- 1Conservation Ecology, Department of Biology, Philipps-Universität Marburg, Karl-von-Frisch-Straße, Marburg, Germany
- 2Behavioural Ecology and Ecophysiology Group, Department of Animal Ecology and Systematics, Justus-Liebig-Universität Giessen, Heinrich-Buff-Ring, Giessen, Germany
Introduction: Parasites play important roles in ecosystems. Through their interactions with host and vector species, they are capable of changing the behavior and population dynamics of their host species, and the shape of entire communities. Over the past years, many studies have acknowledged the role of parasitism for host populations and communities and discovered their important regulatory functions for many vertebrate populations. Although birds are a well-studied group of vertebrates, the infection patterns of endoparasites at the community level are not fully understood. Some bird species and families are known to have a higher susceptibility to certain endoparasites than others, which may be driven by their abundance in the community.
Methods: Over the course of four consecutive breeding seasons (2019–2022), we monitored the patterns of endoparasite infections in a bird community of a temperate forest ecosystem. We sampled 483 birds belonging to 29 Palearctic species and investigated the prevalence of blood parasites (haemosporidian parasites) and Trichomonas spp. using molecular methods.
Results: We found an overall prevalence of 48.1% of haemosporidians belonging to 53 genetic lineages of the three genera Haemoproteus, Leucocytozoon, and Plasmodium spp. While the bird families Turdidae (94%) and Paridae (76%) showed a high prevalence of haemosporidians, Certhiidae, and Picidae were not infected (0%). Host–parasite network analysis detected high variability in interactions. Infections with Trichomonas spp. were not observed in the forest bird community.
Discussion: We found that the prevalence and lineage diversity of haemosporidian parasites differed between avian families and that the parasite prevalence of a family could not serve as a predictor of lineage diversity. To further assess the consequences of these host–parasite interactions for bird communities, future research should aim to disentangle the infection pathways in different ecosystems while also considering the vector community and environmental factors.
1. Introduction
Parasites play an important role in ecosystems and are known for their ability to influence population dynamics and shape communities (Gómez and Nichols, 2013; Poulin, 2014). However, these species have long been underrepresented in conservation and ecological studies (Gómez and Nichols, 2013; Okamura et al., 2018). Over the past few years, more studies have focused on the role of parasites in ecosystems and revealed that the effects on their hosts are manifold (Gómez and Nichols, 2013). Parasites can lead to a decrease in the abundance of hosts, loss of fitness, or behavioral changes in infected individuals (Gómez and Nichols, 2013). Overall, parasites are capable of modulating populations and communities of host species and help maintain ecosystem integrity (Gómez et al., 2012). These findings highlight the importance of monitoring parasites in different ecosystems to understand their functions and to consider them in conservation strategies.
Birds are a group of hosts that are of special interest for studies on the spread of diseases and parasitism. Due to their high mobility and the large proportion of migratory species, their parasites and pathogens have the opportunity to spread globally (Fuller et al., 2012). However, information on the spatial distribution of most avian parasites remains limited (Fuller et al., 2012). Mutations in parasites or changes in climatic conditions can lead to changes in distribution ranges, thereby inducing changes in the population of the host species (Atkinson and LaPointe, 2009; Garamszegi, 2011; Fuller et al., 2012; LaPointe et al., 2012; Loiseau et al., 2013; Alkishe et al., 2017).
Blood parasites of the order Haemosporida are a relatively well-studied group of avian parasites (Valkiūnas, 2005; Clark et al., 2014; Ishtiaq, 2021). They are found almost globally in most avian families (Valkiūnas, 2005; Bensch et al., 2009; Quillfeldt et al., 2011; Clark et al., 2014; Vanstreels et al., 2014; Masello et al., 2018). Haemosporida are known to affect plumage coloration (Romano et al., 2019), body condition (Valkiŭnas et al., 2006), reproductive success (Knowles et al., 2010), and survival (Bennett et al., 1993; Bueno et al., 2010) of avian hosts. The severity of the infection depends on the period of infection, infection intensity, initial infection dose, and physiological condition of the host (Valkiūnas, 2005; Dadam et al., 2019).
In recent years, research on host–parasite interactions has expanded to study single bird species (Ots and Hõrak, 1998; Atkinson et al., 2000; Garvin et al., 2003; Dadam et al., 2019; Romano et al., 2019; Names et al., 2021) using a community approach, investigating parasite prevalence differences and the effects of climate change, seasonality, habitat quality, and fragmentation on transmission and infection rates (Bonneaud et al., 2009; Garamszegi, 2011; Laurance et al., 2013; Ellis et al., 2015, 2017; Ferreira Junior et al., 2017; Harvey and Voelker, 2019; Ellis et al., 2020; Šujanová et al., 2021). Previous studies on host–parasite interactions at the community level have either been conducted in tropical ecosystems (Ferreira Junior et al., 2017; Fecchio et al., 2018; Lopes et al., 2020) or in wetland-associated ecosystems (Ellis et al., 2020; Šujanová et al., 2021). However, there is a lack of research in temperate managed forests, which limits our understanding of the distribution of host–parasite interactions within the community and how host–parasite interactions vary across different ecosystems. Additionally, human activities, such as logging and urbanization, are known to alter the distribution and abundance of bird species (Schulze et al., 2019) and parasites in ecosystems (Laurance et al., 2013; Santiago-Alarcon et al., 2013). Therefore, further research in temperate-managed forests is necessary to bridge this knowledge gap and provide a more comprehensive understanding of haemosporidian host–parasite interactions at the community level. To our knowledge, host–parasite interactions in European-managed forests have not been previously addressed.
Host–parasite characteristics in bird communities exhibit several features. Some bird families are more frequently affected by parasites. Some show high parasite prevalence or diversity, whereas others host only a few but highly specific parasites, and others are rarely infected (Ellis et al., 2017, 2020; Šujanová et al., 2021). It has been suggested that abundant host species show a higher parasite diversity due to more closely related species and conspecifics in the same ecosystem, but also a higher parasite prevalence due to higher exposure to vectors (Ellis et al., 2017). However, the connection between parasite prevalence and diversity in bird families is not yet fully understood.
In this study, we focused on the prevalence of haemosporidian parasites and Trichomonas spp. in a temperate forest bird community. The latter is reported to infest raptors and pigeons (Amin et al., 2014) and is caused by microaerophilic protozoa that induce lesions mainly in the upper digestive tract of birds and is transmitted either directly by feeding on conspecific individuals, e.g., in raptors, or indirectly through shared food or water sources (Atkinson et al., 2008; Amin et al., 2014). In 2005, populations of Chloris chloris declined in Great Britain due to infection with Trichomonas gallinae. Similar infection patterns have been observed in other countries (Peters et al., 2009; Lawson et al., 2012; Amin et al., 2014). Trichomonas gallinae is an example of host switching, as it was indirectly transmitted from Columbiformes to Passeriformes, especially to finches, and caused high mortality in their new host species (Lawson et al., 2012). Although infections with T. gallinae in passerines are best known from C. chloris, other passerines, such as Fringilla coelebs, Erithacus rubecula, and Turdus merula, have also been found to be infected (Quillfeldt et al., 2018).
The aim of this study was to assess and describe the overall prevalence, genetic lineage diversity, and underlying host–parasite network of the endoparasites Haemosporida (Plasmodium, Haemoproteus, and Leucocytozoon) and T. gallinae in a European forest bird community during the breeding season. We created a bipartite network and calculated common network parameters to gain insights into interaction diversity and patterns, such as shared lineages within and across avian host families and host specificity within a well-defined community. We hypothesized the following: (1) Parasite prevalence differs between host bird families and is expected to be higher in abundant families such as Paridae and Turdidae. (2) Some families host a higher diversity of parasite lineages, with abundant families exhibiting a higher diversity, and (3) the parasite diversity of bird species affects the parasite prevalence. Here, we also expected that common bird species, such as Parus major, Erithacus rubecula, Sylvia atricapilla, and Turdus merula have high parasite prevalence and lineage diversity. Unveiling the current infection patterns of endoparasites enables the recognition of potential changes within species or communities in the future.
2. Materials and methods
The study was conducted in the “Marburg Open Forest,” a 250-ha-sized managed forest in Hesse, Germany. The forest consists of mixed stands dominated by Fagus sylvatica and Quercus robur mixed with Pseudotsuga menziesii and Picea abies. Captures were conducted from mid-March to August for four consecutive years, starting in 2019. We set up 10 capture sites in the forest and conducted three sampling rounds in 2019 and six sampling rounds were conducted in each year from 2020 to 2022 (30/60 capture events per year). Birds were captured using a standardized set up with three mist nets (12 m length, 2.5 m height, mesh size 16 mm × 16 mm) in one row per site. Starting in 2020, we used high nets with double the height for every other capture event. At each capture event, the nets were open for 3 h during early morning. Birds were not attracted by food or playback. In addition, extra captures were carried out for some species; raptors and crows were caught using the Dho-Gaza method with a mist net (12 m length, 3 m height, mesh size 60 mm × 60 mm) and a stuffed eagle owl placed in front of the net at the forest edges. Additionally, we captured Phylloscopus sibilatrix during separate mist-netting events (mist net: 12 m length, 2.5 m height, mesh size 16 mm × 16 mm) with playback throughout the forest at locations where the males were singing. All birds were marked with rings to identify the recaptures and permission was obtained from the Heligoland Bird Observatory (Institut für Vogelforschung Heligoland, Germany). We sampled 476 birds belonging to 29 Palearctic species and investigated the prevalence of blood parasites (haemosporidian parasites) and Trichomonas spp. using molecular methods.
