- 1College of Forestry, Hebei Agricultural University, Baoding, China
- 2College of Economics and Management, Hebei Agricultural University, Baoding, China
- 3Mulanweichang National Forestry Administration of Hebei Province, Chengde, China
Atmospheric nitrogen (N) deposition is among the main manifestations of global change and has profoundly affected forest biogeochemical cycles. However, the threshold of N deposition to soil nutrient contents and enzyme activities has rarely been studied in a forest. In this study, we explored the effects of N deposition on soil nutrients and enzyme activities in a Larix principis-rupprechtii plantation on the northern Yanshan Mountain through multigradient N addition experiments (0, 5, 10, 20, 40, 80, and 160 kg N ha−1 year−1) after fertilization for 2 years. Compared with the controls, N addition first led to a decrease in soil NH-N and NO-N, which then increased significantly. N addition had no significant effects on other soil nutrients. N addition overall elevated soil β-glucosidase activity. N application of >40 kg N ha−1 year−1 significantly reduced soil leucine aminopeptidase activity but had no significant effects on soil acid phosphatase, N-acetyl-β-D-glucosidase, and urease activities. N addition increased the overall stoichiometry ratio of EEA C:N and EEA C:P, but EEA N:P started decreasing after N application of 40 kg N ha−1 year−1. The ratios of C, N, and P acquisition activities changed from 1:1.2:1 under the control conditions to 1:1.1:1 under the N application of 160 kg N ha−1 year−1. N addition increased the overall vector length and had no significant effects on the vector angle. Correlation and redundancy analyses revealed that N addition-induced change in available soil N was the main factor affecting soil enzyme activity and stoichiometry. In general, different enzyme activities had different sensitivities to N addition. Moderate N addition or atmospheric N deposition (e.g., <40 kg N ha−1 year−1) had beneficial effects on soil nutrient cycling and microorganisms in a Larix principis-rupprechtii plantation.
1. Introduction
With industrial development, increases in population, and excessive use of fossil fuels, atmospheric nitrogen (N) deposition is increasing year by year. Some studies have predicted that the global nitrogen deposition rate will increase by 2.5 times in the twenty-first century (Davidson, 2009). By the end of the twentieth century, China had become among the three major N deposition regions globally. Although recent studies have shown that N deposition in China has exhibited a stabilizing or even decreasing trend (Yu et al., 2019; Wen et al., 2020), it remains at a high level. Increased N deposition affects the growth and diversity of ecosystem plants and alters the species and structure of soil microorganisms, thereby affecting soil enzyme activity and nutrient cycling (Isbell et al., 2013). Carbon, nitrogen, and phosphorus acquisition enzymes are widely present in soil and have a crucial role in organic matter decomposition and nutrient cycling in soil (Burns et al., 2013), which reflect the microbial requirement for C, N, P, and other nutrient elements and changes in the soil environment (Gutknecht et al., 2010; Guo et al., 2022; Wang et al., 2022). Therefore, they are often used to indicate the response of soil microorganisms to global changes (Kelley et al., 2011) and have a profound terrestrial ecosystem biogeochemical cycle (Sinsabaugh et al., 2009; López-Aizpún et al., 2017). Investigating the effect of N addition on soil nutrients and enzyme activity is crucial for a deeper understanding of soil nutrient cycling in the background of increasing atmospheric N deposition.
The results of studies on the effect of N addition on soil nutrients are generally consistent. Most of them have reported that N addition increases the content of forest soil organic carbon, soluble organic carbon, total nitrogen (TN), and available nitrogen (AN) (Liu and Greaver, 2010), whereas excessive nitrogen input leads to phosphorus limitation (Elser et al., 2009; Deng et al., 2017) and loss of calcium, magnesium, and other nutrients (Huang et al., 2015; Lu et al., 2018). However, the results of studies investigating the effect of N addition on the activity of soil carbon, nitrogen, and phosphorus acquisition enzymes differed considerably. For example, with N addition, the activity of carbon acquisition-related β-1,4-glucosidase (BG) showed an increasing trend in one study (Sinsabaugh et al., 2005), no significant change in another study (Zeglin et al., 2007), and a decreasing trend in other studies (Ramirez et al., 2012; Zhang et al., 2017). Moreover, different enzyme species responded differently to the same N addition treatment. For example, Fan et al. (2018) found that N addition (40 and 80 kg N ha−1 year−1) increased acid phosphatase (ACP) activity but decreased N-acetyl-β-D-glucosidase (NAG) activity in a subtropical Castanopsiscarlesii forest soil. The differential effects of N addition on the activities of different soil enzymes may be closely related to N application rates. However, most current experimental gradients of forest N addition are few (generally 3–4) (Sinsabaugh et al., 2005; Zhang et al., 2017), and the starting fertilizer application is high (generally >40 kg N ha−1 year−1) (Fan et al., 2018), which limits us to determine the sensitivity of soil enzyme activity to nitrogen addition. Finding the threshold at which nitrogen addition starts to influence a certain enzyme activity is difficult.
Larix principis-rupprechtii is among the major silvicultural species on the northern Yanshan Mountain and is widely distributed in warm temperate areas in China. Simultaneously, North China has gradually become one of the new high deposition areas in China (Yu et al., 2019), and studying the effect of N deposition on Larix principis-rupprechtii plantations is important. In this study, we conducted a multigradient N addition experiment in a typical Larix principis-rupprechtii plantation and measured soil nutrients, microbial biomass, and activities of soil carbon and phosphorus acquisition enzymes after 2 years of fertilizer application. We hypothesized that different soil nutrients and enzyme activities have different sensitivities and threshold values to N addition. Available nutrients, such as NH or NO, and nitrogen acquisition enzymes have high sensitivity and low threshold values to N addition. This study clarified the response and threshold values of soil nutrients and enzyme activities to N addition and thus provided a scientific basis for long-term management of Larix principis-rupprechtii plantations under the N deposition background.
2. Methods
2.1. Study sites
The study area is located in Mulanweichang National Forestry Administration of Hebei Province (41°35′N−42°40′N, 116°32′E−118°14′E). This area is in the transition zone between the Inner Mongolia Plateau and the northern mountains of Hebei Province and has a flat terrain and altitude of 1,200–2,000 m. The area has a typical temperate continental plateau monsoon climate. This climate is characterized by cold and dry winters and cool and heat-free summers, with an average annual temperature of ~3.3°C. The average annual precipitation is 445 mm, mostly concentrated in July and August.
