- 1Department of Molecular Genetics and Biotechnology, Yuriy Fedkovych Chernivtsi National University, Chernivtsi, Ukraine
- 2Department of Cell Biology, Institute of Biological Sciences, Maria Curie-Skłodowska University, Lublin, Poland
- 3Institute of Biological Basis of Animal Production, Faculty of Biology, Animal Sciences and Bioeconomy, University of Life Sciences in Lublin, Lublin, Poland
Introduction: Significant losses of honey bee colonies have been observed worldwide in recent decades. Inadequate nutrition is considered to be one of the factors that can reduce honey bee resistance to abiotic and biotic environmental stresses. Accordingly, we assessed the impact of food composition on worker bee survival.
Methods: Bees in cages were fed six different diets, and then their survival, levels of thiobarbituric acid reactive substances and protein carbonyl groups, catalase and lysozyme activities were evaluated.
Results and Discussion: After 17 days of feeding, the lowest mortality was observed in the group of bees that received sucrose solution with the addition of willow pollen or artificial rapeseed beebread or artificial willow beebread (diets 4–6). The highest mortality was found in bees that consumed only sucrose solution (diet 1) or the sucrose solution supplemented with a mixture of amino acids (diet 2), which can be explained by the lack of vitamins and microelements in these diets. In the group of bees that received the sucrose solution with rapeseed pollen (diet 3), mortality was intermediate. To check whether the decrease in insect survival could be related to oxidative damage, we evaluated biomarkers of oxidative stress. Consumption of pollen (diets 3 and 5) and artificial beebread (diets 4 and 6) enhances protein carbonylation in worker bees. Feeding bees artificial beebread also resulted in increase in lipid peroxidation and catalase activity, which is probably due to the presence of hydrogen peroxide in the honey contained in beebread. Remarkably, the increase in biomarkers of oxidative stress was not accompanied by adverse but positive effects on insect survival. A lack of amino acids and proteins in the diet 1 did not cause oxidative stress, but led to an increase in lysozyme activity in hemolymph, a biomarker of immune system status. In conclusion, we believe that the increase in oxidative stress biomarkers we found do not indicate oxidative damage, but rather reflect the changes in redox balance due to consumption of certain dietary options.
Introduction
The western honey bee, Apis mellifera L. (Hymenoptera: Apidae), is an important pollinator in natural and agricultural ecosystems (Potts et al., 2010; Garibaldi et al., 2017; Khalifa et al., 2021). Significant bee colony losses have been observed worldwide in recent decades (Gray et al., 2019, 2022). This issue has drawn particular attention to identifying factors that negatively affect honey bee health, and it is believed that poor nutrition may be one of them. A deficiency or a disturbed composition of dietary components can weaken the resistance of bees to stressors of abiotic and biotic nature and make them more sensitive to adverse weather conditions, infectious diseases, and the effects of environmental contaminants (Brodschneider and Crailsheim, 2010; Goulson et al., 2015; Tosi et al., 2017; Ptaszyńska et al., 2018; Wright et al., 2018; Branchiccela et al., 2019; Dolezal et al., 2019; Negri et al., 2019; Tong et al., 2019; Olate-Olave et al., 2021).
For normal activity of bee colony, all basic nutrients, i.e., proteins, fats, carbohydrates, vitamins, and minerals should be available (Paoli et al., 2014; de Groot et al., 2021). The nectar and honey derived from it are the main sources of carbohydrates, while pollen provides necessary proteins and lipids. Pollen also contains various carbohydrates, such as fructose, glucose, sucrose, polysaccharides, as well as vitamins, minerals, and polyphenolic compounds (Čeksterytė et al., 2008; Rzepecka-Stojko et al., 2015; Bogdanov, 2016; Arathi et al., 2018; Radev, 2018).
Honey bees, unlike other insects, are able to accumulate their own food reserves in the form of honey and beebread, a mixture of pollen and nectar or honey that also include some enzymes and micro-organisms. Beebread contains a large amount of proteins, lipids, free sugars, essential amino acids, mono- and polyunsaturated fatty acids, etc., and is the main food source for worker bees and larvae. The nutritional and biological qualities of beebread vary widely depending on the diversity of flora and the season of bee pollen collection (Urcan et al., 2017; Wright et al., 2018; Bakour et al., 2019).
The results of artificial feeding show that the nutrient composition of diets affects both the physiological state of individual bees and the health of the bee colony as a whole (Branchiccela et al., 2019; Castelli et al., 2020). Such parameters as the lifespan of worker bees (Di Pasquale et al., 2013; Arien et al., 2020), the size of the acini of the hypopharyngeal glands, the protein content in the hemolymph and intestines, the activity of glucooxidase (Alaux et al., 2010), the level of gene expression (De Grandi-Hoffman et al., 2021), etc. depend on the pollen composition. Nevertheless, the influence of pollen from different plant species on the lifespan of worker bees and the health of bee colonies is still insufficiently studied and requires further research.
In all aerobic organisms, even under optimal environmental conditions, reactive oxygen species (ROS), such as hydrogen peroxide, superoxide, and hydroxyl radicals, etc., are constantly generated in various compartments as an inevitable part of cell life. In animals, ROS are produced by respiratory chain in mitochondria and also as byproducts of different metabolic pathways (Barja, 1999; Drahota et al., 2002; Miwa and Brand, 2005; Murphy, 2009; Halliwell and Gutteridge, 2015). ROS levels are under the strict control of a complex system of antioxidant protection, which includes both enzymes and small-molecule non-enzymatic compounds, which contribute to ROS-splitting and reparation of oxidative damage (Corona and Robinson, 2006; Halliwell and Gutteridge, 2015; Jakubczyk et al., 2020; Irato and Santovito, 2021). Various external abiotic (extreme temperatures, dehydration, environmental pollutants, and radiation) and biotic (pathogens attack) stress factors induce excessive production of ROS causing an oxidative stress, i.e., imbalance between ROS formation and antioxidative defense mechanisms and leading to oxidative damage of proteins, lipids, and DNA, which in turn results in numerous violations of physiological functions and even cell death (Roelofs et al., 2008; Birben et al., 2012; Nikolenko et al., 2012; Halliwell and Gutteridge, 2015; Cervoni et al., 2017). Especially, a correlation between antioxidant system activity and longevity has been found in insects, including bees (Phillips et al., 1989; Arking et al., 2000; Corona et al., 2005).
In eukaryotes, ROS not only damage the cell, but also play numerous beneficial roles, serving as tools to combat infectious agents and as signaling molecules involved in the regulation of stress response, senescence, and immunity (Corona et al., 2005; Volkov et al., 2006; Aurori et al., 2013; Scialò et al., 2016; Cervoni et al., 2017; Foyer et al., 2017; Orčić et al., 2017).
Honey, pollen, and beebread contain both pro- and antioxidant compounds, the composition and concentration of which vary depending on the plant species that the honeybees pollinate (Čeksterytė et al., 2008; Rzepecka-Stojko et al., 2015; Bogdanov, 2016). Accordingly, it is to be expected that the level of ROS in bees can depend on the composition of the forage. However, very little is known about the relationship between the type of food, immunocompetence, the state of the antioxidant system, and oxidative stress in bees (Alaux et al., 2010; Li et al., 2012; Wheeler and Robinson, 2014). Therefore, the aim of our work is to evaluate the effect of the consumed feed (sucrose solution supplemented with amino acids, or pollen and beebread of rapeseed and willow) on survival, the state of the antioxidant system (lipid peroxidation, protein carbonylation, and catalase activity) and parameters of immunity (lysozyme activity) in honey bees.
Materials and methods
Experimental conditions and feeding of bees
The research was conducted in June 2018. Worker bees used for the experiment were obtained from three colonies of Apis mellifera carnica from the experimental apiary of the University of Life Sciences in Lublin (Poland). Combs with sealed brood in the late stages of pupal development were transferred from the hives to a thermostat (34°C and 60–70% relative humidity) and maintained until imago hatching. Bees hatched from the combs within 10 h were collected and reared in groups of 40 individuals in cages (12 cm × 12 cm × 3.5 cm) at 24°С and 60% relative humidity.
Firstly, all bees were fed 50% sucrose solution for 2 days for acclimatization. After that, the bees were fed ad libitum for 17 days on one of the six diets: diet 1–50% solution of sucrose (SS, control group); diet 2–10% solution of “Aminosteril N-HEPA 8%” (Fresenius Kabi Deutschland GmbH; 100 ml of “Aminosteril N-HEPA 8%” contain: L-Isoleucine 1.04 g, L-Leucine 1.309 g, L-Lysine monoacetate 0.971 g, L-Lysine 0.688 g, L-Methionine 0.11 g, N-Acetylcysteine 0.07 g, L-Cysteine 0.052 g, L-Phenylalanine 0.088 g, L-Threonine 0.44 g, L-Tryptophan 0.07 g, L-Valine—1.008 g, Arginine 1.072 g, L-Histidine 0.28 g, Aminoacetic acid 0.582 g, L-Alanine 0.464 g, L-Proline 0.573 g, L-Serine 0.224 g, and Glacial acetic acid 0.442 g) in SS; diet 3–10% rapeseed pollen in SS; diet 4–10% artificial rapeseed beebread (rapeseed pollen mixed with honey and fermented for 2 days) in SS; diet 5–10% willow pollen in SS; and diet 6–10% artificial willow beebread in SS. Rapeseed and willow pollen were obtained, respectively, from organic farming or nature conservation area. The amount of feed consumed and the number of dead bees were measured every 2 days.
Biochemical measurements
For biochemical measurements, the bees were taken on the 15th day of the feeding experiment, flash frozen in liquid nitrogen, and stored at −70°C. Biochemical parameters were assayed separately for head and abdomen. The frozen bees were dissected on ice, and then, for one sample, heads and abdomens from 12 individuals were pooled, ground in liquid nitrogen, and homogenized at 0–4° C using a Heildolph high-speed homogenizer (Germany) and different extraction buffers depending on the parameters examined.