2.1. Sample collection
Blood was sampled from the captured birds with the permission of the regional council of Giessen, Hesse, Germany (V 54-19 c 20 15 h 01 MR 20/15 Nr. G 10/2019) by puncturing the brachial vein, and storing a minimal amount of blood on Whatman FTA Classic cards (Whatman, United Kingdom). The blood samples were kept dry until further processing. For the detection of possible infection with Trichomonas spp., we took saliva samples with oral swabs which were then stored in Trichomonas selective culture medium (Trichomonas medium REF EB0861C, OXOID Deutschland GmbH, Wesel, Germany) and incubated at 37°C for five to 7 days. Samples were fixed after the first centrifugation (3,200 rpm for 5 min). We added 1 mL of Phosphate-Buffered Saline (PBS), centrifuged once more (3,200 rpm, 5 min), removed the PBS, and stored the samples in a freezer for further processing (Quillfeldt et al., 2018).
2.2. Sequencing
Blood and saliva samples were further processed for DNA sequencing in an external laboratory (Ecogenics GmbH, Balgach, CH). DNA was extracted and isolated using the DNeasy Blood and Tissue Kit (QIAGEN, Valencia, CA, United States). PCR was conducted using parasite-specific primer pairs, and blood samples were sequenced using nested PCR (Table 1, Hellgren et al., 2004; Quillfeldt et al., 2018). To exclude laboratory errors, positive and negative controls were included in all PCRs. The sequences were uploaded to GenBank® and are available under the accession numbers OQ311003–OQ311317.
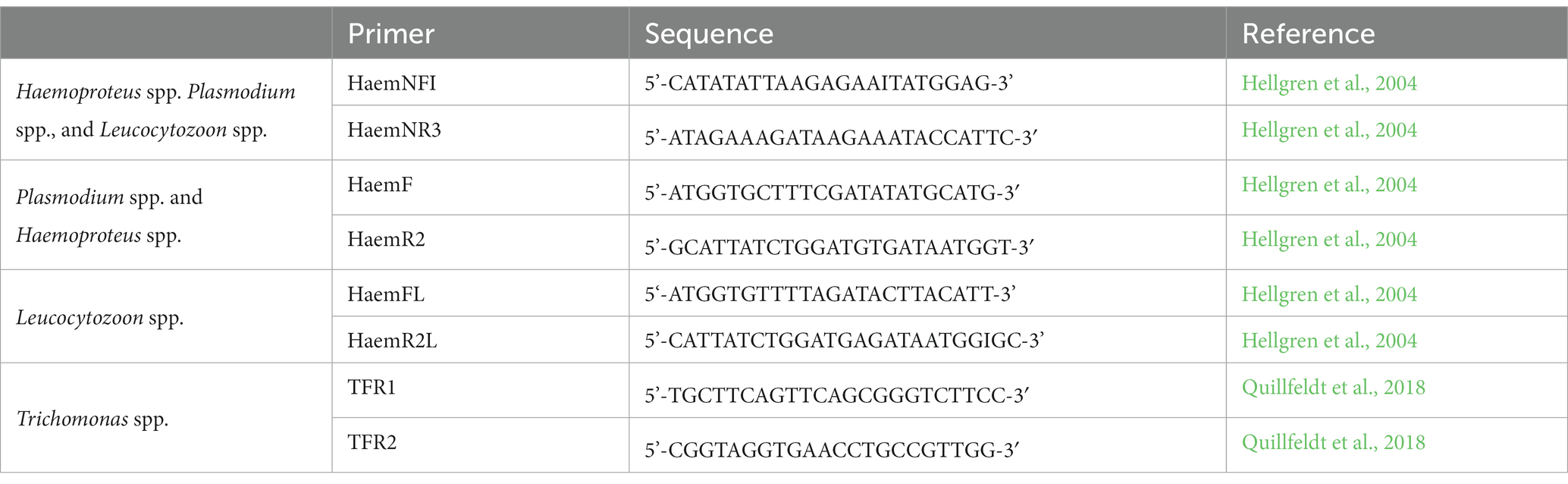
Table 1. Primer pairs for the detection of Haemosporida (cyt b) and Trichomonas spp. (ITS1-5.8S-ITS2).
2.3. Data processing and statistics
The resulting sequences (~480 bp) of the mitochondrial cytochrome b gene of Haemosporida were trimmed and assembled using the CodonCode Aligner software (CodonCode Corp., Dedham, MA). Except for 53 samples determined only to the genus level because of low-quality regions (possibly indicative of multiple infections), all sequences were free from conflicts in the forward–reverse alignment and had the same length as the reference data of the MalAvi database (Bensch et al., 2009). Samples were assigned to a parasite lineage, using the reference data of the MalAvi database (Bensch et al., 2009) and the ‘Identify Sequence’ tool of CodonCode Aligner. We compared the sequences to the best match of the reference sequences, and in the case of a 100% match, assigned to a lineage. Some samples (53) could not be matched 100% to one lineage, owing to low-quality regions. The quality of these samples was maintained even after repeated sequencing. Thus, we determined these samples to the genus level and hereafter refer to them as Lineage undetermined. This was the case for 46 Leucocytozoon spp. samples and eight Haemoproteus spp. samples (one sample was positive for both genera). There were no problems in assigning lineages to any Plasmodium spp. positive samples. For repeat samples from recaptured birds, additional lineages were added to the dataset of the respective birds.
To assess and describe our host–parasite community, we assessed the prevalence of each parasite genus in each bird species. We created a stacked bar chart to show the abundance of bird species and the parasite prevalence of the three genera using R (R Core Team, 2022) and the package ggplot2 (Wickham, 2016). To elucidate interactions between host bird species and parasite lineages, we compiled an interaction network using the bipartite package (Dormann et al., 2008) in R. To describe the host parasite network, we calculated further network-specific parameters and values. The degree indicates the sum of interactions per species or parasite lineage (Dormann et al., 2008), the paired differences index (PDI) provides information on the generality (0) or specificity (1) of the species or parasite lineage (Poisot et al., 2012), and effective partners is the effective number of interacting partners (hosts or parasites) if each partner was equally common (Bersier et al., 2002) at the parasite and host levels (Tables 2, 3).
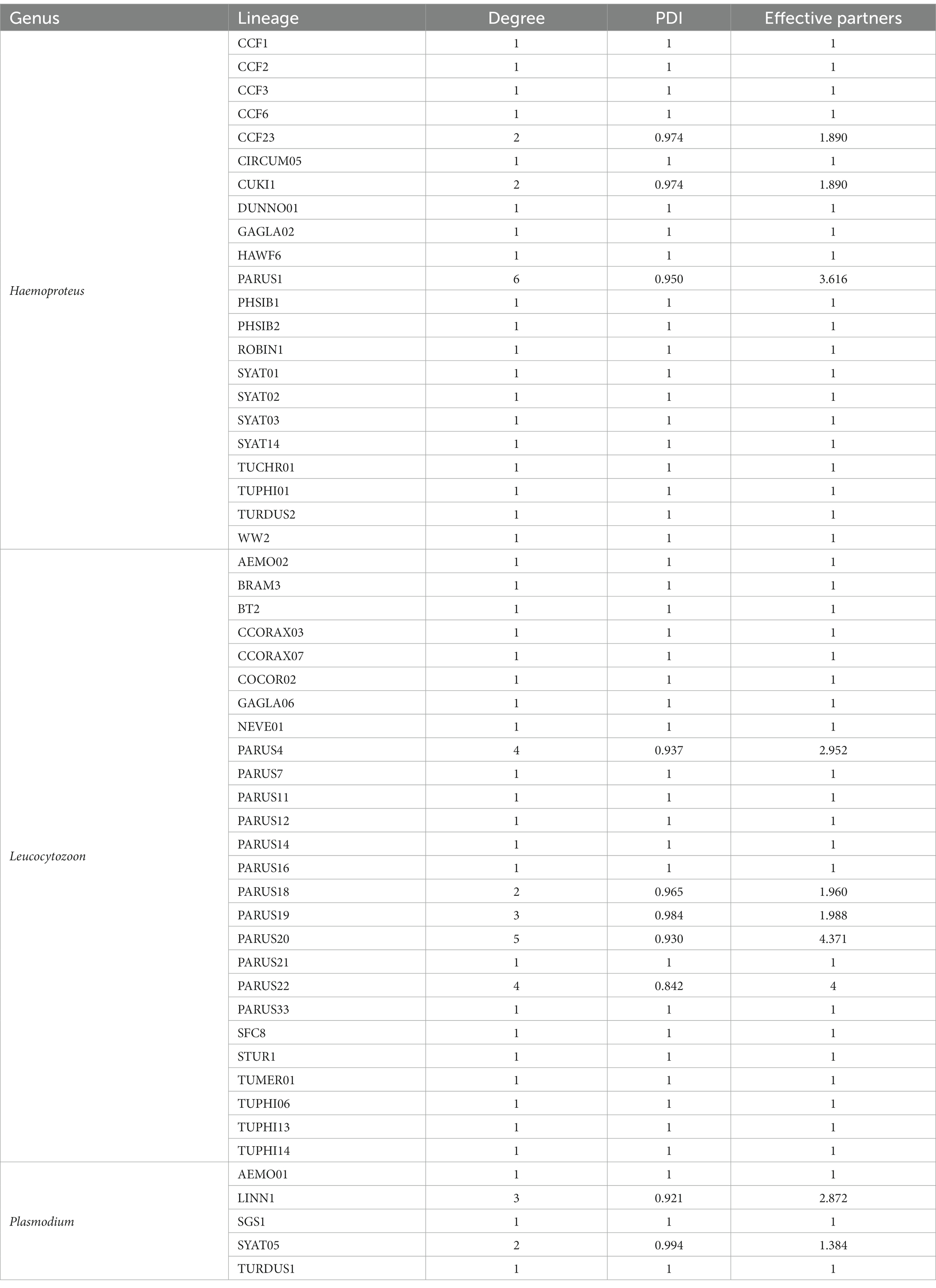
Table 2. Network information on haemosporidian lineages: degree indicates the sum of hosts per parasite lineage, paired differences index (PDI) provides information on the generality (0) or specificity (1) of this lineage and effective partners is the effective number of partners if each partner was equally common (Dormann et al., 2008).
