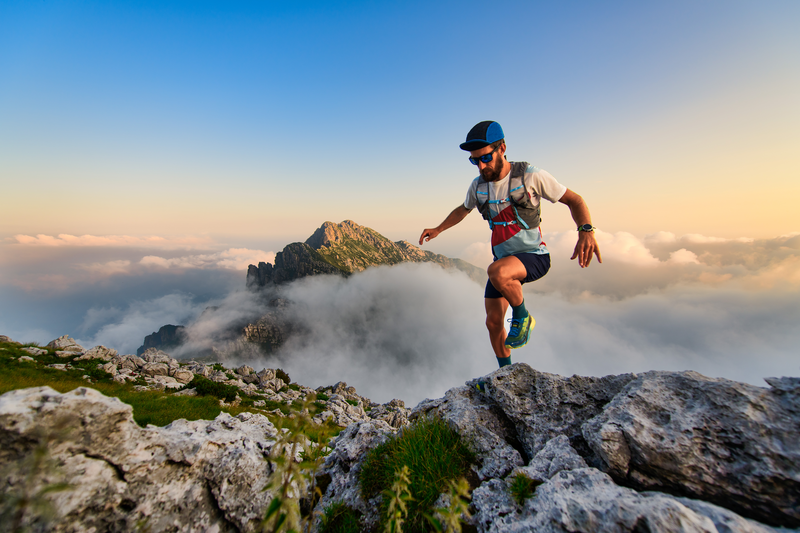
94% of researchers rate our articles as excellent or good
Learn more about the work of our research integrity team to safeguard the quality of each article we publish.
Find out more
ORIGINAL RESEARCH article
Front. Ecol. Evol. , 19 January 2023
Sec. Conservation and Restoration Ecology
Volume 11 - 2023 | https://doi.org/10.3389/fevo.2023.1082321
The mining of mineral resources has caused serious damage to the ecosystems of mining areas, resulting in the degradation of large areas of vegetation. In the Loess Plateau in particular, the ecological environment is fragile, and soil erosion is serious. It is urgent to restore vegetation and to improve the quality of the damaged environment. In the process of restoring the vegetation in the Pingshuo opencast mining area, this paper investigates the growth rates of species and the factors that affect these rates. The results showed that the maximum growth rate of Robinia pseudoacacia was 0.25 m/year in Mingled stand and 0.23 m/year in pure stand. The growth rate of R. pseudoacacia in Mingled stand was higher than that in pure stand, and the diversity indicators in the Mingled stand plots were higher than those in the pure stand plots. The stepwise regression analysis of the species diversity index, soil, and climate with the growth rate of the two plots showed that Margalef’s richness index had a significant effect on the growth rate of R. pseudoacacia in Mingled stands. This indicates that the R. pseudoacacia in our study area grows faster in mingled stand and that the Ulmus pumila and Ailanthus altissima that belong to the broad-leaved forest will grow together with R. pseudoacacia. The configuration pattern of broad-leaved pure stand is more singular than that of broad-leaved mingled stand, so the pure stand is not more suitable for vegetation restoration in the mining area.
Mining is considered to be one of the most aggressive human disturbances in the world to date, and the exploitation of mineral resources can destroy natural ecosystems on a massive scale, making them irreversible, among them, severe land degradation can occur following open-pit mining activities, negatively affecting the vegetation and soil quality of natural ecosystems (Hou X. et al., 2019; Hou X. Y. et al., 2019). In addition, the coordinated relationship between coal mining and ecological protection is not dealt with in the mining process, this can also lead to severe vegetation degradation, as degradation alters the material cycle in the mining ecosystem (Fu et al., 2016). At present, the restoration of open-pit mines mainly includes land reclamation (Yuan et al., 2022), ecotourism (Ashok et al., 2022), and vegetation restoration (Hui et al., 2021), among which vegetation restoration is a cost-effective and prospective green technology for the restoration of contaminated sites (Han et al., 2021). The Pingshuo open-pit mining area was one of the early adopters of vegetation restoration, and the vegetation that has been restored over the years has improved significantly (Liu et al., 2016).