The lipid peroxidation was assayed in head and abdomen by measuring the content of thiobarbituric acid reactive substances (TBARS; μmol/kg fresh weight; Placer et al., 1966) using RIPA-extraction buffer (150 mM NaCl, 1% Triton X-100, 0.5% Sodium deoxycholate, 0.1% SDS, 2 mM EDTA, 50 mM NaF, 50 mМ Tris HCl, and pH 7.4; Margotta et al., 2018). 100–120 mg of frozen tissues were homogenized at 0–4° C in 400 μl of RIPA-buffer, incubated on ice for 10 min, and centrifuged for 15 min at 12,000 g, 4°C. The supernatant was collected and the pellet was re-suspended in 400 μl of RIPA-buffer and centrifuged. Both supernatants were combined and mixed with 800 μl of 0.6% 2-thiobarbituric acid in 20% trichloroacetic acid. The control tube contained 800 μl of RIPA-buffer and 800 μl 0.6% TBA in 20% TCA. The sample and control tubes were incubated at 95°C for 60 min, cooled on ice, and centrifuged for 15 min at 12,000 g. The optical density of the obtained supernatants was determined at wavelengths of 532 and 600 nm and used to calculate the TBARS content.
The content of protein carbonyl groups (PCG; nmol/mg protein), a biomarker of protein carbonylation, was determined in abdomen using the DNPH (2,4-dinitrophenylhydrazine) method (Reznick and Packer, 1994). 100–120 mg of tissues ground in liquid nitrogen were mixed with 800 μl of extraction buffer (100 mM potassium phosphate, pH 7.4, 1 mM PMSF, and 1 mM EDTA), additionally homogenized on ice, and centrifuged for 20 min at 14,000 g, 4°C. 400 μl of to the obtained supernatant was added to (і) a sample tube containing 800 μl of 10 mM DNPH dissolved in 2 M HCl and (ii) a control tube containing 800 μl of 2 M HCl. After 60 min of incubation in the dark at 25°C, 800 μl of 40% TCA was added to both sample and control tubes. After 5 min of incubation on ice, the tubes were centrifuged for 15 min at 10,000 g. The pellets were washed three times with 1 ml of ethanol/ethyl acetate (1:1) mixture followed by centrifugation. Finally, the pellets were dissolved in 1 ml of 6 M guanidinium chloride for 30 min in the dark. The optical density of samples at 370 nm was determined.
Activity of catalase (CAT) was evaluated in head and abdomen by measuring the decrease in hydrogen peroxide content in the samples, applying the previously described method (Korolyuk et al., 1988; Buzduga et al., 2018). 100–120 mg of tissues ground in liquid nitrogen were mixed with six volumes of extraction buffer (100 mM potassium phosphate, pH 7.4, 1 mM PMSF, and 1 mM EDTA), additionally homogenized on ice, and centrifuged for 20 min at 14,000 g, 4°C. The supernatants were used for CAT activity estimation. Two samples, a blank and an experimental, were prepared. 100 μl of protein extract was added to 1.9 ml of reaction buffer containing 100 mM Tris–HCl (pH 6.8) and 70 mM H2O2. The mixture was stirred briefly, and 1 ml was immediately transferred to a tube (blank sample) containing 0.5 ml of a 4% solution of ammonium molybdate, which resulted in termination of reaction due to formation of colored complexes between ammonium molybdate and hydrogen peroxide. The remaining reaction mixture was incubated for 1 min at 25°C; thereafter the reaction was stopped by the addition of 0.5 ml of a 4% ammonium molybdate solution. The optical density of the samples at 410 nm was measured, and the content of hydrogen peroxide was calculated using a calibration curve. CAT activity was expressed in micromoles of hydrogen peroxide spited per minute per milligram of protein (μmol/min mg−1 protein).
The activity of lysozyme in hemolymph was quantified by the diffusion pit method (Snyder and Fritsch, 1984) with Lysozyme from chicken egg white (Sigma-Aldrich, United States) as the standard. Hemolymph was collected from bees as described previously (Borsuk et al., 2017). The samples were kept at −20°C until further analysis.
Protein concentration in the samples was determined according to the method of Bradford (1976).
Statistics
Each diet feeding experiment was performed three times for four pools of 40 bees per cage (i.e., for a total of 12 bee cages or 480 bees per diet). Biochemical measurements were performed in four analytical replicates for each feeding experiment. Differences in survival were determined using the log-rank test for the Kaplan-Meir survival curve. For amount of food consumed by bees and lysozyme activity, the significance of differences between control and each treatment was assessed using Duncan’s one-way ANOVA test. For TBARS and PCG content and CAT activity, the Kruskal-Wallis test followed by the Mann–Whitney test was applied. p values less than 0.05 were considered statistically significant.
Relationships between survival of bees and biochemical parameters were evaluated using no parametric Spearman correlation analysis (Zar, 1996). All tests were performed with the statistical software Statistica 12.5.
Results
Consumption of different diets and survival rate of worker bees
In our experiment, the consumption of six dietary options by worker bees was compared. It was found that the bees consumed the least amount of sucrose solution supplemented with the amino acid-containing drug “Aminosteril,” while solutions with rapeseed pollen or beebread as well as the control sucrose solution were most accepted (Figure 1).
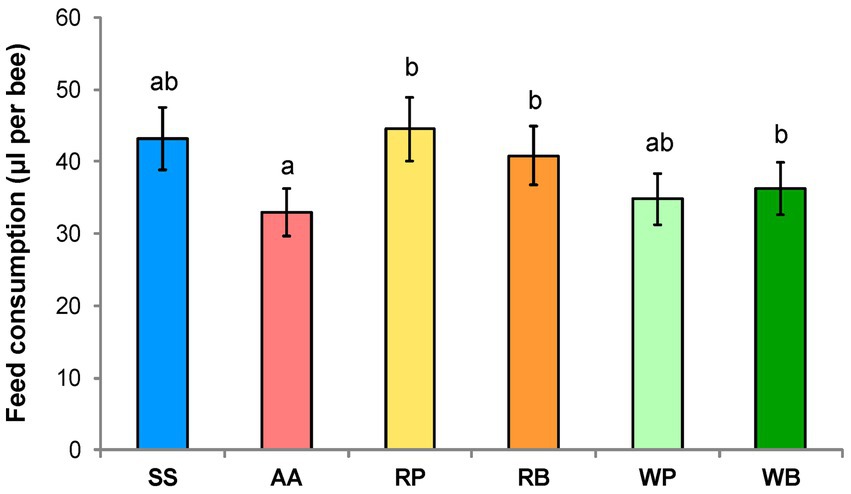
Figure 1. Consumption of different diets (μl per one bee). SS, sucrose solution (control group); AA, sucrose solution + amino acids; RP, sucrose solution + rapeseed pollen; RB, sucrose solution + rapeseed beebread; WP, sucrose solution + willow pollen; and WB, sucrose solution + willow beebread. Different letters indicate significant differences between the values (p < 0.05).
The survival rate of the worker bees was evaluated depending on the composition of the different diets. The obtained data show that in the first 6–8 days after the start of the experiment, the survival rate of the bees in the control group, which only consumed sucrose solution (diet 1), was the highest (Figure 2). At the same time, the highest mortality was observed in individuals that consumed sucrose solution with the addition of rapeseed or willow pollen. However, with increasing duration of the experiment, a gradual increase in the mortality of bees receiving only sucrose solution or sucrose solution with the addition of amino acids mixture was observed. At the end, on day 18 from the beginning of the experiment, these last two feeding groups had the highest mortality, 88 and 91%, while the bees consuming the sugar solution supplemented with willow beebread, willow pollen, or rapeseed pollen showed mortality of 66, 70, and 72%. The experimental group that received rapeseed pollen had an intermediate mortality rate of 81%.
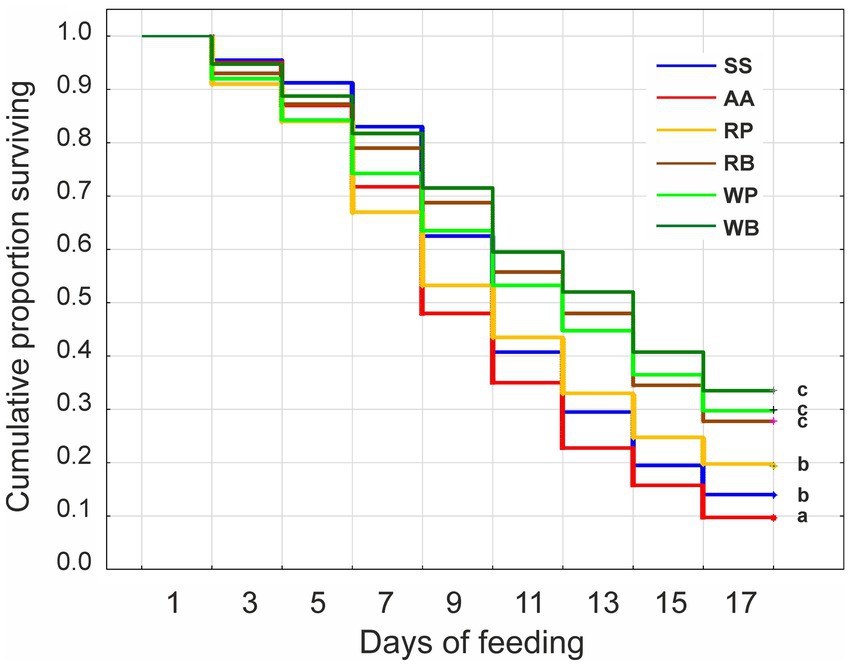
Figure 2. Kaplan–Meier survival curves for worker bees fed different diets for 17 days. The administration of the diets was started 2 days after the hatching of the bees. SS, sucrose solution (control group); AA, sucrose solution + amino acids; RP, sucrose solution + rapeseed pollen; RB, sucrose solution + rapeseed beebread; WP, sucrose solution + willow pollen; and WB, sucrose solution + willow beebread. Different letters indicate significant differences between the values (p < 0.05).