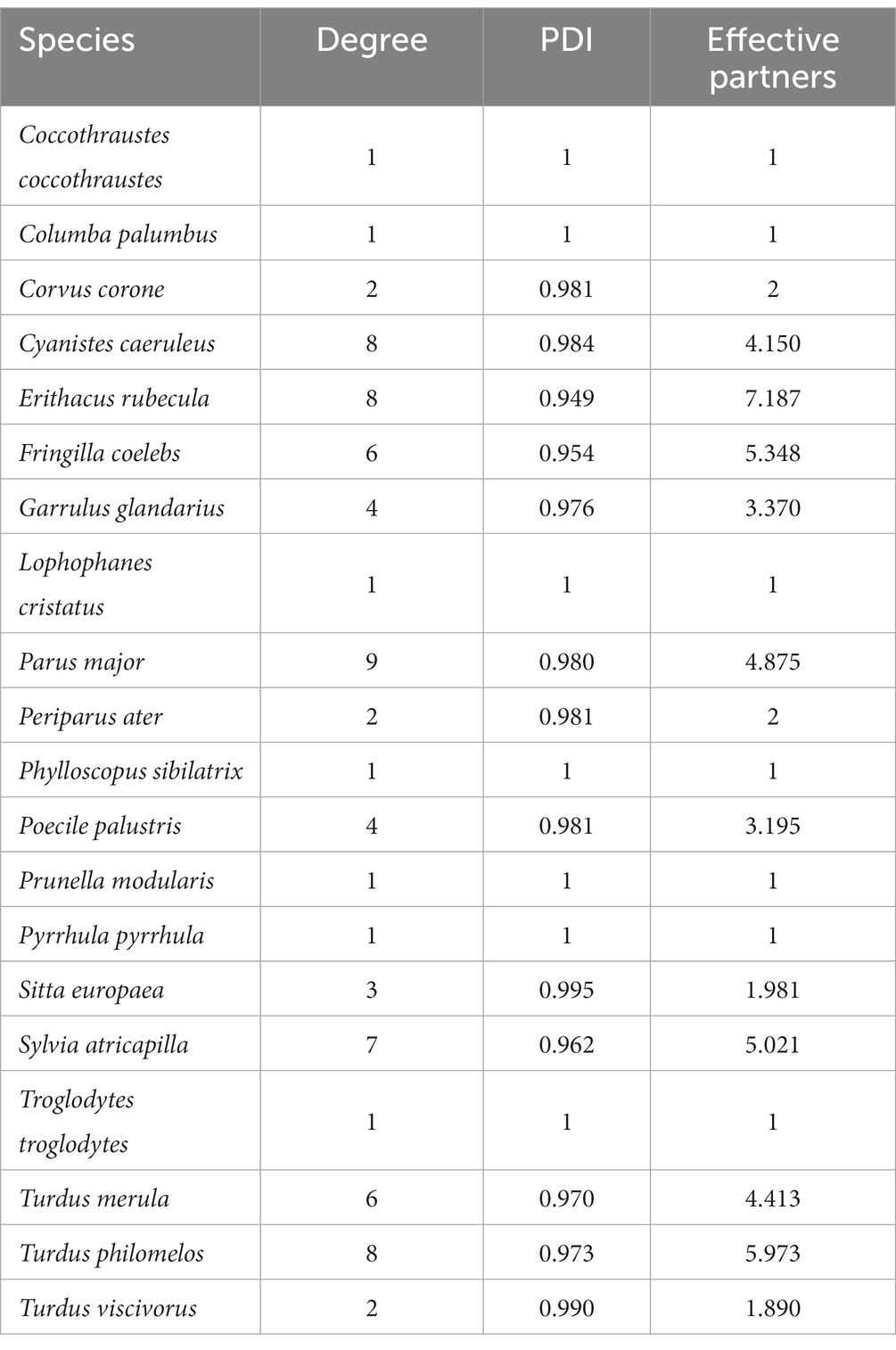
Table 3. Network information on 20 infected bird species: degree indicates the sum of links per species, paired differences index (PDI) provides information on the generality (0) or specificity (1) of this species and effective partners is the effective number of partners if each partner was equally common (Dormann et al., 2008).
We tested for differences in parasite prevalence (proportion of infected individuals) between bird families by performing a chi-square test in R. To assess differences in lineage diversity (number of haemosporidian lineages) among bird families, we used a chi-square goodness-of-fit test, assuming an even distribution of lineages among all bird families. For both tests, we included only bird families with at least ten individuals, to avoid overestimation of parasite prevalence or lineage diversity based on a small sample size. We calculated a mixed-effects model to test the effect of effective partners on parasite prevalence in bird species using the lme4 package (Bates et al., 2015). We included family as a random effect to correct for closely related species and tested for the impact of family by calculating the marginal and conditional r-squared values.
3. Results
Over the course of four breeding seasons, we captured and sampled 483 individual birds (68 recaptures) belonging to 29 species and collected 542 blood and 396 saliva samples. Four of the bird species were represented by only one individual. The species-specific sample sizes are shown in Figure 1.
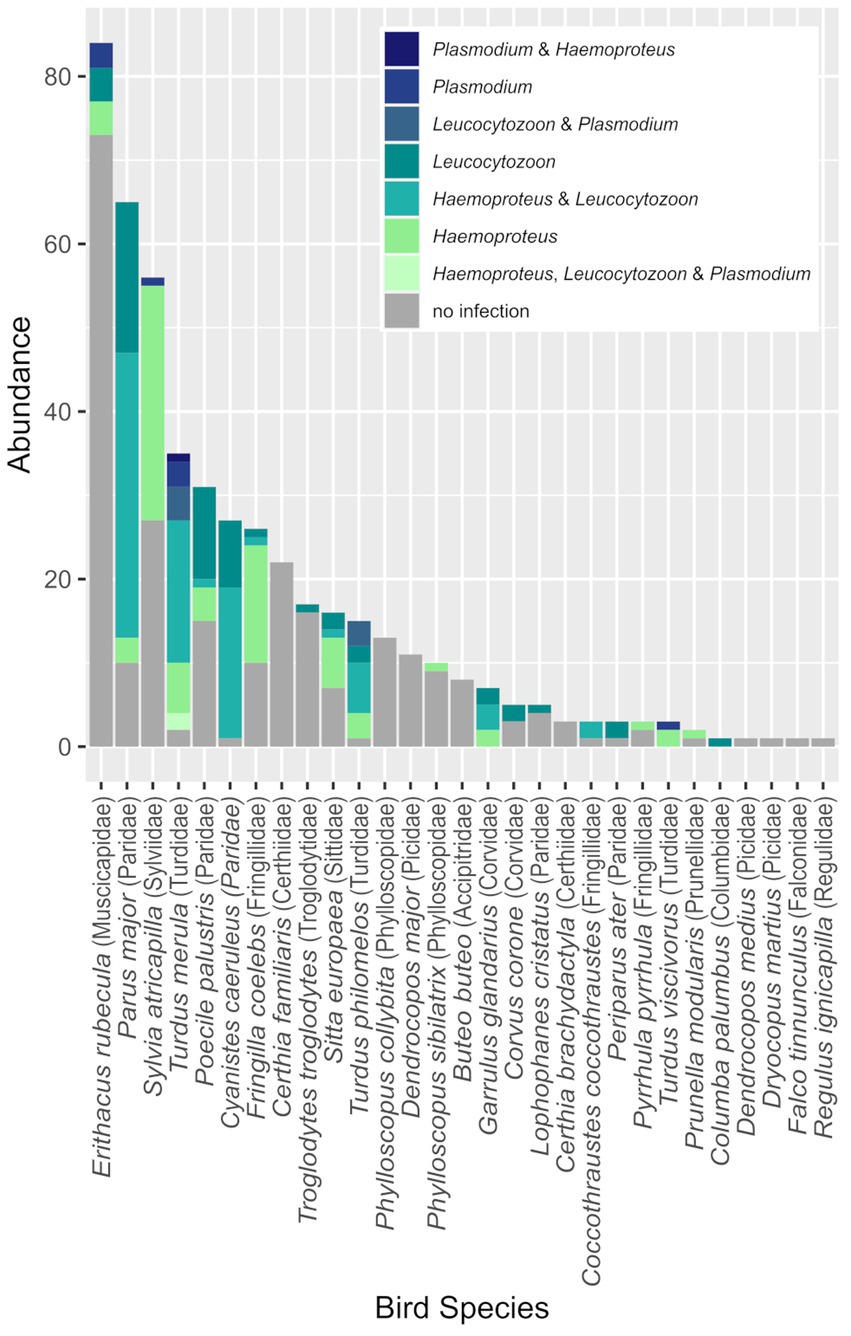
Figure 1. Abundance of the bird species (families given) and the corresponding parasite prevalence. The colors indicate haemosporidian genera as indicated in the legend.