Vegetation growth cannot be achieved without the influence of climate change, which is an important driving factor for vegetation change and provides the heat and moisture needed for vegetation growth (Li et al., 2019). Vegetation growth in an arid and semi-arid region, is very sensitive to changes in precipitation, and vegetation coverage improves significantly as annual precipitation increases (Zhang et al., 2012). Studies have shown that in some areas of the Loess Plateau, vegetation growth is positively affected when the average annual temperature is higher than 8°C and negatively affected when the temperature is lower than 8°C (Sun et al., 2015). Additionally, a contributing element to plant development in recent decades has been the Loess Plateau’s rising temperatures, which has extended the growing season of vegetation (Zhao et al., 2017; Ma et al., 2019).
In addition to climate change, soil conditions are one of the driving factors affecting vegetation growth. Mining areas are generally located harsh environments with low a nutrient content, and this inhibits vegetation growth (Ahirwal et al., 2017). Moreover, the success of vegetation restoration is highly dependent on the organic matter, available phosphorus, available potassium, and total nitrogen content of the soil (Noumi, 2015), because soil organic matter, the available potassium, and total nitrogen have significant effects on the growth and development of vegetation under vegetation growth and development conditions (An et al., 2014). Furthermore, nitrogen and phosphorus play an important role in plant growth and development, influencing the interaction between plant nitrogen and phosphorus and regulating plant growth with each other. Therefore, it is important to determine how soil parameters affect vegetation growth in the process of vegetation restoration (Stoorvogel et al., 2017).
In arid and semi-arid regions, species diversity has had a positive impact on soil protection and plant community stability, especially in the midst of climate change in recent decades (Alarcón et al., 2018). The China Coal Pingshuo open-pit coal mine is a typical area in the Loess Plateau region of China in which the ecosystem has been destroyed, and favorable modes of vegetation restoration include planting trees, shrubs, and grasses. Robinia pseudoacacia was first planted for vegetation restoration in the Loess Plateau region in the 1950s (Jiao et al., 2012). In general, the R. pseudoacacia grows very rapidly because they can photosynthesize more and use resources more efficiently, so they may affect coexisting native species (Fu et al., 2016). However, it has also been shown that while strong competitiveness allows R. pseudoacacia to suppress the growth of native species, it is also thought to benefit the growth of neighboring plants and achieve symbiosis due to its nitrogen fixation capacity (Von Holle et al., 2005; Hellmann et al., 2011).
However, many of the current studies on ecological restoration in mining areas are conducted in the short term, without long-term observation and monitoring, and there are few studies on the changes in vegetation and the impact on the environment, so it is essential that the impact of mining activities on the ecosystem in mining areas be studied in a long-term and dynamic manner and that the process and patterns of ecological restoration be scientifically assessed via long-term monitoring (Dong et al., 2019). The establishment of economically viable permanent vegetation coverage on abandoned soil from mines through reclamation or ecological restoration is an important solution (Dutta and Agrawal, 2003). The long-term positioning and monitoring of the ecological restoration effect of the site vegetation are of great practical significance to solve the current problem of the ecological reconstruction of the damaged land in the mine. Moreover, the dynamic changes in vegetation are influenced by various biotic and abiotic factors, so it is especially important to follow up on the vegetation at each stage of the ecological restoration process of the mine site through long-term positioning monitoring, especially from the early stage of reclamation, and to apply ecological principles for scientific plant allocation and process management to artificially induce positive plant community succession. So we have established a permanent fixed monitoring sample site within the Pingshuo open-pit coal mine dumps to monitor changes in various indicators such as vegetation and soil since the land reclamation process began. The primary issue mentioned in this study is the maximum growth rate and time required to achieve the maximum growth rate of R. pseudoacacia under various vegetation configuration modes as well as the factors that influence the maximum growth rate of R. pseudoacacia. To determine the final influencing factors, soil, species diversity, temperature, and precipitation are analyzed. In this paper, two sample plots with different configuration patterns, a R. pseudoacacia–Ulmus pumila (U. pumila)–Ailanthus altissima (A. altissima) broadleaf Mingled stand and a R. pseudoacacia broadleaf pure stand, were selected from the dumping site of the Pingshuo open-pit coal mine reclamation area. Our hypothesis is that soil and climate factors as well as diversity will jointly affect the growth of R. pseudoacacia in plantations.
