- 1Department of Plant and Wildlife Sciences, Brigham Young University, Provo, UT, United States
- 2Wildlife Section, Utah Division of Wildlife Resources, Salt Lake City, UT, United States
- 3Southeastern Region, Idaho Fish and Game, Pocatello, ID, United States
Recent meta-analyses indicate that predator removal experiments result in marginal increases in prey abundance at best. However, most predator removal studies take place for less than the target prey’s generation time and lack a targeted spatial approach. Our objective was to determine how temporal and spatial aspects of predator control influenced neonate survival of a temperate ungulate. We conducted multiyear coyote (Canis latrans) removals using a crossover experimental design. We found that consecutive years of predator removal increased survival of neonate mule deer (Odocoileus hemionus) more than a single year of removal. We also found that removing coyotes from areas near fawn birth sites increased fawn survival, whereas removing coyotes from areas farther from birth sites did not influence fawn survival. Our results underscore the need for coyote removal programs to (1) employ removal efforts over consecutive years to maximize effectiveness, (2) conduct spatially explicit removal efforts targeting fawning habitat, and (3) occur when the likelihood of additive mortality is high and prey populations have the resources available to grow.
Introduction
Intentionally removing predators from an ecosystem for the purpose of increasing prey populations is a common wildlife management strategy (Connolly, 1978; Salo et al., 2010; Peek et al., 2012). However, a growing number of studies question whether such predator removals are effective (Bergstrom, 2017). Given that predator removal efforts continue in many regions throughout the world, it is important to capitalize on ongoing removal programs by experimentally testing their efficacy and providing stakeholders with data needed to make informed management decisions (Bergstrom, 2017). A recent meta-analysis, for example, found that experimental predator removals only resulted in modest increases in prey abundance and/or survival at best, but that most studies lacked rigor in experimental design (Clark and Hebblewhite, 2021). Importantly, just six of the 52 experiments were conducted over a period of time that exceeded the target prey’s generation time (Clark and Hebblewhite, 2021). This mismatch is problematic because such removal schedules may not be long enough or in the locations needed to generate observable effects on prey survival (Connell and Sousa, 1983).
It is likely that both temporal and spatial aspects of predator removal influence response of prey. Temporal aspects can include both time of year and single vs. multiple years of predator control. An observed increase in survival over multiple years could result from (1) an absolute decrease in the total number of predation events in a given year or (2) a decrease in the proportion of animals taken due to increases in population size in subsequent years of predator removal. Both of these scenarios result in the desired outcome of increasing survival in prey populations. In either case, it is likely that time of year when predators are removed will affect likelihood of success. For example, predator removal during late winter may increase likelihood of increased prey survival because that timing disrupts social structure of predators during the time of pair bonding or reproduction and occurs just prior to birthing of prey species (Blejwas et al., 2002). Nonetheless, some predator species refill home ranges vacated by removed predators quickly. For example, under intense coyote (Canis latrans) removal programs, coyotes have been reported to repopulate areas within months to a short number of years (Beasom, 1974; Connolly and Longhurst, 1975; Connolly, 1995), although there are examples where coyotes do not rapidly recolonize (Mahoney, 2017). Because predators can exhibit compensatory natality and increased survival following reductions in density (Knowlton, 1972; Knowlton et al., 1999), it is likely that removal at the most important time of year and across multiple consecutive years has the greatest likelihood of a resulting increase in survival of prey.
Predation rates on domestic livestock have been correlated with density of coyotes on the landscape (Stoddart et al., 2001) leading to predator control programs that are often non-selective and broad in spatial scale (Mitchell et al., 2004). However, wild ungulates do not live and reproduce evenly or randomly across the landscape. Therefore, predator control programs focused on habitat where prey are most vulnerable have the greatest likelihood of success. Further, survival of prey should increase even if predators backfill the vacated space over several months to a few years since individuals that disperse or backfill tend to be young individuals that are less likely to take large prey items compared to resident adults (Harrison, 1992; Watine and Giuliano, 2017). Coyotes have the greatest effect on mule deer (Odocoileus hemionus) during the first few months of life when mule deer are small and unable to escape or during late winter when some individuals have exhausted energy reserves (Bowyer, 1987; Lingle, 2000). Mule deer susceptible to coyotes in late winter are likely individuals with severely depleted energy reserves (often associated with old age). Therefore, this source of mortality is more likely to be compensatory or have little effect on population growth. Consequently, coyote control programs focused on birthing and early-life rearing habitat have the greatest likelihood of benefiting mule deer by decreasing mortality that is more likely to be additive. Indeed, numerous studies indicate that coyote predation is a common source of mortality for mule deer fawns (Bartmann et al., 1992; Whittaker and Lindzey, 1999; Pojar and Bowden, 2004; Bishop et al., 2009). However, in perhaps the largest coyote removal experiment, mule deer fawn survival increased when coyotes were removed, but increases were modest and only evident when alternate prey (i.e., lagomorph) populations were low (Hurley et al., 2011). While the study by Hurley et al. (2011) employed a robust experimental design over six consecutive years, the number of coyotes removed varied drastically from year to year, ranging from a mean of 31.15–79.96 coyotes per 1,000 km2 and occurred in a traditional manner over a broad spatial scale. This high variability in the number of coyotes removed combined with the broad spatial scale (i.e., non-targeted) leaves questions about the effectiveness of coyote removal during this early life stage of mule deer.
Mule deer have high cultural and economic value as a primary big game ungulate in western North America but have recently experienced fluctuations in population size (Bleich and Taylor, 1998; Unsworth et al., 1999; Peek et al., 2002; Bergman et al., 2015). As in other ungulate species (Gaillard et al., 1998), low fawn survival and subsequent low recruitment may drive fluctuations in mule deer populations (Peek et al., 2002; Lomas and Bender, 2007). In this study, we explicitly tested the effect of two consecutive years vs. a single year of coyote removal on survival of mule deer fawns in Utah, United States. Further, we attempted to examine the effectiveness of spatially explicit removal (i.e., removal from birthing and rearing habitat). Therefore, the objective of our study was to determine the effect of coyote removal on fawn survival using (1) a crossover experimental design in two comparably sized locales, (2) two consecutive years of control and treatment conditions in both locales, and (3) relatively consistent numbers of coyotes removed across years. Further, we examined the relationship between location of removal relative to birthing habitat and the likelihood of fawn survival. We hypothesized that both spatial and temporal aspects of predator removal would influence survival of neonate mule deer. We predicted that multiple years of predator removal would increase survival of mule deer fawns because reproduction by coyotes would be disrupted, densities would be further reduced, and the recovery of coyotes to pre-control levels would be prolonged. In addition, we predicted that control efforts in birthing habitat would be more effective at reducing fawn mortality than control efforts at a broad spatial scale. Importantly, our research site experienced normal climatic patterns (no extremely harsh winters or dry summers) during the years of the study, which enabled the examination of predation effects that were not confounded by severe weather.
