents. In the woodlice Armadillidium vulgare for instance, an indeterminate grower and iteroparous invertebrate as Collembola, old females produce larger clutches with larger eggs than young adults, but the offspring that hatches form these eggs had a lower survival rate than those issued from young adults (Depeux et al., 2020). This shows that a comprehensive vision of ageing requires the exploration of potential transgenerational manifestation of senescence. In our study organism, the slight but detectable decline of egg size in some condition could well have such transgenerational consequences, especially given that we have shown that the smaller juveniles that hatch from smaller eggs survive less well in hash environmental conditions (Tully and Ferrière, 2008). It would be interesting to investigate the extent to which age-related maternal effects shape the offspring survival and actuarial senescence (“Lansing effect”), but also their long-term individual reproductive trajectories (reproductive senescence). Such “intergenerational transfer of ageing” could explain part of the non-genetic and non-environmentally induced phenotypic variability that we have observed between individuals of the same clone in the same environment (Monaghan et al., 2020).
The original contributions presented in the study are publicly available. This data can be found here: https://zenodo.org/doi/10.5281/zenodo.10011211.
The manuscript presents research on animals that do not require ethical approval for their study.
The author confirms being the sole contributor of this work and has approved it for publication.
This study was financed by recurrent funding from the French government allocated to Sorbonne University.
The author declares that the research was conducted in the absence of any commercial or financial relationships that could be construed as a potential conflict of interest.
All claims expressed in this article are solely those of the authors and do not necessarily represent those of their affiliated organizations, or those of the publisher, the editors and the reviewers. Any product that may be evaluated in this article, or claim that may be made by its manufacturer, is not guaranteed or endorsed by the publisher.
The Supplementary Material for this article can be found online at: https://www.frontiersin.org/articles/10.3389/fevo.2023.1112045/full#supplementary-material
Alexandrov I. D., Alexandrova M. V., Goryacheva I. I., RoChina N. V., Shaikevich E. V., Zakharov I. A. (2007). Removing endosymbiotic Wolbachia specifically decreases lifespan of females and competitiveness in a laboratory strain of Drosophila melanogaster. Russian J. Genet. 43 (10), 1147–1152. doi: 10.1134/s1022795407100080
Alonso L., Pommier T., Kaufmann B., Dubost A., Chapulliot D., Doré J., et al. (2019). Anthropization level of Lascaux Cave microbiome shown by regional-scale comparisons of pristine and anthropized caves. Mol. Ecol. 28 (14), 3383–3394. doi: 10.1111/mec.15144
Auld J. R. (2018). The effects of diet and mating system on reproductive (and post-reproductive) life span in a freshwater snail. Ecol. Evol. 8 (23), 12260–12270. doi: 10.1002/ece3.4689
Auld J. R., Henkel J. F. (2014). Diet alters delayed selfing, inbreeding depression, and reproductive senescence in a freshwater snail. Ecol. Evol. 4 (14), 2968–2977. doi: 10.1002/ece3.1146
Baudisch A. (2011). The pace and shape of ageing. Methods Ecol. Evol. 2 (4), 375–382. doi: 10.1111/j.2041-210X.2010.00087.x
Baudisch A., Salguero-Gómez R., Jones O. R., Wrycza T., Mbeau-Ache C., Franco M., et al. (2013). The pace and shape of senescence in angiosperms. J. Ecol. 101 (3), 596–606. doi: 10.1111/1365-2745.12084
Baudisch A., Stott I. (2019). A pace and shape perspective on fertility. Methods Ecol. Evol. 10 (11), 1941–1951. doi: 10.1111/2041-210X.13289