Evaluation of stress biomarkers and activity of protective enzymes
Determination of TBARS content in the tissues of the head and abdomen revealed that this biomarker varies depending on the diet used. In particular, in the tissues of the head of bees whose diet included beebread, the TBARS content was the highest compared to other types of diet (Figure 3). At the same time, the content of TBARS when consuming rapeseed beebread was higher than that of willow beebread. In bees that were fed a sucrose solution with the addition of pollen or an amino acid mixture, the values of the biomarker studied were at the control level. A similar pattern was found in insect abdominal tissues. In general, the content of TBARS in the tissues of the head was higher than in the abdomen.
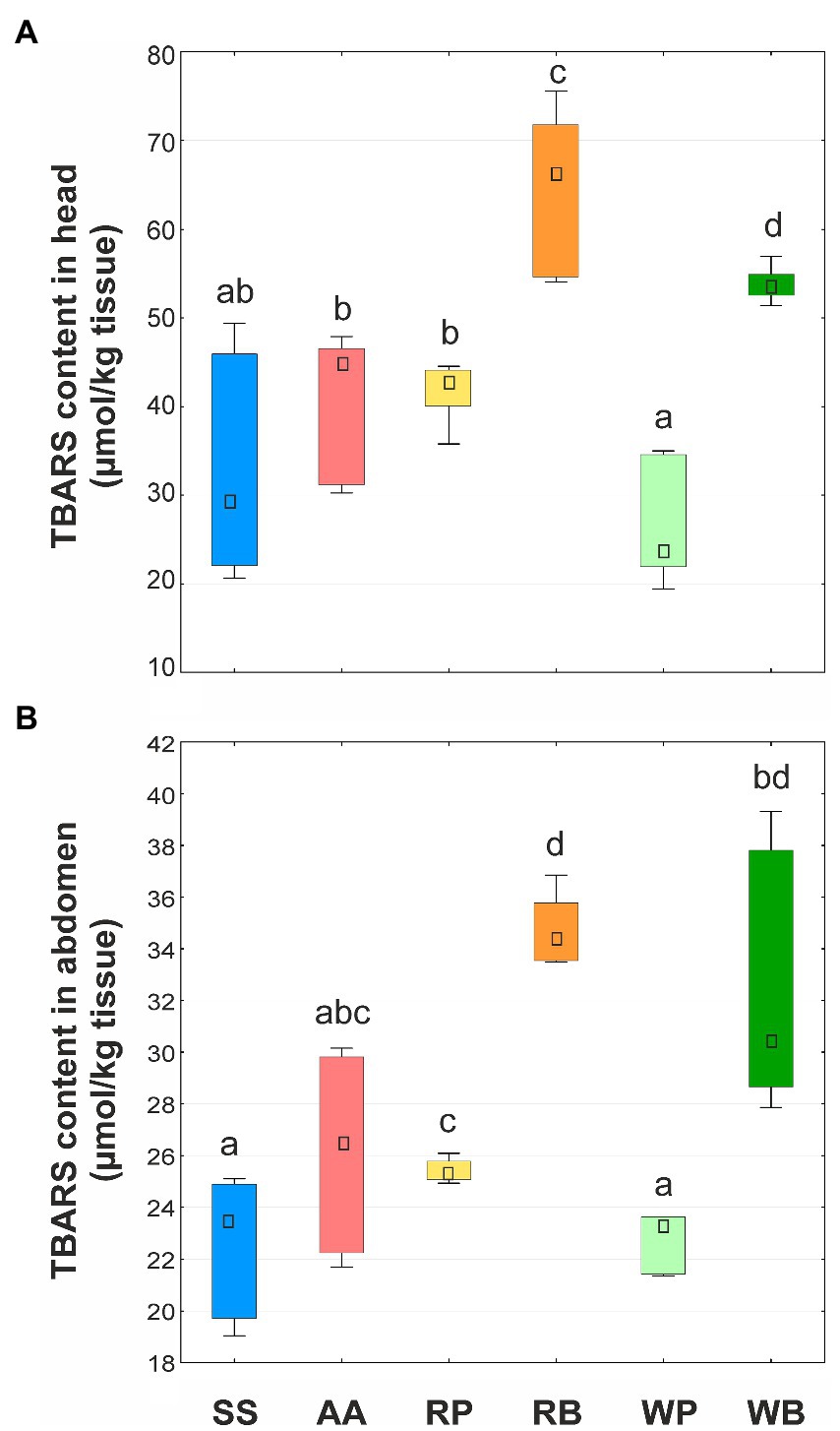
Figure 3. Content of thiobarbituric acid reactive substances (TBARS, μmol/kg tissue) in heads (A) and abdomens (B) of worker bees consuming different diets. SS, sucrose solution (control group); AA, sucrose solution + amino acids; RP, sucrose solution + rapeseed pollen; RB, sucrose solution + rapeseed beebread; WP, sucrose solution + willow pollen; and WB, sucrose solution + willow beebread. Boxes show first and third interquartile ranges with the dot denoting median. Different letters indicate significant differences between the values (p < 0.05).
The content of PCG was determined only in the abdominal tissues of bees (Figure 4). It was shown that in insects that were fed a sucrose solution supplemented with pollen or beebread, this biomarker was higher than in the control group. At the same time, the level of protein carbonylation was higher in bees consuming willow beebread than rapeseed beebread. The lowest level of protein carbonylation was found in the insects that received a mixture of amino acids.
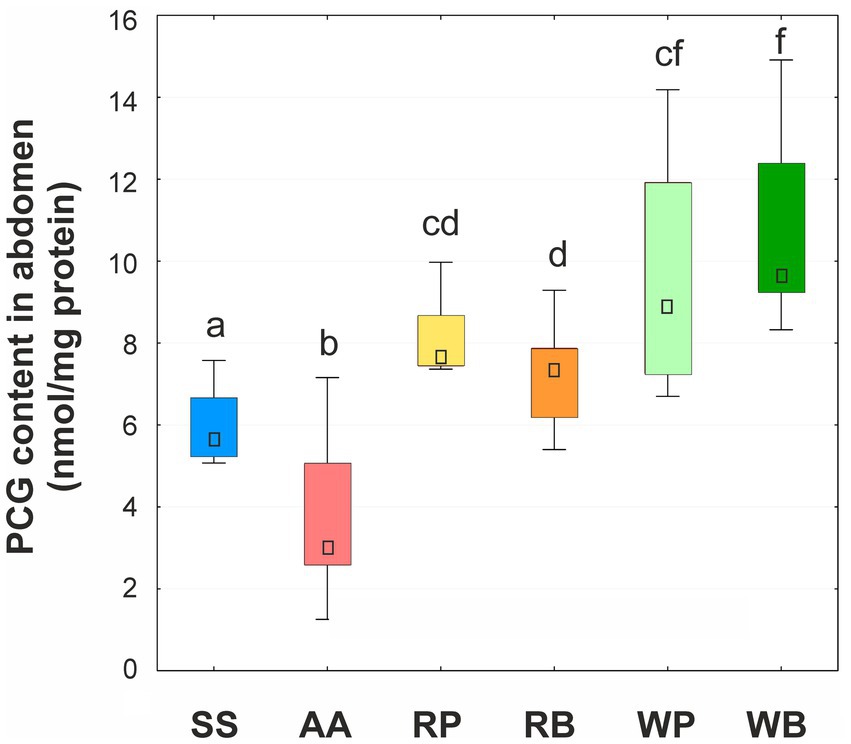
Figure 4. Content of protein carbonylic groups (PCG, nmol/mg protein) in abdomens of worker bees consuming different diets. SS, sucrose solution (control group); AA, sucrose solution + amino acids; RP, sucrose solution + rapeseed pollen; RB, sucrose solution + rapeseed beebread; WP, sucrose solution + willow pollen; WB, sucrose solution + willow beebread. Boxes show first and third interquartile ranges with the dot denoting median. Different letters indicate significant differences between the values (p < 0.05).
Measurement of CAT activity showed its increase in the tissues of the head of bees receiving a sucrose solution with the addition of an amino acid mixture, while the activity of the enzyme remained at the control level when pollen or beebread was consumed. However, in bees whose diet included beebread, the activity of CAT was about 14% higher than in bees that fed on pollen (Figure 5). In the tissues of abdomen, the activity of CAT was 3–4 times higher, depending on the applied diet, than in the tissues of head. In the abdomen, the highest activity of the enzyme was found in bees of the control group, whereas the lowest in those that consumed pollen. In the insects that received a diet containing a mixture of amino acids or beebread, the activity of CAT was higher than in bees consuming pollen, but lower (mixture of amino acids or rapeseed beebread) or equal (willow beebread) as in bees fed sucrose solution. Hence, similar to head tissues, consumption of beebread led to an increase in of CAT activity in abdomen.
