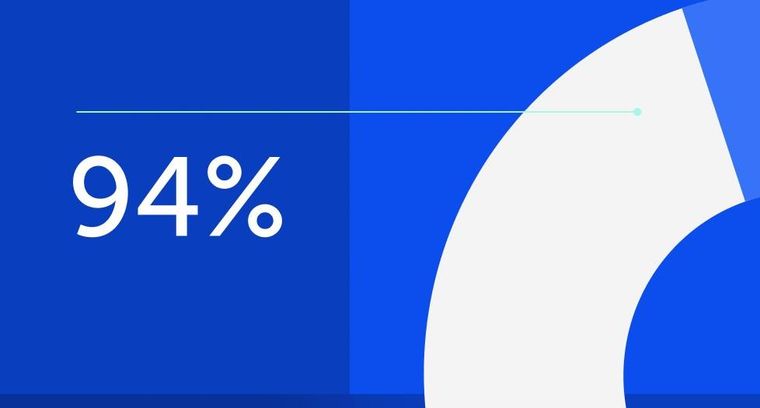
94% of researchers rate our articles as excellent or good
Learn more about the work of our research integrity team to safeguard the quality of each article we publish.
Find out more
ORIGINAL RESEARCH article
Front. Ecol. Evol., 23 March 2023
Sec. Conservation and Restoration Ecology
Volume 11 - 2023 | https://doi.org/10.3389/fevo.2023.1087058
This article is part of the Research TopicAdvances in the Conservation of Large Terrestrial MammalsView all 10 articles
Translocation of large mammals has become common practice for wildlife managers charged with conservation of animals and their genetic integrity on increasingly modified landscapes. Translocations of ungulates have occurred around the world with varying outcomes. Although translocations have been used to manage mule deer (Odocoileus hemionus) in western North America, only recently have the outcomes associated with this management practice been documented. Our objective was to evaluate survival of translocated mule deer in comparison to resident mule deer over multiple years following release and provide information useful in judging the relative value of translocation as a conservation strategy for this species. In January and March 2013, the Utah Division of Wildlife Resources (UDWR) captured and translocated 102 mule deer from winter range near Parowan, Utah to winter range near Holden, Utah (approximately 145 kilometers north of capture location). We fitted each deer with a radio transmitter (n = 102 total: 21 GPS collars, 81 VHF collars) prior to release. We also captured and marked a total of 70 resident deer (9 GPS collars, 61 VHF collars) to serve as a reference group. Survival of translocated deer in the first year was similar among release dates in January (0.51; 95% CI = 0.40–0.63) and March (0.53; 95% CI = 0.40–0.66). Annual survival of translocated deer, however, was lower than survival of resident deer (0.83; 95% CI = 0.72–0.90) in the first year after release. During the second year following release, however, survival of translocated animals (0.85; 95% CI = 0.71–0.93) was not different from that of resident deer (0.80; 95% CI = 0.69–0.88). Additionally, age strongly influenced the survival of translocated deer; young deer (e.g., 1.5 year olds) were more than twice as likely as old deer (e.g., 7.5 year olds) to survive the initial year following translocation. These data highlight the need to monitor translocated animals for multiple years following release and suggest that wildlife managers should expect to see higher survival rates during the second year following translocation and higher survival rates in younger deer compared to older deer.
Translocation is an increasingly common strategy for managing large mammals and this approach has been applied to a variety of species in many parts of the world. Typical goals of translocation include reducing population density in the source area, supplementing existing populations in the release area, reestablishing extirpated populations, introducing new populations, and increasing genetic diversity (Griffith et al., 1989; Baxter et al., 2008). Although there have been successes, translocation efforts do not always produce positive outcomes. In a review of translocations from around the world, it was estimated that more than 25% of those involving mammals ended in failure (Wolf et al., 1996).
Reasons for failure included movement of translocated individuals out of release areas, limited reproduction by translocated individuals, and low genetic diversity due to founder effects (Mock et al., 2004; Dickens et al., 2009a). Recent evidence further suggests that translocation can alter stress physiology, thereby creating survival challenges for released individuals (Dickens et al., 2009b). For some species, a positive relationship with the number of released individuals and translocation success has been observed (Griffith et al., 1989; Wolf et al., 1996; Singer et al., 2000). For others, the details associated with the release itself (e.g., hard versus soft release or time of year) are important predictors of success (Bright and Morris, 1994). Results of translocation for large mammals often vary across species creating a need for species-specific information.
