- 1Institute for Marine and Antarctic Studies, University of Tasmania, Hobart, TAS, Australia
- 2Centre d’Etudes Biologiques de Chizé, Centre National de la Recherche Scientifique-La Rochelle Université, UMR, Villiers en Bois, France
- 3Institut Pluridisciplinaire Hubert Curien, CNRS-UMR, Strasbourg, France
Introduction: In Antarctica, there is growing concern about the potential effect of anthropogenic activities (i.e., tourism, research) on wildlife, especially since human activities are developing at an unprecedented rate. Although guidelines exist to mitigate negative impacts, fundamental data are currently lacking to reliably assess impacts. Physiological tools, such as circulating corticosterone levels, appear promising to assess the potential impact of human disturbance on Antarctic vertebrates.
Methods: In this study, we compared the body condition, and the physiological sensitivity to stress (i.e., basal and stress-induced corticosterone level) of adult and chick Adélie penguins between a disturbed and an undisturbed area (i.e., 2 colonies located in the middle of a research station exposed to intense human activities and 2 colonies located on protected islands with minimal human disturbance).
Results: We did not find any significant impact of human activities on body condition and corticosterone levels in adults (incubating adults, brooding adults). In chicks, there were significant inter-colony variations in stress-induced corticosterone levels. Specifically, the chicks from the disturbed colonies tended to have higher stress-induced corticosterone levels than the chicks from the protected areas although this difference between areas was not significant. In addition, and independently of human disturbance we also found significant differences in adult body condition, and chick corticosterone level between colonies.
Discussion: Overall, our study suggests that this species is not dramatically impacted by human activities, at least when humans and penguins have cohabited for several decades. Our results support therefore the idea that this species is likely to be tolerant to human disturbance and this corroborates with the persistence of Adélie penguin colonies in the middle of the research station. However, our results also suggest that chicks might be more sensitive to human disturbance than adults and might therefore potentially suffer from human disturbance. Our study also suggests that specific individual and environmental variables outweigh the potential minor impact of human disturbance on these variables. Combining corticosterone with complementary stress-related physiological markers, such as heart rate, may strengthen further studies examining whether human disturbance may have subtle detrimental impacts on individuals.
1. Introduction
In recent years, there has been increasing concern about the potential effect of anthropogenic activities on Antarctic wildlife (Tin et al., 2014). Antarctica is often perceived as a pristine wilderness area unaffected by human activities. However, tourism activities have developed to an unprecedent scale over the last decade (IAATO ATCM Information Papers, 2022). Similarly, research activities have increased, including the creation of several new stations on the Antarctic continent (Chown et al., 2012). Importantly, human activities are predicted to continue to grow in the following decades (Woehler et al., 2014; Bender et al., 2016). Therefore, there is an urgent need to propose management policies regulating anthropogenic activities, to develop sustainable Antarctic tourism, and to limit the impact of anthropogenic activities on Antarctic wildlife (Tin et al., 2014; Coetzee et al., 2017; Pertierra et al., 2017).
Despite this urgent need, we currently lack basic data to reliably assess the impact of anthropogenic activities on wildlife (Tin et al., 2014). Surprisingly few studies have examined the impact of anthropogenic activities on wild vertebrate species such as seals and seabirds (reviewed in Coetzee and Chown, 2016). However, these species may be particularly vulnerable to anthropogenic activities in the Antarctic for several reasons. Firstly, these species have not coevolved with human presence or terrestrial predators and their behavioral and physiological systems have not necessarily been selected to cope with terrestrial disturbance. Secondly, seabirds and seals are iconic species, often considered a “must-see” during tourist expeditions (Tin et al., 2016). Among Antarctic species, they are therefore probably the most exposed to tourist activities. Finally, these species often breed on rocky coastlines and islands, which are also most suitable to establish research stations. Therefore, they may be directly impacted by the settlement of new Antarctic stations (Micol and Jouventin, 2001; Woehler et al., 2014).
Assessing the impact of human presence and activities on Antarctic vertebrates can be challenging. Having not coevolved with humans, they are often “tame” and do not necessarily flee in response to human presence. Therefore, they are often considered relatively insensitive to human presence. Coetzee and Chown (2016) recently reported in a meta-analysis that anthropogenic activities do not have consistent and significant effects on seabird and seal behavior. However, despite this apparent insensitivity there is increasing evidence that these species may perceive anthropogenic activities as a threat or a stressor even if they do not behaviorally react. For example, human presence was associated with an increase in heart rate in several sub-Antarctic and Antarctic seabird species without behavioral signs of stress being evident (Weimerskirch et al., 2002; de Villiers et al., 2006; Ellenberg et al., 2013).
Assessing the impact of anthropogenic activities on wild vertebrates warrants the use of alternative and additional tools, such as physiological parameters, which can reflect individual level of stress better than behavioral components (i.e., the concept of “Conservation Physiology”, see Walker et al., 2005; Cockrem et al., 2006; Wikelski and Cooke, 2006). For example, there is growing interest in the use of “stress hormones” in vertebrates to assess the influence of biotic and abiotic factors (Busch and Hayward, 2009; Dickens and Romero, 2013). In response to a stressor the Hypothalamus-Pituitary-Adrenals (HPA) axis is activated, resulting in a rapid release of glucocorticoids into the bloodstream (Wingfield et al., 1998). This increase in circulating glucocorticoid levels mediates important physiological and behavioral changes, which aims to restore homeostasis (McEwen and Wingfield, 2003; Romero et al., 2009). Although circulating glucocorticoid levels generally return to baseline levels when the stressor ends, they can theoretically stay elevated during a prolonged period if homeostasis is not restored. Consequently, ecophysiologists have used baseline glucocorticoids levels to test whether a given individual, or population, is “stressed” (reviewed in Busch and Hayward, 2009; Dickens and Romero, 2013). In addition, the sensitivity of the HPA axis to a standardized restraint stress protocol (i.e., stress-induced glucocorticoid levels) is also used to test individual responses to a stressor (Wingfield et al., 1992; Angelier and Wingfield, 2013; Dickens and Romero, 2013). This stress-induced protocol allows us to understand whether a given individual, or population, could become habituated (low stress-induced glucocorticoid levels) or sensitized (high stress-induced glucocorticoid levels) to stress in a context of chronic anthropogenic disturbance (Angelier and Wingfield, 2013). Therefore, using elevated circulating glucocorticoid levels as a stress indicator has been proposed and successfully implemented as a physiological tool to monitor anthropogenic disturbance effects on wild vertebrates (Walker et al., 2005; Cockrem et al., 2006; Wikelski and Cooke, 2006; Busch and Hayward, 2009; but see Dickens and Romero, 2013).
We sought to assess the impact of anthropogenic activities on an Antarctic bird species widely used as an “ecosystem sentinel”, the Adélie penguin (Pygoscelis adeliae). To do so we examined individual body condition and corticosterone levels (the main avian glucocorticoid) among penguin colonies located both within protected areas and in the close vicinity of a research station. In the protected areas human presence is absolutely limited to research purpose and access is restricted to a small number of scientists per breeding season. In contrast, other colonies are located in the middle of a research station with daily anthropogenic activities including vehicles, frequent human presence, maintenance work and the use of helicopters for transporting cargo and people.
If anthropogenic activities have a detrimental effect on Adélie penguins, we predict that penguins from the disturbed area will have a lower body condition (prediction 1a), higher baseline corticosterone levels (prediction 1b), and higher stress-induced corticosterone levels (prediction 1c) if they become hyper-sensitive to stress, relative to penguins from undisturbed areas. Alternatively, if Adélie penguins have habituated to anthropogenic activities, we predict that penguins from the disturbed area will have a similar body condition (prediction 2a), similar baseline corticosterone levels (prediction 2b), and lower stress-induced corticosterone levels (prediction 2c), relative to penguins from protected areas. Finally, we predict that this effect of anthropogenic activities on corticosterone levels may be exacerbated during specific stages (i.e., early life) during which penguins may be more sensitive to disturbance (prediction 3).
2. Methods
2.1. Adélie penguins and study sites
This study was carried out at Pointe Géologie (66°400’S, 140°010′E) in Adélie Land, Antarctica, between November 2015 and February 2016. Breeding Adélie penguins were captured during the incubation stage (November 24 to December 4 for males; December 8 to December 11 for females) and the early chick-rearing period (December 31 to January 7). During these stages both parents alternate foraging trips at sea with incubating or brooding periods at the colony. A total of 127 incubating (78 males and 49 females) and 92 chick-rearing (43 males and 50 females) penguins were captured. In addition, 54 chicks (27 males and 27 females) were captured after thermal emancipation when they are left alone at the colony (end of January-beginning of February).
