- 1Department of Biology, Brigham Young University-Idaho, Rexburg, ID, United States
- 2Department of Natural Resources and Environmental Science, University of Nevada, Reno, NV, United States
- 3Institute of Arctic Biology, University of Alaska Fairbanks, Fairbanks, AK, United States
- 4Department of Fisheries, Wildlife, and Conservation Sciences, Oregon State University, Corvallis, OR, United States
Mammals are imperiled worldwide, primarily from habitat loss or modification, and exhibit downward trends in their populations and distributions. Likewise, large-bodied herbivores have undergone a collapse in numbers and are at the highest extinction risk of all mammals. Bighorn sheep (Ovis canadensis) are among those large-bodied herbivores that possess a slow-paced life history, suffer from debilitating diseases, and have experienced range contractions across their historical distribution since the late 1800s. Translocations and reintroductions of these mountain ungulates are key aspects of restoration and often are used to re-establish populations in historical habitat or to supplement declining herds. Millions of US dollars and much effort by state and federal natural resource agencies, as well as public and private organizations, have been expended to restore bighorn sheep. Despite those efforts, translocated populations of bighorn sheep have not always been successful. We assessed restoration of bighorn sheep to provide insights in the context of conservation of populations of bighorn sheep, because this management tool is a frequently used to re-establish populations. We focused briefly on past efforts to restore bighorn sheep populations and followed with updates on the value of habitat enhancements, genetic issues, the importance of ecotypic or phenotypic adaptations when restoring populations, predation, and disease transmission. We also raised issues and posed questions that have potential to affect future decisions regarding the restoration of bighorn sheep. This information will help conservationists improve the success of conserving these iconic large mammals.
1. Introduction
Mammals are imperiled worldwide (Bowyer et al., 2019). Rates of recent extinctions far exceed previous levels, constituting a conservation crisis for many species (Ceballos and Ehrlich, 2002; Ceballos et al., 2017). Indeed, numerous mammals exhibit downward trends in their populations and distributions (Schipper et al., 2008; Ceballos et al., 2017), which necessitate efforts to ensure their continued survival (Goble et al., 2012). Threat of extinction and body size are positively correlated (Purvis et al., 2000; Cardillo et al., 2005), and large mammals are especially vulnerable to threats to their existence. Loss, modification, and fragmentation of habitat pose the greatest threats to mammals worldwide (Wilcox and Murphy, 1985; Schipper et al., 2008), and such threats typically are driven by human population density or climate change, which present increasingly greater dangers to the continued existence of mammals (Vitousek et al., 1997; McKee et al., 2013; Bowyer et al., 2019).
Large herbivores have undergone a collapse in numbers worldwide (Figure 1 in Ripple et al., 2015), and are at the highest extinction risk of all mammals (Atwood et al., 2020). High risks of extinction among large herbivores also are related to intrinsic characteristics, including susceptibility to disease, limited geographic distributions, and a slow-paced life history typified by low adult mortality, iteroparity, small litter size, high maternal investment in large young, long generation times, and low intrinsic rates of increase (Caughley and Krebs, 1983; Davidson et al., 2017; Bowyer et al., 2019). Those life-history characteristics are linked with strong density-dependent processes in the dynamics of ungulate populations, and such populations are connected intrinsically with the habitats they occupy (Bowyer et al., 2014).
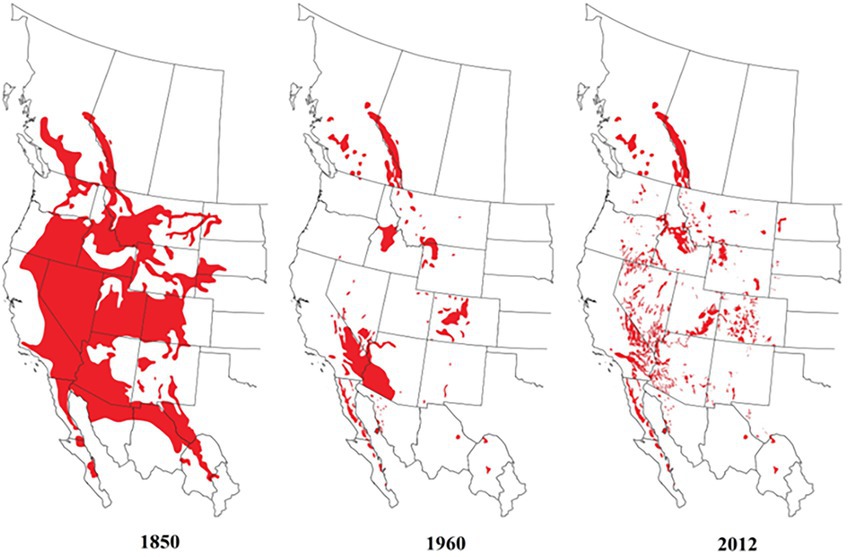
Figure 1. Historical (1850), at ostensibly the lowest extent of distribution (1960), and more recent distribution of bighorn sheep based on restoration of these ungulates in North America (Wild Sheep Foundation and WAFWA Wild Sheep Working Group, 2012).
Ungulates have experienced massive contractions in their distributions across North America (Laliberte and Ripple, 2004), and are undergoing elevated threats of extinction (Bowyer et al., 2019; Berger et al., 2020). Bighorn sheep (Ovis canadensis) are endemic to North America, are among the large-bodied ungulates that have experienced range contractions, and populations have declined considerably since the late 1800s (Buechner, 1960; Geist, 1971; Krausman, 2000; Figure 1). These ungulates are listed as least concern under The IUCN Red List of Threatened Species although some subspecies are endangered.1 Krausman (2000), and more recently Donovan et al. (2020), analyzed the status of many bighorn sheep populations in western North America. Restoration of populations has been an important tool for the conservation of bighorn sheep (Seddon, 2010; Taylor et al., 2017), but there is much to be learned from an examination of the overall circumstances leading to success or failure of efforts to conserve populations of these iconic large mammals.
Translocations and reintroductions (herein referred to as restoration), both key aspects of restoration, often are used to re-establish bighorn sheep in historical habitat or to supplement declining populations (Risenhoover et al., 1988; Roy and Irby, 1994; Singer et al., 2000a; Figure 2). Despite those efforts, translocations have not always been successful (Roy and Irby, 1994; Krausman, 2000; Singer et al., 2000b). Translocations and reintroductions, however, can be critically important to the restoration of bighorn sheep, particularly for endangered taxa or distinct population segments of those specialized mountain ungulates (Rubin et al., 1998; Ostermann et al., 2001; Cahn et al., 2011).
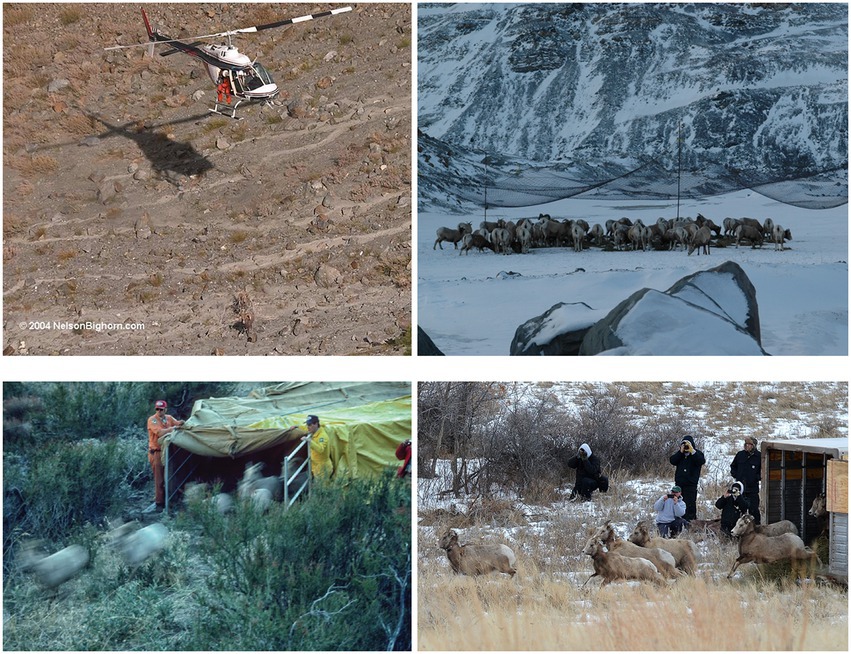
Figure 2. Examples of capturing bighorn sheep using a helicopter and a net gunner (top left) and a drop net (top right), as well as methods of releasing bighorn sheep after captures (bottom; Jessup et al., 2015).
Translocation has three forms: introduction, reintroduction, and restocking (IUCN, 1987). Hale and Koprowski (2018) refer to these as: (1) intended or unintended movement of an organism out of its native range; (2) intentional movement of an organism into native range from which it has been extirpated, and (3) movement of members of a species to augment the number of individuals in an original habitat. Whether in the context of introduction, reintroduction, or restocking, translocation has been, and remains, an essential component of wildlife management and conservation biology (Armstrong and Seddon, 2008; Seddon, 2010; Polak and Saltz, 2011), and is a positive step in restoring function to ecosystems of western North America, whether with bighorn sheep or other species (Kie et al., 2003; Bleich, 2020). Millions of US dollars and much effort by state and federal natural-resource agencies, as well as public and private organizations, have been expended to restore bighorn sheep to historical ranges (Krausman, 2000; Krausman et al., 2001; Hurley et al., 2015; Donovan et al., 2020).
Twenty-three years ago, Singer et al. (2000b) assessed factors associated with translocation success and provided recommendations for future translocations of bighorn sheep (Singer et al., 2000a). Herein, we conducted a review of translocation as a management tool for bighorn sheep with emphasis on new insights stemming from subsequent research, particularly regarding disease, evolutionary history, local adaptation, genetics, and climate change, as well as habitat considerations and the role of hunting.
2. Past success and habitat considerations
Bighorn sheep are endemic to North America. Suitable habitat extends from the northern Rocky Mountains in western Canada south through the western United States to northern Mexico (Buechner, 1960; Geist, 1971; Trefethen and Corbin, 1975; Valdez and Krausman, 1999; Figure 1). Bighorn sheep populations declined as human settlement expanded through much of their range; that downward trend in numbers began with the human settlement of vast, uninhabited areas (Grinnell, 1928; Buechner, 1960). Much attention has been accorded to unregulated market hunting and habitat loss, or modification, as causes of population declines (Grinnell, 1928; Buechner, 1960). Another, more onerous, factor that exacerbated declines was the introduction of livestock, primarily domestic sheep (O. aries), across much of the range of bighorn sheep (Buechner, 1960). As a result, naïve bighorn sheep populations were exposed to novel bacterial pathogens, resulting in epizootics of respiratory disease and depressed population performance (Grinnell, 1928; Skinner, 1928; Marsh, 1938), which may have begun during the late 1700s in some locations. Indeed, an ancient Kaliwa legend makes reference to a pestilence that destroyed many of the bighorn sheep in northern Baja California within a few years after Spanish padres arrived with their livestock (Tinker, 1978).
Cary (1911) provided additional evidence that disease was a factor in the early decline of bighorn sheep. Such declines were thought to have coincided with the advent of domestic livestock grazing on ranges occupied by bighorn sheep (Warren, 1910; Grinnell, 1928; Shillinger, 1937; Honess and Frost, 1942). Epizootics among native bighorn herds were reported in various locations following European settlement and establishment of domestic livestock grazing throughout the central and southern Rocky Mountains. Diseases, therefore, likely were the primary cause of decline among bighorn sheep populations across much of western North America (Beecham et al., 2007). Many native populations fell to <10% of historical numbers (Wild Sheep Working Group, 2012); as a result, bighorn sheep currently occur in far fewer locations and in fewer numbers than in the past (Figure 1). Despite an estimate of at least 1.5 million bighorn sheep occupying North America in 1850 (Seton, 1929), a number that was popularized by Buechner (1960), Valdez (1988) suggested that the number of wild sheep (O. canadensis and O. dalli combined) inhabiting North America probably never exceeded 500,000. Similarly, others have noted the absence of reliable historical population estimates and acknowledged that the number of wild sheep inhabiting North America in pristine times likely was in the hundreds of thousands (Wild Sheep Working Group, 2015). A combined total (Larkins, 2010; Sandoval et al., 2019) of about 81,000 bighorn sheep currently exist across the range of the species in Canada (~12,000), Mexico (~12,000), and the United States (~57,000).
Bighorn sheep occur largely in a metapopulation structure (Schwartz et al., 1986; Bleich et al., 1990a, 1996; Epps et al., 2006) and, as habitat specialists, are slow to colonize vacant habitat (Geist, 1971), although such events may occur more frequently than previously recognized (Bleich et al., 2021). The use of translocations to restore wild sheep to historical ranges began in 1922 with the capture of 20 Rocky Mountain bighorn sheep (O. c. canadensis) in Alberta, Canada, and release of 12 animals in Montana and eight animals in South Dakota. As of 2015 at least 1,460 additional projects have resulted in the translocation of ≥21,500 bighorn sheep in the United States and Canada (Wild Sheep Working Group, 2015; Figures 2–5). In the United States, states from which bighorn sheep had been extirpated—Nebraska, North Dakota, Oregon, South Dakota, Texas, and Washington—were completely dependent on the availability of translocation stock originating from outside of their jurisdictions.
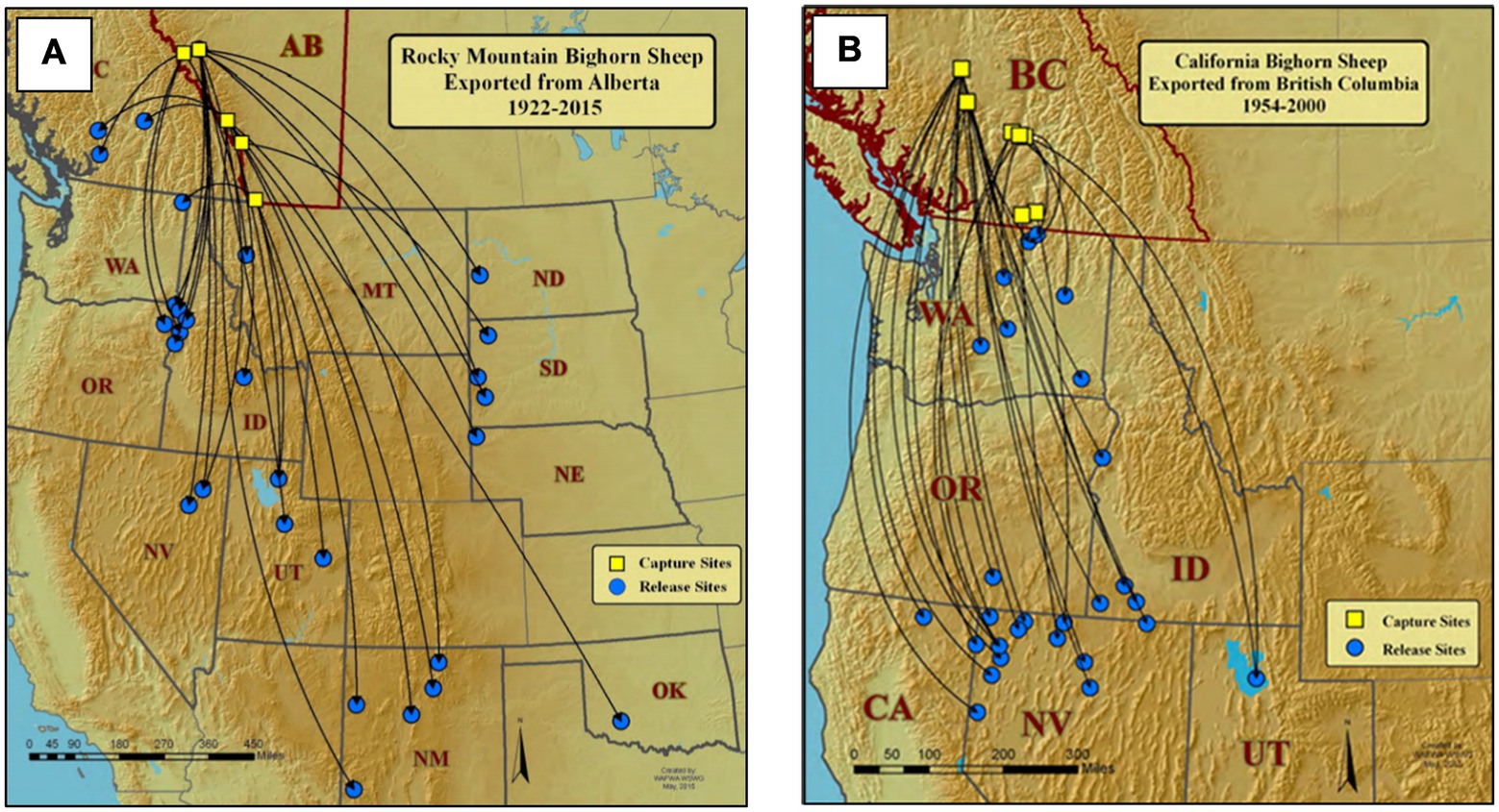
Figure 3. Examples of historical restoration of Rocky Mountain (A) and California bighorn sheep (B) from Canadian provinces to states of the western United States (Wild Sheep Working Group, 2015).