In this study, one side of a hill with consistent stand conditions was selected as the experimental sample site for N addition. The stand type was a Larix principis-rupprechtii plantation on the northern slope of the hill, a shady slope of ~15°. This 30-year-old stand has an average tree diameter at a breast height of 13.2 cm, an average tree height of 10.5 m, and a density of ~1,575 trees hm−2. The understory shrubs mainly include Rosa dahurica, Lespedeza bicolor, Armeniacasibirica, and Spiraea pubescens Turcz. The main herbs are Carex siderosticta, Campanula punctata, Moehringia trinervia, Artemisia mongolicau, etc.
2.2. Experimental design
In July 2018, 28 sample plots (area of each plot: 20 m × 20 m) were set up in a row along the slope's contour. The plots were separated by a 10-m separation zone to avoid the interaction between different N application treatments. All plots were fenced to exclude human and animal interference. Before fertilization, 0–10 cm of surface soil was collected from each site by using a five-point sampling method, and soil indices such as pH, soil organic carbon (SOC), TN, total phosphorus (TP), available phosphorus (AP), ammonium nitrogen (NH-N), nitrate nitrogen (NO-N), and available nitrogen (AN) were measured (Table 1). No significant differences were observed in the soil background of each site and were moderate for the nutrient addition control experiments.
The N addition gradient fertilization experiments were conducted in August 2019. N addition was divided into seven levels with four replications for each treatment in a randomized block design. Using the rain gauge method, we found that the annual atmospheric wet N deposition flux in this area is 5.03 kg N ha−1 year−1, and 88% of it is deposited in the growing season from May to September. Thus, N concentrations for addition to the seven levels were 0 (N0), 5 (N5), 10 (N10), 20 (N20), 40 (N40), 80 (N80), and 160 (N160) kg N ha−1 yr−1 from low to high, and the fertilizer type was urea (CH4N2O). Urea was dissolved in 40 L of water in each sample plot and sprayed in each plot evenly. Equal amounts of water were sprayed in the control sample plot. The soil was fertilized every month in the growing season.
2.3. Field sampling
Soil surface (0–10 cm) sampling was conducted in September 2020, 2 years after fertilization was completed in the sample plots. Soil from five points on each plot was sampled and mixed into one sample. The soil were passed through a 2-mm soil sieve to remove debris such as animal residues and stones. A part of the sieved soil sample was stored in a refrigerator at 4°C to determine soil enzyme activity and microbial carbon. Another part of the soil was stored at room temperature and dried naturally to determine basic soil nutrient indices.
2.4. Measurement methods
2.4.1. Soil chemical properties
Soil organic carbon was determined using the potassium dichromate-external heating method; recalcitrant organic carbon (ROC) was determined through acid hydrolysis; soil easily oxidized organic carbon (EOC) was determined using the potassium permanganate colorimetric method; and microbial quantity carbon (MBC) was determined using the chloroform fumigation-potassium sulfate leaching method. Soil TN was determined through potassium dichromate-sulfuric acid digestion; ammonium nitrogen (NH-N) and nitrate nitrogen (NO-N) were extracted with 2 mol L−1 KCI and determined using a continuous flow analyzer (Skalar san++, Skalar, Netherlands); AN was a sum of NH-N and NO-N. Soil TP and AP were detected using the continuous flow analyzer (Hedley et al., 1982). Soil pH was determined using an acidity meter with the soil–water ratio (1:2.5). These methods can be seen in Bao (2000).
2.4.2. Determination of enzyme activity and calculation of enzyme stoichiometric ratio
Soil urease (UE), soil leucine aminopeptidase (LAP), soil ACP, soil NAG, and soil β-glucosidase (BG) were determined using the microplate fluorometric method (Chen et al., 2018). According to the requirements of the test kit, soil samples were placed in 96-well cell culture plates with the corresponding reagents and detected using a microplate reader (Thermo Scientific™, USA). The substrates of BG, NAG, UE, LAP, and ACP are 4-MUB-β-D-glucopyranoside, 4-MUB-N-acetyl-β-D-glucosaminide, urea, L-leucine-7-amino-4-methylcoumarin, and 4-MUB-phosphate, respectively.
The equations for determining the stoichiometry of soil extracellular enzymes (Keeler et al., 2009) are as follows:
where EEA represents the soil extracellular enzyme activity, BG represents soil β-glucosidase, NAG represents soil N-acetyl-β-D-glucosidase, LAP represents leucine aminopeptidase, and ACP represents acid phosphatase.
2.4.3. Vector characteristics of enzyme activity
To quantify the limitation of microbial metabolism of C, N, and P, vector analysis was performed on the relative proportion of enzyme activity. Vector length represents the C limitation, and the vector angle represents relative P and N limitations. Microbial C limitation increases with the vector length. A vector angle of >45° represents microbial P limitation, and that of < 45° represents N limitation (Fanin et al., 2016; Moorhead et al., 2016).
2.5. Data analysis
Data statistics and analysis were performed using Microsoft Excel 2019 and SPSS 22.0. One-way ANOVA and Duncan multiple comparisons of enzyme activities for N addition treatments were performed at a significance level of p < 0.05 and plotted using SigmaPlot 14.0 software. The Pearson correlation analysis analyzed the correlation between soil enzyme activities and their stoichiometric ratios with soil physicochemical properties and other indicators. Redundancy analysis (RDA) was performed using Canoco 5.0 software to determine the inter-relationships between soil enzyme activities and their environmental conditions. In the forward selection step, the variable was excluded due to its collinearity with the currently selected variable. The constrained step based on a Monte Carlo permutation moved unimportant explanatory variables, and significant variables (p < 0.05) were used in the final analyses.
3. Results and analysis
3.1. Effects of N addition on soil chemical properties
Table 2 and Figure 1 present the effects of N addition on total soil nutrients, effective nutrients, and pH. SOC, TN, and TP with their ratios, pH, AP, and MBC exhibited no significant difference among the different levels of N added (p > 0.05). Soil EOC content tended to increase with increasing N addition, but it did not reach a significant level (p > 0.05). Soil NH-N and NO-N displayed a decreasing trend with low N applications and tended to increase after the N20 or N40 treatment (Figure 1), and soil AN also exhibited the same trend as soil NO-N.