All penguins were captured from four colonies: two located within the station area considered as a “disturbed area”, each hereafter termed a “station colony”; and two were located on protected islands considered as “undisturbed area”, each hereafter termed a “protected colony” (Figure 1). The station colonies (station colony 1 and 2) are located in the middle of the Dumont d’Urville research station – here, birds are breeding nearby to the helicopter landing platform (10 to 50 m proximity) and are located within 1–2 m from paths or roads frequently used by people and vehicles. Noisy human activities related to the daily station operations are thus very frequent, however there is no tourism in the area. In contrast, the protected colonies (Bernard Island, protected colony 1; Lamarck Island, protected colony 2) both belong within an Antarctic Specially Protected Area (ASPA) where human activities are strictly limited to scientific research purposes. There are no paths nor roads, vehicles or recreational activities are prohibited, and helicopters cannot overfly these islands. The access permitted to scientists is restricted to small groups (2–3 persons) only a few times per breeding season. Sample size was evenly distributed among colonies (in the order of station colony 1, 2 and protected colony 1, 2; sample size is: incubating adults: 30, 33, 32, 31; rearing adults: 23, 23, 23, 23; chicks: 15, 14, 13, 12).
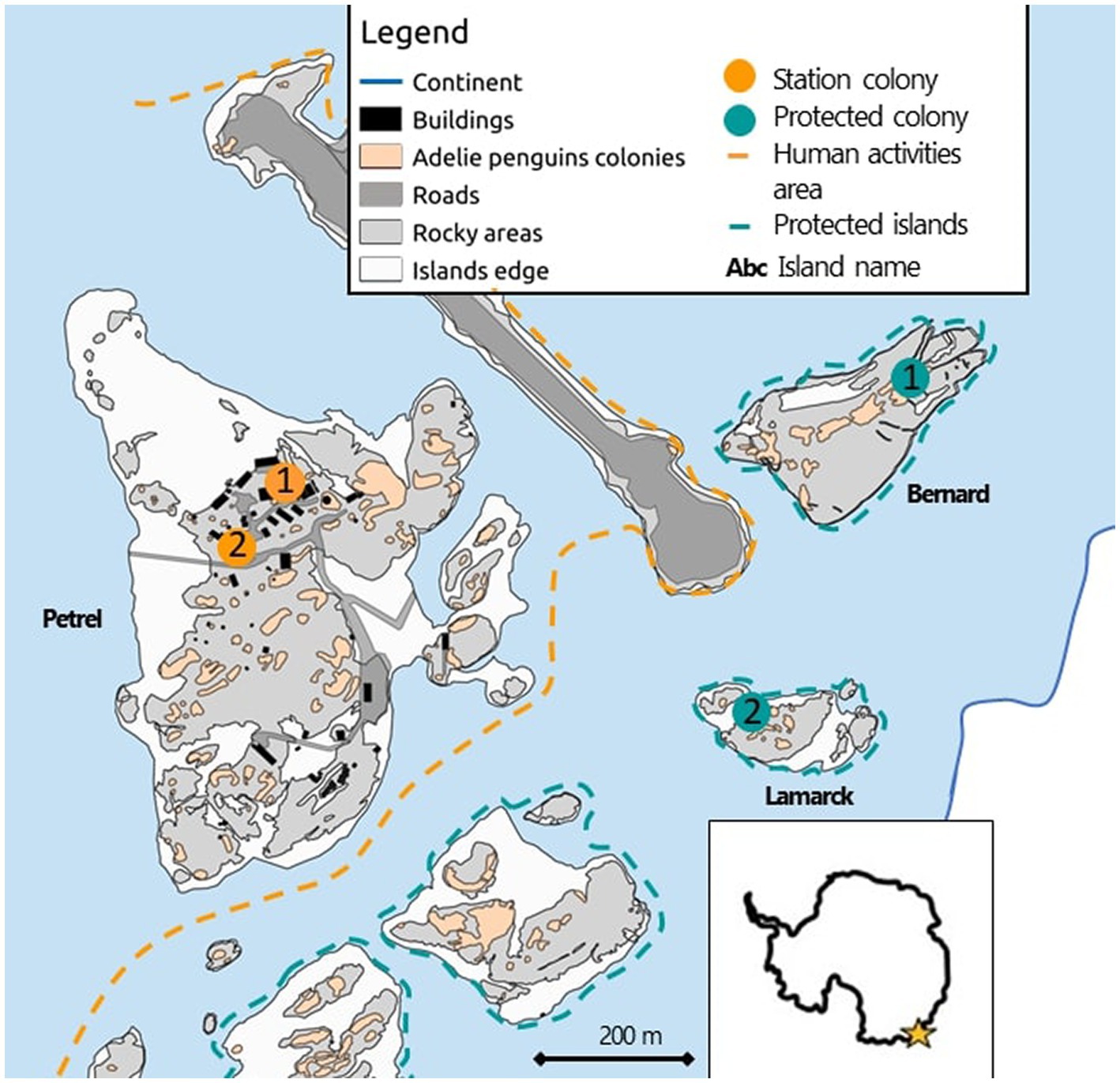
Figure 1. Study area in Adelie Land, East Antarctica. Representation of the disturbed (orange) and undisturbed (green) areas with sampled colonies highlighted with large colored dots.
2.2. Handling procedures, body measurements, and standardized stress protocol
All birds were captured by hand. A hood was immediately placed over the head and individuals were sampled for blood (0.5 mL of blood drawn from the tarsal vein within 3 min of capture) to obtain basal corticosterone levels (Romero and Reed, 2005; Angelier et al., 2010). Flipper length was measured using a ruler (±1 mm) and weight using a digital scale (±10 g). Body condition was calculated as the residuals from the linear regression between mass and flipper length (adults: F1,218 = 20.02, p < 0.001; chicks: F1,49 = 20.99, p < 0.001). Birds were then restrained for 15 min (standardized stress protocol, see Wingfield et al., 1992) and a second blood sample collected to measure stress-induced corticosterone levels. Birds were marked with a dye spot (Porcimark®) before being released near their nest to avoid recapturing the same individual twice.
2.3. Corticosterone assay and molecular sexing
Sex determination was performed by molecular sexing at the CEBC (‘Service d’Analyses Biologiques’) as described in Marciau et al. (2023). Plasma concentrations of corticosterone were determined in 50 μL samples by radio-immunoassay following procedures described in Lormée et al. (2003). Inter-assay and intra-assay precisions were, respectively, 8.00 and 12.34%. Corticosterone lowest detectable concentration was 0.28 ng/mL.
2.4. Statistical analysis
To examine the effect of anthropogenic activities on Adelie penguins in this region we tested models for four response variables: two morphometric, (A) flipper length and (B) body condition, and two physiological, (C) basal and (D) stress-induced corticosterone levels. All statistical analyses were performed using the R software (version 4.2.1 R Core Team, 2022). Linear mixed effect models and generalized least squares models were performed with the nlme package (Pinheiro et al., 2023) and model selection with the MuMIn package (Bartoń, 2022).
To first examine the appropriate random effect structure, we considered a relatively full linear mixed model (LMM) fitted using REML with all covariates included (Table 1, main fixed effect being Area) and including a hierarchical random effect with Colony nested within Area. However, only two colonies per area could not support estimation of this random effects structure and resulted in singularity errors. We secondly considered including only Colony as a random effect and used AIC and likelihood ratio tests (LRTs) to compare models with i. the nested random effect Area/Colony, ii. Colony only as a random effect, or iii. no random effect. In all cases, the AIC and LRTs provided no support for including a random effects structure and further analyses (detailed below) were developed using generalized least squares models fitted using ML estimation.
Our primary aim was to investigate for effects between disturbed (station) and undisturbed (protected) areas acknowledging our sampling was limited to two colonies per area and other potential influential factors (stage, sex etc.). To determine the best fixed-effects structure we therefore proceeded with a model selection approach using the dredge() function–to generate a model selection table of models with all possible subsets of terms – with models ranked using AICc and Akaike weights (AICw). In this model selection procedure, we considered Area OR Colony, excluding models containing both these terms (Table 1). This approach investigates disturbance effects between areas while acknowledging that effects may not manifest identically across colonies.
Following this workflow, we tested successively models for the four response variables, fitting separate models for the three stages in each case, i.e., incubating adults, rearing adults, chicks (12 final models in total, Table 1). We present results from the best-ranked model based on AICc and AICw criterion. In general, this approach follows the scientific rule of parsimony in selecting the simplest parameterization supported by the available data. However, for disturbance studies the burden of proof for demonstrating no effect (accepting a null hypothesis) is necessarily higher. In a conservative approach, we therefore also present and discuss the second-ranked models when needed, i.e., when AICw are very similar.