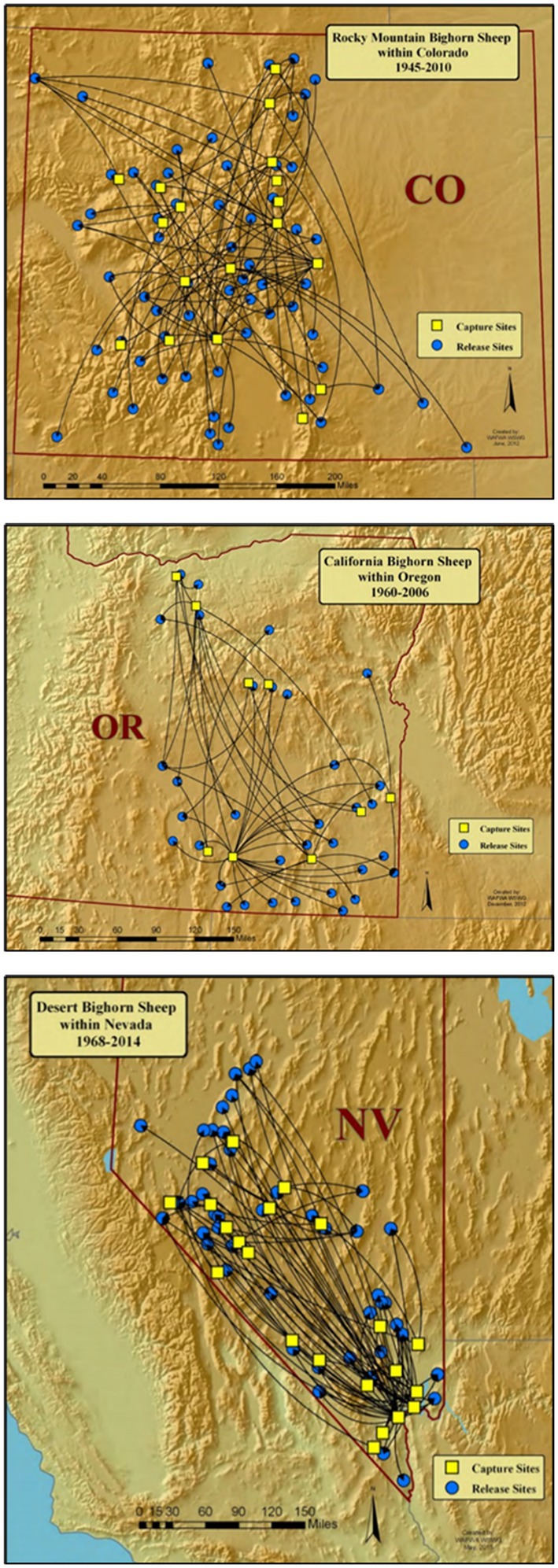
Figure 4. Examples of historical interstate restoration of Rocky Mountain bighorn sheep in Colorado, United States (top), California bighorn sheep in Oregon, United States (middle), and desert bighorn sheep in Nevada, United States (bottom; Wild Sheep Working Group, 2015).
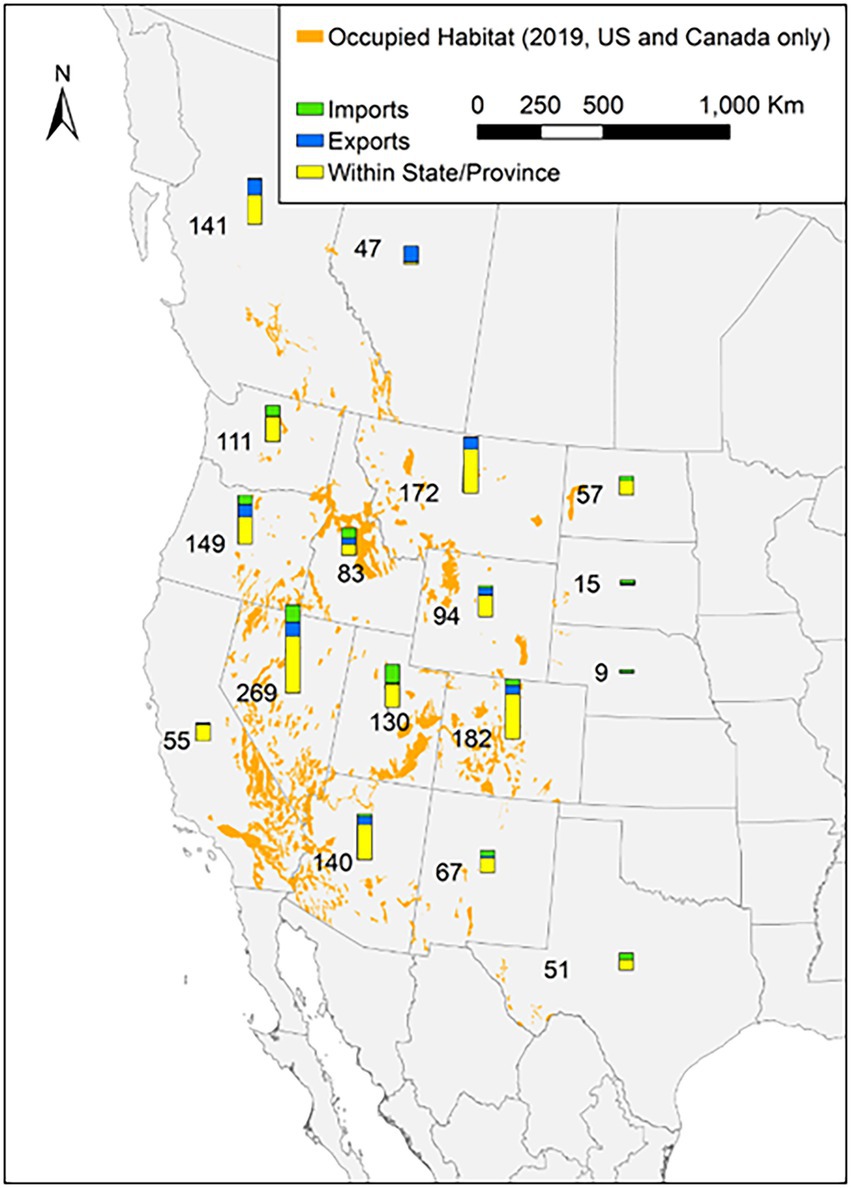
Figure 5. Currently occupied habitat of bighorn sheep populations in western North America with the number of restoration events (imported, exported, and moved within states and provinces).
Bighorn sheep formerly occurred in at least six Mexican states, but native populations remain only in Baja California, Baja California Sur, and Sonora (Valdez, 2011). Bighorn sheep occur in only 40% of their historical range in Baja California and Baja California Sur (DeForge et al., 1997). Elsewhere in Mexico, bighorn sheep have been re-established in historically occupied habitat in Sonora, Chihuahua, and Coahuila, and bighorn sheep in Mexico now number nearly 13,000 individuals (Sandoval et al., 2019; Brewer and McEnroe, 2020).
Bighorn sheep used to restore formerly occupied habitat in Mexico have originated largely from insular populations, captive populations, or from free-ranging populations on privately owned land (Sandoval et al., 2019). Mexico has a successful captive-breeding program, and current laws allow individual landowners to maintain facilities for the captive propagation of wildlife (Gonzalez-Rebeles Islas et al., 2019; Valdez, 2019). Economic considerations have been an important force driving those efforts, and they have occurred largely in the context of a demand for hunting opportunities for this iconic species (Lee, 2011; Gonzalez-Rebeles Islas et al., 2019). These efforts have resulted in establishment of ≥40 management areas in Sonora alone, with similar programs existing elsewhere in Mexico where the benefits to conservation are becoming widely recognized (Sandoval et al., 2019; Brewer and McEnroe, 2020).
Recovery of bighorn sheep throughout North America largely has been a function of successful programs, carried out by state or provincial wildlife agencies, to return those mountain ungulates to their historical ranges wherever possible. Most restoration efforts have involved the translocation of animals from existing, free-ranging populations to areas from which the species had been extirpated. Although uncommon, some successful restorations or augmentations have depended on captive populations to produce stock for translocation in Nebraska (Fairbanks et al., 1987), Texas (Hailey, 1971; Kilpatrick, 1980), New Mexico (Snyder, 1980), California (Ostermann et al., 2001), and perhaps other jurisdictions. There are, however, no commercial enterprises that breed bighorn sheep to be released in the wild. Regardless of the origin of translocation stock, recovery has restored an important biological component of many desert and alpine ecosystems. For example, in the most arid portions of the Mojave Desert in California, United States, bighorn sheep are the only large native herbivore present and serve as prey for native predators, including mountain lions (Puma concolor), bobcats (Lynx rufus), coyotes (Canis latrans), and golden eagles (Aquila chrysaetos; Ober, 1931; Wehausen, 1996; Bleich et al., 1997).
Among habitat characteristics shared throughout the distribution of bighorn sheep is their association with steep, rugged terrain, which plays a vital role in predator-evasion strategies and in survival of lambs (Berger, 1991; Bleich et al., 1997; Bleich, 1999; Schroeder et al., 2010). Additionally, openness of terrain and vegetation enhances the ability of bighorn sheep to detect predators (Berger, 1991; Bleich et al., 1997) and spend less time vigilant while foraging (Berger, 1978; Risenhoover and Bailey, 1985), with probable energetic benefits to individuals. Additionally, surface water (as well as condensation and as preformed water in forage) is a critically important resource for bighorn sheep occupying desert ecosystems in which those large mammals are unable to meet their physiological needs via metabolic water during the hot summer (Turner, 1973; Leslie and Douglas, 1979; Epps et al., 2004). The availability of water and vegetation, however, in historical range may not be suitable for reintroduction because the landscape and land use may have changed. There are four attributes of critical habitat for bighorn sheep: availability of surface water in desert ranges, vegetation composition or structure, isolation from diseases carried by domestic sheep, and rugged terrain. All of these attributes can be considered in selecting a translocation site but water, vegetation, and diseases are much more dynamic than terrain if the animals remain in the selected area. Despite this limitation, the potential for success of translocations can be enhanced by selecting areas with appropriately rugged terrain for translocations (Smith et al., 1991; Zeigenfuss et al., 2000), or by manipulating and emphasizing the importance of several other habitat features that are important to bighorn sheep (Smith et al., 1999; Bleich, 2009; Whiting et al., 2011a).
Seasonal differences in habitat selection between males and females are a consequence of differential distributions between sexes of bighorn across the landscape, resulting principally from modifications in digestive morphology and physiology (Barboza and Bowyer, 2000, 2001) and varying susceptibility to predation (Bleich et al., 1997) by the sexes—those differences have important implications for the conservation of bighorn sheep (Bowyer, 2022). Males and females exhibit disparate life-history patterns (Bleich et al., 1997; Schroeder et al., 2010; Whiting et al., 2010a,b), and the restoration of bighorn sheep to previously occupied ranges is complicated by these behavioral differences in their habitat requirements (Bleich et al., 1997; Villepique et al., 2015). Unfortunately, the differential selection of habitat by males and females during much of the year has not often been considered in translocation efforts but is necessary for successful restoration of these large mammals (Bowyer, 2022).
2.1. Benefits of water development
In the Chihuahuan, Sonoran, and Mojave deserts, and to a lesser extent in the Great Basin Desert, water development has been a prominent and widespread habitat-enhancement technique (Rosenstock et al., 1999; Bleich, 2009; Larsen et al., 2012) albeit, in some instances, a contentious activity, as bighorn sheep may not always rely on these features (Cain et al., 2008). That aside, water-development projects have played a critical role in managing habitat for extant populations and have been implemented widely to ensure the availability of that important resource prior to restoration efforts in desert ecosystems (Bleich, 2009; Whiting et al., 2011a).
Numerous techniques for providing surface water have been developed, ranging from enhancing storage of water flowing from natural springs, the use of horizontal wells to create surface water where it previously was unavailable, modification of natural water sources that previously were unavailable because bighorn sheep could not negotiate the near-vertical walls of tenajas (natural features that retain run-off water for long periods of time), the use of sand dams for underground storage, and construction of artificial catchments that trap and store rainfall in areas where no surface water previously existed (Bleich et al., 2020). Water developments can accomplish several objectives simultaneously, among which is provision of a resource without which bighorn sheep cannot persist. This is particularly important where water has become unavailable in desert environments because of anthropogenic activities that have resulted in shifts in the water table, or loss of free water resulting from a changing climate (Epps et al., 2004; Whiting et al., 2011a; Larsen et al., 2012).
Given the metapopulation structure of bighorn sheep in desert regions (Schwartz et al., 1986; Bleich et al., 1990a, 1996; Epps et al., 2007), enhancement of water availability also facilitates metapopulation processes, encourages colonization events, increases population size (albeit not necessarily population density), enhances gene flow among subpopulations, and increases the probability of individual survival during periods of drought (Bleich, 2009). As such, water provision has played a major role in translocation of desert bighorn sheep (O. c. nelsoni) to areas from which they had been extirpated and helps to ensure their persistence in currently occupied areas.
Early habitat-assessment models (Smith et al., 1991; Zeigenfuss et al., 2000) identified the need to consider availability of reliable water sources to existing populations, and prior to re-establishing bighorn sheep, particularly in deserts. Recent work has highlighted the need to consider water sources that will benefit males and females separately, because sexual segregation is a life-history characteristic of these ungulates (Bleich et al., 1997; Bowyer, 2004; Rubin and Bleich, 2005; Bleich, 2009; Schroeder et al., 2010). For example, in one re-established population, although female and male bighorn sheep used small, adjacent areas during segregation, the sexes still visited different sources of water (Whiting et al., 2010a). This information highlights the value of water sources in areas used by sexes regardless of the size of the area occupied by these animals. Whiting et al. (2010a) also reported that all water sources were within 3.2 km of 50% core areas used by either sex of re-established bighorn sheep. Use of that resource differed, however, according to the proximity of water to habitats used by males or females. Indeed, some sources of water were used rarely by either males or females during particular seasons (Whiting et al., 2010a; Larsen et al., 2012).
2.2. Vegetation management
In addition to maintaining or providing reliable sources of surface water, manipulation of vegetation often is desirable, and in some situations is necessary to enhance success of translocation efforts or ensure the persistence of populations. Numerous methods that are mechanical or biological in nature, as well as the application of prescribed fire, offer viable approaches (Green, 1977a,b; Bleich and Holl, 1982; Bleich et al., 2020). Prescribed burning is a tool for reducing fuels and restoring a process to landscapes that historically experienced fire, but that have been altered by decades of fire exclusion or fire suppression. Results from prescribed ignitions are dependent, however, on numerous variables, each of which can affect the final outcome (Stephens et al., 2009). Nevertheless, vegetation manipulation in the form of prescribed fire is widely recognized as an important method of enhancing forage quality or availability for bighorn sheep (Peek et al., 1979; Hobbs and Spowart, 1984; Seip and Bunnell, 1985;Smith et al., 1999; Clapp and Beck, 2016) and other large herbivores, or enhancing habitat quality indirectly by decreasing barriers to visibility (Risenhoover and Bailey, 1980; Smith et al., 1999). Vegetation management to enhance habitat quality is likely to be successful in those areas of the southwestern United States occupied by bighorn sheep and dominated by coastal chaparral, or by Madrean evergreen woodland, vegetation types in which shrub density increases, and forage quality declines with time elapsed since previous fire or other types of perturbation (Green, 1977a; Cain et al., 2005; Bleich et al., 2008; Holl et al., 2012). Vegetation types differ, but similar positive results have been obtained in areas occupied by Rocky Mountain bighorn sheep (Peek et al., 1979; Smith et al., 1999; Clapp and Beck, 2016).
