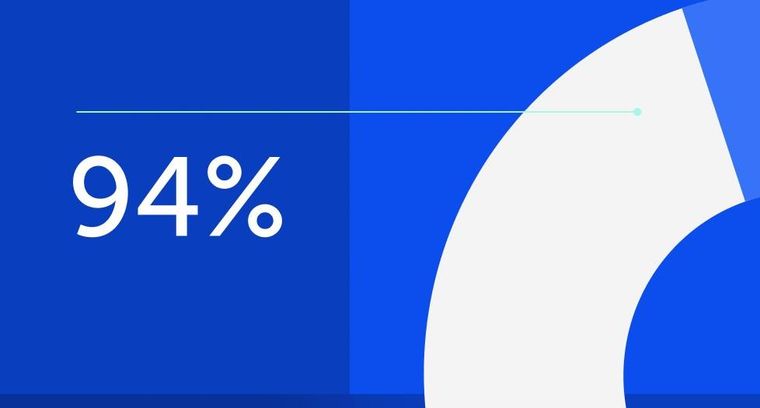
94% of researchers rate our articles as excellent or good
Learn more about the work of our research integrity team to safeguard the quality of each article we publish.
Find out more
ORIGINAL RESEARCH article
Front. Ecol. Evol., 20 February 2023
Sec. Population, Community, and Ecosystem Dynamics
Volume 11 - 2023 | https://doi.org/10.3389/fevo.2023.1110594
This article is part of the Research TopicThe Effects of Climate Change and Anthropogenic Activities on Patterns, Structures and Functions of Terrestrial EcosystemsView all 18 articles
Introduction: China’s forests have sequestrated a significant amount of carbon over the past two decades. However, it is not clear whether China’s forests will be able to continue to have as much carbon sequestration potential capacity in the future.
Methods: In order to research China’s forest carbon storage and carbon sequestration potential capacities at spatial and temporal scales, we built a digital forest model for each province of China using the data from The China Forest Resources Report (2014– 2018) and calculated the carbon storage capacity and sequestration potential capacity of each province with the current management practices without considering natural successions.
Results: The results showed that the current forest carbon storage is 10.0 Pg C, and the carbon sequestration potential in the next 40 years (from year 2019 to 2058) will be 5.04 Pg C. Since immature forests account for the majority of current forests, the carbon sequestration capacity of the forest was also high (0.202 Pg C year−1). However, the forest carbon storage reached the maximum with the increase of stand maturity. At this time, if scenarios such as afforestation and reforestation, human and natural disturbances, and natural succession are not considered, the carbon sequestration capacity of forests will continue to decrease. After 90 years, all stands will develop into mature and over-mature forests, and the forest carbon sequestration capacity is 0.008 Pg year−1; and the carbon sequestration rate is ~4% of what it is nowadays. The change trend of forest carbon in each province is consistent with that of the country. In addition, considering the large forest coverage area in China, the differences in tree species and growing conditions, the forest carbon storage and carbon sequestration capacities among provinces were different. The growth rate of carbon density in high-latitude provinces (such as Heilongjiang, Jilin, and Inner Mongolia) was lower than that in the south (Guangdong, Guangxi, or Hunan), but the forest carbon potential was higher.
Discussion: Planning and implementing targeted forest management strategies is the key to increasing forest carbon storage and extending the service time of forest carbon sinks in provinces. In order to reach the national carbon neutrality goals, we recommend that each province have an informative strategic forest management plan.
Since the Industrial Revolution, a large amount of fossil fuel has been utilized and a significant amount of carbon dioxide has been released into the atmosphere. The carbon emission is far more than the amount absorbed by the marine and terrestrial ecosystems. Forest carbon sequestration is the most cost effective nature-based way to reduce the CO2 in the atmosphere (Münnich Vass, 2017). Accurate estimates of carbon storage and the carbon sequestration potential of forests are critical for achieving the strategic goal of carbon neutrality (Broadstock et al., 2021). In order to reduce CO2 emission, many countries and regions have promised to achieve carbon neutrality within certain time frames. At the United Nations Climate Action Summit in September 2019, 66 parties committed to achieve net-zero emissions, and China committed to achieve carbon neutrality by the year 2060 (UNFCCC, 2019). The carbon sequestration capacity depends on the photosynthesis (P) and respiration (R) potential of the trees (Jiang et al., 2020). E.P.Odum’s ecological succession hypothesis is one of the main hypotheses to explain the overall development of forest community. Trees sequestrated CO2 through photosynthesis and released carbon through respiration. According to the hypothesis, the biomass increases in the early stage of ecological succession when P/R > 1. The value of P/R will be close to 1 when the stands in the forest reach mature growth. The carbon budget of the ecosystem will reach a balance, and the forest biomass will reach the maximum limit (Odum Eugene, 1969). In other words, the forest carbon sink tends to be zero when the stands in the forest reach mature stages. Meanwhile, a crucial issue has emerged for our world: if forests lost the function of doing carbon sequestration, what would be the fate of the CO2 emitted by the industry? This is why scientists need to estimate and predict future changes in forest carbon storage and carbon sequestration capacity.
There are 4.1 billion ha forests in the world, and the annual forest carbon sequestration is about 1.1 ± 0.8 Pg C year−1 (Pan et al., 2011). Existing forests will continue to absorb large amounts of CO2, but the future sequestration capacities will depend on forest management. Studies have shown signs of carbon sequestration saturation in tropical rainforests in Africa and in the Amazon (Hubau et al., 2020), tropical Borneo (Qie et al., 2017) and in parts of Europe (Nabuurs et al., 2013). The carbon sequestration rate of forests is closely related to their age structure (Pan et al., 2004). The carbon sequestration rate of young and middle-aged forest is relatively high, and the carbon sequestration capacity rate begins to decline gradually (Pregitzer and Euskirchen, 2004; Zhou et al., 2015) because of stand aging. In theory, by the end of the life cycle, trees will eventually die and become a carbon source, resulting in carbon release (Maia et al., 2020).
Since the 1970s, China has implemented large-scale afforestation and reforestation programs to protect the ecological environment. At present, the area of plantation forests in China ranks the first in the world, and the forest area has increased from 12.7% in early 1970 to 21.6% in 2013. China’s forests are making a positive response to global carbon neutralization (China, S.F.A.O, 2014). Previous forest carbon sequestration studies have predicted the future carbon sequestration capacities, for example, He et al. (2017) predicted the change of carbon storage and carbon sequestration rate of Chinese forests from 2010 to 2050, Cai et al. (2022) predicted carbon sequestration rate for the time period 2010–2060, while Xu et al. (2010) predicted carbon sequestration rates for the period 2000–2050, and Yao et al. (2018) predicted carbon sequestration rate for the period 2010–2040. These results show that Chinese forests will be able to maintain a higher carbon sequestration rate and absorb more CO2 from the atmosphere in the next 30–50 years. These studies play an important role in the specific contribution of forests to conduct carbon neutralization by the year 2060. However, how the forest carbon sequestration capacity will change after 2060 is unknown. We need to learn more about the forest carbon sequestration capacity to make smart forest management decisions to achieve and maintain carbon neutrality after the year 2060.