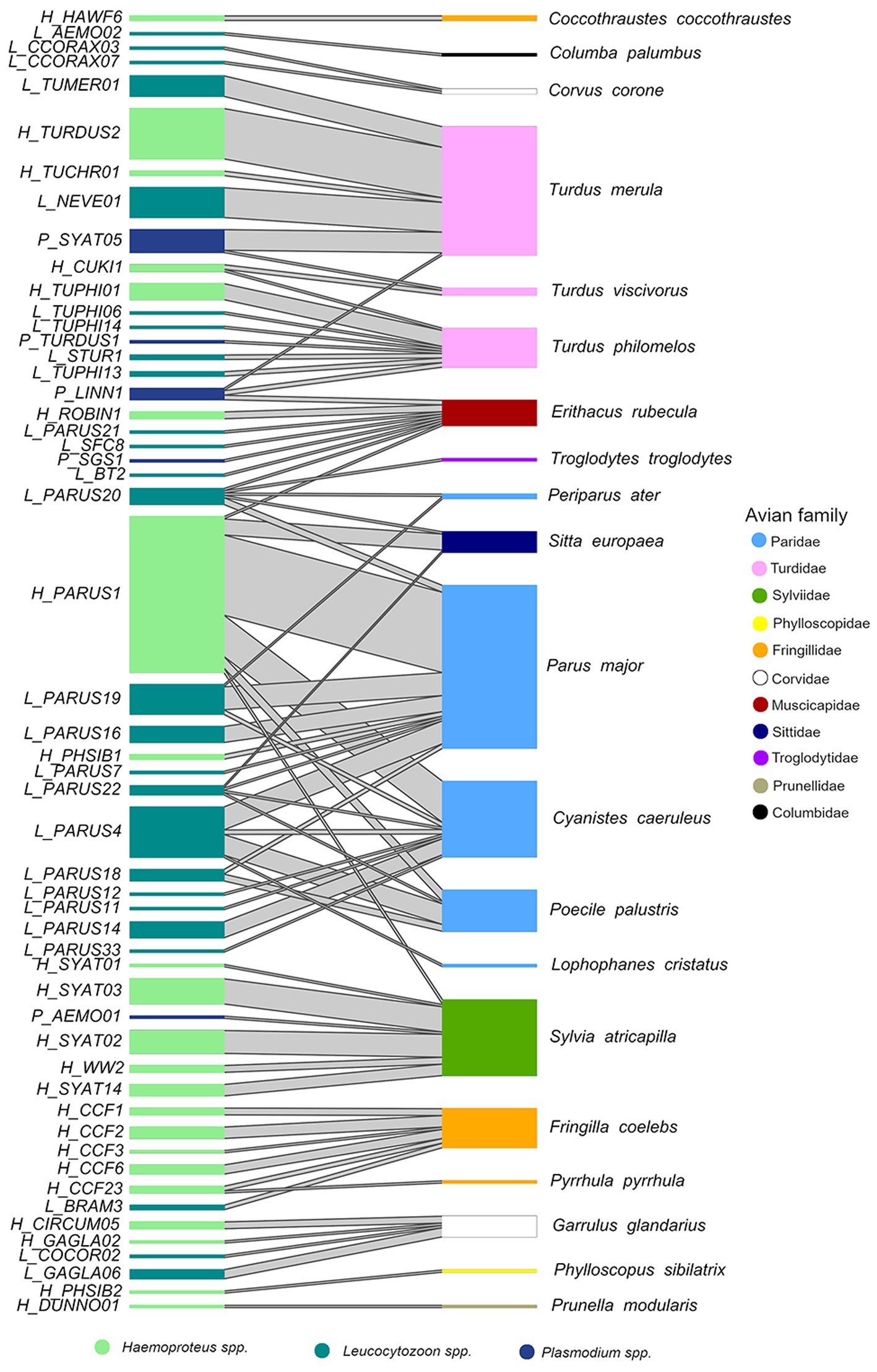
Figure 2. Interaction network of parasite lineages (left) and the host bird species (right). The first letter of the lineage indicates the genus: H (Haemoproteus spp.), P (Plasmodium spp.), and L (Leucocytozoon spp.). The width of the boxes indicates the abundance of the species (e.g., C. palumbus = 1, P. major = 68) or lineage (e.g., L_AEMO02 = 1, H_PARUS1 = 68) and the width of the connecting line indicates the abundance of this interaction (e.g., H_PARUS1 – P. major = 35).
We detected Haemosporida infections in almost half of our samples (48.1%, including 53 samples with genus undetermined). In 14 bird species, we detected blood parasites in at least half of the individuals (note that one of the 14 species was represented by only one individual; Figure 1). Infections with Haemoproteus spp. were the most common (67.7% of infected samples), followed by Leucocytozoon spp. infections (59.8%). Only 7.9% of Haemosporida-positive individuals were infected with lineages belonging to Plasmodium spp. Distribution of the three blood parasite genera was unequal within the bird community. Sylvia atricapilla was not infected with Leucocytozoon spp., whereas all but one (uninfected) of the sampled individuals of Cyanistes caeruleus were positive for Leucocytozoon spp., most of them in combination with Haemoproteus spp. Plasmodium spp. were found in only five of the sampled species: three Turdus spp., E. rubecula, and S. atricapilla (Figure 1).
We did not detect any infection with T. gallinae within the bird community.
3.1. Host–parasite network
Overall, we identified 53 haemosporidian parasite lineages, including 22 lineages of Haemoproteus spp., 26 lineages of Leucocytozoon spp., and five lineages of Plasmodium spp. (Supplementary Figures S1-3). All the detected parasite lineages have been previously described. The most frequent lineage, H_PARUS1 (H. majoris, found in 98 birds), was detected in P. major (66.3% of all H_PARUS1 positive samples), C. caeruleus (18.4%), E. rubecula (1.0%), Poecile palustris (5.1%), Sitta europaea (7.1%), and S. atricapilla (2.0%). The second most common lineages were L_PARUS4 and H_TURDUS2 (22 birds each). L_PARUS4 was found in four host species, namely C. caeruleus, Lophophanes cristatus, P. major, and P. palustris, whereas H_TURDUS2 was only found in T. merula (Figure 2).
At the parasite level the Haemoproteus spp. lineage PARUS1 had the highest degree value (6) and compared to the other Haemoproteus spp. lineages, it was the most generalist lineage (PDI = 0.950) and had the highest number of effective partners (3.616). The most generalized lineage in our network was Leucocytozoon spp. PARUS20 (PDI = 0.84) which was also the lineage with the most effective partners (4.37) among the three genera. PARUS20 also had the highest degree (5) of Leucocytozoon spp. lineages. In the Plasmodium spp. lineages, LINN1 had the most interactions (3), the lowest PDI (0.921), and the most effective partners (2.872). On the host side of the network, the species with the highest degree value, and thus most parasite lineages, were P. major (9), followed by E. rubecula (8), C. caeruleus (8), and T. philomelos (8). Although we detected a low parasite prevalence in E. rubecula (13.1%), it was the most generalized host species (PDI = 0.949) and had the highest number of effective partners (7.187).
3.2. Parasite prevalence and lineage diversity
As hypothesized, parasite prevalence was not evenly distributed among bird families (N ≥ 10) in the studied community (chi-square test: χ2 (10, N = 462) = 196.4, p < 0.01). The prevalence of haemosporidian parasites was the highest in Turdidae (94.3%, 50/53 individuals) and Paridae (75.6%, 99/131 individuals). In contrast, the Certhiidae (25 individuals) and Picidae (13 individuals) were not infected (Figure 1, Supplementary Table S4). Likewise, lineage diversity (number of parasite lineages per host family) was significantly different between avian families, as confirmed by the chi-square goodness-of-fit test, χ2 (10, N = 59) = 43.0, p < 0.01.
There was no significant effect of effective partners on parasite prevalence (estimate = 0.039; std. error = 0.034, p = 0.257). To estimate the proportion of variation explained by the fixed and random effects, we computed the marginal and conditional R-squared values. While the fixed effect of effective partners had a modest effect (R2 = 0.03) in explaining the variation in parasite prevalence among infected bird species, the random effect of bird family had a more substantial effect (R2 = 0.39).
4. Discussion
We detected an overall prevalence of haemosporidian parasites of 48% (N = 229/475) in a forest bird community consisting of 29 bird species belonging to 16 avian families and detected 53 haemosporidian lineages. The recorded infections were not equally distributed across the community, with some abundant families and species being more frequently infected (e.g., Turdidae and Paridae) than others. Other rare families, such as Picidae and Certhiidae, were not infected. In contrast to the high prevalence of Haemosporida, we did not detect a single infection of T. gallinae in the studied bird community.
4.1. Haemosporidian prevalence and host-specificity
Infections with the three haemosporidian genera were commonly found in the forest bird communities. Compared with another study from central Germany, we found a higher prevalence of Haemoproteus spp. and Leucocytozoon spp. (current study: H: 66.7%, L: 59.8%; Schumm et al., 2019: H: 31.3%, L: 47.5%), whereas Plasmodium spp. was less commonly found in our area (current study: 7.9%, Schumm et al., 2019: 12.5%). Another study conducted in a woody wetland in Slovakia found a higher prevalence of Haemoproteus spp. and Plasmodium spp. (96%) and a lower prevalence of Leucocytozoon spp. than those in our study (37%, Šujanová et al., 2021); however, they did not address Haemoproteus spp. and Plasmodium spp. separately. To date, the drivers of such regional differences are not completely known, but habitat suitability and vector abundance may contribute to these different patterns. For instance, Schumm et al., 2019 discussed that the high prevalence of Leucocytozoon spp. could be driven by the number of forests and running water bodies in and around the study area, which are suitable for the reproduction of Simuliidae, the vectors of Leucocytozoon spp. (Zahar, 1951; Hellgren et al., 2008). However, whether and how climatic and environmental factors determine the distribution of haemosporidian parasites remain unclear. Whereas Ellis et al. (2015) found that the local distributions of Haemoproteus spp. and Plasmodium spp. were driven by local host populations rather than by environmental factors, Gonzalez-Quevedo et al. (2014) found that the minimum temperature throughout the year was an important predictor of the prevalence of Plasmodium spp. Valkiūnas (2005) suggested that the transmission of Plasmodium spp. decreased in Europe north of 55° N, potentially because of low temperatures in winter, which is one possible explanation for the low prevalence of Plasmodium spp. in our study. The other two Haemosporida genera, Haemoproteus spp. and Leucocytozoon spp., are known to be transmitted at lower temperatures than those at which Plasmodium spp. is transmitted (Mozaffer et al., 2022). The diversity and prevalence of Leucocytozoon spp. increased at higher latitudes (Fecchio et al., 2020). The local adaptations of hosts and parasites have also been discussed to cause regional differences (Ellis et al., 2015). To the best of our knowledge, this hypothesis has not been tested yet. It is likely that the regional differences we found were not driven by a single factor, but rather by a combination of climatic factors, vector abundance, and regional host and vector community structures.
