- 1Laboratory of Genetics, Department of Plant Sciences, Wageningen University and Research, Wageningen, Netherlands
- 2Biosystematics Group, Department of Plant Sciences, Wageningen University and Research, Wageningen, Netherlands
- 3Olive and Agroecological Production Systems Laboratory, Department of Agriculture, Hellenic Mediterranean University, Heraklion, Greece
- 4Laboratory of Biological Control, Department of Entomology and Agricultural Zoology, Benaki Phytopathological Institute, Kifissia, Greece
Foraging parasitoids rely on infochemicals, derived from the habitat, host plant and/or host insect itself. Here, we studied the olive fruit fly Bactrocera oleae (Rossi) (Diptera: Tephritidae), a major pest in olive agroecosystem and its larval parasitoid, Psyttalia concolor (Szépligeti) (Hymenoptera: Braconidae), a well-established and abundant wasp in Crete. To improve parasitoid’s efficiency as biological control agent, more knowledge on its host location behavior is required. The aim of this study was to investigate the role of oviposition- (OIPVs) and herbivore-induced plant volatiles (HIPVs) emitted by olive trees upon infestation by B. oleae as well as cues emitted by B. oleae, e.g., pheromones, therein. We conducted two-choice bioassays to test the preference of P. concolor to different olfactory stimuli. Moreover, we collected headspace volatiles from olive fruits and branches to investigate whether egg or larval infestation changes volatile emissions locally or/and systemically. We found that P. concolor wasps showed a strong preference to volatiles of B. oleae larval-infested fruits (HIPVs) compared to clean air, non-infested, egg-infested, and mechanical-damaged fruits. But they were not able to discriminate between volatiles of non-infested and larval-infested branches. In addition, the wasps were attracted to cues emitted from the virgin and mated B. oleae females. They were neither attracted to cues emitted by the virgin and mated males nor to the olive fruits with or without the cues of the oviposition marking of the B. oleae. We found important qualitative differences in volatile profiles of egg- and larval-infested fruits: nineteen volatile compounds were only detected in infested fruits, such as the terpenoids (E)-β-ocimene and (E,E)-α-farnesene. Moreover, volatile analysis showed that olives change volatile emissions locally and systemically in response to larval infestation. Our results suggest that both HIPVs and host cues play an important role to P. concolor during host location. Further knowledge on the chemical compounds utilized by the parasitoid to locate infested fruits could help application in olive orchards and to improve the control of the olive fruit fly by natural enemies.
Introduction
Successfully locating a suitable host increases the Darwinian fitness of parasitic wasps and is therefore strongly influenced by natural selection (van Alphen and Vet, 1986). For this reason, parasitoids have developed various strategies to optimize their searching efficiency. These host foraging strategies can be divided into different phases, i.e., host habitat location, host location, and host acceptance (Vinson, 1998). On one hand, stimuli that derive from plants after insect herbivory (host plants) are highly detectable and can be used by parasitoid wasps to find the host-habitat in a long distance (Vet et al., 1991). On the other hand, stimuli that derive from the host itself (insect host) are less detectable at longer distances but more reliable in close vicinity, e.g., during host location (Vet et al., 1991). During host finding, hymenopteran parasitoids highly depend on chemical cues, so-called infochemicals or semiochemicals, mostly deriving from the host plants (i.e., cues that may serve as synomones) and/or their insect hosts (i.e., cues that may serve as host kairomones) (Vet and Dicke, 1992; Godfray, 1994; Jayanthi et al., 2020; Ayelo et al., 2021). Infochemicals that mediate the communication between different species are characterized as (a) synomones when it is beneficial for both the emitter and the receiver (e.g., plant and natural enemies of pests) and (b) kairomones when it is beneficial for the receiver and detrimental for the emitter (e.g., natural enemies of pests and pests) (Vet and Dicke, 1992).
Plants emit a plethora of volatile organic compounds (VOCs) that facilitate insect-plant interactions (Kessler and Baldwin, 2002). Volatile emission can be induced either by egg deposition (i.e. oviposition-induced plant volatiles – OIPVs) or feeding damage (i.e. herbivore-induced plant volatiles – HIPVs) by herbivorous insects (Dicke, 2009; Mumm and Dicke, 2010). Both OIPVs and HIPVs have been shown to play an important role in host or prey location for parasitoids and predators and could act as synomones (Mattiacci et al., 1994; Dicke and Vet, 1999; Calvitti et al., 2002; Tamiru et al., 2011; Fatouros et al., 2012; Giunti et al., 2016; Milonas et al., 2019). Additionally, parasitoids have evolved strategies to exploit host-derived chemical cues, such as pheromones (Vet and Dicke, 1992; Fatouros et al., 2008). Parasitoids are known to eavesdrop on pheromones originated from their insect hosts. They may use these chemical signals to detect their host which then function as kairomones (Vet and Dicke, 1992; Benelli et al., 2014a; Greenberg et al., 2023). Both plant-derived (OIPVs or HIPVs) and host-derived volatiles (pheromones) may have a synomonal or kairomonal effect, respectively, and could perceived by parasitoids (Vet and Dicke, 1992; Fatouros et al., 2008; Milonas et al., 2009). Parasitoids are often used as biological control agents in agriculture (van Lenteren, 2012). Exploring the kairomone- and synomone-based foraging behavior of parasitoids could enhance biological control (Peri et al., 2018; Turlings and Erb, 2018; Ayelo et al., 2021).
The olive fruit fly, Bactrocera oleae is the major pest of the olive tree (Olea europaea L., Oleales: Oleaceae). It is a monophagous frugivorous insect that exclusively attacks the fruits of cultivated and wild olive trees (Daane and Johnson, 2010). Bactrocera oleae infestation causes considerable damage to olive fruits with larvae feeding on the mesocarp of the fruits and results to dramatic yield losses and decrease of olive quality (Daane and Johnson, 2010). The control of this pest is mainly based on application of synthetic pesticides, such as the organophosphate and pyrethroid insecticides, that lead to olive fruit fly resistance (Daane and Johnson, 2010; Kampouraki et al., 2018), residues in olive products (Amvrazi and Albanis, 2009; Daane and Johnson, 2010), and environmental degradation (Daane and Johnson, 2010). Furthermore, synthetic pesticides can be harmful for the non-target organisms and beneficial insects (Daane and Johnson, 2010; Calvo-Agudo et al., 2020). Hence, an environmentally friendly method to effectively suppress this pest is of great importance.
Psyttalia concolor is a koinobiont generalist larval endoparasitoid which is known to parasitize many Tephritidae species, including the 2nd and 3rd instar larvae of B. oleae (Sime et al., 2006). Psyttalia concolor was first introduced to Europe (Italy in 1914 and France in 1919) from north Africa and used in classical biological control programs to suppress the olive fruit fly and the Mediterranean fruit fly, Ceratitis capitata (Wiedmann) (Diptera: Tephritidae) (Daane and Johnson, 2010). It is a species that is well adapted to the introduced areas but its effectiveness as biocontrol agent gave mixed results (Daane and Johnson, 2010). It has been reported that after mass releases of this parasitoid wasp, their effectiveness does not last more than one season and inundative releases are needed to keep the pest density low (Daane and Johnson, 2010). Thus, a better understanding of the host location behavior of this parasitoid wasp is required in order to improve its efficiency as biocontrol agent and to optimize the olive fruit fly control.
Previous studies have reported that P. concolor wasps respond to olfactory stimuli released by plants and that they are able to discriminate between volatiles from infested and non-infested plants with a preference for cues released by infested ones (Benelli et al., 2013; Giunti et al., 2016). For instance, infestation by C. capitata on peach and apple, and by B. oleae on olive fruits alters the volatile blend of the infested fruits which was exploited by P. concolor wasps (Benelli et al., 2013; Giunti et al., 2016). The HIPV induction by the feeding damage by B. oleae on olive fruits (Alagna et al., 2016; Giunti et al., 2016) is an indication that olive may have evolved indirect defense mechanism in response to B. oleae herbivory. However, the effect of OIPVs on the larval parasitoid P. concolor has not been tested so far. Recent research showed that OIPVs are not exclusively exploited by egg parasitoids but can be used by larval parasitoids as well in order to find their hosts on time before they even start feeding on the plant (Tamiru et al., 2011; Fatouros et al., 2012; Pashalidou et al., 2015). In addition, previous studies mainly focused on the olive fruits (Malheiro et al., 2015; Kokkari et al., 2021) and only a few analyzed also the volatile profile of olive leaves (Scarpati et al., 1993; Malheiro et al., 2016). However, none of them have investigated yet the volatile profile of olive branches carrying fruits and leaves upon infestation by B. oleae.
Sex pheromones of the host adult stage have been recorded to attract many parasitoid species belonging to Trichogrammatidae, Eupelmidae, Eulophidae, and Braconidae families (Benelli et al., 2014a). In addition, oviposition marking pheromones have been reported to be detected by entomophagous insects, such as parasitoids, in order to find their host (Nufio and Papaj, 2001). For example, Trichogramma egg parasitoids and the parasitoid Halticoptera rosae (Burks) (Hymenoptera, Pteromalidae) are able to exploit the sex pheromones and oviposition marking pheromones, respectively, in order to locate their hosts (Vet et al., 1991; Nufio and Papaj, 2001). Attraction of the P. concolor female wasps was tested against synthetic compounds of the two major sex pheromones produced by B. oleae female (i.e., 1,7-dioxaspiro[5.5]undecane) and male (i.e., (Z)-9-tricosene) flies. The wasps were found to be attracted only to male-borne (Z)-9-tricosene (Benelli et al., 2014a). However, the chemical cues released directly by B. oleae adults (males and females) or cues deposited on the surface of the fruits during egg deposition, have not been investigated yet in relation to P. concolor host location behavior.
Yet it remains unclear, which chemical cues do the P. concolor specifically use that mediate O. europaea-B. oleae-P. concolor interactions. This is the first study in which a thorough chemical analysis of olive branches (with fruits and leaves) after being exposed to B. oleae infestation is conducted. Moreover, this is the first time that the role of OIPVs and volatiles from B. oleae infested branches in the foraging behavior of P. concolor wasps is investigated. We studied the host location behavior of the parasitoid P. concolor subject to different olfactory stimuli, both from the plant as well as from its host B. oleae. The aim of this study was to elucidate the role of olfactory cues emitted from the plant-host complex (i.e., OIPVs and HIPVs – plant synomones) and from the herbivore itself (i.e., pheromones and cues left on fruits by ovipositing B. oleae females - host kairomones) to parasitoid attractiveness. We hypothesized that (a) B. oleae egg and larval-infestation activate olive indirect defense mechanism by altering the volatile blend of the infested fruits, (b) B. oleae larval-infestation induces HIPVs production in leaves that are adjacent to infested fruits, and (c) the pheromones released by the host attract P. concolor wasps. More specifically, we investigated: (a) whether volatiles induced by egg deposition or larval feeding of B. oleae attract the parasitoid P. concolor, (b) whether the olive tree responds locally or/and systemically to feeding damage by the B. oleae larvae, and (c) role of the adult host kairomones, i.e., cues from fruits that just received egg deposition and chemical cues released directly by male and female B. oleae flies, on parasitoid’s attractiveness. Finally, we analyzed the chemical composition of the volatiles emitted by the olive fruits alone (local plant response) and branches carrying fruits and leaves (systemic plant response) to assess the biochemical changes that occur after B. oleae infestation and whether the olive tree invest in indirect resistance to counteract the pest infestation.
Materials and methods
Plants and insects
Olive fruits and branches from about thirty-five-year-old olive trees of ‘Koroneiki’ cv. used in the experiments were collected from the experimental organic olive orchard of the Laboratory of Olive and Agroecological Production Systems, Hellenic Mediterranean University in Heraklion, Greece (35°19΄03΄΄N, 25°06΄20΄΄E). Laboratory colonies of B. oleae and P. concolor were established by using adults that emerged from B. oleae infested olive fruits collected from the experimental organic olive orchard. Bactrocera oleae flies were fed with a mixture of sugar, yeast flakes, and milk powder (2:1:1 volume ratio), 50% honey solution and water ad libitum. Olive fruit flies used in the experiments were obtained from the laboratory colony reared on olive fruits under controlled conditions (21 ± 1°C, 50–60% R.H. and natural photoperiod). The parasitic wasp P. concolor was reared on B. oleae L2-L3 instar larvae of the laboratory colony. The parasitoid colony was maintained in the laboratory under controlled conditions (21 ± 1°C, 50–60% R.H. and natural photoperiod) and adult parasitoids were fed on 50% honey solution and water. Female parasitoids were assumed mated and used for the behavioral experiments at six to eight days after emergence.
Plant and insect treatments
Olive fruits alone and branches were used for the behavioral bioassays as well as for the headspace collection of volatiles (Figure 1). Only olive fruits with yellowish-green and yellowish epidermis color (maturation index 0–1) (Guzmán et al., 2015), which are preferred for oviposition by the olive fruit fly, were used for the experiments while fruits with purple and black epidermis were discarded. All plant material (fruits and branches) acclimated to laboratory conditions (21 ± 1°C, 50–60% R.H.) for 24 h prior to behavioral experiments and VOC collection. Furthermore, B. oleae male and female adults were used in the behavioral experiments. Figure 1 illustrates the treatments used in each of the experiments.