Materials and methods
Study area
Our study areas were located on Monroe Mountain in south-central Utah. Monroe Mountain is approximately 70 km long (north to south) and 20 km across. Several thousand mule deer inhabit the mountain and surrounding winter range. Land ownership is split between federal agencies (Forest Service and Bureau of Land Management), state lands, and private lands. The mountain was divided into two study areas and a buffer zone (south study area, north study area, and a central buffer; Figure 1). The buffer area was an east to west corridor that separated the north study area from the south study area. This buffer was 5 to 10 km wide and was delineated by large canyons on the north and south where it bordered the study areas (an effort to ensure independence of the treatments). Habitat types on the mountain include areas dominated by big sagebrush (Artemesia tridentata), mountain mahogany (Cercocarpus ledifolius), Gambel oak (Quercus gambelii), aspen (Populus tremuloides), pinion pine (Pinus edulis), Utah juniper (Juniperus osteosperma), ponderosa pine (Pinus ponderosa), and Douglas fir (Pseudotsuga menziesii). Potential predators of mule deer inhabiting this area were coyotes (Canis latrans), cougars (Puma concolor), bobcats (Lynx rufus), and black bears (Ursus americanus), although black bears are uncommon.
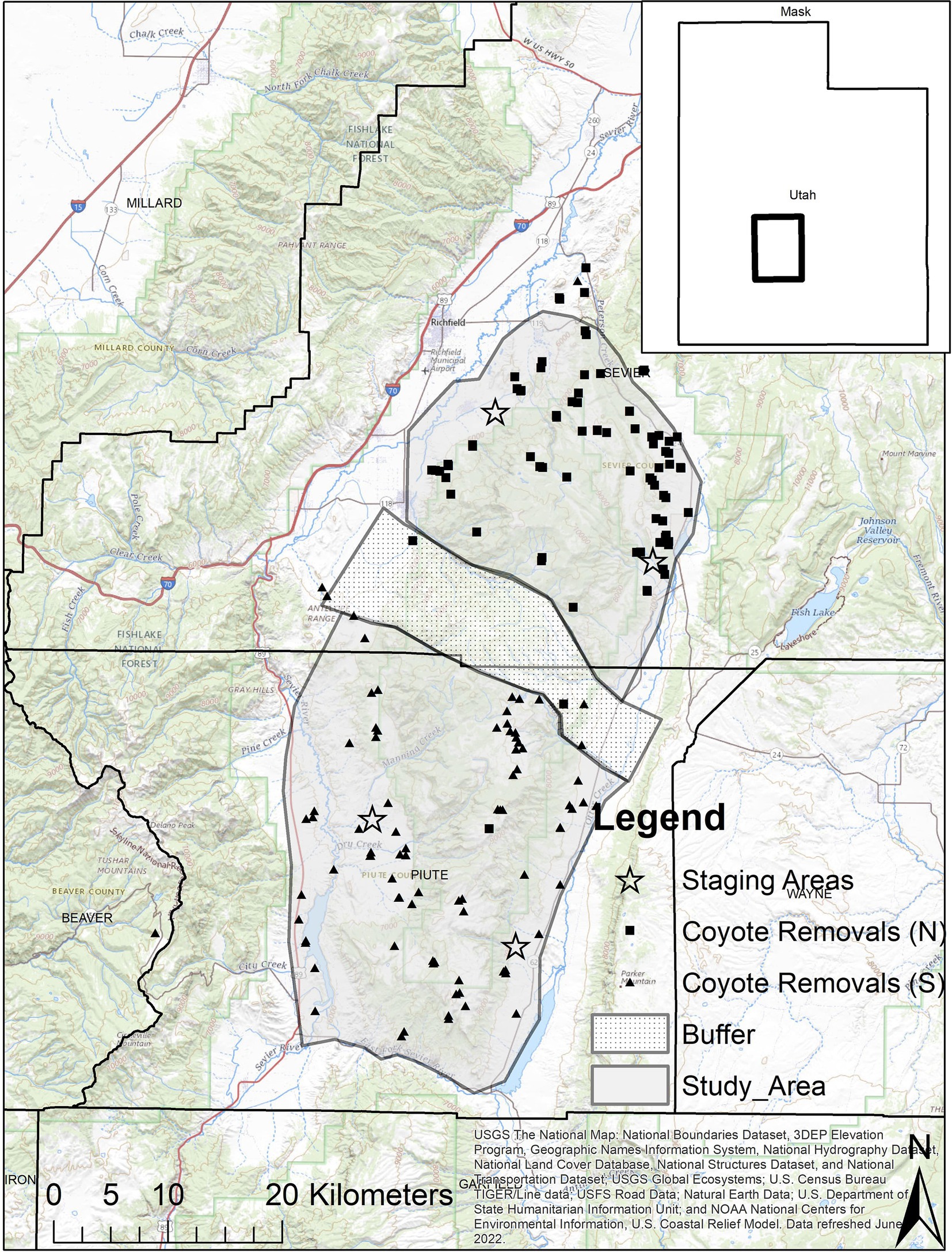
Figure 1. Map of Monroe Mountain, Utah, United States with polygons delineating north (coyote removal area 2012–2013) and south (coyote removal area 2014–2015) study areas where we evaluated survival of neonate mule deer. The stippled area indicates the buffer zone between the two study areas and was bordered on the north and south by deep canyons that traversed the mountain. Squares and triangles indicate the location of coyotes that were removed from the mountain during 2012–2013 and 2014–2015, respectively. Stars represent the centroid of areas on winter range where adult females were captured each March of the study.
Experimental design
We determined the effects of predator removal on survival of neonate mule deer (birth to 4 months of age) during 2012–2015. To evaluate the effects of predator removal, we implemented an experimental crossover design. During 2012–2013 (first 2 years of the study), USDA Wildlife Services used standard techniques, including aerial gunning, to remove coyotes from the north study area, but not from the south study area. In 2014, treatments were switched; coyote removal occurred on the south study area, but not the north study area for the final 2 years. Similar numbers of coyotes were removed from both the north and south study areas during the first 2 years and last 2 years of the study (Mahoney, 2017).
For coyote removals, Wildlife Services focused on high elevation habitat where birthing was more likely to occur. However, removals were influenced by snow and other weather conditions (e.g., wind and cloud cover) and, therefore, there was spatial variation in the locations of removals. All removal flights were performed within 3 days of a snow event. Wildlife Services would systematically fly the entire study area. Once a coyote track was located, they would follow that track to locate the animal and attempt to remove that animal. Once removed, they would record a GPS location of the removal and return to the original path to continue the search effort. With the GPS location of each removed animal, we were able to examine the spatial effects of coyote removal on the survival of neonate mule deer as describe below.
To determine survival of neonate mule deer, we captured neonates on each study area during each year. To aid in the capture of neonate mule deer, we captured adult female mule deer (via helicopter net-gunning) in March of each year from four locations on winter range of the study areas (Angle, Burrville, Thompson Basin, and Elbow Ranch; Figure 1). We assessed body size (weight, hind foot length, chest and neck girth), condition (body condition score method; Cook et al., 2007), and age (estimated based on tooth wear and eruption pattern) of each captured individual (Severinghaus, 1949; Robinette et al., 1957). Additionally, we determined body condition and pregnancy via ultrasonography (E.I. Medical Imaging portable ultrasound; Smith and Lindzey, 1982). We fitted pregnant females with VHF collars [Telonics Inc., Advanced Telemetry Systems (ATS)] and vaginal implant transmitters (VIT; ATS). We used a vaginoscope to insert VITs until the antennae did not protrude from the opening of the vagina (Bishop et al., 2009).
