- 1Laboratoire PALEVOPRIM, Université de Poitiers, Poitiers, France
- 2Department of Geosciences, Eberhard Karls Universität Tübingen, Tübingen, Germany
- 3Yangon University of Distance Education, Yangon, Myanmar
- 4Mandalay University of Distance Education, Mandalay, Myanmar
- 5Senckenberg Centre for Human Evolution and Palaeoenvironment at the University of Tübingen, Tübingen, Germany
The late Middle Eocene Pondaung Fm. is a window to understand the environment and ecosystem dynamics of a past greenhouse world and the paleoenvironments where modern mammal clades such as anthropoid primates originated. Previous studies focused on the overall climate and vegetation of this Eocene habitat and provided first evidence for an early monsoon onset before the orogenesis of the Himalayan-Tibetan range. Here, we wanted to investigate how the mammal fauna used this habitat and which different ecological niches and microhabitats they occupied. We analyzed the carbonate fraction of dental enamel of a fossil mammal assemblage from various localities of the Pondaung Fm. in Myanmar. Bayesian niche modelling of the δ13C and δ18O values allowed us to quantify aspects of the ecological core niches occupied by these taxa, to calculate niche overlap and to use these data to infer directional competition potential in this mammal assemblage. Furthermore, comparison of different areas of the Pondaung Fm. revealed two different microhabitats whose distribution is consistent with existing vegetation models. Most primate taxa were found in both described environments, which gives a first indication about their ecological flexibility.
1. Introduction
The climate today is changing fast and towards conditions never before experienced by humans. Some geological time periods might however be important analogues for possible climate scenarios in the future. One of these epochs is the Eocene that spans from 56 to 34 Ma (million years ago). The Eocene Earth was a greenhouse world with mean annual temperatures around 5°C higher than today in the Late Eocene (Zachos et al., 2001; Pagani et al., 2005). It was a world not unlike the possible man-made greenhouse world in our future (Burke et al., 2018; Tierney et al., 2020). Studying this time period, the paleoenvironments, and the organization of fossil mammal communities can therefore provide valuable information to better understand various scenarios of future environments on Earth. In addition, it was also the period when modern mammals started their ecological radiation (e.g., Coster et al., 2018; Ducrocq et al., 2019; Jaeger et al., 2020). Here we want to focus on one mammal community, characterize its habitat and niche partitioning and explore if and to what extent approaches from ecology such as directional competition potential and individual specialization can be applied to paleoecology.
The mammal assemblage we focus on is from the late Middle Eocene Pondaung Formation (Fm.) located in modern day Myanmar. Southeast Asia is a hotspot for understanding mammal evolution during that time, as many radiations of important clades such as early anthropoid primates (Jaeger et al., 2020) and anthracotheres that are believed to be ancestral to the extant hippopotamids (Lihoreau et al., 2015), but also ruminants, pigs and hystricognath and anomalurid rodents took place there (Marivaux et al., 2005; Beard, 2016; Coster et al., 2018). The Pondaung Fm. is one of the fossil formations that document the rich biodiversity in this important biogeographic region. In this study we report the first substantial sample of isotopic data from herbivorous mammals and we correlate it to previously studied paleoenvironmental proxies. Our research questions can be structured around three topics: paleoseasonality, microhabitat differences, and niche partitioning from which we try to infer competition potential of the Pondaung mammal fauna with a focus on the Anthracotheriidae.
It has been thought that the Tibetan-Himalayan orogenesis was a prerequisite for a strong monsoonal climate in Southeast Asia, which could therefore not have originated earlier than 25 to 22 Ma. Studies on different climatic proxies including stable isotope analysis of gastropod shells and mammal teeth from the Pondaung Fm. revealed however that a monsoon-like climate was already present in the Eocene (Licht et al., 2014c; Toumoulin et al., 2022; Huang et al., 2023). With our larger data set, covering some of the localities represented in the previous study by Licht et al. (2014c) and more, we will try to test whether the same pattern is present. Furthermore, we investigated possibilities to compare monsoon intensity. For this we are comparing the Eocene data to a specimen of modern Bos from the Central Myanmar Basin (Habinger et al., 2022). δ18O values of the Eocene mammals as low or lower than in the modern specimen together with a sinusoidal variation pattern would be evidence for a seasonal precipitation regime similar or even more pronounced as in Myanmar today. Our goal is not only to substantiate the hypothesis of an early onset Eocene monsoon-like precipitation regime, but also to characterize the environmental conditions linked to this kind of climate in more detail.
The localities of the Pondaung Fm. represent a terrestrial paleoecosystem with several river channels. They are situated in the Central Myanmar Basin (17–21°N, 92–96°E) west of the Chindwin River and northwest of the Irrawaddy River. However, in the late Middle Eocene the Pondaung Fm. was part of the Trans-Tethyan arc and therefore located farther south close to the equator (Westerweel et al., 2019, 2020). According to paleoenvironmental reconstructions, the ancient Eocene coastline with its mangrove forests lay farther to the west of the fossil localities. The vertebrate fossil bearing area was located further inland in a deltaic plain with reconstructed environments ranging from littoral to riparian forests and open-forested seasonal wetlands to dry dipterocarp and Schima forests in the upstream areas (Licht et al., 2013, 2014a, 2015; Gentis et al., 2021). Morphological adaptations to leaping in the primate taxa suggest that the Eocene forest density was high enough to allow for such a locomotive repertoire for example in the medium sized sivaladapid Kytchaungia takaii (body mass 1.6–1.8 kg; Kay et al., 2004; Beard et al., 2007; Marivaux et al., 2008a,b). The elevated temperatures during the Eocene led to a northward expansion of tropical and subtropical climatic zones, where rainforests could grow (Morley, 2018). We will test if microhabitat differences between the three geographic clusters of localities—the Bahin, Pangan, and Mogaung areas (Figure 1)—can be detected. Based on their geographic distribution and the vegetation model published by Licht et al. (2015) we have formulated two hypotheses. First, as the Mogaung area is further upstream the paleoriver system we are expecting the habitat there to be more densely forested and humid, as suggested by the different modelled vegetation regimes described above. Thus, both the δ13C and δ18O values of the mammals from this areas should be lower than at the other two areas. Second, should we detect microhabitat differences the probability that these differences are in fact correlated with spatial factors and not differences in exact dating of the localities of the different areas rise, if the Bahin and Pangan areas are more similar to one another than to the Mogaung area, and if they exhibit higher δ13C and δ18O values than the mammals from Mogaung.
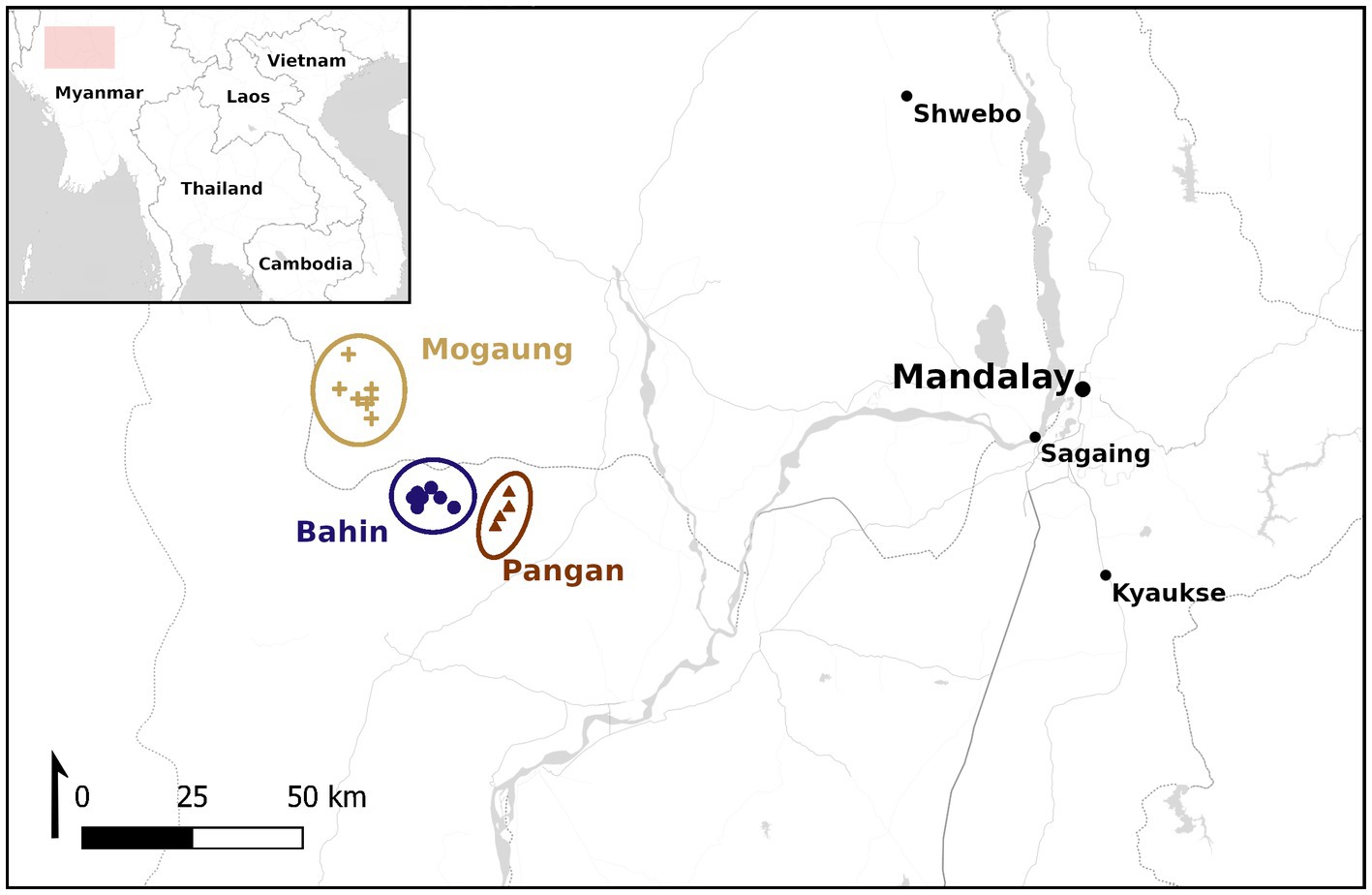
Figure 1. Map showing the location of the different localities of the Pondaung Fm. and their division into the three area clusters. Coordinates for all the localities from which samples were analyzed in this study are reported in Supplementary Table SI 1. The location of the detailed map is marked in the overview with a colored rectangle. Map tiles by Stamen Design, under CC BY 3.0. Data by OpenStreetMap, under ODbL.
As described above, the paleoenvironment of the Pondaung Fm. has already been characterized using different approaches, from ecomorphology to vegetation modelling based on fossil plant remains (Licht et al., 2015). However, to our knowledge no studies on niche partitioning and habitat use of this mammal fauna have been conducted yet. Increasing our knowledge on the organization of this fossil mammals has also implications on our knowledge about the palaeoecology of the earliest anthropoid primates, which makes studying it so important. Though these primates are not directly part of the data set used in this study, they were part of this same fossil mammal assemblage occurring sympatric with them in all three areas with fossil localities (see Supplementary Table SI 4). Reconstructing the paleoecology and niche partitioning among other members of this mammal fauna forms the basis on which we can discuss the paleoecology of the anthropoid primates in more detail. With our approach we can model aspects of the ecological niche of a taxonomic group reflecting the habitat and its use as well as dietary ecology. Should we detect microhabitat differences, but have some of the fossil primates occur in more than one this indicates a certain degree of ecological flexibility in these taxonomic groups.
Many open questions remain also regarding the anthracotheres, a group widely considered to be ancestral to hippos (Lihoreau et al., 2015), which are of special interest given their abundance and diversity in the Pondaung mammal fauna and the possibility to detect an ecological transition from a fully terrestrial to a semi-aquatic lifestyle around that time. As of today, three genera are known in this fossil assemblage. The taxonomy of this clade has been discussed and revised several times (Tsubamoto et al., 2002; Lihoreau and Ducrocq, 2007; Soe, 2008). With our isotopic data, we want to characterize aspects of their ecological niches at the genus and species level to see if there are any notable differences. In addition, we will calculate the niche overlap and explore the possibility to infer directional competition potential from these calculations. To our knowledge, this approach used by ecologists (Ogloff et al., 2019) has not yet been applied to fossil mammal communities. We expect that anthracothere taxa with higher body mass share more of their niche space with other anthracotheres, as they can balance the higher degree of competition potential this implies with their size. For several medium to large sized anthracotheres from periods younger than the Eocene, such as Arretotherium, Merycopotamus, and Libycosaurus with body masses ranging from 150 to 1,000 kg, semi-aquatic lifestyle similar to extant hippos has been inferred (Orliac et al., 2013). They also displayed morphological adaptation to such a lifestyle that have often been referred to as hippo-ecomorph (Clementz et al., 2008), such as elevated orbits, external nares at the top of the snout, and massive limbs with high bone density (Lihoreau and Ducrocq, 2007). None of these morphological adaptations is present in the anthracotheres from the Pondaung Fm. We nevertheless wanted to test if their oxygen isotopic abundances already indicate semi-aquatic behavior before morphological adaptations to this lifestyle are visible. To do so we will test, if their δ18O values are lower than the ones of the other taxonomic groups and if the variation in these values is reduced. Similar studies have been conducted on Eocene early whales (Thewissen et al., 2007; Cooper et al., 2016).
To answer all of these questions, we conducted stable isotope analysis on the carbonate fraction of fossil tooth enamel on the terrestrial mammal fauna from the Pondaung Fm. Due to its high content of inorganic hydroxyapatite and the fine and dense crystalline structure, enamel is very resistant to diagenetic changes. In addition, the Pondaung sediments that we analyzed are low in carbonate content, thus reducing the probability of diagenetic alteration of the original biological isotopic signal in the fossil teeth (for details see Methods section).
2. Materials and methods
2.1. The geological context
The mammal fauna that we analyzed for this study originates from the Pondaung Fm. in the Central Basin of Myanmar. During annual fieldwork campaigns of the French-Myanmar expedition, the fossils were collected from different localities that can be attributed to one of three areas—Bahin, Pangan, and Mogaung (Figure 1).