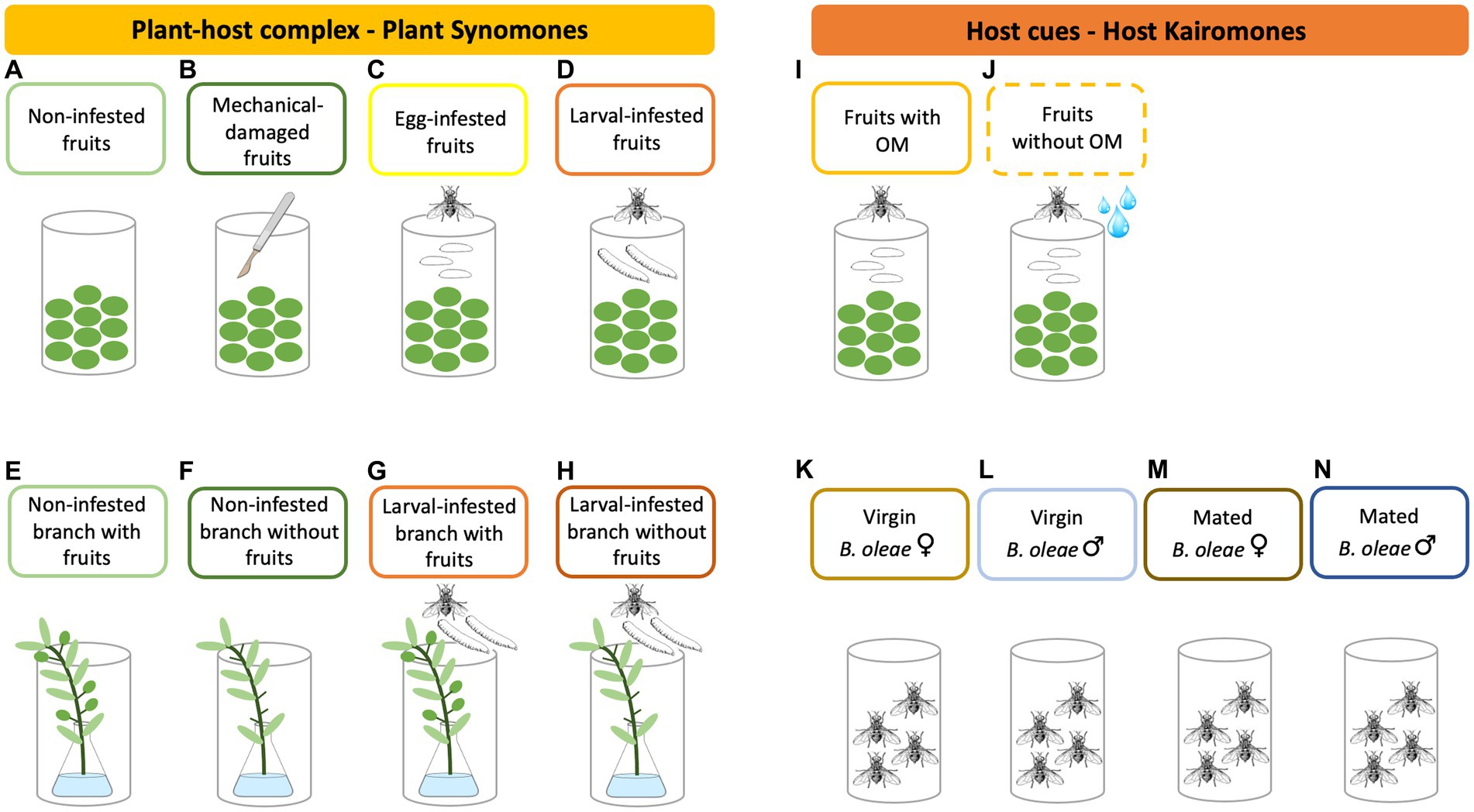
Figure 1. Schematic representation of the plant and insect treatments used in the experiments to assess: (a) the preference of the parasitic wasp Psyttalia concolor to volatile cues emitted by the host plant (Oleae europaea L.) and host insect (Bactrocera oleae) and (b) whether B. oleae infestation changes volatile emission in fruits and/or branches. From the plant-host complex, we used for both the behavioral bioassays and the volatile analysis olive fruits alone as well as olive branches. Olive fruits treatments: non-infested (A) (control) (light green), mechanical-damaged (B) (to mimic the oviposition puncture of B. oleae) (dark green), B. oleae egg-infested (C) (yellow), and B. oleae larval-infested (D) (orange) fruits. We used 100 fruits (200–250 g) per replicate for the behavioral bioassays and 500–800 g fruits per replicate for the volatile analysis. Olive branches treatments: non-infested branch with fruits (E) (light green), non-infested branch without fruits (F) (fruits were removed prior testing) (dark green), larval-infested branch with fruits (G) (orange), and larval-infested branch without fruits (H) (fruits were removed prior testing) (brown-red). For both behavioral bioassays and volatile analysis, a 25–30 cm long branch with 20–30 leaves and 15–20 fruits were used per replicate. From the host cues, we used in the behavioral bioassays freshly deposited fruits by B. oleae females (100 fruits with the oviposition marking (OM) of B. oleae or without per replicate) and B. oleae adults (twenty adults per replicate). Treatments of freshly deposited fruits: fruits with the OM of B. oleae (I) (gold) and fruits without the OM of B. oleae (J) (fruits were washed to remove the cues from the fly) (gold dashes). Treatments with B. oleae adults (8–10 days old): virgin females (K) (light yellow-brown), virgin males (L) (light blue), mated females (M) (dark yellow-brown), and mated males (N) (dark blue).
Olive fruits
Non-infested fruits were carefully collected from the experimental olive orchard and used as control treatment avoiding damaged or unhealthy fruits (Figure 1A). To obtain mechanically damaged fruits, non-infested fruits were carefully wounded with a sterile scalpel blade without damaging the endocarp 24 h prior testing, in order to mimic the oviposition puncture of the B. oleae adult females (Figure 1B). This procedure was performed directly in the field in non-infested fruits and then the branch with the treated fruits was covered with a mosquito net to avoid further damage or pest infestation. Furthermore, we carefully collected B. oleae infested fruits directly from the trees. Specifically, we selected those fruits that were either infested with two to three oviposition punctures (egg-infested fruits) or with L2-L3 instar larvae (larval-infested fruits) with no exit holes or any other pest infestation, disease, or mechanical damage on the epidermis of the fruits (Figures 1C,D). Then the collected fruits (non-infested, infested and mechanical-damaged fruits) were transferred to the laboratory and stored in a refrigerator at 4–5°C for 24 h up to 48 h before using them for the experiments. For the behavioral bioassays, we used one hundred fruits (200–250 g) while for the collection of VOCs 500–800 g fruits were used.
Olive branches
Olive branches, 25–30 cm long with 20–30 leaves and 15–20 fruits, were collected from the experimental olive orchard. Branches with non-infested and larval-infested fruits (L2-L3 instar larvae) were cut from the trees and were immediately transferred to the laboratory and kept in water up to 24 h prior the experiments as well as during the period of testing (Figures 1E–H). All branches tested during the behavioral experiments and the VOC collection were carrying both leaves and fruits except of those that had to be tested without their fruits. More specifically, shortly prior the behavioral experiments and VOC collection the fruits (non-infested or infested fruits) were carefully removed from the branches and only the leaves left during the experiments.
Cues left on fruits by ovipositing olive fruit fly females
Non-infested fruits were placed in bugdorm cages for an hour with twenty females B. oleae to obtain fruits with fresh B. oleae oviposition markings (fresh deposited fruits) (Figure 1I). The female adults were observed during the oviposition to make sure that the fruits were freshly deposited and that they can be used immediately in the behavioral experiments. Moreover, to obtain fruits without the cues left by the female fly during oviposition (Figure 1J), the deposited fruits were soaked in a solution of distilled water and 1% bleach for 30 min. After treatment, the fruits were rinsed with distilled water, washed gently with a sponge in a solution of distilled water and 1% bleach, were rinsed and then soaked overnight in distilled water. The next day the fruits were rinsed with distilled water, washed with a sponge and dry them before conducting the behavioral experiments. After egg deposition, B. oleae females use their labellum to take out the juice of the olive fruits from the oviposition punctures in order to mark the fruit (Benelli et al., 2014b). There is a possibility that host marking pheromones (HMPs) could be left on fruits surface but we did not specifically test them.
Cues emitted by the olive fruit fly
Twenty adult male or female and virgin or mated B. oleae flies were used for the behavioral experiments at eight to ten days after emergence (Figures 1K–N). Twenty-four hours prior the behavioral bioassays, twenty males and twenty females (1 male:1 female) were placed in a bugdorm cage in order to obtain mated B. oleae males and females. Whereas the virgin males and females were kept in separate cages from the first day after emergence.
Behavioral bioassays in dynamic Y-tube olfactometer
The response of P. concolor wasps to chemical cues emitted by the plant (olive tree) and by the host (olive fruit fly) was tested in a dynamic air-flow Y-tube glass olfactometer (main arm length 10 cm, side arm length 8 cm, ∅1 cm) (Figure 2A) as previously described by Milonas et al. (2019). Each arm of the olfactometer was connected with Teflon tubing to a 2 L cylindrical plexiglass container holding the odor source. Air was pumped (Dymax 5 air-sampling vacuum pump, Supelco, Charles Austen Pumps Ltd., UK) through an active charcoal filter, humidified by passing it through a conical 2 L glass flask contained tap water and split into the two arms of the olfactometer at a flow rate of 30 mL min−1. The olfactometer was placed in a panel covered with black fabric in order to prevent the wasps from visually spotting the tested material in odor containers as well as to prevent light entering from the side. The bioassays were conducted at 21 ± 1°C and 50–60% R.H. using three 18 W cool fluorescent light bulbs above the olfactometer providing uniform lighting. In this two-choice set-up we offered to the wasps two different odor sources (testing pair) to choose from. Naive female parasitoids were individually released at the downwind end of the main arm of the olfactometer and observed for 5 min until making a choice. The first choice of the wasps was used as parameter to estimate the attraction to different odor source offered. A choice was recorded when the parasitoid crossed at least two-thirds of one of the side arms and stayed there for 15 s. When the wasps did not make a choice within 5 min, it was counted as ‘no response’ and excluded from the statistical analysis (Giunti et al., 2016; Ponzio et al., 2016). The number of wasps that did not make any choice was excluded only for the statistical analysis but included in the calculations to determine the percentage of the total wasps’ response. The wasp was used only once and then discarded. After every trial, the position of the odor sources was switched and the olfactometer was rotated to avoid any position bias. The olfactometer was washed with mild soap, hot water and acetone after every replicate. A total of fifteen wasps were released for each plant-pair (experiments with fruits or branches) or insect-pair (experiments with the B. oleae) combination. Each testing pair (bioassay combination) consisted of fifteen or six replicates (plant pairs or insect pairs). In each testing pair, we tested 225 or 90 wasps.
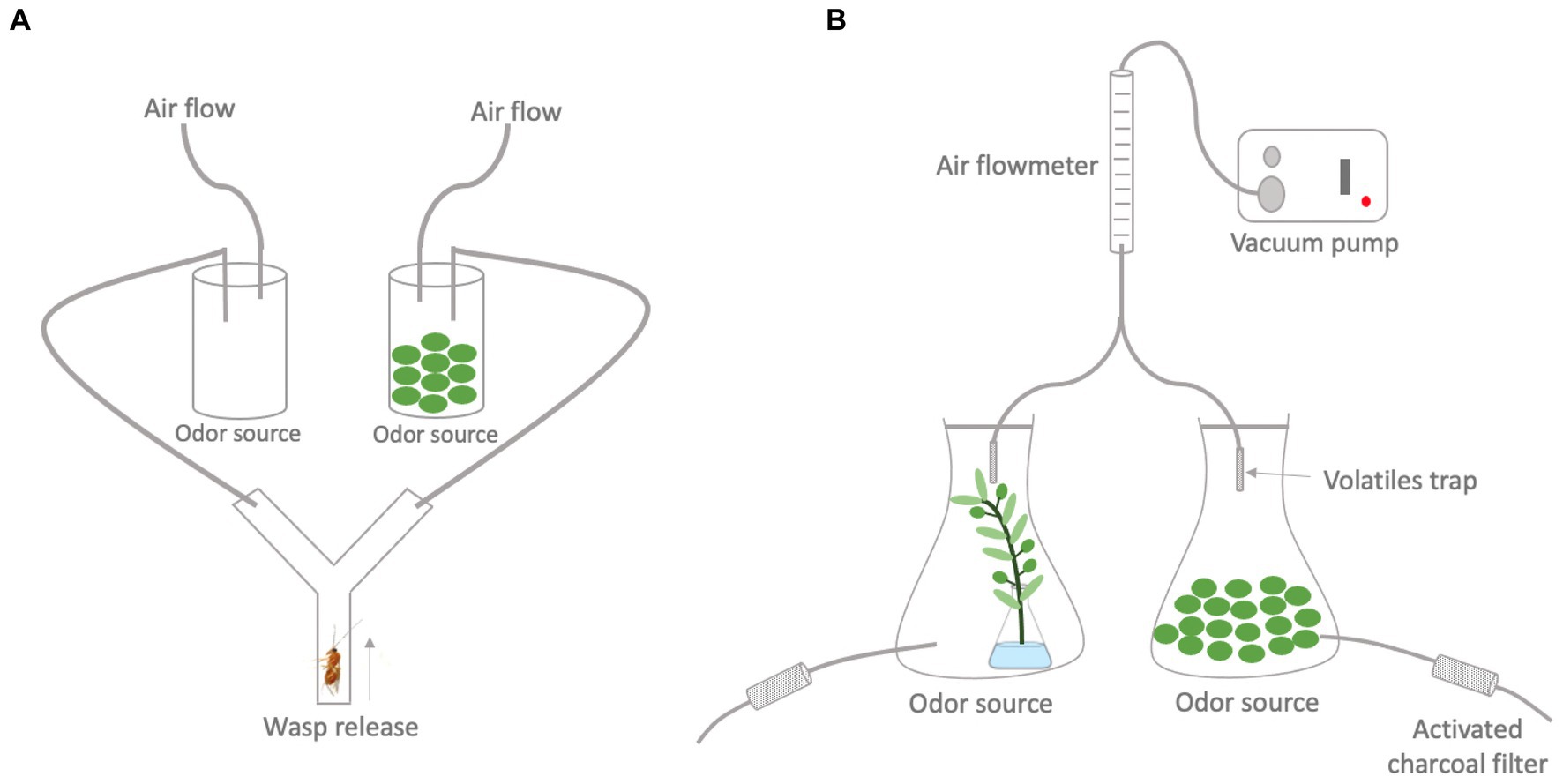
Figure 2. Schematic representation of the (A) Y-tube olfactometer set-up used in the behavioral bioassays to test volatile preferences of Psyttalia concolor wasps and (B) set-up for the headspace collection of plant volatiles.