The Pingshuo mine is located along the border area between Jin, Shaanxi, and Mongolia on the Loess Plateau, in the northern part of Shanxi Province, in the Pinglu District of Shuozhou City, with the geographical coordinates of 112°10′–113°30′ East and 39°23′–39°37′ North (Figure 1). The mine is located in the northern part of the Ningwu coalfield and is bounded on the east, west, and north by coal seam outcrops and on the south by the Danshuigou fault, which spans 21 km from north to south and is 22 km wide from east to west, covering an area of 380 km2, with a geological reserve of 12.75 billion tons. The area where the Antaibao open-pit coal mine is located belongs to the low hills of the Loess Plateau in Shanxi, and the whole area is mostly covered by loess. The loess plateau in the area was once subject to strong erosion and cutting. Coupled with the sparse vegetation in the area, the terrain is variable from the highlands to the river valley, with large changes in height. The highest point is 1,505.72 m, the lowest point is 1,270 m, and the relative height difference is 235.72 m. The continental monsoon climate in the mining area is extremely typical, with cool summers, strong winds in spring and winter, severe sand, and dry and cold weather as its characteristics. The temperature is generally low, characterized by a large annual temperature difference and daily temperature difference, with an average annual temperature of 5.4–13.8°C. The longest sunshine period in the mining area is 2,883.1 h, the shortest annual period is 2,444.5 h, and the average is 2,693.3 h. The annual precipitation distribution is extremely uneven, and the intensity of heavy rainfall is high. The annual rainfall is 345.3–682.2 mm. Due to the long history of development and the high reclamation index, natural secondary forests have been destroyed, and large grassland communities are rarely seen but are scattered and have low vegetation cover, so the current agricultural landscape is generally appropriate for farming. The zone soil in this area is the transition zone of chestnut soil and chestnut cinnamon soil. Drought-tolerant plants such as Stipa bungcana, Stipa krylovii, Agropyron cristatum, Thymus mongolicus, and Lespedeza davurica are widely distributed in the area. The main zone soil is chestnut soil, which is distributed in alluvial plain. Most of the weathering products are granite and gneiss. Thus, the physical weathering of the soil is strong. The soil is sandy, arid, well-ventilated (Zhao et al., 2018).
The south dump site of the Antaibao open-pit coal mine started land reclamation in 1993, with an early reclamation time, various configuration patterns, and significant reclamation effects. Together, these factors have formed a biodiversity-rich vegetation restoration area that basically covers the original bare surface of the dump site, with vegetation cover reaching over 90%. For this reason, a fixed monitoring sample site was set up in the south dump site of the Antaibao open-pit coal mine.