The Middle Eocene Pondaung Fm. is framed by the underlying Early Eocene Tabyin and the overlying Late Eocene Yaw Fm., both of which are marine deposits (Bender and Bannert, 1983). The Pondaung Fm. itself is up to 2 km thick (Bender and Bannert, 1983) with a succession of marine to terrestrial sediments (Stamp, 1922), but only the upper part (Upper Member) with a thickness of around 500 m (Oo et al., 2015) yielded significant amount of fossils representing a terrestrial mammal fauna. The stratigraphic positions of the different fossil localities to one another has not been studied in depth as of today. Nevertheless, the fossiliferous sediments of some localities from the Bahin area (PK1, PK2, PK3, PK4, PK5, and PK8) belong to the same traceable claystone named Ayoedawpon Taung Claystone ranging in thickness from 8 to 20 m. It is underlain directly by another widely traceable sediment, the Ayoedawpon Taung Sandstone. For two other fossil localities from the same region, the stratigraphic relationship is well constrained. The fossil outcrops at PK9 are part of the Nyaungpinle Claystone, which is stratigraphically lower than the Ayoedawpon Taung Claystone. The claystones at the PK12 locality on the other hand overlay directly the sandstones above the Ayoedawpon Taung Claystone (Maung et al., 2005; Suzuki et al., 2006). It has been dated to 40.31 ± 0.65 Ma and 40.22 ± 0.86 Ma using LA-ICP-MS, U–Pb zircons on tuffaceous sandstones from the Bahin area (PK4 and PK8; Zaw et al., 2014). This means that the Pondaung Fm. dates to around 40 Ma, which corresponds to the late Middle Eocene or Bartonian.
Its depositional environment was characterized by fluvial sediments of meandering river channels and is mostly comprised of sandstone with interspersed silt/clay bands. Fossils are mostly associated with lithofacies indicative of small fluvial or crevasse channels, swale fills and point bar deposits. Some evidence of peat layers and coal seams might indicate peat swamp formation of the inter-channel areas (Soe et al., 2002).
In their magnetostratigraphic study on a 319 m thick section of the Upper Member of the Pondaung Fm., Benammi et al. (2002) detected a single normal polarity. Although this homogenous paleomagnetism makes correlation with a specific Bartonian chron difficult, it indicates a high sedimentation rate of the floodplain (>0.3 mm yr.−1; Licht et al., 2014b) as the maximum length of the possible Bartonian polar chron is smaller than 1 Myr (Benammi et al., 2002). This inference can be further substantiated by the results of another study on sedimentation rates in Asia (Métivier et al., 1999). Given this high sedimentation rate and the observed homogeneity of the fauna documented notably by rodents, amynodontids, anthracotheres and primates, we will consider that the Pondaung fossil remains correspond to a contemporaneous mammal fauna assemblage (Ducrocq et al., 2019). We give a detailed evaluation of sympatry of the different taxa in our sample per locality and area in Table SI 1.
2.2. The Pondaung mammal fauna
The fossil mammal fauna preserved in the Pondaung Fm. is famous for its unique and diverse primate assemblage. Two Sivaladapid adapiformes (Kyitchaungia takaii, and Paukkaungia parva), one Eosimiiforme (Afrasia djijidae), one Eosimiidae (Bahinia pondaungensis), a yet undescribed tarsid and most notably three different Amphipithecidae (Ganlea megacanina, Pondaungia cotteri, and Myanmarpithecus yarshensis) were found there (Marivaux et al., 2008b; Chaimanee et al., 2012; Fleagle, 2013; Jaeger et al., 2020). The phylogenetic position of the amphipithecids has been highly debated in the past century, but these primates are now firmly established as stem anthropoids by cranial, postcranial, and phylogenetic evidence (Jaeger et al., 2020). The high diversity in species underlines the importance of the Pondaung Fm. and Southeast Asia as the biogeographic region of early diversification and origin of this clade. This fact, together with the co-occurrence of three different stem anthropoid species together with eosimiids, which are basal anthropoids, and other primate taxa raises the interest in the paleoenvironment in which they all existed.
The diet of the Pondaung primates has been inferred from both dental morphology as well as dental microwear analysis. Pondaungia was likely predominantly frugivorous and also included some hard items in its diet. Myanmarpithecus subsisted on a mix of fruit and leaves (Kay et al., 2004; Ramdarshan et al., 2010). Although the unique tooth morphology of Ganlea complicates the dietary interpretations, this amphipithecid likely also ingested a mixture of leaves and fruit, but also engaged in seed predation (Beard et al., 2009; Jaeger et al., 2020). For the smaller primates Bahinia and Paukkaungia an insectivorous diet has been suggested (Kay et al., 2004; Ramdarshan et al., 2010). Only few primate postcranial remains have been found from the Pondaung Fm. Both Pondaungia and Ganlea have been described as active arboreal quadrupeds (Marivaux et al., 2003, 2010; Jaeger et al., 2020). For the Sivaladapidae a generalized locomotor profile as an arboreal quadruped has been suggested as well (Kay et al., 2004; Beard et al., 2007), with some evidence for leaping capabilities due to hip and pelvis morphology in K. takaii (Beard et al., 2007; Marivaux et al., 2008a,b). Given the small size of the teeth and their importance and rarity it was not possible to sample any of the Eocene anthropoid primates for stable isotope analysis.
Four large herbivore groups make up the majority of our sample of the Pondaung mammal fauna. The numbers in brackets correspond to the number of specimens sampled. There are three groups of perissodactyls, Brontotheriidae (n = 24), Amynodontidae (n = 16), Rhinocerotoidea indet. (n = 27), and the artiodactyl Anthracotheriidae (n = 56). Of these tooth specimens, 38 were sampled serially (9 Brontotheriidae, 9 Amynodontidae, 8 Rhinocerotoidea indet., and 12 Anthracotheriidae), hence providing a time series across the mineralization time of the fossil enamel allowing the investigation of seasonal variations. Brontotheriidae are described as being obligate browsers due to their brachydont teeth with bunoselenodont morphology and therefore preferring forest to woodland habitats in warm temperate to subtropical environments (Mader, 1998). Some of the brontotherids in our data set have been identified as Bunobrontops a more primitive genus in comparison to two other known genera, Metatelmatherium and Sivatitanops, from the Pondaung Fm. (Holroyd and Ciochon, 2000). Most of the Rhinocerotoidea are probably Amynodontidae, the most abundant family in the Pondaung Fm., but the fragmentary nature of the sampled fossils did not allow for an assignment of this taxonomic label with enough certainty. Previous work on fossil Rhinocerotoidea from Late Eocene sites in Vietnam characterized them as forest dwellers with their brachydont teeth and as being obligate browsers as well (Böhme et al., 2013). These brief descriptions of two of the main herbivorous taxonomic groups at the Pondaung Fm. reveal once more the lack of systematic studies especially on the Perissodactyl fauna. In addition, the tapiromorph Bahinolophus (n = 2), Eomoropidae (n = 1), and Ruminantia (n = 2) are also part of our data set. In four cases two teeth were sampled per individual (Siamotherium PNG-20 M2/M3 and PNG-141 M2/M2, Anthracokeryx tenuis PNG-22/23, Anthracotherium crassum PNG-56/57, and Amynodontidae PNG-92/93). In general, the ungulate fauna in the Pondaung mammal fauna is considered to be representative of the original living community, while a sampling bias is likely in the case of rodents and other small mammals including primates. This is due to the fieldwork method applied by the different teams of researchers, which mainly conduct surface prospecting in the area (Tsubamoto et al., 2005).
The Pondaung Fm. has also yielded some creodont (Egi and Tsubamoto, 2000; de Bonis et al., 2018), fish, reptile (crocodilian, lizard, and turtle; Hutchison et al., 2004; Tsubamoto et al., 2006a; Head et al., 2013) and bird fossils (Tsubamoto et al., 2006b). However, no extensive systematic studies on this material exist as of now and it has therefore not been included in our data set for isotopic analysis.
2.3. The anthracotheres
In the Pondaung Fm., we find a high diversity of anthracothere taxa. This comes as no surprise, as this taxonomic group likely originated in Southeast Asia and experienced a radiation there in the late Middle Eocene. Later on, anthracotheres spread from Asia to Europe, Africa, and North America. Three different anthracothere genera are known from the Pondaung Fm.—Anthracotherium, Anthracokeryx, and Siamotherium. In contrast to the perissodactyls from the Pondaung mammal fauna that were strictly herbivorous according to their tooth morphology, anthracotheres were more opportunistic feeders. They likely had a much more diversified diet that included leaves, fruits, roots, and invertebrates.
Anthracotherium (n = 20) was a medium to large sized anthracothere and the largest of the three genera found at the Pondaung Fm. It has bunodont teeth suitable for crushing tougher food like roots or even scavenge carcasses from time to time. Hence, its cranio-dental morphology indicates a more generalist diet not unlike the one of extant pigs (Ducrocq, 1999). Therefore, we hypothesize that it occupied more open forests than the other two and probably lived closer to the river system, meaning that it was more water dependent than the other anthracothere genera known from the Pondaung Fm. Two species could be identified in our sample, the smaller A. crassum (n = 5) and the larger A. pangan (n = 11).
The second genus, Anthracokeryx (n = 13) with the two species, the larger Anthracokeryx birmanicum (n = 4) and the smaller A. tenuis (n = 4), represents a more typical forest dweller with bunoselenodont teeth. These are typical for a more specialized herbivorous foraging ecology (Ducrocq, 1999). It has a body mass, of about 20–25 kg (Lihoreau and Ducrocq, 2007). This and its cranio-dental morphology are consistent with dwelling in denser forests and having a more specialized folivorous diet.
Siamotherium (n = 4) is the smallest and most primitive of the three genera with an estimated body mass of 7.5 kg for S. pondaungensis. It was a small, slender animal with a short snout showing first signs of selenodonty on its molars (Ducrocq et al., 2001; Soe et al., 2017; Ducrocq et al., 2021). Its subsistence strategy has been described as more omnivorous including occasional scavenging, and it is thought to have lived in open forested areas (Soe et al., 2017). For this study, we could sample four teeth from two individuals.
2.4. Sample preparation and analysis
We sampled the specimens during a museum visit to the National Museum in Nay Pyi Taw in February 2020 and the fieldwork campaign in the following weeks. The specimens were cleaned from dust and sediment using ethanol and the outermost layer of fossil enamel was removed on the sampling site as these would be most susceptible to diagenetic alteration. During sampling 6–12 mg of fossil enamel powder were drilled using a portable diamond coated micro drill station. Dentine samples from these specimens were also sampled using the same sampling methodology. Sediment attached to the specimens was also collected in some instances, depending on availability. Both of these tissue types were used to assess diagenesis (see SI). They are reported in Supplementary Table SI 3.
The pretreatment and analysis in the IRMS (isotopic ratio mass spectrometer) was conducted in the laboratories of the Biogeology working group (Department of Geosciences) at the University of Tübingen using a modified Koch method (Bocherens et al., 1994; Koch et al., 1997; Wright and Schwarcz, 1999). We added 1.35 ml of NaOCl at a concentration of 2.5% to each of the powdered samples and left it react for 24 h to remove organic matter that might be present in the sample. Then the liquid was removed and after rinsing the samples three times with distilled water, 1.35 ml of 1 M acetic acid buffer solution (CH3COOH) was added to the enamel and dentine samples and let to react with the samples for 24 h. Given difference in crystalline structure between enamel and other exogenous sources of carbonates, the latter ones were removed by this step. The samples were once again rinsed three times with distilled water and then dried at 35°C for 72 h. With each set of samples two internal standards of elephant and hippo enamel were treated following the same protocol. Prior to analysis one internal (LM = Laaser Marmor) and two international (IAEA-603, NBS-18) pure carbonate standards were added to the data set after every 15 samples. These were not subjected to any pretreatment prior to analysis.
For the analysis with the Elementar IsoPrime 100 IRMS 2.5–3 mg of enamel or 0.1 mg of pure carbonate were reacted with 99% phosphoric acid (H3PO4) at 70°C for 4 h. The isotopic ratios of the resulting CO2 gas were measured four times for each sample over a time span of 15 min. This allowed us to monitor measurement precision by calculating the mean and standard deviation of these repeated measurements for each sample (Szpak et al., 2017). In addition, measurement uncertainty was assessed using the standards. It is reported for each sample in Supplementary Table SI 2. Isotopic ratios are reported using the δ-notation (in per mill). The calculation is based on formula (1) (Coplen, 2011; Bond and Hobson, 2012) where j marks the heavier and i the lighter isotope.
With the internal enamel standards the isotopic ratios were calibrated relative to VPDB (Vienna Pee Dee Belemnite). Oxygen isotopic ratios have been subsequently converted to δ18OVSMOW values (Vienna Mean Ocean Water) using the formula (2) by (Coplen, 1988).
In Supplementary Tables SI 2, SI 3 we also report the CaCO3 content of each sample. It was calculated with the Ion Vantage software based on an estimated elemental composition with sample weight, peak area and the internal LM standard as variables. The resulting CaCO3 values are subsequently scaled up until one of the international pure carbonate standards reaches 100%. CaCO3 content of sediment samples from various Pondaung localities was too low when we used the regular amount of 0.1 mg of powdered sediment sample in the IRMS. Even when we raised the sample size to 2.5–3 mg of powdered sample, CaCO3 content remained very low for the Pondaung sediments (Supplementary Table SI 3). We discuss the results of the sediment and dentine samples in the Supplementary Information to evaluate the risk of diagenetic alteration of the original biogenic isotopic signal in our data set.
2.5. Data correction and statistical analysis
Prior to data analysis and isotopic niche modelling two types of data corrections were applied to account for differences in enrichment of δ13C values between diet and bioapatite as well as changing δ13C baselines, due to shifts of the isotopic composition of CO2 in the atmosphere. All the data before and after the corrections were applied as well as the specific enrichment factor used to calculate δ13Cdiet values are reported in Table 1.