Responses of Psyttalia concolor wasps to different olfactory stimuli
In the behavioral experiments, we tested the attraction of the wasps to volatiles emitted by the olive tree (fruits and branches) and to cues emitted by its host B. oleae (Table 1). The response of the wasps to (i) clean air, (ii) non-infested fruits, (iii) egg-infested fruits, (iv) larval-infested fruits, and (v) mechanical-damaged fruits was tested in order to determine whether B. oleae infestation (egg- or larval-infested fruits) induce the release of volatiles that could attract the wasps. Moreover, the mechanical-damaged fruits (to mimic the oviposition puncture of the B. oleae) were used to test if artificial damaging of the fruits could induce the emission of volatiles that may attract the wasps. To investigate whether the feeding damage by the olive fruit fly alters the volatile blend of the infested olive branches and whether the induction of the volatiles is restricted to the infested fruits or also to the adjacent leaves (systemic induction of the volatiles) we tested the response of the wasps to: (i) clean air, (ii) non-infested branches with fruits, (iii) non-infested branches without the fruits (fruits removed prior testing), (iv) larval-infested branches with fruits, and (v) larval-infested branches without the fruits (fruits removed prior testing). Wasps’ response was tested also to cues left on fruits by B. oleae ovipositing females: (i) clean air, (ii) fruits with the oviposition marking, and (iii) fruits without the oviposition marking (washed fruits). Additionally, we investigated wasps’ response to cues emitted directly by the olive fruit flies: (i) clean air, (ii) B. oleae virgin females, (iii) B. oleae virgin males, (iv) B. oleae mated females, and (v) B. oleae mated males.
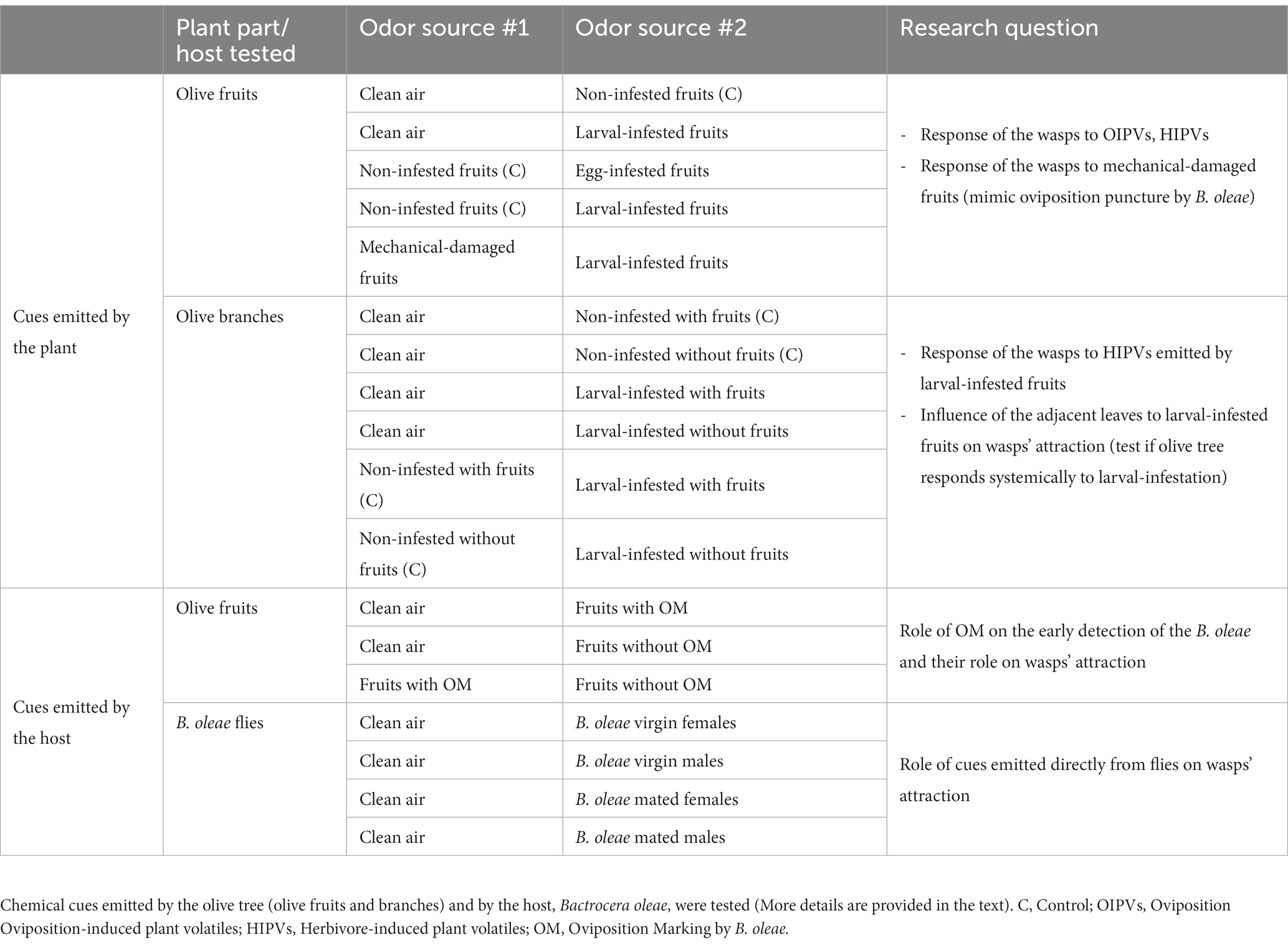
Table 1. An overview of the behavioral experiments and treatments conducted in a two-choice Y-tube olfactometer to test the response of Psyttalia concolor wasps (first choice) to two different odor sources.
Headspace collection of plant volatiles and chemical analysis
To investigate whether B. oleae infestation changes plant volatile emission in fruits and/or branches, and thus P. concolor wasps’ preference, volatiles from the headspace of olive fruits and branches were collected (Figure 2B). Headspace volatiles were collected by enclosing the fruits and branches with the different treatments in an oven bag made by polyethylene terephthalate (PET), with thickness 12 μm, and dimensions 35 × 43 × 43 cm (SANITAS, Sarantis Group, Greece). A tape was wrapped around the top and bottom end to close the oven bag and each of the treatments were left for 30 min for acclimatization prior to volatile collection. In all cases, plant volatile collection was performed by using dynamic headspace sampling as outlined in Anastasaki et al. (2018). Ambient air was purified through an activated charcoal filter (10 cm length × 1.5 cm i.d.) containing 0.5 g of activated charcoal (Merck, Germany) tapped with glass wool (extra fine, Assistent, Germany) by inserting Teflon tubing through an opening in the bottom part of the bag. Air was sucked out and headspace volatiles were collected in Teflon-made traps for four hours (at 20 ± 1°C, 50–65% RH), at a flow rate of 360 mL min−1 (Dymax 5 air-sampling vacuum pump, Supelco, Charles Austen Pumps Ltd., UK) by inserting Teflon tubing through an opening in the upper part of the bag. The Teflon-made traps (5 cm length × 4 mm i.d.) were filled with 75 mg Porapak Q 80/100 mesh (Supelco, Bellefonte, PA, USA), tapped with a 2 mm glass wool and 3 mm Teflon tubes on each end. Prior volatile collection, the Teflon-made traps were sequentially washed with 1 mL of methanol, diethyl ether, and n-pentane (Fisher Chemicals, Bishop, UK) and blown dry with N2. After use oven bags were discarded. After the collection, the adsorbent traps were extracted immediately with 500 μL of n-pentane and stored in a freezer (−20°C) in a sealed vial with a conical inserter until chemical analysis. Prior to chemical analysis, the samples were concentrated to 100 μL under a gentle stream of nitrogen. Blank collections of the system (oven bag with no plant material) were performed following elution with 500 μL n-pentane as described above. Background volatiles from these experiments were subtracted from treatment collections.
Headspace samples were analyzed in a gas chromatograph (GC) (Nexis™ GC-2030, Shimadzu, Japan) connected to a single quadrupole mass spectrometer (MS) (GCMS-QP2020 NX Shimadzu, Japan). One μL of the extract was used for the analysis and injected in GC–MS instrument. Separation of the analytes was performed with a MEGA-5 MS capillary column (5% diphenyl / 95% dimethyl polysiloxane) with the following dimensions: 30 m length, 0.25 mm i.d., and 0.25 μm film thickness (Mega, Legnano, Milan, Italy). Spitless mode was set for 1 min. The flow rate of the carrier gas helium was 1 mL min−1. The oven temperature was maintained at 50°C for 5 min, increased at a rate of 3°C min−1 to 170°C and at a rate of 20°C min−1 to the final temperature of 250°C. The mass spectrometer was operated in Electron ionization (EI) mode with ion energy of −70 eV, filament current 50 μA and source temperature 200°C. Data acquisition was performed in full scan (MS) with scanning range 40–300 amu. Compounds were identified by comparing their elution order, the mass spectra with those from mass spectra libraries (Adams, 2007; NIST 17, Wiley7) and literature data (Adams, 2007; NIST – WebBook site). We also used as an additional criterion retention index (RI) of a series of n-alkane (C8-C20). Identified compounds were confirmed by comparing RIs with those from the literature (Adams, 2007; NIST – WebBook site). Wherever possible, RI, retention time and mass spectra were compared with commercial standards. The total ion chromatogram was processed by LAB solutions software (version 4) based on the retention time and mass spectrum. Emission rates were calculated for compounds that were detected in at least 50% of the samples for each treatment. Six replicates per treatment were used. Chemical analysis was performed in Benaki Phytopathological Institute, Kifissia, Greece.
Statistical analysis
Wasps’ preference to different olfactory stimuli were analyzed using binary logistic regression [i.e., a generalized linear model (GLM)] with a binomial distribution and a logit link function. We used the wasps’ first choice as the response variable, while the plant or insect treatment and the replicates used as fixed factors. Differences in VOC profile were analyzed using Projection to Latent Structures Discriminant Analysis (PLS-DA) (SIMCA 16.0.1, Umetrics AB, Umeå, Sweden). Volatile compounds measured as peak area divided by the fresh weight of the plant part tested. Data were log-transformed, mean-centered and scaled to unit variance before PLS-DA processing. Additionally, for each model, compounds with a variable importance for the projection (VIP) value higher than one was generated as well. VIP values estimate the importance of each variable (compound) in the projection and is often used for variable selection. Moreover, the composition of the volatile blend was analyzed using a cluster analysis and data visualized with heatmaps (MetaboAnalyst 5.0). Clustering was performed using Ward’s clustering algorithm with Euclidean distances. Data were log-transformed, range scaled and averaged per treatment. To assess the differences regarding volatile emission per compound between non-infested, B. oleae-infested and mechanically damaged fruits and branches, we used one-way ANOVA with Tukey’s post hoc tests for pairwise comparisons or Kruskal-Wallis H tests depending on data normality. Plant treatment used as the independent variable and the volatile emission per compound as the dependent variable. Data normality was assessed by the Shapiro–Wilk test. Statistical analyses were performed using IBM SPSS Statistics 28.0 (IBM corp.).
Results
Psyttalia concolor responses to plant-derived chemical cues
When testing the wasps’ responses to volatiles from non-infested fruits against clean air, the wasps significantly preferred volatiles of non-infested fruits (Figure 3A; GLM, x2 = 90.652, df = 1, p ≤ 0.001). Psyttalia concolor wasps were more attracted to volatiles emitted by B. oleae larval-infested fruits (HIPVs) (Figure 3A) when tested either against clean air (GLM, x2 = 77.984, df = 1, p ≤ 0.001), non-infested fruits (GLM, x2 = 160.747, df = 1, p ≤ 0.001), or mechanical-damaged fruits (GLM, x2 = 129.091, df = 1, p ≤ 0.001). In contrast, volatiles of B. oleae egg-infested fruits (OIPVs) did not influence the attraction of the wasps when tested against volatiles of non-infested fruits (Figure 3A; GLM, x2 = 0.786, df = 1, p = 0. 074).