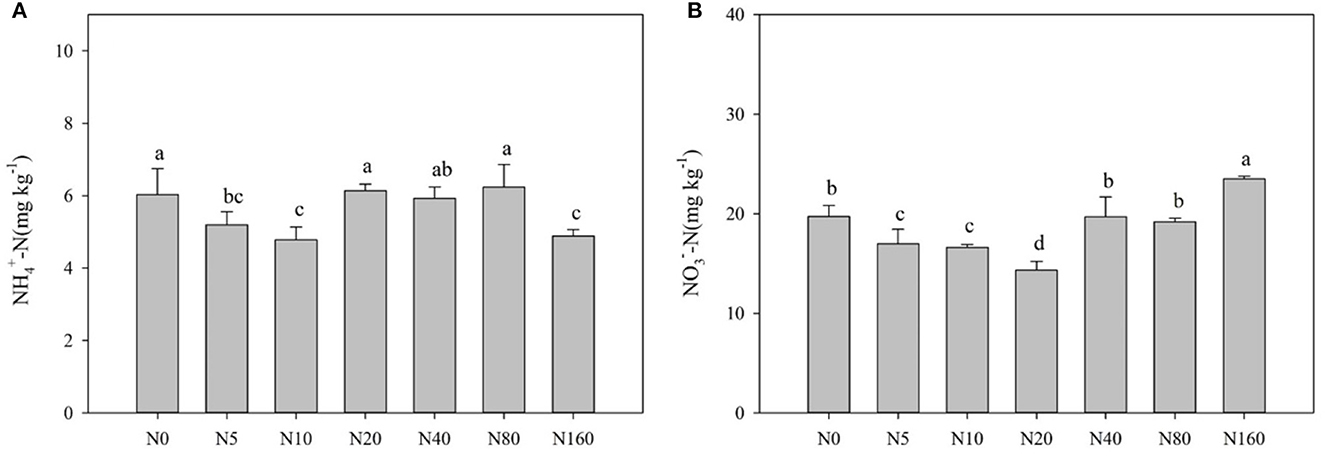
Figure 1. Effects of nitrogen addition on soil ammonium nitrogen (A) and nitrate nitrogen (B) in a Larix principis-rupprechtii plantation in North China.
3.2. Effects of N addition on soil enzyme activity and enzyme stoichiometry
Figure 2 presents the effects of N addition on the activity of each soil enzyme. Among them, N addition influenced BG activity, with an overall trend of increase and then decrease, with the highest activity observed after the N5 treatment (Figure 2A). NAG, LAP, and UE presented different responses to N addition. NAG displayed large variations in activity among N treatments and showed no obvious regularity with an increase in N addition. LAP activity first increased and then decreased with the increase in N addition, reaching its peak after the N40 treatment. No significant change was observed in UE activity after the N treatments. ACP activity also did not exhibit significant changes (Figure 2D).
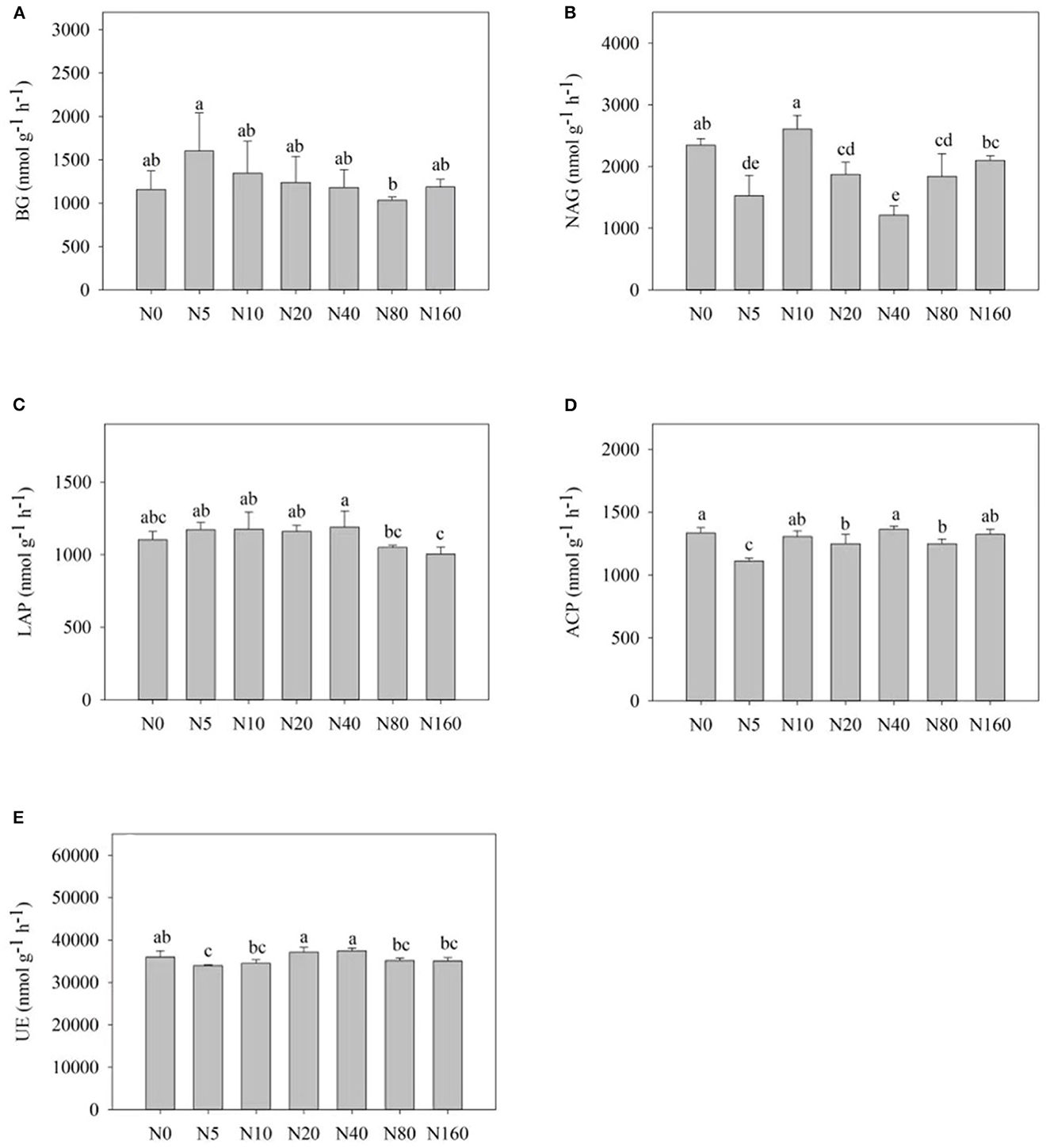
Figure 2. Effects of nitrogen addition on soil enzyme activity of a Larix principis-rupprechtii plantation in North China. (A–E) BG, NAG, LAP, ACP, and UE, respectively.
Figure 3 presents the influences of N addition on the stoichiometric ratio of soil enzymes. With an increase in N addition, EEA C:N and EEA C:P presented a trend of first increasing and then decreasing. At the same time, EEA N:P exhibited an overall trend of decreasing and reached its highest value after the N10 treatment (Figure 3C).