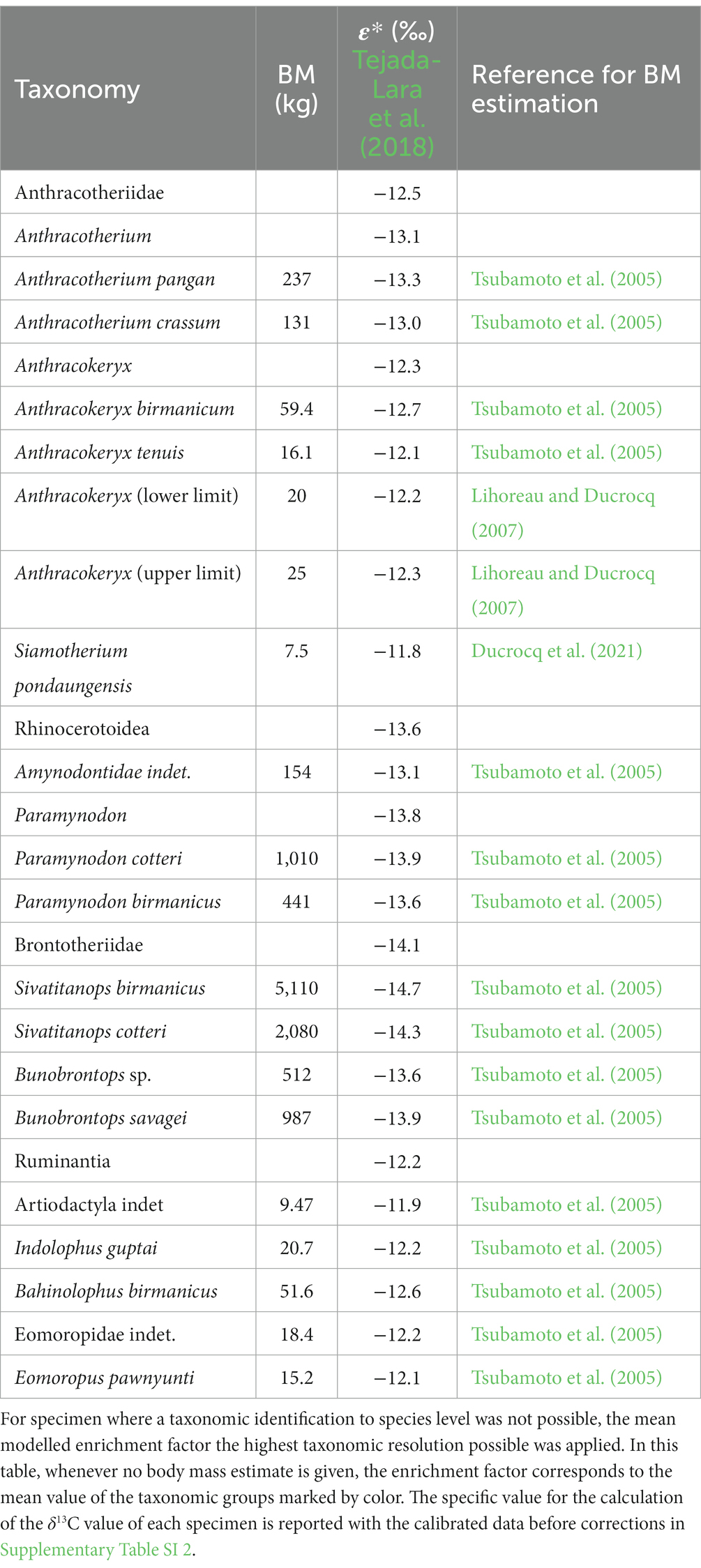
Table 1. Summary of the body mass (BM) estimates and the modelled isotopic enrichment factor based on formula (3).
In the past one uniform enrichment factor of 14‰ for the carbon isotope ratio from diet to apatite in dental enamel has been used for all medium to large bodied herbivores (Cerling and Harris, 1999). Recent studies however showed, that many different factors such as body mass and digestive physiology influence this enrichment factor (Passey et al., 2005; Tejada-Lara et al., 2018). Conducting an experimental study to establish a specific ε* for a species is only possible for extant animals. For our study we therefore apply the regression formula (3) proposed by Tejada-Lara et al. (2018) to account for differences of ε* due to variations in body mass. This is the conservative formula, which is not specific to foregut or hindgut fermenters.
Here, BM stands for the log transformed (ln) body mass in kg. The resulting enrichment factor is in per mill (‰), which can then be applied to the data prior to isotopic modelling (Table 1) to calculate δ13Cdiet values (4).
Instead of correcting the individual δ13C values for changes in δ13CCO2 of the atmosphere, which is the pool from which plants extract the carbon in their tissue during photosynthesis, we chose to apply the corrections to the thresholds used for the interpretation of vegetation type and density. Post-1,930 the δ13CCO2 values were −8‰ (Zachos et al., 2001; Cerling et al., 2010 but see Keeling et al., 2005 for fluctuations within this time period). Tipple et al. (2010) reconstructed Cenozoic δ13CCO2 values based on stable isotope analysis of benthic foraminifera. For the middle Eocene at 40 Ma their model suggest higher δ13CCO2 values than today with an estimate of −5.5‰ (ranging from −6‰ to −5.2‰ at a confidence interval of 90%). Thus, we corrected the modern thresholds by +2.5‰ before interpreting the Eocene data.
Following the Hutchinsonian niche concept (Hutchinson, 1957, 1978), we calculated core ecological niches for each taxonomic group using the R package SIBER (version 2.1.6; Jackson et al., 2011). For this study, we quantify niches using standard ellipse area corrected for small sample sizes (SEAC). They correspond to a confidence interval (CI) of 40%. As this modelling approach is based on a maximum likelihood estimation in a Bayesian framework, the limiting effect of small sample sizes on statistical power of our analysis is counteracted to a certain extent. Should the sample size of a taxonomic group be below five individuals we mention it explicitly during the interpretation of the results.
For the evaluation of niche packing and directional competition potential, we calculated three metrics to quantify niche overlap between two taxonomic groups. These are the percentage of the total niche space of the two taxa that is shared between them (% overlap), which is calculated with formula (5; Ogloff et al., 2019), the percentage of niche area of taxon A (AA) that overlaps the niche space of taxon B (AB), and vice versa. The latter two are reported as AA–AB and AB–AA, respectively. AO which is the area shared between taxon A and taxon B was acquired by using the maxLikOverlap() function of the SIBER package. AA and AB are calculated using AO and the unitary method.
Based on the modelled ecological niches we also calculated the relative individual niche (RINI; Sheppard et al., 2018) for the individuals from which serial samples were taken. RINI is a metric that relates the individual niche area to the population niche area. Here, it expresses the proportion the SEACs (CI = 40%) of an individual that is shared with the union of all the SEACs of the serially sampled individuals from a taxonomic group. For this we used the siberKapow() function that is part of the SIBER package. Code examples can be found in the explanatory vignettes at the SIBER Github repository.
Given the small sample sizes we are working with and the fact that normal distribution of our data cannot be attested in all cases non-parametric Wilcox rank sum tests are used to test for statistically significant differences in this study. To screen for relationships between δ13C and δ18O values in the specimen where intra-individual serial samples were taken, we performed Spearman correlation analyses. Habinger et al. (2022) identified one statistical outlier in the modern Bos data we also use in our study. This outlier was not included in the statistical analysis, but is shown in the plots. Statistical significance is ascribed to test results where p = <0.05. In cases where several statistical tests were run on the same data set, value of ps have been adjusted with the Bonferroni correction, a conservative method to correct for multiple comparisons (Bonferroni, 1936). All statistical tests and models were run in R version 4.1.2.
3. Results
A comparison of the δ18O values of the modern bovid from the Central Basin of Myanmar and the fossil mammals from the Pondaung Fm. showed a significant difference (W = 0, value of p <0.001), with the Eocene δ18O values being lower. Sinusoidal patterns were present in both the modern and the fossil specimen. Two of the 38 specimens that were sampled serially are plotted together with the modern Bos in Figure 2. Figures of all the serially sampled individuals are provided in the Supplementary Information. We also tested if there was a correlation between the δ13C and δ18O values, which would indicate a consistent influence of seasonal precipitation on the plants consumed. We found no constant relationship of these values across all the mammal data (rho = 0.10, S = 2,366,301, p = 0.106). However, when we sorted the specimen based on taxonomy (family level), we found significant positive correlation in Anthracotheriidae (rho = 0.56, S = 9,147.2, p = 2.28e-05) and Amynodontidae (rho = −0.37, S = 125,705, p = <0.001) as well as significant negative correlation in Rhinocerotoidea (rho = 0.33, S = 18,683, p = 0.015). Only in the Brontotheriidae no statistically significant correlation was present (rho = 0.1923064, S = 35,280, p = 0.128). The negative correlation in the modern Bos also was not statistically significant (rho = −0.34, S = 1,299, p = 0.170).
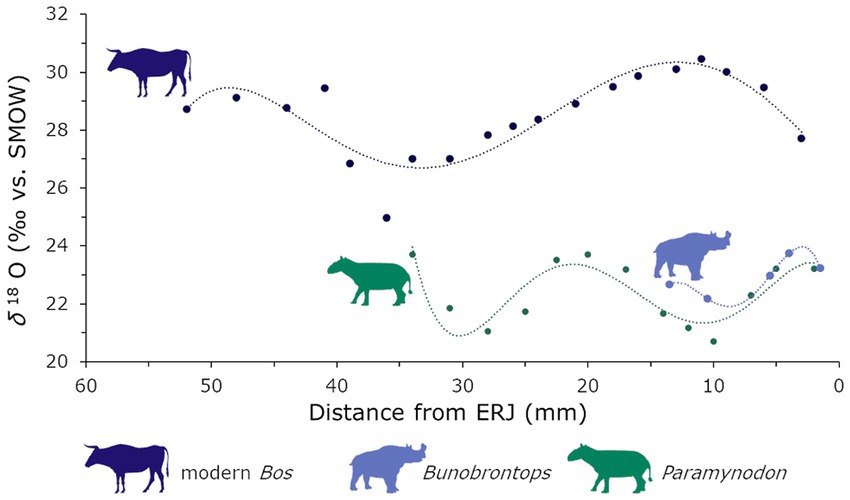
Figure 2. Visualization of some exemplary serially sampled specimen, the modern bovid and two of the Eocene specimen from the Pondaung Fm. (Bunobrontops PNG-47, Paramynodon PNG-119), illustrating different seasonal precipitation regimes. The distance from the ERJ (enamel root junction) on the x-axis has been inverted. This way it represents the values in an intuitive temporal sequence from the oldest ones to the left to the youngest ones to the right. The statistical outlier (lowest δ18O value) in the modern Bos is included in this figure, but has been excluded from the statistical analysis. Silhouettes via Phylopic under CC 3.0 license (modern Bos by T. Michael Keesey, Bunobrontops and Paramynodon by Zimices).
The different localities where the fossil specimens analyzed in this study have been found can be separated into three areas. Therefore, we wanted to test if the isotopic ratios indicate a difference in environment between them. Visual inspection of the δ13C and δ18O values per area (Figure 3) showed some inter-area differences. The comparison of δ13C and δ18O values from the Bahin and Pangan localities using Wilcox rank sum tests showed no significant difference (p = 1 in both cases). Hence, these two areas will be grouped together for the ecological niche modelling. We then conducted a Wilcox rank sum test, to see if the δ13C and δ18O values were significantly different in the Mogaung area than in the Bahin and Pangan ones. All of these test results showed significant differences (Mogaung—Bahin C: p = 0.001, O: p = 0.001; Mogaung—Pangan C: p = 0.003, O: p = 0.110). Hence, we modelled the ecological niches separately for the mammals from the Mogaung localities.
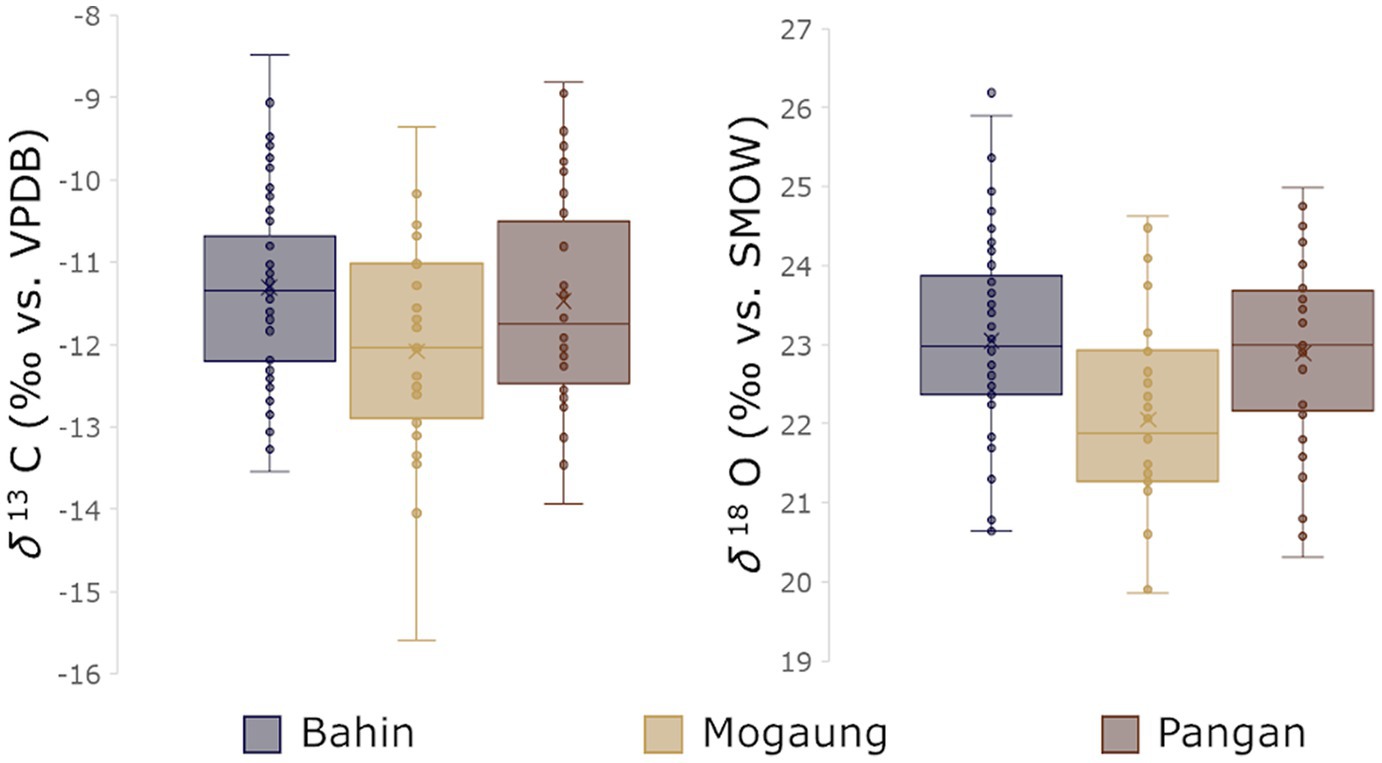
Figure 3. Box plots of the compiled δ13C (left) and δ18O (right) values per area. The Mogaung values are lower for both isotopes which is consistent with a difference in microhabitats.
The Bahin and Pangan areas (δ13C: −27.2 to −20.7‰; δ18O: 26.2 to 19.8‰) do not only have significantly higher δ13C and δ18O values, the variation of these data is also smaller than in the Mogaung area (δ13C: −29.2 to −21.2‰; δ18O: 25.9 to 18.1‰). Bulk and averaged serial sampling are visualized in Figure 4. Summary statistics for the mammal communities from the Bahin/Pangan and Mogaung area are reported in Table 2 and results of the niche overlap calculations in Table 3. The median % overlap of the Bahin/Pangan area is higher (17.8%) than in the Mogaung area (16.3%). This difference is not statistically significant (W = 9, value of p = 0.786). The median SEAC is almost identical in both areas with 3.6 in Bahin/Pangan and 3.5 in Mogaung.