Despite decades of intensive management in western North America, outcomes associated with translocation of mule deer (Odocoileus hemionus) have only recently been documented. Typical results show increased movements and reduced survival for translocated animals in the initial year following release (Wakeling, 2003; Cain et al., 2018; Howard, 2018; Smedley et al., 2019; Wright et al., 2020). Annual survival of translocated mule deer during the initial year following release, for example, ranged from 15 to 79 percent which is low for this species (Wakeling, 2003; Cain et al., 2018; Wright et al., 2020). Most of these efforts, however, did not involve monitoring of translocated mule deer during their second year following release or comparison with resident deer in the release area.
Our objective was to document the outcomes associated with translocation of mule deer. More specifically, we evaluated the survival of translocated mule deer in relation to timing of release (early versus late winter) and individual covariates such as age and body condition. Mule deer released in early winter would have more time to integrate with resident deer prior to spring migration and be in relatively good condition compared to deer released in March that were expected to be in relatively poor body condition and would have less time to integrate with resident deer prior to spring migration, but perhaps more likely to stay near release areas due to their relatively poor condition. We predicted that translocated mule deer would experience lower survival rates than resident deer during the first year following release. During the second year following release, however, we predicted survival rates for translocated deer would be higher than those observed in year one, similar to what has been documented for white-tailed deer (Odocoileus viginianus) and elk (Cervus canadensis) (sensu Frair et al., 2007; Foley et al., 2008). We further expected covariates such as age and body condition to influence survival rates (Hawkins and Montgomery, 1969; Jones and Witham, 1990; Haydon et al., 2008).
Translocated deer were captured from the Parowan front in southern Utah, United States which is winter range for mule deer in the Panguitch management unit (Figure 1). The predominant geographic feature in the Panguitch management unit is the Markagaunt Plateau which is approximately 91 km long (north to south) and 34 km wide (at its widest point). Mean high air temperatures during the summer and winter months over the past century were 29.4° C and 6.6° C respectively, with average annual precipitation of 31.0 cm at 1862 m (Western Regional Climate Center). Elevations across this mountain range varied from 1762 to 3,446 m. Mule deer in this area were thought to migrate seasonally using high-elevation areas in summer and low-elevation areas during winter. Habitat types at high-elevation included areas dominated by aspen (Populus tremuloides), bitterbrush (Purshia tridentata), mountain mahogany (Cercocarpus ledifolius), and Gambel’s oak (Quercus gambelli). The winter range along the Parowan front was dominated by juniper (Juniperus sp.), pinion (Pinus edulis), and sagebrush (Artemisia sp.). Over the last decade, population estimates for this deer herd have exceeded management objectives and the quality of the winter range was rated in only poor to fair condition (UDWR, 2013, 2014).
Figure 1. Map of Utah, United States showing our study area where the Utah Division of Wildlife Resources (UDWR) captured (circle) and released (star) mule deer (Odocoileus hemionus) in January and March of 2013.
Translocated deer were released by the Utah Division of Wildlife Resources (UDWR) onto the Pahvant mountain range (approximately 144 km north of capture areas) in central Utah (Figure 1). The Pahvant study area was chosen for release due to similarities with the Parowan capture area. These similarities included north to south mountain ranges, migratory deer herds with west to east migration, similar climates, and winter ranges bordered by Interstate 15 on the west and high-elevation mountains on the east. The Pahvant Mountain Range is approximately 54 km long (north to south) and 22 km wide at its widest point. Elevations across this mountain range varied from 1,520–3,117 m. Mean high temperatures during the summer and winter months over the past century were 31.4° C and 5.7° C, respectively, with average annual precipitation of 38.1 cm at 1,552 m (Western Regional Climate Center). The winter range along the foothills of the Pahvant mountain range was dominated by bitterbrush, cliffrose (Purshia stansburiana), Gambel’s oak, juniper, mountain mahogany, and sagebrush. Higher elevation areas were composed of mixed brush communities, aspen, and a variety of conifers (e.g., genus Abies and Pinus) and juniper. Unlike the Parowan front, the deer population on the Pahvant range has consistently been below management objectives and the release area was considered by UDWR to consist of high-quality winter range (UDWR, 2012). Potential predators of mule deer inhabiting both the area where deer were captured from and the release area included black bears (Ursus americanus), bobcats (Lynx rufus), coyotes (Canis latrans), and mountain lions (Puma concolor).