The Bonn Challenge1 launched in 2011, encourages countries to restore 150 million ha of forest by 2020 and 350 million ha (Hawes, 2018) by 2030, and countries around the world have actively responded to global climate change. China has implemented a series of measures since the 1970s, such as the Three-North Shelter Forest program, the Natural Forest Protection program and many other programs. From 2001 to 2010, the total carbon sequestration of these programs was 132 Tg C year−1 (Lu et al., 2018), which made a significant contribution to China’s current and future CO2 emission reduction (Wang et al., 2018). So far, China has nearly 60 million ha of plantation forests, accounting for 31.76% of the total forest area in China, and most areas are still in the juvenile stages. Due to excessive deforestation over the last decades, more than 60% of the second growth natural forests are still at young and middle-age stages (Dai et al., 2018). The potential carbon sequestration capacity for the near future (from 2010 to 2060) will be significant, however, the carbon sequestration capacity for the distant future (after 2060) is not clear yet. Therefore, the analysis of forest carbon storage and carbon sequestration capacity is required at both a national scale and provincial scales.
The carbon storage and carbon sequestration capacities are particularly important for China’s long-term forest management. The objectives of this research are to predict the carbon storage and sequestration capacities of China in the long-term based on the current management practice and the data of the China Forest Resources Report (2014–2018).
China is located in the northern hemisphere, with forests covering a wide range of latitudes from north to south. The latitude and climatic differences between the north and south of China make the country’s climate complex and diverse. The latitudinal spatial of forests makes China’s forest types diverse, such as tropical rain forests, subtropical evergreen broad-leaved forests, warm temperate deciduous broad-leaved forests, temperate coniferous and broad-leaved mixed forests, and cold temperate coniferous forests. The longitude spatial makes the country’s forests mainly concentrated in the east, and the west is mostly grassland and desert.
Since the 1970s, every 5 years, the State Forestry Administration of China has published statistics about forest resources. According to the data, forests are divided into three major categories: arborescence forest, economic forests and bamboo forests (this study does not include economic forests or bamboo forests). Furthermore, according to the dominant tree species, the forest was divided into 36 forest types, and each forest type was divided into five age classes: young forest, middle-aged forest, premature forest, mature forest and over-mature forest. The classification of age classes will be carried out with the age classes and age groups of main tree species in the Technical Regulations on Forest Resource Inventory of China (Supplementary Table 1; Administration, C.S.F, 2014). The study covers the entire national forest area of 31 provinces of China.
The key to the prediction of forest carbon storage is to understand the relationship between biomass accumulation and forest ages. In recent years, many studies have found that the Logistic equation can better describe the relationship between biomass density and forest ages (Zhou et al., 2014; He et al., 2017). Xu et al. (2010) used the logistic function to fit the biomass growth curves statistically. In this study, we used the logistic curves of forest stand types by Xu, combined with the latest national forest inventory data in China, to calculate and predict the forest carbon storage capacities of each province.
where B is the biomass density (Mg C ha−1); t is the forest age (year); w k and c are the model parameters. Xu used China’s forest data to fit logistic curves of biomass and age of different forest types. We can see the fitted parameter values (w k and c) for each forest type in Supplementary Table 2.
China Certified Emission Reduction (CCER) was launched in 2011, from which the development of forestry carbon sequestration projects has attracted widespread attention from around the world (Ye et al., 2021). At present, the CCER forestry carbon sequestration project is divided into Afforestation carbon sequestration methodology, forest management and carbon sequestration methodology, bamboo afforestation carbon sequestration methodology, and bamboo management and carbon sequestration methodology. Those methodologies accord with the present situation of ecological development in China, and have the characteristics of being universal, cost effective, and environmentally integrated as well as being scientific and reasonable (Li L. et al., 2019).
Forest management and carbon calculation methodology was used to calculate the carbon storage amount of each province. The carbon storage amount mainly included four carbon pools, living trees, dead trees, litter, and harvested wood production. The carbon storage of this study was calculated and predicted based on the current forest area without considering afforestation and reforestation, or any human and natural disturbances in the future using Eq. 2.
where is the total forest carbon of the country; represent the carbon pools of live trees, dead wood, litter carbon, and harvested wood production, respectively; A is the total area of each forest stand type; i, t, and j parameters represent the forest stand types, age groups, and provinces. is generally calculated as the biomass multiplied by the carbon density of each tree species. Many studies used the default values of 0.5 to transfer biomass to carbon storage (Taeroe et al., 2017; Cai et al., 2022; Chen et al., 2022), but there were significant differences in carbon density among different species. The average carbon density of the deciduous species was <0.5, and the coniferous species was higher than 0.5. We calculated the carbon storage using the average carbon density of each tree species based on the Forestry Standard—Standing tree Biomass Model and Carbon parameters (forestry standard number: LY/T2258-2014, LY/T2259-2014, LY/T2260-2014, LY/T2261-2014, LY/T2262-2014, LY/T2263-2014, LY/T2264-2014, LY/T2655-2016, LY/T2654-2016, LY/T2659-2016, LY/T2661-2016, LY/T2660-2016, LY/T2656-2016, LY/T2657-2016, LY/T2658-2016). In addition, CHWP is equal to zero because forest harvesting was not involved in this study.
The biomass of the whole tree was mainly composed of two parts: aboveground biomass and belowground biomass. Most studies ignore the belowground biomass or use the average ratio of 0.25 given by FAO (Chen et al., 2022). We calculated the belowground carbon according to the ratio of belowground biomass to aboveground biomass of each tree species given by the National Greenhouse Gas Inventory Report of China 2005 (Commission, N.D.a.r, 2014) as Eqs 3, 4.
where is the carbon density of the tree species i. is the live tree biomass. and represent aboveground biomass and belowground biomass, respectively.
where is the dead wood carbon of tree species i; is live tree carbon; is the ratio of dead wood carbon and live tree carbon of tree species i.
According to the Chinese forest biomass database, we discovered that the correlation between the ratio of litter biomass to the aboveground biomass and aboveground biomass was given by Eq. 6.
where is litter carbon of the tree species i; is the carbon rate of litter in tree species i, and 0.37 is the amount of C per kg of dry litter biomass; is the percentage of litter biomass to the aboveground biomass (%).