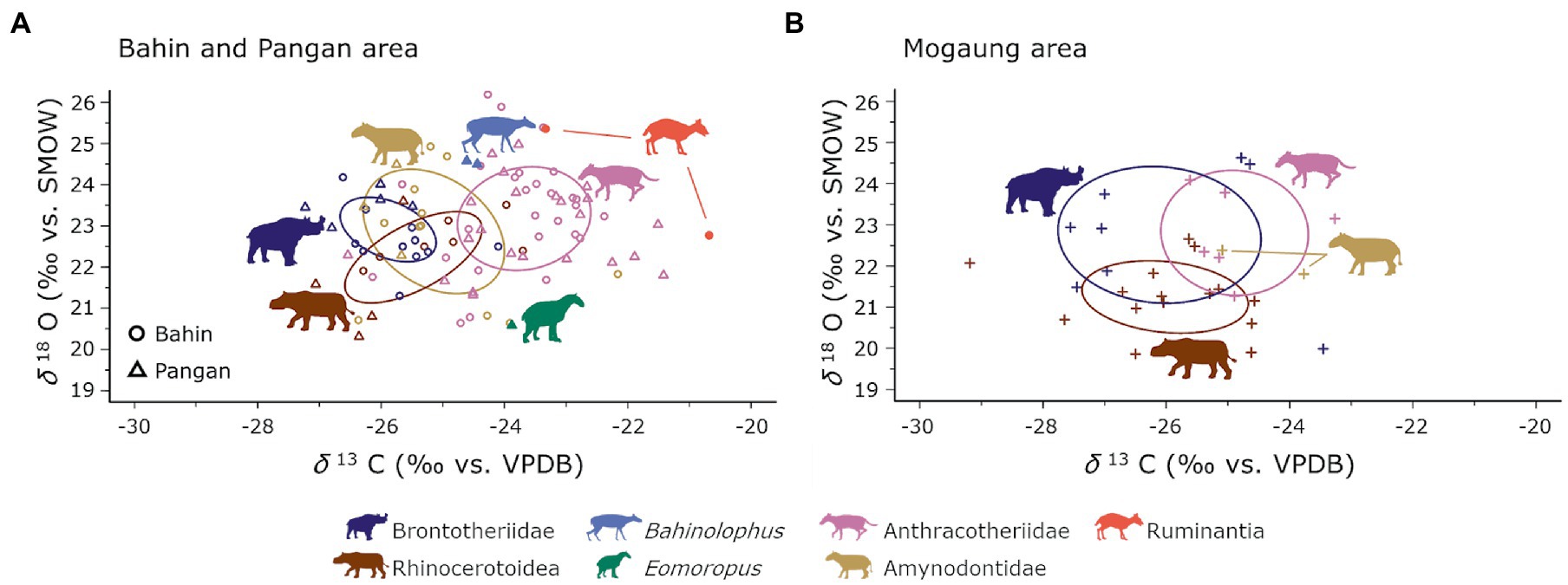
Figure 4. Ecological niche modelling of the Bahin/Pangan (A) and Mogaung (B) mammal communities based on all bulk and averaged serially sampled specimen. Ellipses represent the modelled core ecological niches. Silhouettes are from Phylopic under CC BY 3.0 license by Zimices.
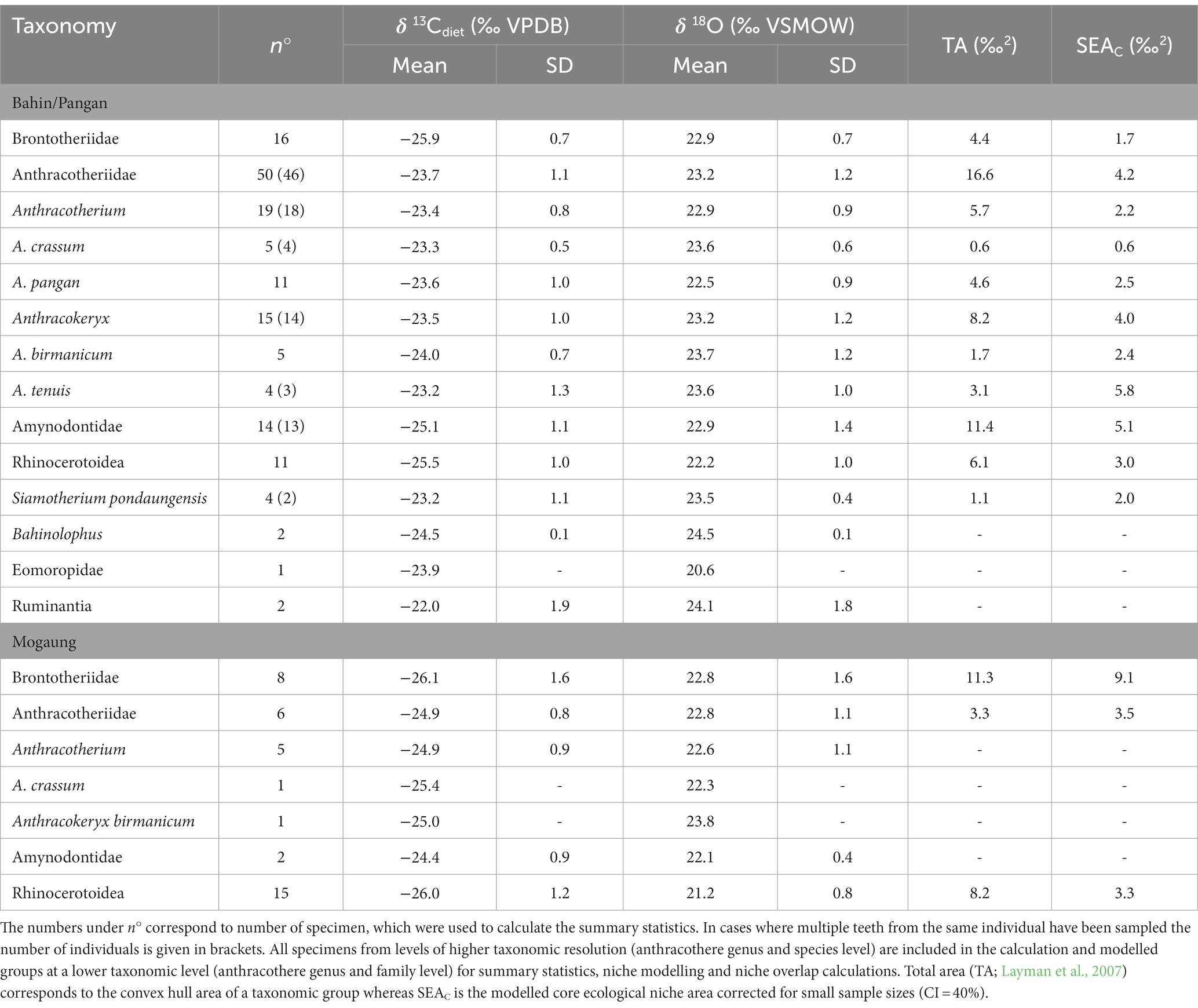
Table 2. Summary statistics from the isotope data used for ecological niche modelling (bulk and averaged intra individual serial samples).
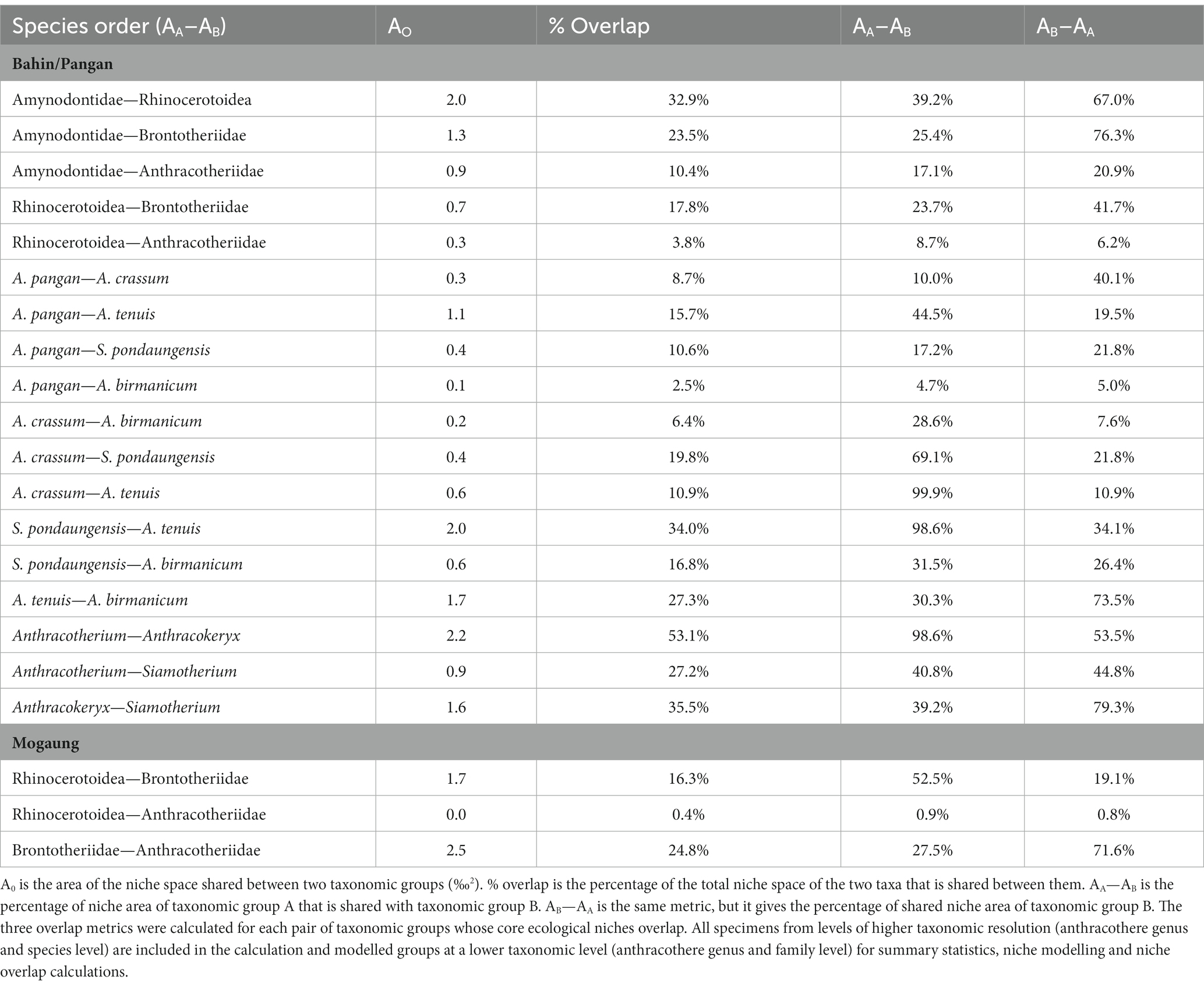
Table 3. Summary of the results of the niche overlap in the Bahin/Pangan and Mogaung mammal communities.
Additionally, we calculated the RINI for the individuals that were sampled serially. A first visual inspection of the patterns between taxonomic groups shows that in Brontotheriidae, as well as the Amynodontidae and Rhinocerotoidea most individuals cluster closely together except for only two or three (Figure 5). In the Anthracotheriidae the individual niches plot more continuously without an apparent split in discrete clusters. It also looks as if the modelled ecological niches of the Anthracotheriidae are slightly bigger than the ones of the other taxonomic groups. This is confirmed when we look at the different median SEACs (Table 4). There we also see that they also have the highest median RINI of all the taxonomic groups. However, these differences are not significant (SEAC: W = 162, value of p = 0.863; RINI: W = 155, value of p = 0.280).
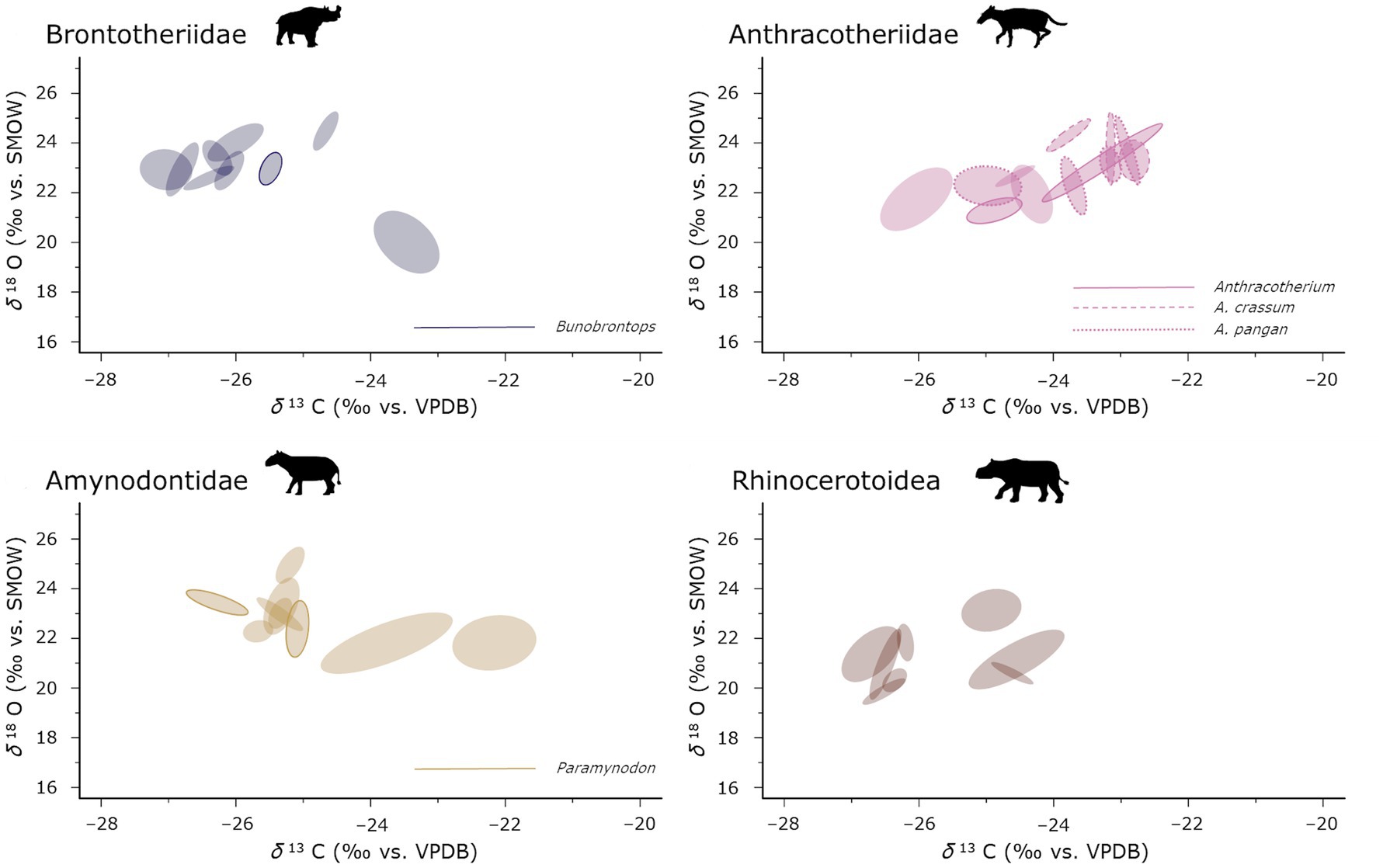
Figure 5. Modelled ecological niches of the serially sampled specimen in separate graphs per taxonomic group. Each ellipse corresponds to the modelled core ecological niche represented by SEAC of one individual (CI = 40%) visualizing different clustering patterns especially between the Anthracotheriidae and the other three taxonomic groups. Silhouettes are from Phylopic under CC BY 3.0 license by Zimices.