3. Results
3.1. Determinants of flipper length
For all stages examined, i.e., incubating and chick-rearing adults and chicks, the best ranked model for flipper length included only sex. In all cases males had significantly longer flippers than females (Table 2; Supplementary Figure S1). For incubating adults, the mean predicted flipper length for males was 192.13 ± 0.73 and for females was 188.43 ± 0.93 (Supplementary Figure S1a). For chick-rearing adults the predicted flipper length for males was 192.23 ± 0.84 and females was 187.36 ± 0.78 (Supplementary Figure S1a). For chicks predicted flipper length for males was 18.37 ± 0.10 and females was 17.83 ± 0.10 (Supplementary Figure S1b).
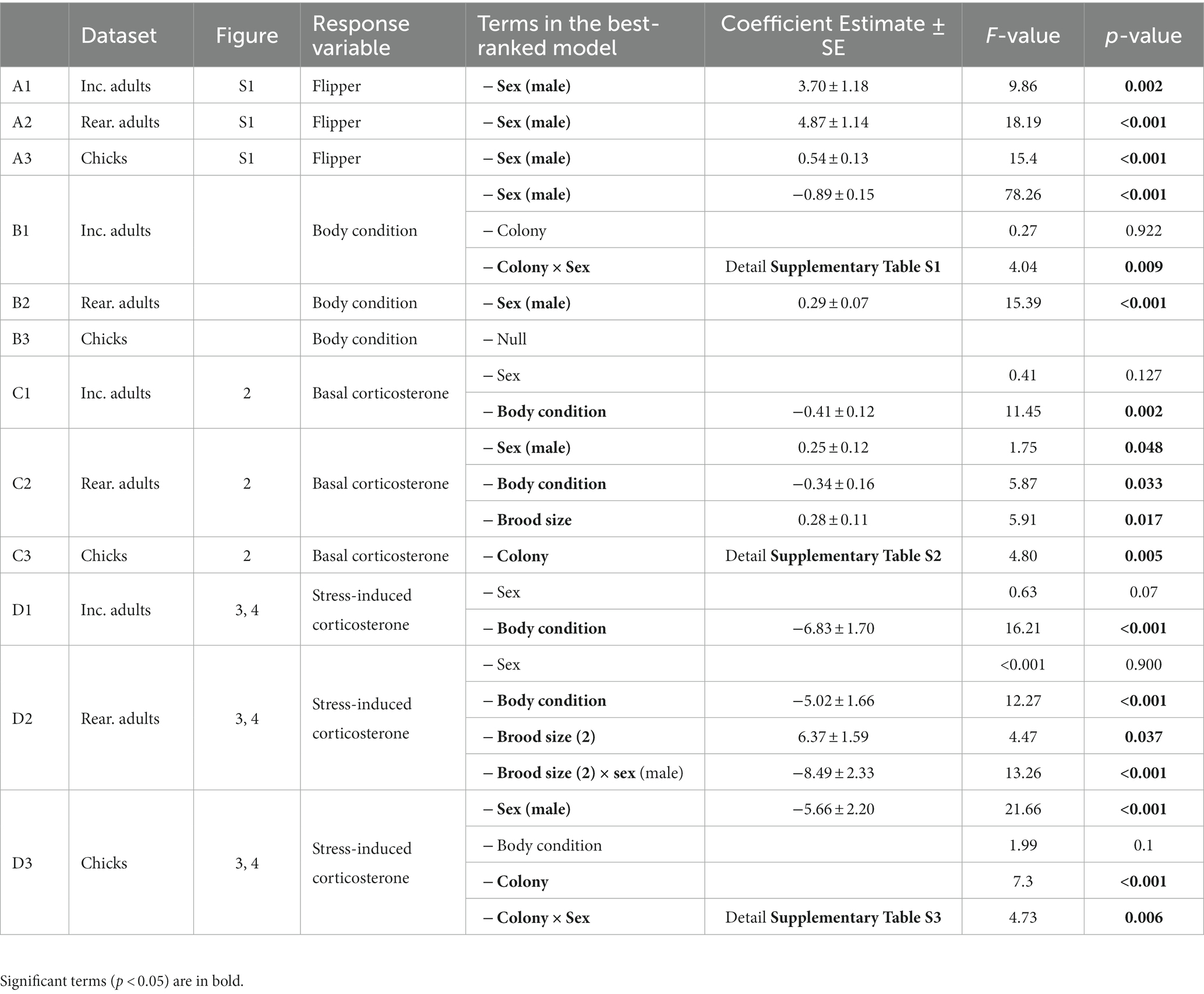
Table 2. Summary of final best-ranked models for (A) flipper length, (B) body condition, (C) basal corticosterone, and (D) stress-induced corticosterone.
3.2. Determinants of body condition
For incubating adults the best ranked model included only sex, with males having a significantly lower body condition than females (F value = 78.26, value of p <0.001, Table 2; male predicted value = −0.26 ± 0.05, female predicted value = 0.41 ± 0.06). However, the model selection procedure showed the second-ranked model to be similarly weighted (AIC weight ratio was only 1.13: AICw1 = 0.40, AICw2 = 0.36). In this second model, Colony and a Colony x sex interaction effect were retained (F-value = 4.04, value of p = 0.009, Table 2). Females from protected colony 2 (Lamarck Is) presented a significantly lower condition (predicted value = 0.14 ± 0.12) than females from protected colony one (Bernard Is; predicted value = 0.58 ± 0.12) and from the station colony 1 (predicted values = 0.55 ± 0.12; contrasts detailed in Supplementary Table S1). For adults in chicks rearing, the best ranked model included only sex. Males had a significantly higher body condition than females (p < 0.001, male predicted value = 0.16, female predicted value = −0.14, Figure 2). For chicks the best-ranked model was the null model, indicating body condition did not vary in relation to sex or Area/Colony.
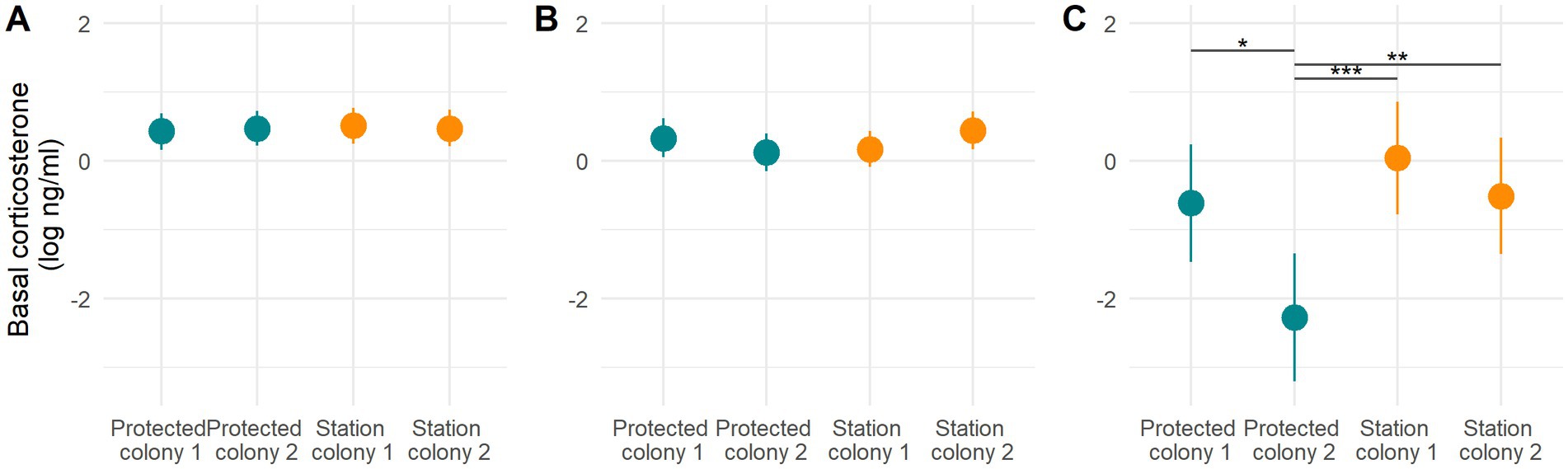
Figure 2. Model results for basal corticosterone levels (log) in relation to the colony in incubating adults (A), rearing adults (B), and chicks (C). Predicted mean values ± SE are plotted. Asterisks denote statistical significance between two groups (*p < 0.05, **p < 0.005, ***p < 0.001) based on contrast analyses (presented in Supplementary Table S2).