Baudisch A., Vaupel J. (2010). Senescence vs. sustenance: Evolutionary-demographic models of aging. Demographic Res. 23, 655–668. doi: 10.4054/DemRes.2010.23.23
Beamonte-Barrientos R., Velando A., Drummond H., Torres R. (2010). Senescence of maternal effects: aging influences egg quality and rearing capacities of a long-lived bird. Am. Nat. 175 (4), 469–480. doi: 10.1086/650726
Booth R. G. (1983). Effects of plaster-charcoal substrate variation on the growth and fecundity of Folsomia candida (Collembola, Isotomidae). Pedobiologia 25, 187–195. doi: 10.1016/S0031-4056(23)05923-1
Breheny P., Burchett W. (2017). Visualization of regression models using visreg. R J. 9 (2), 56–71. doi: 10.32614/RJ-2017-046
Briti N. W. (1951). Observations on the life history of the Collembolan Achorutes armatus. Trans. Am. Microscopical Soc. 70 (2), 119–132. doi: 10.2307/3223425
Cayuela H., Lemaître J. F., Bonnaire E., Pichenot J., Schmidt B. R. (2020a). Population position along the fast-slow life-history continuum predicts intraspecific variation in actuarial senescence. J. Anim. Ecol. 89 (4), 1069–1079. doi: 10.1111/1365-2656.13172
Cayuela H., Lemaître J.-F., Muths E., McCaffery R. M., Frétey T., Le Garff B., et al. (2021). Thermal conditions predict intraspecific variation in senescence rate in frogs and toads. Proc. Natl. Acad. Sci. 118 (49), e2112235118. doi: 10.1073/pnas.2112235118
Cayuela H., Lemaître J.-F., Rugiero L., Capula M., Luiselli L. (2020b). Asynchrony of actuarial and reproductive senescence: a lesson from an indeterminate grower. Biol. J. Linn. Soc. 131 (3), 667–672. doi: 10.1093/biolinnean/blaa127
Chen J., Carey J. R., Ferris H. (2001). Comparative demography of isogenic populations of Caenorhabditis elegans. Exp. Gerontol 36 (3), 431–440. doi: 10.1016/S0531-5565(00)00225-4
Colchero F., Jones O. R., Conde D. A., Hodgson D., Zajitschek F., Schmidt B. R., et al. (2019). The diversity of population responses to environmental change. Ecol. Lett. 22 (2), 342–353. doi: 10.1111/ele.13195
Congdon J. D., Nagle R. D., Kinney O. M., Van R. C. (2003). Testing hypotheses of aging in long-lived painted turtles (Chrysemys picta). Exp. gerontol 38 (7), 765–772. doi: 10.1016/S0531-5565(03)00106-2
Cumming G. (2009). Inference by eye: reading the overlap of independent confidence intervals. Stat. Med. 28 (2), 205–220. doi: 10.1002/sim.3471
Dasgupta P., Halder S., Dari D., Nabeel P., Vajja S. S., Nandy B. (2022). Evolution of a novel female reproductive strategy in Drosophila melanogaster populations subjected to long-term protein restriction. Evolution 76 (8), 1836–1848. doi: 10.1111/evo.14560
Depeux C., Lemaître J. F., Moreau J., Dechaume-Moncharmont F. X., Laverre T., Pauhlac H., et al. (2020). Reproductive senescence and parental effects in an indeterminate grower. J. Evolutionary Biol. 33 (9), 1256–1264. doi: 10.1111/jeb.13667
Fontana L., Partridge L. (2015). Promoting health and longevity through diet: from model organisms to humans. Cell 161 (1), 106–118. doi: 10.1016/j.cell.2015.02.020
Fountain M. T., Hopkin S. P. (2005). Folsomia candida (Collembola): a “standard” soil arthropod. Annu. Rev. Entomol 50, 201–222. doi: 10.1146/annurev.ento.50.071803.130331
Frati F., Negri I., Fanciulli P. P., Pellecchia M., De Paola V., Scali V., et al. (2004). High levels of genetic differentiation between Wolbachia-infected and non-infected populations of Folsomia candida (Collembola, Isotomidae). Pedobiologia 48 (5-6), 461–468. doi: 10.1016/j.pedobi.2004.04.004
Gaillard J. M., Lemaître J. F. (2017). The Williams’ legacy: A critical reappraisal of his nine predictions about the evolution of senescence. Evolution 71 (12), 2768–2785. doi: 10.1111/evo.13379
Grandison R. C., Wong R., Bass T. M., Partridge L., Piper M. D. (2009). Effect of a standardised dietary restriction protocol on multiple laboratory strains of Drosophila melanogaster. PloS One 4 (1), e4067. doi: 10.1371/journal.pone.0004067