Between the original capture of adult females and parturition, we located collared females using radio telemetry. During March and April, individuals were located sporadically from the ground and a fixed-wing aircraft. Beginning in May, we attempted to locate every female twice a week. Beginning the last week of May, we located each female at least every other day until all VITs had been expelled. When an expelled VIT was detected (pulse rate doubled after a reduction in temperature), we located the VIT and conducted an extensive search for the neonate(s). In addition, we opportunistically captured neonate mule deer while searching for those associated with a VIT or while observing female mule deer not previously captured.
To determine survival of neonate mule deer, we attached VHF radio collars to captured individuals. We handled neonates with latex gloves while we fitted collars, and we recorded morphological measurements. Additionally, we estimated the age of individuals using hoof condition/length, pelage, and behavior (Lomas and Bender, 2007). Radio collars placed on neonates were designed to expand with the growing animal and drop off after approximately 8 months.
We monitored collared neonate mule deer on a schedule designed to ensure that mortalities were located promptly. Early detection of mortalities minimized the likelihood of confusion between the true cause of mortality and scavenging. Specifically, we relocated neonates at least 3 times weekly between the time of initial capture and the end of August. We decreased monitoring frequency to once a week beginning in September because most mortality of neonate mule deer occurs in the first few months of life (Pojar and Bowden, 2004; Lomas and Bender, 2007).
We attempted to locate deceased animals whenever a transmitter was in mortality mode, which was reflected by a doubling of the pulse rate of the collar after 8 h of no movement. After locating a collar, we searched for the deceased neonate mule deer and determined a probable cause of death based on evidence found at that location. If the probable cause of death was attributed to predation, we used a suite of indicators that are predator specific to determine what species of predator was most likely responsible for the mortality (Gese and Grothe, 1995). In a few cases, assignment of the predator responsible for death was ambiguous (e.g., most of the carcass was consumed and there was sign from multiple species of predators) and, therefore, we classified these mortalities as unknown predation. Other cause-of-death categories included starvation, accidents (e.g., vehicle strikes, fences, etc.), disease, and unknown.
Statistical analyses
We used multi-model inference within Program MARK and a known-fate model (White and Burnham, 1999) to estimate survival of neonate mule deer in both treatments (removal and non-removal), and to investigate factors potentially influencing survival. Preliminary analysis revealed that coyotes have the greatest impact on survival of neonate mule deer during the first 16 weeks of life and, therefore, we modeled survival during the first 16 weeks of life in our subsequent analyses. Rather than use a staggered entry into the model when an animal was born, we set week one for each mule deer neonate to begin at birth, regardless of calendar date (Bishop et al., 2008). We used a hierarchical approach to draw inferences regarding a priori hypotheses about potential influences on survival rates (Burnham and Anderson, 1998). We first tested for temporal effects by comparing models where survival varied linearly through time (T), quadratically through time (T2), by week (Week), and by year (Year); we also tested models that used interactions of these time components. We advanced models to the next step based on Akaike’s Information Criterion adjusted for small sample sizes (AICc) if they had at least 5% AICc weight ω i .
In our second step, we tested the influence of removal of coyotes by adding variables to models that advanced from our first step. Covariates related to removal of coyotes included study area (north or south; StdArea), treatment (Treatment), year of treatment (YrofTrtmt), number of coyotes removed within 2,250 meters (the average summer home range diameter of mule deer; Webb et al., 2013) surrounding the capture location of each neonate (HRCKills), and lagomorph abundance [LagAbund (a measure of alternate prey for coyotes)]. We obtained estimates of lagomorph abundance from a concurrent study of predators on the study site (Mahoney, 2017).
In our final step, we added various individual characteristics of neonate mule deer as covariates. These characteristics included sex of each individual (Sex), new hoof growth (NewGrowth), whether or not the neonate was a twin (Twin), and weight (Weight). We included neonate mule deer age at capture in all our models that included weight to account for the effects of age-related weight gain.
Fate of siblings is not completely independent and, therefore, using siblings as independent observations can lead to over-dispersion in known-fate models. To account for potential dependence among the fate of siblings, we estimated ĉ (degree of overdispersion) by bootstrapping our data using methods described by Bishop et al. (2008). We then ranked final models based on Quasi-AICc values (QAICc) adjusted for ĉ. We checked models in our final list for uninformative parameters and then produced model-averaged estimates of β coefficients and survival (Arnold, 2010). We judged the importance of variables in top models based on overlap in 85% confidence intervals around these β estimates (Arnold, 2010).
To further visualize the relationship between survival of neonates and years of predator removal, we used a Kaplan–Meier function to produce survival curves for neonates (Kaplan and Meier, 1958; Jager et al., 2008). We grouped individual neonates into the following three groups: no coyote removal (0), 1 year of removal (1), and 2 years of removal (2). We then plotted these survival curves with time on the x-axis and survival on the y-axis.
Results
During 2012–2015 we captured 287 adult female mule deer from winter-range locations surrounding Monroe Mountain (Table 1). Range of percent ingesta-free body fat (IFBF) was 2.9%–12.1% and was normally distributed around the mean. Of the 287 females captured, 95% (n = 273) were pregnant. Vaginal Implant Transmitters were inserted into 260 of these 273 pregnant females; 13 females were not used because they were recaptured and known to summer outside the two study areas (n = 5), their vaginas were too small for VIT insertion (n = 5), or they experienced capture-related injury (n = 3). Of the 260 animals that received VITs, 146 moved onto one of our study areas on Monroe Mountain. The remaining females either died before parturition or transitioned onto summer range outside the study areas and could not be included in the study (Table 1).
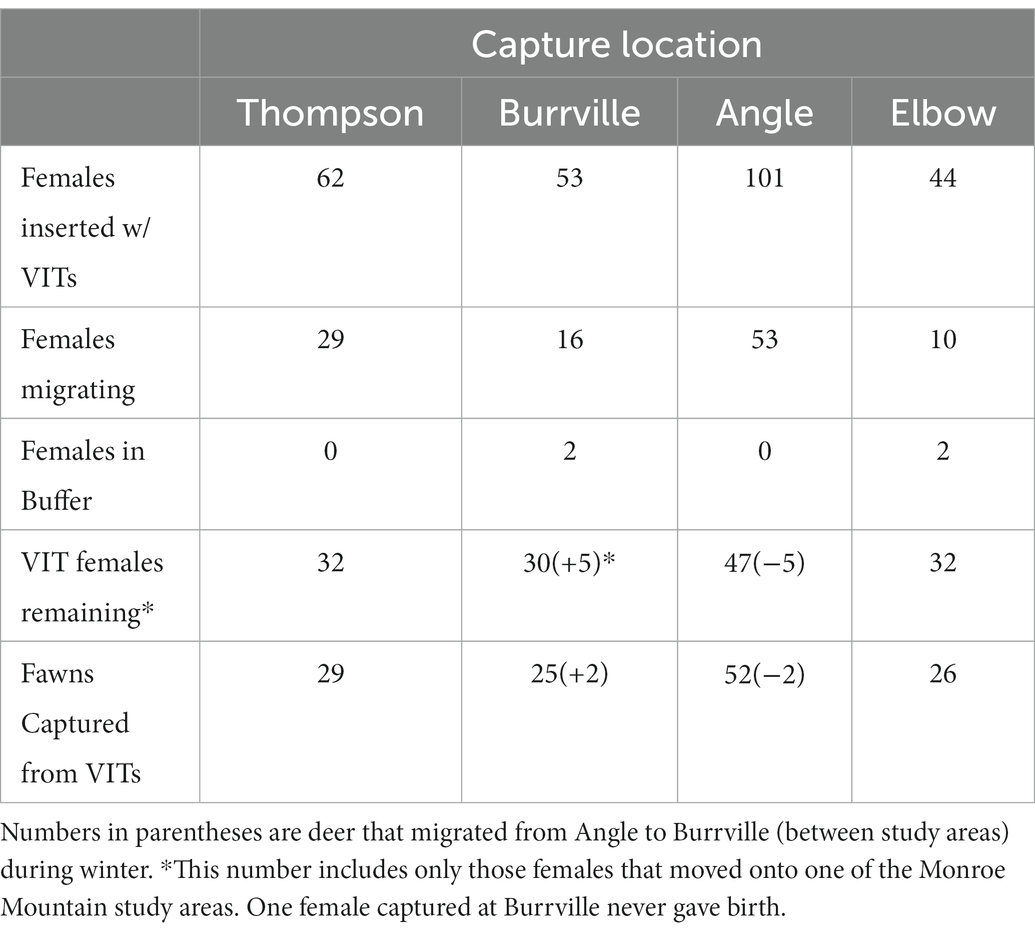
Table 1. The distribution of female mule deer captured from four regions of wintering range around Monroe Mountain, Utah during 2012–2015, including the number of captured animals that migrated to a different summer range and those with VITs that moved onto Monroe Mountain.