3.3. Determinants of basal corticosterone levels
For incubating adults the best ranked model included both body condition and sex, however this model had a similar weight to the second-ranked model excluding sex (i.e., AIC weight ratio was only 1.14: AIC w1 = 0.28, AIC w2 = 0.25) and the sex difference was not significant (Table 2). Individuals in poorer condition had higher basal levels of corticosterone (coefficient estimate = −0.41 ± 0.12; F-value = 11.45, value of p = 0.002, Table 2). For chick-rearing adults the best ranked model included body condition, brood size, and sex. Individuals in poorer condition tended to have higher basal levels of corticosterone (coefficient estimate = −0.34 ± 0.16, F-value = 5.87, value of p = 0.033, Table 2). Individuals rearing 2 chicks had higher basal corticosterone levels than individuals rearing a single chick (F-value = 5.91, value of p = 0.017, Table 2; predicted value for single chick individual = 1.28 ± 1.08, two chicks = 1.70 ± 1.08). Males had higher basal corticosterone levels than females (F-value = 1.75, value of p = 0.048, Table 2; predicted value for females = 1.28 ± 1.08, males = 1.65 ± 1.09). For chicks the best ranked model included only the Colony term (F-value = 4.80, value of p = 0.005, Table 2). Chicks from protected colony 2 (Lamarck Is) presented lower basal corticosterone levels (Table 2; Figure 2, contrasts detailed in Supplementary Table S1) while the other three colonies were not different (predicted value for station colony 1 = 1.04 ± 1.52; station colony 2 = 0.60 ± 1.54; protected colony 1 = 0.54 ± 1.54; protected colony 2 = 0.10 ± 1.60).
3.4. Determinants of stress-induced corticosterone levels
For incubating adults the best ranked model included body condition and sex, but it did not include the terms colony or area. Birds in poorer body condition had higher stress-induced corticosterone levels (F-value = 16.21, value of p <0.001, Table 2; Figure 3A) and there was weak evidence that males tended to have lower stressed-induced corticosterone levels than females (Table 2; predicted value for males = 19.37 ± 1.02, for females = 22.77 ± 1.35).
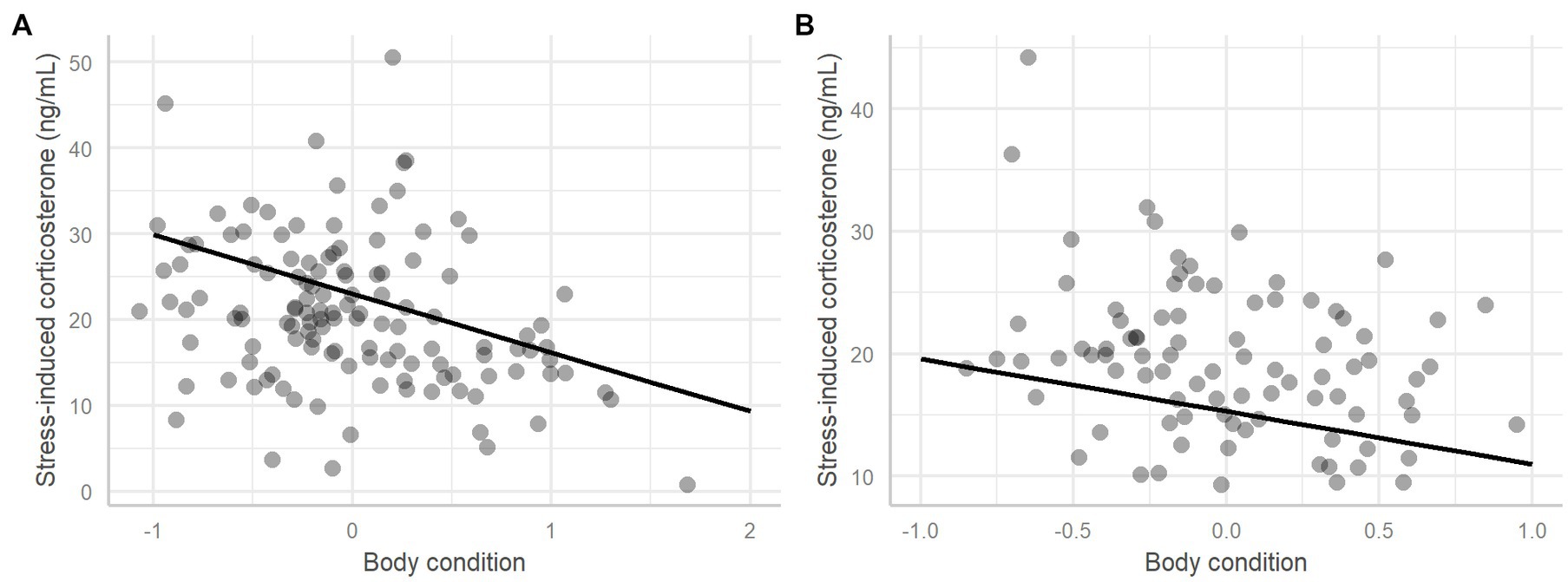
Figure 3. Stress-induced corticosterone in relation to the body condition, in incubating adults (A) and rearing adults (B). The dots represent the raw data and the regression line is extracted from the fitted model.
For chick-rearing adults the best ranked model included body condition, brood size, sex and the interaction between brood size and sex, but it did not include the terms colony or area. Again, individuals in poorer condition had higher levels of stress-induced corticosterone (F-value = 12.27, p = <0.001, Table 2; Figure 3B). Females with a higher brood size had higher levels of stress-induced corticosterone (F-value = 13.26, p = <0.001, Table 2; predicted value for females with one chick = 16.24 ± 1.15, two chicks = 22.71 ± 1.13), but this was not the case for males. The model selection procedure showed the second-ranked model to be similarly weighted (i.e., AIC weight ratio was only 1.10). In this second model the area term was retained indicating lower stress-induced corticosterone in the undisturbed area, but this effect was not significant (coefficient estimate = −1.69 ± 1.19, t-value = −1.43, value of p = 0.16; predicted value for station colonies = 20.44 ± 0.83, protected colonies = 18.75 ± 0.83). Incubating and chick-rearing adults did not present any significant differences in stress-induced corticosterone levels between colonies (Figures 4A and B).
For chicks the best ranked model included body condition, colony, sex and the interaction between sex and colony. The colony-level effects showed male chicks from station colony 1 had a higher level of stress-induced corticosterone than chicks from the two protected colonies (p = 0.009 and 0.069; Table 2; Figure 4C; contrasts detailed in Supplementary Table S3) and female chicks from station colony 2 presented higher levels of stress-induced corticosterone than the three other colonies (all p < 0.001; Table 2; Figure 4D; contrasts detailed in Supplementary Table S3).