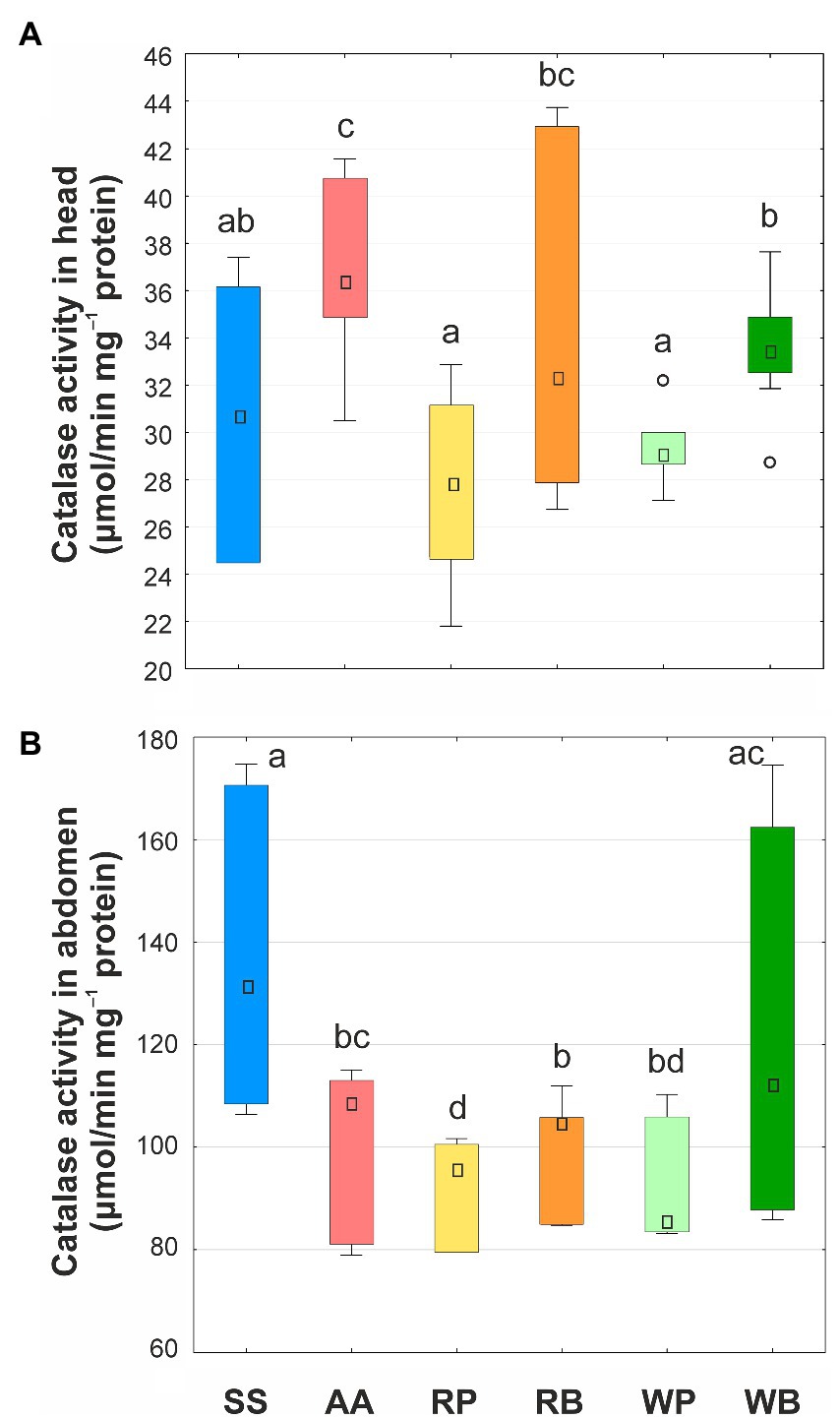
Figure 5. Catalase activity (μmol/min mg−1 protein) in heads (A) and abdomens (B) of worker bees consuming different diets. SS, sucrose solution (control group); AA, sucrose solution + amino acids; RP, sucrose solution + rapeseed pollen; RB, sucrose solution + rapeseed beebread; WP, sucrose solution + willow pollen; WB, sucrose solution + willow beebread. Boxes show first and third interquartile ranges with the dot denoting median. Whiskers encompass 90% of individuals, beyond which outliers are represented by circles. Different letters indicate significant differences between the values (p < 0.05).
The activity of lysozyme in the hemolymph of bees of the control group that consumed sucrose solution was found to be 4–9 times higher than in bees that received other diet variants. The lowest lysozyme activity was found in bees receiving willow pollen or beebread, but the difference in this parameter for different diets (except for the control group) was not statistically significant (Figure 6).
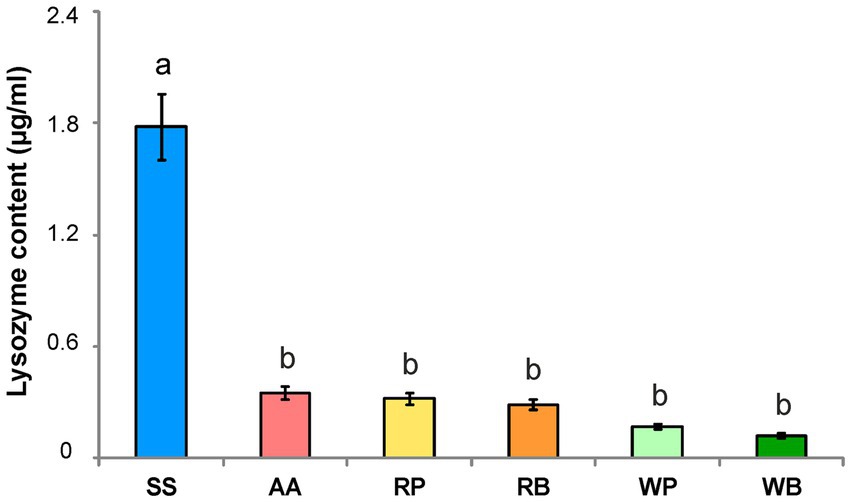
Figure 6. Lysozyme content (μg/ml) in the hemolymph of worker bees consuming different diets. SS, sucrose solution (control group); AA, sucrose solution + amino acids; RP, sucrose solution + rapeseed pollen; RB, sucrose solution + rapeseed beebread; WP, sucrose solution + willow pollen; WB, sucrose solution + willow beebread. Different letters indicate significant differences between the values (p < 0.05).
Correlation analysis
In order to reveal a possible relationship between survival of bees and biomarkers of oxidative stress, we applied correlation analysis (Table 1). A strong positive statistically significant relationship was found only in two cases: (i) the survival of bees when consuming different diets was correlated with the level of protein carbonylation and (ii) the level of lipid peroxidation in tissues of head correlated with that in abdomen.
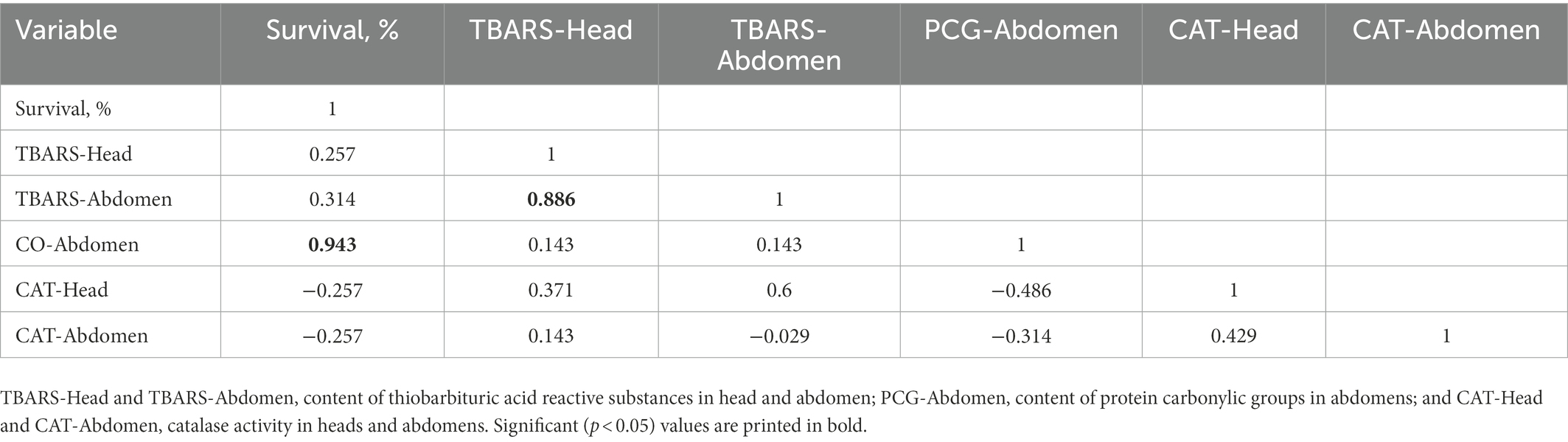
Table 1. Spearman rank order correlations among survival of bees and biomarkers of oxidative stress.
Discussion
Effect of diet on the lifespan of worker bees
In nature, foraging honey bees collect nectar and pollen from various flowers to provide the necessary nutrients for the colony. The main source of energy for bees are carbohydrates (fructose, glucose, sucrose, etc.), which are part of flower nectar and honey obtained from it. Also, a mandatory components of the diet must be pollen and beebread obtained from it, which provide bees with amino acids (especially with essential amino acids—EAAs), lipids, sterols, vitamins, and minerals (De Groot, 1953; Day et al., 1990; Herbert, 1992; Cook et al., 2003; Paoli et al., 2014; Bogdanov, 2016). Behavioral castes of adult worker honeybees differ in dietary requirements for EAAs/proteins and carbohydrates. In particular, young nurse bees need relatively more EAAs, while older forager bees, which have increased energy expenditure due to flight, consume relatively more carbohydrates (Crailsheim, 1990; Crailsheim et al., 1992; Paoli et al., 2014).