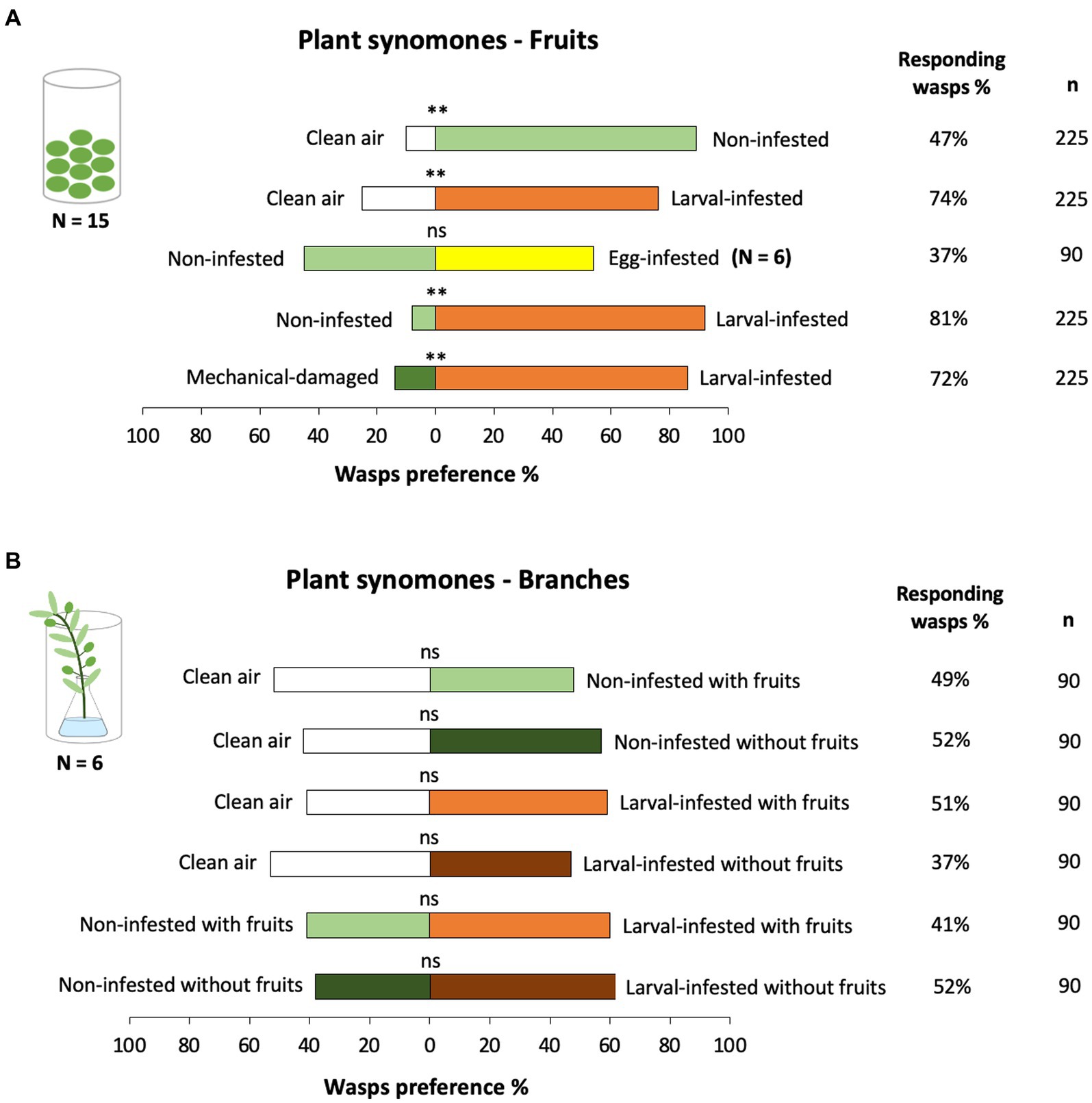
Figure 3. Percentage of first choice (preference %) of Psyttalia concolor female wasps to different chemical cues emitted by olive fruits and branches tested in two-choice bioassays in a Y-tube olfactometer. Volatiles of: (A) non-infested fruits (control) (light green), Bactrocera oleae egg- (green) or larval-infested (orange) fruits, and mechanical-damaged fruits (dark green) and (B) non-infested (control) (light green) and larval-infested (orange) branches with fruits, and non-infested (control) (dark green) and larval-infested (brown-red) branches without the fruits (fruits were removed prior behavioral bioassays) were tested. Asterisks indicate significant differences between treatments: **p ≤ 0.001, ns = not significant at the 0.05 level (GLM). Fifteen or six replicates were conducted for each bioassay combination and fifteen wasps were tested per plant-pair. N indicates the number of replicated testing pairs. The percentage of responding wasps and the total number of the tested female wasps (n) per testing pair is given on the right side of the bars.
Psyttalia concolor wasps were not attracted to volatiles from the differently treated branches (Figure 3B), neither when testing branches (a) with non-infested fruits (GLM, x2 = 0.182, df = 1, p = 0.670), (b) with non-infested fruits from which the fruits were removed prior testing (GLM, x2 = 0.392, df = 1, p = 0.531), (c) with larval-infested fruits (GLM, x2 = 2.754, df = 1, p = 0.097), nor (d) with larval-infested fruits from which the fruits were removed prior testing (GLM, x2 = 0.971, df = 1, p = 0.325) against clean air. Similarly, we observed no differences in wasps’ preference when testing volatiles of non-infested branches with fruits against larval-infested branches with fruits (GLM, x2 = 2.697, df = 1, p = 0.101) or non-infested branches with fruits that have been removed prior testing against larval-infested branches with the fruits that have been removed prior testing (GLM, x2 = 2.752, df = 1, p = 0.097).
Psyttalia concolor responses to host-derived chemical cues
To test the wasps’ response to cues deposited by female flies on the fruit (potential HMPs), we used fruits that were in contact with ovipositing female flies and tested them against fruits that were washed before testing. The parasitoid was not attracted to cues emitted from the fruits with the oviposition marking cue (GLM, x2 = 0.725, df = 1, p = 0.394) nor to fruits without the oviposition marking cue (GLM, x2 = 1.492, df = 1, p = 0.222) when tested against clean air. Similarly, the wasps were not able to discriminate between fruits with the oviposition marking cue and fruits without the oviposition marking cue (GLM, x2 = 0.000, df = 1, p = 1.000) (Figure 4A). Psyttalia concolor wasps significantly preferred the volatiles emitted by virgin female B. oleae (Figure 4B; GLM, x2 = 7.571, df = 1, p = 0.006) and mated female B. oleae (GLM, x2 = 6.028, df = 1, p = 0.014) when tested against clean air. In contrast, the parasitoid was neither attracted to cues emitted by virgin males (GLM, x2 = 2.724, df = 1, p = 0.099) nor mated males (GLM, x2 = 1.599, df = 1, p = 0.206) B. oleae when tested against clean air (Figure 4B).
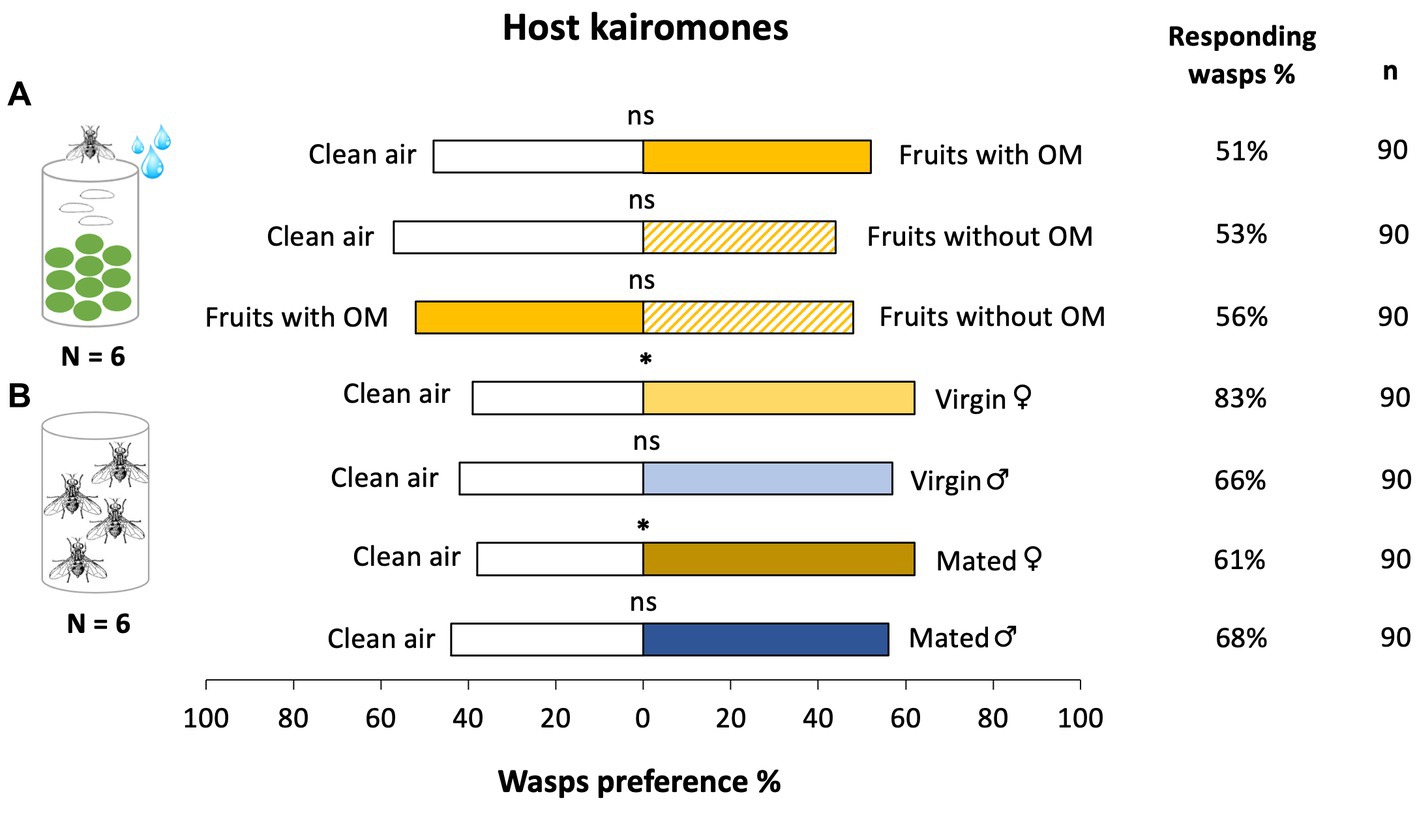
Figure 4. Percentage of first choice (preference %) of Psyttalia concolor female wasps to different chemical cues emitted by Bactrocera oleae (host) tested in two-choice bioassays in a Y-tube olfactometer. Cues emitted from (A) fruits with the oviposition marking (OM) of B. oleae (freshly deposited fruits) (gold) and fruits without the OM (B. oleae deposited fruits were washed prior testing) (gold dashes) and (B) virgin and mated female (light yellow-brown and dark yellow-brown respectively) or male (light blue and dark blue respectively) B. oleae flies. Asterisk indicates significant differences between treatments: *p < 0.05, ns = not significant at the 0.05 level (GLM). Six replicates were conducted for each bioassay combination and fifteen wasps were tested per plant- or insect-pair. N indicates the number of replicated testing pairs. The percentage of responding wasps and the total number of the tested female wasps (n) per testing pair is given on the right side of the bars.
Headspace analysis of plant volatiles
A total of fifty-one volatile organic compounds were detected in the headspace of the olive fruits alone and forty-five compounds from the olive branches. Most of the detected compounds belong to five major classes of plant volatiles released after herbivory: benzenoids and phenylpropanoids, monoterpenoids, homoterpenoids, sesquiterpenoids, and fatty-acid and amino-acid derivatives (Supplementary Tables S1, S2). Overall, qualitative and quantitative differences were observed in the volatile profiles of οlive fruits alone as well as in branches with or without fruits (Figures 5A, 6A, 7; Supplementary Tables S1, S2; Supplementary Figures S1, S2).