In our study, the entire carbon pool change was affected by the changes of trees, dead wood, and litter. We did not consider the changes of soil organic carbon pool and carbon storage of shrub and grass under the forest. In addition, the change of carbon pool caused by extreme climate (fire, flood, and drought) was not within the scope of our study. This function was used to determine forest carbon sequestration capacity:
where is the total carbon stock in year t1; is the current carbon storage; , and represent the variation of the change of carbon storage of trees, dead wood, and litter during t1-t0, respectively. Forest Simulation and Optimization System (FSOS)2 were used for the analysis in this study (Liu et al., 2006). FSOS is a forest planning system with artificial intelligence algorithm to balance and optimize multiple forest functions using cloud computing. FSOS is a widely used decision support system with stand dynamic modeling and landscape modeling.
The current forest carbon storage in China is 10.0 Pg, and this rate will continue to increase in the future. The total carbon storage will increase from 10.0 Pg to 16.2 Pg, with a total growth potential of 62% in the next 200 years (Figure 1). The total forest carbon storage will increase gradually and attain a stable stage with the increase of time. On the national scale, the carbon sequestration capacity has already reached its peak and has begun to gradually decrease. Forest carbon sequestration capacity is currently the greatest (0.202 Pg year−1), and the decline of forest carbon sequestration capacity has changed from fast to slow, which is what it will be in the future. If forests continue to develop at this trend, they will play a smaller role in achieving the goal of carbon neutrality.
Figure 1. The carbon storage and carbon sequestration capacity of China’s forests in contemporary times and in the future.
Using the age and age classification of each forest type, we drew a national forest age structure figure. At present, the forest area of each age class accounts for 32.67% (young forest), 30.39% (middle-aged forest), 15.59% (premature forest), 14.11% (mature forest), and 7.24% (overmature forest) of the total forest area, respectively (Figure 2). Combined with the changes of forest carbon sequestration capacity, the current forests are mainly young, and the carbon sequestration capacity is at its peak. The proportion of young and middle-aged forests gradually decreased with stands beginning to age. The forest will change from a young state to a mature state, and the carbon sequestration capacity also will decrease. After 35 years, all the young forests will have been transformed into middle-aged forests, and the carbon sequestration capacity will drop from 0.202 Pg year−1 to 0.063 Pg year−1; additionally, the carbon sequestration rate might decrease 69%. The middle-aged forest will develop into premature forests over the next 70 years, the carbon sequestration capacity of the forest will be 0.016 Pg year−1, and the carbon sequestration capacity will decrease by 92%. After 90 years, all the stands will have developed into mature and overmature forests, and the carbon sequestration capacity of forest will be 0.008 Pg year−1, and the carbon sequestration rate will be ~4% of the current forest carbon sequestration capacity. When the forest is all overmature (after 130 years), the carbon sequestration capacity will be 0.0017 Pg year−1, and the carbon sequestration rate will be reduced by 99%.
There are great differences in carbon storage capacities among the different provinces (Figure 3). The regions with higher carbon storage capacities are mainly in northeastern and southwestern China. Heilongjiang province has the highest carbon storage of 1.49 Pg C, followed by Sichuan (1.28 Pg C), Inner Mongolia (1.16 Pg C) and Yunnan (0.87 Pg C). The forest carbon storage of the eastern coastal provinces is lower than that in the northeastern or southwestern provinces. The lowest carbon storage was in the central area (Henan, Anhui, Shandong, Shanxi, Jiangsu, and Shanghai) of China, which ranged from 0.002 Pg to 0.16 Pg. Although the land area of this western regions is very large, the forest area is relatively small. Due to the harsh environment, there are few types of woodlands suitable for tree growth, the trees grow slowly, and the forest carbon storage potential is lower than that in other areas.
The carbon sequestration capacities in forest ecosystems were distributed heterogeneously across China. The current forest carbon sequestration capacities in the southwestern and eastern regions are at their peaks, and have greatly reduced with stand aging (Figure 4). Most regions in the central-southern region (Guangdong, Guangxi, Hunan, Hubei et al.) have not yet reached the maximum carbon sequestration capacities yet and they will reach the maximum around the year 2028. Through the comparative analysis of carbon storage and the carbon sequestration capacity of 31 provinces in China, generally speaking, there is a positive correlation between forest carbon sequestration capacity and carbon storage, such as in Heilongjiang or in Inner Mongolia, Sichuan or the Yunnan provinces. The corresponding carbon sequestration capacity is also higher than that of other provinces. Interestingly, although the carbon sequestration capacity of forests in the Guangxi Province is lower than that in the Yunnan Province, it is still higher than that in Heilongjiang, Inner Mongolia or Sichuan. In addition, the carbon sequestration capacity of other provinces in the southern regions (Guangdong, Hunan, Hubei) is also higher than that of most other provinces in China.
Figure 4. Carbon sequestration capacities (Tg C year−1) of 31 provinces over the next 200 years. Forests in China are divided into six regions: Northwestern region (Shaanxi, Gansu, Xinjiang, Qinghai, and Ningxia), Northern region (Inner Mongolia, Hebei, Inner Mongol, Shanxi, Beijing, and Tianjin), Northeastern region (Heilongjiang, Jilin, and Liaoning), Southwestern region (Sichuan, Yunnan, Tibet, Guizhou, and Chongqing), Central southern region (Guangxi, Guangdong, Hubei, Hunan, Henan, and Hainan), and Eastern region (Fujian, Jiangxi, Zhejiang, Shanghai, Anhui, Shandong, and Jiangsu).
At present, the average carbon density of all forest is 56.69 Mg C ha−1 in China, and only 9 provinces are above the average level (Figure 5). The areas with higher carbon densities are mainly concentrated in the northeast, northwest and southwest of China, and the lower carbon density areas are in the eastern and south-central regions. The forest carbon density (91.68 Mg C ha−1) of Xinjiang is the highest, followed by Tibet (84.78 Mg C ha−1), Sichuan (83.18 Mg C ha−1), Jilin (75.57 Mg C ha−1), Heilongjiang (75.07 Mg C ha−1) and other regions. The carbon density in Tianjin, Jiangsu and Guangxi is much lower than other provinces, and the forests of Guangxi are mainly young plantation forests. The carbon density of provinces in the eastern and southeastern regions is low, and the carbon density is between 20 and 40 Mg C ha−1, but the future growth rate is expected to be faster.
In the next 40 years, the average forest carbon density of China will increase greatly in the eastern, central and southern regions of China, from 56.69 Mg C ha−1 in 2018 to 86.38 Mg C ha−1 in 2058. We also found that the increment rate of carbon density in most provinces in southern China was higher than that in the north, but the potential of forest carbon sequestration in southern China will likely account for a major part of the whole carbon uptake process in the future.