Although the U.S. Forest Service has changed its policy from fire suppression to fire management (DeBruin, 1974), use of prescribed fire to enhance or maintain conditions suitable for restoring bighorn sheep is infrequent and, even when implemented, is limited in scope (Smith et al., 1999; Clapp and Beck, 2016). Further, the phenomenon of sexual segregation dictates that habitat requirements of male and female bighorn sheep be considered when implementing prescribed fire for habitat enhancement, and physical characteristics of the landscape have important implications for success (Bowyer, 2022). Regardless of the amount of ‘high-quality’ habitat created as a result of prescribed ignition, the relative increase in habitat possessing suitable physical or geological characteristics (e.g., steepness or ruggedness) will dictate the degree of benefit independently of any increase in forage availability, quality, or visibility (Holl, 1982). Further, many areas identified for reintroductions, even though occupied historically by bighorn sheep, likely have changed considerably since bighorn sheep were extirpated (Risenhoover et al., 1988), and decreases in suitability of habitat may have been subtle and likely not evaluated prior to translocations (Wakelyn, 1984; Wakelyn, 1987). Moreover, management of vegetation specifically to enhance bighorn sheep habitat has been severely constrained within legislated wilderness areas in the United States since the Wilderness Act was passed in 1964, and existing policy will continue to complicate such efforts (Bleich, 2005, 2016; Bleich et al., 2019).
2.3. Managing disease susceptibility
In addition to the physical or vegetational characteristics of sites to be considered, the potential for exposure to pathogens remains a key consideration. Disease, in addition to habitat quality, has primary consequences for conservation of wild sheep in North America (Bleich, 2009). Thus, the third aspect of bighorn sheep habitat that can be manipulated or managed prior to a restoration is the proximity of translocation sites to domestic sheep, whether on private or public lands. Bighorn sheep are especially vulnerable to Mycoplasma ovipneumoniae as an agent predisposing them to respiratory disease; Pasteurella multocida, Mannheimia haemolytica, and Bibersteinia trehalosi also occur widely among domestic sheep (Besser et al., 2013; Cassirer et al., 2013, 2018). As a result, even areas that are geologically or topographically suitable, provide vegetation characteristics that are adequate to meet predator-evasion strategies and nutritional requirements, and meet the physiological needs of bighorn sheep through the availability of reliable surface water, may be excluded from consideration because of the potential for pathogen transmission from domestic sheep.
Prudent and responsible stewardship dictates that the potential for pathogen transfer to bighorn sheep be a primary factor when considering restoration locations. Current guidelines caution strongly against implementing such actions where the probability of translocated animals coming into contact with domestic sheep does not approach zero, or where there is substantial uncertainty of the disease status of either source or recipient populations (Brewer et al., 2014; Jex et al., 2016). Efforts to restore native bighorn sheep to historical ranges that otherwise were suitable have been postponed, and even canceled, because of risks associated with the presence of, or proximity to, grazing of domestic sheep (Shannon et al., 2014). Until such risks are eliminated, proposed restoration efforts should be halted. While survival of desert bighorn sheep infected by M. ovipneumoniae has been observed to be higher when forage conditions are good (Dekelaita et al., 2020), the risks of allowing novel pathogen strains to enter a restored system likely outweigh the quality of the habitat.
2.4. Climate change and future habitat conditions
Climate change provides challenges for managing wildlife populations, and the need for climate-informed management of species, particularly in restoration of alpine specialists such as bighorn sheep, is now well-recognized (Gude et al., 2022). Studies have attempted to estimate effects of past and future climate variation on bighorn sheep, including both native and restored populations (Epps et al., 2004; Colchero et al., 2009). Nonetheless, a study investigating temporal mismatch between vegetation green-up and parturition date in Rocky Mountain bighorn sheep, an expected consequence of climate change, concluded that mismatch did not have major consequences for fitness (Renaud et al., 2022).
Changes in precipitation regimes across western North America may be more dramatic and less predictable than current conditions and could have major implications for management of both natural and artificial water sources (Dolan, 2006; Terry et al., 2022), snowpack and avalanche risk (Conner et al., 2018), forage quantity and quality (Epps et al., 2004), or metapopulation dynamics in desert systems (Epps et al., 2004, 2006). For instance, in Colorado, increased precipitation increased deposition of inorganic nitrogen, acidifying surface waters and potentially affecting the bottom of the food chain with consequences for bighorn sheep foraging (Williams et al., 2002). Increased variation in rainfall in Baja California Sur, Mexico, is expected to disrupt conservation of bighorn sheep and the associated hunting-based economy (Zamora-Maldonado et al., 2021); indeed, translocation of individuals might be necessary to sustain huntable populations under some climate conditions, although the long-term success of such an approach is questionable if nutrition limitations are the cause of the decline. Alternatively, Creech et al. (2020) considered vulnerability of desert bighorn sheep across a portion of their range with respect to climate change as a function of adaptive capacity (genetic diversity and connectivity) and predicted rates of environmental change. Some reintroduced populations ranked poorly in that assessment because of low genetic diversity caused by founder effects from translocations (Creech et al., 2020).
Across the range of bighorn sheep, managers of restored systems or those contemplating restoration would be well advised to consider habitat resources in a changing climate, and whether historically occupied but now unoccupied habitats would still support populations if restored (Wehausen and Epps, 2021). Likewise, climate change is a primary reason to maintain the ability of restored bighorn sheep to adapt by preserving genetic diversity and connectivity where not precluded by other considerations.
3. Genetic considerations
Efforts to restore bighorn sheep in North America during the 20th and 21st centuries exemplify the challenges posed by an incomplete understanding of their evolutionary histories. Two primary problems exist: first, when both local and regional extirpation of species have occurred, which potential source stocks are most evolutionarily and ecologically appropriate for restoration? Second, how can genetic diversity in translocated populations be maximized and maintained without precipitating outbreeding depression? Both questions warrant serious consideration and require a spatially detailed and correct assessment of the evolutionary history of extirpated and remaining populations. The second question also requires careful assessment of population history or genetic variation in remaining populations (Bleich et al., 2021).
Wild sheep colonized North America from Asia approximately 2 million years ago (Bunch et al., 2006; Rezaei et al., 2010), diverging subsequently into two species, bighorn sheep and Dall’s sheep. Subspecies designations remain unresolved, but specific divergence likely resulted from isolation of bighorn sheep in different refugia during the glacial cycles of the Pleistocene (Buchalski et al., 2016). Cowan (1940) recognized up to seven subspecies on the basis of morphology, but subsequent morphometric and genetic analyses indicated that all four desert subspecies (O. c. nelsoni, O. c. mexicana, O. c. cremnobates, and likely O. c. weemsi; although O. c. weemsi was not formally included in the analyses) should be synonymized and referred to taxonomically as O. c. nelsoni (Wehausen and Ramey, 1993). Specifically, PCA and discriminant function analyses of 17 skull and horn measurements from 198 rams and 145 ewes did not support clear distinction among those taxa, nor did RFLP analyses of mitochondrial DNA haplotypes (Ramey, 1993a,b). More recent genetic analyses, however, show some evidence for distinction of populations previously described as O. c. cremnobates (in part, although the lines of distinction differ somewhat from the estimation of Cowan, 1940) and O. c. mexicana from O. c. nelsoni (Buchalski et al., 2016). Sierra Nevada bighorn sheep represent a deeply divergent evolutionary lineage (Buchalski et al., 2016) and are now recognized as a distinct subspecies (O. c. sierrae; Wehausen and Ramey, 2000; Wehausen et al., 2005). Based on morphological analysis of horn and skull characters, Wehausen and Ramey (2000) proposed synonymizing Rocky Mountain and California (O. c. californiana), as well as the extinct O. c. auduboni, as O. c. canadensis. Wildlife agencies in North America, however, mostly continue to manage bighorn sheep from those lineages separately, and microsatellite analysis of populations in British Columbia, Washington, Oregon, and Idaho showed clear distinction by lineage (Barbosa et al., 2021).
Bighorn sheep in the northwestern U.S. and southwestern Canada pose a particular management challenge. Bighorn sheep were extirpated from Oregon, Washington, southeastern Idaho, and northwestern Nevada by the first one-half of the 20th century (Buechner, 1960). At that time, Cowan (1940) considered the range of California bighorn to extend from British Columbia southward to the Sierra Nevada of California (Figure 3). Subsequent restoration efforts relied largely on bighorn sheep translocated from British Columbia. Since then, Wehausen and Ramey (2000) assigned extinct and extant populations in Washington and British Columbia to the Rocky Mountain subspecies, but argued that extirpated native populations in Oregon, southeastern Idaho, northeastern California, and northwestern Nevada represented a Great Basin form of desert bighorn sheep. Managers now question what the most appropriate source for future translocations should be—whether translocation stock representing California and Rocky Mountain bighorn sheep can or should be mixed, or even whether poorly performing populations in the Great Basin region should be augmented with desert bighorn sheep (Epps et al., 2019). Across much of the range of bighorn sheep, resolving questions of evolutionary history thus remains relevant to future management.
Determining appropriate strategies to manage genetic diversity of translocated bighorn sheep populations continues to generate keen interest and debate (Epps et al., 2019; Flesch et al., 2020), but will require distinctive approaches in different systems. In largely intact native systems of bighorn sheep, such as the metapopulations of desert bighorn occurring in the Mojave and Sonoran deserts of California (Schwartz et al., 1986; Bleich et al., 1990a, 2021), genetic diversity is strongly shaped by connectivity among populations (Epps et al., 2005; Flesch et al., 2020), habitat quality (Epps et al., 2006; Creech et al., 2016), and extinction and colonization dynamics (Epps et al., 2010, 2018). Connectivity, in turn, is influenced by both natural and anthropogenic fragmentation (Bleich et al., 1996; Epps et al., 2007).
Translocations of desert bighorn sheep in that region largely have addressed localized extirpations. As a result, and in most instances, potential for those translocated populations to be integrated into the existing metapopulation by dispersal is high. Indeed, for most translocated populations in that region, dispersal has been documented to or from nearby native populations (e.g., Epps et al., 2010, 2018; Bleich et al., 2021) except in instances of extreme isolation (e.g., the Sespe population near San Rafael Peak in Ventura County, California, United States; Bleich et al., 2019). Similarly, integration of translocated populations into current, native population structure has been observed elsewhere, as on the north rim of the Grand Canyon in Arizona (Gille et al., 2019). Nonetheless, relationships between genetic diversity and geographic and genetic measures of isolation largely were decoupled for translocated populations in that region, indicating that genetic equilibrium has not yet been reached (Creech et al., 2020). Over time, genetic diversity of translocated populations can be expected to benefit from connections to nearby native populations, thereby potentially increasing genetic diversity of nearby native populations, as has occurred in the Coxcomb Mountains in southern California, United States (Epps et al., 2010).
As the proportion of translocated populations increases within a particular system, effects of genetic bottlenecks and founder effects can become more apparent. Founder effects are well-documented among translocated and subsequently isolated populations of bighorn sheep (Gutiérrez-Espeleta et al., 2000; Hedrick et al., 2001; Hogg et al., 2006; Johnson et al., 2011; Barbosa et al., 2021). In the Sierra Nevada of California, where bighorn sheep likely declined to fewer than 100 individuals, restoration efforts have relied on translocation as a primary tool, and much consideration has been given to conserving what genetic diversity survived bottlenecks by this subspecies (Few et al., 2013; Bleich et al., 2021). In other systems with significant numbers of translocations but greater connectivity and more source populations, bottlenecks are less apparent, but translocation histories still leave readily detectable genetic footprints of source populations, sometimes admixed with other source populations or native individuals (Jahner et al., 2019; Flesch et al., 2022).
In some parts of the range of bighorn sheep, restoration at the regional scale was necessary, leading to more serious challenges from a genetic standpoint. Restoration of bighorn sheep to historically occupied areas in Washington, Oregon, southern Idaho, northwestern Nevada, and northeastern California, United States, was conducted using animals from British Columbia, Canada (subspecies californiana), or Rocky Mountain bighorn (subspecies canadensis), which likely were of a different evolutionary lineage than animals that were present before extirpation (Wehausen and Ramey, 2000). Using translocated populations as sources for later translocations led to a complicated history of bottlenecks and severe, repeated founder effects, resulting in very low genetic diversity in many contemporary populations in those regions (Whittaker et al., 2004; Barbosa et al., 2021). Recognition of the potential negative consequences of that history led to efforts in Oregon to restore genetic diversity in several populations through additional translocations from other sources (Olson et al., 2012). Maintaining or improving genetic diversity in those regions remains a concern, and further questions, including how well adapted those animals may be to Great Basin environments, and whether bighorn sheep from California or Rocky Mountain sources represent evolutionarily divergent lineages, also have been raised or are now being evaluated (Olson et al., 2013; Epps et al., 2019; Bleich et al., 2021).
Moving forward, low genetic diversity and inbreeding depression remain a common concern for management of small, isolated populations of bighorn sheep, particularly because of growing evidence for links between genetic diversity and fitness. Genetic diversity has been linked to nematode resistance in Soay sheep (Hayward et al., 2014). In the Mojave Desert of California, desert bighorn sheep populations with higher genetic diversity had lower prevalence of exposure to and infection by M. ovipneumoniae, and more genetically diverse individuals had stronger adaptive immune responses (Dugovich et al., 2023). Genetic rescue of an isolated population of bighorn sheep exhibiting inbreeding depression has been reported (Hogg et al., 2006; Miller et al., 2012), but outbreeding depression (Dobzhansky, 1948) is also a concern when selecting source stock for translocations or augmentations. Outbreeding depression can result from inappropriate mixing of individuals from different environments because of disruption of co-adapted gene complexes (Waits and Epps, 2015). Further, individuals from different environments may fare poorly when compared with locally adapted individuals (e.g., Whiting et al., 2012; Wiedmann and Sargeant, 2014; Bleich et al., 2018). Finally, any movement of individuals to actively manage genetic diversity increases the risk of introducing or spreading disease (see Disease section). In areas such as the northwestern United Sates, where extirpations were especially widespread, better understanding of evolutionary history, inbreeding, and adaptive genetic variation in bighorn sheep are urgently needed.
4. Ecotypic and phenotypic considerations
Bighorn sheep are habitat specialists (i.e., dependence on steep, rugged terrain of variable elevations), and the successful restoration of these iconic animals to historical ranges is conditioned on a number of critically important factors that are shared among the various clades (Buchalski et al., 2016), subspecies (Wehausen and Ramey, 2000), or ecotypes (i.e., those possessing physiological, reproductive, or behavioral adaptations to localized environmental conditions or habitats; Bleich et al., 2018). In the past, translocation of bighorn sheep to historically occupied ranges was based largely on selection of a source population thought to represent the appropriate taxon according to subspecies boundaries recognized at the time (Cowan, 1940), the availability of translocation stock (Leslie, 1980), or in an effort to maintain subspecific purity (Ramey, 1993a,b). Although emphasized strongly by Leslie (1980), the importance of similarities between habitat occupied by a source population and the location to which bighorn sheep are translocated has become widely recognized within recent decades (Whiting et al., 2012; Wiedmann and Sargeant, 2014; Bleich et al., 2018, 2021), just as have the genetic consequences of translocations (Whittaker et al., 2004; Jahner et al., 2019; Barbosa et al., 2021). In some instances, hybridization of subspecies or putative subspecies has occurred because of underestimation of the ability of bighorn sheep to make long distance dispersal movements (e.g., Jahner et al., 2019), and managers have stated the need to prevent hybridization between strongly divergent subspecies—e.g., Rocky Mountain and desert bighorn, (Latch et al., 2006), because of concerns about outbreeding depression.
Indeed, because of the variation in success of translocations (Singer et al., 2000b), the importance of local adaptations or ecological similarities between habitat occupied by source populations and that at proposed translocation sites now receives greater emphasis (Whiting et al., 2012; Brewer et al., 2014; Wiedmann and Sargeant, 2014; Bleich et al., 2018). Timing of birthing seasons, diet, composition of predator communities, climate, and vegetative structure all have implications for the success or failure of a restoration effort. Although some traits such as birthing season and migration appear to be plastic and do adjust over time (Whiting et al., 2011b, 2012; Jesmer et al., 2018), others may be less malleable because of a genetic basis, such as body size, or size and rates of horn growth (Ramey, 1993a,b).