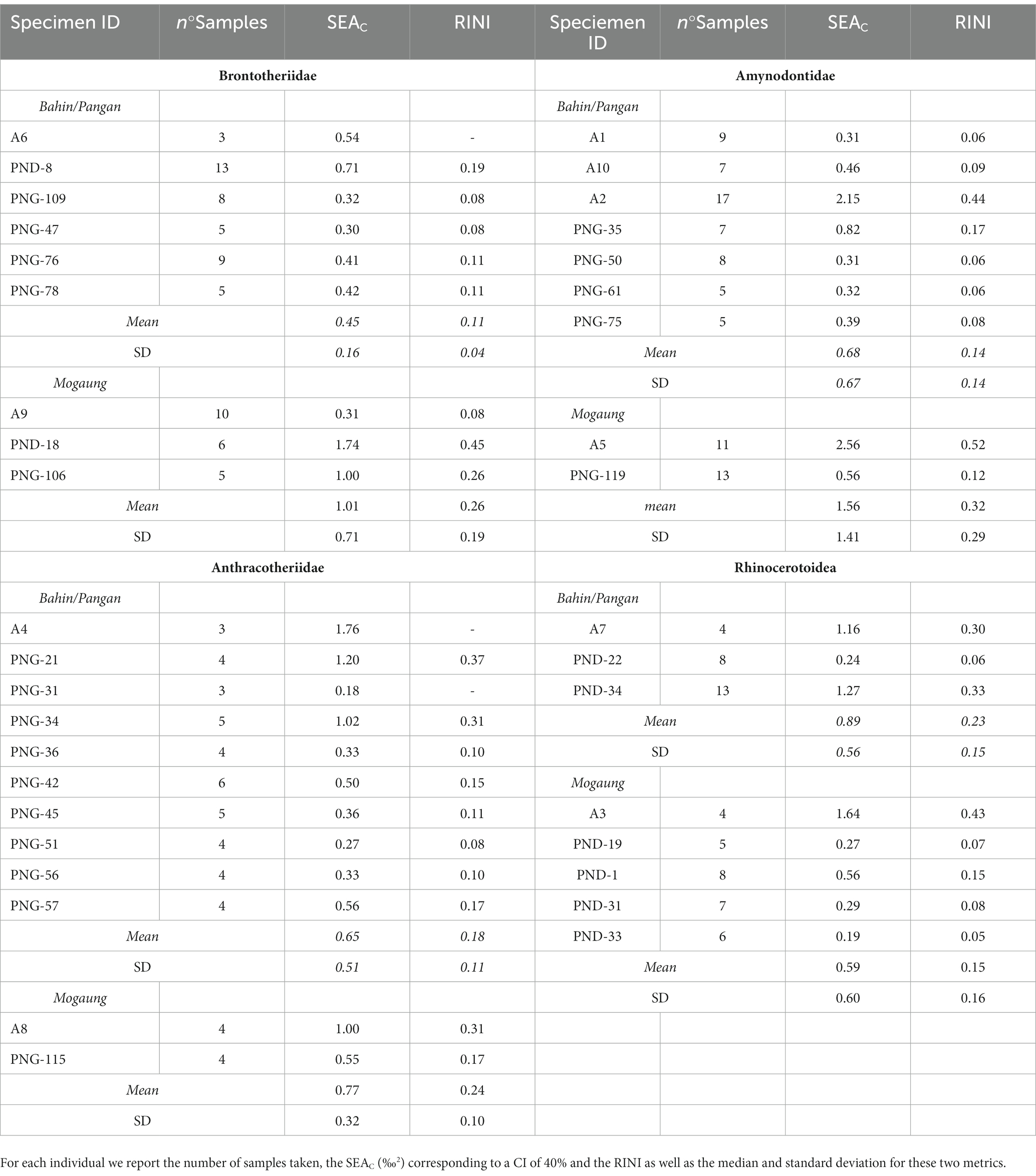
Table 4. Summary of the calculation of RINI for the specimen from which intra-individual serial samples were taken.
4. Discussion
4.1. Paleoseasonality
The δ18O values including the minimum values, which represent the seasonal precipitation maxima in tropical environments (Dansgaard, 1964; Sun et al., 2021), of the modern bovid are higher than the Eocene (Figure 2) ones even though the temperatures nowadays are around 5°C lower than they were back then. The temperature effect leads to higher δ18O values under warmer conditions (Dansgaard, 1964). However, the pattern in the comparison of the modern bovid with the Eocene mammals is reversed. This would be consistent with a generally wetter, more humid climate in the Eocene than in Myanmar today. Consistently lower minimum δ18O might even indicate an increased monsoon intensity in the Eocene, although they could have also been influenced by the possible closer paleogeographic proximity of the fossil localities to the coast at 40 Ma (Westerweel et al., 2019, 2020).
We screened for a correlation between the δ13C and δ18O values of the serially sampled specimen; as such a relationship would be consistent with an influence of seasonality on variation in the diet (e.g., the type of plant or plant part ingested) or habitat. We do see that there is a statistically significant correlation between seasonal precipitation and diet/habitat in the Anthracotheriidae, Amynodontidae, and Rhinocerotoidea as opposed to the Brontotheriidae. This relation however is not uniform across the taxonomic groups. This pattern is consistent with an interpretation of different strategies of the taxonomic groups to react to seasonal habitat changes such as for example adapting their subsistence strategy or migration. As there is no correlation of the δ13C or δ18O values diagenesis is likely not the cause for these correlations (see discussion in the Supplementary Information). The correlation in Anthracotheriidae and Amynodontidae is positive, whereas it is negative in Rhinocerotoidea. This difference between Amynodontidae and Rhinocerotoidea is surprising, since as stated earlier, most specimens which are classified as Rhinocerotoidea are likely amynodonts as no other genus from this superfamily has been described yet. Of course, this difference could be introduced by a sampling bias. If not, it could indicate that either there are two groups of amynodonts that have different ecological strategies to cope with seasonality or that these different ecological strategies also coincide with a taxonomic distinction on a generic level that has not been described as of yet. As there are no experimental studies that focus on this relationship, we cannot precisely characterize the difference between a positive versus a negative correlation of δ13C and δ18O values in plants or herbivorous animals.
Nevertheless, the occurrence of significant correlation of δ13C and δ18O values can be interpreted as an increased influence, e.g., due to the reconstructed monsoon intensity in the Eocene, of seasonality on the diet/habitat on some of the mammal groups from the Pondaung Fm when compared with the modern cow. Two important limitations of this interpretation are the small sample size for modern Bos, which is only represented by one individual and the fact that we are comparing wild Eocene mammals with a modern domestic Bos, whose precise diet is unknown. But even the statistically significant correlations of δ13C and δ18O values in the Eocene alone are another indication of an intense Eocene monsoon.
4.2. Paleoenvironmental differences between the Bahin, Pangan, and Mogaung areas
The Pondaung Fm. was a habitat with only C3 plants present. When we consider the changes in δ13CCO2 values of the atmosphere discussed in the Methods section, modern C3 plants would lead to δ13Cdiet values of −17.5 to −34.5‰ (modern thresholds from Kohn, 2010). Hence, δ13Cdiet values higher than −17.5‰ would be evidence for the presence of C4 plants in the Eocene. The δ13C values of all the mammals in the data set used in this study range from −20.7 to −29.2‰, and thus falling within the range of Eocene C3 plants. As C4 plants with their photosynthetic pathway adapted to dry and hot climatic conditions did not evolve and spread to Asia until the Late Miocene (Cerling et al., 1993, 1997) we did not expect to find any values that high in our data set. In Myanmar, the first evidence of the presence of C4 plants based on isotopic data is from 6 Ma (Thein et al., 2011; Habinger et al., 2022).
The variation of δ13Cdiet values that we observed within and between the two study areas is caused by differences in vegetation structure. Due to the canopy effect, including factors such as low irradiance and carbon recycling, δ13C values decrease with an increased canopy density (van der Merwe and Medina, 1989; Jackson et al., 1993; Bonal et al., 2000; Leffler and Enquist, 2002; Bonafini et al., 2013). This effect is less pronounced high up in the canopy and more open patches of a habitat. In these microhabitats C3 plants yield delta-values of −18.5 to −24.5‰, whereas δ13Cdiet values of −29‰ and below are indicative for the understory of a closed canopy forest (modern thresholds from Kohn, 2010). δ13C values from both the Bahin/Pangan (−20.7 to −27.2‰) and the Mogaung area (−21.2 to −29.2‰) are consistent with open-forested seasonal woodlands, like the ones reconstructed for the upper deltaic plain by Licht et al. (2015). These woodlands were denser in the Mogaung area, given the significantly lower δ13C values (Figure 3), and with specimen with the lowest δ13Cdiet values falling just below the threshold for closed canopy vegetation. This possibly indicates proximity to the more closed dipterocarp forests in the upstream areas from the same vegetation model (Licht et al., 2015). An interpretation of the microhabitat differences as being caused by such spatial factors is also consistent with the paleoenvironmental similarities of the geographically close Bahin and Pangan areas with one another. However, given the currently available dates and correlations of the stratigraphic sequences of the different fossil localities of the Pondaung Fm. we cannot rule out the possibility of the two microhabitats representing a changing environment at two different late Middle Eocene time intervals completely.
The significant difference in the δ18O values between the Bahin/Pangan and the Mogaung area is consistent with a more humid climate in the latter. As the Mogaung area was probably more densely forested as well, the data are also consistent with a consumption of drinking water from water sources that are more protected from direct sunlight. Water sources that are subjected to evaporation would have higher δ18O values as the lighter oxygen isotope would evaporate more readily. However, there is no significant difference in the ranges of δ18O values of the intra-individually sampled individuals between the Bahin/Pangan and the Mogaung area (W = 104, value of p = 0.07336). Range here is defined as the minimum δ18O value subtracted from the maximum one. This indicates that there is no difference in the intensity of the seasonality.
Considering the occurrences of fossil primates (Supplementary Table SI4) in relation with these two different habitat types, we see that so far for only two of the four genera of the anthropoid Amphipithecidae have been found at all three localities, Aseanpithecus and Pondaungia. So at least for these two amphipithecid genera we can propose a certain degree of ecological flexibility. A more detailed discussion of the fossil primate occurrences and how they relate to the localities and the other mammal taxa in our data set can be found in the Supplementary Information.
4.3. Niche partitioning and directional competition potential
An evaluation of differences in size of combined ecological niches on taxonomic family level, which potentially consist of multiple species, and their % overlap showed no significant differences between the two areas. In addition to these similarities the relative positions of the taxonomic groups to one another are very similar as well (Figure 4). Anthracotheriidae were occupying the more open patches of the habitat, which is most apparent in the Bahin/Pangan area. However, the high δ13C values that would indicate a more open vegetation in the anthracothere habitat can also be partly influenced by their more omnivorous subsistence strategy. As the Anthracotheriidae are more omnivorous than the other taxonomic group they had an ecological advantage there. They were able to reduce competition potential as evidenced by the indication of their use of more open habitats in the Bahin/Pangan area than in the more closed Mogaung habitat. Brontotheriidae, Rhinocerotoidea, and in the Bahin/Pangan area also Amynodontidae shared their aspects of their ecological niches as modelled by the carbon and oxygen isotopes in parts of the paleolandscape. The differentiation between the Brontotheriidae and Rhinocerotoidea niche space is mostly due to the δ18O, which would be consistent with the former drinking from more evaporated water sources than the latter. Given all these similarities in the relative positioning of the different taxonomic groups to one another, the differences that we saw between the paleoenvironments between the Bahin/Pangan and the Mogaung area, apparently did not translate to a different organization of the habitat use of the mammal communities there.
We took our analysis of the niche modelling of the Pondaung mammal fauna one step further and looked at the serially sampled individuals (Figure 5) separately. This revealed different patterns of the distribution of modelled ecological niches especially in the Anthracotheriidae. While the Anthracotheriidae appear to share the modelled aspects of their ecological niches, there is more diversity in the Brontotheriidae, Amynodontidae, and Rhinocerotoidea. This makes sense as the taxonomic resolution in the Anthracotheriidae is better (Figure 5), where all but two individuals could be identified as to at least belonging to the genus Anthracotherium, whereas potentially multiple genera or species with different ecologies could be included in the sample for the other taxonomic groups. Several individuals of the Brontotheriidae, Amynodontidae, and Rhinocerotoidea seem to have occupied different microhabitat niches than the other specimen of these groups. Given that this approach requires multiple samples per individual, it becomes apparent why the species diversity in Anthracotheriidae is not reflected here in its entirety as this sampling technique could only be applied to larger teeth, such as the two Anthracotherium species and some undetermined Anthracotheriidae.
Based on the ecological niche modelling on the individual level for the four main taxonomic groups we calculated a metric used in ecology to differentiate generalist from specialist individuals. Large modelled niche areas for a taxon or a taxonomic group are consistent with an overall generalist behavior. It can however, be caused by two different reasons. It can result either from many specialist individuals with different ecologies that would have a low RINI, or from generalist individuals that all more or less use the entire niche space of their taxonomic group and thus having a high RINI. As the total modelled niche area of the taxonomic groups is based on higher level taxa (families) there is the possibility of an overestimation of the isotopically modelled niche aspect, if there are big differences in habitat preferences and use in the lower level taxa. Hence, the RINIs could be lower in these cases. Both SEAC and RINI were not significantly different between Anthracotheriidae and the rest of the taxonomic groups. The fact the same pattern is present in both metrics is an indication that no particular generalist or specialist individuals were present, and that the bias towards an underestimation of the RINIs has no significant effect on this comparison. The clustering pattern in Anthracotheriidae seems to be different than in the other three taxonomic groups. The lack of individuals plotting apart from the main cluster of modelled ecological core niches without overlapping them is consistent with a more continuous variation of ecological requirements in this group whereas the pattern in Brontotheriidae, Amynodontidae, and Rhinocerotoidea indicates more discrete ecological niches reflecting aspects of habitat use and dietary ecology.
4.4. Paleoecology of the Pondaung Anthracotheriidae
Although the localities are located along a paleo river system, none of the taxonomic groups has δ18O values low enough to suggest semi-aquatic behavior. Previous studies showed that depending on the humidity of the terrestrial habitat, this offset can be 2–4‰ lower in freshwater living semi-aquatic species than in terrestrial ones (Clementz et al., 2008). An additional indication of semi-aquatic behavior in a taxonomic group is a reduced standard deviation (SD) of ≤0.5‰ (Yoshida and Miyazaki, 1991; Clementz and Koch, 2001). In our dataset such low SDs are only present in S. pondaungensis, Bahinolophus, and Amynodontidae from Mogaung (Table 2). These are all taxonomic groups with very low sample sizes (MNI = minimum number of individuals = 2). Therefore, we do not consider the low SD as an indication for semi-aquatic behavior in any of these cases. Differences in δ18O values can however result from the use of different water sources. The lower δ18O values in Rhinocerotoidea in comparison to Brontotheriidae would be consistent with them drinking from less evaporated water sources. This could mean shaded water or flowing and not stagnant water.