The study area is located within the south dump of the Antaibao open-pit mine in the Pingshuo mining area. The height of R. pseudoacacia seedlings was uniformly 0.3 m when reclamation began in 1993. The vegetation had been severely damaged after mining (Figures 2A, B). Additionally, the sample site was constructed in 2010, and all vegetation in the sample site was surveyed in both 2015 and 2020. We selected two plots of 1 hm2 (100 × 100 m) of flat land, one of which was a mingled R. pseudoacacia–U. pumila–A. altissima broadleaf forest and the other with a pure R. pseudoacacia broadleaf forest (Figures 2C, D), with the same planting density in both plots (Table 1). We selected R. pseudoacacia species that were still alive in 2020 and over 1.3 m in height and selected the same species in 2010 and 2015 according to the tree number of each surviving R. pseudoacacia. Other species were also counted (Table 2). Additionally, we divided the 1 hm2 plots into 100 quadrats of 10 m × 10 m (Figure 3), the maximum growth rate of all R. pseudoacacia within each 10 m × 10 m sample plot was calculated, and the two plots were compared. The height of the trees was measured using altimetry poles, and both plots were 1 hm2, that is, each plot was 100 m × 100 m.
Figure 2. Vegetation types before and after restoration. (A) Original vegetation; (B) damaged vegetation; (C) mingled stand; (D) pure stand.
Figure 3. Sample division and working order diagram. The left figure shows the schematic diagram of sample number, 0 is the origin, each small square is 10 m × 10 m, and the total is 100 m × 100 m. The right figure shows the schematic diagram of work order for each 10 m × 10 m sample.
To evaluate species variety, measures like the Pielou evenness index and the Patrick richness index are frequently utilized. The diversity index is determined by the number of species in a sample of a specific size according to the Margalef richness index, which also takes into account the total number of members in the community. The Simpson Ecological Diversity Index has been used previously to evaluate economic inequality. The Shannon (or Shannon Weiner) diversity index was originally a measure of entropy, which was then used in information theory. In this manuscript, the species diversity in the two sample sites surveyed was calculated as follows (Song, 2004):
S is the total number of species, N is the total number of individuals of each species, InS is the natural logarithm of species, InN is the natural logarithm of individuals, and pi denotes the proportion of individuals of the ith species to all individuals.
The soil in the study area was severely damaged after mining (Figure 4). This manuscript refers to the CTFS (Center for Tropical Forest Science) soil sampling plan and the actual situation of the study area, the sample area was divided into nine 30 m ×30 m grids, and the node of each grid was used as a reference point for sampling. Then one of the eight directions from each reference point was randomly selected, and two places within this direction were randomly selected at 2, 5, or 15 m for additional sampling. Thus, a total of 96 sampling points were set up in this study (Figure 5).
Three soil samples at depths of 0–10 cm (auger diameter of 5 cm) were collected with a soil auger at a distance of 20 cm around the sampling points designed above, and these three soil samples were mingled and used as samples (500 g each) for that sampling point. The obtained soil samples are dried in an oven at 105°C for 48 h before testing, and then stones larger than 2 mm were removed from the dried soil (using a 2 mm sieve). The soil indicators tested in this study included pH, soil organic matter (SOM), total nitrogen (TN), available phosphorus (AP), and available potassium (AK)(Shi et al., 2021). The 48 soil sampling data from the two sample plots were interpolated separately, and five soil factors from the 48 points were interpolated into each 10 m × 10 m small sample square using the kriging method, with soil sampling values for each 10 m × 10 m small sample square.
The analysis in this paper uses temperature and precipitation data for Pinglu District, Shuozhou City, Shanxi Province, from the China Meteorological Data Service Centre.1
In this paper, we used a three-parameter logistic function to describe the growth dynamics across growing seasons, the “stats” and “deSolve” packages were employed for the growth rates (Paine et al., 2012). The data were processed and analyzed using SPSS 20.0, ANOVA was used to analyze the significance of differences in growth rates of different pairs of R. pseudoacacia, and the significance of differences test with Bonferroni (p < 0.05). Correlation analysis was done using the “corrplot” package in Matlab 2020. Stepwise regression was used to analyze the final influencing factors.
In this manusript, the growth rates of R. pseudoacacia in the two sample plots were subjected to ANOVA to compare whether the results were significantly different.