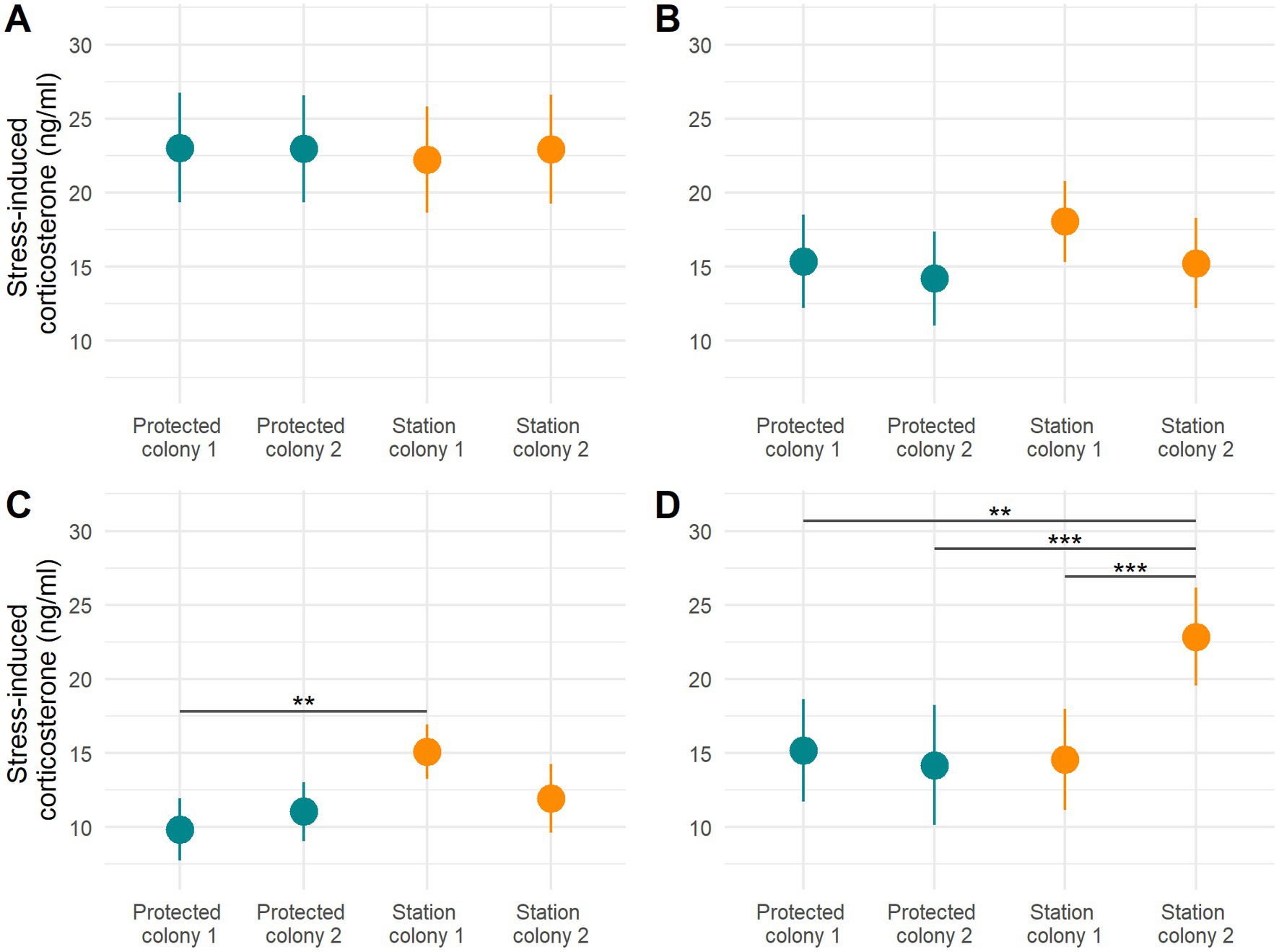
Figure 4. Stress-induced corticosterone in relation to the colony in incubating adults (A), rearing adults (B), male chicks (C), and female chicks (D). Predicted mean values ± SE are plotted. Asterisks denote statistical significance between two groups (*p < 0.05, **p < 0.005, ***p < 0.001) based on contrast analyses (presented in Supplementary Table S3).
4. Discussion
4.1. What impact of human activities on body condition and corticosterone levels?
Contrary to our hypotheses, there was no difference in either body condition or corticosterone levels between the disturbed and undisturbed areas for breeding adult Adelie penguins. In chicks, the individuals from some disturbed colonies, but not others, had higher corticosterone levels that the chicks from undisturbed colonies, indicating that there was no strong and consistent difference in corticosterone level and body condition between disturbed and undisturbed areas (see Table 3). Our study therefore suggests that human activities have no major effect on the reproduction of this species in our study sites. Supporting this interpretation, the Adélie penguin population on the Pointe Géologie archipelago has been increasing over the last 10 years despite the human activities on this site (Barbraud et al., 2020). Indeed, this Adélie penguin population had increased even during major building construction (Micol and Jouventin, 2001). Similarly, other studies have found no evidence that human disturbance effects reproductive success and population trends in several sites of western Antarctica (Carlini et al., 2007; Lynch et al., 2010; Villanueva et al., 2014; but see Woehler et al., 1994; Giese, 1996).
The lack of difference in body condition between the disturbed and undisturbed areas in any group (breeding adults or chicks) suggests that human activities have no detrimental effect on energy expenditure or acquisition. Nonetheless, human presence can induce increased heart rate in some Antarctic birds including Adélie penguins (e.g., Culik et al., 1990; Weimerskirch et al., 2002; de Villiers et al., 2006; Ellenberg et al., 2013) which may be associated with higher energy expenditure, and a quicker depletion of energy reserves in fasting animals (e.g., Groscolas et al., 2010). In addition, human presence can affect foraging efficiency of breeding penguins if this delays departure from the colony or return from the sea (French et al., 2019). Body condition is a crucial determinant of breeding success in Adélie penguins (Vleck and Vleck, 2002). First, nest desertion occurs when adults reach a low threshold in body condition (Spée et al., 2010; Thierry et al., 2013). Second, chick’s body condition is thought to be the main determinant of fledging success and post-fledging survival (Ainley et al., 2018). Because we did not find any evidence of an effect of human activities on body condition, our study suggests that Adélie penguins at DDU are tolerant to interactions with humans and this could explain why colonies were able to persist in the middle of the station, perhaps due to individuals being habituated to this level of human activity.
Adult baseline and stress-induced corticosterone levels did not differ between the disturbed and undisturbed areas. We had hypothesized that human disturbance would be the main determinant of corticosterone levels (predictions 1b, 1c, 2b, and 2c), but instead corticosterone levels were mainly affected by body condition and brood size. The similarity in both baseline and stress-induced corticosterone levels between disturbed and undisturbed areas suggest that the functioning of the HPA axis was not affected by human disturbance in these colonies.
In contrast, there were variations in chick basal and stress-induced corticosterone levels that were consistently (but not exhaustively) in the direction of higher corticosterone levels in some of the disturbed colonies (i.e., sensitization). The best model retained the colony term rather than the area (i.e., level of disturbance) but because the colonies with the highest corticosterone levels were within the disturbed areas there is some support for a weak and inconsistent disturbance effect. This inter-colony variability in corticosterone levels shows the necessity of replication because only one disturbed and one protected colony could have led to misleading conclusions. Previous studies have reported that human activities did influence corticosterone levels in penguins and other species (reviewed in Dantzer et al., 2014), for example, and according to our predictions 1b and 1c, Ellenberg et al. (2007) reported Yellow-eyed penguins (Megadyptes antipodes) had higher baseline and stress-induced corticosterone levels and lower reproductive performance in disturbed areas. Similarly, Müllner et al. (2004) reported that tourist activity was associated with increased stress-induced corticosterone levels, lower body mass, and reduced survival in hoatzin juveniles (Opisthocomus hoazin). In contrast, other studies reported that individuals have lower stress-induced corticosterone levels or fecal corticosterone levels in response to human disturbance in penguins species (Barbosa et al., 2013; Scheun et al., 2021).
We predicted a dampened stress response (prediction 2c) because Adélie penguins may have habituated to human disturbance in the disturbed area (Rich and Romero, 2005; Cyr and Romero, 2009). Habituation enables individuals to avoid a detrimental state of chronic stress (Angelier and Wingfield, 2013). Adult Magelanic (Spheniscus magellanicus) penguins had lower stress-induced corticosterone levels in response to frequent tourism activities Walker et al. (2006) and this habituation was not associated with any apparent fitness costs. Other studies have also reported no significant effect of human disturbance on plasma (Walker et al., 2005; Villanueva et al., 2012) or fecal corticosterone levels (Ozella et al., 2017; Lynch et al., 2019). In our disturbed site, penguins live in the middle of the station (and have done for 60 years), but human activities do not seem to represent a threat to them. Adélie penguins have not co-evolved with terrestrial predators, and so adults and chicks may not perceive human presence a threat, and not modulate their corticosterone stress response. Altogether, these studies suggest that the response of the HPA axis to human disturbance (e.g., sensitization, habituation, or no effect) may be a key determinant of the ability of wild vertebrate populations to coexist with humans (Angelier and Wingfield, 2013).
The sensitivity of individuals to human disturbance may depend on life-history stages. For example, Magellanic penguin chicks are more sensitive to disturbance than adults because, contrary to adults, chicks do not show a dampened stress response in response to human presence, but even show increased stress-induced corticosterone levels during their first day of life (Walker et al., 2006). We therefore predicted that Adélie penguins may be more sensitive during specific stages (prediction 3). Despite only weak support and an inability to separate this effect from confounding factors (sex, colony), we observed this effect in chicks which presented higher levels of corticosterone (although not in all colonies even in the disturbed area). In addition, at our study site, a recent study reported that Adélie penguin chicks from the disturbed area had shorter telomeres relative to those from the undisturbed area, suggesting some hidden physiological costs of growing in disturbed areas at this stage (Caccavo et al., 2021). Further studies are therefore required to fully evaluate the behavioral and physiological impact of human disturbance on Adélie penguin chicks (possibly at different stages of development), and on their probability to recruit into the population.