During late winter and early spring 2012–2015, Wildlife Services removed a total of 166 coyotes. These removals included 47, 34, 38, and 47 in 2012, 2013, 2014, and 2015, respectively. In 2012 and 2013, coyote removal efforts were on the north study area whereas removal efforts focused on the south study area in 2014 and 2015 (Figure 1). Ten coyotes were removed from the buffer area or from outside the study area. Each of these animals had at least part of their home range within the study area as all tracking events to locate a coyote were initiated within the removal study area.
Searches for neonate mule deer associated with expulsion of VITs began on 29 May, when the first VIT was expelled, and continued through early July. Based on expulsion of VITs, we obtained dates of parturition for 140 of the 146 females that remained in the study area during parturition. Five females died prior to parturition, and one female that received a VIT never gave birth. Mean dates of parturition were June 13th, 16th, 15th, and 14th for 2012–2015, respectively. Using VITs and opportunistic searching, we captured 266 neonate mule deer, including 71 sets of twins, between the two study areas. We excluded six neonates from our sample because they were stillborn (n = 2) or their deaths were human-caused (poaching, vehicle strike; n = 4); consequently, 260 neonates were used in final analyses.
We attributed mortality of neonate mule deer to predation, starvation, disease, stillbirth, roadkill, and unknown (Table 2). Predation was the leading cause of mortality accounting for approximately 68% of all mortalities. Coyotes killed 16% of all collared neonates on the north study area and 13% of all neonates monitored on the south study area. Approximately 90% of coyote-related mortality occurred during the first 16 weeks of life for neonates.
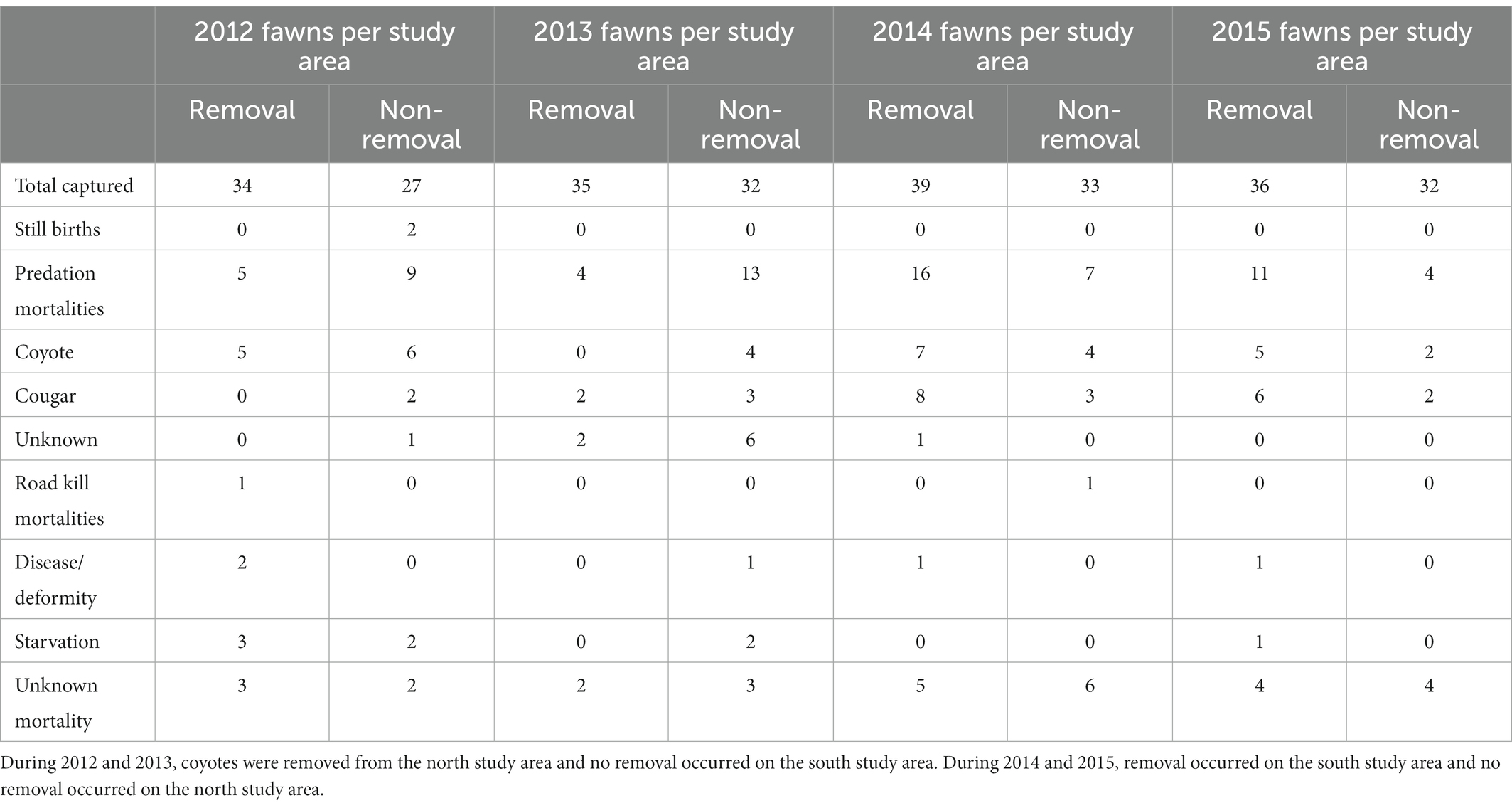
Table 2. The distribution and probable causes of mortality of neonate mule deer that were captured on Monroe Mountain, Utah during 2012–2015.