The potential for adjustment of life-history characteristics and behaviors, thus, is an essential consideration when translocating bighorn sheep that may be adapted to environments different than those that occur, or historically occurred, at a particular release site. For example, female bighorn sheep captured in Canada and released in Utah, United States, did not adjust maternal care to compensate for late-born young within the first 3 years following release, which possibly influenced survivorship of young (Whiting et al., 2010b). Young males and a low male-to-female ratio of bighorn sheep captured in Canada and Montana, United States, and released in Utah did not affect the number of young born per female or timing and synchrony of births (Whiting et al., 2008). Moreover, some of those populations adjusted timing and synchrony of parturition to environmental conditions of their release site within 5 years after release (Whiting et al., 2011b). Slow adjustment of those life-history characteristics by females, however, possibly contributed to lower survival of young to their first winter, which could slow successful establishment of bighorn sheep populations (Whiting et al., 2011b).
In another example, bighorn sheep from British Columbia performed poorly in terms of population dynamics and survival rates when compared with those from Montana, an area much more similar to the Badlands of western North Dakota, United States, where animals had been released (Wiedmann and Sargeant, 2014; Bleich et al., 2018). Thus, consideration should be given to the adjustment of timing and synchrony of births when restoring populations of bighorn sheep, and biologists should select source populations occupying areas that are ecologically similar to proposed release sites (Whiting et al., 2012). Further, ecotypic differences among source stocks may have long-term implications for recruitment and demographic performance of reintroduced populations, and use of appropriate source stock may greatly improve prospects for successful reintroductions of bighorn sheep (Wiedmann and Sargeant, 2014).
5. Predation and its implications for restoration
Mountain lions, coyotes, bobcats, and golden eagles are important predators of adult bighorn sheep (Bleich, 1996, 1999; Bleich et al., 1997, 2004). Relative abundance of coyotes was up to 12× greater on ranges occupied by male bighorn sheep when compared to areas occupied primarily by females and young during sexual segregation, but relative abundance of bobcats was similar between male and female ranges (Bleich et al., 1997). Mountain lions generally occur at exceedingly low densities (0.4–7.0/100 km2; Pierce and Bleich, 2003, 2014). Nevertheless, predation by mountain lions is a common source of mortality for bighorn sheep (Ross et al., 1997; Hayes et al., 2000; Rominger, 2018) and can impede restoration of populations of this ungulate (Wehausen, 1996; Rominger et al., 2004; McKinney et al., 2006). An additional aspect of predation involves apparent competition (Holt, 1977; Holt and Lawton, 1994; Sinclair et al., 1998). Predation can affect a rare competitor negatively if the population dynamics of a shared predator are associated primarily with a more numerous species of sympatric prey (Keddy, 2001). Consequently, the two species of prey appear to be in competition with each other, and the rare competitor may be threatened by increased predation (DeCesare et al., 2010; Wielgus, 2017). The endangered Sierra Nevada bighorn sheep is preyed upon by mountain lions, but the dynamics of the mountain lion population are dependent upon more numerous mule deer (Odocoileus hemionus), and results in the bighorn sheep population being threatened by higher rates of predation from mountain lions than if mule deer occurred at lower densities or were not present (Johnson et al., 2013).
In western North America, mountain lions, mule deer, and bighorn sheep often share ranges, seasonally or year-round (Wehausen, 1996; Holl et al., 2004). In some areas, mule deer have been implicated in negatively affecting bighorn sheep by potentially impeding population growth or contributed to declining populations of bighorn sheep (Wehausen, 1996; Holl et al., 2004; Rominger et al., 2004; Holl and Bleich, 2009, 2010). Such outcomes ostensibly have occurred because mountain lions have switched prey from mule deer to bighorn sheep following declines in numbers of mule deer (Holl et al., 2004; Holl and Bleich, 2009, 2010; Rominger, 2018), although prey switching may not always occur (Villepique et al., 2011). Risk of predation also may have caused bighorn sheep to abandon useable habitat (Wehausen, 1996; Rominger, 2018), although other environmental explanations are plausible (Villepique et al., 2015). Thus, the abundance and distribution of predators are essential considerations when re-establishing populations of bighorn sheep, and likely will receive increasing emphasis in the future.
6. Disease and restoration
Disease long has been recognized as a primary hindrance to the persistence of bighorn sheep populations (Gross et al., 2000; Zeigenfuss et al., 2000; Singer et al., 2000b). Nonetheless, new discoveries, particularly with respect to ovine pneumonia as subsequently described, have greatly clarified the scope and significance of the problem. Indeed, the issue of pathogen transmission has become one of the central challenges to bighorn sheep restoration. Since attempts to restore bighorn sheep to historic range began, disease likely has played a primary role in failures noted in some reviews of translocations (Singer et al., 2000c, 2001). For instance, in 1971, bighorn sheep were translocated to an enclosure within the Lava Beds National Monument in northeastern California (Blaisdell, 1972). In 1980, after contact with domestic sheep, all individuals within the enclosure died from respiratory pneumonia (Foreyt and Jessup, 1982). Subsequently, as agencies struggled to manage new outbreaks, elimination of entire populations—whether native or restored—at times has been deemed necessary to contain outbreaks, albeit with unclear success (Cassirer et al., 2018).
Evidence obtained over nearly 40 years underscores the risk of disease transmission to wild sheep from domestic sheep or goats (Capra hircus; Wild Sheep Working Group, 2012). Managers and researchers consistently have recommended temporal or spatial separation of domestic sheep or goats from wild sheep to reduce the potential for disease in the latter—evidence supporting this recommendation is overwhelming. The risks associated with contact between bighorn sheep and domestic sheep or domestic goats have been clearly identified by recent risk assessments and reviews (Beecham et al., 2007; Schommer and Woolever, 2008; Baumer et al., 2009;Wehausen et al., 2011; Cassirer et al., 2018), conservation management strategies or plans (George, 2009), modeling exercises or locally specific risk assessments (Clifford et al., 2009; Cahn et al., 2011; Carpenter et al., 2014; Anderson et al., 2022), and many wildlife biologists or veterinarians (Dubay et al., 2004; Garde et al., 2005; Jansen et al., 2006; Foreyt et al., 2009).
Although bighorn sheep suffer from a variety of diseases—including scabies (Boyce and Weisenberger, 2005), sinusitis (Paul and Bunch, 1978), contagious echthyma (Jansen et al., 2007), lungworm (Luikart et al., 2008), bluetongue (Singer et al., 2000a,b,c), and others—ovine respiratory pneumonia remains the primary concern for conservation of this unique large mammal (Wehausen et al., 2011; Cassirer et al., 2018). Despite clear evidence that contact between domestic sheep and bighorn sheep often resulted in fatal pneumonia among bighorn sheep, the primary pathogens had, until recently, remained unclear. Bacteria, including Pasteurella multocida, Mannheimia haemolytica, and Bibersteinia trehalosi were implicated, but presence of those species was not always consistent with a disease outbreak (Cassirer et al., 2018). The role of Mycoplasma ovipneumoniae as an agent predisposing bighorn sheep to other pathogens that result in respiratory disease has become increasingly apparent (Besser et al., 2008, 2012, 2013; Dassanayake et al., 2010). This pathogen is spread from domestic sheep or domestic goats to bighorn sheep by incidental contact, but then can spread readily among bighorn sheep populations following conspecific contact with infected individuals.
At first contact with a novel strain of M. ovipneumoniae, all-age die-offs of bighorn sheep frequently are observed (Cassirer et al., 2018). Although animals that survive an initial outbreak appear to maintain immunity for some years, juveniles are naïve, and mortality within the first 4–6 months after birth frequently is high for several years following exposure (Cassirer and Sinclair, 2007; Smith et al., 2014), leading to consistent suppression of recruitment (Butler et al., 2018). Moreover, adult immunity is strain-specific (Cassirer et al., 2017). Thus, continued contact with domestic sheep, or with other bighorn sheep infected with differing strains of M. ovipneumoniae, can result in a new cycle of all-age mortality, followed by suppression of juvenile recruitment that slows, or even prevents, population growth (Manlove et al., 2016; Cassirer et al., 2017), as well as reduction in size and growth rate of sexually selected traits (e.g., horn length; Martin et al., 2022). Virulence appears to differ among strains of M. ovipneumoniae (Johnson et al., 2022), and other factors (host-related or environmental) likewise also cause epidemics to have widely varying effects on adult and juvenile survival (Manlove et al., 2022).
Recent research has demonstrated that removal of chronically infected adult females from wild populations can increase juvenile recruitment (Garwood et al., 2020), but that strategy may be logistically challenging to implement in some systems; when prevalence is low, natural mortality may also remove carriers and allow recovery at least temporarily (Besser et al., 2021). Modeling of M. ovipneumoniae disease dynamics indicates that depopulation and restoration can assist recovery following an epidemic (Almberg et al., 2022). Indeed, following rapid depopulation of bighorn sheep in the Montana Mountains of northwestern Nevada, United States, in 2015 after detection of a new outbreak of M. ovipneumoniae with high mortality, that strain was not detected in subsequent monitoring of the nearby Trout Creek metapopulation of bighorn in southeastern Oregon (Spaan, 2022). Depopulation is likely considered more readily in systems of restored rather than native populations.
Earlier cautions that translocations pose a risk of establishing new lines of transmission of pathogens (Bleich et al., 1990a) have been verified. Newly translocated individuals often range widely and explore new habitats (Robinson et al., 2019), during which contact with livestock becomes more likely, and translocated animals may return to their native area even if moved far from their initial point of capture (Torres et al., 2000). Translocated individuals also may be linked to other nearby populations through natural movements (Epps et al., 2007, 2010). Thus, establishing a new population in an area with a high risk of contracting pathogens, either from wild conspecifics or domestic sheep, could result in inadvertent spread of the pathogen to previously unexposed populations within the region. Consequently, some otherwise suitable habitats may not be available for restoration under existing conditions (Shannon et al., 2014).
The lingering debate over which pathogens play a primary role in outbreaks of respiratory pneumonia clarifies one of the greatest problems with translocation: how can surveillance be conducted for pathogens for which their pathogenicity is unknown? Although managers were well-aware of the risks of disease from contact with domestic livestock, translocations prior to ca. 2012 may have spread M. ovipneumoniae to other herds or metapopulations of bighorn sheep. For instance, archived serum samples demonstrated that populations within California’s Mojave Desert showed evidence of previously unrecognized exposure to M. ovipneumoniae as early as 1986 (Shirkey et al., 2021), including some bighorn sheep used as source stock for within-state translocations (Bleich et al., 1990b).
Recently, a transmissible sinus tumor has been identified (Fox et al., 2011, 2016) that is associated with co-infection by M. ovipneumoniae, and may contribute to chronic shedding of that pathogen (Fox et al., 2015). Adult females that persistently carry and chronically shed M. ovipneumoniae appear to be an important mechanism causing persistent juvenile mortality (Plowright et al., 2017; Garwood et al., 2020). Thus, sinus tumors may have played a role in facilitating chronic shedding of M. ovipneumoniae and furthered the spread of the pathogen through translocation. Therefore, any movement of animals must be acknowledged as having some risk of spreading previously unrecognized pathogens.
Management guidelines developed in the recent past advocate health assessments for source and recipient populations prior to and following translocations. If the presence of pathogens is confirmed, or if substantial uncertainty regarding health status of either the source or recipient (or nearby) populations exists, translocations should be avoided (Brewer et al., 2014). Even when such guidelines are followed, however, the potential for spillover or pathogen transmission between wild populations remains a reality (Werdel et al., 2020).
Finally, tradeoffs between the benefits and costs of inter-population connectivity are of concern, especially for landscapes where large-scale restoration of bighorn sheep was, or is, required. In most ecosystems, bighorn sheep exist in naturally or anthropogenically fragmented populations or groups. As described previously, movement among such populations is critically important to maintain genetic diversity, facilitate demographic rescue, or allow for recolonization of vacant habitat following a local extirpation (Bleich et al., 1990a; Epps et al., 2006). Therefore, when re-establishing bighorn sheep across landscapes, a metapopulation is the ideal distribution to enable these processes to approximate demographics observed in largely intact systems by creating local populations that could be linked by natural dispersal. Yet, that same inter-connectedness likewise provides simultaneous opportunities for the spread of pathogens by contact, as observed in Rocky Mountain bighorn sheep in the Hells Canyon system (Cassirer et al., 2017). One strategy to ameliorate this tradeoff would be to establish systems of populations potentially linked by dispersal but separated from other such systems either by distance or by potential barriers.
7. Conservation, restoration, and the role of hunting
The genesis of current efforts to restore bighorn sheep began with total protection in many jurisdictions during the late 1800s. At that time, total protection was a good-faith effort to halt the decline of bighorn sheep, and it occurred at a time when it was desperately needed; nevertheless, that status ultimately hindered or confounded efforts to conserve or restore bighorn sheep to their historical ranges. For example, in California, such protection was completely insufficient, and indirectly hindered conservation by discouraging allocation of resources to non-game species; the results were inadequate inventory data, absence of awareness that populations were disappearing—with the result that there were no efforts to stop those losses—and, subsequently, a lack of incentive for active management (Wehausen et al., 1987; Bleich, 2006; Bleich and Weaver, 2007).
Continuing losses indicated that legislative protection did not affect factors that led to extirpations; the notion that total protection would stop any declines likely revolved around the mistaken assumptions that: (1) over-hunting was a cause of extirpations, and (2) that protected populations would increase in size and expand into unoccupied habitat. Both of those assumptions were faulty (Wehausen et al., 1987). The first failed to consider the potential role of diseases and habitat destruction, and the second was erroneous, because some mountain sheep may be slow to disperse from occupied ranges (Geist, 1971).
Many may view with irony the essential role that hunting has played in the restoration of bighorn sheep and other large mammals in North America. Thus far, funds from hunting that have helped with restoration have been a remarkable conservation success, as a result of efforts by wildlife and land management agencies, conservation organizations, concerned members of the academic community, private landowners, and other stakeholders (Heffelfinger et al., 2013; Krausman and Bleich, 2013; Hurley et al., 2015; Bleich, 2018). Among those stakeholders have been individuals that hunt “big game,” and numerous conservation organizations that support the scientific management and legal harvest of bighorn sheep (Hurley et al., 2015). Indeed, regulated hunting has played an extremely important role in the restoration of bighorn sheep, both directly and especially indirectly, through provision of funds to implement restoration efforts (Williamson, 1987; Regan, 2010).
Bighorn sheep likely experience the most conservative management of hunted species in North America (LaSharr et al., 2019). As a result, both government and non-governmental organizations have seized opportunities to generate funds specifically for restoration, and millions of US dollars are raised for that purpose through the sale of special permits on an annual basis (Hurley et al., 2015). In the United States and Canada, where most hunting opportunities occur on federal or crown lands, sale of those permits are largely cooperative efforts between state or provincial wildlife agencies and nongovernmental organizations having a special interest in the conservation of bighorn sheep (DiGrazia, 1999). In Mexico, however, most efforts to re-establish bighorn sheep populations have occurred on private land. Financial incentives through the sale of permits by private landowners have resulted in widespread and incredibly successful efforts to restore bighorn sheep to historically occupied mountain ranges in that country (Brewer and McEnroe, 2020). Regardless of the source, available funds will be stretched further as the human population continues to increase, and subsequent demands on the environment create new challenges to conservation (Krausman and Bleich, 2013; Bowyer et al., 2019).
The restoration of bighorn sheep populations through translocation is extremely costly, and costs continue to escalate; for example, from 1983 to 1989 the average cost of translocating a bighorn sheep (n = 336) was $ 2,257 (Bleich, 1990). Corrected for inflation, the average cost was $6,025/animal translocated during 2022. Although agencies provide the bulk of funds associated with translocation efforts, monies generated through the sale of hunting permits, whether through special opportunity permits (i.e., fund-raising tags) made available by conservation agencies, First Nations or Tribes, or enterprising landowners that have realized the financial benefits of bighorn sheep on their property, have played important roles in the conservation of these iconic ungulates. For example, one organization that is comprised largely of hunter-conservationists has raised approximately $136 M since 1977, and from 2011 to 2021 alone, that organization contributed $49.75 M toward bighorn sheep conservation (Wild Sheep Foundation, 2022). Dozens of similar organizations have contributed millions of dollars more specifically to conserve or restore bighorn sheep on an annual basis (Hurley et al., 2015). Fortunately, an increasing proportion of the public is gaining an appreciation of the importance of a broad funding base for wildlife conservation, and “…it is appropriate [and essential] that all citizens contribute to the cost of wildlife conservation” (Regan, 2010), nonetheless, it is essential that even more people understand and practice a conservation ethic. Funds raised through the sale of special opportunity tags are but one example of that conservation ethic and continue to play an important role in efforts to conserve bighorn sheep across North America. We anticipate that source of stakeholder funding will become even more important in the future.