In our feeding studies, we determined the effect of different diets on lifespan, an important integral indicator reflecting the physiological state of bees. It was found that using a 50% aqueous sucrose solution (diet 1) to feed young worker bees ensured the highest survival rate within 6–8 days from the start of the experiment. Thereafter, however, the survival rate of the insects consuming this diet decreased and was the lowest on the 16th day after the start of the experiment. This effect can be explained by the gradual depletion of the pool of amino acids and other nutrients (vitamins and mineral elements) when using for feeding a sucrose solution without additives. It should be noted that adding a mixture of amino acids (diet 2) to the sucrose solution did not increase the survival rate of the bees. Moreover, when using this diet, the highest mortality compared to other feeding variants was observed starting from the 10th day (Figure 2). These results are consistent with the data that an optimal ratio between carbohydrate and protein content in the feed is necessary for high survival of worker bees, while consuming diets high in EAAs (Paoli et al., 2014) or proteins (Bouchebti et al., 2022) leads to a shortened life span of worker honey bees. Accordingly, it can be assumed that the concentration of amino acids we used in the diet 2 was too high and thus did not lead to an increase in the bee survival rate compared to the control group. Our results also show that the consumption rate of diet 2 was lower than that of the other diet variants (Figure 1), indicating that bees were trying to avoid this diet.
The highest survival rate of worker bees in our experiments was observed when consuming sucrose syrup with added pollen or beebread of rapeseed (Brassica napus) or willow (Salix spp.). These data further support the results of Di Pasquale et al. (2013, 2016) who found that adding pollen to sucrose syrup increased bee survival. However, Li et al. (2019) found that pollen supplementation did not significantly affect the lifespan of bees as long as their gut microbiome was not disrupted.
In our study, we chose for comparison pollen of rapeseed and willow since these plant species are ubiquitous in the Northern Hemisphere and represent an important source of nectar and pollen for honey bees and other pollinators (Čeksterytė et al., 2008; Ostaff et al., 2015). In particular, many species of willow begin flowering very early in spring, as the first wild pollinators become active following winter, and stop flowering by mid-May. A later-flowering willow species normally begins flowering in mid-May and stops flowering by mid-June (Ostaff et al., 2015). If willow pollen is particularly important for bees in spring, in the absence of other sources of amino acids and protein, the value of rapeseed pollen increases in summer, especially when bees are used to pollinate this crop. For instance, in Lithuania, beebread, collected in spring, contained almost equal parts of rapeseed and willow pollen, 45.1 and 41.8%, respectively. The content of rapeseed pollen in summer beebread was significantly higher, from 61.7 to 78.7%, while the content of willow pollen decreased, from 17.9 to 5.6% (Čeksterytė et al., 2008).
The nutritional value of pollen from different plant species depends on the protein and amino acid content, with the EAA content being particularly important (Day et al., 1990; Cook et al., 2003). In choice-test experiments, bees preferred pollen of rapeseed over that of field bean (Vicia faba) because rapeseed pollen contained a greater proportion of the most EAA, suggesting that rapeseed pollen is of greater nutritional quality (Cook et al., 2003). Our current results show that willow pollen has a nutritional value similar to that of rapeseed pollen, which agree well with the finding that positive influence of pollen consumption on honey bee health and survival was mostly independent of the pollen type (with the exception of Cistus spp. and Zea mais pollen; Di Pasquale et al., 2013, 2016).
It should also be noted that in our experiments, using rapeseed or willow beebread instead of pollen for feeding resulted in an increase in bee survival, although this difference was insignificant in the case of willow. This result can be explained by the fact that the artificial beebread contained honey in addition to pollen. Also, the beebread was fermented for 2 days (see the section Materials and methods), which could additionally affect its assimilation by insects. Hence, the exact mechanism of the beneficial effect of artificial beebread on bee survival compared to pollen is still unclear and needs further clarification.
Oxidative stress biomarkers in bees consuming of different diets
Previous studies have shown that extended longevity in insects correlates with enhanced expression of antioxidative defense system genes and increased activities of antioxidant enzymes, while defects of antioxidant system results in lifespan reduction (Phillips et al., 1989; Arking et al., 2000; Corona et al., 2005). Accordingly, it is believed that further investigation of ROS metabolism and antioxidative defense mechanisms can contribute to our understanding of bee survival and conservation of this species (Orčić et al., 2017). However, the effect of diet on the antioxidative status of worker bees is still poorly understood (Li et al., 2012; Wheeler and Robinson, 2014). Respectively, to assess the cell damage caused by production of ROS, we have measured two oxidative stress-related parameters, TBARS and PCG levels, which reflect membrane lipid peroxidation and the oxidation of cellular proteins, respectively (Levine et al., 1994; Reznick and Packer, 1994; Grotto et al., 2009; Cervoni et al., 2017).
Our results revealed that diet composition can significantly affect the intensity of lipid peroxidation in different body parts of worker bees. In particular, the consumption of sucrose solution with the addition of artificial willow and rapeseed beebread for 15 days led to an increase in the TBARS content, respectively, by 1.2–2.8 times in the head and 1.2–1.5 times in the abdomen compared to the bees receiving all other dietary variants, including pollen (Figure 3). Using the six experimental diets, a strong positive correlation was observed between TBARS levels in the head and abdomen (Table 1), indicating a systemic character of lipid peroxidation changes in different body parts of worker bees.
As mentioned above, the artificial beebread we used represents pollen mixed with honey and fermented for 2 days. Thus, the increase in lipid peroxidation in bees that consumed the artificial beebread must be caused by a compound absent from pollen but present in honey or produced in the fermentation process. Honey contains several substances that have pro-oxidant properties. The main components of honey are fructose (30–45%), glucose (24–40%), and sucrose (0.1–4.8%) those concentrations vary depending on the nectar source (White, 1957). According to our recent data, feeding worker bees a solution of fructose or a mixture of fructose and glucose resulted in an increase in lipid peroxidation compared to bees consuming glucose or sucrose solutions (Karavan et al., 2021). Besides, honey also contains 5 mM hydrogen peroxide, which has antimicrobial properties (White et al., 1963; Kwakman et al., 2010). This compound can activate oxidation of membrane phospholipids (Birben et al., 2012; Halliwell and Gutteridge, 2015). Thus, the presence of fructose and hydrogen peroxide seems to be a possible reason for the increase in lipid peroxidation in bees consuming artificial beebread.
Consumption of rapeseed beebread resulted in a slightly higher increase in lipid peroxidation than that of willow beebread. This can be explained by the different composition of the pollen of different plant species, in particular, the presence of different compounds that have pro- or antioxidant properties, such as carotenoids, flavonoids, anthocyanins, etc. (Rzepecka-Stojko et al., 2015; Bogdanov, 2016; Arathi et al., 2018; Radev, 2018). Especially, willow and rapeseed pollen differ in the content of polyunsaturated ω-3 and ω-6 fatty acids (Čeksterytė et al., 2008), tocopherols, ascorbate, polyphenols, etc. (Bogdanov, 2016).
Ingestion of rapeseed and willow pollen or beebread also resulted in an increase in another biomarker of oxidative stress and a PCG level in abdominal tissues (Figure 4). This result agrees with the data from Korayem et al. (2012), who showed an increase in hydrogen peroxide levels in worker bees in Egypt during the active season and suggested that this could be due to the intensive consumption of pollen and nectar, which contain phenolic compounds whose oxidation leads to increased ROS production (Thiboldeaux et al., 1998; Barbehenn et al., 2001; Mittapalli et al., 2007).
In contrast to the increase in the TBARS content, the PCG level in the group of bees consuming pollen of both plant species studied did not differ from that in bees obtaining beebread. That is, the pro-oxidant compounds present in beebread enhance lipid peroxidation, but do not affect the carbonylation of proteins. Measurement of PCG levels also showed that consumption of rapeseed pollen caused a greater increase in this biomarker than willow pollen. This difference can be explained by the different composition of the pollen of the two species—see above.
In our feeding experiments, PCG levels were strongly correlated with survival (Table 1), suggesting that increasing the oxidative stress biomarker does not necessarily mean deleterious consequences for the bees. We believe that in our case, increased ROS levels when consuming a particular diet may reflect an overall activation of metabolism and/or ROS-dependent cellular signaling, resulting in increased bee survival. Such an interpretation agrees with the data of Scialò et al. (2016) that increasing mitochondrial ROS improves health and extends lifespan in Drosophila.
It should also be noted that the consumption of a mixture of amino acids (diet 2) led to a decrease in protein carbonylation. To explain this phenomenon, several mechanisms can be proposed: (i) Dietary amino acids protect cellular proteins from oxidative damage by interacting with ROS; (ii) Saturation of the cellular pool of amino acids promotes the synthesis of new protein molecules instead of ROS-damaged ones that undergo accelerated proteolysis; and (iii) Some amino acids have antioxidant properties and can affect the redox balance in the cell.
Activity of catalase
Cellular levels of ROS are under the control of the antioxidant system. One of the most important antioxidant enzymes is CAT, which splits hydrogen peroxide directly and is considered an biomarker of the general condition of the antioxidant system in insects (Corona and Robinson, 2006; Badiou-Bénéteau et al., 2012). Accordingly, the activity of this enzyme was determined in the next step of our research.
Ingestion of artificial beebread was revealed to cause a moderate (14%) increase in CAT activity in the head of worker bees (Figure 5), which can be considered a protective response to the consumption of diets containing hydrogen peroxide. Interestingly, an increase in the activity of CAT was also observed when consuming a mixture of amino acids, although no signs of oxidative stress (e.g., an increase in lipid peroxidation) were observed. Hence, this last effect requires further explanation.
Our measurements showed that for all diets used, CAT activity in the abdomen was significantly higher than in the head. It can be assumed that the high activity of CAT in the abdomen is necessary for the splitting of hydrogen peroxide, the formation of which is associated with the digestion of food or some other process characteristic of this part of the body. When the bees were fed different diets, the highest activity of CAT in the abdomen was observed in the control group of bees consuming the sucrose solution. The increase in CAT activity is thought to represent a component of the stress response activated by poor nutrition, namely depletion of the amino acid pool. Accordingly, when the “Aminosteril” or another source of proteins/amino acids was added to the diet, the activity of CAT became lower. Moreover, the lowest values of CAT activity were observed when pollen was consumed, i.e., with a more balanced diet. At the same time, when feeding with beebread, the activity of CAT in the abdomen increased, similar to that in the head.