Survival of neonate mule deer to 16 weeks averaged 65% across all years. Sex and weight were influential variables in our models (Table 3). Females had a higher likelihood of survival than males. Additionally, survival of neonate mule deer increased as weight increased. None of our models contained uninformative parameters (Arnold, 2010).
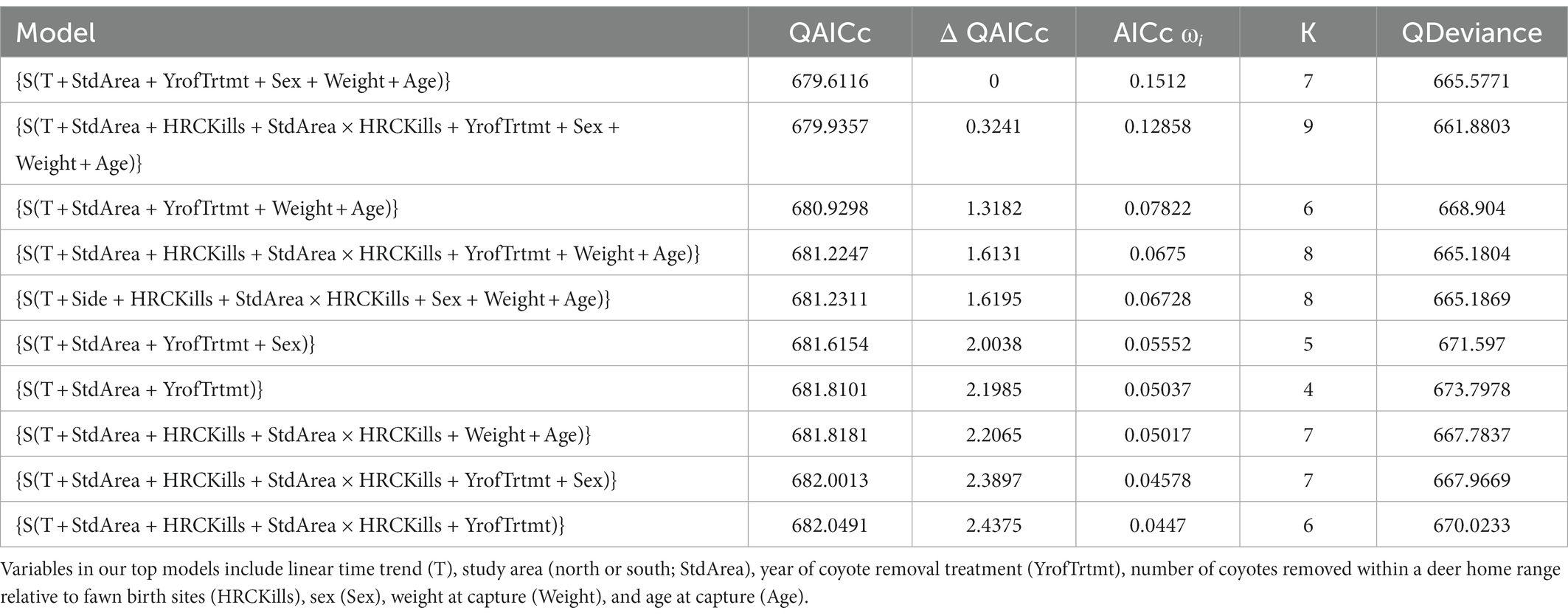
Table 3. Model selections results for survival of neonate mule deer through 16 weeks of age on Monroe Mountain, Utah during 2012–2015.
Number of consecutive years of treatment (YrofTrtmt) was a prominent variable in our known-fate analyses, appearing in 8 of the top 10 models (Table 3). Likelihood of survival to 16 weeks of age for fawns in a treatment area with no coyote removal was 57.6% ± 5.1% (±SE). In contrast, likelihood of survival to 16 weeks of age increased to 65.4% ± 7.9% and 72.8% ± 5.1% with one and two consecutive years of coyote removal, respectively. Survival curves from a Kaplan–Meier function illustrated the pattern of survival relative to year of treatment (Figure 2).
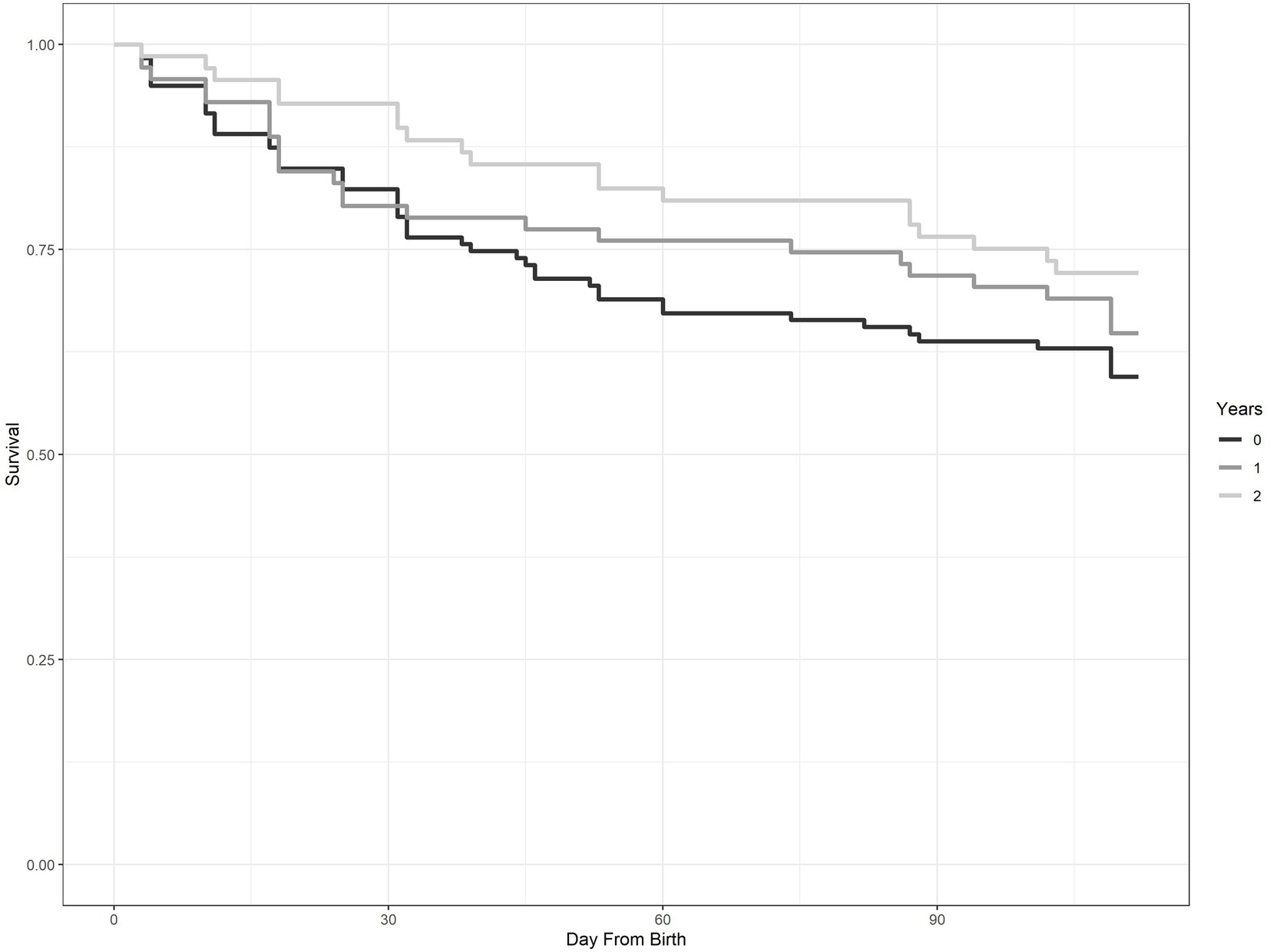
Figure 2. Survival curves for neonate mule deer produced using a Kaplan–Meier function. The three curves illustrate survival of fawns with no removal of coyotes (0), 1 year of removal of coyotes (1), and 2 years of removal of coyotes (2). This study was conducted on Monroe Mountain, Utah, United States during 2012–2015.