8. Future considerations
While translocation has been the primary tool used to re-establish bighorn sheep in alpine, desert, and other ecosystems across western North America, future use of that method may be more limited (Bleich et al., 2021). Reducing risk of spillover of disease from domestic livestock is critically important to the effective restoration of this species, and future translocation efforts must squarely confront ways to minimize contact with domestic sheep or goats. In many regions, particularly those with large numbers of small private landowners with livestock, the risk of pathogen spillover simply may be too great to overcome. Thus, managers may need to shift from a paradigm where “excess” individuals from successful populations were used primarily as translocation stock to a new paradigm where both males and females are subject to hunter harvest.
Harvest of female bighorn sheep remains uncommon, but it is practiced in several states and provinces (Monteith et al., 2013, 2018; LaSharr et al., 2019). A male-biased harvest, however, has a limited effect on population dynamics of ungulates, because abundance of males has little influence on nutrition of females and, consequently, recruitment of young into the population (McCullough, 1979, 2001; Bowyer et al., 2014, 2020; Monteith et al., 2018). Thus, female harvest offers a beneficial, but underused, management option for regulating density-dependent processes for many ungulate populations by holding populations below K (ecological carrying capacity; Monteith et al., 2018). Consequently, females occupy the principal role in the dynamics of most ungulate populations, and female harvest can allow managers to manipulate population size to decrease nutritional limitations and competition for resources (McCullough, 1979, 2001; Solberg et al., 2002), or to decrease the potential for pathogen transmission by reducing population density. Moreover, body mass and fat reserves of females are strongly related to horn size in mature male bighorn sheep—a management objective for many populations of these ungulates (LaSharr et al., 2019). The decision to implement such harvests, however, rests on several biological and sociological considerations.
Translocation is subject to other constraints as well—the growing recognition that moving animals always includes risk of moving diseases. In some instances, lack of public support for efforts that may increase risk of death of translocated individuals may require removal of apex predators, or otherwise raise concerns about animal welfare. Managers also face continued uncertainty about the appropriateness of allowing mixing of bighorn sheep from different evolutionary lineages, or continued questions about the most appropriate source of bighorn sheep to be used in restoration efforts (Epps et al., 2019). Finally, because of the lingering legacies of founder effects and genetic bottlenecks, managers face questions about how best to manage for genetic diversity while limiting disease transmission risk among bighorn sheep—both from natural movement and translocation. Little is yet known about how variation in genetic diversity contributes to success of restored populations, beyond examples of extreme isolation and inbreeding (e.g., Hogg et al., 2006), and this remains an active and important area of consideration for ensuring the long-term success of extensive restoration efforts in the 21st century.
Author contributions
JW, VB, RB, and CE designed the study and wrote an initial draft of the manuscript and finalized it. All authors contributed to the article and approved the submitted version.
Acknowledgments
We thank colleagues who, throughout the years, have participated in stimulating discussions about bighorn sheep conservation. This is Professional Paper 135 from the Eastern Sierra Center for Applied Population Ecology.
Conflict of interest
The authors declare that the research was conducted in the absence of any commercial or financial relationships that could be construed as a potential conflict of interest.
Publisher’s note
All claims expressed in this article are solely those of the authors and do not necessarily represent those of their affiliated organizations, or those of the publisher, the editors and the reviewers. Any product that may be evaluated in this article, or claim that may be made by its manufacturer, is not guaranteed or endorsed by the publisher.
Footnotes
References
Almberg, E. S., Manlove, K. R., Cassirer, E. F., Ramsey, J., Carson, K., Gude, J., et al. (2022). Modelling management strategies for chronic disease in wildlife: predictions for the control of respiratory disease in bighorn sheep. J. Appl. Ecol. 59, 693–703. doi: 10.1111/1365-2664.14084
Anderson, K., Cahn, M. L., Stephenson, T. R., Few, A. P., Hatfield, B. E., German, D. W., et al. (2022). Cost distance models to predict contact between bighorn sheep and domestic sheep. Wildl. Soc. Bull. 46:e1329. doi: 10.1002/wsb.1329
Armstrong, D. P., and Seddon, P. J. (2008). Directions in reintroduction biology. Trends Ecol. Evol. 23, 20–25. doi: 10.1016/j.tree.2007.10.003
Atwood, T. B., Valentine, S. A., Hammill, E., McCauley, D. J., Madin, E. M., Beard, K. H., et al. (2020). Herbivores at the highest risk of extinction among mammals, birds, and reptiles. Sci. Adv. 6:eabb8458. doi: 10.1126/sciadv.abb8458
Barbosa, S., Andrews, K. R., Harris, R. B., Gour, D. S., Adams, J. R., Cassirer, E. F., et al. (2021). Genetic diversity and divergence among bighorn sheep from reintroduced herds in Washington and Idaho. J. Wildl. Manag. 85, 1214–1231. doi: 10.1002/jwmg.22065
Barboza, P. S., and Bowyer, R. T. (2000). Sexual segregation in dimorphic deer: a new gastrocentric hypothesis. J. Mammal. 81, 473–489. doi: 10.1644/1545-1542(2000)081<0473:SSIDDA>2.0.CO;2
Barboza, P. S., and Bowyer, R. T. (2001). Seasonality of sexual segregation in dimorphic deer: extending the gastrocentric model. Alces 37, 275–292.
Baumer, A., East, N., Echenique, J., Haworth, M., Leinssar, M., Papouchis, C., et al. (2009). A process for identifying and managing risk of contact between Sierra Nevada bighorn sheep and domestic sheep. US Fish and Wildlife Service, Ventura, California, USA.
Beecham, J., Collins, C., and Reynolds, T. D. (2007). Rocky Mountain Bighorn Sheep (Ovis canadensis): A Technical Conservation Assessment. USDA Forest Service, Rocky Mountain Region
Berger, J. (1978). Group size, foraging, and antipredator ploys: analysis of bighorn sheep decisions. Behav. Ecol. Sociobiol. 4, 91–99. doi: 10.1007/BF00302563
Berger, J. (1991). Pregnancy incentives, predation constraints and habitat shifts: experimental and field evidence for wild bighorn sheep. Anim. Behav. 41, 61–77. doi: 10.1016/S0003-3472(05)80503-2
Berger, J., Wangchuk, T., Briceno, C., Vila, A., and Lambert, J. E. (2020). Disassembled food webs and messy projections: modern ungulate communities in the face of unabating human population growth. Front. Ecol. Evol. 8:128. doi: 10.3389/fevo.2020.00128
Besser, T. E., Cassirer, E. F., Highland, M. A., Wolff, P., Justice-Allen, A., Mansfield, K., et al. (2013). Bighorn sheep pneumonia: sorting out the cause of a polymicrobial disease. Prev. Vet. Med. 108, 85–93. doi: 10.1016/j.prevetmed.2012.11.018
Besser, T. E., Cassirer, E. F., Lisk, A., Nelson, D., Manlove, K. R., Cross, P. C., et al. (2021). Natural history of a bighorn sheep pneumonia epizootic: source of infection, course of disease, and pathogen clearance. Ecol. Evol. 11, 14366–14382. doi: 10.1002/ece3.8166
Besser, T. E., Cassirer, E. F., Potter, K. A., Vander Schalie, J., Fischer, A., Knowles, D. P., et al. (2008). Association of Mycoplasma ovipneumoniae infection with population-limiting respiratory disease in free-ranging Rocky Mountain bighorn sheep (Ovis canadensis canadensis). J. Clin. Microbiol. 46, 423–430. doi: 10.1128/JCM.01931-07
Besser, T. E., Highland, M. A., Baker, K., Cassirer, E. F., Anderson, N. J., Ramsey, J. M., et al. (2012). Causes of pneumonia epizootics among bighorn sheep, western United States, 2008-2010. Emerg. Infect. Dis. 18, 406–414. doi: 10.3201/eid1803.111554
Blaisdell, J. (1972). Progress report—lava beds bighorn re-establishment. Desert Bighorn Coun. Trans. 16, 84–87.
Bleich, V. C. (1990). “Costs of translocating mountain sheep” in Managing Wildlife in the Southwest. eds. P. R. Krausman and N. S. Smith (Phoenix, Arizona: Arizona Chapter of The Wildlife Society), 67–75.
Bleich, V. C. (1996). Interactions between coyotes (Canis latrans) and mountain sheep (Ovis canadensis). Southwest. Nat. 41, 81–82.
Bleich, V. C. (1999). Mountain sheep and coyotes: patterns of predator evasion in a mountain ungulate. J. Mammal. 80, 283–289. doi: 10.2307/1383228
Bleich, V. C. (2005). Politics, promises, and illogical legislation confound wildlife conservation. Wildl. Soc. Bull. 33, 66–73. doi: 10.2193/0091-7648(2005)33[66:IMOPPA]2.0.CO;2
Bleich, V. C. (2006). Mountain sheep in California: perspectives on the past, and prospects for the future. Proc. North. Wild Sheep Goat Coun. 15, 1–13.
Bleich, V. C. (2009). Factors to consider when reprovisioning water developments used by mountain sheep. Cal. Fish Game 95, 153–159.
Bleich, V. C. (2016). Wildlife conservation and wilderness: wishful thinking? Nat. Areas J. 36, 202–206. doi: 10.3375/043.036.0213
Bleich, V. C. (2018). Maintaining momentum for conservation: bighorn sheep as an example. Wildl. Soc. Bull. 42, 540–546. doi: 10.1002/wsb.914
Bleich, V. C. (2020). Mountain lions, minimum viable populations, and intact ecosystems: a cautionary note. Ecol. Appl. 30:e01990. doi: 10.1002/eap.1990
Bleich, V. C., Blum, M. E., Shoemaker, K. T., Sustaita, D., and Holl, S. A. (2019). Habitat selection by bighorn sheep in a mesic ecosystem: the San Rafael Mountains, California, USA. Calif. Fish Game 105, 205–224.
Bleich, V. C., Bowyer, R. T., and Wehausen, J. D. (1997). Sexual segregation in mountain sheep: resources or predation? Wildl. Monogr. 134, 1–50.
Bleich, V. C., Cassirer, E. F., Coggins, V. L., Oldenburg, L. E., and Hunter, D. E. (2004). Predation by a golden eagle, Aquila chrysaetos, on a juvenile mountain sheep. Cal. Fish Game 90, 91–93.
Bleich, V. C., and Holl, S. A. (1982). Management of chaparral habitat for mule deer and mountain sheep in southern California. USDA For. Serv. Gen. Tech. Rep. PSW-58, 247–254.
Bleich, V. C., Johnson, H. E., Holl, S. A., Konde, L., Torres, S. G., and Krausman, P. R. (2008). Fire history in a chaparral ecosystem: implications for conservation of a native ungulate. Rangel. Ecol. Manag. 61, 571–579. doi: 10.2111/07-016.1
Bleich, V. C., Oehler, M. W., and Kie, J. G. (2020). “Managing rangelands for wildlife” in The Wildlife Management Techniques Manual. ed. N. J. Silvy (Baltimore, MD: Johns Hopkins University Press), 126–148.
Bleich, V. C., Sargeant, G. A., and Wiedmann, B. P. (2018). Ecotypic variation in population dynamics of reintroduced bighorn sheep: implications for management. J. Wildl. Manag. 82, 8–18. doi: 10.1002/jwmg.21381
Bleich, V. C., and Weaver, R. A. (2007). Status of mountain sheep in California: comparisons between 1957 and 2007. Desert Bighorn Coun. Trans. 49, 55–67.
Bleich, V. C., Wehausen, J. D., and Holl, S. A. (1990a). Desert-dwelling mountain sheep: conservation implications of a naturally fragmented distribution. Conserv. Biol. 4, 383–390. doi: 10.1111/j.1523-1739.1990.tb00312.x
Bleich, V. C., Wehausen, J. D., Jones, K. R., and Weaver, R. A. (1990b). Status of bighorn sheep in California, 1989 and translocations from 1971 through 1989. Desert Bighorn Coun. Trans. 34, 24–26.
Bleich, V. C., Wehausen, J. D., Ramey, R. R., and Rechel, J. L. (1996). “Metapopulation theory and mountain sheep: implications for conservation” in Metapopulations and Wildlife Conservation. ed. D. R. McCullough (Washington, DC: Island Press), 353–373.
Bleich, V. C., Wehausen, J. D., Torres, S. G., Anderson, K. A., and Stephenson, T. R. (2021). Fifty years of bighorn sheep translocations: details from California (1971–2020). Desert Bighorn Coun. Trans. 56, 1–32.
Bowyer, R. T. (2004). Sexual segregation in ruminants: definitions, hypotheses, and implications for conservation and management. J. Mammal. 85, 1039–1052. doi: 10.1644/BBL-002.1
Bowyer, R. T. (2022). Sexual Segregation in Ungulates: Ecology, Behavior, and Conservation. Maryland: Johns Hopkins University Press
Bowyer, R. T., Bleich, V. C., Stewart, K. M., Whiting, J. C., and Monteith, K. L. (2014). Density dependence in ungulates: a review of causes, and concepts with some clarifications. Calif. Fish Game 100, 550–572.
Bowyer, R. T., Boyce, M. S., Goheen, J. R., and Rachlow, J. L. (2019). Conservation of the world’s mammals: status, protected areas, community efforts, and hunting. J. Mammal. 100, 923–941. doi: 10.1093/jmammal/gyy180
Bowyer, R. T., Stewart, K. M., Bleich, V. C., Whiting, J. C., Monteith, K. L., Blum, M. E., et al. (2020). Metrics of harvest for ungulate populations: misconceptions, lurking variables, and prudent mangement. Alces 56, 15–38.
Boyce, W. M., and Weisenberger, M. E. (2005). The rise and fall of psoroptic scabies in bighorn sheep in the San Andres Mountains, New Mexico. J. Wildl. Dis. 41, 525–531. doi: 10.7589/0090-3558-41.3.525
Brewer, C., Bleich, V. C., Foster, J., Hosch-Hebdon, T., McWhirter, D., Rominger, E., et al. (2014). Bighorn sheep: Conservation challenges and management strategies for the 21st century. Western Association of Fish and Wildlife Agencies.
Brewer, C., and McEnroe, A. (2020). Justice league: WSF partner Mexico Council's stunning achievements. Wild Sheep Fall, 46–56.
Buchalski, M. R., Sacks, B. N., Gille, D. A., Penedo, M. C. T., Ernest, H. B., Morrison, S. A., et al. (2016). Phylogeographic and population genetic structure of bighorn sheep (Ovis canadensis) in north American deserts. J. Mammal. 97, 823–838. doi: 10.1093/jmammal/gyw011
Buechner, H. K. (1960). The bighorn sheep in the United States, its past, present, and future. Wildl. Monogr. 4, 1–174.