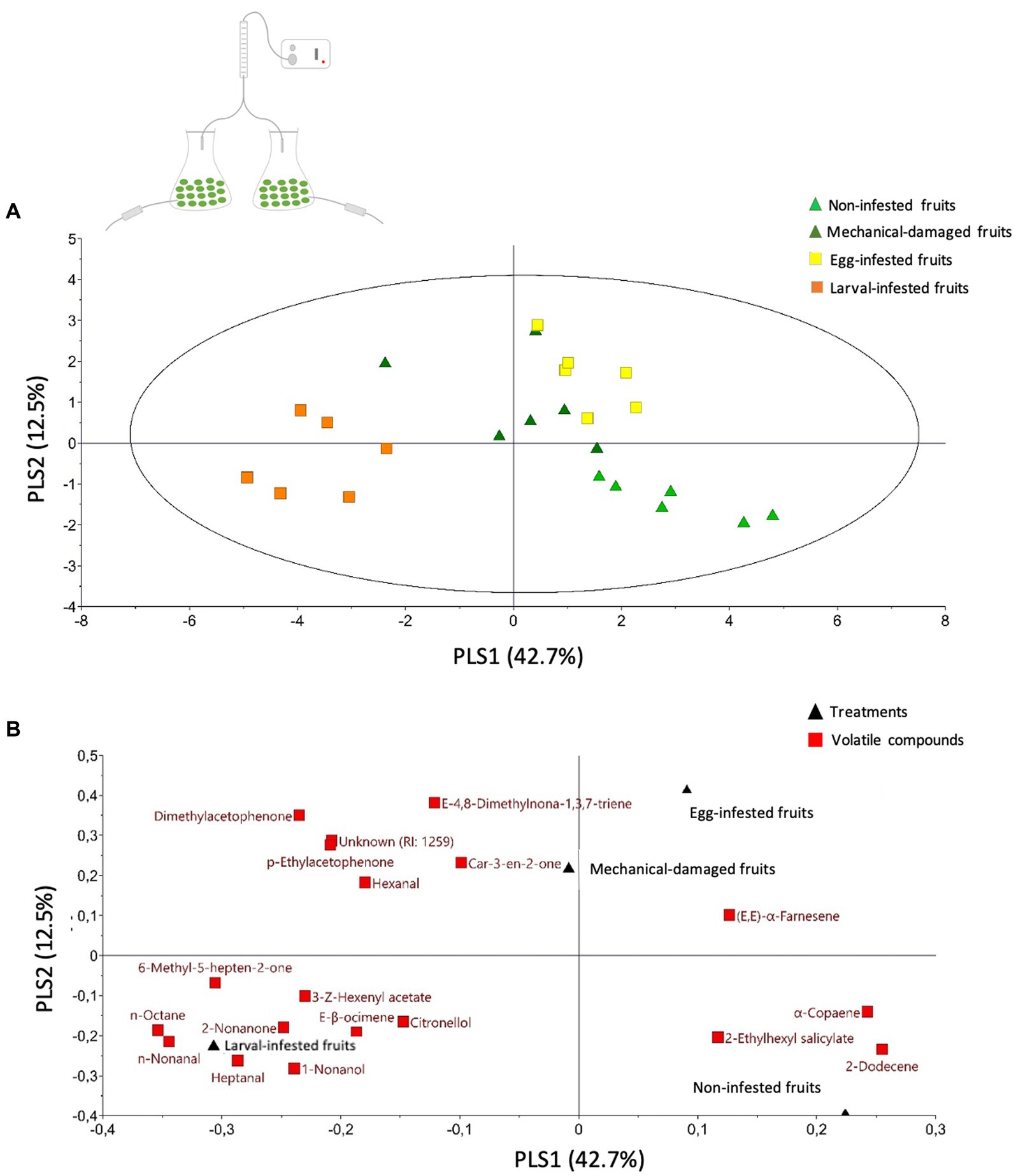
Figure 5. Volatile profile of the headspace of olive fruits exposed to Bactrocera oleae infestation (egg- or larval-infestation) or not exposed to herbivory at all. Projection to Latent Structures Discriminant Analysis (PLS-DA) of volatile compounds with VIP values >1 emitted from differently treated olive fruits. Olive fruits were either: non-infested (control) (light green triangle), mechanical-damaged (dark green triangle), or B. oleae egg-infested (yellow square) or larval-infested fruits (orange square). (A) Score plot visualizing the grouping pattern of the samples based on the first two principal components. The ellipse defines Hotelling’s T2 confidence region (95%). (B) Loading plot of the PLS-DA components shows the contribution of each of the compounds (red square) to the first two principal components. Each treatment (black triangle) consists of six replicates.
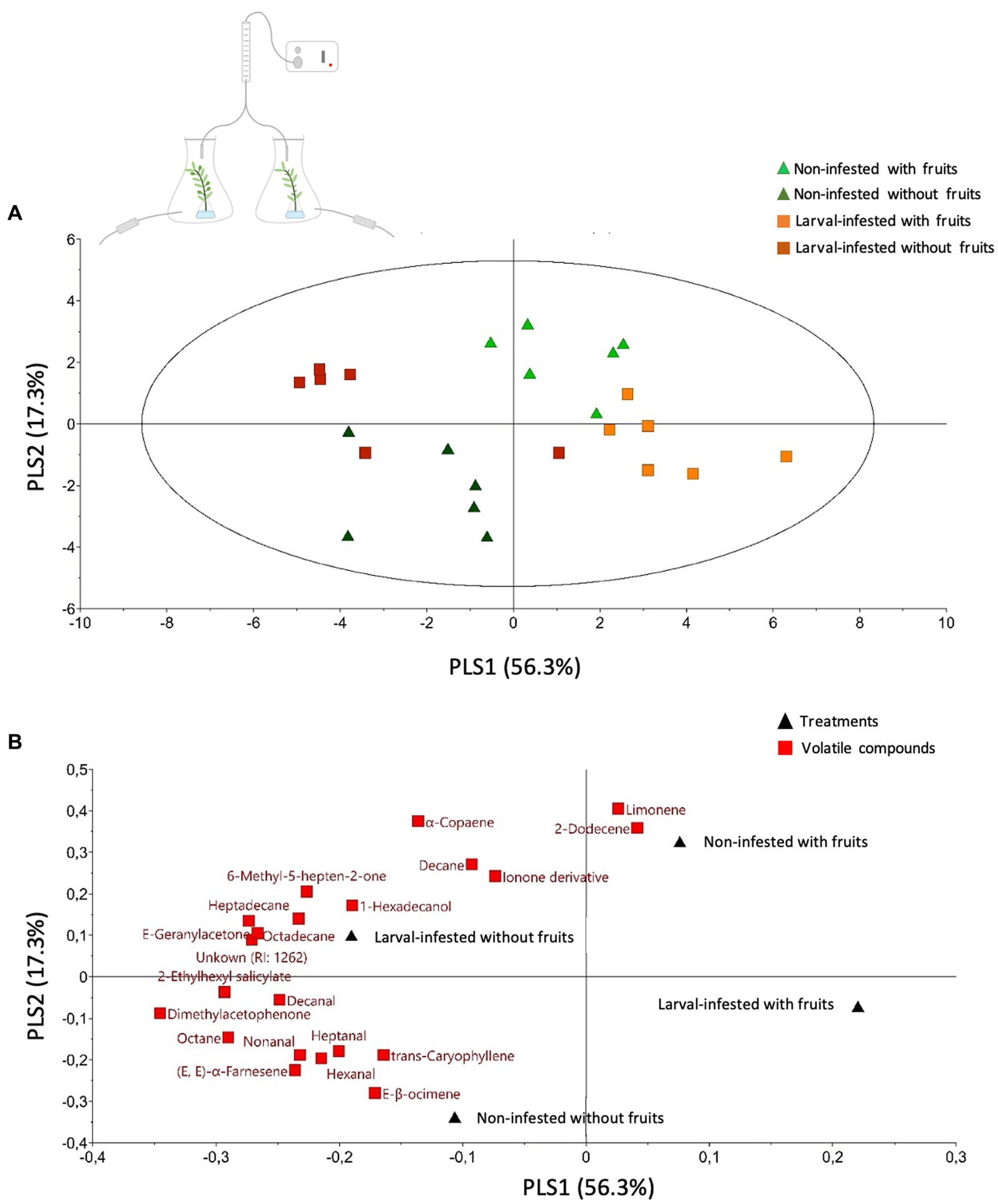
Figure 6. Volatile profile of the headspace of olive branches exposed to Bactrocera oleae larval-infestation or not exposed to herbivory at all. Projection to Latent Structures Discriminant Analysis (PLS-DA) of volatile compounds with VIP values >1 emitted from differently treated olive branches. Olive branches were either: non-infested branch with fruits (light green triangle), non-infested branch without fruits (fruits removed prior testing) (dark green triangle), larval-infested branch with fruits (orange square), and larval-infested branch without fruits (fruits removed prior testing) (brown-red square). In non-infested or larval-infested branches without the fruits, the fruits were removed from the branches prior to volatile collection. (A) Score plot visualizing the grouping pattern of the samples based on the first two principal components. The ellipse defines Hotelling’s T2 confidence region (95%). (B) Loading plot of the PLS-DA components shows the contribution of each of the compounds (red square) to the first two principal components. Each treatment (black triangle) consists of six replicates.
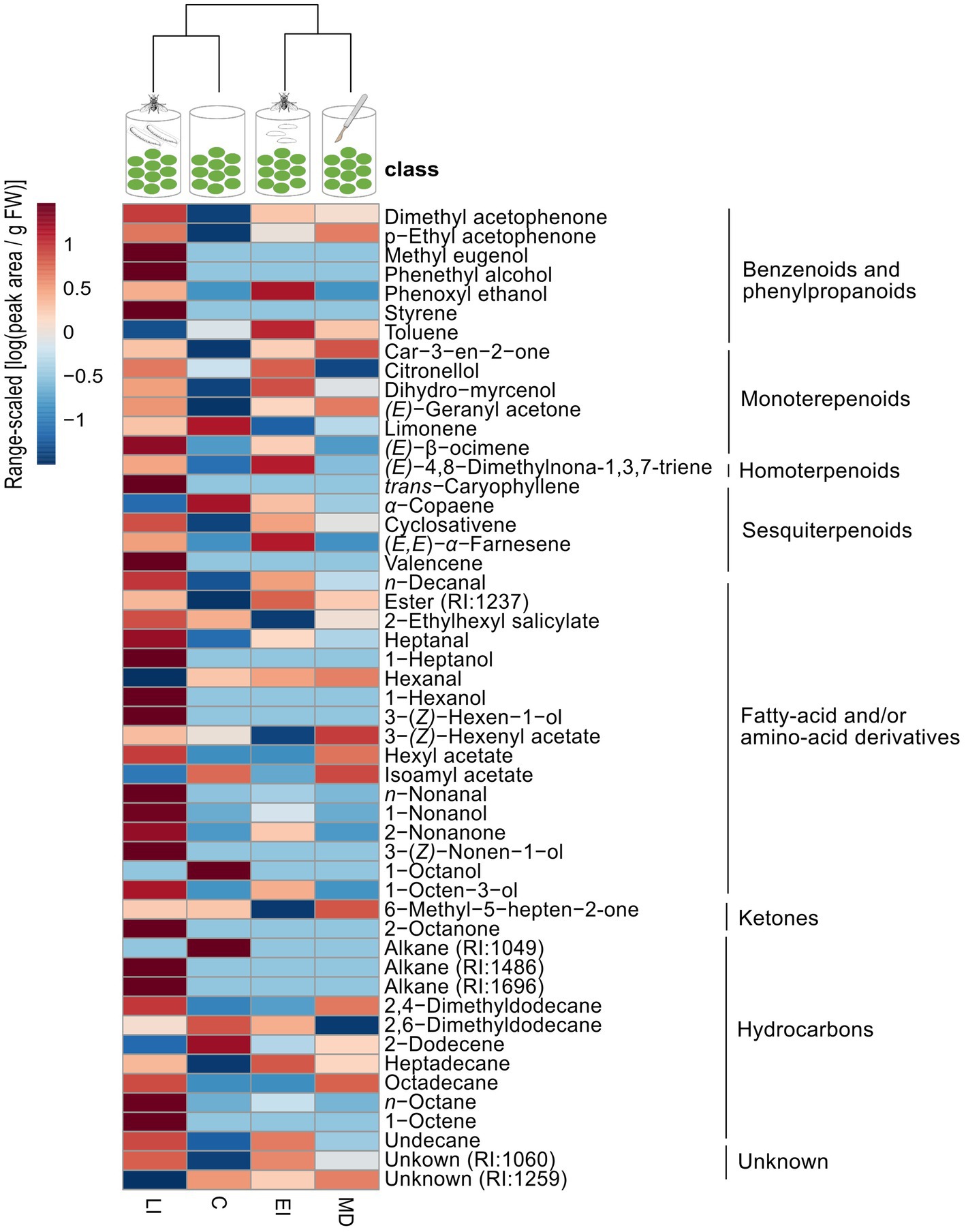
Figure 7. Dendrogram and heatmap of the emission of volatile organic compounds of olive fruits exposed to Bactrocera oleae infestation (egg- or larval-infestation) or not exposed to herbivory at all. Headspace volatiles were collected from non-infested (control) (C), mechanical-damaged (MD), Bactrocera oleae egg-infested (EI) or larval-infested (LI) fruits. Dendrogram clustering was performed using Ward’s clustering algorithm with Euclidean distances. For the heatmap, volatile emission (peak area/g FW) values for each compound were log-transformed, range-scaled and averaged per treatment. In the heatmap, the red color indicates high volatile emission, whereas blue color represents low volatile emission. Each treatment consists of six replicates.
Concerning the headspace volatiles from the olive fruits alone, a clustering analysis revealed that each treatment differently affected the volatile profile by altering the emission of specific compounds (Figure 7). In particular, we found that the volatile profiles of non-infested fruits and larval-infested fruits grouped together as well as those of the egg-infested fruits and mechanical-damaged fruits, suggesting that each of the groups have a similar volatile profile (Figure 7). The projection to latent structures discriminant analysis (PLS-DA) resulted in a model with the first two principal components explained 42.7 and 12.5% of the total variance, respectively, (Figure 5A). The first principal component clearly separates the larval-infested fruits from the other three treatments, suggesting that upon herbivore infestation by B. oleae larvae, the olive fruits respond with changes in the volatile emission. In particular, clustering analysis shows that forty-two compounds were emitted in higher amounts upon B. oleae herbivory, either after egg deposition or feeding damage, while twenty-two compounds were produced in higher amounts after mechanically damaging the fruits (Figure 7). From the forty-two compounds that were emitted in higher amounts upon B. oleae infestation, six compounds were detected only in both egg- and larval-infested fruits and thirteen compounds were specific to larval-infested fruits (Supplementary Table S1). Interestingly, from these nineteen compounds the most important ones, with VIP values >1, that contribute to the separation of the volatile profile between the egg- and larval-infested fruits from the other two treatments were: (E)-β-ocimene, (E,E)-α-farnesene, 2-nonanone, and heptanal (Table 2). Furthermore, the loading plot illustrates the contribution of each of the compounds to the differentiation of the volatile blend among treatments based on the VIP values of the compounds (Figure 5B). More specifically, the model identified nineteen compounds with VIP values >1 (Figure 5B; Table 2), suggesting that these compounds contributed the most to the differences observed between treatments. Interestingly, the volatile emission of eleven compounds out of the total nineteen shown in Table 2 was significantly induced in larval-infested fruits compared to the control treatment (Supplementary Table S1). Thus, the compounds that contributed the most to the separation of the VOC profile of the larval-infested fruits from the controls were: n-nonanal, n-octane, heptanal, dimethyl acetophenone, 1-nonanol, (E)-4,8-dimethylnona-1,3,7-triene, 6-methyl-5-hepten-2-one, 2-nonanone, p-ethyl acetophenone, (E)-β-ocimene, and (E,E)-α-farnesene. While the compounds that contributed the most to the separation of the VOC profile of the larval-infested fruits from all the other treatments were: n-nonanal, n-octane, heptanal, 1-nonanol, 2-nonanone, and (E)-β-ocimene (Table 2; Supplementary Table S1).