In January and March 2013, UDWR contracted with a private helicopter company to capture female mule deer via helicopter net-gunning (Krausman et al., 1985; Van de Kerk et al., 2020) and sling them to 3 different staging areas along the Parowan front in southern Utah (Figure 1). During handling, we weighed deer and then estimated age (via tooth wear and eruption pattern; Severinghaus, 1949; Robinette et al., 1957), body size (chest, hind foot length, neck girth), condition (body condition score method; Cook et al., 2007), and pregnancy (via transabdominal ultrasound; E.I. Medical Imaging portable ultrasound; Smith and Lindzey, 1982). Preliminary results suggest >80% accuracy within 2 years when aging mule deer in Utah from tooth wear (Hinton et al., 2023). UDWR administered 3 cc banimine and 1.5 cc ivermectin to each individual deer and fitted them with a radio collar (VHF or GPS) and unique ear tag. UDWR also conducted rectal biopsies to test for chronic wasting disease (Thomsen et al., 2012) and collected blood samples to verify pregnancy. Following the handling process, UDWR loaded mule deer in stock trailers and drove them to the Pahvant range (Figure 1) where the majority were immediately released (hard release; average of 6.9 h between capture and release; range 1.5 to 17.9 h). To serve as a reference group, resident deer in the Pahvant study area were also captured and radio-marked (VHF or GPS). Resident deer were fitted with a radio collar by the capture company and released immediately at point of capture. For additional detail on capture and release protocols see Smedley (2016) or Smedley et al. (2019).
Following release, we used radio telemetry from the ground (weekly) and fixed-wing aircraft (approximately monthly, n = 19 different flights over 2 years) to locate and assess the status (alive or dead) of each radio-marked deer throughout the year. When mortality signals were detected (triggered after 8 h of inactivity), we located the carcass as soon as possible to determine cause of death by postmortem examination and evidence (tracks, cached carcass, feces, etc.) from the surrounding area (Rominger et al., 2004; Kilgo et al., 2012). When we found carcasses that showed no signs of predation or vehicle impact, we collected them for necropsy by the Utah State University, Veterinary Diagnostics Laboratory. We classified mortalities as predation, undetermined, capture-related (capture myopathy and capture-related injuries), roadkill, poached, or other which included diseases not directly associated with capture.
We used model selection and known-fate models within Program MARK (White and Burnham, 1999) to estimate seasonal and annual survival for each group of mule deer (resident, January release, and March release) and evaluate support for covariates that included age, body mass, body condition, and pregnancy. We formatted our encounter history by month and year beginning 1 January and ending 31 December for both 2013 and 2014. Structuring our encounter history by year (i.e., year as a group) allowed us to graduate deer (in age) and easily obtain unique estimates of annual survival for resident and translocated deer in year 1 and year 2 following release. We evaluated relative model support using Akaike’s Information Criterion (Akaike, 1973) adjusted for small sample sizes (AICc) and then used model averaging based on AICc weights to produce estimates of annual survival (Burnham and Anderson, 2002). To evaluate differences across groups (e.g., resident versus translocated deer), we looked for overlap in 95 percent confidence intervals associated with estimates of annual survival. To assess the influence of individual covariates, we examined confidence intervals (95%) surrounding β estimates.
We used a 3-stage, hierarchical approach to model selection to provide structure to our analysis and allow us to identify the most-supported seasonal (time), group (translocated or resident), and individual covariates (e.g., age). First, we identified the best model of time (seasonal and annual structure) while keeping survival for all groups equal. Our time models were based on month, season, year, and migration dates as well as time trends (linear and quadratic) following release. Our seasonal models included 2, 3, and 4-season models based on spring, summer, fall, and winter as well as average migration dates. Our 4-season model, for example, allowed for differential survival during the spring, summer, fall, and winter whereas the 2 and 3-season models collapsed those seasons into different combinations (e.g., spring/summer and fall/winter). We defined spring as March–May, summer as June–August, fall as September–November, and winter as December–February. We determined migration dates based on when deer left either winter or summer range and did not return (sensu Northrup et al., 2014). Second, we added the grouping structure to supported models (i.e., ≥ 0.05 AICc weight) of time from step 1. Our groups included the following: resident (2013), January translocations (2013), March translocations (2013), resident (2014), and surviving January and March translocations from 2013 that were combined in 2014 to maintain adequate precision around estimates of survival. In our final step, we evaluated the influence of individual covariates (age, age2, percent body fat, body mass, and pregnancy when available for the specific year evaluated) to models with ≥0.05 AICc weight from step 2. Inclusion of age2 allowed us to represent a potential asymptotic relationship with age. We evaluated the final list of supported models for evidence of uninformative parameters and used model averaging to avoid any potential bias (Arnold, 2010). In addition, we used a logistic regression to estimate odds ratios for annual survival in relation to group and age.