There were not only differences in the overall prevalence of Haemosporida infection, but we also found differences in the host–parasite network compared to other studies. When comparing the number of lineages of each haemosporidian genus with those in other studies, our study revealed a comparably low number of lineages (Ellis et al., 2020; Šujanová et al., 2021). The proportion of lineages per genus, defined as the number of lineages within a genus divided by the total number of lineages found, was found to be very similar between Sweden and Slovakia. Specifically, the proportions of Plasmodium spp., Haemoproteus spp., and Leucocytozoon spp. were 14%/13, 46%/47, and 40%/40%, respectively (Ellis et al., 2020; Šujanová et al., 2021). However, our analysis revealed a lower percentage of Plasmodium spp. lineages (9%) and a relatively higher percentage of Leucocytozoon spp. lineages (49%), as reported by Ellis et al. (2020) and Šujanová et al. (2021). This could be caused by fewer host species and a drier and less diverse habitat in our study compared to those of studies from Sweden and Slovakia, where the study area consisted of woody wetlands and forest meadows (Šujanová et al., 2021) or deciduous forests mixed with planted spruce, partly wet soils with small ponds, reed beds, willow thickets, and wet meadows surrounded by dry-grazed grasslands on sandy soils (Ellis et al., 2020).
To further resolve geographical differences in host–parasite interactions, better knowledge of the transmission sites is needed. Migratory birds may have been infected at their stopover sites or their wintering grounds. They have been found to have a higher parasite prevalence and diversity than resident species (de Angeli Dutra et al., 2021a; Huang et al., 2022) and have the potential to disperse parasites along their migratory routes, enhancing the spread of parasites (de Angeli Dutra et al., 2021b). Previous studies have found that most lineages that infect migratory birds are acquired during migration rather than in their breeding grounds (Huang et al., 2022). However, it is not possible to determine whether the lineages found in this study were transmitted in our forest ecosystem or even in Europe, as some of the host species we sampled were migratory birds. Because of the low proportion of juvenile birds that had not yet migrated and the fact that we did not sample potential vectors, we were not able to disentangle the transmission sites in this study.
In terms of the host specificity of the parasite network presented here, three out of 22 Haemoproteus spp. lineages (13.6%), five out of 26 Leucocytozoon spp. lineages (19.2%) and two out of five Plasmodium spp. lineages (40%) were found in several hosts. This suggests that, in general, Plasmodium spp. are generalists, whereas Haemoproteus spp. seemed to be more host-specific, which is in line with the findings of other studies (Ellis et al., 2020). However, the most generalist lineage of our network was not a Haemoproteus spp. lineage but a Leucocytozoon spp. lineage that was not found in the Swedish study (Ellis et al., 2020) and was slightly more specific in the Slovakian study (Šujanová et al., 2021). Contrary to the hypothesis posited by Poulin (1998) that a higher prevalence of host-specific parasite lineages than generalist lineages, our findings revealed that the most frequently detected lineage (Haemoproteus spp. PARUS1) is a generalist one. Notably, this lineage demonstrated the highest prevalence across three host species, and also infected three additional species, albeit with a lower prevalence. Our findings are in line with those of Hellgren et al. (2009), who reported that parasites exhibiting a broad host range were the most prevalent in their respective host species. The ability to infect multiple host species enhances parasite transmission by increasing the number of potential hosts and the likelihood of successful transmission by vectors (Hellgren et al., 2009). This could explain why some generalist parasites attain a high prevalence within their host species.
4.2. Parasite prevalence and lineage diversity of avian hosts
As expected, at the host level of the network, we found significant differences in parasite prevalence and lineage diversity among bird families. Turdidae (94.3%) and Paridae (75.6%) had a particularly high parasite prevalence. Valkiūnas (2005) has earlier described that Passeriformes host the greatest number of haemosporidian lineages. Even within the order Passeriformes, some families such as Fringillidae, Muscicapidae, Paridae, Sylviidae, and Turdidae appear to be more susceptible to haemosporidian parasites (Valkiūnas, 2005). All these families, except Muscicapidae, also had a parasite prevalence of over 50% in our study (see Supplementary Table S4). We found similar patterns at the species level, with some abundant species having particularly high parasite prevalence, such as C. caeruleus (96.3%), P. major (78.3%), and T. merula (94.3%). In contrast, certain Passeriformes, such as Certhiidae, Troglodytidae, and Sittidae, exhibited a comparatively lower parasite prevalence (Valkiūnas, 2005). With the exception of the family Sittidae, which had a prevalence of over 50% in our study, our results are consistent with previous findings (Valkiūnas, 2005). Several species were not infected at all in our study, such as P. collybita or C. familiaris. In contrast, Šujanová et al. (2021) detected that two of nine C. familiaris individuals were infected with Plasmodium spp. in their study in Slovakia.
The high parasite prevalence in certain bird families and species may be driven by several factors. One possible explanation could be that the highly abundant species and families found in our study are not only common in forests, but are also abundant in different ecosystems (Gedeon et al., 2022). This could lead to an increased vector-host encounter rate (Medeiros et al., 2013; Ellis et al., 2017). Furthermore, the phylogenetic relationships among birds could lead to a high parasite prevalence (O’Connor et al., 2016; Ellis et al., 2020; Huang et al., 2022). Birds with many closely related species have similar immune functions that allow the parasite to utilize several closely related species as hosts, leading to both high parasite prevalence and similar parasite communities (Davies and Pedersen, 2008; O’Connor et al., 2016; Clark and Clegg, 2017; Ellis et al., 2020). Specifically, phylogenetically closely related host species display comparable haemosporidian parasite prevalence and lineage composition (Ellis et al., 2020; Huang et al., 2022). A common bird family showing this pattern is the Paridae family, which shared a considerable number of lineages in our study. In contrast, only a few shared lineages have been identified in the Turdidae. The reasons for the high degree of lineage sharing among Paridae birds and their rarity in the Turdidae remain to be elucidated. Several possible explanations exist, including a weaker immune response of Paridae species to haemosporidian parasites, making them more vulnerable to haemosporidian infections than Turdidae species (O’Connor et al., 2016). Furthermore, ecological factors could have contributed to this observation, as Paridae birds tend to occur at higher densities, and various species of Paridae coexist within a given ecosystem, whereas Turdidae birds generally have lower densities and do not overlap as much in their use of habitats (Poulin, 1998). The degree to which lineage sharing is influenced by phylogenetic relationships versus ecological and behavioral factors is uncertain. Nevertheless, it is likely that multiple factors contribute to lineage sharing among different families.
As observed in the overall prevalence of haemosporidian genera, there are regional differences in the susceptibility of host species to Haemosporida. These differences could be attributed to several factors. Host-specific factors for high parasite prevalence include breeding abundance, higher exposure to vectors (Kilpatrick et al., 2006; Ellis et al., 2017), and evolutionary distinctiveness, as closely related host species are often infected by the same generalist parasites (Parker et al., 2015; Ellis et al., 2020). In addition to that there are also environmental factors promoting high parasite prevalence and diversity such as habitat quality and seasonal dynamics (Wood et al., 2007; Belo et al., 2011; Ferreira Junior et al., 2017; Šujanová et al., 2021). Parasite prevalence appears to be higher in urbanized areas close to rivers, tropical rainforests, and pastures (Wood et al., 2007; Belo et al., 2011; Ferreira Junior et al., 2017). To our knowledge, information on parasite prevalence in managed beech forests in Europe is not yet available. This makes it difficult to assess whether the parasite prevalence in our study is high due to habitat type or quality, or whether this is the typical parasite prevalence in managed temperate forests. Furthermore, we only captured and sampled birds during the breeding season, which is known for its high parasite prevalence (Huang et al., 2020) and could also partly explain the high parasite prevalence we found. The condition of birds, resistance to certain parasites, migratory patterns, nest type, and height of the foraging stratum have also been discussed as predictors of parasite prevalence and diversity; however, these were not assessed in this study (Hing et al., 2016; Ellis et al., 2017; Ricklefs et al., 2017; Ilgūnas et al., 2019; Names et al., 2021; Šujanová et al., 2021).