According to the results of the ANOVA (Figure 6), the significance p < 0.01 indicates that there is a significant difference in the comparison between the two sample sites, and the means of the two groups are significantly unequal. The maximum growth rate of R. pseudoacacia was 0.25 m/year in the Mingled stand and 0.23 m/year in the pure stand. The maximum growth rate of R. pseudoacacia was higher in the Mingled stand sample than in the pure stand sample, and the maximum growth rate was reached earlier in the Mingled stand sample than in the pure stand sample, but the rapid growth of R. pseudoacacia in both samples ended in 2012 and 2014 (Table 3). The mine site studied in this paper was reclaimed in 1993, and the maximum growth rate was reached in 2006 and 2007, which means that R. pseudoacacia reached its maximum growth rate after 13 years in the Mingled stand and the rapid growth stage in years 7–19. In the pure stand, the maximum growth rate was reached in the 14th year and the rapid growth stage in years 8–21.
The diversity indices calculated in this manuscript included all trees and shrubs in the sample plots, and five commonly used diversity indices were selected for each species in the two sample plots that were surveyed.
In the main species diversity results (Figure 7), the Mingled stand sample plots contained the main three species, including R. pseudoacacia, U. pumila, and A. altissima, and the pure stand sample plots included R. pseudoacacia and U. pumila. The U. pumila in the pure stand sample plots were invasive species. In the total species diversity results (Figure 8), we combined all trees and shrubs in the two sample plots, with nine species in the Mingled stand sample plot and three species in the pure stand sample plot. It was evident that Margalef index (M), Simpson index (D), and Shannon–Wiener index (H’) increased as the Patrick index (S) increased, and the Pielou index (J) decreased as the Patrick index (S) increased in both sample plots, and all of the diversity indices within the Mingled stand sample plots were much higher than those in the pure stand sample plots.
Figure 7. Major species diversity. The blue is the Mingled stand diversity index, and the red is the pure forest diversity index. It includes Patrick index, Margalef’s index, Simpson’s index, Shannon–Wiener index, Pielou’s index.
Figure 8. Total species diversity. The blue is the Mingled stand diversity index, and the red is the pure forest diversity index. It includes Patrick index, Margalef’s index, Simpson’s index, Shannon–Wiener index, Pielou’s index.
We correlated the diversity indices, soil factors, and climatic data (temperature and precipitation) with the growth rates (Figures 9, 10) and found no correlation (p > 0.05) between the diversity indices and growth rates for all five species in the two sample plots. Species richness was significantly correlated with species diversity M, D, and H′ (p < 0.01) but not with evenness in Mingled stand samples, while species richness was significantly correlated with species diversity M, D, and H′ as well as evenness (p < 0.01) in pure stand samples, which also indicates that the increase in species affects the species evenness. All five soil factors in the Mingled stand sample were not correlated with R (p > 0.05), while in the pure stand sample, four of the soil factors (SOM, TN, AP, and AK) were not correlated with R (p > 0.05). However, soil pH and R were significantly correlated (p < 0.01), indicating that soil pH affects the growth rate of R. pseudoacacia in the pure stand sample. The results in Table 1 show the time range of rapid growth of R. pseudoacacia, and we used the average monthly temperature and precipitation in this range to analyze whether R was related to the temperature and precipitation in it. From the results, it can be seen that the growth rate of R. pseudoacacia was not correlated with temperature and precipitation in either the mingled or pure stands (p > 0.05).
Figure 9. Correlation matrix between R. pseudoacacia growth rate and different properties in mingled stands. Blue color indicates positive correlation and red color indicates negative correlation. Precipitation (mm); Temperature (°C); pH; SOM (g/kg); TN (g/kg); AP (mg/kg); AK (mg/kg). The closer the graph is to a straight line (that is, closer to 1 or –1), the stronger the correlation.