Bunch, T. D., Wu, C., Zhang, Y.-P., and Wang, S. (2006). Phylogenetic analysis of snow sheep (Ovis nivicola) and closely related taxa. J. Hered. 97, 21–30. doi: 10.1093/jhered/esi127
Butler, C. J., Edwards, W. H., Paterson, J. T., Proffitt, K. M., Jennings-Gaines, J. E., Killion, H. J., et al. (2018). Respiratory pathogens and their association with population performance in Montana and Wyoming bighorn sheep populations. PLoS One 13:e0207780. doi: 10.1371/journal.pone.0207780
Cahn, M. L., Conner, M. M., Schmitz, O. J., Stephenson, T. R., Wehausen, J. D., and Johnson, H. E. (2011). Disease, population viability, and recovery of endangered Sierra Nevada bighorn sheep. J. Wildl. Manag. 75, 1753–1766. doi: 10.1002/jwmg.232
Cain, J. W., Johnson, H. E., and Krausman, P. R. (2005). Wildfire and desert bighorn sheep habitat, Santa Catalina Mountains, Arizona. Southwest. Nat. 50, 506–513. doi: 10.1894/0038-4909(2005)050[0506:WADBSH]2.0.CO;2
Cain, J. W., Krausman, P. R., Morgart, J. R., Jansen, B. D., and Pepper, M. P. (2008). Responses of desert bighorn sheep to removal of water sources. Wildl. Monogr. 171, 1–32. doi: 10.2193/2007-209
Cardillo, M., Mace, G. M., Jones, K. E., Bielby, J., Bininda-Emonds, O. R., Sechrest, W., et al. (2005). Multiple causes of high extinction risk in large mammal species. Science 309, 1239–1241. doi: 10.1126/science.1116030
Carpenter, T. E., Coggins, V. L., McCarthy, C., O'Brien, C. S., O'Brien, J. M., and Schommer, T. J. (2014). A spatial risk assessment of bighorn sheep extirpation by grazing domestic sheep on public lands. Prev. Vet. Med. 114, 3–10. doi: 10.1016/j.prevetmed.2014.01.008
Cassirer, E. F., Manlove, K. R., Almberg, E. S., Kamath, P. L., Cox, M., Wolff, P., et al. (2018). Pneumonia in bighorn sheep: risk and resilience. J. Wildl. Manag. 82, 32–45. doi: 10.1002/jwmg.21309
Cassirer, E. F., Manlove, K. R., Plowright, R. K., and Besser, T. E. (2017). Evidence for strain-specific immunity to pneumonia in bighorn sheep. J. Wildl. Manag. 81, 133–143. doi: 10.1002/jwmg.21172
Cassirer, E. F., Plowright, R. K., Manlove, K. R., Cross, P. C., Dobson, A. P., Potter, K. A., et al. (2013). Spatio-temporal dynamics of pneumonia in bighorn sheep. J. Anim. Ecol. 82, 518–528. doi: 10.1111/1365-2656.12031
Cassirer, E. F., and Sinclair, A. R. E. (2007). Dynamics of pneumonia in a bighorn sheep metapopulation. J. Wildl. Manag. 71, 1080–1088. doi: 10.2193/2006-002
Caughley, G., and Krebs, C. J. (1983). Are big mammals simply little mammals writ large? Oecologia 59, 7–17. doi: 10.1007/BF00388066
Ceballos, G., and Ehrlich, P. R. (2002). Mammal population losses and the extinction crisis. Science 296, 904–907. doi: 10.1126/science.1069349
Ceballos, G., Ehrlich, P. R., and Dirzo, R. (2017). Biological annihilation via the ongoing sixth mass extinction signaled by vertebrate population losses and declines. Proc. Natl. Acad. Sci. U. S. A. 114, E6089–E6096. doi: 10.1073/pnas.1704949114
Clapp, J. G., and Beck, J. L. (2016). Short-term impacts of fire-mediated habitat alterations on an isolated bighorn sheep population. Fire Ecol. 12, 80–98. doi: 10.4996/fireecology.1203080
Clifford, D. L., Schumaker, B. A., Stephenson, T. R., Bleich, V. C., Cahn, M. L., Gonzales, B. J., et al. (2009). Assessing disease risk at the wildlife-livestock interface: a study of Sierra Nevada bighorn sheep. Biol. Conserv. 142, 2559–2568. doi: 10.1016/j.biocon.2009.06.001
Colchero, F., Medellin, R. A., Clark, J. S., Lee, R., and Katul, G. G. (2009). Predicting population survival under future climate change: density dependence, drought and extraction in an insular bighorn sheep. J. Anim. Ecol. 78, 666–673. doi: 10.1111/j.1365-2656.2009.01528.x
Conner, M. M., Stephenson, T. R., German, D. W., Monteith, K. L., Few, A. P., and Bair, E. H. (2018). Survival analysis: informing recovery of Sierra Nevada bighorn sheep. J. Wildl. Manag. 82, 1442–1458. doi: 10.1002/jwmg.21490
Cowan, I. M. (1940). Distribution and variation in the native sheep of North America. Ornithology 24, 505–580. doi: 10.2307/2420858
Creech, T. G., Epps, C. W., Monello, R. J., and Wehausen, J. D. (2016). Predicting diet quality and genetic diversity of a desert-adapted ungulate with NDVI. J. Arid Environ. 127, 160–170. doi: 10.1016/j.jaridenv.2015.11.011
Creech, T., Epps, C. W., Wehausen, J. D., Crowhurst, R. S., Jaeger, J. R., Longshore, K., et al. (2020). Genetic and environmental indicators of climate change vulnerability for desert bighorn sheep. Front. Ecol. Evol. 8:279. doi: 10.3389/fevo.2020.00279
Dassanayake, R. P., Shanthalingam, S., Herndon, C. N., Subramaniam, R., Lawrence, P. K., Bavananthasivam, J., et al. (2010). Mycoplasma ovipneumoniae can predispose bighorn sheep to fatal Mannheimia haemolytica pneumonia. Vet. Microbiol. 145, 354–359. doi: 10.1016/j.vetmic.2010.04.011
Davidson, A. D., Shoemaker, K. T., Weinstein, B., Costa, G. C., Brooks, T. M., Ceballos, G., et al. (2017). Geography of current and future global mammal extinction risk. PLoS One 12:e0186934. doi: 10.1371/journal.pone.0186934
DeBruin, H. W. (1974). From fire control to fire management: a major policy change in the Forest Service. Proc. Tall Timbers Fire Ecol. Conf. 14, 11–17.
DeCesare, N., Hebblewhite, M., Robinson, H., and Musiani, M. (2010). Endangered, apparently: the role of apparent competition in endangered species conservation. Anim. Conserv. 13, 353–362. doi: 10.1111/j.1469-1795.2009.00328.x
DeForge, J., Jimenez, S., Ostermann, S., Barrett, E., Valdez, R., and Cecilia, C. (1997). Translocation and population modeling of Weems desert bighorn in Baja California Sur, Mexico. Desert Bighorn Coun. Trans. 41, 44–50.
Dekelaita, D. J., Epps, C. W., Stewart, K. M., Sedinger, J. S., Powers, J. G., Gonzales, B. J., et al. (2020). Survival of adult female bighorn sheep following a pneumonia epizootic. J. Wildl. Manag. 84, 1268–1282. doi: 10.1002/jwmg.21914
DiGrazia, R. E. (1999). “Fund raising for the future of wild sheep” in Putting Sheep on the Mountain—The Foundation for North American Wild Sheep: Twenty-Five Years Dedicated to Wild Sheep 1974 to 1999. eds. R. A. Schultz, D. A. Pedrotti, and S. C. Reneau (Wyoming, USA: Foundation for North American Wild Sheep)
Dobzhansky, T. (1948). Genetics of natural populations. XVIII. Experiments on chromosomes of Drosophila pseudoobscura from different geographic regions. Genetics 33:588.
Dolan, B. F. (2006). Water developments and desert bighorn sheep: implications for conservation. Wildl. Soc. Bull. 34, 642–646. doi: 10.2193/0091-7648(2006)34[642:WDADBS]2.0.CO;2
Donovan, V. M., Roberts, C. P., Wonkka, C. L., Beck, J. L., Popp, J. N., Allen, C. R., et al. (2020). Range-wide monitoring of population trends for Rocky Mountain bighorn sheep. Biol. Conserv. 248:108639. doi: 10.1016/j.biocon.2020.108639
Dubay, S., Schwantje, H., de Vos, J., and McKinney, T. (2004). Bighorn sheep (Ovis canadensis) diseases: a brief literature review and risk assessment for translocation. Proc. Annu. Meet. United States Anim. Health Assoc. 107, 600–621.
Dugovich, B. S., Beechler, B. R., Dolan, B. P., Crowhurst, R. S., Gonzales, B. J., Powers, J. G., et al. (2023). Population connectivity patterns of genetic diversity, immune responses, and exposure to infectious pneumonia in a metapopulation of desert bighorn sheep. J. Anim. Ecol. doi: 10.1111/1365-2656.13885
Epps, C. W., Bowen, E., Buchalski, M. R., Cassirer, F., Coltman, D., Conway, W. C., et al. (2019). “Frequently-asked questions about wild sheep genetics and genomics.” in Proceedings of 2018 North American Wild Sheep Goat Symposium, 2018, 45–75.
Epps, C. W., Crowhurst, R. S., and Nickerson, B. S. (2018). Assessing changes in functional connectivity in a desert bighorn sheep metapopulation after two generations. Mol. Ecol. 27, 2334–2346. doi: 10.1111/mec.14586
Epps, C. W., McCullough, D. R., Wehausen, J. D., Bleich, V. C., and Rechel, J. L. (2004). Effects of climate change on population persistence of desert-dwelling mountain sheep in California. Conserv. Biol. 18, 102–113. doi: 10.1111/j.1523-1739.2004.00023.x
Epps, C. W., Palsboll, P. J., Wehausen, J. D., Roderick, G. K., and McCullough, D. R. (2006). Elevation and connectivity define genetic refugia for mountain sheep as climate warms. Mol. Ecol. 15, 4295–4302. doi: 10.1111/j.1365-294X.2006.03103.x
Epps, C. W., Palsboll, P. J., Wehausen, J. D., Roderick, G. K., Ramey, R. R., and McCullough, D. R. (2005). Highways block gene flow and cause a rapid decline in genetic diversity of desert bighorn sheep. Ecol. Lett. 8, 1029–1038. doi: 10.1111/j.1461-0248.2005.00804.x
Epps, C. W., Wehausen, J. D., Bleich, V. C., Torres, S. G., and Brashares, J. S. (2007). Optimizing dispersal and corridor models using landscape genetics. J. Appl. Ecol. 44, 714–724. doi: 10.1111/j.1365-2664.2007.01325.x
Epps, C. W., Wehausen, J. D., Palsbøll, P. J., and McCullough, D. R. (2010). Using genetic tools to track desert bighorn sheep colonizations. J. Wildl. Manag. 74, 522–531. doi: 10.2193/2008-448
Fairbanks, W. S., Bailey, J. A., and Cook, R. S. (1987). Habitat use by a low-elevation, semicaptive bighorn sheep population. J. Wildl. Manag. 51, 912–915. doi: 10.2307/3801759
Few, A. P., German, D. W., Wehausen, J. D., Pierce, B. M., and Stephenson, T. R. (2013). "Translocation Plan for Sierra Nevada Bighorn sheep." Bishop, California: California Department of Fish and Game
Flesch, E., Graves, T., Thomson, J., Proffitt, K., and Garrott, R. (2022). Average kinship within bighorn sheep populations is associated with connectivity, augmentation, and bottlenecks. Ecosphere 13:e3972. doi: 10.1002/ecs2.3972
Flesch, E. P., Graves, T. A., Thomson, J. M., Proffitt, K. M., White, P., Stephenson, T. R., et al. (2020). Evaluating wildlife translocations using genomics: a bighorn sheep case study. Ecol. Evol. 10, 13687–13704. doi: 10.1002/ece3.6942
Foreyt, W. J., Jenkins, E., and Appleyard, G. (2009). Transmission of lungworms (Muellerius capillaris) from domestic goats to bighorn sheep on common pasture. J. Wildl. Dis. 45, 272–278. doi: 10.7589/0090-3558-45.2.272
Foreyt, W. J., and Jessup, D. A. (1982). Fatal pneumonia of bighorn sheep following association with domestic sheep. J. Wildl. Dis. 18, 163–168. doi: 10.7589/0090-3558-18.2.163
Fox, K. A., Rouse, N. M., Huyvaert, K. P., Griffin, K. A., Killion, H. J., Jennings-Gaines, J., et al. (2015). Bighorn sheep (Ovis canadensis) sinus tumors are associated with coinfections by potentially pathogenic bacteria in the upper respiratory tract. J. Wildl. Dis. 51, 19–27. doi: 10.7589/2014-05-130
Fox, K., Wootton, S., Marolf, A., Rouse, N., LeVan, I., Spraker, T., et al. (2016). Experimental transmission of bighorn sheep sinus tumors to bighorn sheep (Ovis canadensis canadensis) and domestic sheep. Vet. Pathol. 53, 1164–1171. doi: 10.1177/0300985816634810
Fox, K., Wootton, S., Quackenbush, S., Wolfe, L., Levan, I., Miller, M., et al. (2011). Paranasal sinus masses of Rocky Mountain bighorn sheep (Ovis canadensis canadensis). Vet. Pathol. 48, 706–712. doi: 10.1177/0300985810383873
Garde, E., Kutz, S., Schwantje, H., Veitch, A., Jenkins, E., and Elkin, B. (2005). Examining the risk of disease transmission between wild Dall's sheep and mountain goats, and introduced domestic sheep, goats, and llamas in the Northwest Territories. Zoo. Wildl. Dis. 29, 1–149.
Garwood, T. J., Lehman, C. P., Walsh, D. P., Cassirer, E. F., Besser, T. E., and Jenks, J. A. (2020). Removal of chronic Mycoplasma ovipneumoniae carrier ewes eliminates pneumonia in a bighorn sheep population. Ecol. Evol. 10, 3491–3502. doi: 10.1002/ece3.6146
Geist, V. (1971). Mountain Sheep: A Study in Behavior and Evolution. Chicago: The University of Chicago Press
George, J. (2009). Colorado Bighorn sheep management plan 2009–2019. Colorado Division of Wildlife, Department of Natural Resources.
Gille, D. A., Buchalski, M. R., Conrad, D., Rubin, E. S., Munig, A., Wakeling, B. F., et al. (2019). Genetic outcomes of translocation of bighorn sheep in Arizona. J. Wildl. Manag. 83, 838–854. doi: 10.1002/jwmg.21653
Goble, D. D., Wiens, J. A., Scott, J. M., Male, T. D., and Hall, J. A. (2012). Conservation-reliant species. Bioscience 62, 869–873. doi: 10.1525/bio.2012.62.10.6
Gonzalez-Rebeles Islas, C., Mendez, M., and Valdez, R. (2019). “Evolution of wildlife laws and policy in Mexico” in Wildlife Ecology and Management in Mexico. eds. R. Valdez and J. Ortega-S (College Station, Texas: Texas A&M University Press), 366–377.
Green, L. R. (1977a). Fuel reduction without fire: current technology and ecosystem impact. USDA For. Serv. Gen. Tech. Rep. WO-3, 163–171.
Green, L. R. (1977b). Fuelbreaks and Other Fuel Modification for Wildland Fire Control 499. Washington, D.C.: US Department of Agriculture, Agricultural Handbook, 1–79.
Gross, J. E., Singer, F. J., and Moses, M. E. (2000). Effects of disease, dispersal, and area on bighorn sheep restoration. Restor. Ecol. 8, 25–37. doi: 10.1046/j.1526-100x.2000.80063.x
Gude, J. A., DeCesare, N. J., Proffitt, K. M., Sells, S. N., Garrott, R. A., Rangwala, I., et al. (2022). Demographic uncertainty and disease risk influence climate-informed management of an alpine species. J. Wildl. Manag. 86. doi: 10.1002/jwmg.22300
Gutiérrez-Espeleta, G. A., Kalinowski, S. T., Boyce, W. M., and Hedrick, P. W. (2000). Genetic variation and population structure in desert bighorn sheep: implications for conservation. Conserv. Genet. 1, 3–15. doi: 10.1023/A:1010125519304
Hailey, T. L. (1971). Reproduction and release of Texas transplanted desert bighorn sheep. Desert Bighorn Counc. Trans. 15, 97–100.
Hale, S. L., and Koprowski, J. L. (2018). Ecosystem‐level effects of keystone species reintroduction: a literature review. Restor. Ecol. 26, 439–445.
Hayes, C. L., Rubin, E. S., Jorgensen, M. C., Botta, R. A., and Boyce, W. M. (2000). Mountain lion predation of bighorn sheep in the peninsular ranges, California. J. Wildl. Manag. 64, 954–959. doi: 10.2307/3803204
Hayward, A. D., Garnier, R., Watt, K. A., Pilkington, J. G., Grenfell, B. T., Matthews, J. B., et al. (2014). Heritable, heterogeneous, and costly resistance of sheep against nematodes and potential feedbacks to epidemiological dynamics. Am. Nat. 184, S58–S76. doi: 10.1086/676929
Hedrick, P. W., Gutierrez-Espeleta, G. A., and Lee, R. N. (2001). Founder effect in an island population of bighorn sheep. Mol. Ecol. 10, 851–857. doi: 10.1046/j.1365-294X.2001.01243.x
Heffelfinger, J. R., Geist, V., and Wishart, W. (2013). The role of hunting in North American wildlife conservation. Int. J. Environ. Stud. 70, 399–413. doi: 10.1080/00207233.2013.800383
Hobbs, N., and Spowart, R. (1984). Effects of prescribed fire on nutrition of mountain sheep and mule deer during winter and spring. J. Wildl. Manag. 48, 551–560. doi: 10.2307/3801188
Hogg, J. T., Forbes, S. H., Steele, B. M., and Luikart, G. (2006). Genetic rescue of an insular population of large mammals. Proc. Roy. Soc. London Ser. B. Biol. Sci. 273, 1491–1499. doi: 10.1098/rspb.2006.3477
Holl, S. A., and Bleich, V. C. (2009). Reconstructing the San Gabriel Mountains bighorn sheep population. Calif. Fish Game 95, 77–87.