Given that parasite prevalence and lineage diversity differ between bird families and species, we hypothesized that abundant bird species are simultaneously characterized by both high lineage diversity and thus a higher parasite prevalence at the same time (Ellis et al., 2017). In contrast to this hypothesis, we did not find any effect of effective partners on parasite prevalence in the bird species in our network. This theory might apply for some species, such as P. major which had both a high parasite prevalence and high lineage diversity. However, E. rubecula was our biggest outlier and had a very low parasite prevalence (13.1%) but at the same time a high lineage diversity and was the most frequently caught species in our community. Another study from Hungary reported a similar parasite prevalence in E. rubecula (14.9%, Ágh et al., 2019). However, parasite prevalence was not always as low as that in our Hungarian study (Ágh et al., 2019). A study from Sardinia found an almost five-fold higher parasite prevalence in E. rubecula which might be due to the lower latitude of the study area and thus different vector communities (61.5%, Pellegrino et al., 2021). Since we did not use microscopy to verify the infection, some of the infections found in E. rubecula could have been abortive; thus, the actual lineage diversity might have been lower.
We did not detect an effect of effective partners on parasite prevalence; in fact, the random effect of bird family explained more variance than effective partners. The absence of a correlation between the effective partners and parasite prevalence could be attributed to several factors. There may be a trade-off in parasites between host specificity and the ability to achieve high parasite prevalence (Poulin, 1998; Hellgren et al., 2009). Parasite lineages that are highly specific to a single host species may result in high parasite prevalence, whereas generalist lineages are capable of infecting several hosts but typically do not achieve the same level of prevalence as specific lineages (Poulin, 1998). Furthermore, certain host species might be resistant to certain generalist haemosporidian parasites (Ilgūnas et al., 2019), whereas other species are less exposed to vectors, show a low parasite prevalence, and are therefore not well adapted to the parasites, and thus host a larger range of parasite lineages (Ellis et al., 2017). In contrast, generalist parasite lineages were capable of achieving higher levels of prevalence in their host species than in specific lineages (Hellgren et al., 2009). Even though we could not concretely determine why a high parasite prevalence does not predict high lineage diversity, we found that host abundance did not correlate with either of these factors.
4.3. The absence of Trichomonas spp.
Although we found a high prevalence of haemosporidian parasites, Trichomonas spp. was absent in the studied bird community. However, infections with Trichomonas spp. in Passeriformes are not very common (Forrester and Foster, 2008) despite local outbreaks of trichomonosis in Chloris chloris and F. coelebs since 2005 (Lawson et al., 2011). Climatic factors have been hypothesized to play a role in the emergence of this disease; however, these were not considered in this study (Amin et al., 2014). Dry weather and low rainfall are suggested to promote the transmission of Trichomonas spp., and infections peak in late summer and early fall (Simpson and Molenaar, 2006; Bunbury et al., 2007; Lawson et al., 2012). The absence of Trichomonas spp. in our study is thus not very surprising, as the bird species of the studied community are not known to be frequent hosts, and we did not take samples in late summer or early spring.
4.4. Conclusion
Our study revealed a high prevalence of haemosporidian parasites in a forest bird community, as well as differences in the susceptibility of different avian families and species, which could potentially be driven by host abundance and habitat choice. The prevalence of different haemosporidian genera, however, differed from those reported in other studies from across Europe. This shows the importance of monitoring host–parasite interactions in different habitats, both natural and human-dominated. The causes of the different susceptibilities of bird families and species remain unclear. As endoparasites are spreading globally in response to climate change and can significantly influence the behavior, reproduction, and mortality of their hosts, our findings highlight the need to monitor host–parasite interactions to better understand interaction dynamics. The inclusion of vectors and environmental parameters can further help to identify the drivers of regional differences.
Data availability statement
The original contributions presented in the study are included in the article/Supplementary materials, further inquiries can be directed to the corresponding author.
Ethics statement
The animal study was reviewed and approved with permissions for animal testing by regional council Giessen, Hesse, Germany (V 54-19 c 20 15 h 01 MR 20/15 Nr. G 10/2019).
Funding
This project was part of the Natur 4.0 project funded by LOEWE (National Offensive for the Development of Scientific and Economic Excellence). Open access funding provided by the Open Access Publishing Fund of Philipps-Universität Marburg with support of the Deutsche Forschungsgemeinschaft (DFG, German Research Foundation).
Author contributions
FS contributed to the conception and design of the study, carried out the field and laboratory work, performed the statistical analyses, and wrote the manuscript. NF, DS, and SR designed and structured the study. PQ, JM, and YS helped with conceptual and analytical approaches and supported the lab and fieldwork. MB, KL, DS, and SR contributed to fieldwork. YS introduced and helped with both field and laboratory work. NF, DS, and SR contributed to the statistical analysis. All authors contributed to manuscript revision and have read and approved the submitted version.
Acknowledgments
This study was part of the Project Natur 4.0—Sensing Biodiversity Initiative funded by the LOEWE of the state of Hesse, Germany. We thank M. Ehmig, M. Jappe, S. Thews, C. Guckenbiehl, A. Maier, M. Fuchs, J. Kröger, and J. Schmitt for field and laboratory assistance. We thank Esther Meißner for supporting the field and laboratory work.
Conflict of interest
The authors declare that the research was conducted in the absence of any commercial or financial relationships that could be construed as a potential conflict of interest.
Publisher’s note
All claims expressed in this article are solely those of the authors and do not necessarily represent those of their affiliated organizations, or those of the publisher, the editors and the reviewers. Any product that may be evaluated in this article, or claim that may be made by its manufacturer, is not guaranteed or endorsed by the publisher.
Supplementary material
The Supplementary material for this article can be found online at: https://www.frontiersin.org/articles/10.3389/fevo.2023.1107736/full#supplementary-material
SUPPLEMENTARY FIGURE S1 | A median-joining network of the mitochondrial cytochrome b lineages of Haemoproteus spp. (480 bp fragment, Hellgren et al., 2004). Each circle represents one lineage. The size of the circle indicates the frequencies of the lineages and the colors show in which host species the lineage was found. Hatch marks represent number of mutations between lineages.
SUPPLEMENTARY FIGURE S2 | A median-joining network of the mitochondrial cytochrome b lineages of Leucocytozoon spp. (478 bp fragment, Hellgren et al., 2004). Each circle represents one lineage. The size of the circle indicates the frequencies of the lineages and the colors show in which host species the lineage was found. Hatch marks represent the number of mutations.
SUPPLEMENTARY FIGURE S3 | A median-joining network of the mitochondrial cytochrome b lineages of Plasmodium spp. (480 bp fragment, Hellgren et al., 2004). Each circle represents one lineage. The size of the circle indicates the frequencies of the lineages and the colors show in which host species the lineage was found. Hatch marks represent the number of mutations.
References
Ágh, N., Piross, I. S., Majoros, G., Csörgő, T., and Szöllősi, E. (2019). Malaria infection status of European robins seems to associate with timing of autumn migration but not with actual condition. Parasitology 146, 814–820. doi: 10.1017/S0031182018002184
Alkishe, A. A., Peterson, A. T., and Samy, A. M. (2017). Climate change influences on the potential geographic distribution of the disease vector tick Ixodes ricinus. PLoS One 12:e0189092. doi: 10.1371/journal.pone.0189092
Amin, A., Bilic, I., Liebhart, D., and Hess, M. (2014). Trichomonads in birds—a review. Parasitology 141, 733–747. doi: 10.1017/S0031182013002096
Arneberg, P., Skorping, A., Grenfell, B., and Read, A. F. (1998). Host densities as determinants of abundance in parasite communities. Proc. R. Soc. London B 265, 1283–1289. doi: 10.1098/rspb.1998.0431
Atkinson, C. T., Dusek, R. J., Woods, K. L., and Iko, W. M. (2000). Pathogenicity of avian malaria in experimentally-infected Hawaii Amakihi. J. Wildl. Dis. 36, 197–201. doi: 10.7589/0090-3558-36.2.197
Atkinson, C. T., and LaPointe, D. A. (2009). Introduced avian diseases, climate change, and the future of Hawaiian honeycreepers. J. Avian Med. Surg. 23, 53–63. doi: 10.1647/2008-059.1
Atkinson, C. T., Thomas, N. J., and Hunter, D. B., Eds. (2008). Parasitic Diseases of Wild Birds. Ames, Iowa: Wiley-Blackwell.