Different penguin species may have different sensitivity to human disturbance. Humboldt penguin (Spheniscus humboldti) appears much more sensitive to human disturbance than the Magellanic penguin (Ellenberg et al., 2006). The history of co-habitation of a penguin species or population with humans and terrestrial predators may however modulate its sensibility to human disturbance (see Bricher et al., 2008; Villanueva et al., 2012; Viblanc et al., 2012; Pichegru et al., 2016 for some examples). The specific history of Antarctic penguins could explain why Adélie penguins appear quite tolerant to human disturbance (at least at our study site) because they have not co-evolved with terrestrial predators or human presence until very recently. It is however important to note that a few other studies have found that human presence can affect breeding success of this species in other areas (Woehler et al., 1994; Giese, 1996; Bricher et al., 2008), suggesting that human activities may be detrimental to this species in other populations or maybe under specific environmental circumstances (e.g., low food availability). Although we did not find any difference in our variables of interest between the disturbed and undisturbed areas, it is also important to note that Adélie penguins may benefit to some extent from human presence because buildings can provide shelters against inclement weather and predators such as skuas (some penguins nest under buildings, another sign of high tolerance to human activities). Finally, we cannot exclude that the impact of human disturbance on penguin physiology may vary between populations. For example, declining populations of Magellanic penguins showed chronic stress when exposed to tourism while increasing and stable populations did not (Palacios et al., 2018).
4.2. Inter-colony and individual heterogeneity in body condition and corticosterone levels
In addition to the comparison between the disturbed and undisturbed areas, we also investigated how our variables of interest varied between the four colonies, allowing us to document important variations in body condition and corticosterone levels at a finer scale. Given that differences in body condition or corticosterone levels between the four colonies were not driven by a strong and consistent effect of human disturbance, indicating the importance of other environmental or individual characteristics. All of the penguins breeding in this region forage in the same zones (Michelot et al., 2020), so food availability should not differ between the four studied colonies. Inter-colony differences in corticosterone levels are also unlikely to be linked to brood size as it did not differ between colonies. Similarly, body condition is unlikely to explain these differences in corticosterone levels between colonies because body condition did not dramatically vary between colonies. Abiotic factors, such as slope, orientation, exposition, and predation risk could explain the differences between colonies in our study (Patterson et al., 2003; Bricher et al., 2008; Schmidt et al., 2021), especially if penguins select their breeding site (i.e., colony) according to their individual quality or age. However, we did not find any important difference in body size or body condition between colonies.
Our study demonstrates that sensitivity to abiotic and biotic factors may vary between females and males. Adélie penguins do not have strong sexual dimorphism (Jennings et al., 2016) but breeding female and male Adélie penguins may forage in different areas (Widmann et al., 2015; Lescroël et al., 2020) and may differ in their parental expenditure (Beaulieu et al., 2009; Colominas-Ciuró et al., 2017). There were also differences in chick corticosterone levels between sexes, and sex-dependent differences in corticosterone levels between colonies. Although the origin of these differences remains unclear, they suggest that female chicks may be more sensitive to environmental constraints, and possibly human disturbance than male chicks (Jennings et al., 2016).
4.3. Conclusion
By studying complementary variables (body condition, corticosterone levels and breeding performance) at several colonies in two areas with contrasting levels of human disturbance, our study provides evidence that breeding Adélie penguins were not detrimentally affected by human activities, at least at the adult stage. Rather, in adults, baseline and stress-induced corticosterone levels were primarily influenced by body condition, sex, and brood size. In chicks, there was some support for higher corticosterone levels in the colonies located in the vicinity of the station relative to the colonies located in protected areas. Adélie penguins at Dumont D’Urville station seem to be quite tolerant to human presence, and this may result from the specific history of this species, which has not co-evolved with humans until very recently. Future studies should now investigate complementary and promising stress-related physiological markers, such as heart rate (Weimerskirch et al., 2002; Viblanc et al., 2012; Schaefer and Colombelli-Négrel, 2021) or telomere length (Caccavo et al., 2021; Salmon and Burraco, 2022), to further assess the influence of multiple human disturbance stimuli on Adelie penguins (Nimon et al., 1995; Ellenberg et al., 2013).
Data availability statement
The raw data supporting the conclusions of this article will be made available by the authors, without undue reservation.
Ethics statement
The animal study was reviewed and approved by French Regional Ethical Committee in animal experimentation (No. 84), permit number APAFIS#2111–2,015,092,414,273,394 v4.
Author contributions
FA and TR designed the study. FA, TP, and TR conducted the fieldwork. FA, CM, and SB analyzed the data. CP and CR conducted the laboratory analyses. CB and KD provided additional data from the P109. CM and FA wrote the manuscript with input from TP, YR-C, MAH, SB, CP, TR, CR, KD, CB, and AK. All authors contributed to the article and approved the submitted version.
Funding
The project is part of the research programs 1091 and 109, financially and logistically supported by the French polar institute (IPEV) and the Zone Atelier Antarctique (CNRS-INEE). This project was also funded by the CNRS, the WWF-UK and by a research scholarship allocated by the University of Tasmania (TGRS) to CM.
Acknowledgments
The authors are grateful to the “Service d’Analyses Biologiques du CEBC” for its expertise and technical support in conducting laboratory analyses. The authors acknowledge Julien Vasseur for SIG support in mapping area. The long-term Adelie population survey of the pointe geologie archipelago was made possible thanks to all the fieldworkers involved in the monitoring program over years (program 109 IPEV).
Conflict of interest
The authors declare that the research was conducted in the absence of any commercial or financial relationships that could be construed as a potential conflict of interest.
Publisher’s note
All claims expressed in this article are solely those of the authors and do not necessarily represent those of their affiliated organizations, or those of the publisher, the editors and the reviewers. Any product that may be evaluated in this article, or claim that may be made by its manufacturer, is not guaranteed or endorsed by the publisher.
Supplementary material
The Supplementary material for this article can be found online at: https://www.frontiersin.org/articles/10.3389/fevo.2023.1099028/full#supplementary-material
References
Ainley, D. G., Dugger, K. M., Mesa, M. L., Ballard, G., Barton, K. J., Jennings, S., et al. (2018). Post-fledging survival of Adélie penguins at multiple colonies: chicks raised on fish do well. Mar. Ecol. Prog. Ser. 601, 239–251. doi: 10.3354/meps12687
Angelier, F., and Wingfield, J. C. (2013). Importance of the glucocorticoid stress response in a changing world: theory, hypotheses and perspectives. 10th International Symposium on Avian Endocrinology 190, 118–128. doi: 10.1016/j.ygcen.2013.05.022
Angelier, F., Wingfield, J. C., Weimerskirch, H., and Chastel, O. (2010). Hormonal correlates of individual quality in a long-lived bird: a test of the 'corticosterone-fitness hypothesis'. Biol. Lett. 6, 846–849. doi: 10.1098/rsbl.2010.0376
Barbosa, A., de Mas, E., Benzal, J., Diaz, J. I., Motas, M., Jerez, S., et al. (2013). Pollution and physiological variability in gentoo penguins at two rookeries with different levels of human visitation. Antarct. Sci. 25, 329–338. doi: 10.1017/S0954102012000739
Barbraud, C., Delord, K., Bost, C. A., Chaigne, A., Marteau, C., and Weimerskirch, H. (2020). Population trends of penguins in the French southern territories. Polar Biol. 43, 835–850. doi: 10.1007/s00300-020-02691-6