In January and March 2013, UDWR translocated 102 female mule deer (51 in January; 51 in March) from winter range near Parowan, Utah to winter range near Holden, Utah on the Pahvant mountain range (Figure 1). We marked 81 deer with VHF radio collars (41 in January, 40 in March) and 21 deer with GPS collars (10 in January, 11 in March). Estimated age of captured deer ranged from 1 to 9 (x- = 3.9 years for January and 4.1 years for March, SE = 0.03 for both January and March). Percent ingesta free body fat ranged from 3.9–16.3% and was higher in January 2013 compared to March 2013 as expected (Barboza et al., 2009, 2020). Of the 102 females captured for translocation, 91% (N = 93) were pregnant and none tested positive for chronic wasting disease. Prior to capturing deer for translocation, we marked 50 resident deer in the Pahvant study area (41 VHF, 9 GPS). An additional 20 resident deer (20 VHF) were captured during January 2014 in the Pahvant study area to assess body condition and bolster sample sizes for this reference group.
Our first stage of model selection resulted in 5 supported models of time with at least 5% AICc weight that we advanced to step 2. These models divided the year into 2, 3, and 4 seasons and accounted for 88% of the total AICc weight (Table 1). In stage 2, we added group structure to supported models of time and identified 6 models with at least 5% AICc weight (Table 1). These models included three 2-season models (based on season, year, and group), two 4-season models, and one 3-season model. The two 4-season models were defined by year and migration dates (winter, spring migration, summer, and fall migration). The 3-season model was also defined by year and migration dates with survival rates modeled as equal during winter and summer, but different during the spring and summer migrations. Four of the 6 models included a 3-group structure (residents [2013 and 2014 combined], 2013 translocations combined with January and March releases, and translocations in year 2 [2014]). The other 2 models each had 4 groups (residents [2013 and 2014 combined], 2013 January translocations, 2013 March translocations, and translocations in year 2 [2014] or 2013 residents, 2013 translocations, 2014 residents, and 2014 translocations).
Table 1. Akaike’s Information Criterion selected models of survival (s) for resident and translocated mule deer (Odocoileus hemionus) on the Pahvant Range in central, Utah, USA for 2013 and 2014.
In stage 3, we added covariates to our best models from stage 2. This stage resulted in 6 models (2, 3, and 4 seasons) with an AICc weight ≥ 5% that accounted for 73% of the total AICc weight. The top model was a 2-season model that combined spring with summer and fall with winter periods. This model had 4 separate groups (residents [2013 and 2014 combined], 2013 January translocations, 2013 March translocations, and surviving translocations in 2014) and included age and age2 as individual covariates (Table 2). Age or age2 occurred in all models with wi > 0.05 (Table 2). We found little support for body condition, pregnancy, or body mass as influencing survival as models with these covariates received <5% of AICc weight.
Table 2. Akaike’s Information Criterion selected models of survival (s) for resident and translocated mule deer (Odocoileus hemionus) on the Pahvant Range in central, Utah, United States during 2013 and 2014.
We experienced low rates of capture myopathy and capture-related deaths. Four of 102 (3.9%) deer captured and translocated during 2013 died of capture-related causes. All of these deer died within 3 days of release and 2 of the 4 deaths were attributed to injuries (e.g., broken bones) sustained during capture. Two of 70 (3%) resident deer died of capture-related causes. Predation accounted for the majority of mortalities (n = 54) for translocated deer (50%) followed by undetermined (28%), other including disease (8%), poached (8%), and roadkill (6%). Predation was also the highest cause of mortality (n = 21 deaths) for resident deer (63%) followed by undetermined (32%) and other (5%).
Overall annual survival of resident deer during 2013 was estimated at 0.83 (95% CI = 0.72–0.90). Annual survival of mule deer translocated in January during 2013 (year one following release) was 0.51 (95% CI = 0.40–0.63) compared to 0.53 (95% CI = 0.40–0.66) for mule deer translocated in March. During their second year following release, translocated deer maintained much higher survival rates with annual rates estimated at 0.85 (95% CI = 0.71–0.93). This rate was not different from that of residents in 2014 (0.80; 95% CI = 0.69–0.88; Figure 2). Resident and translocated deer experienced similar monthly survival rates during their initial year following release in winter and spring months (January through April). Beginning in May, however, and lasting through September of 2013, number of mortalities for translocated deer increased compared to those of resident deer. In October of 2013, survival rates of translocated deer stabilized and were again similar to resident deer.
Figure 2. Annual survival rates (± 95% CI) of resident (reference group) and translocated mule deer (Odocoileus hemionus) released in January of 2013 and March of 2013 on the Pahvant Range in southern Utah, United States during 2013 (year one following release) and 2014 (year two following release).
We found support for age influencing survival of translocated deer. The β estimate for age was negative in the top model (β = −0.73), although the 95% CI slightly overlapped zero (−1.61–0.14). The β estimate for age2 in the top model was positive with the 95% CI slightly overlapping zero (β = 0.06, 95% CI = −0.03-0.14). A plot of odds ratios for annual survival showed reduced likelihood of survival during the initial year for animals translocated in January and March compared to resident deer, but not during the second year following release (Figure 3). This plot also showed reduced odds of survival as age of animals increased. Estimates of annual survival for 2-year old mule deer during year 1 following release were more than double (0.71; 95% CI = 0.52–0.84) those of 7 year olds (0.35; 95% CI = 0.17–0.58) (Figure 4).