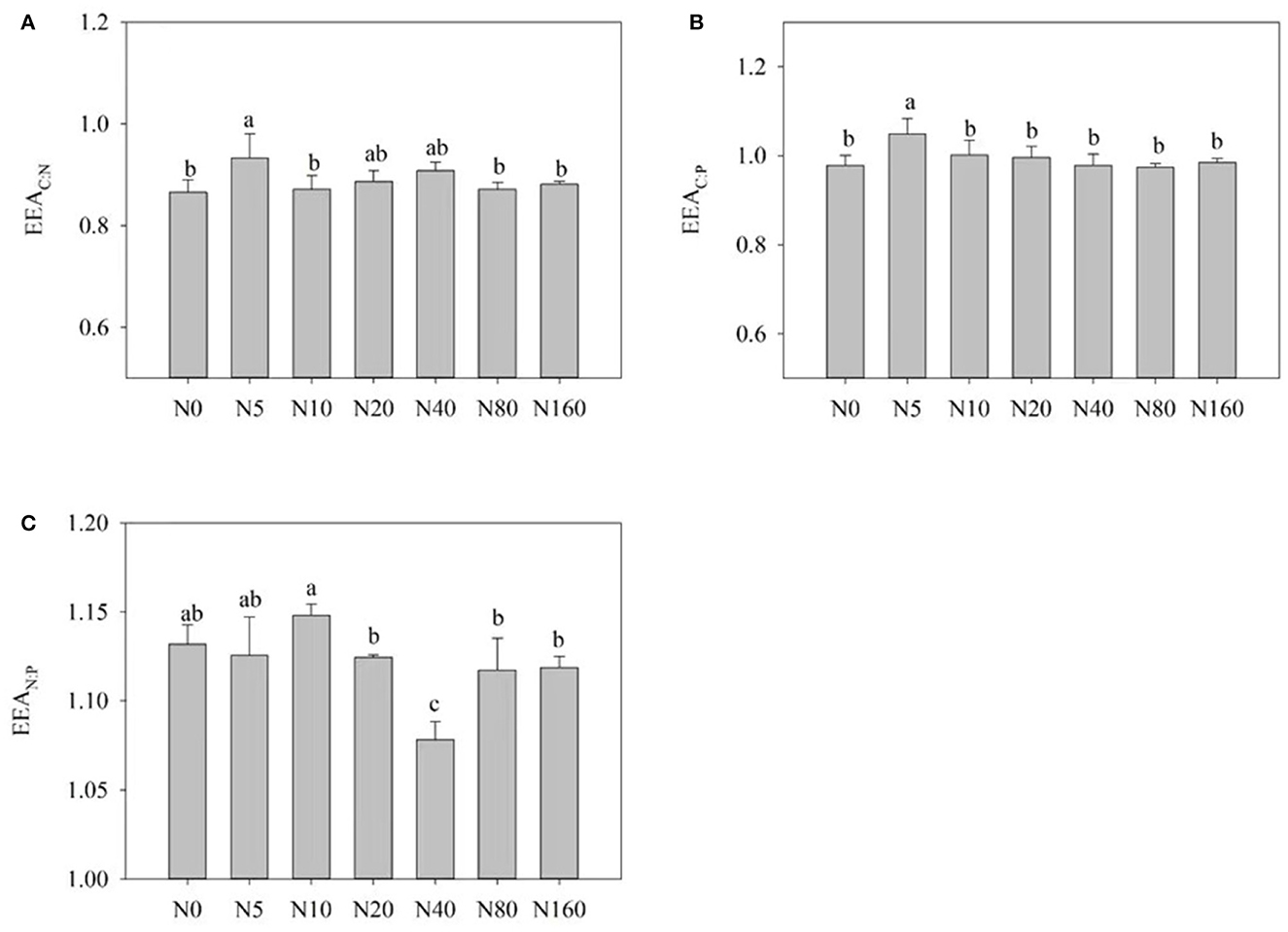
Figure 3. Effects of nitrogen addition on soil enzyme stoichiometry in a Larix principis-rupprechtii plantation in North China. (A–C) BG: (LAP+NAG), BG:ACP, and (LAP+NAG):ACP, respectively.
3.3. Effect of N addition on vector characteristics of soil enzyme activity
Overall, the vector length increased with N addition (p < 0.05), except after the N80 treatment (Figure 4A). The vector length was the longest after the N5 treatment, thereby indicating that microorganisms experience the largest carbon limitation at the N5 level. Compared with the controls, N addition had no significant effects on the vector angle (Figure 4B). Vector angles under different gradient N addition enzymes were all < 45°. This indicates that microbial communities experience nitrogen limitation to some extent, and short-term N addition did not significantly change this status.
3.4. Analysis of soil enzyme activities and stoichiometric ratios with soil environmental factors
3.4.1. Correlation analysis
The results of correlation analysis between soil enzyme activities, stoichiometric ratios, and soil environmental factors in a Larix principis-rupprechtii plantation after N addition are presented in Table 3. Soil BG activity significantly and negatively correlated with pH and EOC and significantly and positively correlated with MBC (p < 0.05). Soil LAP activity was significantly and negatively correlated with NO-N and AN. Soil ACP activity was extremely and positively correlated with TP (p < 0.01) and significantly and negatively correlated with MBC. Soil UE activity was significantly and positively correlated with NH-N. Soil NAG activity was not significantly correlated with any of the environmental factors (p > 0.05).