Forest carbon sequestration provides a low-cost, nature-based solution for national carbon neutral strategies with enormous potential capacities for the future. Piao et al. (2022a) compared and analyzed four large-scale ecosystem carbon storage and carbon sequestration capacity methods. The existing carbon storage estimation methods of forest vegetation have been relatively mature. Our study uses the inventory method to calculate the carbon storage of China’s forests without including economic forests and bamboo forests. The total carbon storage is 10.0 Pg C of various vegetation types of 31 provinces. Tang et al. (2018) also calculated the carbon storage of China’s forests by vegetation types. The carbon storage of Chinese forests is 10.5 ± 2.0 Pg C. Chen et al. (2022) calculated that the carbon storage of natural forest of China was 9.4 ± 1.45 Pg C using a similar method and the data of 762 natural forest sites in 2010. The previous studies estimated that the carbon storage is 1.4 Pg C in the plantation forests (Zhang et al., 2015), and 10.8 Pg C in the natural forests. The results of all these studies are consistent with those of this study. Due to the lack of economic forest and bamboo forest data, the total carbon storage of this study is slightly lower than that of other studies.
To better understand the characteristics of forest carbon sequestration and the contribution to carbon neutrality, based on the calculation of carbon storage, we forecasted the forest carbon storage and sequestration capacities of China for the next 200 years. From 2019 to 2048, the increment of forest carbon storage will be 4.48 Pg C, and the average carbon sequestration will be 0.15 Pg C year−1. He et al. (2017) predicted that the increment of carbon storage of forest vegetation of China will be 13.92 Pg C and the average carbon sequestration will be 0.34 Pg C year−1 from 2010 to 2050, which is much higher than our results. While Yao et al. (2018) predicted that the increment from 2010 to 2050 will be 6.69 Pg C (the average carbon sequestration is 0.17 Pg C year−1). Xu et al. (2010) predicted 7.23Pg C (0.14 Pg C year−1) from 2000 to 2050, and Cai et al. (2022) predicted 0.211 Pg C year−1 from 2010 to 2060. These results are similar to ours. Our results showed that in the next 40 years, the carbon storage will increase from 10.0 Pg C to 15.04 Pg C, and the average carbon sequestration will be only 0.13 Pg C year−1. However, it is worth noting that after 90 years, when all forests have transitioned to a mature state, the carbon sequestration capacity of forests will be only 4% of the current level, and forests almost lost their carbon sequestration capacity. Our results supported E.P.Odum’s ecological succession hypothesis. The forests will gradually enter the old seral stages, the carbon sequestration capacities will decrease greatly, and the carbon storage of the whole forest will gradually reach the upper limit in stable environment conditions (Odum Eugene, 1969; Hubau et al., 2020; Piao et al., 2022b). Of course, our research object is only the current forests in China. In the future, due to afforestation and reforestation, human management, natural succession of forests, and natural disturbances such as hurricanes, floods, droughts, and wildfires that forests may suffer. These factors will affect the carbon storage and carbon sequestration capacity of forests (Fekete et al., 2017; Ceccherini et al., 2020; Walker et al., 2020; Anderegg et al., 2022; Phillips et al., 2022). Studies showed that China’s terrestrial carbon sequestrations will offset 2.8%–18.7% of fossil fuel emissions by the year 2060 (Cai et al., 2022; Yang et al., 2022). However, China will also face more severe challenges in achieving the goal of carbon neutrality because of the decrease in forest carbon sequestration capacities. The carbon sequestration capacity will tend to be zero as the forest gradually enters the mature stage (Odum Eugene, 1969).
The study showed that the carbon storage and carbon sequestration capacities have large spatial heterogeneity in China, and the carbon storage capacity in the northeastern and southwestern regions is much higher than that in other regions. The difference in carbon storage among the different provinces is mainly related to the forest areas of each province. In the last 20–25 years, carbon storage in the northeast of China has increased from 1.79 Pg C from 1994 to 1998 to 2.17 Pg C from 1997 to 1999 (Fang et al., 2001), a 45.95% increase because of plantations (Luo et al., 2020), mainly due to the 3-North Shelter Forest Program that has been implemented by China since 1979. The project requires that the afforestation areas in the three northern areas increase from 5.05% to 15.95% by 2050. The fast-growing trees absorb CO2 quickly because of the good climatic conditions in the southeast of the Tibet Plateau, with suitable temperature and sufficient precipitation. Another main reason for the carbon storage increase is that the area is less disturbed (Keith et al., 2009). Forest disturbances have always been the major factors affecting forest carbon storage and carbon sequestration capacity (Seidl et al., 2014). Storms, droughts, pests, wildfires and human disturbances have a great impact on the global carbon storage. For example, Kurz et al. (2008) predicted that continued insect outbreaks in British Columbia will lead to forest loss of about 374,000 square kilometers of forest area between 2000 and 2020, and they also indicated that the forest fires in western Canada have also released a huge amount of carbon. With global warming, climate extremes such as droughts and storms can decrease the regional ecosystem carbon stocks (Reichstein et al., 2013). Lasslop et al. (2020) used simulations from seven global vegetation models to estimate the fire impacts on global tree cover and the carbon cycle, and found that global fires reduce forest cover and vegetation carbon storage by 10%. In addition, there is sufficient evidence to prove that deforestation has reduced forest carbon stocks in the Amazon by 5.1 ± 3.7% (Li et al., 2022).