Between the three different anthracothere genera found in the Pondaung Fm. there is a lot of niche overlap (Figure 6), which was already suggested by the niche modelling of the serially sampled anthracotheres (Figure 5), although none of the smaller genera (Anthracokeryx and Siamotherium) were in this sample group. Apparently, the three genera did share habitats with similar ecological characteristics. Hence, the different feeding ecologies and habitat preferences in regard to forest density inferred from tooth and bone morphology (Ducrocq, 1999) do not translate to different habitats occupied by them based on the modelled core ecological niches. No clear patterns are visible that would differentiate the anthracothere genera based on canopy closure or isotopically different water sources. We described the hypothetical ecology of Anthracotherium as being both more water dependent and more reliant on open spaces in the habitat for their subsistence. Anthracotherium however does not have significantly higher δ13C values or lower δ18O values than the other genera, thus the isotopic data are not consistent with this hypothesis.
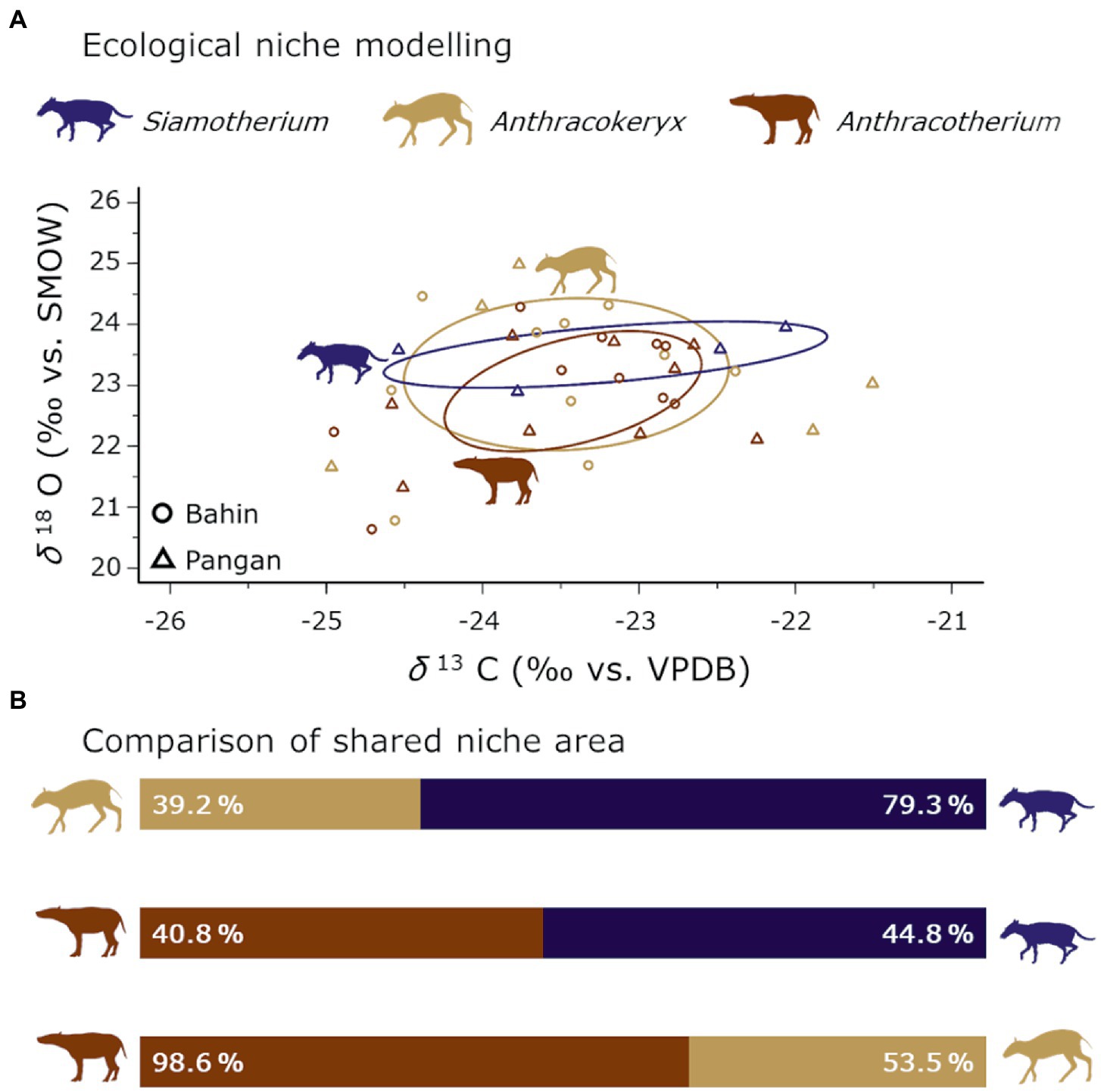
Figure 6. Ecological niche modelling of the anthracotheres from Bahin/Pangan per genus (A). Ellipses represent the core ecological niches. In (B) the results of the shared niche area (AA—AB, and AB—AA) between each taxonomic group are visualized. Silhouettes are from Phylopic under CC BY 3.0 license (Anthracotherium by Zimices, Anthracokeryx by Nobu Tamura and vectorised by T. Michael Keelsey, and Siamotherium is Public Domain).
Anthracotherium shares almost all of its modelled ecological core niche space with Anthracokeryx (Figure 6B). This can indicate that Anthracotherium experienced a higher competition potential for its ecological niche than Anthracokeryx (Ogloff et al., 2019). However, the larger body mass of Anthracotherium counteracts this effect. The higher %overlap of the Anthracotherium with the Anthracokeryx niche could also mean that the advantage of being larger and more generalist in its diet enabled Anthracotherium to use more of the Anthracokeryx niche space (Schoener, 1983; Persson, 1985; Law et al., 1997). A similar pattern is also visible in the comparison of Anthracokeryx and Siamotherium, which would be consistent with similar interpretations. However, it is important to keep in mind that the Siamotherium niche is based on only two individuals, with two sampled teeth each, which differ vastly in δ13C values, and further interpretations will depend on a larger sample size for Siamotherium.
The limitation of sample size of course is even more prevalent when we are looking at niche partitioning between the anthracothere species (Figure 7A). Nevertheless, we wanted to investigate possible interesting patterns of niche partitioning and directional competition potential detectable to open new directions for future research. Given the severe problems with sample size in S. pondaungensis discussed in the previous section, we cannot discuss its niche overlap in comparison with the other species. It is apparent that there is a lot of niche overlap between the anthracothere species, leading to more diffuse competition than niche partitioning in this context (Figure 7). The Pondaung habitat must have provided sufficient area to fulfil the ecological requirements of the anthracotheres and sustain all of the species sampled in this study.
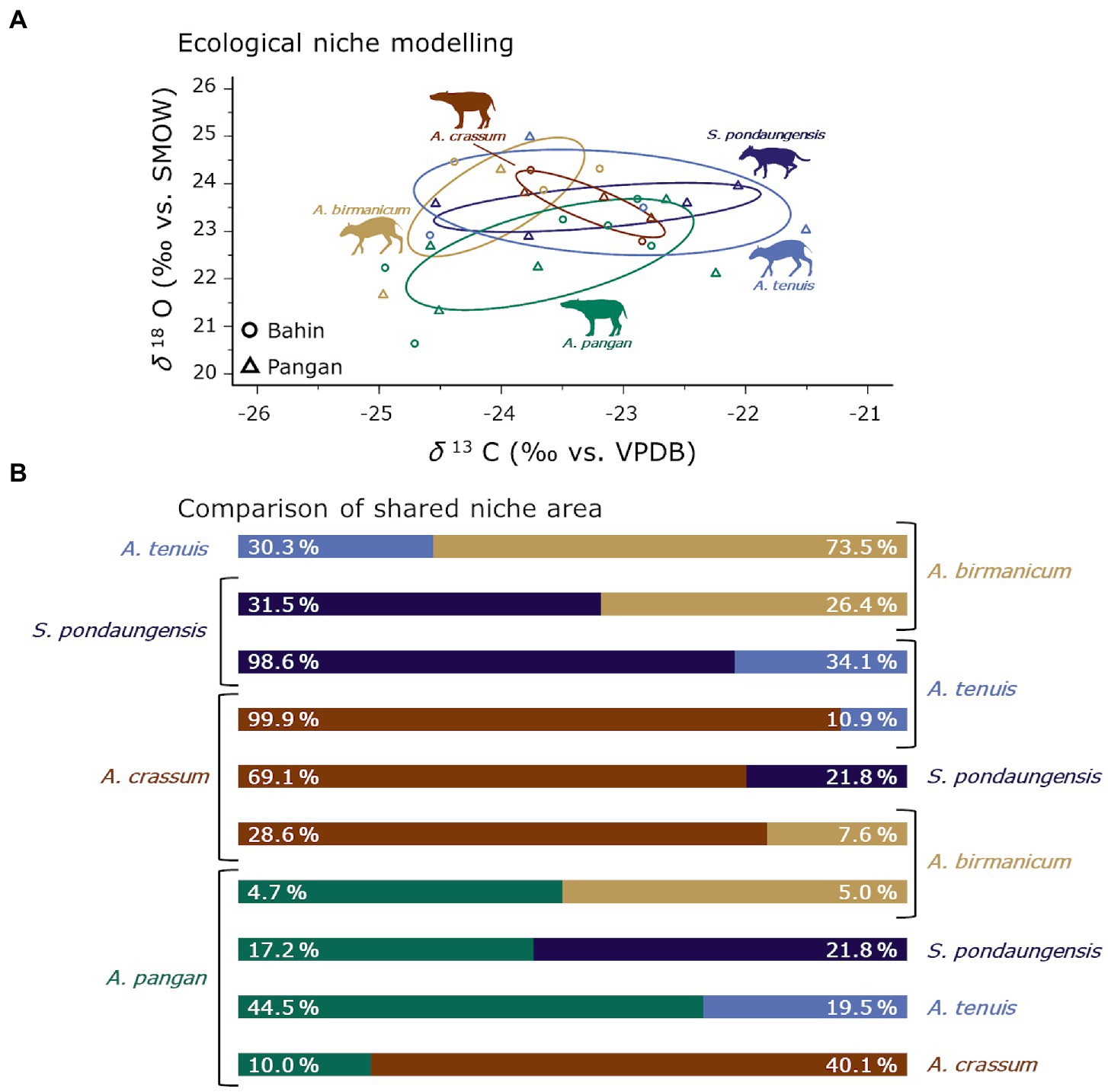
Figure 7. Ecological niche modelling of the anthracotheres from Bahin/Pangan per species (A). Ellipses represent the core ecological niches. In (B) the results of the shared niche area (AA—AB, and AB—AA) between each taxonomic group are visualized. Silhouettes are from Phylopic under CC BY 3.0 license (Anthracotherium by Zimices, Anthracokeryx by Nobu Tamura and vectorised by T. Michael Keelsey, and Siamotherium is Public Domain).
When we generalize the results formed based on the comparison Anthracotherium and Anthracokeryx in the previous section we propose the following hypothesis: Species with higher body mass have a competition advantage; hence, we expect them to have a higher percentage of shared niche space with the smaller species (Figure 7B). Anthracokeryx tenuis, the smallest of these anthracotheres, follows this prediction in every instance and always shares a smaller percentage of its niche area than the bigger species with which it is compared. For the other species this relationship is not as simple. Anthracotherium pangan is the largest anthracothere from Pondaung. However, the second largest A. crassum shares more of its core niche with it than vice versa. This might be because A. crassum also has a much narrower core ecological niche than A. pangan. This fact would be consistent with the latter having more generalist ecological requirements than the former. Anthracokeryx birmanicum mostly fulfils the expectations based on our hypothesis. The shared niche space between it and A. pangan however, are the same. They only share around 5% of their ecological core niches with one another. Given this small overlap area, distinct ecological requirements of these two anthracothere species probably led to a reduced competition potential where the body mass advantage of A. pangan was irrelevant.
To sum up, we could further prove the existence of a proto-monsoon already in the Eocene even at the low paleolatitude of 5°N of the equator. The δ13C and δ18O values also showed significant differences in both overall humidity and vegetation structure between the Bahin/Pangan and Mogaung areas. Although our results are consistent with the hypothesis that favor a spatial pattern of the vegetation structure, temporal differences cannot be ruled out and require more detailed investigation of the dating and stratigraphic relationship of the different fossil localities of the Pondaung Fm. Although the Mogaung area was more humid and more densely forested than the other two, the organization of the mammal fauna was not different, which is consistent with ecological flexibility of this Middle Eocene greenhouse mammal fauna. A high degree of niche overlap was observed on genus and species level resolution for the anthracotheres. Interestingly, there is no evidence for semi-aquatic adaptations in any of the taxonomic groups, when we test for significant offsets in δ18O values and a reduction of their variability. This means that at the same time when ancestral whales were already returning to aquatic life, anthracotheres that are ancestral to hippos still were fully terrestrial even though the paleo river channel associated with the Pondaung Fm. would have offered enough suitable habitats for this lifestyle. That the primate fossil record from the Pondaung Fm. also does not show any significant differences in the occurrences of the different taxa is also consistent with this and also indicates ecological flexibility in this taxonomic group. Therefore, the Pondaung Fm. represents diverse microhabitats that were used by a dynamically organized mammal fauna. This environment proved to be conducive for the diversification and radiation of many taxonomic groups such as primates, anthracotheres and rodents.
Data availability statement
The original contributions presented in the study are included in the article/Supplementary material, further inquiries can be directed to the corresponding author.
Author contributions
SH, OC, and HB contributed to conception and design of the study and worked together with SD on data analysis. SH and SS performed the sampling, sample preparation and SH did the statistical analysis and wrote the first draft of the manuscript. OC, YC, JJ, CS, and AS were essential for fieldwork during which the fossil specimens were collected. All authors contributed to the article and approved the submitted version.
Funding
This research was funded by the ANR (ANR-18-CE92-0029) and DFG (BO 3478/7-1). Sample preparation and analysis took place at the Eberhard Karls Universität Tübingen in the laboratories of the AG Biogeology (Department of Geosciences).
Acknowledgments
We want to thank our collaborators from Myanmar that supported us during the fieldwork and sampling campaign in 2020, M. Rugbumrung for fossil preparation, and P. Tung for conducting the isotopic analysis and proofreading the manuscript. We also want to thank our reviewers for their constructive criticism and ideas that helped us to further improve this article.