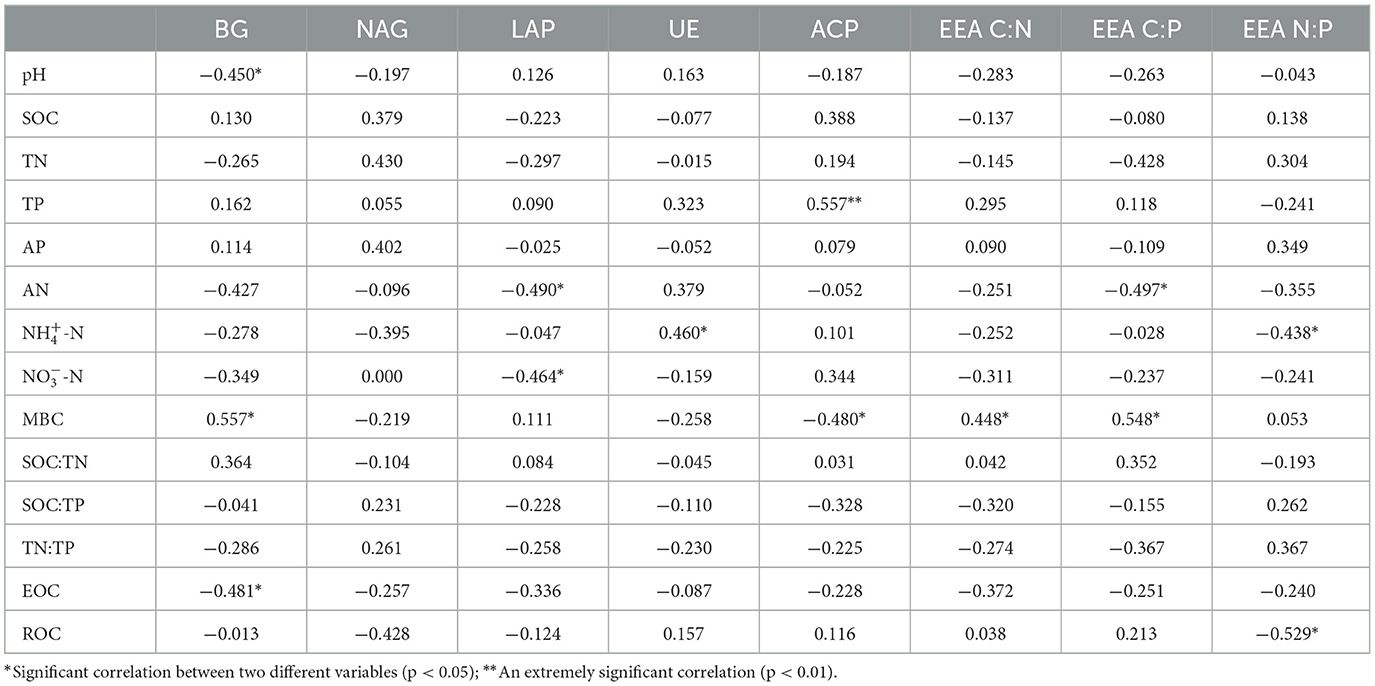
Table 3. Pearson correlation between soil enzymes, stoichiometric ratios, and soil physicochemical properties.
EEA C:N was significantly and positively correlated with soil MBC. EEA C:P was significantly and negatively correlated with AN and significantly and positively correlated with MBC. EEA N:P significantly and positively correlated with soil NH-N and ROC (p < 0.05).
3.4.2. Redundancy analysis
As shown in Figure 5, the total interpretation rate of environmental variables on soil enzyme activities was 65.66%. The total interpretation rates on the first and second axes were 57.65% and 8.01%, respectively. NH-N and ROC were the first two factors for explaining the variation in soil enzyme activities and enzyme stoichiometry, and their interpretation rates were 20.6% and 18.6%, respectively.
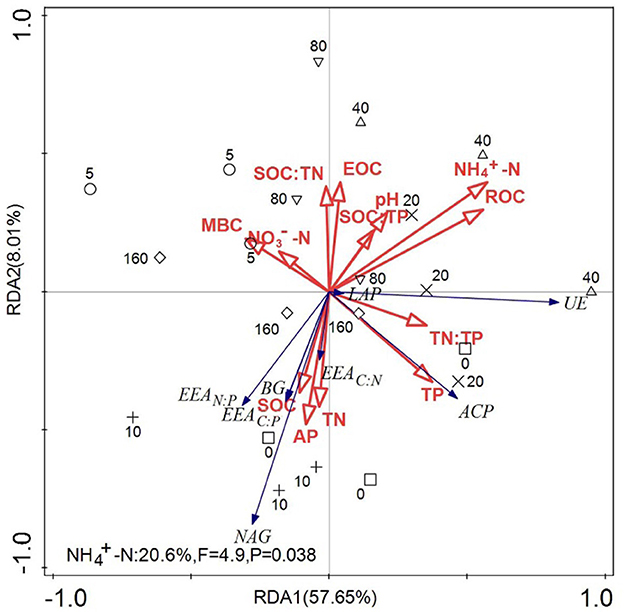
Figure 5. Redundant analysis diagram of the influence of soil nutrients on soil enzyme activity and stoichiometric ratios. Soil enzyme activity and the ecological enzyme stoichiometric ratio were used as response variables, and soil environmental factors were used as explanatory variables for redundancy analysis (RDA). The arrows connecting soil microbial enzyme activities and enzyme stoichiometric ratios are in blue, and the arrows connecting the soil environmental factors are in red.
4. Discussion
4.1. Effects of N addition on soil chemical properties
In this study, short-term fertilizer application exhibited no significant effect on soil SOC, TN, TP, TK, ROC, and pH but had significant effects on AN, NH-N, NO-N, and MBC (Figure 1; Table 2). Most studies have reported an increase in soil AN, NH-N, and NO-N contents with high N addition (Fang et al., 2009; Hu et al., 2009; Xu et al., 2009). In the present study, these factors also increased with high N addition, but they first decreased with low N application. This phenomenon has rarely been reported in forest ecosystems because the starting fertilizer application rate was usually high in previous studies (Fan et al., 2018). Boreal forests are typically nitrogen-deficient ecosystems (Du et al., 2020). A study also found that nitrogen addition increased plant N uptake and plant biomass in North China (Du et al., 2014). N addition stimulated Larix principis-rupprechtii growth, but this effect was greater at a low N addition level (< 40 kg N ha−1 year−1) and lower with high N addition (unpublished data). Therefore, high N uptake by trees with low N addition is possibly the main reason for the decreased available N. Simultaneously, N addition promotes the mineralization of soil organic N, and decomposition of soil organic N after exogenous N addition directly increases the soil NH-N content (Aber, 2002) and the nitrification reaction (Bejarano et al., 2014). Therefore, when the content of available soil N exceeds the range that plants could absorb, soil NH-N and NO-N accumulate and increase with high N addition.
Soil microorganisms are the most active part of soil organic matter, and MBC is a sensitive index that reflects small changes in the soil environment. Most studies have indicated that N addition causes a reduction in soil MBC content (Treseder, 2008; Janssens et al., 2010; Liu and Greaver, 2010; Zhang et al., 2017), whereas N addition did not reduce soil MBC content in this study (Table 2). In a meta-analysis, Treseder (2008) concluded that two main reasons lead to a decrease in MBC content with N addition. The first is “aluminum toxicity” in soil due to the decrease in soil pH with N addition, and the second is the microbial “carbon starvation” caused by the decrease in lignose activity, increase in melanin content, or decrease in plant carbon allocation to the subsurface with N addition. Soil MBC did not decrease in the present study because, on the one hand, short-term fertilizer application had not led to soil acidification, and on the other hand, the herbaceous plant community under the larch forest in North China was growing vigorously, and N addition significantly increased its biomass (unpublished data). The rapid turnover of herbaceous plants might have increased the soil EOC (Table 2), thereby alleviating the microbial “carbon starvation” possibly after N addition. Therefore, the role of understory plants in connection to the effect of N addition on soil nutrients needs to be further explored.