Figure 3. Odds ratios for annual survival of resident (reference group) and translocated mule deer (Odocoileus hemionus) released in January of 2013 and March of 2013 on the Pahvant Range in southern Utah, United States during 2013 (year one following release) and 2014 (year two following release). Intercept is set to resident deer during 2013.
Figure 4. Annual survival of translocated mule deer (Odocoileus hemionus) in relation to estimated age (tooth eruption and tooth wear; Severinghaus, 1949; Robinette et al., 1957) during the first 2 years (2013, year 1; 2014, year 2) following release on the Pahvant Range in central Utah, United States.
We observed very few capture-related deaths and documented low mortality rates immediately following release for translocated mule deer. Our observed 3.9% rate of mortalities associated with capture was similar to that observed with resident deer released at point of capture (3%) as well as rates common in traditional capture, radio-marking, and release projects that do not involve translocation (general range 3–5%; Quinn et al., 2012; Lendrum et al., 2014; Van de Kerk et al., 2020). The majority of mortalities we observed for translocated deer occurred during the spring and summer (May–September) months and were similar to causes reported in other areas (primarily predation, but also poaching and vehicle strikes) for mule deer (Beringer et al., 2002; Rominger et al., 2004; Frair et al., 2007; McIntosh et al., 2014).
Survival rates for translocated mule deer, during the first year post release, were lower than rates commonly observed for animals not translocated. We observed annual survival for resident deer of 0.83, which was similar to data from Colorado, Idaho, and Montana (annual survival estimated at 0.85) for mule deer (Unsworth et al., 1999). Survival rates for translocated mule deer in year one (0.51 and 0.53) were lower than those of resident deer (0.83). These lower rates during the initial year following release, however, are similar to those reported for black-tailed or mule deer translocated in other areas using a variety of methods (O’Bryan and McCullough, 1985; Martinez-Garcia, 2009; Cain et al., 2018; Wright et al., 2020). During the second year, survival rates for translocated mule deer were higher and not different from resident deer (Figure 2) suggesting that survival challenges related to translocation were transitory and dissipated by the end of the initial year. This finding supports our prediction that translocated deer would experience lower survival rates than resident deer during the first year after release, but higher rates of survival (when compared to survival for translocated deer in year 1) during the second year once acclimated to release areas. While low survival of ungulates is a common observance following translocation (Beringer et al., 2002; Frair et al., 2007), there are few translocation studies that have documented survival for multiple years following release (Haydon et al., 2008; McIntosh et al., 2014; Wright et al., 2020). These data reinforce the need to monitor translocated animals for multiple years following release.
We found no difference in annual survival rates for deer translocated in early versus late winter. Deer released in early winter (January) were in better condition at time of release than deer released later in winter, but these differences did not influence survival rates and we only observed limited mortality during winter and spring months. Moreover, we did not detect a relationship between survival and body condition. However, winter conditions during our study years were mild as temperatures were above the long-term mean and precipitation below the long-term average (Western Regional Climate Center). Translocating mule deer during a severe winter may yield different results. The results of our study did not support our hypothesis of differences in survival between early and late winter as survival rates were not different for deer released at either time. These results suggest managers could translocate mule deer throughout the winter period as survival rates were not related to the timing of release (early or late winter) when winter conditions are not severe.
We found strong support for age as a predictor of survival, with younger animals more likely to survive the initial year following translocation than older animals (Figure 4). Two year old deer, for example, were approximately 2 times more likely to survive the initial year post release than 7 year old animals. Jones and Witham (1990) found that translocated white-tailed deer fawns had higher survival that translocated adults while Hawkins and Montgomery (1969) and Parker et al. (2008) found no difference in survival based on age of translocated white-tailed deer. Moreover, higher survival for young mule deer from urban environments translocated to non-urban areas was observed in British Columbia (Wright et al., 2020). Younger animals may have more plasticity in their behavior and may have responded to novel environments better than older animals in our study. The specific mechanisms explaining this result, however, are unclear.
Our results suggest that translocation is a strategy that could be used to address conservation and management objectives for mule deer populations. We experienced low rates of capture myopathy. We observed survival rates for translocated mule deer that were lower than resident deer during the first year following translocation. During the second year after translocation, however, translocated mule deer had much higher survival rates that were not different from resident deer suggesting challenges to survival were transitory. Moreover, we found a strong relationship with age as young deer survived the initial year following translocation much better than older deer. Given the difference we observed between survival in year one compared to year two, we recommend that translocated animals be monitored for at least 2 years following release. Results from multiple years provide critical data when considering the relative value of translocation as a conservation and management strategy. In our study, there was no difference in survival rates for deer translocated in January or March. Although winters during our study period were mild, this result suggests managers can use translocation throughout winter months to address management concerns.