According to the 2014 IPCC’s report, forest carbon sequestration is the most cost-effective way for national carbon neutral strategies. Forest carbon sequestration capacities can be increased by reducing deforestation, sustainable management and afforestation (IPCC, 2004). In China, forests are widely distributed, with large latitude and longitude spans, and complex terrain. In general, the national forest area, the forest carbon storage and the carbon sequestration capacity have been positively correlated. In areas with high carbon storage, forest carbon sequestration capacity will also be stronger. However, it is worth noting that some low-latitude provinces have lower carbon storage than high-latitude provinces, but their carbon sequestration capacity is stronger than that of high-latitude provinces. For example, the total carbon storage of the Guangxi Province is lower than that of Heilongjiang and Inner Mongolia, but its carbon sequestration capacity is also greater. The reasons may be as follows: First, the proportion of young and middle-aged forests in the Guangxi Province is 84% higher than that in Heilongjiang (61%) and in Inner Mongolia (53%), and the carbon sequestration capacity of young and middle-aged forests is still relatively strong. Second, there are great differences in forest types among the provinces. The southern forests are dominated by short-life cycle tree species with a short maturity period, while the northern tree species have a longer life cycle. The southern forests mainly have the fastest growth and shortest rotation species (Populus spp., Cunninghamia lanceolata, Eucalyptus robusta, Larix spp.), which have greater carbon sequestration capacities if we manage them properly. Third, the environment in the southern region is more suitable for tree growth, with suitable temperatures and precipitation enabling trees to grow year-round, while the annual growth of the cycle of trees in the northern region is short. Here, we also found that although the current carbon sequestration capacity of some south-central provinces (Guangdong, Hunan, and Hubei) is not much different from that of Heilongjiang and Inner Mongolia, the carbon sequestration capacity in the future will decline much more rapidly than in these other provinces. This is related to the growth characteristics of the forest ecosystems in each province. Long life-cycle tree species have a longer carbon sink service time. To maintain a higher level of forest carbon sequestration capacity after achieving the carbon neutralization, China needs to manage forests more effectively to improve forest carbon sequestration capacity as much as possible. Ameray et al. (2021) proposed three management strategies for boreal, temperate and tropical forests: extensive forest management, intensive forest management and old-growth forest conservation. Old-growth forests can store more carbon than young forests, and although older forests have a slower rate of carbon sequestration, old-growth forest will release more carbon after logging (Harmon et al., 1990). Intensive forest management is one way to increase the carbon sequestration capacity of fast-growing tree species. Extensive forest management increases forest carbon sequestration capacity and soil carbon storage through partial cutting (Dalmonech et al., 2022). Due to the strong spatial heterogeneity of China’s forest carbon, it is very necessary to implement corresponding management strategies for forest conditions in different regions. For example, forests in Guangxi, Guangdong, and Fujian should focus on intensive forest management to improve the carbon sequestration capacity of fast-growing species such as Cunninghamia lanceolata and Eucalyptus robusta. Provinces such as Heilongjiang, Inner Mongolia, Jilin, and Liaoning have long forest life cycles. It is recommended to adopt extensive forest management and increase forest and soil carbon storage through partial cutting. Forests in Yunnan, Sichuan and Tibet are less disturbed and have higher carbon density, so it is recommended to focus on protection.
Currently, the forests of China will have largest carbon storage potential and strongest carbon sequestration capacities by the year 2058, but the role of forest carbon sequestration will decline gradually. Therefore, the key to maintaining the high carbon sequestration capacity of forests lies in two points. First, increase the forest area as much as possible and increase the carbon storage potential. From the late 1990s to 2010, the cumulative afforestation area of six key forests projects begun in China increased by 106.31 × 106 ha. The potential afforestation area in the future (2010–2050) is about 300,000 ha, and the carbon sequestration potential of afforestation may reach 2.52 Pg. These afforestation projects are the key to increasing carbon storage in China’s forest ecosystems (Huang et al., 2012). Second, maintain a reasonable forest age structure and maintain a certain proportion of young and middle-aged forests through forest management measures. Here we suggest that national-scale forest planning should implement targeted measures on a province-by-province basis. For forests with fast growing trees in the south, high-intensity management and reforestation methods are used to maintain the high carbon sequestration capacity of forests (Busch et al., 2019; Veldkamp et al., 2020). Aiming at the slow-growing forests in the north, increasing the forest area is not the main goal, but improving forest quality through low-intensity management is the key (Canadell and Raupach, 2008; Feng et al., 2022; Kreier, 2022). The objectives of this research are to comprehensively analyze forest carbon reserves and carbon sequestration potential at a national scale as well as to understand the changes of forest carbon sequestration capacities, and provide a reference for decision-makers so that they can make more effective and more intelligent decisions.
Forest stand ages are important factors for the carbon sequestration capacities of forest ecosystems because the carbon accumulation greatly depends on forest stand ages (Yao et al., 2018). At present, 63.06% of China’s forests are young and middle-aged forests, so China’s forests currently maintain a high forest carbon sequestration capacity. But the carbon sequestration capacity declines rapidly with the succession of ecosystems. When the forest is fully succeeded to a mature state (after 90 years), the carbon sequestration capacity of the forest decreases by 68%. Therefore, to maintain the high carbon sequestration capacity of forests, a certain proportion of young forests must be maintained. The forest carbon sequestration capacities of China have increased by 34% through afforestation and reforestation after the forests were destroyed before the 1990s (Pan et al., 2011). The high proportion of young and middle-age forests make China’s current forest carbon density (56.69 Mg C ha−1) lower than the United States (88 Mg C ha−1; Pan et al., 2011; Nelson et al., 2017). The areas with high carbon densities in China are mainly concentrated in the Northeast, Northwest and Southwest, and the carbon densities in South-Central and Eastern areas are relatively small. The mature and over-mature forests are mainly located in the Northeast, Northwest and Southwest.
The current forest is dominated by immature forest stands, with strong carbon sequestration capacities, and the carbon density will increase from 56.69 Mg C ha−1 to 86.38 Mg C ha−1 over the next 40 years (by 2058). However, the forest carbon sequestration capacities will decrease after the stands reach the mature and over-mature stageswithin the next 40 years. The average carbon density of the national forest will reach the stable stage 91.93 Mg C ha−1 after 100 years, and the carbon density of New Zealand’s natural forest will be 227 Mg C ha−1 when it reaches the stable stage. There are two main reasons for the differences: the natural forest of New Zealand Forest Inventory is mainly composed of primordial forest, which has a more stable structure and a higher carbon storage capacity with a longer growing season and good rainfall (Paul et al., 2021). Reasonable age structure is the key to maintaining high carbon sequestration capacity of forests (Li Y. et al., 2019).
The results of this study analyzed the changes of forest carbon storage and carbon sequestration capacities in China. The current forest carbon storage in China is 10.0 Pg C. Before the national carbon neutrality strategic goal is achieved in 2060, the forest still has 5.04 Pg increment space. However, in the next 40 years, the carbon sequestration capacity will be greatly reduced, from 0.202 Pg year−1 to 0.050 Pg year−1, and the carbon sequestration capacity will decrease by 75%. These changes in the trends of forest carbon in the provinces are basically the same as those in the country, and as the new forests, the possible disturbances in the future, and the forest succession are not considered, the carbon sequestration capacity of forest tends to zero with the forest age. Therefore, in order to maintain a high forest carbon sequestration capacity and a longer service time for forest carbon sinks, we have to make more comprehensive strategic plans for our forests. At present, restoration, afforestation, reforestation and sustainable forest development will be the main methods through which to extend the service time of forest carbon sinks. Because of the strong spatial–temporal heterogeneity of forest carbon in China, to formulate and implement afforestation and management strategies according to the changes of forest carbon pools and the carbon sequestration capacity in each province, strategies need to be developed and implemented.