Gregoire-Wibo C. (1974). Bioecology of folsomia quadrioculata. Pedobiologia 14, 199–207. doi: 10.1016/S0031-4056(23)00105-1
Gribble K. E., Moran B. M., Jones S., Corey E. L., Mark Welch D. B. (2018). Congeneric variability in lifespan extension and onset of senescence suggest active regulation of aging in response to low temperature. Exp. Gerontol 114, 99–106. doi: 10.1016/j.exger.2018.10.023
Grimnes K. A., Snider R. M. (1981). An analysis of egg production in four strains of Folsomia candida (Collembola). Pedobiologia 22 (4), 224–231. doi: 10.1016/S0031-4056(23)03689-2
Hafer N., Pike N. (2010). Shape change in viable eggs of the collembolan Folsomia candida provides insight into the role of Wolbachia endosymbionts. Zoological Res. 31 (6), 623–626. doi: 10.3724/SP.J.1141.2010.06623
Hayward A. D., Moorad J., Regan C. E., Berenos C., Pilkington J. G., Pemberton J. M., et al. (2015). Asynchrony of senescence among phenotypic traits in a wild mammal population. Exp. gerontol 71, 56–68. doi: 10.1016/j.exger.2015.08.003
Hoekstra L. A., Schwartz T. S., Sparkman A. M., Miller D. A. W., Bronikowski A. M. (2020). The untapped potential of reptile biodiversity for understanding how and why animals age. Funct. Ecol. 34 (1), 38–54. doi: 10.1111/1365-2435.13450
Jones O. R., Scheuerlein A., Salguero-Gómez R., Camarda C. G., Schaible R., Casper B. B., et al. (2014). Diversity of ageing across the tree of life. Nature 505, 169–173. doi: 10.1038/nature12789
Kassambara A., Kosinski M., Biecek P., Scheipl F. (2021). survminer: Drawing Survival Curves using ‘ggplot2’. Available at: https://cran.r-project.org/package=survminer.
Kasule F. K. (1991). Egg size increases with maternal age in the cotton stainer bugs Dysdercus fasciatus and D.cardinalis (Hemiptera: Pyrrhocoridae). Ecol. Entomol. 16 (3), 345–349. doi: 10.1111/j.1365-2311.1991.tb00226.x
Kelly M. A., Zieba A. P., Buttemer W. A., Hulbert A. J. (2013). Effect of temperature on the rate of ageing: an experimental study of the blowfly Calliphora stygia. PloS One 8 (9), e73781. doi: 10.1371/journal.pone.0073781
Kirkwood T. B. L. (2002). Evolution of ageing. Mech. Ageing Dev. 123 (7), 737–745. doi: 10.1016/S0047-6374(01)00419-5
Kirkwood T. B. L., Austad S. N. (2000). Why do we age? Nature 408 (6809), 233–238. doi: 10.1038/35041682
Kirkwood T. B. L., Shanley D. P. (2005). Food restriction, evolution and ageing. Mech. Ageing Dev. 126 (9), 1011–1016. doi: 10.1016/j.mad.2005.03.021
Kraus C., Pavard S., Promislow D. E. L. (2013). The size-life span trade-off decomposed: Why large dogs die young. Am. Nat. 181 (4), 492–505. doi: 10.1086/669665
Krivtsov V., Garside A., Bezginova T., Thompson J., Palfreyman J. W., Salmond R., et al. (2006). Ecological study of the forest litter meiofauna of a unique Scottish woodland. Anim. Biol. 56 (1), 69–93. doi: 10.1163/157075606775904687
Krivtsov V., Illian J. B., Liddell K., Garside A., Bezginova T., Salmond R., et al. (2003). Some aspects of complex interactions involving soil mesofauna: analysis of the results from a Scottish woodland. Ecol. Model. 170 (2-3), 441–452. doi: 10.1016/s0304-3800(03)00244-8
Lemaître J. F., Ronget V., Tidière M., Allainé D., Berger V., Cohas A., et al. (2020). Sex differences in adult lifespan and aging rates of mortality across wild mammals. Proc. Natl. Acad. Sci. 117 (15), 8546–8553. doi: 10.1073/pnas.1911999117
Li G.-Y., Zhang Z.-Q. (2021). Age-specific mortality and fecundity of a spider mite under diet restriction and delayed mating. Insect Sci. 29 (3), 889-899. doi: 10.1111/1744-7917.12948
Liefting M., Ellers J. (2008). Habitat-specific differences in thermal plasticity in natural populations of a soil arthropod. Biol. J. Linn. Soc. 94 (2), 265–271. doi: 10.1111/j.1095-8312.2008.00969.x