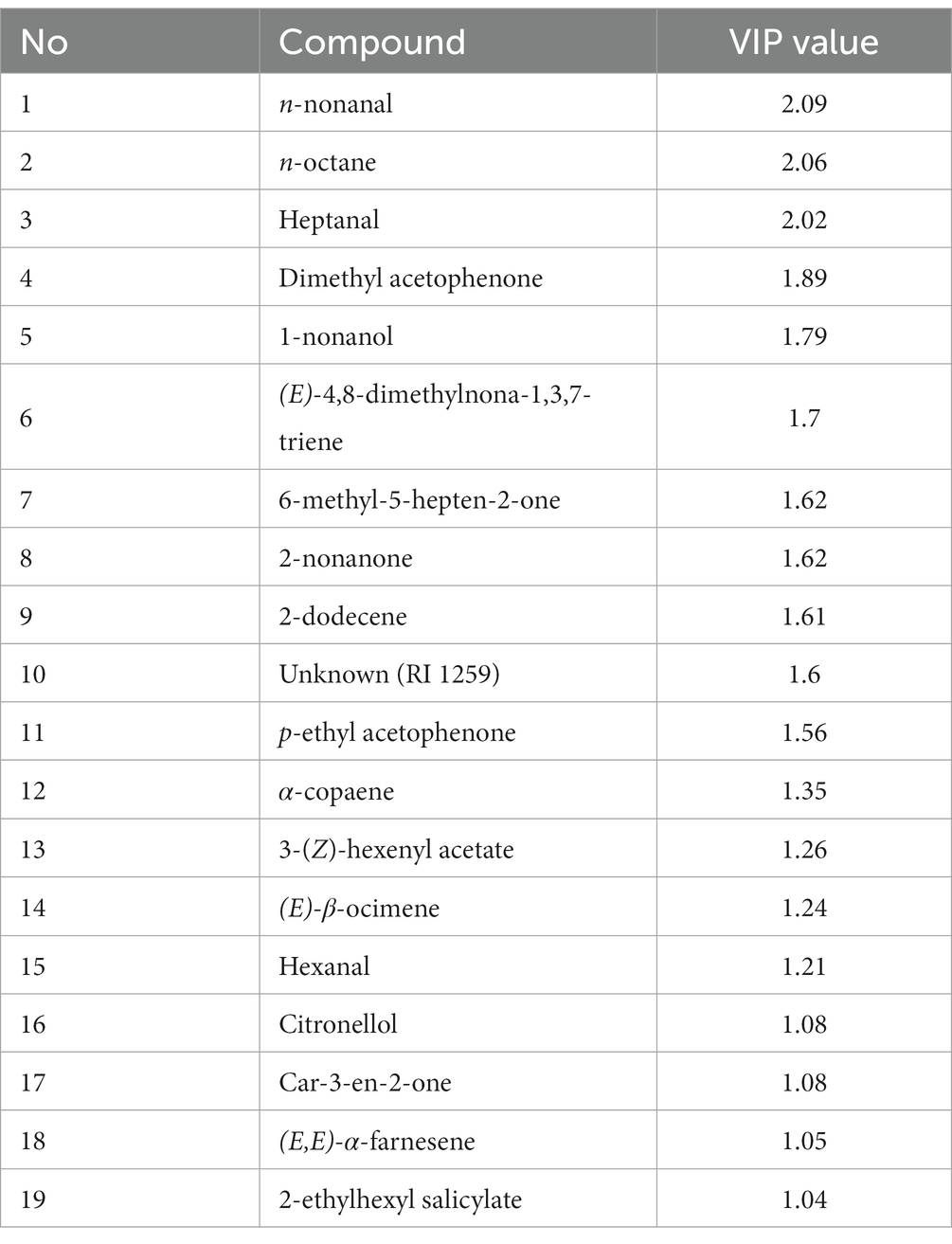
Table 2. Values of variable importance to the projection (VIP) of volatiles collected from olive fruits.
Regarding the headspace volatiles from olive branches, clustering analysis revealed that the volatile profiles of the branches with the non-infested fruits and the branches with the larval-infested fruits grouped together indicating that they have a similar volatile profile (Supplementary Figure S3). More specifically, the analysis shows that twelve compounds were emitted in higher amounts from branches with larval-infested fruits, and twenty-four compounds were emitted in higher amounts in branches with larval-infested fruits removed prior testing (Supplementary Figure S3). The PLS-DA shows that the first principal component, which explains 56.3% of the total variance, clearly separates the branches with the non-infested fruits and the branches with the larval-infested fruits from the branches with the non-infested fruits removed prior testing and the branches with the larval-infested fruits removed prior testing (Figure 6A). Moreover, PLS-DA shows that the second principal component, which explains 17.3% of the total variance, clearly separates (a) the branches with the non-infested fruits from the branches with the larval-infested fruits and (b) the branches with the non-infested fruits removed prior testing from the branches with the larval-infested fruits removed prior testing (Figure 6A). This could be evidence that the olive tree responds both locally and systemically to herbivore infestation by B. oleae larvae with changes in volatile emission, both in fruits and leaves. The loading plot shows the contribution of each of the compounds to the differentiation of the volatile blend between different treatments (Figure 6B). In detail, the model identified twenty-one compounds with VIP values >1 which suggests that these compounds contributed the most to the differences observed among the treatments (Figure 6B; Table 3). Notably, the volatile emission of two compounds out of the total twenty-one, namely hexadecanol and heptanal, was significantly induced in the branches with larval-infested fruits (Table 3). Another six compounds, different from the two previous, were emitted in higher amounts in branches with larval-infested fruits removed prior testing (Supplementary Table S2).
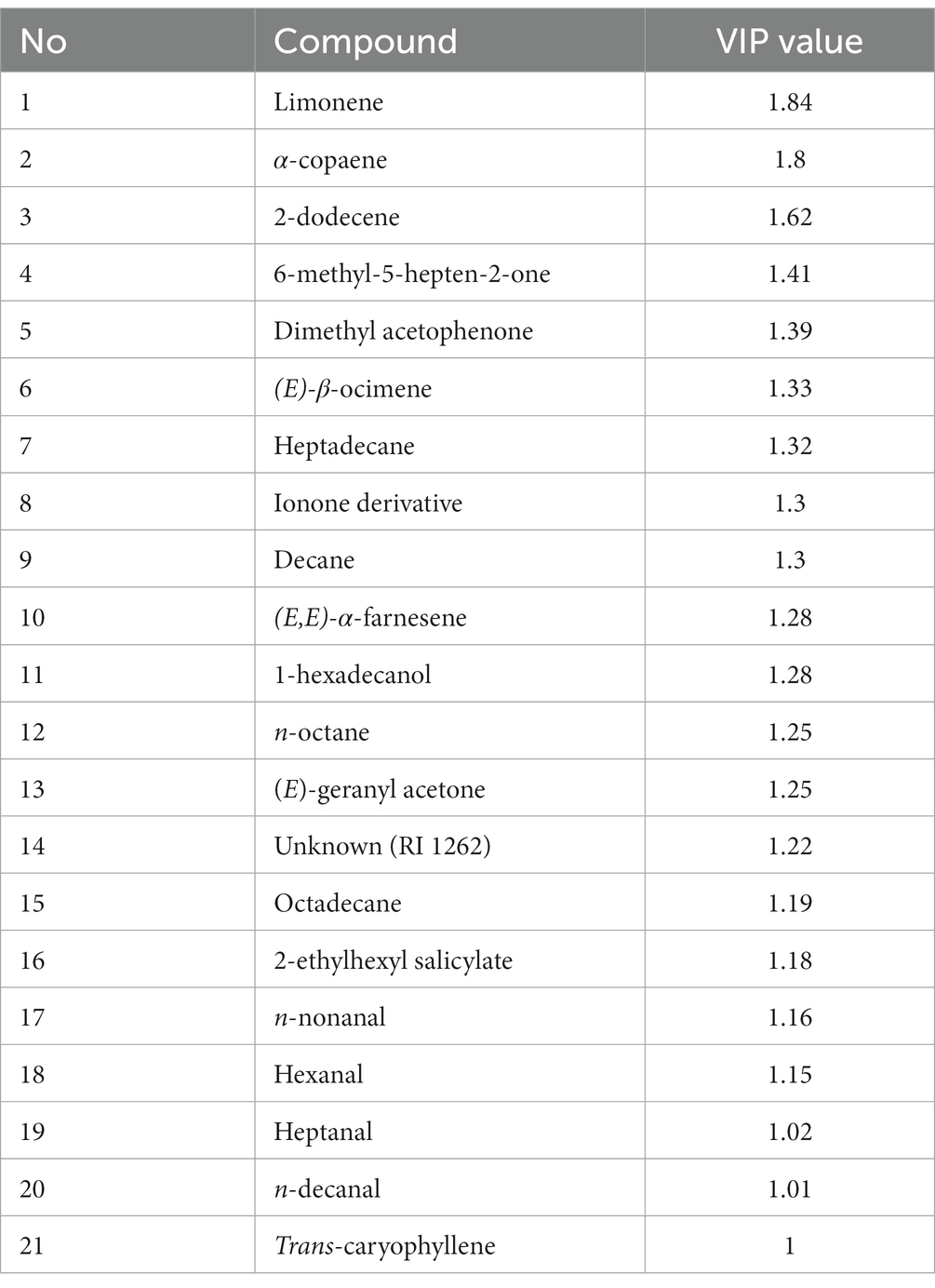
Table 3. Values of variable importance to the projection (VIP) of volatiles collected from olive branches.
Discussion
Overall, our study revealed that volatiles emitted by B. oleae larval-infested fruits and released by virgin and mated female B. oleae adults attracted P. concolor wasps. Volatile analysis of fruits alone showed that B. oleae feeding damage altered the volatile profile of infested fruits, suggesting that HIPVs may play a role in the attraction of P. concolor wasps. However, volatiles emitted from egg-infested fruits did not attract the wasps. Although, the chemical analysis showed that B. oleae egg infestation induces changes in the volatile blend of the egg-infested fruits compared to non-infested fruits, suggesting a role of the OIPVs in this system. For instance, OIPVs by B. oleae might be attractive to another parasitoid species than P. concolor parasitizing eggs or younger larvae of B. oleae. Furthermore, P. concolor wasps were neither attracted to volatiles emitted either from larval-infested branches nor to non-infested ones. This could be related to the quantity of plant material used for the bioassays, as we used about six times more fruits in the bioassays with the fruits alone. However, the chemical analysis showed that the volatile profile of larval-infested branches with the fruits and that of larval-infested branches without the fruits was different compared to their controls (non-infested branches), suggesting that B. oleae feeding damage elicits the release of HIPVs, both in fruits (larval-infested branches with the fruits treatment) and leaves (larval-infested branches without the fruits treatment). This suggests that the olive tree might respond both locally and systemically to infestation by B. oleae larvae. In addition, we found that P. concolor wasps were not able to discriminate between fruits with the cues left by the ovipositing female (potential HMPs) and washed fruits, suggesting that the HMPs are non-volatile compounds and only detectable by contact. Furthermore, the parasitoid was attracted to cues, likely sex pheromones, emitted by virgin and mated B. oleae females but were not attracted to cues released by virgin male and male B. oleae adults.
Effect of plant-derived cues on the wasps’ host location behavior
In the behavioral bioassays, P. concolor wasps exhibit a strong preference toward volatiles of larval-infested fruits (HIPVs) of the olive ‘Koroneiki’ cv. This is in accordance with a study by Giunti et al. (2016) that found that larval infestation by B. oleae induces HIPVs, in three different cultivars of ‘Frantoio’, ‘Leccino’, and ‘Arbequina’, and enhances their attractiveness to P. concolor. From previous studies it is known that artificial wounding and elicitors in insect oral secretion or oviduct secretions are needed to induce plant volatile changes (Meiners and Hilker, 2000; Acevedo et al., 2015). For instance, fatty acid amino acid conjugates, that found to be synthesized in vitro in the larval body of the fruit fly Drosophila melanogaster (Meigen) (Diptera, Drosophilidae) (Yoshinaga et al., 2014), are known to activate receptor-like kinases which then activate specific signaling transduction pathways that trigger the release of HIPVs. Meiners and Hilker (2000) found that a combination of artificial wounding on the leaves surface and oviduct secretion released together with eggs by the elf leaf beetle Xanthogaleruca luteola (Müller) (Coleoptera, Chrysomelidae), induce the release of OIPVs that are attractive to the egg parasitoid Oomyzus gallerucae (Fonscolombe) (Hymenoptera, Eulophidae). Similarly, we observed that P. concolor wasps were more attracted to volatiles from larval-infested fruits but not to mechanical-damaged fruits, which indicates that wounding alone does not elicit the induction of volatiles that could attract P. concolor wasps. Thereby, oral secretion and/or saliva from the B. oleae larvae likely contains an elicitor that induces the emission of HIPVs that serve as a synomone for P. concolor wasps. Moreover, we observed that the wasps were not attracted to the OIPVs from the egg-infested fruits. This suggests that B. oleae egg-infestation could not serve as an early herbivore cue for this parasitoid species. From the larval parasitoids’ point of view, the detection of the presence of the host eggs in fruits might be too early before the actual host stage is present as P. concolor wasps parasitize the 2nd and 3rd instar larvae of B. oleae. Furthermore, a previous study found that the egg-larval parasitoid Fopius arisanus (Sonan) (Hymenoptera, Braconidae) was attracted to volatiles from B. oleae egg-infested fruits (Calvitti et al., 2002), indicating that the OIPVs released after B. oleae egg deposition serve as synomones for this parasitoid wasp. This suggests that secretions from the eggs of the olive fruit fly, during oviposition, could have caused the changes in the emission of VOCs to attract F. arisanus wasps. Although OIPVs are well documented from several plant-host systems (Hilker and Fatouros, 2015), no previous knowledge is available from the olive system. Interestingly, chemical analysis of volatiles from egg-infested fruits revealed that, indeed, B. oleae egg infestation induces changes in VOC emission of the olive fruits and this could hint to a cue possibly used by other parasitoids of the olive fruit fly for host location such as the egg-larval parasitoid F. arisanus.