The raw data supporting the conclusions of this article will be made available by the authors, without undue reservation.
The animal study was reviewed and approved by Brigham Young University's IACUC Committee.
DS collected these data as part of his MS thesis. BM and RL provided oversight as graduate advisors. KH and JS helped design the study and collect the data as part of their roles as biologists for the Utah Division of Wildlife Resources. All authors contributed to the article and approved the submitted version.
This project was funded by Sportsmen for Fish and Wildlife, the Utah Division of Wildlife Resources, and Brigham Young University.
The authors thank Sportsmen for Fish and Wildlife who initiated this study and provided the necessary funding to capture, translocate, and monitor mule deer. We also thank Brigham Young University and the Utah Division of Wildlife Resources who provided additional funding and logistical support. We recognize the contributions of many field technicians and R. Peck, the district biologist in the study area, who assisted immensely throughout the study.
The authors declare that the research was conducted in the absence of any commercial or financial relationships that could be construed as a potential conflict of interest.
All claims expressed in this article are solely those of the authors and do not necessarily represent those of their affiliated organizations, or those of the publisher, the editors and the reviewers. Any product that may be evaluated in this article, or claim that may be made by its manufacturer, is not guaranteed or endorsed by the publisher.
Akaike, H. (1973). “Information theory and an extension of the maximum likelihood principle” in International Symposium on Information Theory. eds. B. N. Petran and F. Csáki. 2nd ed (Budapest, Hungary: Akadémiai Kiadi), 267–287.
Arnold, T. W. (2010). Uninformative parameters and model selection using Akaikes information criterion. J. Wildl. Manag. 74, 1175–1178. doi: 10.1111/j.1937-2817.2010.tb01236.x
Barboza, P. S., Parker, K., and Hume, I.. (2009). Integrative Wildlife Nutrition. Springer. Heidelberg, Germany.
Barboza, P. S., Shively, R., Gustine, D., and Addison, J. (2020). Winter is coming: conserving body protein in female reindeer, Caribou, and muskoxen. Front. Ecol. Evol. 8:150. doi: 10.3389/fevo.2020.00150
Baxter, R. J., Flinders, J. T., and Mitchell, D. L. (2008). Survival, movements, and reproduction of translocated greater sage-grouse in Strawberry Valley, Utah. J. Wildl. Manag. 72, 179–186. doi: 10.2193/2006-402
Beringer, J., Hansen, L. P., Demand, J. A., Sartwell, J., Wallendorf, M., and Mange, R. (2002). Efficacy of translocation to control urban deer in Missouri: costs, efficiency, and outcome. Wildl. Soc. Bull. 30, 767–774. doi: 10.2307/3784230
Bright, P. W., and Morris, P. A. (1994). Animal translocation for conservation - performance of dormice in relation to release methods, origin and season. J. Appl. Ecol. 31, 699–708. doi: 10.2307/2404160
Burnham, K. P., and Anderson, D. A.. (2002). Model Selection and Multimodel Inference: A Practical Information-Theoretic Approach. Springer-Verlag, New York,NY.
Cain, J. W. I. I. I., Ashling, J. B., and Liley, S. G. (2018). Survival and cause-specific mortality of translocated female mule deer in southern New Mexico, USA. Wildl. Res. 45, 325–335. doi: 10.1071/WR17173
Cook, R. C., Stephenson, T. R., Myers, W. L., Cook, J. G., and Shipley, L. A. (2007). Validating predictive models of nutritional condition for mule deer. J. Wildl. Manag. 71, 1934–1943. doi: 10.2193/2006-262
Dickens, M. J., Delehanty, D. J., Reed, J. M., and Romero, L. M. (2009a). What happens to translocated game birds that 'disappear'? Anim. Conserv. 12, 418–425. doi: 10.1111/j.1469-1795.2009.00265.x
Dickens, M. J., Delehanty, D. J., and Romero, L. M. (2009b). Stress and translocation: alterations in the stress physiology of translocated birds. Proc. Biol. Sci. 276, 2051–2056. doi: 10.1098/rspb.2008.1778
Foley, A. M., Pierce, B., Hewitt, D. G., DeYoung, R. W., Campbell, T. A., Hellickson, M. W., et al. (2008). Survival and movements of translocated white-tailed deer in South Texas. Proc. Annu. Con. Southeast Assoc. Fish Wildl. Agencies 62, 25–30.