It was shown recently that during wintering activity of CAT in worker bees was increased while activity of other antioxidative enzymes, superoxide dismutase (SOD), and glutathione transferase (GST) as well as lipid peroxidation were reduced indicating a decrease in production of ROS (Orčić et al., 2017). Considering our new results, it is tempting to speculate that the increase in CAT activity is necessary for the splitting of hydrogen peroxide contained in honey, consumption of which increases in winter compared to summer.
In winter, worker bees are relatively inactive and have a longer lifespan compared to summer. Accordingly, it was hypothesized that this lengthening of lifespan is associated with a decreased ROS production observed in winter, while the high activity of bees in summer is accompanied by an increase in ROS levels, which causes oxidative stress and leads to a shortening of lifespan (Münch et al., 2008; Orčić et al., 2017). Conversely, in our experiments, the lowest values of oxidative stress biomarkers were observed in bees that consumed only sucrose solution or sucrose solution with the addition of a mixture of amino acids and had the shortest lifespan. At the same time, bees that received pollen or beebread had the longest lifespan. Consumption of these dietary variants enhances lipid peroxidation and protein carbonylation in head and/or abdominal tissues of worker bees, which, however, was not detrimental but beneficial to insect survival.
We believe that the observed increase in biomarkers of oxidative damage does not reflect intracellular redox imbalance and oxidative stress, but is a direct consequence of dietary pro-oxidant intake, which is effectively compensated by antioxidant defenses. This example shows that the biomarkers of oxidative stress should be considered with caution: certain dietary options can induce a moderate oxidative damage, but do not have a negative impact on life expectancy.
Activity of lysozyme
Reactive oxygen species (ROS) are not only damaging compounds, but are also used by insects as a weapon to fight pathogens (Nikolenko et al., 2012; Orčić et al., 2017). Accordingly, it could be expected that changes in the redox state caused by different diets would affect the immunity of bees. Therefore, at the next stage of our research, we determined the activity of lysozyme, an enzyme possessing antibacterial and antifungal activity that is synthesized in fat body (Gillespie et al., 1997; Imler and Bulet, 2005). We found that the activity of lysozyme in the hemolymph of worker bees fed sucrose solution was significantly higher than after consumption of all other diets (Figure 6). Thus, the lack of amino acids/proteins is the main factor causing the increase in lysozyme activity, while other nutrients present in pollen and beebread have less influence on this biomarker.
Previously, the influence of different diets on the immune competence of honey bees was examined. Protein deficiency caused a significant increase in the percentage of granular hemocytes in hemolymph, a significant decrease in other types and lower metabolic activity of hemocytes, indicating a reduced ability to phagocytosis. Therefore, it was supposed that haemocyte concentration might increase in bees fed without protein to compensate for their decreased activity (Szymaś and Jędruszuk, 2003; Alaux et al., 2010). Also, a relative mass of fat body, the main site of antimicrobial peptide synthesis (Imler and Bulet, 2005; Alaux et al., 2010), and activity of glucose oxidase, which generates hydrogen peroxide and contributes to colony-food sterilization (White et al., 1963), were decreased in bees consuming a non-proteinaceous diet (Alaux et al., 2010). It was also shown that p-coumaric acid present in pollen specifically up-regulates select antimicrobial peptide genes, those expressions were compromised in bees consuming only sucrose (Mao et al., 2013). Taken together, these results show that the consumption of non-protein diets leads to a decrease in the immunocompetence of worker bees. Our new data suggest that the increase in lysozyme activity can be viewed as an alternative protective mechanism induced in the absence of adequate nutrition, namely amino acid and protein deficiencies.
Data availability statement
The original contributions presented in the study are included in the article/supplementary material, further inquiries can be directed to the corresponding author.
Author contributions
GB and RV conceived the ideas for this study. GB, RV, and LY designed the research program. GB, IP, LY, and MD conceived and designed the experiments. LY, VK, GB, and MD performed the experiments. LY, GB, IP, and VK analyzed the data. RV, LY, and IP wrote the manuscript. All authors contributed to the article and approved the submitted version.
Funding
This work was supported by the Ministry of Education and Science of Ukraine (grant No 0120U102119).
Acknowledgments
The authors are very grateful to the Frontiers staff for exempting our article from paying the publication fee to support Ukrainian scientists during the Russian invasion.
Conflict of interest
The authors declare that the research was conducted in the absence of any commercial or financial relationships that could be construed as a potential conflict of interest.
Publisher’s note
All claims expressed in this article are solely those of the authors and do not necessarily represent those of their affiliated organizations, or those of the publisher, the editors and the reviewers. Any product that may be evaluated in this article, or claim that may be made by its manufacturer, is not guaranteed or endorsed by the publisher.
References
Alaux, C., Ducloz, F., Crauser, D., and Le Conte, Y. (2010). Diet effects on honeybee immunocompetence. Biol. Lett. 6, 562–565. doi: 10.1098/rsbl.2009.0986
Arathi, H. S., Bjostad, L., and Bernklau, E. (2018). Metabolomic analysis of pollen from honey bee hives and from canola flowers. Metabolomics 14:86. doi: 10.1007/s11306-018-1381-5
Arien, Y., Dag, A., Yona, S., Tietel, Z., Lapidot Cohen, T., and Shafir, S. (2020). Effect of diet lipids and omega-6:3 ratio on honey bee brood development, adult survival and body composition. J. Insect Physiol. 124:104074. doi: 10.1016/j.jinsphys.2020.1040
Arking, R., Burde, V., Graves, K., Hari, R., Feldman, E., and Zeevi, A. (2000). Forward and reverse selection for longevity in drosophila is characterized by alteration of antioxidant gene expression and oxidative damage patterns. Exp. Gerontol. 35, 167–185. doi: 10.1016/s0531-5565(99)00094-7
Aurori, C. M., Buttstedt, A., Dezmirean, D. S., Mărghitaş, L. A., Moritz, R. F. A., and Erler, S. (2013). What is the main driver of ageing in long-lived winter honeybees: antioxidant enzymes, innate immunity, or vitellogenin? J. Gerontol. 69, 633–639. doi: 10.1093/gerona/glt134
Badiou-Bénéteau, A., Carvalho, S. M., Brunet, J.-L., Carvalho, G. A., Buleté, A., Giroud, B., et al. (2012). Development of biomarkers of exposure to xenobiotics in the honey bee Apis mellifera: application to the systemic insecticide thiamethoxam. Ecotoxicol. Environ. Saf. 82, 22–31. doi: 10.1016/j.ecoenv.2012.05.005
Bakour, M., Fernandes, Â., Barros, L., Sokovic, M., Ferreira, I. C. F. R., and Badiaa, L. (2019). Bee bread as a functional product: chemical composition and bioactive properties. LWT 109, 276–282. doi: 10.1016/j.lwt.2019.02.008
Barbehenn, R. V., Bumgarner, S. L., Roosen, E. F., and Martin, M. M. (2001). Antioxidant defenses in caterpillars: role of the ascorbate-recycling system in the midgut lumen. J. Insect Physiol. 47, 349–357. doi: 10.1016/s0022-1910(00)00125-6
Barja, G. (1999). Mitochondrial oxygen radical generation and leak: sites of production in states 4 and 3, organ specificity, and relation to aging and longevity. J. Bioenerg. Biomembr. 31, 347–366. doi: 10.1023/A:1005427919188
Birben, E., Sahiner, U. M., Sackesen, C., Erzurum, S., and Kalayci, O. (2012). Oxidative stress and antioxidant defense. World Allergy Organ. J. 5, 9–19. doi: 10.1097/wox.0b013e3182439613
Bogdanov, S. (2016). “Pollen: nutrition, functional properties, health” in The Pollen Book. ed. S. Bogdanov (Switzerland: Bee Product Science), 1–34.