Bates, D., Mächler, M., Bolker, B., and Walker, S. (2015). Fitting linear mixed-effects models using lme4. J. Stat. Soft. 67, 1–48. doi: 10.18637/jss.v067.i01
Belo, N. O., Pinheiro, R. T., Reis, E. S., Ricklefs, R. E., and Braga, É. M. (2011). Prevalence and lineage diversity of avian haemosporidians from three distinct cerrado habitats in Brazil. PLoS One 6:e17654. doi: 10.1371/journal.pone.0017654
Bennett, G. F., Peirce, M. A., and Ashford, R. W. (1993). Avian haematozoa: mortality and pathogenicity. J. Nat. Hist. 27, 993–1001. doi: 10.1080/00222939300770621
Bensch, S., Hellgren, O., and Pérez-Tris, J. (2009). MalAvi: a public database of malaria parasites and related haemosporidians in avian hosts based on mitochondrial cytochrome B lineages. Mol. Ecol. Resour. 9, 1353–1358. doi: 10.1111/j.1755-0998.2009.02692.x
Bersier, L.-F., Banašek-Richter, C., and Cattin, M.-F. (2002). Quantitative descriptors of food-web matrices. Ecology 83, 2394–2407. doi: 10.1890/0012-9658(2002)083[2394:QDOFWM]2.0.CO;2
Bonneaud, C., Sepil, I., Milá, B., Buermann, W., Pollinger, J., Sehgal, R. N., et al. (2009). The prevalence of avian plasmodium is higher in undisturbed tropical forests of Cameroon. J. Trop. Ecol. 25, 439–447. doi: 10.1017/S0266467409006178
Bueno, M. G., Lopez, R. P. G., Menezes, R. M. T.De, and Costa-Nascimento, M. D. J., Lima, G. F. M. D. C., and Araújo, R. A. D. S.,, et al. (2010). Identification of plasmodium relictum causing mortality in penguins (Spheniscus magellanicus) from São Paulo zoo, Brazil. Vet. Parasitol. 173, 123–127. doi: 10.1016/j.vetpar.2010.06.026
Bunbury, N., Jones, C. G., Greenwood, A. G., and Bell, D. J. (2007). Trichomonas gallinae in Mauritian columbids: implications for an endangered endemic. J. Wildl. Dis. 43, 399–407. doi: 10.7589/0090-3558-43.3.399
Clark, N. J., and Clegg, S. M. (2017). Integrating phylogenetic and ecological distances reveals new insights into parasite host specificity. Mol. Ecol. 26, 3074–3086. doi: 10.1111/mec.14101
Clark, N. J., Clegg, S. M., and Lima, M. R. (2014). A review of global diversity in avian haemosporidians (plasmodium and Haemoproteus: Haemosporida): new insights from molecular data. Int. J. Parasitol. 44, 329–338. doi: 10.1016/j.ijpara.2014.01.004
Dadam, D., Robinson, R. A., Clements, A., Peach, W. J., Bennett, M., Rowcliffe, J. M., et al. (2019). Avian malaria-mediated population decline of a widespread iconic bird species. R. Soc. Open Sci. 6:182197. doi: 10.1098/rsos.182197
Davies, T. J., and Pedersen, A. B. (2008). Phylogeny and geography predict pathogen community similarity in wild primates and humans. Proc. Biol. Sci. 275, 1695–1701. doi: 10.1098/rspb.2008.0284
de Angeli Dutra, D., Fecchio, A., Braga, É. M., and Poulin, R. (2021a). Migratory birds have higher prevalence and richness of avian haemosporidian parasites than residents. Int. J. Parasitol. 51, 877–882. doi: 10.1016/j.ijpara.2021.03.001
de Angeli Dutra, D., Filion, A., Fecchio, A., Braga, É. M., and Poulin, R. (2021b). Migrant birds disperse haemosporidian parasites and affect their transmission in avian communities. Oikos 130, 979–988. doi: 10.1111/oik.08199
Dormann, C. F., Gruber, B., and Fruend, J. (2008). Introducing the bipartite package: analysing ecological networks. R. News 8, 8–11.
Ellis, V. A., Collins, M. D., Medeiros, M. C. I., Sari, E. H. R., Coffey, E. D., Dickerson, R. C., et al. (2015). Local host specialization, host-switching, and dispersal shape the regional distributions of avian haemosporidian parasites. Proc. Natl. Acad. Sci. U. S. A. 112, 11294–11299. doi: 10.1073/pnas.1515309112
Ellis, V. A., Huang, X., Westerdahl, H., Jönsson, J., Hasselquist, D., Neto, J. M., et al. (2020). Explaining prevalence, diversity and host specificity in a community of avian haemosporidian parasites. Oikos 129, 1314–1329. doi: 10.1111/oik.07280
Ellis, V. A., Medeiros, M. C. I., Collins, M. D., Sari, E. H. R., Coffey, E. D., Dickerson, R. C., et al. (2017). Prevalence of avian haemosporidian parasites is positively related to the abundance of host species at multiple sites within a region. Parasitol. Res. 116, 73–80. doi: 10.1007/s00436-016-5263-3
Fecchio, A., Bell, J. A., Bosholn, M., Vaughan, J. A., Tkach, V. V., Lutz, H. L., et al. (2020). An inverse latitudinal gradient in infection probability and phylogenetic diversity for leucocytozoon blood parasites in New World birds. J. Anim. Ecol. 89, 423–435. doi: 10.1111/1365-2656.13117
Fecchio, A., Pinheiro, R., Felix, G., Faria, I. P., Pinho, J. B., Lacorte, G. A., et al. (2018). Host community similarity and geography shape the diversity and distribution of haemosporidian parasites in Amazonian birds. Ecography 41, 505–515. doi: 10.1111/ecog.03058
Ferreira Junior, F. C., Rodrigues, R. A., Ellis, V. A., Leite, L. O., Borges, M. A. Z., and Braga, É. M. (2017). Habitat modification and seasonality influence avian haemosporidian parasite distributions in southeastern Brazil. PLoS One 12:e0178791. doi: 10.1371/journal.pone.0178791
Forrester, D. J., and Foster, G. W. (2008). “Trichomonosis” in Parasitic Diseases of Wild Birds. eds. C. T. Atkinson, N. J. Thomas, and D. B. Hunter (Oxford, UK: Wiley-Blackwell), 120–153.
Fuller, T., Bensch, S., Müller, I., Novembre, J., Pérez-Tris, J., Ricklefs, R. E., et al. (2012). The ecology of emerging infectious diseases in migratory birds: an assessment of the role of climate change and priorities for future research. EcoHealth 9, 80–88. doi: 10.1007/s10393-012-0750-1
Garamszegi, L. Z. (2011). Climate change increases the risk of malaria in birds. Glob. Chang. Biol. 17, 1751–1759. doi: 10.1111/j.1365-2486.2010.02346.x
Garvin, M. C., Basbaum, J. P., Ducore, R. M., and Bell, K. E. (2003). Patterns of Haemoproteus beckeri parasitism in the gray catbird (Dumatella carolinensis) during the breeding season. J. Wildl. Dis. 39, 582–587. doi: 10.7589/0090-3558-39.3.582
Gedeon, K., Grüneberg, C., Mitschke, A., and Sudfeldt, C. (2022). Atlas Deutscher Brutvogelarten: Atlas of German Breeding Birds. Berlin: Favoritenpresse.
Gómez, A., and Nichols, E. (2013). Neglected wild life: parasitic biodiversity as a conservation target. Int. J. Parasitol. Parasites Wildl. 2, 222–227. doi: 10.1016/j.ijppaw.2013.07.002
Gómez, A., Nichols, E. S., and Perkins, S. L. (2012). “Parasite conservation, conservation medicine, and ecosystem health” in New Directions in Conservation Medicine: Applied Cases of Ecological Health. eds. A. A. Aguirre, R. Ostfeld, and P. Daszak (Oxford: Oxford University Press).
Gonzalez-Quevedo, C., Davies, R. G., and Richardson, D. S. (2014). Predictors of malaria infection in a wild bird population: landscape-level analyses reveal climatic and anthropogenic factors. J. Anim. Ecol. 83, 1091–1102. doi: 10.1111/1365-2656.12214
Harvey, J. A., and Voelker, G. (2019). Host associations and climate influence avian haemosporidian distributions in Benin. Int. J. Parasitol. 49, 27–36. doi: 10.1016/j.ijpara.2018.07.004
Hellgren, O., Bensch, S., and Malmqvist, B. (2008). Bird hosts, blood parasites and their vectors—associations uncovered by molecular analyses of blackfly blood meals. Mol. Ecol. 17, 1605–1613. doi: 10.1111/j.1365-294X.2007.03680.x
Hellgren, O., Pérez-Tris, J., and Bensch, S. (2009). A jack-of-all-trades and still a master of some: prevalence and host range in avian malaria and related blood parasites. Ecology 90, 2840–2849. doi: 10.1890/08-1059.1
Hellgren, O., Waldenström, J., and Bensch, S. (2004). A new PCR assay for simultaneous studies of leucocytozoon, plasmodium, and haemoproteus from avian blood. J. Parasitol. 90, 797–802. doi: 10.1645/GE-184R1
Hing, S., Narayan, E. J., Thompson, R. C. A., and Godfrey, S. S. (2016). The relationship between physiological stress and wildlife disease: consequences for health and conservation. Wildl. Res. 43:51. doi: 10.1071/WR15183
Huang, X., Chen, Z., Yang, G., Xia, C., Luo, Q., Gao, X., et al. (2022). Assemblages of plasmodium and related parasites in birds with different migration statuses. Int. J. Mol. Sci. 23:10277. doi: 10.3390/ijms231810277
Huang, X., Jönsson, J., and Bensch, S. (2020). Persistence of avian haemosporidians in the wild: a case study to illustrate seasonal infection patterns in relation to host life stages. Int. J. Parasitol. 50, 611–619. doi: 10.1016/j.ijpara.2020.05.006
Ilgūnas, M., Palinauskas, V., Platonova, E., Iezhova, T., and Valkiūnas, G. (2019). The experimental study on susceptibility of common European songbirds to plasmodium elongatum (lineage pGRW6), a widespread avian malaria parasite. Malar. J. 18:290. doi: 10.1186/s12936-019-2926-4
Ishtiaq, F. (2021). Ecology and evolution of avian malaria: implications of land use changes and climate change on disease dynamics. J. Indian Inst. Sci. 101, 213–225. doi: 10.1007/s41745-021-00235-3