Effect of host-derived cues on wasps’ host location behavior
Our olfactory bioassays showed that P. concolor wasps were attracted to host cues emitted by virgin and mated B. oleae females and might use these cues as kairomones. The ability of parasitoids to eavesdrop on host cues to easily detect their host is well documented (Vet and Dicke, 1992; Fatouros et al., 2008; Benelli et al., 2014a). These chemical cues may be derived from the host either as long-range (i.e., host pheromones) or short-range/contact (e.g., from the wing scales, feces, cuticle) host cues (Vet and Dicke, 1992; Fatouros et al., 2008). The two major sex pheromone components of B. oleae are 1,7-Dioxaspiro[5.5]undecane produced by virgin female and young males, and (Z)-9-Tricosene exclusively produced by sexually mature males but not by young males (Benelli et al., 2014b). When testing the attraction of P. concolor wasps to the synthetic compounds 1,7-Dioxaspiro[5.5]undecane and (Z)-9-Tricosene the wasps were more attracted to (Z)-9-tricosene (Benelli et al., 2014b). However, in our bioassay experiments, we found that the wasps preferred the virgin and mated B. oleae females. The female-derived 1,7-Dioxaspiro[5.5]undecane is a compound with high volatility (Benelli et al., 2014a) and therefore it is unlikely to influence the wasps’ behavior as it is not stay long in the environment. Thus, we suggest that other compounds produced by virgin and mated B. oleae females, e.g., methyl dodecanoate, nonanal, or α-pinene (Benelli et al., 2014b; Canale et al., 2015), could play a role on the wasps’ attraction. Furthermore, we cannot exclude the possibility that other type of pheromones could influence the attraction of the wasps. Several Tephritidae, including B. oleae, appear to exhibit an oviposition marking behavior after egg deposition (Benelli et al., 2014b). Bactrocera oleae females have been observed to use their labellum to take out the secretions of the olive pulp from the oviposition puncture and spread it in the fruit surface which functions as oviposition deterrent by conspecifics (Benelli et al., 2014b). It has been reported that HMPs can be exploited by the parasitoids as kairomones to increase their searching efficiency and thus to find a suitable host for their offspring (Vet and Dicke, 1992; Nufio and Papaj, 2001). However, our data did not support this because when we offered fruits with and without cues left by the ovipositing female fly to P. concolor, the wasps were not able to discriminate between the two odor sources. We hypothesize that due to the non-volatility or low volatility of the cues (Nufio and Papaj, 2001), the wasps may be only able to detect them with their contact chemoreceptors. Moreover, cuticular hydrocarbons (CHCs) that are present on B. oleae (Galhoum, 2017; Scolari et al., 2021) could potentially been left as traces on the surface of the fruits. Egg parasitoids of true bugs are known to respond to footprints of their hosts mediated by the absorption of contact kairomone in the epicuticular wax layer of plants walked upon by the host bugs (Conti and Colazza, 2012). It would be interesting to further investigate this in the B. oleae - P. concolor relationship.
Changes in VOCs of olive fruits and branches by Bactrocera oleae infestation
In the present study, we found that larval-infested fruits exclusively emit several benzenoids/phenylpropanoids, sesquiterpenoids and green leaf volatiles, which have been reported to be induced upon herbivory and the volatiles emitted serve as attractants for predators and parasitoids (Kessler and Baldwin, 2002; Mumm and Dicke, 2010). Important qualitative and quantitative differences were detected in the volatile blend of egg- and larval-infested fruits compared to non-infested and mechanically damaged fruits of ‘Koroneiki cv. From the fifty-one identified compounds in treatments with olive fruits alone, forty-two compounds were significantly increased after egg- and larval-infestation. Six compounds were exclusively produced in egg- and larval-infested fruits and thirteen compounds were specific to larval-infested fruits. Two previous studies have reported qualitative and quantitative differences in the volatile blend of the B. oleae larval-infested fruits as well. More specifically, they found that specific volatile compounds significantly increased in the larval-infested fruits compared to non-infested fruits (Alagna et al., 2016; Giunti et al., 2016), while, specific volatile compounds exclusively emitted from the larval-infested fruits (Giunti et al., 2016). In the present study, we found volatile differences between the egg- and larval-infested fruits from the controls and mechanical damaged fruits. These differences were influenced the most by (E)-β-ocimene, (E,E)-α-farnesene, 2-nonanone, and heptanal. Compounds that were only found in the volatile blend of larval-infested fruits were: n-nonanal, n-octane, heptanal, 1-nonanol, 2-nonanone, and (E)-β-ocimene. All three cultivars that Giunti et al. (2016) examined had in common two volatile compounds, (E)-β-ocimene and (E,E)-α-farnesene, that had been induced upon larval infestation. Compounds that were also detected in our study. Additionally, it has been reported that P. concolor wasps were attracted to larval-infested fruits (Giunti et al., 2016). These findings suggest that (E)-β-ocimene and (E,E)-α-farnesene might play a key role during P. concolor host location. It seems that the presence of compounds found to be emitted only by B. oleae infested fruits or those that significantly increased might act as attractants to parasitoid P. concolor. Notably, in our study system we found many qualitative changes in VOC emission upon B. oleae infestation apart of changes in ratios of specific compounds. This is quite interesting, as not many systems report qualitative differences in VOC emission upon infestation, rather changes in VOC ratios. For instance, several studies in apple (Benelli et al., 2013), bean (Birkett et al., 2003), tomato (Milonas et al., 2019), and brassicaceous plants (Fatouros et al., 2012) have reported mainly changes in ratios of the volatile emission rather than qualitative differences in volatile compounds upon larval and egg-infestation.
Infochemical use for agricultural pest management
The knowledge obtained from our study could be used in biological pest control programs to improve parasitoid’s important biological control traits such as searching efficiency, host finding, host recognition, host location, host acceptance, and high parasitization rates (Leung et al., 2020). To improve the wasps’ effectiveness, selection of the appropriate wasp genotypes with the desired traits, through selective breeding, is needed. In such breeding programs, knowledge on intraspecific genetic variation of the desired traits of the candidate biocontrol agent is of great importance as it will define its effectiveness as a natural enemy of a specific insect pest (Lommen et al., 2017; Kruitwagen et al., 2018). To enhance the parasitoid’s efficiency, and thus to improve pest control, various approaches must be considered of such as the use of allelochemical-based tactics in order to enhance the efficiency of the biocontrol agent by manipulating their foraging behavior (Peri et al., 2018). This can be achieved, for instance, by using host kairomones (e.g., sex pheromones) and synthetic HIPVs (plant synomones) that are attractive to the targeted parasitoid to enhance the parasitism rates in the field (Peri et al., 2018), or by manipulating the HIPVs emission of the crops by applying HIPV inducers, such as phytohormones, to prime the plants’ defense response to herbivory (Turlings and Erb, 2018) in order to attract natural enemies.
Conclusions and future perspectives
In this study, we attempted to unravel the mechanisms that mediate O. europaea-B. oleae-P. concolor interactions and to better understand the role of olfactory cues emitted from the plant-host complex (i.e., OIPVs and HIPVs – plant synomones) and cues from the B. oleae (e.g., pheromones – host kairomones) exploited by P. concolor wasps. This was the first time that the OIPVs and chemical cues directly released by B. oleae flies were investigated in relation to parasitoid P. concolor and plant volatile emissions analyzed thoroughly. Our findings suggest that both cues from the plant and host might play an important role to P. concolor wasps during host location of the B. oleae. However, the cut plant material used in this study could affect the results, thus further investigation with intact plant material by conducting the experiments directly in the field should be considered. Moreover, further studies are needed in order to elucidate which specific chemical compounds could be used by P. concolor wasps. More specifically, the compounds that we found that may play an important role in P. concolor attraction, (E)-β-ocimene and (E,E)-α-farnesene, could be further tested by electroantennography and behavioral assays of the wasps to these compounds. Individual compound or a mixture of compounds in various ratios and combinations could be used to evaluate their electrophysiological activity (stimulation of olfactory sensilla of the wasps) on the wasp’s antenna. Similarly, chemical cues from virgin and mated female B. oleae flies could be extracted, identified, and tested in order to use them and to confirm their kairomonal effect. Finally, research is required to assess the potential of the application of these semiochemicals under field conditions.
Data availability statement
The original contributions presented in the study are publicly available. This data can be found here: Zenodo, doi: 10.5281/zenodo.7987959.
Author contributions
GB, EK, BZ, BP, and NF planned and designed the study. GB collected the data. GB, EA, PM, and AP conducted the chemical analyses. GB and EA analyzed the data. GB and NF prepared and wrote the first draft. All authors read and drafted the final version of the manuscript.
Funding
This research was financially supported by the Ecology Fund Grant of the Royal Netherlands Academy of Arts and Sciences (KNAW Fonds Ecologie 2020 – KNAWWF/DA/973/Eco2006) and by the LIFE program of the European Union, project LIFE IGIC - “Improvement of green infrastructure (GI) in agroecosystems: reconnecting natural areas by countering habitat fragmentation” (LIFE16 NAT/GR/000575).
Acknowledgments
The authors thank Antonia Vogiatzaki-Bagkeri and the intern students of the Laboratory of Olive & Agroecological Production Systems of the Hellenic Mediterranean University for their help regarding the insects rearing and the collection of plant material, and Georgios Partsinevelos from the Laboratory of Biological Control of Benaki Phytopathological Institute for providing us with field collected plant material during the trials of volatile collection.
Conflict of interest
The authors declare that the research was conducted in the absence of any commercial or financial relationships that could be construed as a potential conflict of interest.
Publisher’s note
All claims expressed in this article are solely those of the authors and do not necessarily represent those of their affiliated organizations, or those of the publisher, the editors and the reviewers. Any product that may be evaluated in this article, or claim that may be made by its manufacturer, is not guaranteed or endorsed by the publisher.
Supplementary material
The Supplementary material for this article can be found online at: https://www.frontiersin.org/articles/10.3389/fevo.2023.1100983/full#supplementary-material
Abbreviations
OIPVs, oviposition induced plant volatiles; HIPVs, herbivore induced plant volatiles; VOC, volatile organic compounds; HMP, host marking pheromone; VIP, variable importance for the projection; RI, retention index; CHCs, cuticular hydrocarbons.
References
Acevedo, F. E., Rivera-Vega, L. J., Chung, S. H., Ray, S., and Felton, G. W. (2015). Cues from chewing insects – the intersection of DAMPs, HAMPs, MAMPs and effectors. Curr. Opin. Plant Biol. 26, 80–86. doi: 10.1016/j.pbi.2015.05.029
Adams, R. P. (2007). Identification of essential oil components by gas chromatography/mass spectroscopy. Carol Stream, IL: Allured Pub Corp.