Frair, J. L., Merrill, E. H., Allen, J. R., and Boyce, M. S. (2007). Know thy enemy: experience affects elk translocation success in risky landscapes. J. Wildl. Manag. 71, 541–554. doi: 10.2193/2006-141
Griffith, B., Scott, J. M., Carpenter, J. W., and Reed, C. (1989). Translocation as a species conservation tool - status and strategy. Science 245, 477–480. doi: 10.1126/science.245.4917.477
Hawkins, R. E., and Montgomery, G. G. (1969). Movements of translocated deer as determined by telemetry. J. Wildl. Manag. 33, 196–203. doi: 10.2307/3799672
Haydon, D. T., Morales, J. M., Yott, A., Jenkins, D. A., Rosatte, R., and Fryxell, J. (2008). Socially informed random walks: incorporating group dynamics into models of population spread and growth. Proc. Biol. Sci. 275, 1101–1109. doi: 10.1098/rspb.2007.1688
Hinton, M. S., McMillan, B. R., Hersey, K. R., and Larsen, R. T. (2023). Estimating age of mule deer in the field: Can we move beyond broad age categories? In revision. PLoS One
Howard, C. R. (2018). Efficacy of Translocation as a Management Tool for Urban Mule deer in Utah. MS Thesis, Utah State University, Logan, Utah, United States.
Jones, J. M., and Witham, J. H. (1990). Post-translocation survival and movements of metropolitan white-tailed deer. Wildl. Soc. Bull. 18, 434–441.
Kilgo, J. C., Ray, H. S., Vukovich, M., Goode, M. J., and Ruth, C. (2012). Predation by coyotes on white-tailed deer neonates in South Carolina. J. Wildl. Manag. 76, 1420–1430. doi: 10.1002/jwmg.393
Krausman, P. R., Hervert, J. J., and Ordway, L. L. (1985). Capturing deer and mountain sheep with a net-gun. Wildl. Soc. Bull. 13, 71–73.
Lendrum, P. E., Anderson, C. R., Monteith, K. L., Jenks, J. A., and Bowyer, R. T. (2014). Relating the movement of a rapidly migrating ungulate to spatiotemporal patterns of forage quality. Mamm. Biol. 79, 369–375. doi: 10.1016/j.mambio.2014.05.005
Martinez-Garcia, J. L. (2009). Site Fidelity and Post Release Movements of Translocated Mule deer in Northern Coahuila, Mexico. M.S. Thesis, Sul Ross State University, Alpine, Texas, United States.
McIntosh, T. E., Rosatte, R. C., Hamr, J., and Murray, D. L. (2014). Patterns of mortality and factors influencing survival of a recently restored elk population in Ontario, Canada. Restor. Ecol. 22, 806–814. doi: 10.1111/rec.12145
Mock, K. E., Latch, E. K., and Rhodes, O. E. (2004). Assessing losses of genetic diversity due to translocation: long-term case histories in Merriam's Turkey (Meleagris gallopavo merriami). Conserv. Genet. 5, 631–645. doi: 10.1007/s10592-004-1849-x
Northrup, J. M., Anderson, C. R. Jr., and Wittemyer, G. (2014). Effects of helicopter capture and handling on movement behavior of mule deer. J. Wildl. Manag. 78, 731–738. doi: 10.1002/jwmg.705
O’Bryan, M. K., and McCullough, D. R. (1985). Survival of black-tailed deer following relocation in California. J. Wildl. Manag. 49, 115–119. doi: 10.2307/3801854
Parker, I. D., Watts, D. E., Lopez, R. R., Silvy, N. J., Davis, D. S., McCleery, R. A., et al. (2008). Evaluation of the efficacy of Florida key deer translocations. J. Wildl. Manag. 72, 1069–1075. doi: 10.2193/2007-025
Quinn, A. C. D., Williams, D. M., and Porter, W. F. (2012). Postcapture movement rates can inform data-censoring protocols for GPS-collared animals. J. Mammal. 93, 456–463. doi: 10.1644/10-MAMM-A-422.1
Robinette, W. L., Jones, D. A., Rogers, G., and Gashwiler, J. S. (1957). Notes on tooth development and wear for Rocky Mountain mule deer. J. Wildl. Manag. 21, 134–153. doi: 10.2307/3797579
Rominger, E. M., Whitlaw, H. A., Weybright, D. L., Dunn, W. C., and Ballard, W. B. (2004). The influence of mountain lion predation on bighorn sheep translocations. J. Wildl. Manag. 68, 993–999. doi: 10.2193/0022-541X(2004)068[0993:TIOMLP]2.0.CO;2
Severinghaus, C. W. (1949). Tooth development and wear as criteria of age in white-tailed deer. J. Wildl. Manag. 13:195. doi: 10.2307/3796089
Singer, F. J., Papouchis, C. M., and Symonds, K. K. (2000). Translocations as a tool for restoring populations of bighorn sheep. Restor. Ecol. 8, 6–13. doi: 10.1046/j.1526-100x.2000.80061.x
Smedley, D. C. (2016). Influence of Release Timing on Survival and Movements of Tranlocated Mule Deer (Odocoileus hemionus) in Utah. MS Thesis, Brigham Young University, Provo, Utah, United States.