Holl, S. A., and Bleich, V. C. (2010). Responses of bighorn sheep and mule deer to fire and rain in the San Gabriel Mountains, California. Proc. North. Wild Sheep Goat Counc. 17, 139–156.
Holl, S. A., Bleich, V. C., Callenberger, B. W., and Bahro, B. (2012). Simulated effects of two fire regimes on bighorn sheep: the San Gabriel Mountains, California, USA. Fire Ecol. 8, 88–103. doi: 10.4996/fireecology.0803088
Holl, S. A., Bleich, V. C., and Torres, S. G. (2004). Population dynamics of bighorn sheep in the San Gabriel Mountains, California, 1967-2002. Wildl. Soc. Bull. 32, 412–426. doi: 10.2193/0091-7648(2004)32[412:PDOBSI]2.0.CO;2
Holt, R. D. (1977). Predation, apparent competition, and the structure of prey communities. Theor. Popul. Biol. 12, 197–229. doi: 10.1016/0040-5809(77)90042-9
Holt, R. D., and Lawton, J. H. (1994). The ecological consequences of shared natural enemies. Annu. Rev. Ecol. Evol. Syst. 25, 495–520. doi: 10.1146/annurev.es.25.110194.002431
Honess, R. F., and Frost, N. M. (1942). A Wyoming bighorn sheep study. Bull. Wyoming Game Fish Depart. 1, 1–127.
Hurley, K., Brewer, C., and Thornton, G. N. (2015). The role of hunters in conservation, restoration, and management of North American wild sheep. Int. J. Environ. Stud. 72, 784–796. doi: 10.1080/00207233.2015.1031567
IUCN (International Union for the Conservation of Nature). (1987). Position statement on translocations of living organisms: introductions, reintroductions, and re-stocking. Gland, Switzerland: International Union for the Conservation of Nature and Natural Resources Council.
Jahner, J. P., Matocq, M. D., Malaney, J. L., Cox, M., Wolff, P., Gritts, M. A., et al. (2019). The genetic legacy of 50 years of desert bighorn sheep translocations. Evol. Appl. 12, 198–213. doi: 10.1111/eva.12708
Jansen, B. D., Heffelfinger, J. R., Noon, T. H., Krausman, P. R., and deVos Jr, J. C. (2006). Infectious keratoconjunctivitis in bighorn sheep, Silver Bell Mountains, Arizona. Bull. Wildlife Dis. Assoc. 42, 407–411. doi: 10.7589/0090-3558-42.2.407
Jansen, B. D., Krausman, P. R., Heffelfinger, J. R., Noon, T. H., and Devos, J. C. (2007). Population dynamics and behavior of bighorn sheep with infectious keratoconjunctivitis. J. Wildl. Manag. 71, 571–575. doi: 10.2193/2005-617
Jesmer, B. R., Merkle, J. A., Goheen, J. R., Aikens, E. O., Beck, J. L., Courtemanch, A. B., et al. (2018). Is ungulate migration culturally transmitted? Evidence of social learning from translocated animals. Science 361, 1023–1025. doi: 10.1126/science.aat0985
Jessup, D. A., DeJesus, S. R., Clark, W. E., and Bleich, V. C. (2015). Evolution of ungulate capture techniques in California. Calif. Fish Game 100, 491–526.
Jex, B. A., Ayotte, J. B., Bleich, V. C., Brewer, C. E., Bruning, D. L., Hegel, T. M., et al. (2016). "Thinhorn sheep: Conservation challenges and management strategies for the 21st century". Boise, Idaho: Western Association of Fish and Wildlife Agencies.
Johnson, H. E., Hebblewhite, M., Stephenson, T. R., German, D. W., Pierce, B. M., and Bleich, V. C. (2013). Evaluating apparent competition in limiting the recovery of an endangered ungulate. Oecologia 171, 295–307. doi: 10.1007/s00442-012-2397-6
Johnson, H. E., Mills, L. S., Wehausen, J. D., Stephenson, T. R., and Luikart, G. (2011). Translating effects of inbreeding depression on component vital rates to overall population growth in endangered bighorn sheep. Conserv. Biol. 25, 1240–1249. doi: 10.1111/j.1523-1739.2011.01739.x
Johnson, B. M., Stroud-Settles, J., Roug, A., and Manlove, K. (2022). Disease ecology of a low-virulence Mycoplasma ovipneumoniae strain in a free-ranging desert bighorn sheep population. Animals 12:1029. doi: 10.3390/ani12081029
Kie, J. G., Bowyer, R. T., and Stewart, K. M. (2003). “Ungulates in western coniferous forests: habitat relationships, population dynamics, and ecosystem processes” in Mammal Community Dynamics: Management and Conservation in the Coniferous Forests of Western North America. eds. C. J. Zabel and R. G. Anthony (New York: Cambridge University Press), 296–340.
Krausman, P. R. (2000). An introduction to the restoration of bighorn sheep. Restor. Ecol. 8, 3–5. doi: 10.1046/j.1526-100x.2000.80060.x
Krausman, P. R., Bangs, P., Kunkel, K., Phillips, M. K., Parsons, Z., and Rominger, E. (2001). “Mountain sheep restoration through private/public parternership” in Large Mammal Restoration. eds. D. S. Maehr, R. F. Noss, and J. L. Larkin (Washington: Island Press), 231–242.
Krausman, P. R., and Bleich, V. C. (2013). Conservation and management of ungulates in North America. Int. J. Environ. Stud. 70, 372–382. doi: 10.1080/00207233.2013.804748
Laliberte, A. S., and Ripple, W. J. (2004). Range contractions of North American carnivores and ungulates. Bioscience 54, 123–138. doi: 10.1641/0006-3568(2004)054[0123:RCONAC]2.0.CO;2
Larkins, A. (2010). Wild sheep status and management in western North America: summary of state, province, and territory status report surveys. North. Wild Sheep Goat Counc. Proc. 17, 8–28.
Larsen, R. T., Bissonette, J. A., Flinders, J. T., and Whiting, J. C. (2012). Framework for understanding the influences of wildlife water developments in the western United States. Calif. Fish Game 98, 148–163.
LaSharr, T. N., Long, R. A., Heffelfinger, J. R., Bleich, V. C., Krausman, P. R., Bowyer, R. T., et al. (2019). Hunting and mountain sheep: do current harvest practices affect horn growth? Evol. Appl. 12, 1823–1836. doi: 10.1111/eva.12841
Latch, E. K., Heffelfinger, J. R., Wakeling, B. F., Hanna, J., Conrad, D., and Rhodes, O. E. Jr. (2006). “Genetic subspecies identification of a recently colonized bighorn sheep population in Central Arizona.” in Proceedings of the Managing Wildlife in the Southwest Symposium. Southwest Section of the Wildlife Society, eds. J. W. Cain and P. R. Krausman, 1–9.
Lee, R. (2011). Economic aspects of and the market for desert bighorn sheep. Desert Bighorn Coun. Trans. 51, 46–49.
Leslie, D. (1980). Remnant populations of desert bighorn sheep as a source for transplantation. Desert Bighorn Coun. Trans. 24, 36–44.
Leslie, D. M., and Douglas, C. L. (1979). Desert bighorn sheep of the River Mountains, Nevada. Wildl. Monogr. 66, 1–56.
Luikart, G., Pilgrim, K., Visty, J., Ezenwa, V. O., and Schwartz, M. K. (2008). Candidate gene microsatellite variation is associated with parasitism in wild bighorn sheep. Biol. Lett. 4, 228–231. doi: 10.1098/rsbl.2007.0633
Manlove, K., Cassirer, E. F., Cross, P. C., Plowright, R. K., and Hudson, P. J. (2016). Disease introduction is associated with a phase transition in bighorn sheep demographics. Plant World 97, 2593–2602. doi: 10.1002/ecy.1520
Manlove, K. R., Roug, A., Sinclair, K., Ricci, L. E., Hersey, K. R., Martinez, C., et al. (2022). Bighorn sheep show similar in-host responses to the same pathogen strain in two contrasting environments. Ecol. Evol. 12:e9109. doi: 10.1002/ece3.9109
Marsh, H. (1938). Pneumonia in Rocky Mountain bighorn sheep. J. Mammal. 19, 214–219. doi: 10.2307/1374617
Martin, A. M., Hogg, J. T., Manlove, K. R., LaSharr, T. N., Shannon, J. M., McWhirter, D. E., et al. (2022). Disease and secondary sexual traits: effects of pneumonia on horn size of bighorn sheep. J. Wildl. Manag. 86:e22154. doi: 10.1002/jwmg.22154
McCullough, D. R. (1979). The George Reserve Deer Herd: Population Ecology of a K-Selected Species. Ann Arbor, Michigan: The University of Michigan Press
McCullough, D. R. (2001). Male harvest in relation to female removals in a black-tailed deer population. J. Wildl. Manag. 65, 46–58. doi: 10.2307/3803276
McKee, J., Chambers, E., and Guseman, J. (2013). Human population density and growth validated as extinction threats to mammal and bird species. Hum. Ecol. 41, 773–778. doi: 10.1007/s10745-013-9586-8
McKinney, T., Devos, J. C., Ballard, W. B., and Boe, S. R. (2006). Mountain lion predation of translocated desert bighorn sheep in Arizona. Wildl. Soc. Bull. 34, 1255–1263. doi: 10.2193/0091-7648(2006)34[1255:MLPOTD]2.0.CO;2
Miller, J., Poissant, J., Hogg, J., and Coltman, D. (2012). Genomic consequences of genetic rescue in an insular population of bighorn sheep (Ovis canadensis). Mol. Ecol. 21, 1583–1596. doi: 10.1111/j.1365-294X.2011.05427.x
Monteith, K. L., Long, R. A., Bleich, V. C., Heffelfinger, J. R., Krausman, P. R., and Bowyer, R. T. (2013). Effects of harvest, culture, and climate on trends in size of horn-like structures in trophy ungulates. Wildl. Monogr. 183, 1–28. doi: 10.1002/wmon.1007
Monteith, K. L., Long, R. A., Stephenson, T. R., Bleich, V. C., Bowyer, R. T., and LaSharr, T. N. (2018). Horn size and nutrition in mountain sheep: can ewe handle the truth? J. Wild Mgmt. 82, 67–84. doi: 10.1002/jwmg.21338
Olson, Z. H., Whittaker, D. G., and Rhodes, O. E. Jr. (2012). Evaluation of experimental genetic management in reintroduced bighorn sheep. Ecol. Evol. 2, 429–443. doi: 10.1002/ece3.97
Olson, Z. H., Whittaker, D. G., and Rhodes, O. E. Jr. (2013). Translocation history and genetic diversity in reintroduced bighorn sheep. J. Wildl. Manag. 77, 1553–1563. doi: 10.1002/jwmg.624
Ostermann, S. D., DeForge, J. R., and Edge, W. D. (2001). Captive breeding and reintroduction evaluation criteria: a case study of peninsular bighorn sheep. Conserv. Biol. 15, 749–760. doi: 10.1046/j.1523-1739.2001.015003749.x
Paul, S. R., and Bunch, T. D. (1978). Chronic frontal sinusitis and osteolysis in desert bighorn sheep. J. Amer. Vet. Med. Assoc. 173, 1178–1180.
Peek, J. M., Riggs, R. A., and Lauer, J. L. (1979). Evaluation of fall burning on bighorn sheep winter range. J. Extra-corp. Technol. 32, 430–432. doi: 10.2307/3898552
Pierce, B. M., and Bleich, V. C. (2003). “Mountain lion” in Wild Mammals of North America. eds. G. A. Feldhamer, B. C. Thompson, and J. A. Chapman Second ed (Baltimore: Johns Hopkins University Press), 744–757.
Pierce, B. M., and Bleich, V. C. (2014). Enumerating mountain lions: a comparison of two indices. Calif. Fish Game 100, 527–537.
Plowright, R. K., Manlove, K. R., Besser, T. E., Páez, D. J., Andrews, K. R., Matthews, P. E., et al. (2017). Age-specific infectious period shapes dynamics of pneumonia in bighorn sheep. Ecol. Lett. 20, 1325–1336. doi: 10.1111/ele.12829
Polak, T., and Saltz, D. (2011). Reintroduction as an ecosystem restoration technique. Conserv. Biol. 25, 424–425. doi: 10.1111/j.1523-1739.2011.01669.x
Purvis, A., Gittleman, J. L., Cowlishaw, G., and Mace, G. M. (2000). Predicting extinction risk in declining species. Proc. Biol. Sci. 267, 1947–1952. doi: 10.1098/rspb.2000.1234
Ramey, R. R. (1993b). A morphometric re-evaluation of the peninsular bighorn subspecies. Desert Bighorn Coun. Trans. 37, 1–10.
Ramey, R. R. (1993a). Evolutionary genetics and systematics of North American mountain sheep: Implications for conservation. Ph. D. Dissertation. Cornell University, Ithaca, New York.
Renaud, L. A., Festa-Bianchet, M., and Pelletier, F. (2022). Testing the match–mismatch hypothesis in bighorn sheep in the context of climate change. Glob. Change Biol. Bioenerg. 28, 21–32. doi: 10.1111/gcb.15923
Rezaei, H. R., Naderi, S., Chintauan-Marquier, I. C., Taberlet, P., Virk, A. T., Naghash, H. R., et al. (2010). Evolution and taxonomy of the wild species of the genus Ovis (Mammalia, Artiodactyla, Bovidae). Mol. Phylogenet. Evol. 54, 315–326. doi: 10.1016/j.ympev.2009.10.037
Ripple, W. J., Newsome, T. M., Wolf, C., Dirzo, R., Everatt, K. T., Galetti, M., et al. (2015). Collapse of the world’s largest herbivores. Sci. Adv. 1:e1400103. doi: 10.1126/sciadv.1400103
Risenhoover, K., and Bailey, J. (1980). Visibility: an important factor for an indigenous, low-elevation bighorn herd in Colorado. Bie. Symp. North. Wild Sheep Goat Coun. 2, 18–28.
Risenhoover, K. L., and Bailey, J. A. (1985). Foraging ecology of mountain sheep: implications for habitat management. J. Wildl. Manag. 49, 797–804. doi: 10.2307/3801714
Risenhoover, K. L., Bailey, J. A., and Wakelyn, L. A. (1988). Assessing the Rocky Mountain bighorn sheep management problem. Wildl. Soc. Bull. 16, 346–352.
Robinson, R. W., Whiting, J. C., Shannon, J. M., Olson, D. D., Flinders, J. T., Smith, T. S., et al. (2019). Habitat use and social mixing between groups of resident and augmented bighorn sheep. Sci. Rep. 9:14984. doi: 10.1038/s41598-019-51370-y
Rominger, E. M. (2018). The Gordian knot of mountain lion predation and bighorn sheep. J. Wild Manag. 82, 19–31. doi: 10.1002/jwmg.21396
Rominger, E. M., Whitlaw, H. A., Weybright, D. L., Dunn, W. C., and Ballard, W. B. (2004). The influence of mountain lion predation on bighorn sheep translocations. J. Wildl. Manag. 68, 993–999. doi: 10.2193/0022-541X(2004)068[0993:TIOMLP]2.0.CO;2
Rosenstock, S. S., Ballard, W. B., and Devos, J. C. (1999). Viewpoint: benefits and impacts of wildlife water developments. J. Extra-corp. Technol. 52, 302–311. doi: 10.2307/4003538
Ross, P. I., Jalkotzy, M. G., and Festa-Bianchet, M. (1997). Cougar predation on bighorn sheep in southwestern Alberta during winter. Can. J. Zool. 75, 771–775. doi: 10.1139/z97-098
Roy, J. L., and Irby, L. R. (1994). Augmentation of a bighorn sheep herd in southwest Montana. Wildl. Soc. Bull. 22, 470–478.