Liefting M., Weerenbeck M., Van Dooremalen C., Ellers J. (2010). Temperature-induced plasticity in egg size and resistance of eggs to temperature stress in a soil arthropod. Funct. Ecol. 24 (6), 1291–1298. doi: 10.1111/j.1365-2435.2010.01732.x
Mair W., Dillin A. (2008). Aging and survival: the genetics of life span extension by dietary restriction. Annu. Rev. Biochem. 77, 727–754. doi: 10.1146/annurev.biochem.77.061206.171059
Mair W., Goymer P., Pletcher S. D., Partridge L. (2003). Demography of dietary restriction and death in Drosophila. Science 301 (5640), 1731–1733. doi: 10.1126/science.1086016
Mair W., McLeod C. J., Wang L., Jones D. L. (2010). Dietary restriction enhances germline stem cell maintenance. Aging Cell 9 (5), 916–918. doi: 10.1111/j.1474-9726.2010.00602.x
Mair W., Piper M. D. W., Partridge L. (2005). Calories do not explain extension of life span by dietary restriction in Drosophila. PloS Biol. 3 (7), e223. doi: 10.1371/journal.pbio.0030223
Mallard F., Farina M., Tully T. (2015). Within-species variation in long-term trajectories of growth, fecundity and mortality in the Collembola Folsomia candida. J. Evolutionary Biol. 28 (12), 2275–2284. doi: 10.1111/jeb.12752
Mallard F., Le Bourlot V., Le Coeur C., Avnaim M., Péronnet R., Claessen D., et al. (2019). From individuals to populations: How intraspecific competition shapes thermal reaction norms. Funct. Ecol. 34, 669–683. doi: 10.1111/1365-2435.13516
Marshall V. G., Kevan D. K. M. (1962). Preliminary observations on the biology of Folsomia candida Willem 1902 (Collembola: Isotomidae). Can. Entomologist 94 (06), 575–586. doi: 10.4039/Ent94575-6
Martínez D. E. (1998). Mortality patterns suggest lack of senescence in Hydra. Exp. Gerontol 33 (3), 217–225. doi: 10.1016/S0531-5565(97)00113-7
Massot M., Clobert J., Montes-Poloni L., Haussy C., Cubo J., Meylan S. (2011). An integrative study of ageing in a wild population of common lizards. Funct. Ecol. 25 (4), 848–858. doi: 10.1111/j.1365-2435.2011.01837.x
Mattison J. A., Roth G. S., Beasley T. M., Tilmont E. M., Handy A. M., Herbert R. L., et al. (2012). Impact of caloric restriction on health and survival in rhesus monkeys from the NIA study. Nature 489 (7415), 318-321. doi: 10.1038/nature11432
Merry B. J. (2005). Dietary restriction in rodents - delayed or retarded ageing? Mech. Ageing Dev. 126 (9), 951–959. doi: 10.1016/j.mad.2005.03.015
Monaghan P., Maklakov A. A., Metcalfe N. B. (2020). Intergenerational transfer of ageing: parental age and offspring lifespan. Trends Ecol. Evol. 35 (10), 927–937. doi: 10.1016/j.tree.2020.07.005
Münch D., Amdam G. V., Wolschin F. (2008). Ageing in a eusocial insect: molecular and physiological characteristics of life span plasticity in the honey bee. Funct. Ecol. 22 (3), 407–421. doi: 10.1111/j.1365-2435.2008.01419.x
Naciri M., Aars J., Blanchet M.-A., Gimenez O., Cubaynes S. (2022). Reproductive senescence in polar bears in a variable environment. Front. Ecol. Evol. 10. doi: 10.3389/fevo.2022.920481
Nielsen M. L. K., Ellis S., Towers J. R., Doniol-Valcroze T., Franks D. W., Cant M. A., et al. (2021). A long postreproductive life span is a shared trait among genetically distinct killer whale populations. Ecol. Evol. 11 (13), 9123–9136. doi: 10.1002/ece3.7756
Partridge L., Piper M. D. W., Mair W. (2005). Dietary restriction in drosophila. Mech. Ageing Dev. 126 (9), 938–950. doi: 10.1016/j.mad.2005.03.023
Pike N., Kingcombe R. (2009). Antibiotic treatment leads to the elimination of Wolbachia endosymbionts and sterility in the diplodiploid collembolan Folsomia candida. BMC Biol. 7, 1–6. doi: 10.1186/1741-7007-7-54
Piper M. D. W., Partridge L., Raubenheimer D., Simpson S. J. (2011). Dietary restriction and aging: a unifying perspective. Cell Metab. 14 (2), 154–160. doi: 10.1016/j.cmet.2011.06.013
Rebora P., Salim A., Reilly M. (2018). bshazard: nonparametric smoothing of the hazard function. Available at: https://CRAN.R-project.org/package=bshazard.