The location of coyote removal relative to birth sites (HRCKills) appeared in 6 of the top 10 models which accounted for 40% QAICc weight. The support for HRCKills in our models indicated that proximity of coyote removal to fawning locations positively influenced the likelihood of survival of neonate mule deer (Table 3). The probability of a neonate mule deer surviving to 16 weeks increased with increasing numbers of coyotes removed in a deer home range surrounding birth locations (Figure 3).
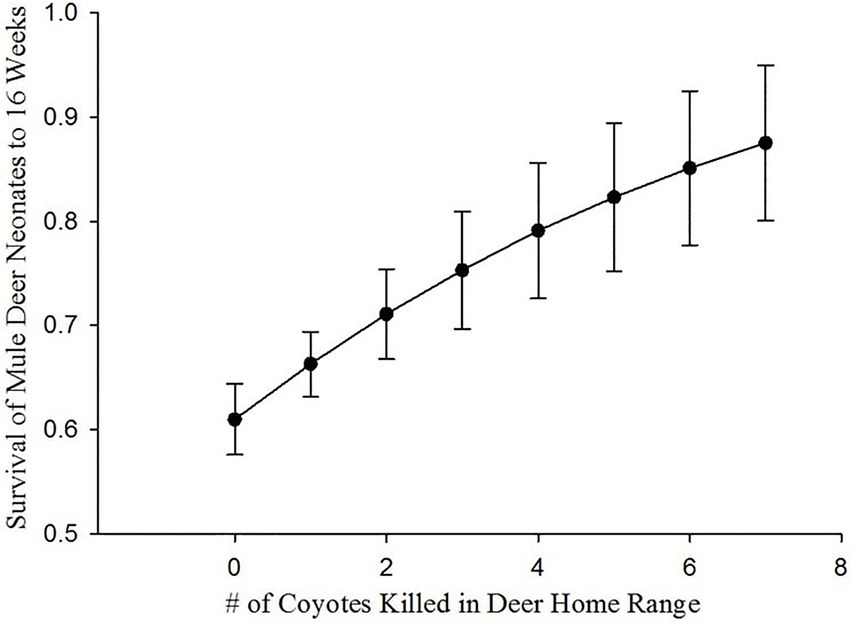
Figure 3. Probability of neonate mule deer surviving to 16 weeks of age on Monroe Mountain, Utah, United States during 2012–2015 based on the number of coyotes removed within 2.25 km (average diameter of a mule deer home range) of the birth site.
Discussion
Removing coyotes had a positive effect on survival of neonate mule deer to 16 weeks of age regardless of the density of alternate prey. Importantly, subsequent years of coyote control in the same area had a greater effect than a single year of control (Figure 2). This result is consistent with a study on pronghorn (Antilocapra americana) that highlighted the need for multi-year coyote removal to increase fawn survival (Smith et al., 1986). Even under intense coyote removal programs, coyotes have been reported to repopulate areas within months or a short number of years (Beasom, 1974; Connolly and Longhurst, 1975; Connolly, 1995). Additionally, some coyote populations have returned to pre-treatment levels through recolonization, compensatory breeding, and increased survival rates following coyote removal (Knowlton, 1972; Knowlton et al., 1999). Our findings underscore the need for consecutive annual removal efforts, with consistent removal among years, to have positive effects on survival of mule deer fawns.
Removing coyotes near birth sites of fawns increased fawn survival, whereas removing coyotes from areas distant from birth sites did not influence survival of neonates. Coyote removal is often conducted during winter months because coyotes leave tracks in the snow that managers can use to locate and remove them. Since coyotes generally use the same areas in winter as they do in summer on Monroe Mountain (Mahoney, 2017), removing coyotes from potential fawning habitat in winter would likely benefit neonate mule deer the following summer. Our results support this prediction. This finding is notable because many coyote removal studies do not consider proximity to prey birth sites when removing coyotes, which may explain the equivocal impacts on fawn survival that have been reported, if some studies removed coyotes far away from prey birth sites. Our results underscore the need for coyote removal programs to target coyotes near fawning habitat in order to be effective, whereas removal programs targeting coyotes distant from fawning habitat likely have no effect on prey populations.
Predation was the leading cause of mortality of neonate mule deer accounting for at least 68% of all mortalities. It is likely this estimate, however, is low and underestimates the actual effects of predators. For example, it is probable that at least some of the cases we assigned to starvation were due to the mother being killed by a predator resulting in the subsequent starvation of the neonate. In fact, we had multiple instances where we were able to determine this was the case by locating the deceased collared mother of the neonate mule deer that had starved. However, we do not know the full extent of this type of mortality because many of our collared neonates were opportunistically captured from unmarked adults. If populations are not limited by resources or there is capacity for the landscape to support a larger population, it is likely that reducing predation, the leading cause of mortality, will lead to an increase in population size.
The availability of alternative prey did not appear in any of our top models suggesting that alternative food was not a driving factor in the patterns of predation on mule deer that we observed. This finding is inconsistent with previous research demonstrating that alternative prey can influence the rate of predation on neonate mule deer (Hamlin et al., 1984). However, Sacks and Neale (2002) demonstrated that small prey comprise only a minor portion of coyote diet when neonate sheep are present on the landscape. We suggest that neonate mule deer are an optimal food source for coyotes due to their large size and relative ease of capture. Therefore, coyotes potentially take neonate mule deer selectively when available. Coyotes did not start killing neonate mule deer until approximately the mean date of parturition each year (Hall, 2018), suggesting that the predators were unaware of the presence of the new food source on the landscape initially. Once coyotes started killing neonates, loss to coyotes became common suggesting that the predators shifted their behavior to target neonate mule deer. Indeed, some generalist predators switch to being a specialist when a potentially preferred prey becomes available (Roughgarden, 1974). A possible alternative explanation for the pattern we observed is that lagomorphs (i.e., alternative prey) were not randomly distributed with greater distribution at low elevations, whereas fawning habitat was at relatively high elevations. If coyotes do not transition up and down the mountain as suggested by Mahoney (2017), coyotes living in areas with mule deer neonates did not have access to lagomorphs, and therefore, shifts in abundance of lagomorphs should not influence taking of neonate mule deer.