Rubin, E. S., and Bleich, V. C. (2005). “Sexual segregation: a necessary consideration in wildlife conservation” in Sexual Segregation in Vertebrates: Ecology of the Two Sexes. eds. K. E. Ruckstuhl and P. Neuhaus (Cambridge: Cambridge University Press), 379–391.
Rubin, E. S., Boyce, W. M., Jorgensen, M. C., Torres, S. G., Hayes, C. L., O'Brien, C. S., et al. (1998). Distribution and abundance of bighorn sheep in the peninsular ranges. Wildl. Soc. Bull. 26, 539–551.
Sandoval, A. V., Valdez, R., and Espinosa-T, A. (2019). “Desert bighorn sheep in Mexico” in Wildlife Ecology and Management in Mexico. eds. R. Valdez and J. Ortega-S (College Station, Texas: Texas A&M University Press), 350–365.
Schipper, J., Chanson, J. S., Chiozza, F., Cox, N. A., Hoffmann, M., Katariya, V., et al. (2008). The status of the world's land and marine mammals: diversity, threat, and knowledge. Science 322, 225–230. doi: 10.1126/science.1165115
Schommer, T. J., and Woolever, M. M. (2008). "A review of disease related conflicts between domestic sheep and goats and bighorn sheep", in: U S Forest Service Rocky Mountain Research Station general technical report RMRS-GTR-209 (Fort Collins: U. S. Department of Agriculture, Forest Service, Rocky Mountain Research Station).
Schroeder, C. A., Bowyer, R. T., Bleich, V. C., and Stephenson, T. R. (2010). Sexual segregation in Sierra Nevada bighorn sheep, Ovis canadensis sierrae: ramifications for conservation. Arct. Antarct. Alp. Res. 42, 476–489. doi: 10.1657/1938-4246-42.4.476
Schwartz, O. A., Bleich, V. C., and Holl, S. A. (1986). Genetics and the conservation of mountain sheep Ovis canadensis nelsoni. Biol. Conserv. 37, 179–190. doi: 10.1016/0006-3207(86)90090-X
Seddon, P. J. (2010). From reintroduction to assisted colonization: moving along the conservation translocation spectrum. Restor. Ecol. 18, 796–802. doi: 10.1111/j.1526-100X.2010.00724.x
Seip, D., and Bunnell, F. (1985). Nutrition of Stone's sheep on burned and unburned ranges. J. Wildl. Manag. 49, 397–405. doi: 10.2307/3801541
Shannon, J. M., Whiting, J. C., Larsen, R. T., Olson, D. D., Flinders, J. T., Smith, T. S., et al. (2014). Population response of reintroduced bighorn sheep after observed commingling with domestic sheep. Eur. J. Wildl. Res. 60, 737–748. doi: 10.1007/s10344-014-0843-y
Shillinger, J. (1937). Disease relationship of domestic stock and wildlife. Second North Am. Wildl. Con. 2, 298–302.
Shirkey, N., Roug, A., Besser, T. E., Bleich, V. C., Darby, N., Dekelaita, D., et al. (2021). Previously unrecognized exposure of desert bighorn sheep (Ovis canadensis nelsoni) to Mycoplasma ovipneumoniae in the California Mojave Desert. J. Wildl. Dis. 57, 447–452. doi: 10.7589/JWD-D-20-00098
Sinclair, A. R. E., Pech, R. P., Dickman, C. R., Hik, D., Mahon, P., and Newsome, A. E. (1998). Predicting effects of predation on conservation of endangered prey. Conserv. Biol. 12, 564–575. doi: 10.1046/j.1523-1739.1998.97030.x
Singer, F. J., Bleich, V. C., and Gudorf, M. A. (2000a). Restoration of bighorn sheep metapopulations in and near western National Parks. Restor. Ecol. 8, 14–24. doi: 10.1046/j.1526-100x.2000.80062.x
Singer, F. J., Papouchis, C. M., and Symonds, K. K. (2000b). Translocations as a tool for restoring populations of bighorn sheep. Restor. Ecol. 8, 6–13. doi: 10.1046/j.1526-100x.2000.80061.x
Singer, F. J., Williams, E., Miller, M. W., and Zeigenfuss, L. C. (2000c). Population growth, fecundity, and survivorship in recovering populations of bighorn sheep. Restor. Ecol. 8, 75–84. doi: 10.1046/j.1526-100x.2000.80067.x
Singer, F. J., Zeigenfuss, L. C., and Spicer, L. (2001). Role of patch size, disease, and movement in rapid extinction of bighorn sheep. Conserv. Biol. 15, 1347–1354. doi: 10.1046/j.1523-1739.2001.99488.x
Smith, T. S., Flinders, J. T., and Winn, D. S. (1991). A habitat evaluation procedure for Rocky Mountain bighorn sheep in the intermountain west. Great Basin Nat. 51, 205–225.
Smith, T. S., Hardin, P. J., and Flinders, J. T. (1999). Response of bighorn sheep to clear-cut logging and prescribed burning. Wildl. Soc. Bull. 27, 840–845.
Smith, J. B., Jenks, J. A., Grovenburg, T. W., and Klaver, R. W. (2014). Disease and predation: sorting out causes of a bighorn sheep (Ovis canadensis) decline. PLoS One 9:e88271. doi: 10.1371/journal.pone.0088271
Solberg, E. J., Loison, A., Ringsby, T. H., Saether, B. E., and Heim, M. (2002). Biased adult sex ratio can affect fecundity in primiparous moose Alces alces. Wildl. Biol. 8, 117–128. doi: 10.2981/wlb.2002.016
Spaan, R. (2022). Characterizing the spread and consequences of Mycoplasma ovipneumoniae on bighorn sheep (Ovis canadensis) in the northern basin and range ecosystem. Master’s Thesis. Corvallis, OR: Oregon State University.
Stephens, S. L., Moghaddas, J. J., Edminster, C., Fiedler, C. E., Haase, S., Harrington, M., et al. (2009). Fire treatment effects on vegetation structure, fuels, and potential fire severity in western US forests. Ecol. Appl. 19, 305–320. doi: 10.1890/07-1755.1
Taylor, G., Canessa, S., Clarke, R. H., Ingwersen, D., Armstrong, D. P., Seddon, P. J., et al. (2017). Is reintroduction biology an effective applied science? Ecol. Evol. 32, 873–880. doi: 10.1016/j.tree.2017.08.002
Terry, P. J., Alvidrez, A. C., and Black, C. W. (2022). Factors affecting bighorn sheep activity at water developments in southwestern Arizona. J. Wildl. Manag. 86:e22134. doi: 10.1002/jwmg.22134
Torres, S. G., Mulcahcy, G., Gonalez, B., Pauli, A., and Andrew, N. G. (2000). Human induced migration and homing behavior of a desert bighorn ram in the Whipple Mountains, California or, Herman, the trailer park ram. Desert Bighorn Coun. Trans. 44:13.
Trefethen, J. B., and Corbin, P. (1975). American Crusade for Wildlife. Winchester, Ontario, CA: Winchester Press.
Turner, J. C. (1973). Water, energy, and electrolyte balance in the desert bighorn sheep, Ovis canadensis. Dissertation. Riverside, California: University of California, Riverside.
Valdez, R. (1988). Wild Sheep and Wild Sheep Hunters of the New World. Mesilla, New Mexico: Wild Sheep and Goat International
Valdez, R. (2011). “Bighorn sheep” in Handbook of Mammals of the World. eds. D. E. Wilson and A. Mittermeier (Barcelona, Spain: Lynx Edicions)
Valdez, R. (2019). “Historical and conservation perspectives of wildlife in Mexico” in Wildlife Ecology and Management in Mexico. eds. R. Valdez and J. Ortega-S (College Station, Texas: Texas A&M University Press), 1–18.
Valdez, R., and Krausman, P. R. (1999). “Description, distribution, and abundance of mountain sheep in North America” in Mountain Sheep of North America. eds. R. Valdez and P. R. Krausman (Arizona: University of Arizona Press), 3–22.
Villepique, J. T., Pierce, B. M., Bleich, V. C., Andic, A., and Bowyer, R. T. (2015). Resource selection by an endangered ungulate: a test of predator-induced range abandonment. Arch. Gén. Méd. 2015:357080. doi: 10.1155/2015/357080
Villepique, J. T., Pierce, B. M., Bleich, V. C., and Bowyer, R. T. (2011). Diet of cougars (Puma concolor) following a decline in a population of mule deer (Odocoileus hemionus): lack of evidence for switching prey. Southwest. Nat. 56, 187–192. doi: 10.1894/F07-TAL.1
Vitousek, P. M., Mooney, H. A., Lubchenco, J., and Melillo, J. M. (1997). Human domination of Earth's ecosystems. Science 277, 494–499. doi: 10.1126/science.277.5325.494
Waits, L., and Epps, C. W. (2015). “Population Genetics and Wildlife Habitat” in Wildlife Habitat Conservation: Concepts, Challenges, and Solutions. eds. Michael L. Morrison and Heather A. Mathewson (Baltimore, USA: Johns Hopkins University Press), 63–83.
Wakelyn, L. A. (1984). Analysis and Comparison of Existing and Historic Bighorn Sheep Ranges in Colorado. Master’s thesis. Fort Collins, Colorado: Colorado State University
Wakelyn, L. A. (1987). Changing habitat conditions on bighorn sheep ranges in Colorado. J. Wildl. Manag. 51, 904–912. doi: 10.2307/3801758
Warren, E. R. (1910). The Mammals of Colorado: An Account of the Several Species Found Within the Boundaries of the State, Together with a Record of their Habits and of their Distribution. New York, New York: GP Putnam's Sons
Wehausen, J. D. (1996). Effects of mountain lion predation on bighorn sheep in the Sierra Nevada and Granite Mountains of California. Wildl. Soc. Bull. 24, 471–479.
Wehausen, J. D., Bleich, V. C., and Ramey, R. R. (2005). Correct nomenclature for Sierra Nevada bighorn sheep. Calif. Fish Game 91, 216–218.
Wehausen, J. D., Bleich, V. C., and Weaver, R. A. (1987). Mountain sheep in California: a historical perspective on 108 years of full protection. Trans. West. Sec. Wildl. Soc. 23, 65–74.
Wehausen, J. D., and Epps, C. W. (2021). Population extinction and conservation planning for desert bighorn sheep in California. Trans. Desert Bighorn Coun. 56, 54–74.
Wehausen, J. D., Kelley, S. T., and Ramey, R. R. (2011). Domestic sheep, bighorn sheep, and respiratory disease: a review of the experimental evidence. Calif. Fish Game 97, 7–24.
Wehausen, J. D., and Ramey, R. R. (1993). A morphometric reevaluation of the Pennisular bighorn subspecies. Desert Bighorn Coun. Trans. 37, 1–10.
Wehausen, J. D., and Ramey, R. R. (2000). Cranial morphometric and evolutionary relationships in the northern range of Ovis canadensis. J. Mammal. 81, 145–161. doi: 10.1644/1545-1542(2000)081<0145:CMAERI>2.0.CO;2
Werdel, T. J., Jenks, J. A., Besser, T. E., Kanta, J. T., Lehman, C. P., and Frink, T. J. (2020). Restoration of a bighorn sheep population impeded by Mycoplasma ovipneumoniae exposure. Restor. Ecol. 28, 387–395.
Whiting, J. C., Bleich, V. C., Bowyer, R. T., and Larsen, R. T. (2011a). “Water availability and bighorn sheep: life-history characteristics and persistence of populations” in Advances in Environmental Research. ed. J. A. Daniels (Nova Publishers, Inc.), 131–163.
Whiting, J. C., Bowyer, R. T., and Flinders, J. T. (2008). Young bighorn (Ovis canadensis) males: can they successfully woo females? Ethology 114, 32–41. doi: 10.1111/j.1439-0310.2007.01442.x
Whiting, J. C., Bowyer, R. T., Flinders, J. T., Bleich, V. C., and Kie, J. G. (2010a). Sexual segregation and use of water by bighorn sheep: implications for conservation. Anim. Conserv. 13, 541–548. doi: 10.1111/j.1469-1795.2010.00370.x
Whiting, J. C., Bowyer, R. T., Flinders, J. T., and Eggett, D. L. (2011b). Reintroduced bighorn sheep: fitness consequences of adjusting parturition to local environments. J. Mammal. 92, 213–220. doi: 10.1644/10-mamm-a-145.1
Whiting, J. C., Olson, D. D., Shannon, J. M., Bowyer, R. T., Klaver, R. W., and Flinders, J. T. (2012). Timing and synchrony of births in bighorn sheep: implications for reintroduction and conservation. Wildl. Res. 39, 565–572. doi: 10.1071/WR12059
Whiting, J. C., Stewart, K. M., Bowyer, R. T., and Flinders, J. T. (2010b). Reintroduced bighorn sheep: do females adjust maternal care to compensate for late-born young? Eur. J. Wildl. Res. 56, 349–357. doi: 10.1007/s10344-009-0323-y
Whittaker, D. G., Ostermann, S. D., and Boyce, W. M. (2004). Genetic variability of reintroduced California bighorn sheep in Oregon. J. Wildl. Manag. 68, 850–859. doi: 10.2193/0022-541X(2004)068[0850:GVORCB]2.0.CO;2
Wiedmann, B. P., and Sargeant, G. A. (2014). Ecotypic variation in recruitment of reintroduced bighorn sheep: implications for translocation. J. Wildl. Manag. 78, 394–401. doi: 10.1002/jwmg.669
Wielgus, R. B. (2017). Resource competition and apparent competition in declining mule deer (Odocoileus hemionus). Can. J. Zool. 95, 499–504. doi: 10.1139/cjz-2016-0109
Wilcox, B. A., and Murphy, D. D. (1985). Conservation strategy: the effects of fragmentation on extinction. Am. Nat. 125, 879–887. doi: 10.1086/284386
Wild Sheep Foundation (2022). Funding our mission. Available at: https://www.wildsheepfoundation.org/mission-and-programs/mission-funding (Accessed January 20, 2023).
Wild Sheep Foundation, and WAFWA Wild Sheep Working Group (2012). North American Bighorn Sheep Distribution. Available at: https://www.wildsheepfoundation.org/cache/DOC392016-06-01NABHSDISTRIBUTION1850vs1960vs2012.pdf?20160717115807
Wild Sheep Working Group (2012). Recommendations for domestic sheep and goat management in wild sheep habitat. Western Association of Fish and Wildlife Agencies.
Wild Sheep Working Group (2015). Records of wild sheep translocations—United States and Canada, 1922–present [online]. Boise, Idaho: Western Association of Fish and Wildlife Agencies. (Accessed October 12, 2020).
Williams, M. W., Losleben, M. V., and Hamann, H. B. (2002). Alpine areas in the Colorado front range as monitors of climate change and ecosystem response. Geogr. Rev. 92, 180–191. doi: 10.2307/4140969
Williamson, L. L. (1987). “Evolution of a landmark law” in Restoring American Wildlife 1937–1987. eds. H. Kallman, C. P. Agee, W. R. Goforth, and J. P. Linduska (Washington, DC: United States Department of the Interior, Fish and Wildlife Service), 1–17.
Zamora-Maldonado, H. C., Avila-Foucat, V. S., Sánchez-Sotomayor, V. G., and Lee, R. (2021). Social-ecological resilience modeling: water stress effects in the bighorn sheep management system in Baja California Sur, Mexico. Ecol. Complex. 45:100884. doi: 10.1016/j.ecocom.2020.100884
Keywords: bighorn sheep, mountain sheep, Ovis canadensis, reintroduction, translocation
Citation: Whiting JC, Bleich VC, Bowyer RT and Epps CW (2023) Restoration of bighorn sheep: History, successes, and remaining conservation issues. Front. Ecol. Evol. 11:1083350. doi: 10.3389/fevo.2023.1083350
Edited by:
Emiliano Lasagna, University of Perugia, ItalyReviewed by:
Hovirag Lancioni, University of Perugia, ItalyPerry S. Barboza, Texas A&M University, United States
Abdulmojeed Yakubu, Nasarawa State University, Nigeria
Copyright © 2023 Whiting, Bleich, Bowyer and Epps. This is an open-access article distributed under the terms of the Creative Commons Attribution License (CC BY). The use, distribution or reproduction in other forums is permitted, provided the original author(s) and the copyright owner(s) are credited and that the original publication in this journal is cited, in accordance with accepted academic practice. No use, distribution or reproduction is permitted which does not comply with these terms.
*Correspondence: Jericho C. Whiting, whitingj@byui.edu
†These authors have contributed equally to this work and share first authorship