Beaulieu, M., Ropert-Coudert, Y., Maho, Y. L., Ancel, A., and Criscuolo, F. (2009). Foraging in an oxidative environment: relationship between δ13C values and oxidative status in Adélie penguins. Proc. R. Soc. Lond. B Biol. Sci. 277, 1087–1092. doi: 10.1098/rspb.2009.1881
Bender, N. A., Crosbie, K., and Lynch, H. J. (2016). Patterns of tourism in the Antarctic peninsula region: a 20-year analysis. Antarct. Sci. 28, 194–203. doi: 10.1017/S0954102016000031
Bricher, P. K., Lucieer, A., and Woehler, E. J. (2008). Population trends of Adélie penguin (Pygoscelis adeliae) breeding colonies: a spatial analysis of the effects of snow accumulation and human activities. Polar Biol. 31, 1397–1407. doi: 10.1007/s00300-008-0479-z
Busch, D. S., and Hayward, L. S. (2009). Stress in a conservation context: a discussion of glucocorticoid actions and how levels change with conservation-relevant variables. Biol. Conserv. 142, 2844–2853. doi: 10.1016/j.biocon.2009.08.013
Caccavo, J. A., Raclot, T., Poupart, T., Ropert-Coudert, Y., and Angelier, F. (2021). Anthropogenic activities are associated with shorter telomeres in chicks of Adélie penguin (Pygoscelis adeliae). Polar Biol. 44, 1391–1399. doi: 10.1007/s00300-021-02892-7
Carlini, A. R., Coria, N. R., Santos, M. M., Libertelli, M. M., and Donini, G. (2007). Breeding success and population trends in Adélie penguins in areas with low and high levels of human disturbance. Polar Biol. 30, 917–924. doi: 10.1007/s00300-006-0251-1
Chown, S. L., Lee, J. E., Hughes, K. A., Barnes, J., Barrett, P. J., Bergstrom, D. M., et al. (2012). Conservation. Challenges to the future conservation of the Antarctic. Science 337, 158–159. doi: 10.1126/science.1222821
Cockrem, J. F., Potter, M. A., and Candy, E. J. (2006). Corticosterone in relation to body mass in Adelie penguins (Pygoscelis adeliae) affected by unusual sea ice conditions at Ross Island. Antarctica. Gen. Comp. Endocrinol. 149, 244–252. doi: 10.1016/j.ygcen.2006.06.002
Coetzee, B. W. T., and Chown, S. L. (2016). A meta-analysis of human disturbance impacts on Antarctic wildlife. Biol. Rev. 91, 578–596. doi: 10.1111/brv.12184
Coetzee, B. W. T., Convey, P., and Chown, S. L. (2017). Expanding the protected area network in Antarctica is urgent and readily achievable. Conserv. Lett. 10, 670–680. doi: 10.1111/conl.12342
Colominas-Ciuró, R., Santos, M., Coria, N., and Barbosa, A. (2017). Reproductive effort affects oxidative status and stress in an Antarctic penguin species: an experimental study. PLoS One 12:e0177124. doi: 10.1371/journal.pone.0177124
Culik, B., Adelung, D., and Woakes, A. J. (1990). “The effect of disturbance on the heart rate and behaviour of Adélie penguins (Pygoscelis adeliae) during the breeding season” in Antarctic ecosystems. eds. K. R. Kerry and G. Hempel (Berlin, Heidelberg: Springer), 177–182. doi: 10.1007/978-3-642-84074-6_18
Cyr, N. E., and Romero, L. M. (2009). Identifying hormonal habituation in field studies of stress. Gen. Comp. Endocrinol. 161, 295–303. doi: 10.1016/j.ygcen.2009.02.001
Dantzer, B., Fletcher, Q. E., Boonstra, R., and Sheriff, M. J. (2014). Measures of physiological stress: a transparent or opaque window into the status, management and conservation of species? Conserv. Physiol. 2:cou023. doi: 10.1093/conphys/cou023
de Villiers, M., Bause, M., Giese, M., and Fourie, A. (2006). Hardly hard-hearted: heart rate responses of incubating northern Giant petrels (Macronectes halli) to human disturbance on sub-Antarctic Marion Island. Polar Biol. 29, 717–720. doi: 10.1007/s00300-006-0137-2
Dickens, M. J., and Romero, L. M. (2013). A consensus endocrine profile for chronically stressed wild animals does not exist. Gen. Comp. Endocrinol. 191, 177–189. doi: 10.1016/j.ygcen.2013.06.014
Ellenberg, U., Mattern, T., and Seddon, P. J. (2013). Heart rate responses provide an objective evaluation of human disturbance stimuli in breeding birds. Conserv. Physiol. 1:cot013. doi: 10.1093/conphys/cot013
Ellenberg, U., Mattern, T., Seddon, P. J., and Jorquera, G. L. (2006). Physiological and reproductive consequences of human disturbance in Humboldt penguins: the need for species-specific visitor management. Biol. Conserv. 133, 95–106. doi: 10.1016/j.biocon.2006.05.019
Ellenberg, U., Setiawan, A. N., Cree, A., Houston, D. M., and Seddon, P. J. (2007). Elevated hormonal stress response and reduced reproductive output in yellow-eyed penguins exposed to unregulated tourism. Gen. Comp. Endocrinol. 152, 54–63. doi: 10.1016/j.ygcen.2007.02.022
French, R. K., Muller, C. G., Chilvers, B. L., and Battley, P. F. (2019). Behavioural consequences of human disturbance on subantarctic yellow-eyed penguins Megadyptes antipodes. Bird Conserv. Int. 29, 277–290. doi: 10.1017/S0959270918000096
Giese, M. (1996). Effects of human activity on adelie penguin Pygoscelis adeliae breeding success. Biol. Conserv. 75, 157–164. doi: 10.1016/0006-3207(95)00060-7
Groscolas, R., Viera, V., Guerin, N., Handrich, Y., and Côté, S. D. (2010). Heart rate as a predictor of energy expenditure in undisturbed fasting and incubating penguins. J. Exp. Biol. 213, 153–160. doi: 10.1242/jeb.033720
IAATO ATCM Information Papers (2022). IAATO overview of Antarctic tourism: a historical review of growth, the 2021–22 season, and preliminary estimates for 2022–23. https://iaato.org/information-resources/data-statistics/iaato-atcm-information-papers/
Jennings, S., Varsani, A., Dugger, K. M., Ballard, G., and Ainley, D. G. (2016). Sex-based differences in Adélie penguin (Pygoscelis adeliae) Chick growth rates and diet. PLoS One 11:e0149090. doi: 10.1371/journal.pone.0149090
Lescroël, A., Lyver, P. O., Jongsomjit, D., Veloz, S., Dugger, C. M., Kappes, P., et al. (2020). Inter-individual differences in the foraging behavior of breeding Adélie penguins are driven by individual quality and sex. MEPS 636, 189–205. doi: 10.3354/meps13208
Lormée, H., Jouventin, P., Trouve, C., and Chastel, O. (2003). Sex-specific patterns in baseline corticosterone and body condition changes in breeding red-footed boobies Sula sula. Ibis 145, 212–219. doi: 10.1046/j.1474-919X.2003.00106.x
Lynch, H. J., Fagan, W. F., and Naveen, R. (2010). Population trends and reproductive success at a frequently visited penguin colony on the western Antarctic peninsula. Polar Biol. 33, 493–503. doi: 10.1007/s00300-009-0726-y
Lynch, M. A., Youngflesh, C., Agha, N. H., Ottinger, M. A., and Lynch, H. J. (2019). Tourism and stress hormone measures in Gentoo penguins on the Antarctic peninsula. Polar Biol. 42, 1299–1306. doi: 10.1007/s00300-019-02518-z
Marciau, C., Costantini, D., Bestley, S., Hicks, O., Hindell, M. A., Kato, A., et al. (2023). Environmental drivers of growth and oxidative status during early life in a long-lived Antarctic seabird, the Adélie penguin. Physiol. Biochem. Zool. :000. doi: 10.1086/724686
McEwen, B. S., and Wingfield, J. C. (2003). The concept of allostasis in biology and biomedicine. Horm. Behav. 43, 2–15. doi: 10.1016/S0018-506X(02)00024-7
Michelot, C., Kato, A., Raclot, T., Shiomi, K., Goulet, P., Bustamante, P., et al. (2020). Sea-ice edge is more important than closer open water access for foraging Adélie penguins: evidence from two colonies. Mar. Ecol. Prog. Ser. 640, 215–230. doi: 10.3354/meps13289
Micol, T., and Jouventin, P. (2001). Long-term population trends in seven Antarctic seabirds at pointe Géologie (Terre Adélie) human impact compared with environmental change. Polar Biol. 24, 175–185. doi: 10.1007/s003000000193
Müllner, A., Eduard Linsenmair, K., and Wikelski, M. (2004). Exposure to ecotourism reduces survival and affects stress response in hoatzin chicks (Opisthocomus hoazin). Biol. Conserv. 118, 549–558. doi: 10.1016/j.biocon.2003.10.003
Nimon, A. J., Schroter, R. C., and Stonehouse, B. (1995). Heart rate of disturbed penguins. Nature 374:415. doi: 10.1038/374415a0
Ozella, L., Anfossi, L., Di Nardo, F., and Pessani, D. (2017). Effect of weather conditions and presence of visitors on adrenocortical activity in captive African penguins (Spheniscus demersus). Gen. Comp. Endocrinol. 242, 49–58. doi: 10.1016/j.ygcen.2015.12.002
Palacios, M. G., D’Amico, V. L., and Bertellotti, M. (2018). Ecotourism effects on health and immunity of Magellanic penguins at two reproductive colonies with disparate touristic regimes and population trends. Conservation Physiology 6, coy060. doi: 10.1093/conphys/coy060
Patterson, D. L., Easter-Pilcher, A., and Fraser, W. (2003). The effects of human ctivity and environmental variability on long-term changes in Adelie penguin populations at Palmer Station, Antarctica. In Antarctic biology in a global context. eds. A. H. L. Huiskes, W. W. C. Gieskes, J. Rozema, R. M. L. Schorno, S. M. van der Vies, and W. J. Wolff (Leiden, The Netherlands: Backhuys Publishers), 301–307.