Our study focused on spatial aspects of predator control and the effect of two consecutive years vs. a single year of coyote removal on fawn survival. Our results indicate that predator control efforts focused on fawning habitat have the greatest likelihood of leading to increased population growth. This finding is especially meaningful because there were significant sources of error (e.g., variation in the spatial density of predators and prey) that our study could not control. In addition to the spatial effects of predator control efforts, we found that two consecutive years of predator control are better than a single year. While it would be ideal to test the effect of additional consecutive years, it is difficult to maintain optimal experimental conditions for extended durations. As we described in the Introduction, the study by Hurley et al. (2011) took place over 6 years, but coyote removal numbers varied drastically from year to year in that study (we maintain that their study was a feat despite this limitation). Focusing on only 2 years of removal using a crossover design allowed us to maintain consistent removal efforts and carry out our desired goal of experimentally evaluating the effect of removal years, but it also precluded our ability to thoroughly examine population growth in response to our removal efforts given the short timeframe. Although increasing the survival rates of neonate ungulates can increase recruitment and the rate of population growth, it is possible that predator-related mortality is compensatory and not additive—especially for populations that are limited by the availability of nutritional resources. Therefore, it is crucial for future studies to determine the parameters of a prey population where increased fawn survival resulting from multiple consecutive years of consistent coyote removal translates to positive population growth. Further, future studies should determine how long the effect of predator removal lasts after removal efforts cease. Nevertheless, our results are valuable given that we were able to experimentally demonstrate the effect of multiple years vs. a single year of removal on fawn survival in a controlled naturalistic setting.
Managing for robust deer populations is often a high priority for agencies charged with managing wildlife. Our results indicate that coyote control can increase survival of neonate mule deer. Coyote control increased survival when (1) control efforts occurred for multiple consecutive years, and (2) when control efforts occurred in or near fawning habitat. Therefore, efforts to control predators should occur at relatively high elevation with shrubby understory consistent with the location of fawning habitat (Long et al., 2009; Freeman, 2014). Likewise, efforts should occur when populations have room to grow (size is below carrying capacity) to decrease the likelihood of compensatory mortality.
Data availability statement
The raw data supporting the conclusions of this article will be made available by the authors, without undue reservation.
Ethics statement
The animal study was reviewed and approved by Brigham Young University Institutional Animal Care and Use Committee.
Author contributions
BM, KH, and RL conceived the ideas and designed the study and its methods. JH and EF conducted much of the fieldwork and data collection. JH and RL conducted the statistical analyses and produced the figures. BM and JH drafted the initial version of the manuscript. All authors contributed to the article and approved the submitted version.
Funding
Funding was provided by Sportsmen for Fish and Wildlife, Mule Deer Foundation, Utah Division of Wildlife Resources (Contract #126240), Brigham Young University, and Federal Aid in Wildlife Restoration.
Acknowledgments
We acknowledge the efforts of district biologist, V. Mumford, for overseeing captures of adult deer and coordination of volunteers. Locating and capturing the many neonate animals incorporated into this study would not have been possible without the contributions of many volunteers and field technicians. We also acknowledge Quicksilver Air, Inc., Leading Edge Aviation, and Helicopter Wildlife Services for mule deer capture efforts. USDA Wildlife Services performed the predator removal portion of the study. We thank S. Lee for providing comments and edits on a previous version of this manuscript.
Conflict of interest
The authors declare that the research was conducted in the absence of any commercial or financial relationships that could be construed as a potential conflict of interest.
Publisher’s note
All claims expressed in this article are solely those of the authors and do not necessarily represent those of their affiliated organizations, or those of the publisher, the editors and the reviewers. Any product that may be evaluated in this article, or claim that may be made by its manufacturer, is not guaranteed or endorsed by the publisher.
References
Arnold, T. W. (2010). Uninformative parameters and model selection using Akaike’s information criterion. J. Wildl. Manag. 74, 1175–1178. doi: 10.1111/j.1937-2817.2010.tb01236.x
Bartmann, R. M., White, G. C., and Carpenter, L. H. (1992). Compensatory mortality in a Colorado mule deer population. Wildl. Monogr. 121, 1–39.
Beasom, S. L. (1974). Relationships between predator removal and white-tailed deer net productivity. J. Wildl. Manag. 38, 854–859. doi: 10.2307/3800056
Bergman, E. J., Doherty, P. F., White, G. C., and Holland, A. A. (2015). Density dependence in mule deer: a review of evidence. Wildl. Biol. 21, 18–29. doi: 10.2981/wlb.00012
Bergstrom, B. J. (2017). Carnivore conservation: shifting the paradigm from control to coexistence. J. Mammal. 98, 1–6. doi: 10.1093/jmammal/gyw185
Bishop, C. J., White, G. C., Freddy, D. J., Watkins, B. E., and Stephenson, T. R. (2009). Effect of enhanced nutrition on mule deer population rate of change. Wildl. Monogr. 172, 1–28. doi: 10.2193/2008-107
Bishop, C. J., White, G. C., and Lukacs, P. M. (2008). Evaluating dependence among mule deer siblings in fetal and neonatal survival analyses. J. Wildl. Manag. 72, 1085–1093. doi: 10.2193/2007-423
Bleich, V. C., and Taylor, T. J. (1998). Survivorship and cause-specific mortality in five populations of mule deer. Great Basin Naturalist 58, 265–272.
Blejwas, K. M., Sacks, B. N., Jaeger, M. M., and McCullough, D. R. (2002). The effectiveness of selective removal of breeding coyotes in reducing sheep predation. J. Wildl. Manag. 66, 451–462. doi: 10.2307/3803178
Bowyer, R. T. (1987). Coyote group size relative to predation on mule deer. Mammalia 51, 515–526. doi: 10.1515/mamm.1987.51.4.515
Burnham, K. P., and Anderson, D. R. (1998). Practical use of the information-theoretic approach. In model selection and inference. New York: Springer Publishing Company.
Clark, T. J., and Hebblewhite, M. (2021). Predator control may not increase ungulate populations in the future: a formal meta-analysis. J. Appl. Ecol. 58, 812–824. doi: 10.1111/1365-2664.13810
Cook, R. C., Stephenson, T. R., Myers, W. L., Cook, J. G., and Shipley, L. A. (2007). Validating predictive models of nutritional condition for mule deer. J. Wildl. Manage. 71, 1934–1943.
Connell, J. H., and Sousa, W. P. (1983). On the evidence needed to judge ecological stability or persistence. Am. Nat. 121, 789–824. doi: 10.1086/284105
Connolly, G. E. (1978). “Predators and predator control” in Big game of North America: Ecology and management. eds. D. L. Gilbert and J. L. Schmidt (Mechanicsburg, Pennsylvania: Stackpole Books), 369–394.
Connolly, G. E. (1995). The effects of control on coyote populations: another look. In: Symposium proceedings--coyotes of the southwest: a compendium of our knowledge, 36.
Connolly, G. E., and Longhurst, W. M. (1975). The effects of control on coyote populations: a simulation model. Univ. California Division Agricult. Sci. Bull. 1872, 1–37.
Freeman, E. D. (2014). Parturition of mule deer in southern Utah: Management implications and habitat selection. M.S. Thesis. Brigham Young University.