Alagna, F., Kallenbach, M., Pompa, A., de Marchis, F., Rao, R., Baldwin, I. T., et al. (2016). Olive fruits infested with olive fly larvae respond with an ethylene burst and the emission of specific volatiles. J. Integr. Plant Biol. 58, 413–425. doi: 10.1111/jipb.12343
Amvrazi, E. G., and Albanis, T. A. (2009). Pesticide residue assessment in different types of olive oil and preliminary exposure assessment of Greek consumers to the pesticide residues detected. Food Chem. 113, 253–261. doi: 10.1016/j.foodchem.2008.06.073
Anastasaki, E., Drizou, F., and Milonas, P. G. (2018). Electrophysiological and oviposition responses of Tuta absoluta females to herbivore-induced volatiles in tomato plants. J. Chem. Ecol. 44, 288–298. doi: 10.1007/s10886-018-0929-1
Ayelo, P. M., Pirk, C. W. W., Yusuf, A. A., Chailleux, A., Mohamed, S. A., and Deletre, E. (2021). Exploring the Kairomone-based foraging behaviour of natural enemies to enhance biological control: a review. Front. Ecol. Evol. 9:974. doi: 10.3389/fevo.2021.641974
Benelli, G., Carpita, A., Simoncini, S., Raspi, A., and Canale, A. (2014a). For sex and more: attraction of the tephritid parasitoid Psyttalia concolor (Hymenoptera: Braconidae) to male sex pheromone of the olive fruit fly, Bactrocera oleae. J. Pest. Sci. 87, 449–457. doi: 10.1007/s10340-014-0595-1
Benelli, G., Daane, K. M., Canale, A., Niu, C. Y., Messing, R. H., and Vargas, R. I. (2014b). Sexual communication and related behaviours in Tephritidae: current knowledge and potential applications for integrated Pest management. J. Pest. Sci. 87, 385–405. doi: 10.1007/s10340-014-0577-3
Benelli, G., Revadi, S., Carpita, A., Giunti, G., Raspi, A., Anfora, G., et al. (2013). Behavioral and electrophysiological responses of the parasitic wasp Psyttalia concolor (Szépligeti) (Hymenoptera: Braconidae) to Ceratitis capitata-induced fruit volatiles. Biol. Control 64, 116–124. doi: 10.1016/j.biocontrol.2012.10.010
Birkett, M. A., Chamberlaln, K., Guerrieri, E., Pickett, J. A., Wadhams, L. J., and Yasuda, T. (2003). Volatiles from whitefly-infested plants elicit a host-locating response in the parasitoid, Encarsia formosa. J. Chem. Ecol. 29, 1589–1600. doi: 10.1023/A:1024218729423
Calvitti, M., Antonelli, M., Moretti, R., and Bautista, R. C. (2002). Oviposition response and development of the egg-pupal parasitoid Fopius arisanus on Bactrocera oleae, a tephritid fruit fly pest of olive in the Mediterranean basin. Entomol. Exp. Appl. 102, 65–73. doi: 10.1046/j.1570-7458.2002.00925.x
Calvo-Agudo, M., González-Cabrera, J., Sadutto, D., Picó, Y., Urbaneja, A., Dicke, M., et al. (2020). IPM-recommended insecticides harm beneficial insects through contaminated honeydew. Environ. Pollut. 267:581. doi: 10.1016/j.envpol.2020.115581
Canale, A., Benelli, G., Germinara, G. S., Fusini, G., Romano, D., Rapalini, F., et al. (2015). Behavioural and electrophysiological responses to overlooked female pheromone components in the olive fruit fly, Bactrocera oleae (Diptera: Tephritidae). Chemoecology 25, 147–157. doi: 10.1007/s00049-014-0183-0
Conti, E., and Colazza, S. (2012). Chemical ecology of egg parasitoids associated with true bugs. Psyche A J. Entomol. 2012, 1–11. doi: 10.1155/2012/651015
Daane, K. M., and Johnson, M. W. (2010). Olive fruit Fly: managing an ancient pest in modern times. Annu. Rev. Entomol. 55, 151–169. doi: 10.1146/annurev.ento.54.110807.090553
Dicke, M. (2009). Behavioural and community ecology of plants that cry for help. Plant Cell Environ. 32, 654–665. doi: 10.1111/j.1365-3040.2008.01913.x
Dicke, M., and Vet, L. E. M. (1999). “Plant-carnivore interactions: evolutionary and ecological consequences for plant, herbivore and carnivore” in Herbivores: Between plants and predators. eds. H. Olff, V. K. Brown, and R. H. Drent (Hoboken, NJ: Blackwell Science Ltd), 483–520.
Fatouros, N. E., Dicke, M., Mumm, R., Meiners, T., and Hilker, M. (2008). Foraging behavior of egg parasitoids exploiting chemical information. Behav. Ecol. 19, 677–689. doi: 10.1093/beheco/arn011
Fatouros, N. E., Lucas-Barbosa, D., Weldegergis, B. T., Pashalidou, F. G., van Loon, J. J. A., Dicke, M., et al. (2012). Plant volatiles induced by herbivore egg deposition affect insects of different trophic levels. PLoS One 7:3607. doi: 10.1371/journal.pone.0043607
Galhoum, A. M. M. (2017). Taxonomic studies on two Tephritid species (order: Diptera), Bactrocera Oleae and B. zonata, using the cuticular hydrocarbons profile. Al Azhar Bullet. Sci. 28, 45–54. doi: 10.21608/absb.2017.8166
Giunti, G., Benelli, G., Conte, G., Mele, M., Caruso, G., Gucci, R., et al. (2016). VOCs-mediated location of olive fly larvae by the braconid parasitoid Psyttalia concolor: a multivariate comparison among VOC bouquets from three olive cultivars. Biomed. Res. Int. 2016:7827615. doi: 10.1155/2016/7827615
Godfray, H. C. J. (1994). Parasitoids: Behavioral and evolutionary ecology, 67. Princeton, NJ: Princeton University Press.
Greenberg, L. O., Huigens, M. E., Groot, A. T., Cusumano, A., and Fatouros, N. E. (2023). Finding an egg in a haystack: variation in chemical cue use by egg parasitoids of herbivorous insects. Curr. Opin. Insect Sci. 55:1002. doi: 10.1016/j.cois.2022.101002
Guzmán, E., Baeten, V., Pierna, J. A. F., and García-Mesa, J. A. (2015). Determination of the olive maturity index of intact fruits using image analysis. J. Food Sci. Technol. 52, 1462–1470. doi: 10.1007/s13197-013-1123-7
Hilker, M., and Fatouros, N. E. (2015). Plant responses to insect egg deposition. Annu. Rev. Entomol. 60, 493–515. doi: 10.1146/annurev-ento-010814-020620
Jayanthi, P. D. K., Raghava, T., and Kempraj, V. (2020). “Functional diversity of infochemicals in Agri-ecological networks” in Innovative Pest management approaches for the 21st century. ed. A. Chakravarthy (Singapore: Springer Singapore), 187–208.
Kampouraki, A., Stavrakaki, M., Karataraki, A., Katsikogiannis, G., Pitika, E., Varikou, K., et al. (2018). Recent evolution and operational impact of insecticide resistance in olive fruit fly Bactrocera oleae populations from Greece. J. Pest. Sci. 91, 1429–1439. doi: 10.1007/s10340-018-1007-8
Kessler, A., and Baldwin, I. T. (2002). Plant responses to insect herbivory: the emerging molecular analysis. Annu. Rev. Plant Biol. 53, 299–328. doi: 10.1146/annurev.arplant.53.100301.135207
Kokkari, A. I., Milonas, P. G., Anastasaki, E., Floros, G. D., Kouloussis, N. A., and Koveos, D. S. (2021). Determination of volatile substances in olives and their effect on reproduction of the olive fruit fly. J. Appl. Entomol. 145, 841–855. doi: 10.1111/jen.12929
Kruitwagen, A., Beukeboom, L. W., and Wertheim, B. (2018). Optimization of native biocontrol agents, with parasitoids of the invasive pest Drosophila suzukii as an example. Evol. Appl. 11, 1473–1497. doi: 10.1111/eva.12648
Leung, K., Ras, E., Ferguson, K. B., Ariëns, S., Babendreier, D., Bijma, P., et al. (2020). Next-generation biological control: the need for integrating genetics and genomics. Biol. Rev. 95, 1838–1854. doi: 10.1111/brv.12641
Lommen, S. T. E., de Jong, P. W., and Pannebakker, B. A. (2017). It is time to bridge the gap between exploring and exploiting: prospects for utilizing intraspecific genetic variation to optimize arthropods for augmentative pest control – a review. Entomol. Exp. Appl. 162, 108–123. doi: 10.1111/eea.12510
Malheiro, R., Casal, S., Cunha, S. C., Baptista, P., and Pereira, J. A. (2015). Olive volatiles from Portuguese cultivars Cobrançosa, Madural and Verdeal Transmontana: role in oviposition preference of Bactrocera oleae (Rossi) (Diptera: Tephritidae). PLoS One 10:e0125070. doi: 10.1371/journal.pone.0125070
Malheiro, R., Casal, S., Cunha, S. C., Baptista, P., and Pereira, J. A. (2016). Identification of leaf volatiles from olive (Olea europaea) and their possible role in the ovipositional preferences of olive fly, Bactrocera oleae (Rossi) (Diptera: Tephritidae). Phytochemistry 121, 11–19. doi: 10.1016/j.phytochem.2015.10.005
Mattiacci, L., Dicke, M., and Posthumus, M. A. (1994). Induction of parasitoid attracting synomone in Brussels sprouts plants by feeding of Pierris brassicae larvae: role of mechenical damage and herbivore elicitor. Chem. Ecol. 20, 2229–2247. doi: 10.1007/BF02033199
Meiners, T., and Hilker, M. (2000). Induction of plant synomones by oviposition of a phytophagous insect. J. Chem. Ecol. 26, 221–232. doi: 10.1023/A:1005453830961
Milonas, P. G., Anastasaki, E., and Partsinevelos, G. (2019). Oviposition-induced volatiles affect electrophysiological and behavioral responses of egg parasitoids. Insects 10:437. doi: 10.3390/insects10120437
Milonas, P., Mazomenos, B. E., and Konstantopoulou, M. A. (2009). Kairomonal effect of sex pheromone components of two lepidopteran olive pests on Trichogramma wasps. Insect Sci. 16, 131–136. doi: 10.1111/j.1744-7917.2009.00264.x
Mumm, R., and Dicke, M. (2010). Variation in natural plant products and the attraction of bodyguards involved in indirect plant defense. Can. J. Zool. 88, 698–724. doi: 10.1139/Z10-029
Nufio, C. R., and Papaj, D. R. (2001). Host marking behavior in phytophagous insects and parasitoids. Entomol. Exp. Appl. 99, 273–293. doi: 10.1046/j.1570-7458.2001.00827.x
Pashalidou, F. G., Gols, R., Berkhout, B. W., Weldegergis, B. T., van Loon, J. J. A., Dicke, M., et al. (2015). To be in time: egg deposition enhances plant-mediated detection of young caterpillars by parasitoids. Oecologia 177, 477–486. doi: 10.1007/s00442-014-3098-0
Peri, E., Moujahed, R., Wajnberg, E., and Colazza, S. (2018). “Applied chemical ecology to enhance insect parasitoid efficacy in the biological control of crop pests” in Chemical ecology of insects. Applications and associations with plants and microbes. ed. T. Jun. 1st ed (Boca Raton, FL: CRC Press), 234–267.
Ponzio, C., Cascone, P., Cusumano, A., Weldegergis, B. T., Fatouros, N. E., Guerrieri, E., et al. (2016). Volatile-mediated foraging behaviour of three parasitoid species under conditions of dual insect herbivore attack. Anim. Behav. 111, 197–206. doi: 10.1016/j.anbehav.2015.10.024
Scarpati, M. L., Scalzo, R. L., and Vita, G. (1993). Olea europaea volatiles attractive and repellent to the olive fruit fly (Dacus oleae, Gmelin). J. Chem. Ecol. 19, 881–891. doi: 10.1007/BF00985017
Scolari, F., Valerio, F., Benelli, G., Papadopoulos, N. T., and Vaníčková, L. (2021). Tephritid fruit fly semiochemicals: current knowledge and future perspectives. Insects 12:408. doi: 10.3390/insects12050408
Sime, K. R., Daane, K. M., Messing, R. H., and Johnson, M. W. (2006). Comparison of two laboratory cultures of Psyttalia concolor (Hymenoptera: Braconidae), as a parasitoid of the olive fruit fly. Biol. Control 39, 248–255. doi: 10.1016/j.biocontrol.2006.06.007
Tamiru, A., Bruce, T. J. A., Woodcock, C. M., Caulfield, J. C., Midega, C. A. O., Ogol, C. K. P. O., et al. (2011). Maize landraces recruit egg and larval parasitoids in response to egg deposition by a herbivore. Ecol. Lett. 14, 1075–1083. doi: 10.1111/j.1461-0248.2011.01674.x
Turlings, T. C. J., and Erb, M. (2018). Tritrophic interactions mediated by herbivore-induced plant volatiles: mechanisms, ecological relevance, and application potential. Annu. Rev. Entomol. 63, 433–452. doi: 10.1146/annurev-ento-020117-043507
van Alphen, J. J. M., and Vet, L. E. M. (1986). “An evolutionary approach to host finding and selection” in Insect parasitoids. eds. J. K. Waage and D. J. Greathead (Cambridge, MA: Academic Press), 23–61.
van Lenteren, J. C. (2012). The state of commercial augmentative biological control: plenty of natural enemies, but a frustrating lack of uptake. BioControl 57, 1–20. doi: 10.1007/s10526-011-9395-1
Vet, L. E. M., and Dicke, M. (1992). Ecology of info chemical use by natural enemies in a tritrophic context. Annu. Rev. Entomol. 37, 141–172. doi: 10.1146/annurev.en.37.010192.001041
Vet, L. E. M., Wackers, F. L., and Dicke, M. (1991). How to hunt for hiding hosts: the reliability-detectability problem in foraging parasitoids. Netherlands J. Zool. 41, 202–213.
Vinson, S. B. (1998). The general host selection behavior of parasitoid Hymenoptera and a comparison of initial strategies utilized by Larvaphagous and Oophagous species. Biol. Control 11, 79–96. doi: 10.1006/bcon.1997.0601
Keywords: plant-derived synomones, host-derived kairomones, tritrophic interactions, foraging behavior, indirect plant resistance, semiochemicals, biological control
Citation: Bogka G, Anastasaki E, Milonas PG, Psoma A, Kabourakis EM, Zwaan BJ, Pannebakker BA and Fatouros NE (2023) Chemical cues involved in the host foraging behavior of Psyttalia concolor wasps to locate the olive fruit fly Bactrocera oleae. Front. Ecol. Evol. 11:1100983. doi: 10.3389/fevo.2023.1100983
Edited by:
Thomas Schmitt, Julius Maximilian University of Würzburg, GermanyReviewed by:
Salvatore Guarino, Institute of Biosciences and Bioresources - CNR, ItalyVictoria Moris, University of Hull, United Kingdom
Copyright © 2023 Bogka, Anastasaki, Milonas, Psoma, Kabourakis, Zwaan, Pannebakker and Fatouros. This is an open-access article distributed under the terms of the Creative Commons Attribution License (CC BY). The use, distribution or reproduction in other forums is permitted, provided the original author(s) and the copyright owner(s) are credited and that the original publication in this journal is cited, in accordance with accepted academic practice. No use, distribution or reproduction is permitted which does not comply with these terms.
*Correspondence: Nina E. Fatouros, nina.fatouros@wur.nl