Reichard M. (2016). Evolutionary ecology of aging: time to reconcile field and laboratory research. Ecol. Evol. 6 (9), 2988–3000. doi: 10.1002/ece3.2093
Reinke B. A., Cayuela H., Janzen F. J., Lemaître J.-F., Gaillard J.-M., Lawing A. M., et al. (2022). Diverse aging rates in ectothermic tetrapods provide insights for the evolution of aging and longevity. Science 376 (6600), 1459–1466. doi: 10.1126/science.abm0151
Reznick D., Bryant M., Holmes D. (2006). The evolution of senescence and post-reproductive lifespan in guppies (Poecilia reticulata). PloS Biol. 4 (1), e7. doi: 10.1371/journal.pbio.0040007
Roach D. A., Carey J. R. (2014). Population biology of aging in the wild. Annu. Rev. Ecol Evolution Systematics 45 (1), 421–443. doi: 10.1146/annurev-ecolsys-120213-091730
Schaible R., Scheuerlein A., Dańko M. J., Gampe J., Martínez D. E., Vaupel J. W. (2015). Constant mortality and fertility over age in Hydra. Proc. Natl. Acad. Sci. 112(51), 201521002. doi: 10.1073/pnas.1521002112
Snider R. J. (1983). Observations on the oviposition, egg development and fecundity of Onychiurus (Onychiurus) folsomi at constant temperature. Pedobiologia 25, 241–252. doi: 10.1016/S0031-4056(23)05929-2
Sparkman A. M., Arnold S. J., Bronikowski A. M. (2007). An empirical test of evolutionary theories for reproductive senescence and reproductive effort in the garter snake Thamnophis elegans. Proc. R. Soc. B: Biol. Sci. 274 (1612), 943–950. doi: 10.1098/rspb.2006.0072
Stam E. M., de Leemkule M. A. V., Ernsting G. (1996). Trade-offs in the life history and energy budget of the parthenogenetic Collembolan Folsomia candida (Willem). Oecologia 107 (3), 283–292. doi: 10.2307/4221335
Stroustrup N., Anthony W. E., Nash Z. M., Gowda V., Gomez A., López-Moyado I. F., et al. (2016). The temporal scaling of Caenorhabditis elegans ageing. Nature 530(7588), 103-107. doi: 10.1038/nature16550
Sultanova Z., Ivimey-Cook E. R., Chapman T., Maklakov A. A. (2021). Fitness benefits of dietary restriction. Proc. R. Soc. B 288 (1963), 20211787. doi: 10.1098/rspb.2021.1787
The R Development Core Team. (2021). R: A language and environment for statistical computing. Available at: http://www.R-project.org/.