Conflict of interest
The authors declare that the research was conducted in the absence of any commercial or financial relationships that could be construed as a potential conflict of interest.
Publisher’s note
All claims expressed in this article are solely those of the authors and do not necessarily represent those of their affiliated organizations, or those of the publisher, the editors and the reviewers. Any product that may be evaluated in this article, or claim that may be made by its manufacturer, is not guaranteed or endorsed by the publisher.
Supplementary material
The Supplementary material for this article can be found online at: https://www.frontiersin.org/articles/10.3389/fevo.2023.1110331/full#supplementary-material
References
Beard, K. C. (2016). Out of Asia: anthropoid origins and the colonization of Africa. Annu. Rev. Anthropol. 45, 199–213. doi: 10.1146/annurev-anthro-102215-100019
Beard, K. C., Marivaux, L., Chaimanee, Y., Jaeger, J.-J., Marandat, B., Tafforeau, P., et al. (2009). A new primate from the Eocene Pondaung formation of Myanmar and the monophyly of Burmese amphipithecids. Proc. Biol. Sci. 276, 3285–3294. doi: 10.1098/rspb.2009.0836
Beard, K. C., Marivaux, L., Tun, S. T., Soe, A. N., Chaimanee, Y., Htoon, W., et al. (2007). New Sivaladapid primates from the Eocene Pondaung formation of Myanmar and the anthropoid status of Amphipithecidae. Bull. Carnegie Museum Nat. Hist. 39, 67–76. doi: 10.2992/0145-9058(2007)39[67:NSPFTE]2.0.CO;2
Benammi, M., Soe, A. N., Tun, T., Bo, B., Chaimanee, Y., Ducrocq, S., et al. (2002). First magnetostratigraphic study of the Pondaung formation: implications for the age of the middle Eocene anthropoids of Myanmar. J. Geol. 110, 748–756. doi: 10.1086/342868
Bocherens, H., Fizet, M., and Mariotti, A. (1994). Diet, physiology and ecology of fossil mammals as inferred from stable carbon and nitrogen biogeochemistry: implications for Pleistocene bears. Palaeogeogr. Palaeoclimatol. Palaeoecol. 107, 213–225. doi: 10.1016/0031-0182(94)90095-7
Böhme, M., Aiglstorfer, M., Antoine, P.-O., Appel, E., Havlik, P., Métais, G., et al. (2013). Na Duong (northern Vietnam)—an exceptional window into Eocene ecosystems from Southeast Asia. Zitteliana A 53, 121–167. doi: 10.5282/UBM/EPUB.19019
Bonafini, M., Pellegrini, M., Ditchfield, P., and Pollard, A. M. (2013). Investigation of the ‘canopy effect’ in the isotope ecology of temperate woodlands. J. Archaeol. Sci. 40, 3926–3935. doi: 10.1016/j.jas.2013.03.028
Bonal, D., Barigah, T. S., Granier, A., and Guehl, J. M. (2000). Late-stage canopy tree species with extremely low δ13 C and high stomatal sensitivity to seasonal soil drought in the tropical rainforest of French Guiana. Plant Cell Environ. 23, 445–459. doi: 10.1046/j.1365-3040.2000.00556.x
Bond, A. L., and Hobson, K. A. (2012). Reporting stable-isotope ratios in ecology: recommended terminology, guidelines and best practices. Waterbirds 35, 324–331. doi: 10.1675/063.035.0213
Bonferroni, C. (1936). Teoria statistica delle classi e calcolo delle probabilità. Pubblicazioni del R Instituto Superiore di Scienze Economiche e Commericiali di Firenze 8, 3–62.
Burke, K. D., Williams, J. W., Chandler, M. A., Haywood, A. M., Lunt, D. J., and Otto-Bliesner, B. L. (2018). Pliocene and Eocene provide best analogs for near-future climates. Proc. Natl. Acad. Sci. U. S. A. 115, 13288–13293. doi: 10.1073/pnas.1809600115
Cerling, T. E., and Harris, J. M. (1999). Carbon isotope fractionation between diet and bioapatite in ungulate mammals and implications for ecological anc paleoecological studies. Oecologia 120, 347–363. doi: 10.1007/s004420050868
Cerling, T. E., Harris, J. M., Leakey, M. G., Passey, B. H., and Levin, N. E. (2010). “Stable carbon and oxygen isotopes in east African mammals: modern and fossil” in Cenozoic Mammals of Africa. ed. L. Werdelin (Berkeley, Los Angeles: University of California Press), 941–952.
Cerling, T. E., Harris, J. M., MacFadden, B. J., Leakey, M. G., Quade, J., Eisenmann, V., et al. (1997). Global vegetation change through the Miocene/Pliocene boundary. Nature 389, 153–158. doi: 10.1038/38229
Cerling, T. E., Wang, Y., and Quade, J. (1993). Expansion of C4 ecosystems as an indicator of global ecological change in the late Miocene. Nature 361, 344–345. doi: 10.1038/361344a0
Chaimanee, Y., Chavasseau, O., Beard, K. C., Kyaw, A. A., Soe, A. N., Sein, C., et al. (2012). Late middle Eocene primate from Myanmar and the initial anthropoid colonization of Africa. Proc. Natl. Acad. Sci. U. S. A. 109, 10293–10297. doi: 10.1073/pnas.1200644109
Clementz, M. T., Holroyd, P. A., and Koch, P. L. (2008). Identifying aquatic habits of herbivorous mammals through stable isotope analysis. PALAIOS 23, 574–585. doi: 10.2110/palo.2007.p07-054r
Clementz, M. T., and Koch, P. L. (2001). Differentiating aquatic mammal habitat and foraging ecology with stable isotopes in tooth enamel. Oecologia 129, 461–472. doi: 10.1007/s004420100745
Cooper, L. N., Clementz, M. T., Usip, S., Bajpai, S., Hussain, S. T., and Hieronymus, T. L. (2016). Aquatic habits of cetacean ancestors: integrating bone microanatomy and stable isotopes. Integr. Comp. Biol. 56, 1370–1384. doi: 10.1093/icb/icw119
Coplen, T. B. (1988). Normalization of oxygen and hydrogen isotope data. Chem. Geol. (Isotope Geosci. Sect.) 72, 293–297. doi: 10.1016/0168-9622(88)90042-5
Coplen, T. B. (2011). Guidelines and recommended terms for expression of stable-isotope-ratio and gas-ratio measurment results. Rapid Commun. Mass Spectrom. 25, 2538–2560. doi: 10.1002/rcm.5129
Coster, P. M., Soe, A. N., Beard, K. C., Chaimanee, Y., Sein, C., Lazzari, V., et al. (2018). Astragalus of Pondaungimys (Rodentia, Anomaluroidea) from the late middle Eocene Pondaung formation, Central Myanmar. J. Vertebr. Paleontol. 38:e1552156. doi: 10.1080/02724634.2018.1552156
Dansgaard, W. (1964). Stable isotopes in precipitation. Tellus 16, 436–468. doi: 10.1111/j.2153-3490.1964.tb00181.x
de Bonis, L., Solé, F., Chaimanee, Y., Naing Soe, A., Sein, C., Lazzari, V., et al. (2018). New hyaenodonta (Mammalia) from the middle Eocene of Myanmar. Comptes Rendus Palevol 17, 357–365. doi: 10.1016/j.crpv.2017.12.003
Ducrocq, S. (1999). The late Eocene Anthracotheriidae (Mammalia, Artiodactyla) from Thailand. Palaeontogr. Abt. A 252, 93–140. doi: 10.1127/pala/252/1999/93
Ducrocq, S., Chaimanee, Y., Soe, A. N., Sein, C., Jaeger, J.-J., and Chavasseau, O. (2021). First report of the lower dentition of Siamotherium pondaungensis (Cetartiodactyla, Hippopotamoidea) from the late middle Eocene of the Pondaung formation Myanmar. Neues Jb. Geol. Paläontol. Abh. 301, 217–228. doi: 10.1127/njgpa/2021/1010
Ducrocq, S., Soe, A. N., Aung, A. K., Benammi, M., Bo, B., Chaimanee, Y., et al. (2001). A new anthracotheriid artiodactyl from Myanmar, and the relative ages of the Eocene anthropoid primate-bearing localities of Thailand (Krabi) and Myanmar (Pondaung). J. Vertebr. Paleontol. 20, 755–760. doi: 10.1671/0272-4634(2000)020[0755:ANAAFM]2.0.CO;2
Ducrocq, S., Soe, A. N., Chavasseau, O., Sein, C., Chaimanee, Y., Lazzari, V., et al. (2019). New basal ruminants from the Eocene of the Pondaung formation, Myanmar. J. Vertebrate Paleontol. 39:e1722682. doi: 10.1080/02724634.2019.1722682
Egi, N., and Tsubamoto, T. (2000). A preliminary report on carnivorous mammals from Pondaung fauna. Asian Paleoprimatol. 1, 103–114.
Gentis, N., Licht, A., Boura, A., Franceschi, D.de, Win, Z., Wa Aung, D., et al. (2021). Fossil woods from the lower Miocene of Myanmar (Natma formation): Paleoenvironmental and biogeographic implications. Geodiversitas 44, 853–909. doi: 10.5252/geodiversitas2022v44a28
Habinger, S. G., Chavasseau, O., Jaeger, J.-J., Chaimanee, Y., Soe, A. N., Sein, C., et al. (2022). Evolutionary ecology of Miocene hominoid primates in Southeast Asia. Sci. Rep. 12:11841. doi: 10.1038/s41598-022-15574-z
Head, J. J., Gunnell, G. F., Holroyd, P. A., Hutchison, J. H., and Ciochon, R. L. (2013). Giant lizards occupied herbivorous mammalian ecospace during the Paleogene greenhouse in Southeast Asia. Proc. Biol. Sci. 280:20130665. doi: 10.1098/rspb.2013.0665
Holroyd, P. A., and Ciochon, R. L. (2000). Bunobrontops savagei a new genus and species of brontotheriid perissodactyl from the Eocene Pondaung fauna of Myanmar. J. Vertebr. Paleontol. 20, 408–410. doi: 10.1671/0272-4634(2000)020[0408:BSANGA]2.0.CO;2
Huang, H., Morley, R. J., Licht, A., Dupont-Nivet, G., Pérez-Pinedo, D., Westerweel, J., et al. (2023). A proto-monsoonal climate in the late Eocene of Southeast Asia: evidence from a sedimentary record in Central Myanmar. Geosci. Front. 14:101457. doi: 10.1016/j.gsf.2022.101457
Hutchinson, G. E. (1957). Concluding remarks. Cold Spring Harb. Symp. Quant. Biol. 22, 415–427. doi: 10.1101/SQB.1957.022.01.039
Hutchinson, G. E. (1978). An Introduction to Population Ecology. New Haven, London: Yale University Press.
Hutchison, J. H., Holroyd, P. A., and Ciochon, R. L. (2004). Preliminary report on Southeast Asia’s oldest Cenozoic turtle Fauna from the late middle Eocene Pongaung formation, Myanmar. Asiatic Herpetol. Res. 10, 38–52. https://escholarship.org/uc/item/2xf1v8tb
Jackson, A. L., Inger, R., Parnell, A. C., and Bearhop, S. (2011). Comparing isotopic niche widths among and within communities: SIBER—stable isotope Bayesian ellipses in R. J. Anim. Ecol. 80, 595–602. doi: 10.1111/j.1365-2656.2011.01806.x
Jackson, P. C., Meinzer, F. C., Goldstein, G., Holbrook, N. M., Cavelier, J., and Rada, F. (1993). “Environmental and physiological influences on carbon isotope composition of gap and understory plants in a lowland tropical Forest” in Stable Isotopes and Plant Carbon-Water Relations. eds. J. R. Ehleringer, A. E. Hall, and G. D. Farquhar (San Diego: Academic Press), 131–140.
Jaeger, J.-J., Sein, C., Gebo, D. L., Chaimanee, Y., Nyein, M. T., Oo, T. Z., et al. (2020). Amphipithecine primates are stem anthropoids: cranial and postcranial evidence. Proc. R. Soc. B 287:20202129. doi: 10.1098/rspb.2020.2129
Kay, R. F., Schmitt, D., Vinyard, C. J., Perry, J. M. G., Shigehara, N., Takai, M., et al. (2004). The paleobiology of Amphipithecidae, south Asian late Eocene primates. J. Hum. Evol. 46, 3–25. doi: 10.1016/j.jhevol.2003.09.009
Keeling, C. D., Piper, S. C., Bacastow, R. B., Wahlen, M., Whorf, T. P., Heimann, M., et al. (2005). “Atmospheric CO2 and 13CO2 exchange with the terrestrial biosphere and oceans from 1978 to 2000: observations and carbon cycle implications” in A History of Atmospheric CO2 and its Effects on Plants, Animals, and Ecosystems. eds. J. R. Ehleringer, T. E. Cerling, and M. D. Dearing (New York, Great Britain: Springer), 83–113.
Koch, P. L., Tuross, N., and Fogel, M. L. (1997). The effects of sample treatment and diagenesis on the isotopic integrity of carbonate in biogenic Hydroxylapatite. J. Archaeol. Sci. 24, 417–429. doi: 10.1006/jasc.1996.0126
Kohn, M. J. (2010). Carbon isotope compositions of terrestrial C3 plants as indicators of (paleo)ecology and (paleo)climate. Proc. Natl. Acad. Sci. 107, 19691–19695. doi: 10.1073/pnas.1004933107
Law, R., Marrow, P., and Dieckmann, U. (1997). On evolution under asymmetric competition. Evol. Ecol. 11, 485–501. doi: 10.1023/A:1018441108982
Layman, C. A., Arrington, D. A., Montaña, C. G., and Post, D. M. (2007). Can stable isotope ratios provide for community-wide measures of trophic structure? Ecology 88, 42–48. doi: 10.1890/0012-9658(2007)88[42:CSIRPF]2.0.CO;2
Leffler, J. A., and Enquist, B. J. (2002). Carbon isotope composition of tree leaves from Guanacaste, Costa Rica: comparison across tropical forests and tree life history. J. Trop. Ecol. 18, 151–159. doi: 10.1017/S0266467402002109
Licht, A., Boura, A., Franceschi, D. de, Ducrocq, S., Soe, A. N., and Jaeger, J.-J. (2014a). Fossil woods from the late middle Eocene Pondaung formation, Myanmar. Rev. Palaeobot. Palynol. 202, 29–46. doi: 10.1016/j.revpalbo.2013.12.002
Licht, A., Boura, A., Franceschi, D. de, Utescher, T., Sein, C., and Jaeger, J.-J. (2015). Late middle Eocene fossil wood of Myanmar: implications for the landscape and the climate of the Eocene Bengal Bay. Rev. Palaeobot. Palynol. 216, 44–54. doi: 10.1016/j.revpalbo.2015.01.010
Licht, A., Cojan, I., Caner, L., Soe, A. N., Jaeger, J.-J., and France-Lanord, C. (2014b). Role of permeability barriers in alluvial hydromorphic palaeosols: the Eocene Pondaung formation, Myanmar. Sedimentology 61, 362–382. doi: 10.1111/sed.12059
Licht, A., France-Lanord, C., Reisberg, L., Fontaine, C., Soe, A. N., and Jaeger, J.-J. (2013). A palaeo Tibet-Myanmar connection? Reconstructing the late Eocene dranage system of Central Myanmar using a multi-proxy approach. J. Geol. Soc. Lond. 170, 929–939. doi: 10.1144/jgs2012-126
Licht, A., van Cappelle, M., Abels, H. A., Ladant, J.-B., Tracucho-Alexandre, J., France-Lanord, C., et al. (2014c). Asian monsoons in a late Eocene greenhouse world. Nature 513, 501–506. doi: 10.1038/nature13704
Lihoreau, F., Boisserie, J.-R., Manthi, F. K., and Ducrocq, S. (2015). Hippos stem from the longest sequence of terrestrial cetartiodactyl evolution in Africa. Nat. Commun. 6:6264. doi: 10.1038/ncomms7264
Lihoreau, F., and Ducrocq, S. (2007). “Family Anthracotheriidae” in The Evolution of Atiodactyls. eds. D. R. Prothero and S. E. Foss (Baltimore: The Johns Hopkin University Press), 89–105.