4.2. Effect of N addition on soil enzyme activities and stoichiometric ratios
Atmospheric N deposition can affect the balance of energy flow and nutrient cycle within forest ecosystems (Liu et al., 2017). Soil enzymes are more sensitive to environmental changes, so they act as an indicator of the soil response to N deposition (Sinsabaugh et al., 2005). We here focused on the activities of five soil carbon, nitrogen, and phosphorus acquisition enzymes. A total of 2 years after N addition, BG and LAP activities exhibited regular changes, whereas NAG, UE, and ACP activities exhibited no significant regular changes. LAP activity was significantly lower after high N treatment (>80 kg N ha−1 year−1) than in the control and low N groups. High N addition reduced N acquisition enzyme activity, which is consistent with the results of most studies (Dong et al., 2019). According to the resource allocation model (Allison and Vitousek, 2005), N addition can alter soil enzyme activity by affecting the effectiveness of soil nutrients (Wallenius et al., 2011), and enzyme activity associated with N acquisition decreases because of an increase in the effective soil N content under N addition conditions (Berg and Matzner, 2011). The correlation analysis results revealed that the increase in soil AN and NO-N after N addition was the primary cause of the decreased LAP activity (Table 3).
The resource allocation model also suggested that the activities of carbon acquisition enzymes increase after N addition. The increase in BG activity after N addition, in this study, is consistent with the results obtained from the USA (Sinsabaugh et al., 2005). N application generally stimulates microbial activity, thereby increasing the demand for carbon and the activity of carbon-associated soil enzymes (Keeler et al., 2009). In the present study, pH was negatively correlated with BG activity, which agrees with the results of a meta-analysis (Sinsabaugh et al., 2008). The decreased pH and the high H+ concentration could inhibit the biomass of soil microorganisms, which in turn had an inhibitory effect on enzyme activity. In addition, the change in pH induces a change in the binding state of soil particles, thus leading to a consequent change in soil enzyme activity. In this study, EOC was negatively correlated with BG activity. This may be because when the content of EOC, as a more active part of organic carbon, increases, the organic carbon-decomposing extracellular enzymes would have negative feedback. Soil microorganisms reduce the energy required to synthesize enzymes to a certain extent. This would allow the effective promotion of microbial growth by nutrients, thereby limiting the release of nutrients and inhibiting carbon hydrolase activity in the soil (Enrique et al., 2008).
At the global scale, the ratio of specific C, N, and P acquisition activities is 1:1:1 (Sinsabaugh et al., 2008). In this study, this ratio changed from 1:1.2:1 under the control condition to 1:1.1:1 after treatment with 160 kg N ha−1 year−1, thereby indicating that N limitation occurred in this area. The vector angle also exhibited this phenomenon (Figure 4B). Short-term N addition alleviated this N limitation to some extent but led to no change in this situation. In the present study, the overall increase in BG activity, enzyme EEA C:N, and enzyme vector length with N addition also indicated that N addition exacerbated carbon limitation, which accorded to the “optimal allocation” principle of ecological economics (Sinsabaugh and Moorhead, 1994). Therefore, soil microorganisms in this area were simultaneously under C and N limitation, and N addition aggravated the C limitation. These results were obtained due to short-term N addition, and we believe that it can be alleviated when plant litter increases after long-term N addition.
4.3. Implication for forest ecosystem management
Notably, in this study, the sensitivities of enzyme activities related to carbon, nitrogen, and phosphorus differed in response to N addition in Larix principis-rupprechtii plantation soils. The activities of carbon acquisition enzymes (e.g., BG) were altered with low N addition, and those of nitrogen acquisition enzymes (e.g., LAP) responded significantly with medium N addition (~40 kg N ha−1 yr−1). By contrast, the activities of phosphorus acquisition enzymes (e.g., ACP) did not change significantly. The different sensitivities of these soil enzymes to the N addition rate had important implications for ecosystem management. Unlike plants, which are typically limited from accessing nitrogen in terrestrial ecosystems (LeBauer and Treseder, 2008), heterotrophic microorganisms are more susceptible to carbon limitation. In this study, soil microorganisms were simultaneously under C and N limitations. Moreover, with high N addition (>40 kg N ha−1 year−1), the soil enzyme N:P ratio decreased, and the vector angle increased slightly, thereby indicating a risk of phosphorus limitation. A healthy forest needs a nutritive element balance between C, N, and P. Therefore, in this study, moderate N addition or atmospheric N deposition had beneficial effects on soil microbial growth and nutrient cycling and promoted forest growth with positive ecological effects on the ecosystem.
5. Conclusion
In this study, the effects of 2 years of N addition on soil nutrients, enzyme activities, and stoichiometric ratios were investigated in a Larix principis-rupprechtii plantation on the northern Yanshan Mountain in China. Our results revealed that short-term N addition had no significant effects on total nutrients, AP, soil pH, or MBC in larch plantations. Soil AN, NH-N, and NO-N contents decreased with low N addition and significantly increased with high N addition. The sensitivities of different enzyme activities in response to N addition varied. BG activity increased with low N treatment, and LAP activity started to decrease significantly with medium N application (40 kg N ha−1 year−1) treatment. The other three enzymes exhibited no sensitivity to short-term N addition. Soil NH-N and NO-N contents were the main environmental factors influencing soil enzyme activity and altered enzyme stoichiometric ratios under N addition. In general, moderate N addition or atmospheric N deposition was beneficial for soil nutrient cycling and microorganisms in Larix principis-rupprechtii plantations.
Data availability statement
The original contributions presented in the study are included in the article/supplementary material, further inquiries can be directed to the corresponding author.
Author contributions
YJ and ZX contributed to the design of the study. XY, LY, XL, and GX performed the experiment. XY performed the statistical analysis. XY, QL, and YJ wrote the first draft of the manuscript. All authors contributed to the manuscript revision and reading and approved the submitted version.
Funding
This study was supported by the National Natural Science Foundation of China (31700377) and the Natural Science Foundation of Hebei (C2018204096).
Conflict of interest
The authors declare that the research was conducted in the absence of any commercial or financial relationships that could be construed as a potential conflict of interest.
Publisher's note
All claims expressed in this article are solely those of the authors and do not necessarily represent those of their affiliated organizations, or those of the publisher, the editors and the reviewers. Any product that may be evaluated in this article, or claim that may be made by its manufacturer, is not guaranteed or endorsed by the publisher.