Therneau T. M., Grambsch P. M. (2000). Modeling survival data: extending the cox model (New York: Springer). doi: 10.1007/978-1-4757-3294-8
Timmermans M. J. T. N., Ellers J. (2009). Wolbachia endosymbiont is essential for egg hatching in a parthenogenetic arthropod. Evolutionary Ecol. 23 (6), 931–942. doi: 10.1007/s10682-008-9282-0
Tully T., D’Haese C. A., Richard M., Ferriere R. (2006). Two major evolutionary lineages revealed by molecular phylogeny in the parthenogenetic collembola species Folsomia candida. Pedobiologia 50 (2), 95–104. doi: 10.1016/j.pedobi.2005.11.003
Tully T., Ferrière R. (2008). Reproductive flexibility: genetic variation, genetic costs and long-term evolution in a Collembola. PloS One 3 (9), e3207. doi: 10.1371/journal.pone.0003207
Tully T., Lambert A. (2011). The evolution of postreproductive life span as an insurance against indeterminacy. Evolution 65 (10), 3013–3020. doi: 10.1111/j.1558-5646.2011.01347.x
Tully T., Le Galliard J., Baron J. (2020). Micro-geographic shift between negligible and actuarial senescence in a wild snake. J. Anim. Ecol. 89 (11), 2704–2716. doi: 10.1111/1365-2656.13317
Tully T., Potapov M. (2015). Intraspecific phenotypic variation and morphological divergence of strains of Folsomia candida (Willem)(Collembola: Isotomidae), the” standard” test springtail. PloS One 10 (9), e0136047. doi: 10.1371/journal.pone.0136047
Usher M. B., Hider M. (1975). Studies on populations of Folsomia candida (Insecta: Collembola): causes of aggregation. Pedobiologia 15, 276–283. doi: 10.1016/S0031-4056(23)00045-8
Van Amelsvoort P. A. M., Usher M. B. (1989). Egg production related to food quality in Folsomia candida (Collembola: Isotomidae): effects on life history strategies. Pedobiologia 33 (1), 61–66.
Vaupel J. W., Baudisch A., Dölling M., A. Roach D., Gampe J. (2004). The case for negative senescence. Theor. Population Biol. 65 (4), 339–351. doi: 10.1016/j.tpb.2003.12.003
Vedder O., Bichet C., Tschirren B. (2022). The effect of manipulated prenatal conditions on growth, survival, and reproduction throughout the complete life course of a precocial bird. Front. Ecol. Evol. 10. doi: 10.3389/fevo.2022.834433
Willem V. (1902). Note préliminaire sur les Collemboles des Grottes de Han et de Rochefort. Annales la Société Entomologique Belgique 46, 275–283. Available at: https://biostor.org/reference/236824.
Williams G. C. (1957). Pleiotropy, natural selection, and the evolution of senescence. Evolution 11 (4), 398–411. doi: 10.2307/2406060
Keywords: age trajectory, ageing, phenotypic plasticity, dietary restriction, egg size, negligible senescence, lifespan, indeterminate growth
Citation: Tully T (2023) Diversity, plasticity and asynchrony of actuarial and reproductive senescence in the Collembola Folsomia candida (Willem, 1902). Front. Ecol. Evol. 11:1112045. doi: 10.3389/fevo.2023.1112045
Received: 30 November 2022; Accepted: 02 October 2023;
Published: 27 October 2023.
Edited by:
Jean-Michel Gaillard, Université Claude Bernard Lyon 1, FranceReviewed by:
Sophie Beltran-Bech, UMR7267 Ecologie et biologie des interactions (EBI), FranceCopyright © 2023 Tully. This is an open-access article distributed under the terms of the Creative Commons Attribution License (CC BY). The use, distribution or reproduction in other forums is permitted, provided the original author(s) and the copyright owner(s) are credited and that the original publication in this journal is cited, in accordance with accepted academic practice. No use, distribution or reproduction is permitted which does not comply with these terms.
*Correspondence: Thomas Tully, dGhvbWFzLnR1bGx5QHNvcmJvbm5lLXVuaXZlcnNpdGUuZnI=
Disclaimer: All claims expressed in this article are solely those of the authors and do not necessarily represent those of their affiliated organizations, or those of the publisher, the editors and the reviewers. Any product that may be evaluated in this article or claim that may be made by its manufacturer is not guaranteed or endorsed by the publisher.
Research integrity at Frontiers
Learn more about the work of our research integrity team to safeguard the quality of each article we publish.