Smedley, D. C., van de Kerk, M., McMillan, B. R., Hersey, K. R., Whiting, J. C., and Larsen, R. T. (2019). Movements, space use and site fidelity of translocated and resident mule deer (Odocoileus hemionus). Wildl. Res. 46, 509–517. doi: 10.1071/WR19043
Smith, R. B., and Lindzey, F. G. (1982). Use of ultrasound for detecting pregnancy in mule deer. J. Wildl. Manag. 46, 1089–1092. doi: 10.2307/3808248
Thomsen, B. V., Schneider, D. A., O'Rourke, K. I., Gidlewski, T., McLane, J., Allen, R. W., et al. (2012). Diagnostic accuracy of rectal mucosa biopsy testing for chronic wasting disease within white-tailed deer (Odocoileus virginianus) herds in North America: effects of age, sex, polymorphism at PRNP codon 96, and disease progression. J. Vet. Diagn. Investig. 24, 878–887. doi: 10.1177/1040638712453582
UDWR (2012). Utah Big Game Range Trend Studies Central and Southern Regions. Utah Division of Wildlife Resources, vol. 2. Salt Lake City, Utah, United States: Utah Division of Wildlife Resources.
UDWR. (2013). Utah Big Game Range Trend Studies Southern Region. Utah Division of Wildlife Resources. Salt Lake City, Utah, United States: Utah Division of Wildlife Resources.
UDWR. (2014). Utah Big Game Annual Report. Utah Division of Wildlife Resources. Salt Lake City, Utah, United States: Utah Division of Wildlife Resources.
Unsworth, J. W., Pac, D. F., White, G. C., and Bartmann, R. M. (1999). Mule deer survival in Colorado, Idaho, and Montana. J. Wildl. Manag. 63, 315–326. doi: 10.2307/3802515
Van de Kerk, M., McMillan, B. R., Hersey, K. R., Roug, A., and Larsen, R. T. (2020). Effect of net-gun capture on survival of mule deer. J. Wildl. Manag. 84, 813–820. doi: 10.1002/jwmg.21838
Wakeling, B. (2003). “The use of translocations in deer and elk population management” in Proceedings of the 5th Western States and Provinces deer and elk Workshop 5, 133–138.
White, G. C., and Burnham, K. P. (1999). Program MARK: survival estimation from populations of marked animals. Bird Study 46, S120–S139. doi: 10.1080/00063659909477239
Wolf, C. M., Griffith, B., Reed, C., and Temple, S. A. (1996). Avian and mammalian translocations: update and reanalysis of 1987 survey data. Conserv. Biol. 10, 1142–1154. doi: 10.1046/j.1523-1739.1996.10041142.x
Keywords: ungulate, conservation, wildlife management, movement, deer
Citation: Smedley DC, McMillan BR, Hersey KR, Shannon JM and Larsen RT (2023) Outcomes associated with translocation of mule deer (Odocoileus hemionus): Influence of age, release timing, and year on survival. Front. Ecol. Evol. 11:1087058. doi: 10.3389/fevo.2023.1087058
Received: 01 November 2022; Accepted: 27 February 2023;
Published: 23 March 2023.
Edited by:
Vernon Bleich, University of Nevada, Reno, United StatesReviewed by:
Perry S. Barboza, Texas A & M University, United StatesCopyright © 2023 Smedley, McMillan, Hersey, Shannon and Larsen. This is an open-access article distributed under the terms of the Creative Commons Attribution License (CC BY). The use, distribution or reproduction in other forums is permitted, provided the original author(s) and the copyright owner(s) are credited and that the original publication in this journal is cited, in accordance with accepted academic practice. No use, distribution or reproduction is permitted which does not comply with these terms.
*Correspondence: Randy T. Larsen, cmFuZHlfbGFyc2VuQGJ5dS5lZHU=
Disclaimer: All claims expressed in this article are solely those of the authors and do not necessarily represent those of their affiliated organizations, or those of the publisher, the editors and the reviewers. Any product that may be evaluated in this article or claim that may be made by its manufacturer is not guaranteed or endorsed by the publisher.
Research integrity at Frontiers
Learn more about the work of our research integrity team to safeguard the quality of each article we publish.