Figure 10. Correlation matrix between R. pseudoacacia growth rate and different properties in pure stands. Blue color indicates positive correlation and red color indicates negative correlation. Precipitation (mm); Temperature (°C); pH; SOM (g/kg); TN (g/kg); AP (mg/kg); AK (mg/kg). The closer the graph is to a straight line (that is, closer to 1 or –1), the stronger the correlation.
Through correlation analysis we can see that only pH is significantly correlated with R. pseudoacacia in the pure stand. This is because correlation analysis only considers the relationship between the values of two variables. Stepwise regression is based on the consideration of multiple variables and controls for the effects of a variable after controlling for the other variables. Therefore, we took the five diversity indices calculated in each of the two sample plots, the five soil indicators after interpolation, and the temperature and precipitation data for the Pinglu district and performed stepwise regression analysis. The independent variable was the 12 indices, and the dependent variable was the growth rate of R. pseudoacacia. We analyzed which indices had significant effects on the growth rate of R. pseudoacacia in the two sample plots separately.
As seen in the results of the above analysis (Tables 4, 5), VIF < 10. The data are consistent with the assumptions of the multiple stepwise regression analysis, and there is no multicollinearity problem to be analyzed. Two models in the Mingled stand entered the final stage: the Margalef index of species diversity and nitrogen in the soil, with two-factor coefficients of p < 0.05. The two indicators explained 65.9% of the total indicators, indicating that the factors affecting the maximum growth rate of R. pseudoacacia within the Mingled stand sample plots were M and N, which together influenced the growth rate of R. pseudoacacia within the Mingled stand sample plots. Only one model in the pure stand entered the final stage, with a precipitation coefficient p < 0.05, and the precipitation index explained 61.8% of the total index, indicating that the only factor affecting the maximum growth rate of R. pseudoacacia within the pure stand sample site was precipitation.
The selection of artificially planted species in mining sites for vegetation restoration was done in this article. Since natural vegetation restoration takes decades or even centuries, artificial vegetation restoration is essential (Shoo et al., 2016). For artificially introduced plants, it is essential to select species that are well adapted to the local environment (Tordoff et al., 2000), including having characteristics that are well adapted to nutrient-poor soils, high biomass, and rapid growth, such as Populus alba var. pyramidalis and Astragalus adsurgens (Lei et al., 2016). R. pseudoacacia forests are an important part of China’s boreal broadleaf forests, in our results, R. pseudoacacia in both plots ended its growth peak about 20 years after planting, because they are a fast-growing species and will have a high growth peak before the age of 20 due to its fast growth characteristics (Nicolescu et al., 2020).
As a major factor in determining the duration of vegetation growing seasons, temperature has been confirmed by many studies (Zhou, 2003; Zhao et al., 2019). Our results did not find that temperature can affect the growth of R. pseudoacacia, which should be due to the fact that our study area is located in the Loess Plateau, the effect of precipitation in the growing season on vegetation growth is more important than that of temperature (Zhang et al., 2019), and the current afforestation in China often takes place where the rainfall is close to that of minimum of species survival and growth. We also found that despite the two plots receiving the same amount of precipitation, only the precipitation in the pure stand plot affected the growth rate of R. pseudoacacia. The data in this research do not entirely support the concept that climate can influence R. pseudoacacia growth, this is because the interspecific competition in mingled stand with complementary features is much lower than that in pure stand (Kelty, 2006). Another limitation was the fact that the point data (precipitation and temperature) was interpolated, which may cause errors (Bier and de Souza, 2017).
In addition to climate, the impact of soil on vegetation restoration cannot be ignored (Liu et al., 2018). Our results found that the nitrogen in the soil of Mingled stand plots limited the growth of R. pseudoacacia and affected the growth rate of R. pseudoacacia, this is because Nitrogen is an essential nutrient for plants in soil and a key element for the growth of most forest plants (Laliberté et al., 2012). Some scholars also believe that phosphorus is one of the key substances required for plant growth and metabolism (Wagh and Sayyed, 2013), and adequate potassium also allows rapid plant growth. However, only nitrogen was found to affect the growth of R. pseudoacacia in our results, and this usually due to soil organic matter-related nutrient deficiencies (Ussiri and Lal, 2005), this may also be due to increased inter-species diversity and changes in precipitation reducing the content between the P and K available in the soil for species growth.