We suggest that the carbon trades should include the carbon storage amount and time in the future because the carbon sequestration of all forests will be gradually reduced to zero when the forests recover to the stable stages. Paying fees by carbon storage amount and time will be a more effective way to keep carbon in the forests and reduce the amount of carbon in the atmosphere. The combination of carbon storage fee and the carbon sequestration fee can be a long-term effective way to reduce the greenhouse gas amount in the atmosphere.
The raw data supporting the conclusions of this article will be made available by the authors, without undue reservation.
FC and JT: conceptualization, methodology, formal analysis, investigation, data curation, writing–original draft, and writing–review. JH: data curation and writing–review. HH: conceptualization, writing–review and editing. GL: methodology, formal analysis, investigation, project administration, and funding acquisition. ZZ: investigation, project administration, and funding acquisition. LZ: writing–review and editing. All authors contributed to the article and approved the submitted version.
This project was supported by the Jilin Scientific and Technological Development Program: Impact of Harvesting Disturbance on the Relationship between Coniferous and Broadwood Mixed Forest Productivity and Biodiversity (20210508012RQ); Jilin Scientific and Technological Development Program: Research and Demonstration of Natural Secondary Forest Management Technology in Eastern Mountainous Mountains of Jilin Province (20220202097NC); Jilin Scientific and Technological Development Program: Jilin Province Degraded Forest Ecosystem Restoration and Reconstruction Cross-regional Cooperation Science and Technology Innovation Center (20200602006ZP); Jilin Scientific and Technological Development Program: Research and demonstration of key Technologies for Ecological Restoration of degraded Natural Forest ecosystem (20190303072SF).
We thank numerous who contributed to data collecting. We also thank the editors and reviewers for their very helpful comments.
LZ is employed by Shenyang Forestry Management Institute of China Railway Shenyang Bureau Group Co. Ltd. GL is employed by Nanjing Jialin System Engineering Ltd.
The remaining authors declare that the research was conducted in the absence of any commercial or financial relationships that could be construed as a potential conflict of interest.
All claims expressed in this article are solely those of the authors and do not necessarily represent those of their affiliated organizations, or those of the publisher, the editors and the reviewers. Any product that may be evaluated in this article, or claim that may be made by its manufacturer, is not guaranteed or endorsed by the publisher.
The Supplementary material for this article can be found online at: https://www.frontiersin.org/articles/10.3389/fevo.2023.1110594/full#supplementary-material
Administration, C.S.F (2014). “Technical provisions for continuous inventory of National Forest Resources ”. (Beijing: China Forestry Publishing House).
Ameray, A., Bergeron, Y., Valeria, O., Montoro Girona, M., and Cavard, X. (2021). Forest carbon management: a review of Silvicultural practices and management strategies across boreal, temperate and tropical forests. Curr. For. Rep. 7, 245–266. doi: 10.1007/s40725-021-00151-w
Anderegg, W. R. L., Chegwidden, O. S., Badgley, G., Trugman, A. T., Cullenward, D., Abatzoglou, J. T., et al. (2022). Future climate risks from stress, insects and fire across US forests. Ecol. Lett. 25, 1510–1520. doi: 10.1111/ele.14018
Broadstock, D., Ji, Q., Managi, S., and Zhang, D. (2021). Pathways to carbon neutrality: challenges and opportunities. Resour. Conserv. Recycl. 169:105472. doi: 10.1016/j.resconrec.2021.105472
Busch, J., Engelmann, J., Cook-Patton, S. C., Griscom, B. W., Kroeger, T., Possingham, H., et al. (2019). Potential for low-cost carbon dioxide removal through tropical reforestation. Nat. Clim. Chang. 9, 463–466. doi: 10.1038/s41558-019-0485-x
Cai, W., He, N., Li, M., Xu, L., Wang, L., Zhu, J., et al. (2022). Carbon sequestration of Chinese forests from 2010 to 2060: spatiotemporal dynamics and its regulatory strategies. Sci. Bull. 67, 836–843. doi: 10.1016/j.scib.2021.12.012
Canadell, J. G., and Raupach, M. R. (2008). Managing forests for climate change mitigation. Science 320, 1456–1457. doi: 10.1126/science.1155458
Ceccherini, G., Duveiller, G., Grassi, G., Lemoine, G., Avitabile, V., Pilli, R., et al. (2020). Abrupt increase in harvested forest area over Europe after 2015. Nature 583, 72–77. doi: 10.1038/s41586-020-2438-y
Chen, S., Lu, N., Fu, B., Wang, S., Deng, L., and Wang, L. (2022). Current and future carbon stocks of natural forests in China. For. Ecol. Manag. 511:120137. doi: 10.1016/j.foreco.2022.120137
China, S.F.A.O (2014). Forest resource statistics of China (2009–2013). Beijing: China Forestry Publishing House.
Commission, N.D.a.r (2014). National Greenhouse gas Inventory Report of China (2005). Beijing: China Environmental Science Press.
Dai, L., Li, S., Zhou, W., Qi, L., Zhou, L., Wei, Y., et al. (2018). Opportunities and challenges for the protection and ecological functions promotion of natural forests in China. For. Ecol. Manag. 410, 187–192. doi: 10.1016/j.foreco.2017.09.044
Dalmonech, D., Marano, G., Amthor, J. S., Cescatti, A., Lindner, M., Trotta, C., et al. (2022). Feasibility of enhancing carbon sequestration and stock capacity in temperate and boreal European forests via changes to management regimes. Agric. For. Meteorol. 327:109203. doi: 10.1016/j.agrformet.2022.109203
Fang, J., Chen, A., Peng, C., Zhao, S., and Ci, L. (2001). Changes in forest biomass carbon storage in China between 1949 and 1998. Science 292, 2320–2322. doi: 10.1126/science.1058629
Fekete, I., Lajtha, K., Kotroczó, Z., Várbíró, G., Varga, C., Tóth, J. A., et al. (2017). Long-term effects of climate change on carbon storage and tree species composition in a dry deciduous forest. Glob. Chang. Biol. 23, 3154–3168. doi: 10.1111/gcb.13669
Feng, Y., Schmid, B., Loreau, M., Forrester, D. I., Fei, S., Zhu, J., et al. (2022). Multispecies forest plantations outyield monocultures across a broad range of conditions. Science 376, 865–868. doi: 10.1126/science.abm6363
Harmon, M. E., Ferrell, W. K., and Franklin, J. F. (1990). Effects on carbon storage of conversion of old-growth forests to young forests. Science 247, 699–702. doi: 10.1126/science.247.4943.699
Hawes, M. (2018). Planting carbon storage. Nat. Clim. Chang. 8, 556–558. doi: 10.1038/s41558-018-0214-x
He, N., Wen, D., Zhu, J., Tang, X., Xu, L., Zhang, L., et al. (2017). Vegetation carbon sequestration in Chinese forests from 2010 to 2050. Glob. Chang. Biol. 23, 1575–1584. doi: 10.1111/gcb.13479
Huang, L., Liu, J., Shao, Q., and Xu, X. (2012). Carbon sequestration by forestation across China: past, present, and future. Renew. Sust. Energ. Rev. 16, 1291–1299. doi: 10.1016/j.rser.2011.10.004
Hubau, W., Lewis, S. L., Phillips, O. L., Affum-Baffoe, K., Beeckman, H., Cuní-Sanchez, A., et al. (2020). Asynchronous carbon sink saturation in African and Amazonian tropical forests. Nature 579, 80–87. doi: 10.1038/s41586-020-2035-0
IPCC (2004). Annex II: glossary Climate change 2014: Synthesis report. Contribution of working groups I, II and III to the fifth assessment report of the intergovernmental panel on climate change K. J. Mach, S. Planton, and C. von Stechow (IPCC, Geneva, Switzerland) 151.