Borsuk, G., Ptaszyńska, A. A., Olszewski, K., Domaciuk, M., Krutmuang, P., and Paleolog, J. (2017). A new method for quick and easy hemolymph collection from Apidae adults. PLoS One 12:e0170487. doi: 10.1371/journal.pone.0170487
Bouchebti, S., Wright, G. A., and Shafir, S. (2022). Macronutrient balance has opposing effects on cognition and survival in honey bees. Funct. Ecol. 36, 2558–2568. doi: 10.1111/1365-2435.14143
Bradford, M. M. (1976). A rapid and sensitive method for the quantification of microgram quantities of protein utilizing the principle of protein-dye binding. Anal. Biochem. 72, 248–254. doi: 10.1006/abio.1976.9999
Branchiccela, B., Castelli, L., Corona, M., Díaz-Cetti, S., Invernizzi, C., Martínez de la Escalera, G., et al. (2019). Impact of nutritional stress on the honeybee colony health. Sci. Rep. 9:10156. doi: 10.1038/s41598-019-46453-9
Brodschneider, R., and Crailsheim, K. (2010). Nutrition and health in honey bees. Apidologie 41, 278–294. doi: 10.1051/apido/2010012
Buzduga, I. M., Volkov, R. A., and Panchuk, I. I. (2018). Metabolic compensation in Arabidopsis thaliana catalase-deficient mutants. Cytol. Genet. 52, 31–39. doi: 10.3103/S0095452718010036
Castelli, L., Branchiccela, B., Garrido, M., Invernizzi, C., Porrini, M., Romero, H., et al. (2020). Impact of nutritional stress on honeybee gut microbiota, immunity, and Nosema ceranae infection. Microb. Ecol. 80, 908–919. doi: 10.1007/s00248-020-01538-1
Čeksterytė, V., Račys, J., Kaškonienė, V., and Venskutonis, P. R. (2008). Fatty acid composition in beebread. Biol. Ther. Dent. 54, 253–257. doi: 10.2478/v10054-008-0052-2
Cervoni, M. S., Cardoso-Júnior, C. A. M., Craveiro, G., Souza, A. D. O., Alberici, L. C., and Hartfelder, K. (2017). Mitochondrial capacity, oxidative damage and hypoxia gene expression are associated with age-related division of labor in honey bee (Apis mellifera L.) workers. J. Exp. Biol. 220, 4035–4046. doi: 10.1242/jeb.161844
Cook, S. M., Awmack, C. S., Murray, D. A., and Williams, I. H. (2003). Are honey bees’ foraging preferences affected by pollen amino acid composition? Ecol. Entomol. 28, 622–627. doi: 10.1046/j.1365-2311.2003.00548.x
Corona, M., Hughes, K. A., Weaver, D. B., and Robinson, G. E. (2005). Gene expression patterns associated with queen honey bee longevity. Mech. Ageing Dev. 126, 1230–1238. doi: 10.1016/j.mad.2005.07.004
Corona, M., and Robinson, G. E. (2006). Genes of the antioxidant system of the honey bee: annotation and phylogeny. Insect Mol. Biol. 15, 687–701. doi: 10.1111/j.1365-2583.2006.00695.x
Crailsheim, K. (1990). The protein balance of the honey bee worker. Apidologie 21, 417–429. doi: 10.1051/apido:19900504
Crailsheim, K., Schneider, L. H. W., Hrassnigg, N., Bühlmann, G., Brosch, U., Gmeinbauer, R., et al. (1992). Pollen consumption and utilization in worker honeybees (Apis mellifera carnica): dependence on individual age and function. J. Insect Physiol. 38, 409–419. doi: 10.1016/0022-1910(92)90117-v
Day, S. E., Beyer, R., Mercer, A. R., and Ogden, S. C. (1990). The nutrient composition of honey bee-collected pollen in Otago, New Zealand. J. Apic. Res. 29, 138–146. doi: 10.1080/00218839.1990.11101210
De Grandi-Hoffman, G., Corby-Harris, V., Carroll, M., Toth, A. L., Gage, S., and deJong Watkins, E. (2021). The importance of time and place: nutrient composition and utilization of seasonal pollens by european honey bees (Apis mellifera L.). Insects 12:235. doi: 10.3390/insects12030235
De Groot, A. P. (1953). Protein and amino acid requirements of the honey bee (Apis mellifera L.). Phys. Comp. Oecol. 3, 197–285.
De Groot, G. S., Aizen, M. A., Sáez, A., and Morales, C. L. (2021). Large-scale monoculture reduces honey yield: the case of soybean expansion in Argentina. Agric. Ecosyst. Environ. 306:107203. doi: 10.1016/j.agee.2020.107203
Di Pasquale, G., Alaux, C., Le Conte, Y., Odoux, J.-F., Pioz, M., Vaissière, B. E., et al. (2016). Variations in the availability of pollen resources affect honey bee health. PLoS One 11:e0162818. doi: 10.1371/journal.pone.0162818
Di Pasquale, G., Salignon, M., Le Conte, Y., Belzunces, L. P., Decourtye, A., Kretzschmar, A., et al. (2013). Influence of pollen nutrition on honey bee health: do pollen quality and diversity matter? PLoS One 8:8. doi: 10.1371/journal.pone.0072016
Dolezal, A. G., Carrillo-Tripp, J., Judd, T. M., Allen Miller, W., Bonning, B. C., and Toth, A. L. (2019). Interacting stressors matter: diet quality and virus infection in honeybee health. R. Soc. Open Sci. 6:181803. doi: 10.1098/rsos.181803
Drahota, Z., Chowdhury, S. K. R., Floryk, D., Mráček, T., Wilhelm, J., Rauchová, H., et al. (2002). Glycerophosphate-dependent hydrogen peroxide production by brown adipose tissue mitochondria and its activation by ferricyanide. J. Bioenerg. Biomembr. 34, 105–113. doi: 10.1023/A:1015123908918
Foyer, C. H., Ruban, A. V., and Noctor, G. (2017). Viewing oxidative stress through the lens of oxidative signalling rather than damage. Biochem. J. 474, 877–883. doi: 10.1042/bcj20160814
Garibaldi, L. A., Requier, F., Rollin, O., and Andersson, G. K. (2017). Towards an integrated species and habitat management of crop pollination. Curr. Opin. Insect. Sci. 21, 105–114. doi: 10.1016/j.cois.2017.05.016
Gillespie, J. P., Kanost, M. R., and Trenczek, T. (1997). Biological mediators of insect immunity. Annu. Rev. Entomol. 42, 611–643. doi: 10.1146/annurev.ento.42.1.611
Goulson, D., Nicholls, E., Botías, C., and Rotheray, E. L. (2015). Combined stress from parasites, pesticides and lack of flowers drives bee declines. Science 347:1255957. doi: 10.1126/science.1255957
Gray, A., Brodschneider, R., Adjlane, N., Ballis, A., Brusbardis, V., Charrière, J.-D., et al. (2019). Loss rates of honey bee colonies during winter 2017/18 in 36 countries participating in the COLOSS survey, including effects of forage sources. J. Apic. Res. 58, 479–485. doi: 10.1080/00218839.2019.1615661
Gray, A., Noureddine, A., Arab, A., Ballis, A., Brusbardis, V., and Bugeja Douglas, A. (2022). Honey bee colony loss rates in 37 countries using the COLOSS survey for winter 2019-2020: the combined effects of operation size, migration and queen replacement. J. Apic. Res. 61, 1–7. doi: 10.1080/00218839.2022.2113329
Grotto, D., Maria, L. S., Valentini, J., Paniz, C., Schmitt, G., Garcia, S. C., et al. (2009). Importance of the lipid peroxidation biomarkers and methodological aspects for malondialdehyde quantification. Química Nova. 32, 169–174. doi: 10.1590/s0100-40422009000100032
Halliwell, B., and Gutteridge, J. M. C. (2015). Free Radicals in Biology and Medicine. New York: Oxford University Press
Herbert, E. W. (1992). “Honey bee nutrition” in The Hive and the Honey Bee. ed. J. M. Graham (Hamilton, Illinois: Dadant and Sons), 197–233.
Imler, J.-L., and Bulet, P. (2005). Antimicrobial peptides in drosophila: structures, activities and gene regulation. Chem. Immunol. Allergy 86, 1–21. doi: 10.1159/000086648
Irato, P., and Santovito, G. (2021). Enzymatic and non-enzymatic molecules with antioxidant function. Antioxidants 10:579. doi: 10.3390/antiox10040579
Jakubczyk, K., Kałduńska, J., Dec, K., Kawczuga, D., and Janda, K. (2020). Antioxidant properties of small-molecule non-enzymatic compounds. Pol. Merkur. Lekars. Organ Pol. Towarzyst. Lekarsk. 48, 128–132.
Karavan, V., Gordey, T., Yazlovytska, L., and Panchuk, I. (2021). Combined effects of temperature and nutritional stress on TBARS level in Apis mellifera. Abstract COLOSS Asia Okinawa, 35.
Khalifa, S. A. M., Elshafiey, E. H., Shetaia, A. A., El-Wahed, A. A. A., Algethami, A. F., Musharraf, S. G., et al. (2021). Overview of bee pollination and its economic value for crop production. Insects 12:688. doi: 10.3390/insects12080688
Korayem, A. M., Khodairy, M. M., Abdel-Aal, A. A., and El-Sonbaty, A. M. (2012). The protective strategy of antioxidant enzymes against hydrogen peroxide in honey bee, Apis mellifera during two different seasons. J. Biol. Earth Sci. 2, B93–B109.
Korolyuk, M. A., Ivanova, L. I., Mayorova, I. G., and Tokarev, V. E. (1988). The method for determining the activity of catalase. Lab. Delo 1, 16–18.
Kwakman, P. H., te Velde, A. A., de Boer, L., Speijer, D., Vandenbroucke-Grauls, C. M., and Zaat, S. A. (2010). How honey kills bacteria. FASEB J. 24, 2576–2582. doi: 10.1096/fj.09-150789
Levine, R. L., Williams, J. A., Stadtman, E. P., and Shacter, E. (1994). Carbonyl assays for determination of oxidatively modified. Methods Enzymol. 233, 346–357. doi: 10.1016/s0076-6879(94)33040-9
Li, J., Heerman, M. C., Evans, J. D., Rose, R., Li, W., Rodríguez-García, C., et al. (2019). Pollen reverses decreased lifespan, altered nutritional metabolism, and suppressed immunity in honey bees (Apis mellifera) treated with antibiotics. J. Exp. Biol. 222:jeb.202077. doi: 10.1242/jeb.202077
Li, C., Xu, B., Wang, Y., Feng, Q., and Yang, W. (2012). Effects of dietary crude protein levels on development, antioxidant status, and total midgut protease activity of honey bee (Apis mellifera ligustica). Apidologie 43, 576–586. doi: 10.1007/s13592-012-0126-0
Mao, W., Schuler, M. A., and Berenbaum, M. R. (2013). Honey constituents up-regulate detoxification and immunity genes in the western honey bee Apis mellifera. PNAS 110, 8842–8846. doi: 10.1073/pnas.1303884110
Margotta, J. W., Roberts, S. P., and Elekonich, M. M. (2018). Effects of flight activity and age on oxidative damage in the honey bee, Apis mellifera. J. Exp. Biol. 221:jeb183228. doi: 10.1242/jeb.183228
Mittapalli, O., Neal, J. J., and Shukle, R. H. (2007). Antioxidant defense response in a galling insect. Proc. Natl. Acad. Sci. U. S. A. 104, 1889–1894. doi: 10.1073/pnas.0604722104
Miwa, S., and Brand, M. D. (2005). The topology of superoxide production by complex III and glycerol 3-phosphate dehydrogenase in drosophila mitochondria. Biochim. Biophys. Acta Bioenerg. 1709, 214–219. doi: 10.1016/j.bbabio.2005.08.003
Münch, D., Amdam, G. V., and Wolschin, F. (2008). Ageing in a eusocial insect: molecular and physiological characteristics of life span plasticity in the honey bee. Funct. Ecol. 22, 407–421. doi: 10.1111/j.1365-2435.2008.01419.x
Murphy, M. P. (2009). How mitochondria produce reactive oxygen species. Biochem. J. 417, 1–13. doi: 10.1042/BJ20081386
Negri, P., Villalobos, E., Szawarski, N., Damiani, N., Gende, L., Garrido, M., et al. (2019). Towards precision nutrition: a novel concept linking phytochemicals, immune response and honey bee health. Insects 10:401. doi: 10.3390/insects10110401
Nikolenko, A., Saltykova, E., and Gaifullina, L. (2012). “Molecular mechanisms of antioxidant protective processes in honey bee Apis mellifera” in The Oxidative Stress in Vertebrates and Invertebrates: Molecular Aspects on Cell Signaling. eds. T. Farooqui and A. A. Farooqui (New Jersey: John Wiley & Sons), 279–294.