Mader, B. J. (1998). “Bronthotheriidae,” in Evolution of Tertiary Mammals of North America: Volume 1, Terrestrial Carnivores, Ungulates, and Ungulate Like Mammals, eds. C. M. Janis, K. M. Scott, and L. L. Jacobs (Cambridge: Cambridge University Press), 525–536.
Marivaux, L., Beard, K. C., Chaimanee, Y., Dagosto, M., Gebo, D. L., Guy, F., et al. (2010). Talar morphology, phylogenetic affinities, and locomotor adaptation of a large-bodied amphipithecid primate from the late middle Eocene of Myanmar. Am. J. Phys. Anthropol. 143, 208–222. doi: 10.1002/ajpa.21307
Marivaux, L., Beard, K. C., Chaimanee, Y., Jaeger, J.-J., Marandat, B., Soe, A. N., et al. (2008a). Anatomy of the bony pelvis of a relatively large-bodied strepsirrhine primate from the late middle Eocene Pondaung formation (Central Myanmar). J. Hum. Evol. 54, 391–404. doi: 10.1016/j.jhevol.2007.09.007
Marivaux, L., Beard, K. C., Chaimanee, Y., Jaeger, J.-J., Marandat, B., Soe, A. N., et al. (2008b). Proximal femoral anatomy of a sivaladapid primate from the late middle Eocene Pondaung formation (Central Myanmar). Am. J. Phys. Anthropol. 137, 263–273. doi: 10.1002/ajpa.20866
Marivaux, L., Chaimanee, Y., Ducrocq, S., Marandat, B., Sudre, J., Soe, A. N., et al. (2003). The anthropoid status of a primate from the late middle Eocene Pondaung formation (Central Myanmar): tarsal evidence. Proc. Natl. Acad. Sci. U. S. A. 100, 13173–13178. doi: 10.1073/pnas.2332542100
Marivaux, L., Ducrocq, S., Jaeger, J.-J., Marandat, B., Sudre, J., Chaimanee, Y., et al. (2005). New remains of Pondaungimys anomaluropsis (Rodentia, Anomaluroidea) from the latest middle Eocene Pondaung formation of Central Myanmar. J. Vertebr. Paleontol. 25, 214–227. doi: 10.1671/0272-4634(2005)025[0214:NROPAR]2.0.CO;2
Maung, M., Htike, T., Tsubamoto, T., Suzuki, H., Sein, C., Egi, N., et al. (2005). Stratigraphy of the primate-bearing beds of the Eocene Pondaung formation at Paukkaung area, Myanmar. Anthropol. Sci. 113, 11–15. doi: 10.1537/ase.04S002
Métivier, F., Gaudemer, Y., Tapponnier, P., and Klein, M. (1999). Mass accumulation rates in Asia during the Cenozoic. Geophys. J. Int. 137, 280–318. doi: 10.1046/j.1365-246X.1999.00802.x
Morley, R. J. (2018). Assembly and division of the south and south-east Asian flora in relation to tectonics and climate change. J. Trop. Ecol. 34, 209–234. doi: 10.1017/S0266467418000202
Ogloff, W. R., Yurkowski, D. J., Davoren, G. K., and Ferguson, S. H. (2019). Diet and isotopic niche overlap elucidate competition potential between seasonally sympatric phocids in the Canadian Arctic. Mar. Biol. 166, 1–12. doi: 10.1007/s00227-019-3549-6
Oo, K. L., Zaw, K., Meffre, S., Myitta, Aung D. W., and Lai, C.-K. (2015). Provenance of the Eocene sandstones in the southern Chindwin Basin, Myanmar: Implications for the unroofing history of the Cretaceous–Eocene magmatic arc. J. Asian Earth Sci. 107, 172–194. doi: 10.1016/j.jseaes.2015.04.029
Orliac, M. J., Antoine, P.-O., Charruault, A.-L., Hervet, S., Prodeo, F., and Duranthon, F. (2013). Specialization for amphibiosis in Brachyodus onoideus (Artiodactyla, Hippopotamoidea) from the early Miocene of France. Swiss J. Geosci. 106, 265–278. doi: 10.1007/s00015-013-0121-0
Pagani, M., Zachos, J., Freeman, K., Tipple, B., and Bohaty, S. (2005). Marked decline in atmospheric carbon dioxide concentrations during the Paleogene. Science 309, 600–603. doi: 10.1126/science.1110063
Passey, B. H., Robinson, T. F., Ayliffe, L. K., Cerling, T. E., Sponheimer, M., Dearing, M. D., et al. (2005). Carbon isotope fractionation between diet, breath CO2, and bioapatite in different mammals. J. Archaeol. Sci. 32, 1459–1470. doi: 10.1016/j.jas.2005.03.015
Persson, L. (1985). Asymmetrical competition: are larger animals competitively superior? Am. Nat. 126, 261–266. doi: 10.1086/284413
Ramdarshan, A., Merceron, G., Tafforeau, P., and Marivaux, L. (2010). Dietary reconstruction of the Amphipithecidae (primates, Anthropoidea) from the Paleogene of South Asia and paleoecological implications. J. Hum. Evol. 59, 96–108. doi: 10.1016/j.jhevol.2010.04.007
Schoener, T. W. (1983). Field experiments on interspecific competition. Am. Nat. 122, 240–285. doi: 10.1086/284133
Sheppard, C. E., Inger, R., McDonald, R. A., Barker, S., Jackson, A. L., Thompson, F. J., et al. (2018). Intragroup competition predicts individual foraging specialisation in a group-living mammal. Ecol. Lett. 21, 665–673. doi: 10.1111/ele.12933
Soe, A. N. (2008). A new study of the anthracotheres (mammalia, artiodactyla) from Pondaung formation, Myanmar: systematics implications. Palaeovertebrata 36, 89–157. doi: 10.18563/pv.36.1-4.89-157
Soe, A. N., Chavasseau, O., Chaimanee, Y., Sein, C., Jaeger, J.-J., Valentin, X., et al. (2017). New remains of Siamotherium pondaungensis (Cetartiodactyla, Hippopotamoidea) from the Eocene of Pondaung, Myanmar: Paleoecologic and phylogenetic implications. J. Vertebr. Paleontol. 37:e1270290. doi: 10.1080/02724634.2017.1270290
Soe, A. N., Myitta, T. S. T., Aung, A. K., Thein, T., Marandat, B., Ducrocq, S., et al. (2002). Sedimentary facies of the late middle Eocene Pondaung formation (Central Myanmar) and the palaeoenvironments of its anthropoid primates. Comptes Rendus Palevol 1, 153–160. doi: 10.1016/S1631-0683(02)00020-9
Stamp, L. D. (1922). An outline of the tertiary geology of Burma. Geol. Mag. 59, 481–501. doi: 10.1017/S001675680010915X
Sun, F., Wang, Y., Jablonski, N. G., Hou, S., Ji, X., Wolff, B., et al. (2021). Paleoenvironment of the late Miocene Shuitangba hominoids from Yunnan, Southwest China: insights from stable isotopes. Chem. Geol. 569:120123. doi: 10.1016/j.chemgeo.2021.120123
Suzuki, H., Maung, M., Win, Z., Tsubamoto, T., Thein, Z. M. M., Egi, N., et al. (2006). Stratigraphic positions of the Eocene vertebrate localities in the Paukkaung area (Pondaung formation, Central Myanmar). Asian Paleoprimatol. 4, 67–74. http://hdl.handle.net/2433/199770
Szpak, P., Metcalfe, J. Z., and Macdonald, R. A. (2017). Best practices for calibrating and reporting stable isotope measurments in archaeology. J. Archaeol. Sci. Rep. 13, 609–616. doi: 10.1016/j.jasrep.2017.05.007
Tejada-Lara, J. V., MacFadden, B. J., Bermudez, L., Rojas, G., Salas-Gismondi, R., and Flynn, J. J. (2018). Body mass predicts isotope enrichment in herbivorous mammals. Proc. Biol. Sci. 285:20181020. doi: 10.1098/rspb.2018.1020
Thein, Z. M. M., Takai, M., Uno, H., Wynn, J. G., Egi, N., Tsubamoto, T., et al. (2011). Stable isotope analysis of the tooth enamel of Chaingzauk mammalian fauna (late Neogene, Myanmar) and its implication to paleoenvironment and paleogeography. Palaeogeogr. Palaeoclimatol. Palaeoecol. 300, 11–22. doi: 10.1016/j.palaeo.2010.11.016
Thewissen, J. G. M., Cooper, L. N., Clementz, M. T., Bajpai, S., and Tiwari, B. N. (2007). Whales originated from aquatic artiodactyls in the Eocene epoch of India. Nature 450, 1190–1194. doi: 10.1038/nature06343
Tierney, J. E., Poulsen, C. J., Montañez, I. P., Bhattacharya, T., Feng, R., Ford, H. L., et al. (2020). Past climates inform our future. Science 370, 1–9. doi: 10.1126/science.aay3701
Tipple, B. J., Meyers, S. R., and Pagani, M. (2010). Carbon isotope ratio of Cenozoic CO2: a comparative evaluation of available geochemical proxies. Paleoceanography 25, 1–11. doi: 10.1029/2009PA001851
Toumoulin, A., Tardif, D., Donnadieu, Y., Licht, A., Ladant, J.-B., Kunzmann, L., et al. (2022). Evolution of continental temperature seasonality from the Eocene greenhouse to the Oligocene icehouse –a model–data comparison. Clim. Past 18, 341–362. doi: 10.5194/cp-18-341-2022
Tsubamoto, T., Egi, N., and Takai, M. (2006a). Notes on fish, reptilian, and several fragmentary mammalian dental fossils from the Pondaung formation. Asian Paleoprimatol. 4, 98–110. Available at: http://hdl.handle.net/2433/199768
Tsubamoto, T., Egi, N., Takai, M., Sein, C., and Maung, M. (2005). Middle Eocene ungulate mammals from Myanmar: a review with description of new specimens. Acta Palaeontol. Pol. 50, 117–138.
Tsubamoto, T., Egi, N., Takai, M., Shigehara, N., Suzuki, H., Nishimura, T., et al. (2006b). A summary of the Pondaung fossil expeditions. Asian Paleontol. 4, 1–66. Available at: http://hdl.handle.net/2433/199771
Tsubamoto, T., Takai, M., Egi, N., Shigehara, N., Thura, S., Aung, A. K., et al. (2002). The Anthracotheriidae (Mammalia; Artiodactyla) from the Eocene Pondaung formation (Myanmar) and comments on some other anthracotheres from the Eocene of Asia. Paleontol. Res. 6, 363–384. doi: 10.2517/prpsj.6.363
van der Merwe, N. J., and Medina, E. (1989). Photosynthesis and ratios in Amazonian rain forests. Geochim. Cosmochim. Acta 53, 1091–1094. doi: 10.1016/0016-7037(89)90213-5
Westerweel, J., Licht, A., Cogné, N., Roperch, P., Dupont-Nivet, G., Kay Thi, M., et al. (2020). Burma terrane collision and northward indentation in the eastern Himalayas recorded in the Eocene-Miocene Chindwin Basin (Myanmar). Tectonics 39, 1–30. doi: 10.1029/2020TC006413
Westerweel, J., Roperch, P., Licht, A., Dupont-Nivet, G., Win, Z., Poblete, F., et al. (2019). Burma terrane part of the trans-Tethyan arc during collision with India according to palaeomagnetic data. Nat. Geosci. 12, 863–868. doi: 10.1038/s41561-019-0443-2
Wright, L. E., and Schwarcz, H. P. (1999). Correspondence between stable carbon, oxygen and nitrogen isotopes in human tooth enamel and dentine: infant diets at Kaminaljuyú. J. Archaeol. Sci. 26, 1159–1170. doi: 10.1006/jasc.1998.0351
Yoshida, N., and Miyazaki, N. (1991). Oxygen isotope correlation of cetacean bone phosphate with environmental water. J. Geophys. Res. 96, 815–820. doi: 10.1029/90JC01580
Zachos, J., Pagani, M., Sloan, L., Thomas, E., and Billups, K. (2001). Trends, rhythms, and aberrations in global climate 65 ma to present. Science 292, 686–693. doi: 10.1126/science.1059412
Keywords: isotopic niche, Pondaung Formation, Anthracotheriidae, anthropoid primates, competition potential, Eocene, paleoecology
Citation: Habinger SG, Chavasseau O, Ducrocq S, Chaimanee Y, Jaeger J-J, Sein C, Soe AN, Stern S and Bocherens H (2023) Isotopic niche modelling of the Pondaung mammal fauna (middle Eocene, Myanmar) shows microhabitat differences. Insights into paleoecology and early anthropoid primate habitats. Front. Ecol. Evol. 11:1110331. doi: 10.3389/fevo.2023.1110331
Edited by:
Daniel J. Peppe, Baylor University, United StatesReviewed by:
Manuel Hernández Fernández, Complutense University of Madrid, SpainDavid L. Fox, University of Minnesota Twin Cities, United States
Copyright © 2023 Habinger, Chavasseau, Ducrocq, Chaimanee, Jaeger, Sein, Soe, Stern and Bocherens. This is an open-access article distributed under the terms of the Creative Commons Attribution License (CC BY). The use, distribution or reproduction in other forums is permitted, provided the original author(s) and the copyright owner(s) are credited and that the original publication in this journal is cited, in accordance with accepted academic practice. No use, distribution or reproduction is permitted which does not comply with these terms.
*Correspondence: Sophie G. Habinger, c29waGllLWdhYnJpZWxlLmhhYmluZ2VyQHVuaS10dWViaW5nZW4uZGU=