Jiang, M., Medlyn, B. E., Drake, J. E., Duursma, R. A., Anderson, I. C., Barton, C. V. M., et al. (2020). The fate of carbon in a mature forest under carbon dioxide enrichment. Nature 580, 227–231. doi: 10.1038/s41586-020-2128-9
Keith, H., Mackey, B. G., and Lindenmayer, D. B. (2009). Re-evaluation of forest biomass carbon stocks and lessons from the world’s most carbon-dense forests. Proc. Natl. Acad. Sci. U. S. A. 106, 11635–11640. doi: 10.1073/pnas.0901970106
Kreier, F. (2022). Tropical forests have big climate benefits beyond carbon storage. Nature. doi: 10.1038/d41586-022-00934-6
Kurz, W. A., Dymond, C. C., Stinson, G., Rampley, G. J., Neilson, E. T., Carroll, A. L., et al. (2008). Mountain pine beetle and forest carbon feedback to climate change. Nature 452, 987–990. doi: 10.1038/nature06777
Lasslop, G., Hantson, S., Harrison, S. P., Bachelet, D., Burton, C., Forkel, M., et al. (2020). Global ecosystems and fire: multi-model assessment of fire-induced tree-cover and carbon storage reduction. Glob. Chang. Biol. 26, 5027–5041. doi: 10.1111/gcb.15160
Li, Y., Bao, W., Bongers, F., Chen, B., Chen, G., Guo, K., et al. (2019). Drivers of tree carbon storage in subtropical forests. Sci. Total Environ. 654, 684–693. doi: 10.1016/j.scitotenv.2018.11.024
Li, Y., Brando, P. M., Morton, D. C., Lawrence, D. M., Yang, H., and Randerson, J. T. (2022). Deforestation-induced climate change reduces carbon storage in remaining tropical forests. Nat. Commun. 13:1964. doi: 10.1038/s41467-022-29601-0
Li, L., Ye, F., Li, Y., and Chang, C.-T. (2019). How will the Chinese certified emission reduction scheme save cost for the national carbon trading system? J. Environ. Manag. 244, 99–109. doi: 10.1016/j.jenvman.2019.04.100
Liu, G., Han, S., Zhao, X., Nelson, J. D., Wang, H., and Wang, W. (2006). Optimisation algorithms for spatially constrained forest planning. Ecol. Model. 194, 421–428. doi: 10.1016/j.ecolmodel.2005.10.028
Lu, F., Hu, H., Sun, W., Zhu, J., Liu, G., Zhou, W., et al. (2018). Effects of national ecological restoration projects on carbon sequestration in China from 2001 to 2010. Proc. Natl. Acad. Sci. U. S. A. 115, 4039–4044. doi: 10.1073/pnas.1700294115
Luo, W., Kim, H. S., Zhao, X., Ryu, D., Jung, I., Cho, H., et al. (2020). New forest biomass carbon stock estimates in Northeast Asia based on multisource data. Glob. Chang. Biol. 26, 7045–7066. doi: 10.1111/gcb.15376
Maia, V. A., Santos, A. B. M., De Aguiar-Campos, N., De Souza Cléber, R., De Oliveira, M. C. F., Coelho, P. A., et al. (2020). The carbon sink of tropical seasonal forests in southeastern Brazil can be under threat. Sci. Adv. 6:eabd4548. doi: 10.1126/sciadv.abd4548
Münnich Vass, M. (2017). Renewable energies cannot compete with forest carbon sequestration to cost-efficiently meet the EU carbon target for 2050. Renew. Energy 107, 164–180. doi: 10.1016/j.renene.2017.01.034
Nabuurs, G.-J., Lindner, M., Verkerk, P. J., Gunia, K., Deda, P., Michalak, R., et al. (2013). First signs of carbon sink saturation in European forest biomass. Nat. Clim. Chang. 3, 792–796. doi: 10.1038/nclimate1853
Nelson, R., Margolis, H., Montesano, P., Sun, G., Cook, B., Corp, L., et al. (2017). Lidar-based estimates of aboveground biomass in the continental US and Mexico using ground, airborne, and satellite observations. Remote Sens. Environ. 188, 127–140. doi: 10.1016/j.rse.2016.10.038
Odum Eugene, P. (1969). The strategy of ecosystem development. Science 164, 262–270. doi: 10.1126/science.164.3877.262
Pan, Y., Birdsey, R. A., Fang, J., Houghton, R., Kauppi, P. E., Kurz, W. A., et al. (2011). A large and persistent carbon sink in the world’s forests. Science 333, 988–993. doi: 10.1126/science.1201609
Pan, Y., Luo, T., Birdsey, R., Hom, J., and Melillo, J. (2004). New estimates of carbon storage and sequestration in China’S forests: effects of age–class and method on inventory-based carbon estimation. Clim. Chang. 67, 211–236. doi: 10.1007/s10584-004-2799-5
Paul, T., Kimberley, M. O., and Beets, P. N. (2021). Natural forests in New Zealand – a large terrestrial carbon pool in a national state of equilibrium. For. Ecosyst. 8, 1–21. doi: 10.1186/s40663-021-00312-0
Phillips, C. A., Rogers, B. M., Elder, M., Cooperdock, S., Moubarak, M., Randerson, J. T., et al. (2022). Escalating carbon emissions from north American boreal forest wildfires and the climate mitigation potential of fire management. Sci. Adv. 8:eabl7161. doi: 10.1126/sciadv.abl7161
Piao, S., He, Y., Wang, X., and Chen, F. (2022a). Estimation of China’s terrestrial ecosystem carbon sink: methods, progress and prospects. Sci. China Earth Sci. 65, 641–651. doi: 10.1007/s11430-021-9892-6
Piao, S., Yue, C., Ding, J., and Guo, Z. (2022b). Perspectives on the role of terrestrial ecosystems in the ‘carbon neutrality’ strategy. Sci. China Earth Sci. 65, 1178–1186. doi: 10.1007/s11430-022-9926-6
Pregitzer, K. S., and Euskirchen, E. S. (2004). Carbon cycling and storage in world forests: biome patterns related to forest age. Glob. Chang. Biol. 10, 2052–2077. doi: 10.1111/j.1365-2486.2004.00866.x