Olate-Olave, V. R., Verde, M., Vallejos, L., Perez Raymonda, L., Cortese, M. C., and Doorn, M. (2021). Bee health and productivity in Apis mellifera, a consequence of multiple factors. Vet. Sci. 8:76. doi: 10.3390/vetsci8050076
Orčić, S., Nikolić, T., Purać, J., Šikoparija, B., Blagojević, D. P., Vukašinović, E., et al. (2017). Seasonal variation in the activity of selected antioxidant enzymes and malondialdehyde level in worker honey bees. Entomol. Exp. Appl. 165, 120–128. doi: 10.1111/eea.12633
Ostaff, D. P., Mosseler, A., Johns, R. C., Javorek, S., Klymko, J., and Ascher, J. S. (2015). Willows (Salix spp.) as pollen and nectar sources for sustaining fruit and berry pollinating insects. Can. J. Plant Sci. 95, 505–516. doi: 10.4141/cjps-2014-339
Paoli, P. P., Donley, D., Stabler, D., Saseendranath, A., Nicolson, S. W., Simpson, S. J., et al. (2014). Nutritional balance of essential amino acids and carbohydrates of the adult worker honeybee depends on age. Amino Acids 46, 1449–1458. doi: 10.1007/s00726-014-1706-2
Phillips, J. P., Campbell, S. D., Michaud, D., Charbonneau, M., and Hilliker, A. J. (1989). Null mutation of copper/zinc superoxide dismutase in drosophila confers hypersensitivity to paraquat and reduced longevity. PNAS 86, 2761–2765. doi: 10.1073/pnas.86.8.2761
Placer, Z. A., Cushman, L. L., and Johnson, B. C. (1966). Estimation of product of lipid peroxidation (malonyl dialdehyde) in biochemical systems. Anal. Biochem. 16, 359–364. doi: 10.1016/0003-2697(66)90167-9
Potts, S. G., Biesmeijer, J. C., Kremen, C., Neumann, P., Schweiger, O., and Kunin, W. E. (2010). Global pollinator declines: trends, impacts and drivers. Tree 25, 345–353. doi: 10.1016/j.tree.2010.01.007
Ptaszyńska, A. A., Gancarz, M., Hurd, P. J., Borsuk, G., Wiącek, D., Nawrocka, A., et al. (2018). Changes in the bioelement content of summer and winter western honeybees (Apis mellifera) induced by Nosema ceranae infection. PLoS One 13:e0200410. doi: 10.1371/journal.pone.0200410
Radev, Z. (2018). Variety in protein content of pollen from 50 plants from Bulgaria. Bee World 95, 81–83. doi: 10.1080/0005772X.2018.1486276
Reznick, A. Z., and Packer, L. (1994). Oxidative damage to proteins: spectrophotometric method for carbonyl assay. Methods Enzymol. 233, 357–363. doi: 10.1016/S0076-6879(94)33041-7
Roelofs, D., Aarts, M. G. M., Schat, H., and Van Straalen, N. M. (2008). Functional ecological genomics to demonstrate general and specific responses to abiotic stress. Funct. Ecol. 22:070719055134003. doi: 10.1111/j.1365-2435.2007.01312.x
Rzepecka-Stojko, A., Stojko, J., Kurek-Górecka, A., Górecki, M., Kabała-Dzik, A., Kubina, R., et al. (2015). Polyphenols from bee pollen: structure, absorption, metabolism and biological activity. Molecules 20, 21732–21749. doi: 10.3390/molecules201219800
Scialò, F., Sriram, A., Fernández-Ayala, D., Gubina, N., Lõhmus, M., Nelson, G., et al. (2016). Mitochondrial ROS produced via reverse electron transport extend animal lifespan. Cell Metab. 23, 725–734. doi: 10.1016/j.cmet.2016.03.009
Snyder, J. A., and Fritsch, D. (1984). “Two reliable and inexpensive lysozyme assays for teaching enzymology and microbiology.” in Tested Studies for Laboratory Teaching. Proceedings of the Third Workshop/Conference of the Association for Biology Laboratory Education (ABLE), Vol. 3. ed. C. L. Harris (Dubuque, Iowa: Kendall/Hunt Publishing Company), 167–180.
Szymaś, B., and Jędruszuk, A. (2003). The influence of different diets on haemocytes of adult worker honey bees, Apis mellifera. Apidologie 34, 97–102. doi: 10.1051/apido:2003012
Thiboldeaux, R., Lindroth, R., and Tracy, J. (1998). Effects of juglone (5-hydroxy-1,4-naphthoquinone) on midgut morphology and glutathione status in Saturniid moth larvae. Comp. Biochem. Physiol. Part C. Toxicol. Pharmacol. 120, 481–487. doi: 10.1016/s0742-8413(98)10070-1
Tong, L., Nieh, J. C., and Tosi, S. (2019). Combined nutritional stress and a new systemic pesticide (flupyradifurone, Sivanto) reduce bee survival, food consumption, flight success, and thermoregulation. Chemosphere 237:124408. doi: 10.1016/j.chemosphere.2019.124408
Tosi, S., Nieh, J. C., Sgolastra, F., Cabbri, R., and Medrzycki, P. (2017). Neonicotinoid pesticides and nutritional stress synergistically reduce survival in honey bees. Proc. R. Soc. B Biol. Sci. 284:20171711. doi: 10.1098/rspb.2017.1711
Urcan, A., Al Mărghitaș, L., Dezmirean, D. S., Bobiș, O., Bonta, V., Mureșan, C. I., et al. (2017). Chemical composition and biological activities of beebread. Bull. UASVM Ani. Sci. Biotechnol. 74, 6–14. doi: 10.15835/buasvmcn-asb:12646
Volkov, R. A., Panchuk, I. I., Mullineaux, P. M., and Schöffl, F. (2006). Heat stress-induced H2O2 is required for effective expression of heat shock genes in Arabidopsis. Plant Mol. Biol. 61, 733–746. doi: 10.1007/s11103-006-0045-4
Wheeler, M. M., and Robinson, G. E. (2014). Diet-dependent gene expression in honey bees: honey vs. sucrose or high fructose corn syrup. Sci. Rep. 4:5726. doi: 10.1038/srep05726
White, J. W. (1957). The composition of honey. Bee World 38, 57–66. doi: 10.1080/0005772X.1957.11094976
White, J. W. J., Subers, M. H., and Schepartz, A. I. (1963). The identification of inhibine, antibacterial factor in honey, as hydrogen peroxide, and its origin in a honey glucose oxidase system. Biochem. Biophys. 73, 57–70. doi: 10.1016/0006-3002(63)90359-7
Wright, G. A., Nicolson, S. W., and Shafir, S. (2018). Nutritional physiology and ecology of honey bees. Annu. Rev. Entomol. 63, 327–344. doi: 10.1146/annurev-ento-020117-043423
Keywords: catalase, honey bee, lipid peroxidation, lysozyme, nutrition, oxidative stress, protein carbonylation, biomarkers
Citation: Yazlovytska LS, Karavan VV, Domaciuk M, Panchuk II, Borsuk G and Volkov RA (2023) Increased survival of honey bees consuming pollen and beebread is associated with elevated biomarkers of oxidative stress. Front. Ecol. Evol. 11:1098350. doi: 10.3389/fevo.2023.1098350
Edited by:
Fabio Sgolastra, University of Bologna, ItalyReviewed by:
Iaria Caliani, University of Siena, ItalyAles Gregorc, University of Maribor, Slovenia
Copyright © 2023 Yazlovytska, Karavan, Domaciuk, Panchuk, Borsuk and Volkov. This is an open-access article distributed under the terms of the Creative Commons Attribution License (CC BY). The use, distribution or reproduction in other forums is permitted, provided the original author(s) and the copyright owner(s) are credited and that the original publication in this journal is cited, in accordance with accepted academic practice. No use, distribution or reproduction is permitted which does not comply with these terms.
*Correspondence: Roman A. Volkov, ✉ ci52b2xrb3ZAY2hudS5lZHUudWE=
†ORCID: Liudmyla S. Yazlovytska orcid.org/0000-0001-9296-1201
Volodymyr V. Karavan, orcid.org/0000-0002-5982-7024
Marcin Domaciuk, orcid.org/0000-0002-4294-9850
Irina I. Panchuk, orcid.org/0000-0002-2837-4480
Grzegorz Borsuk, orcid.org/0000-0002-8407-3383
Roman A. Volkov, orcid.org/0000-0003-0673-2598