Kilpatrick, A. M., Daszak, P., Jones, M. J., Marra, P. P., and Kramer, L. D. (2006). Host heterogeneity dominates West Nile virus transmission. Proc. Biol. Sci. 273, 2327–2333. doi: 10.1098/rspb.2006.3575
Knowles, S. C. L., Palinauskas, V., and Sheldon, B. C. (2010). Chronic malaria infections increase family inequalities and reduce parental fitness: experimental evidence from a wild bird population. J. Evol. Biol. 23, 557–569. doi: 10.1111/j.1420-9101.2009.01920.x
LaPointe, D. A., Atkinson, C. T., and Samuel, M. D. (2012). Ecology and conservation biology of avian malaria. Ann. N. Y. Acad. Sci. 1249, 211–226. doi: 10.1111/j.1749-6632.2011.06431.x
Laurance, S. G. W., Jones, D., Westcott, D., McKeown, A., Harrington, G., and Hilbert, D. W. (2013). Habitat fragmentation and ecological traits influence the prevalence of avian blood parasites in a tropical rainforest landscape. PLoS One 8:e76227. doi: 10.1371/journal.pone.0076227
Lawson, B., Cunningham, A. A., Chantrey, J., Hughes, L. A., John, S. K., Bunbury, N., et al. (2011). A clonal strain of trichomonas gallinae is the aetiologic agent of an emerging avian epidemic disease. Infect. Genet. Evol. 11, 1638–1645. doi: 10.1016/j.meegid.2011.06.007
Lawson, B., Robinson, R. A., Colvile, K. M., Peck, K. M., Chantrey, J., Pennycott, T. W., et al. (2012). The emergence and spread of finch trichomonosis in the British Isles. Phil. Trans. R. Soc. London B 367, 2852–2863. doi: 10.1098/rstb.2012.0130
Loiseau, C., Harrigan, R. J., Bichet, C., Julliard, R., Garnier, S., Lendvai, Á. Z., et al. (2013). Predictions of avian plasmodium expansion under climate change. Sci. Rep. 3:1126. doi: 10.1038/srep01126
Lopes, V. L., Costa, F. V., Rodrigues, R. A., Braga, É. M., Pichorim, M., and Moreira, P. A. (2020). High fidelity defines the temporal consistency of host–parasite interactions in a tropical coastal ecosystem. Sci. Rep. 10:16839. doi: 10.1038/s41598-020-73563-6
Masello, J. F., Martínez, J., Calderón, L., Wink, M., Quillfeldt, P., Sanz, V., et al. (2018). Can the intake of antiparasitic secondary metabolites explain the low prevalence of hemoparasites among wild psittaciformes? Parasit. Vectors 11:357. doi: 10.1186/s13071-018-2940-3
Medeiros, M. C. I., Hamer, G. L., and Ricklefs, R. E. (2013). Host compatibility rather than vector-host-encounter rate determines the host range of avian plasmodium parasites. Proc. Biol. Sci. 280:20122947. doi: 10.1098/rspb.2012.2947
Mozaffer, F., Menon, G. I., and Ishtiaq, F. (2022). Exploring the thermal limits of malaria transmission in the western Himalaya. Ecol. Evol. 12:e9278. doi: 10.1002/ece3.9278
Names, G. R., Schultz, E. M., Krause, J. S., Hahn, T. P., Wingfield, J. C., Heal, M., et al. (2021). Stress in paradise: effects of elevated corticosterone on immunity and avian malaria resilience in a Hawaiian passerine. J. Exp. Biol. 224:jeb242951. doi: 10.1242/jeb.242951
O’Connor, E. A., Strandh, M., Hasselquist, D., Nilsson, J.-Å., and Westerdahl, H. (2016). The evolution of highly variable immunity genes across a passerine bird radiation. Mol. Ecol. 25, 977–989. doi: 10.1111/mec.13530
Okamura, B., Hartigan, A., and Naldoni, J. (2018). Extensive uncharted biodiversity: the parasite dimension. Integr. Comp. Biol. 58, 1132–1145. doi: 10.1093/icb/icy039
Ots, I., and Hõrak, P. (1998). Health impact of blood parasites in breeding great tits. Oecologia 116, 441–448. doi: 10.1007/s004420050608
Parker, I. M., Saunders, M., Bontrager, M., Weitz, A. P., Hendricks, R., Magarey, R., et al. (2015). Phylogenetic structure and host abundance drive disease pressure in communities. Nature 520, 542–544. doi: 10.1038/nature14372
Pellegrino, I., Ilahiane, L., Boano, G., Cucco, M., Pavia, M., Prestridge, H. L., et al. (2021). Avian haemosporidian diversity on Sardinia: a first general assessment for the insular mediterranean. Diversity 13:75. doi: 10.3390/d13020075
Peters, M., Kilwinski, J., Reckling, D., and Henning, K. (2009). Epidemic mortality in greenfinches at feeder stations caused by trichomonas Gallinae—a recent problem in northern Germany. Kleintierpraxis 54, 433–438.
Poisot, T., Canard, E., Mouquet, N., and Hochberg, M. E. (2012). A comparative study of ecological specialization estimators. Methods Ecol. Evol. 3, 537–544. doi: 10.1111/j.2041-210X.2011.00174.x
Poulin, R. (1998). Large-scale patterns of host use by parasites of freshwater fishes. Ecol. Lett. 1, 118–128. doi: 10.1046/J.1461-0248.1998.00022.X
Poulin, R. (2014). Parasite biodiversity revisited: frontiers and constraints. Int. J. Parasitol. 44, 581–589. doi: 10.1016/j.ijpara.2014.02.003
Quillfeldt, P., Arriero, E., Martínez, J., Masello, J. F., and Merino, S. (2011). Prevalence of blood parasites in seabirds—a review. Front. Zool. 8:26. doi: 10.1186/1742-9994-8-26
Quillfeldt, P., Schumm, Y. R., Marek, C., Mader, V., Fischer, D., and Marx, M. (2018). Prevalence and genotyping of trichomonas infections in wild birds in Central Germany. PLoS One 13:e0200798. doi: 10.1371/journal.pone.0200798
R Core Team (2022). R: A Language and Environment for Statistical Computing: Reference Index. Vienna, Austria: R Foundation for Statistical Computing.
Ricklefs, R. E., Medeiros, M., Ellis, V. A., Svensson-Coelho, M., Blake, J. G., Loiselle, B. A., et al. (2017). Avian migration and the distribution of malaria parasites in New World passerine birds. J. Biogeogr. 44, 1113–1123. doi: 10.1111/jbi.12928
Romano, A., Nodari, R., Bandi, C., Caprioli, M., Costanzo, A., Ambrosini, R., et al. (2019). Haemosporidian parasites depress breeding success and plumage coloration in female barn swallows Hirundo rustica. J. Avian Biol. 50:325. doi: 10.1111/jav.01889
Santiago-Alarcon, D., Havelka, P., Pineda, E., Segelbacher, G., and Schaefer, H. M. (2013). Urban forests as hubs for novel zoonosis: blood meal analysis, seasonal variation in culicoides (diptera: ceratopogonidae) vectors, and avian haemosporidians. Parasitology 140, 1799–1810. doi: 10.1017/S0031182013001285
Schulze, E. D., Craven, D., Durso, A. M., Reif, J., Guderle, M., Kroiher, F., et al. (2019). Positive association between forest management, environmental change, and forest bird abundance. For. Ecosyst. 6:3. doi: 10.1186/s40663-019-0160-8
Schumm, Y. R., Wecker, C., Marek, C., Wassmuth, M., Bentele, A., Willems, H., et al. (2019). Blood parasites in Passeriformes in Central Germany: prevalence and lineage diversity of Haemosporida (Haemoproteus, plasmodium and Leucocytozoon) in six common songbirds. PeerJ 6:e6259. doi: 10.7717/peerj.6259
Simpson, V., and Molenaar, F. (2006). Increase in trichomonosis in finches. Vet. Rec. 159:606. doi: 10.1136/vr.159.18.606-a
Šujanová, A., Špitalská, E., and Václav, R. (2021). Seasonal dynamics and diversity of haemosporidians in a natural woodland bird Community in Slovakia. Diversity 13:439. doi: 10.3390/d13090439
Valkiŭnas, G., Zickus, T., Shapoval, A. P., and Lezhova, T. A. (2006). Effect of Haemoproteus belopolskyi (Haemosporida: Haemoproteidae) on body mass of the blackcap Sylvia atricapilla. J. Parasitol. 92, 1123–1125. doi: 10.1645/GE-3564-RN.1
Vanstreels, R. E. T., Miranda, F. R., Ruoppolo, V., Reis, A. O. D. A., Costa, E. S., Pessôa, A. R. D. L., et al. (2014). Investigation of blood parasites of pygoscelid penguins at the King George and Elephant Islands, south Shetlands archipelago, Antarctica. Polar Biol. 37, 135–139. doi: 10.1007/s00300-013-1401-x
Wood, M. J., Cosgrove, C. L., Wilkin, T. A., Knowles, S. C. L., Day, K. P., and Sheldon, B. C. (2007). Within-population variation in prevalence and lineage distribution of avian malaria in blue tits, Cyanistes caeruleus. Mol. Ecol. 16, 3263–3273. doi: 10.1111/j.1365-294X.2007.03362.x
Keywords: blood parasites, endoparasites, parasite infection, host–parasite interactions, trichomonas, avian malaria
Citation: Strehmann F, Becker M, Lindner K, Masello JF, Quillfeldt P, Schumm YR, Farwig N, Schabo DG and Rösner S (2023) Half of a forest bird community infected with haemosporidian parasites. Front. Ecol. Evol. 11:1107736. doi: 10.3389/fevo.2023.1107736
Edited by:
Jenny C. Dunn, University of Lincoln, United KingdomReviewed by:
Pavel Munclinger, Charles University, CzechiaWilliam Jones, University of Debrecen, Hungary
Copyright © 2023 Strehmann, Becker, Lindner, Masello, Quillfeldt, Schumm, Farwig, Schabo and Rösner. This is an open-access article distributed under the terms of the Creative Commons Attribution License (CC BY). The use, distribution or reproduction in other forums is permitted, provided the original author(s) and the copyright owner(s) are credited and that the original publication in this journal is cited, in accordance with accepted academic practice. No use, distribution or reproduction is permitted which does not comply with these terms.
*Correspondence: Finja Strehmann, finja.strehmann@biologie.uni-marburg.de