Qie, L., Lewis, S. L., Sullivan, M. J. P., Lopez-Gonzalez, G., Pickavance, G. C., Sunderland, T., et al. (2017). Long-term carbon sink in Borneo’s forests halted by drought and vulnerable to edge effects. Nat. Commun. 8:1966. doi: 10.1038/s41467-017-01997-0
Reichstein, M., Bahn, M., Ciais, P., Frank, D., Mahecha, M. D., Seneviratne, S. I., et al. (2013). Climate extremes and the carbon cycle. Nature 500, 287–295. doi: 10.1038/nature12350
Seidl, R., Schelhaas, M. J., Rammer, W., and Verkerk, P. J. (2014). Increasing forest disturbances in Europe and their impact on carbon storage. Nat. Clim. Chang. 4, 806–810. doi: 10.1038/nclimate2318
Taeroe, A., Mustapha, W. F., Stupak, I., and Raulund-Rasmussen, K. (2017). Do forests best mitigate CO2 emissions to the atmosphere by setting them aside for maximization of carbon storage or by management for fossil fuel substitution? J. Environ. Manag. 197, 117–129. doi: 10.1016/j.jenvman.2017.03.051
Tang, X., Zhao, X., Bai, Y., Tang, Z., Wang, W., Zhao, Y., et al. (2018). Carbon pools in China’s terrestrial ecosystems: new estimates based on an intensive field survey. Proc. Natl. Acad. Sci. U. S. A. 115, 4021–4026. doi: 10.1073/pnas.1700291115
UNFCCC (2019). Climate ambition alliance: nations renew their push to upscale action by 2020 and achieve net zero CO2 emissions by 2050. (UNFCCC, Madrid, Bonn).
Veldkamp, E., Schmidt, M., Powers, J. S., and Corre, M. D. (2020). Deforestation and reforestation impacts on soils in the tropics. Nat. Rev. Earth Environ. 1, 590–605. doi: 10.1038/s43017-020-0091-5
Walker, W. S., Gorelik, S. R., Baccini, A., Aragon-Osejo, J. L., Josse, C., Meyer, C., et al. (2020). The role of forest conversion, degradation, and disturbance in the carbon dynamics of Amazon indigenous territories and protected areas. Proc. Natl. Acad. Sci. U. S. A. 117, 3015–3025. doi: 10.1073/pnas.1913321117
Wang, K., Hu, D., Deng, J., Shangguan, Z., and Deng, L. (2018). Biomass carbon storages and carbon sequestration potentials of the grain for green program-covered forests in China. Ecol. Evol. 8, 7451–7461. doi: 10.1002/ece3.4228
Xu, B., Guo, Z., Piao, S., and Fang, J. (2010). Biomass carbon stocks in China’s forests between 2000 and 2050: a prediction based on forest biomass-age relationships. Sci. China Life Sci. 53, 776–783. doi: 10.1007/s11427-010-4030-4
Yang, Y., Shi, Y., Sun, W., Chang, J., Zhu, J., Chen, L., et al. (2022). Terrestrial carbon sinks in China and around the world and their contribution to carbon neutrality. Sci. China Life Sci. 65, 861–895. doi: 10.1007/s11427-021-2045-5
Yao, Y., Piao, S., and Wang, T. (2018). Future biomass carbon sequestration capacity of Chinese forests. Sci. Bull. 63, 1108–1117. doi: 10.1016/j.scib.2018.07.015
Ye, F., Xiong, X., Li, L., and Li, Y. (2021). Measuring the effectiveness of the Chinese certified emission reduction scheme in mitigating CO2 emissions: a system dynamics approach. J. Clean. Prod. 294:125355. doi: 10.1016/j.jclepro.2020.125355
Zhang, C., Ju, W., Chen, J. M., Wang, X., Yang, L., and Zheng, G. (2015). Disturbance-induced reduction of biomass carbon sinks of China’s forests in recent years. Environ. Res. Lett. 10:114021. doi: 10.1088/1748-9326/10/11/114021
Zhou, W., Lewis, B. J., Wu, S., Yu, D., Zhou, L., Wei, Y., et al. (2014). Biomass carbon storage and its sequestration potential of afforestation under natural forest protection program in China. Chin. Geogr. Sci. 24, 406–413. doi: 10.1007/s11769-014-0702-5
Keywords: forest carbon storage, carbon sequestration, forest carbon modeling, national and provincial scale, spatial–temporal distribution
Citation: Cheng F, Tian J, He J, He H, Liu G, Zhang Z and Zhou L (2023) The spatial and temporal distribution of China’s forest carbon. Front. Ecol. Evol. 11:1110594. doi: 10.3389/fevo.2023.1110594
Received: 29 November 2022; Accepted: 31 January 2023;
Published: 20 February 2023.
Edited by:
Cuicui Jiao, Sichuan University of Science and Engineering, ChinaReviewed by:
Xulin Guo, University of Saskatchewan, CanadaCopyright © 2023 Cheng, Tian, He, He, Liu, Zhang and Zhou. This is an open-access article distributed under the terms of the Creative Commons Attribution License (CC BY). The use, distribution or reproduction in other forums is permitted, provided the original author(s) and the copyright owner(s) are credited and that the original publication in this journal is cited, in accordance with accepted academic practice. No use, distribution or reproduction is permitted which does not comply with these terms.
*Correspondence: Guoliang Liu, ✉ Z2xpdUBhaXRyZWUubHRk; Zhonghui Zhang, ✉ enpoZ2lzenpoQDE2My5jb20=
†These authors have contributed equally to this work and share first authorship
Disclaimer: All claims expressed in this article are solely those of the authors and do not necessarily represent those of their affiliated organizations, or those of the publisher, the editors and the reviewers. Any product that may be evaluated in this article or claim that may be made by its manufacturer is not guaranteed or endorsed by the publisher.
Research integrity at Frontiers
Learn more about the work of our research integrity team to safeguard the quality of each article we publish.