References
Aber, J. D. (2002). Nitrogen saturation in temperate forest ecosystems: current theory, remaining questions and recent advances. Prog. Nutr. 98:179–188. doi: 10.1007/978-94-017-2789-1-13
Allison, S. D., and Vitousek, P. M. (2005). Responses of extracellular enzymes to simple and complex nutrient inputs. Soil Boil. Biochem. 37:937–944. doi: 10.1016/j.soilbio.2004.09.014
Bejarano, M., Etchevers, J. D., Ruíz-Suárez, G., and Julio, C. (2014). The effects of increased N input on soil C and N dynamics in seasonally dry tropical forests: an experimental approach. Appl. Soil Ecol. 73, 105–115. doi: 10.1016/j.apsoil.2013.08.015
Berg, B., and Matzner, E. (2011). Effect of N deposition on decomposition of plant litter and soil organic matter in forest systems. Environ. Rev. 5, 1–25. doi: 10.1139/er-5-1-1
Burns, R. G., Deforest, J. L., Marxsen, J., Sinsabaugh, R. L., Stromberger, M. E., Wallenstein, M. D., et al. (2013). Soil enzymes in a changing environment: current knowledge and future directions. Soil Biol. Biochem. 58, 216–234. doi: 10.1016/j.soilbio.2012.11.009
Chen, H., Li, D. J., Xiao, K. C., and Wang, K. L. (2018). Soil microbial processes and resource limitation in karst and non-karst forests. Funct. Ecol. 32, 1400–1409. doi: 10.1111/1365-2435.13069
Davidson, E. A. (2009). The contribution of manure and fertilizer nitrogen to atmospheric nitrous oxide since 1860. Nat. Geosci. 2, 659–662. doi: 10.1038/ngeo608
Deng, Q., Hui, D. F., Dennis, S., and Reddy, C. (2017). Responses of terrestrial ecosystem phosphorus cycling to nitrogen addition: a meta-analysis. Glob. Ecol. Biogeogr. 26, 713–728. doi: 10.1111/geb.12576
Dong, C. C., Wang, W., Liu, H. Y., Xu, X. T., and Zeng, H. (2019). Temperate grassland shifted from nitrogen to phosphorus limitation induced by degradation and nitrogen deposition: evidence from soil extracellular enzyme stoichiometry. Ecol. Indic. 101, 453–464. doi: 10.1016/j.ecolind.2019.01.046
Du, E. Z., César, T., Adam, F. A. P., Anders, A., Lissa, C. J., Zhang, X., et al. (2020). Global patterns of terrestrial nitrogen and phosphorus limitation. Nat. Geosci. 13, 221–226. doi: 10.1038/s41561-019-0530-4
Du, Z. H., Wang, W., Zeng, W. J., and Zeng, H. (2014). Nitrogen deposition enhances carbon sequestration by plantations in Northern China. PLoS ONE 9, e87975. doi: 10.1371/journal.pone.0087975
Elser, J. J., Andersen, T., Baron, J. S., Bergström, A. K., Jansson, M., Kyle, M., et al. (2009). Shifts in lake N:P stoichiometry and nutrient limitation driven by atmospheric nitrogen deposition. Science 326, 835–837. doi: 10.1126/science.1176199
Enrique, A., Bruno, C., Christopher, A., Virgile, C., and Stéven, C. (2008). Effects of nitrogen availability on microbial activities, densities and functional diversities involved in the degradation of a Mediterranean evergreen oak litter (Quercus ilex L.). Soil Boil. Biochem. 40, 1654–1661. doi: 10.1016/j.soilbio.2008.01.020
Fan, Y. X., Lin, F., Yang, L. M., Zhong, X. J., Wang, M. H., Zhou, J. C., et al. (2018). Decreased soil organic P fraction associated with ectomycorrhizal fungal activity to meet increased P demand under N application in a subtropical forest ecosystem. Biol. Fert. Soils 54, 149–161. doi: 10.1007/s00374-017-1251-8
Fang, Y. T., Gundersen, P., Mo, J. M., and Zhu, W. X. (2009). Nitrogen leaching in response to increased nitrogen inputs in subtropical monsoon forests in southern China. Forest Ecol. Manag. 257, 332–342. doi: 10.1016/j.foreco.2008.09.004
Fanin, N., Moorhead, D., and Bertrand, I. (2016). Eco-enzymatic stoichiometry and enzymatic vectors reveal differential C, N, P dynamics in decaying litter along a land-use gradient. Biogeochemistry 129, 21–36. doi: 10.1007/s10533-016-0217-5
Guo, D. L., Ou, Y. S., Zhou, X. H., Wang, X., Zhao, Y. F., Li, J., et al. (2022). Response of soil enzyme activities to natural vegetation restorations and plantation schemes in a landslide-prone region. Forests 13, 880. doi: 10.3390/f13060880
Gutknecht, J. L. M., Henry, H. A. L., and Balser, T. C. (2010). Inter-annual variation in soil extra-cellular enzyme activity in response to simulated global change and fire disturbance. Pedobiologia 53, 283–293. doi: 10.1016/j.pedobi.2010.02.001
Hedley, M. J., Stewart, J. W. B., and Chauhan, B. S. (1982). Changes in inorganic and organic soil phosphorus fractions induced by cultivation practices and by laboratory incubations. Soil Sci. Soc. Am. J. 46, 970–976. doi: 10.2136/sssaj1982.03615995004600050017x
Hu, Y. L., Han, S. J., Li, X. F., Zhao, Y. T., and Li, T. (2009). Responses of soil available nitrogen of natural forest and secondary forest to simulated N deposition in Changbai Mountain (in Chinese). J. Northeast For. Univ. 37. 36–38. doi: 10.1016/j.elecom.2008.10.019
Huang, Y. M., Kang, R. H., Mulder, J., Zhang, T., and Duan, L. (2015). Nitrogen saturation, soil acidification, and ecological effects in a subtropical pine forest on acid soil in southwest China. J. Geophys Res-Biogeo. 120, 2457–2472. doi: 10.1002/2015JG003048
Isbell, F., Reich, P. B., Tilman, D., Hobbie, S. E., Polasky, S., and Binder, S. (2013). Nutrient enrichment, biodiversity loss, and consequent declines in ecosystem productivity. Proc. Natl. Acad. Sci. USA 110, 11911–11916. doi: 10.1073/pnas.1310880110
Janssens, I. A., Dieleman, W., Luyssaert, S., Subke, J. A., Reichstein, M., Ceulemans, R., et al. (2010). Reduction of forest soil respiration in response to nitrogen deposition. Nat. Geosci. 3, 315–322. doi: 10.1038/ngeo844
Keeler, B. L., Hobbie, S. E., and Kellogg, L. E. (2009). Effects of long-term nitrogen addition on microbial enzyme activity in eight forested and grassland sites: implications for litter and soil organic matter decomposition. Ecosystems 12, 1–15. doi: 10.1007/s10021-008-9199-z