Gaillard, J. M., Festa-Bianchet, M., and Yoccoz, N. G. (1998). Population dynamics of large herbivores: variable recruitment with constant adult survival. Trends Ecol. Evol. 13, 58–63. doi: 10.1016/S0169-5347(97)01237-8
Gese, E. M., and Grothe, S. (1995). Analysis of coyote predation on deer and elk during winter in Yellowstone National Park, Wyoming. Am. Midl. Nat. 133, 36–43. doi: 10.2307/2426345
Hall, J. T. (2018). Survival of neonate mule deer fawns in southern Utah: Effects of coyote removal and synchrony of parturition. M.S. Thesis. Brigham Young University.
Hamlin, K. L., Riley, S. J., Pyrah, D., Dood, A. R., and Mackie, R. J. (1984). Relationships among mule deer fawn mortality, coyotes, and alternate prey species during summer. J. Wildl. Manage. 48, 489–499.
Harrison, D. J. (1992). Dispersal characteristics of juvenile coyotes in Maine. J. Wildl. Manag. 56, 128–138. doi: 10.2307/3808800
Hurley, M. A., Unsworth, J. W., Zager, P., Hebblewhite, M., Garton, E. O., Montgomery, D. M., et al. (2011). Demographic response of mule deer to experimental reduction of coyotes and mountain lions in southeastern Idaho: Résponse Démographique du Cerf Mulet à la Réduction Expérimentale des populations de coyotes et de pumas dans le Sud de l’Idaho. Wildl. Monogr. 178, 1–33. doi: 10.1002/wmon.4
Jager, K. J., van Dijk, P. C., Zoccali, G., and Dekker, F. W. (2008). The analysis of survival data: the Kaplan-Meier method. Kidney Int. 74, 560–565. doi: 10.1038/ki.2008.217
Kaplan, E. L., and Meier, P. (1958). Nonparametric estimation from incomplete observations. J. Am. Stat. Assoc. 53, 457–481. doi: 10.1080/01621459.1958.10501452
Knowlton, F. F. (1972). Preliminary interpretations of coyote population mechanics with some management implications. J. Wildl. Manag. 36, 18–31.
Knowlton, F. F., Gese, E. M., and Jaeger, M. M. (1999). Coyote depredation control: an interface between biology and management. J. Range Manage. 52, 398–412. doi: 10.2307/4003765
Lingle, S. (2000). Seasonal variation in coyote feeding behavior and mortality of white-tailed deer and mule deer. Can. J. Zool. 78, 85–99. doi: 10.1139/z99-171
Lomas, L. A., and Bender, L. C. (2007). Survival and cause-specific mortality of neonatal mule deer fawns, north-Central New Mexico. J. Wildl. Manag. 71, 884–894. doi: 10.2193/2006-203
Long, R. A., Kie, J. G., Bowyer, R. T., and Hurley, M. A. (2009). Resource selection and movements by female mule deer Odocoileus hemionus: effects of reproductive stage. Wildl. Biol. 15, 288–298. doi: 10.2981/09-003
Mahoney, P. J. (2017). Spatial ecology of coyotes and cougars: Understanding the influence of multiple prey on the spatial interactions of two predators. Ph.D. Dissertation. Utah State University.
Mitchell, B. R., Jaeger, M. M., and Barrett, R. H. (2004). Coyote depredation management: current methods and research needs. Wildl. Soc. Bull. 32, 1209–1218.
Peek, J., Dale, B., Hristienko, H., Kantar, L., Loyd, K., Mahoney, S., et al. (2012). “Management of large mammalian carnivores in North America,’’ in The Wildlife Society Technical Review. (Bethesda, Maryland, USA: The Wildlife Society), 12–01.
Peek, J. M., Dennis, B., and Hershey, T. (2002). Predicting population trends of mule deer. J. Wildl. Manag. 66, 729–736. doi: 10.2307/3803138
Pojar, T. M., and Bowden, D. C. (2004). Neonatal mule deer fawn survival in west-Central Colorado. J. Wildl. Manag. 68, 550–560. doi: 10.2193/0022-541X(2004)068[0550:NMDFSI]2.0.CO;2
Robinette, W. L., Jones, D. A., Rogers, G., and Gashwiler, J. S. (1957). Notes on tooth development and wear for Rocky Mountain mule deer. J. Wildl. Manage. 21, 134–153.
Roughgarden, J. (1974). Niche width: biogeographic patterns among Anolis lizard populations. Am. Nat. 108, 429–442. doi: 10.1086/282924
Sacks, B. N., and Neale, J. C. C. (2002). Foraging strategy of a generalist predator toward a special prey: Coyote predation on sheep. Ecol. Appl. 12, 299–306.
Salo, P., Banks, P. B., Dickman, C. R., and Korpimäki, E. (2010). Predator manipulation experiments: impacts on populations of terrestrial vertebrate prey. Ecol. Monogr. 80, 531–546. doi: 10.1890/09-1260.1
Severinghaus, C. W. (1949). Tooth development and wear as criteria for age in white-tailed deer. J. Wildl. Manage. 13, 195–216.
Smith, R. B., and Lindzey, F. G. (1982). Use of ultrasound for detecting pregnancy in mule deer. J. Wildl Manage. 46, 1089–1092.
Smith, R. H., Neff, D. J., and Woolsey, N. G. (1986). Pronghorn response to coyote control: a benefit: cost analysis. Wildl. Soc. Bull. 14, 226–231.
Stoddart, L. C., Griffiths, R. E., and Knowlton, F. F. (2001). Coyote responses to changing jackrabbit abundance affect sheep predation. J. Range Manag. 54, 15–20.
Unsworth, J. W., Pac, D. F., White, G. C., and Bartmann, R. M. (1999). Mule deer survival in Colorado, Idaho, and Montana. J. Wildl. Manag. 63, 315–326. doi: 10.2307/3802515
Watine, L. N., and Giuliano, W. M. (2017). Factors determining coyote (Canis latrans) diets. Open J. Ecol. 07, 650–666. doi: 10.4236/oje.2017.713045
Webb, S. L., Dzialak, M. R., Houchen, D., Koseiuch, K. L., and Winstead, J. B. (2013). Spatial ecology of female mule deer in an areas proposed for wind energy development. Western North American Naturalist 73, 347–356. doi: 10.3398/064.073.0308
White, G. C., and Burnham, K. P. (1999). Program MARK: survival estimation from populations of marked animals. Bird Study 46, S120–S139. doi: 10.1080/00063659909477239
Keywords: mule deer, Odocoileus hemionus, predation, predator control, neonate survival, coyote, Canis latrans
Citation: McMillan BR, Hall JT, Freeman ED, Hersey KR and Larsen RT (2023) Both temporal and spatial aspects of predator management influence survival of a temperate ungulate through early life. Front. Ecol. Evol. 11:1087063. doi: 10.3389/fevo.2023.1087063
Edited by:
Vernon Bleich, University of Nevada, Reno, United StatesReviewed by:
Levi Newediuk, University of Manitoba, CanadaMitchell J. Eaton, United States Geological Survey (USGS), United States
Copyright © 2023 McMillan, Hall, Freeman, Hersey and Larsen. This is an open-access article distributed under the terms of the Creative Commons Attribution License (CC BY). The use, distribution or reproduction in other forums is permitted, provided the original author(s) and the copyright owner(s) are credited and that the original publication in this journal is cited, in accordance with accepted academic practice. No use, distribution or reproduction is permitted which does not comply with these terms.
*Correspondence: Brock R. McMillan, ✉ YnJvY2tfbWNtaWxsYW5AYnl1LmVkdQ==