Pertierra, L. R., Hughes, K. A., Vega, G. C., and Olalla-Tárraga, M. Á. (2017). High resolution spatial mapping of human footprint across Antarctica and its implications for the strategic conservation of avifauna. PLoS One 12:e0168280. doi: 10.1371/journal.pone.0168280
Pichegru, L., Edwards, T. B., Dilley, B. J., Flower, T. P., and Ryan, P. G. (2016). African penguin tolerance to humans depends on historical exposure at colony level. Bird Conserv. Int. 26, 307–322. doi: 10.1017/S0959270915000313
Pinheiro, J., and Bates, D., R Core Team (2023). Nlme: linear and nonlinear mixed effects models. R package version 3, 1–162. Available at: https://CRAN.R-project.org/package=nlme (Accessed January 31, 2023).
R Core Team (2022). R: A language and environment for statistical computing. Vienna, Austria: R Foundation for Statistical Computing.
Rich, E. L., and Romero, L. M. (2005). Exposure to chronic stress downregulates corticosterone responses to acute stressors. Am. J. Physiol.-Regul. Integr. Comp. Physiol. 288, R1628–R1636. doi: 10.1152/ajpregu.00484.2004
Romero, L. M., Dickens, M. J., and Cyr, N. E. (2009). The reactive scope model-a new model integrating homeostasis, allostasis, and stress. Horm. Behav. 55, 375–389. doi: 10.1016/j.yhbeh.2008.12.009
Romero, L. M., and Reed, J. M. (2005). Collecting baseline corticosterone samples in the field: is under 3 min good enough? Comp. Biochem. Physiol. A Mol. Integr. Physiol. 140, 73–79. doi: 10.1016/j.cbpb.2004.11.004
Salmon, P., and Burraco, P. (2022). Telomeres and anthropogenic disturbances in wildlife: A systematic review and meta-analysis. Molecular Ecology 31, 6018–6039. doi: 10.1111/mec.16370
Schaefer, R., and Colombelli-Négrel, D. (2021). Behavioural and heart rate responses to stressors in two populations of little penguins that differ in levels of human disturbance and predation risk. Ibis 163, 858–874. doi: 10.1111/ibi.12925
Scheun, J., Miller, R. J., Ganswindt, A., Waller, L. J., Pichegru, L., Sherley, R. B., et al. (2021). Urofaecal glucocorticoid metabolite concentrations in African penguin (Spheniscus demersus) chick populations experiencing different levels of human disturbance. Conserv. Physiol. 9:coab078. doi: 10.1093/conphys/coab078
Schmidt, A. E., Ballard, G., Lescroël, A., Dugger, K. M., Jongsomjit, D., Elrod, M. L., et al. (2021). The influence of subcolony-scale nesting habitat on the reproductive success of Adélie penguins. Sci. Rep. 11:15380. doi: 10.1038/s41598-021-94861-7
Spée, M., Beaulieu, M., Dervaux, A., Chastel, O., Le Maho, Y., and Raclot, T. (2010). Should I stay or should I go? Hormonal control of nest abandonment in a long-lived bird, the Adélie penguin. Horm. Behav. 58, 762–768. doi: 10.1016/j.yhbeh.2010.07.011
Thierry, A.-M., Massemin, S., Handrich, Y., and Raclot, T. (2013). Elevated corticosterone levels and severe weather conditions decrease parental investment of incubating Adélie penguins. Horm. Behav. 63, 475–483. doi: 10.1016/j.yhbeh.2012.12.011
Tin, T., Lamers, M., Liggett, D., Maher, P. T., and Hughes, K. A. (2014). “Setting the scene: human activities, environmental impacts and governance arrangements in Antarctica” in Antarctic futures: human engagement with the Antarctic environment. eds. T. Tin, D. Liggett, P. T. Maher, and M. Lamers (Dordrecht: Springer)
Tin, T., Summerson, R., and Yang, H. R. (2016). Wilderness or pure land: tourists’ perceptions of Antarctica. Polar J. 6, 307–327. doi: 10.1080/2154896X.2016.1252545
Viblanc, V. A., Smith, A. D., Gineste, B., and Groscolas, R. (2012). Coping with continuous human disturbance in the wild: insights from penguin heart rate response to various stressors. BMC Ecol. 12:10. doi: 10.1186/1472-6785-12-10
Villanueva, C., Walker, B. G., and Bertellotti, M. (2012). A matter of history: effects of tourism on physiology, behaviour and breeding parameters in Magellanic penguins (Spheniscus magellanicus) at two colonies in Argentina. J. Ornithol. 153, 219–228. doi: 10.1007/s10336-011-0730-1
Villanueva, C., Walker, B. G., and Bertellotti, M. (2014). Seasonal variation in the physiological and behavioral responses to tourist visitation in Magellanic penguins. J. Wildl. Manag. 78, 1466–1476. doi: 10.1002/jwmg.791
Vleck, C. M., and Vleck, D. (2002). Physiological condition and reproductive consequences in Adélie Penguins1. Integr. Comp. Biol. 42, 76–83. doi: 10.1093/icb/42.1.76
Walker, B. G., Boersma, P. D., and Wingfield, J. C. (2005). Physiological and behavioral differences in Magellanic penguin chicks in undisturbed and tourist-visited locations of a Colony. Conserv. Biol. 19, 1571–1577. doi: 10.1111/j.1523-1739.2005.00104.x
Walker, B. G., Dee Boersma, P., and Wingfield, J. C. (2006). Habituation of adult Magellanic penguins to human visitation as expressed through behavior and corticosterone secretion. Conserv. Biol. 20, 146–154. doi: 10.1111/j.1523-1739.2005.00271.x
Weimerskirch, H., Shaffer, S. A., Mabille, G., Martin, J., Boutard, O., and Rouanet, J. L. (2002). Heart rate and energy expenditure of incubating wandering albatrosses: basal levels, natural variation, and the effects of human disturbance. J. Exp. Biol. 205, 475–483. doi: 10.1242/jeb.205.4.475
Widmann, M., Kato, A., Raymond, B., Angelier, F., Arthur, B., Chastel, O., et al. (2015). Habitat use and sex-specific foraging behaviour of Adélie penguins throughout the breeding season in Adélie land. East Antarctica. Mov. Ecol. 3:30. doi: 10.1186/s40462-015-0052-7
Wikelski, M., and Cooke, S. J. (2006). Conservation physiology. Trends Ecol. Evol. 21, 38–46. doi: 10.1016/j.tree.2005.10.018
Wingfield, J. C., Maney, D. L., Breuner, C. W., Jacobs, J. D., Lynn, S., Ramenofsky, M., et al. (1998). Ecological bases of hormone—behavior interactions: the emergency life history stage. Integr. Comp. Biol. 38, 191–206. doi: 10.1093/icb/38.1.191
Wingfield, J. C., Vleck, C. M., and Moore, M. C. (1992). Seasonal changes of the adrenocortical response to stress in birds of the Sonoran Desert. J. Exp. Zool. 264, 419–428. doi: 10.1002/jez.1402640407
Woehler, E. J., Ainley, D., and Jabour, J. (2014). “Human impacts to Antarctic wildlife: predictions and speculations for 2060,” in Antarctic Futures. eds. T. Tin, D. Liggett, P. T. Maher, and M. Lamers (Dordrecht: Springer), 27–60.
Keywords: seabird, Pygoscelis adeliae, human activity, stress response, stress-induced corticosterone, basal corticosterone, disturbance, Antarctica
Citation: Marciau C, Raclot T, Bestley S, Barbraud C, Delord K, Hindell MA, Kato A, Parenteau C, Poupart T, Ribout C, Ropert-Coudert Y and Angelier F (2023) Body condition and corticosterone stress response, as markers to investigate effects of human activities on Adélie penguins (Pygoscelis adeliae). Front. Ecol. Evol. 11:1099028. doi: 10.3389/fevo.2023.1099028
Edited by:
Mary Ann Ottinger, University of Houston, United StatesReviewed by:
Marcelo Bertellotti, CONICET Centro de Estudios de Sistemas Marinos (CESIMAR), ArgentinaGregory J. Robertson, Environment and Climate Change Canada (ECCC), Canada
Copyright © 2023 Marciau, Raclot, Bestley, Barbraud, Delord, Hindell, Kato, Parenteau, Poupart, Ribout, Ropert-Coudert and Angelier. This is an open-access article distributed under the terms of the Creative Commons Attribution License (CC BY). The use, distribution or reproduction in other forums is permitted, provided the original author(s) and the copyright owner(s) are credited and that the original publication in this journal is cited, in accordance with accepted academic practice. No use, distribution or reproduction is permitted which does not comply with these terms.
*Correspondence: Coline Marciau, Y29saW5lLm1hcmNpYXVAZ21haWwuY29t