Kelley, A. M., Fay, P. A., Polley, H. W., Gill, R. A., and Jackson, R. B. (2011). Atmospheric CO2 and soil extracellular enzyme activity: a meta-analysis and CO2 gradient experiment. Ecosphere 2, 1–20. doi: 10.1890/ES11-00117.1
LeBauer, D. S., and Treseder, K. K. (2008). Nitrogen limitation of net primary productivity in terrestrial ecosystems is globally distributed. Ecology 89:371–379. doi: 10.1890/06-2057.1
Liu, L., and Greaver, T. L. (2010). A global perspective on belowground carbon dynamics under nitrogen enrichment. Ecol. Lett. 13, 819–828. doi: 10.1111/j.1461-0248.2010.01482.x
Liu, X. F., Yang, Z. J., Lin, C. F., Giardina, C. P., Xiong, D. C., Chen, G. S., et al. (2017). Will nitrogen deposition mitigate warming-increased soil respiration in a young subtropical plantation? Agric. For. Meteorol. 246, 78–85. doi: 10.1016/j.agrformet.2017.06.010
López-Aizpún, M., Arango-Mora, C., Santamaría, C., Elustondo, D., Lasheras, E., Santamaria, J. M., et al. (2017). Atmospheric ammonia concentration modulates soil enzyme and microbial activity in an oak forest affecting soil microbial biomass. Soil Boil. Biochem. 116, 378–387. doi: 10.1016/j.soilbio.2017.10.020
Lu, X. K., Vitousek, P. M., Mao, Q. G., Gilliam, F. S., Luo, Y., Zhou, G., et al. (2018). Plant acclimation to long-term high nitrogen deposition in an N-rich tropical forest. Proc. Natl. Acad. Sci. USA 115, 5187–5192. doi: 10.1073/pnas.1720777115
Moorhead, D. L., Sinsabaugh, R. L., Hill, B. H., and Weintraub, M. N. (2016). Vector analysis of ecoenzyme activities reveal constraints on coupled C, N and P dynamics. Soil Boil Biochem 93, 1–7. doi: 10.1016/j.soilbio.2015.10.019
Ramirez, K. S., Craine, J. M., and Fierer, N. (2012). Consistent effects of nitrogen amendments on soil microbial communities and processes across biomes. Glob. Change Biol. 18, 1918–1927. doi: 10.1111/j.1365-2486.2012.02639.x
Sinsabaugh, R. L., Gallo, M. E., Lauber, C., Waldrop, M. P., and Zak, D. R. (2005). Extracellular enzyme activities and soil organic matter dynamics for northern hardwood forests receiving simulated nitrogen deposition. Biogeochemistry 75, 201–215. doi: 10.1007/s10533-004-7112-1
Sinsabaugh, R. L., Hill, B. H., and Shah, J. J. F. (2009). Ecoenzymatic stoichiometry of microbial organic nutrient acquisition in soil and sediment. Nature 462:795–798. doi: 10.1038/nature08632
Sinsabaugh, R. L., Lauber, C. L., Weintraub, M. N., Ahmed, B., Allison, S. D., Crenshaw, C., et al. (2008). Stoichiometry of soil enzyme activity at global scale. Ecol. Lett. 11, 1252–1264. doi: 10.1111/j.1461-0248.2008.01245.x
Sinsabaugh, R. L., and Moorhead, D. L. (1994). Resource allocation to extracellular enzyme production: a model for nitrogen and phosphorus control of litter decomposition. Soil Biol. Biochem. 26, 1305–1311. doi: 10.1016/0038-0717(94)90211-9
Treseder, K. K. (2008). Nitrogen additions and microbial biomass: a meta-analysis of ecosystem studies. Ecol. Lett. 11, 1111–1120. doi: 10.1111/j.1461-0248.2008.01230.x
Wallenius, K., Rita, H., Mikkonen, A., Lappi, K., Lindström, K., Hartikainen, H., et al. (2011). Effects of land use on the level, variation and spatial structure of soil enzyme activities and bacterial communities. Soil Boil. Biochem. 43, 1464–1473. doi: 10.1016/j.soilbio.2011.03.018
Wang, M. W., Li, J., Shen, F. Y., Meng, J., Wang, J. L., Shan, C. F., et al. (2022). Differential responses of soil extracellular enzyme activity and stoichiometric ratios under different slope aspects and slope positions in Larix olgensis plantations. Forests 13, 845. doi: 10.3390/f13060845
Wen, Z., XU, W., Li, Q., Han, M. J., Tang, A. H., Zhang, Y., et al. (2020). Changes of nitrogen deposition in China from 1980 to 2018. Environ. Int. 144, 106022. doi: 10.1016/j.envint.2020.106022
Xu, X. K., Lin, H., Luo, X. B., and Liu, Z. R. (2009). Effects of nitrogen addition on dissolved N2O and CO2, dissolved organic matter, and inorganic nitrogen in soil solution under a temperate old-growth forest. Geoderma 151, 370–377. doi: 10.1016/j.geoderma.2009.05.008
Yu, G. R., Jia, Y. L., He, N. P., Zhu, J. X., Chen, Z., Wang, Q. F., et al. (2019). Stabilization of atmospheric nitrogen deposition in China over the past decade. Nat. Geosci. 12, 424–429. doi: 10.1038/s41561-019-0352-4
Zeglin, L. H., Stursova, M., Sinsabaugh, R. L., and Collins, S. L. (2007). Microbial responses to nitrogen addition in three contrasting grassland ecosystems. Oecologia 154, 349–359. doi: 10.1007/s00442-007-0836-6
Keywords: nitrogen addition, Larix principis-rupprechtii, soil nutrients, soil enzyme activity, North China
Citation: Yang X, Yang L, Li Q, Li X, Xu G, Xu Z and Jia Y (2023) Short-term responses of soil nutrients and enzyme activities to nitrogen addition in a Larix principis-rupprechtii plantation in North China. Front. Ecol. Evol. 11:1105150. doi: 10.3389/fevo.2023.1105150
Received: 22 November 2022; Accepted: 25 January 2023;
Published: 20 February 2023.
Edited by:
Cuicui Jiao, Sichuan University of Science and Engineering, ChinaReviewed by:
Zhiwei Xu, National School of Geographical Sciences, FranceXingliang Xu, Institute of Geographic Sciences and Natural Resources Research (CAS), China
Copyright © 2023 Yang, Yang, Li, Li, Xu, Xu and Jia. This is an open-access article distributed under the terms of the Creative Commons Attribution License (CC BY). The use, distribution or reproduction in other forums is permitted, provided the original author(s) and the copyright owner(s) are credited and that the original publication in this journal is cited, in accordance with accepted academic practice. No use, distribution or reproduction is permitted which does not comply with these terms.
*Correspondence: Yanlong Jia, jyl198620@163.com