In this study, one of the two sample plots was a mingled forest and the other was a pure forest, although the invasive species U. pumila was found in the pure forest sample plots, the number was sparse and insufficient to affect the growth and development of R. pseudoacacia. The U. pumila is also a light-loving species, dependent on light, drought, and infertile, and grows very quickly (Zhao et al., 2012), it is also very adaptable to different climates. Research has shown that planting R. pseudoacacia mingled forests on the Loess Plateau, especially with a planting ratio of two R. pseudoacacia - one U. pumila (2R1U), can improve the water use efficiency of R. pseudoacacia, and the mingled forest of R. pseudoacacia and U. pumila can effectively improve photosynthetic capacity (Qin et al., 2016). Some studies prove that A. altissima and R. pseudoacacia can co-exist (Call and Nilsen, 2003). The above ratios were not planted in this study, but our results also suggest that R. pseudoacacia can grow synergistically with U. pumila and A. altissima. Moreover, we found that R. pseudoacacia grows faster in mingled forests than pure forests, which is due to the higher species diversity of mingled forests and the richer flora of mingled forests than pure forests, thus providing more abundant resources diversity. This suggests that interspecific competition with complementary characteristics is much lower than the intraspecific competition (Kelty, 2006). And under similar environmental conditions, R. pseudoacacia pure forests resulted in the loss of plant richness and changes in species composition. This is in line with the paper’s hypothesis that specific variety may have an impact on R. pseudoacacia growth.
Through the analysis of different vegetation configuration patterns of mine dumping sites, it is important to explore the optimal configuration pattern of vegetation construction in the process of mine vegetation restoration, which is important for mine vegetation restoration and ecological reconstruction. Population, as a basic biological level, is both the basic unit that constitutes a biome and the basic unit that makes up a species as well as the key level for studying the effects of vegetation restoration. The study of plant populations is of great importance in the study of all aspects of community ecology, such as dynamics, succession, diversity, and stability. Key species groups play a direct or indirect role in regulating the distribution and abundance of other species, determining the stability of vegetation restoration communities, species diversity, and the persistence or modification of many ecological processes. In this paper, we show that the growth rate of species cannot be related to any single factor but that it depends on the interaction of several factors through studying R. pseudoacacia populations in mine dump sites under different configuration patterns. Additionally, in the process, we found that a single type of tree configuration in artificial vegetation is not the best choice because in this case, the selected plants were more dependent on precipitation, resulting in the plant richness and diversity in these communities being lower.
In this paper, the growth rate of R. pseudoacacia and its influencing factors were analyzed in combination with species diversity, soil, climate, and other factors. The results showed that R. pseudoacacia grows faster in mingled broad-leaved forests because U. pumila and A. altissima will grow with R. pseudoacacia, while broad-leaved forests will complement the same species as broad-leaved forests. This is because species richness has a greater effect on the growth of R. pseudoacacia than other factors, and the increase in species can increase the growth rate of R. pseudoacacia. That is to say since species richness is the primary influencing factor in this study area, it can have a significant impact on the growth rate of R. pseudoacacia. It can be seen from the results that the rapid growth of the Mingled stand with R. pseudoacacia started in the seventh year. Therefore, planting 7-year-old R. pseudoacacia in the mining area directly to achieve restoration should be considered. Additionally, it is best to use a broad-leaved Mingled stand with species that can grow synergistically with each other. Therefore, the analysis of vegetation development in mine reclamation sites can provide a good reference for future reclamation actions. However, the research scope of this paper is limited. Only Mingled stand with R. pseudoacacia–U. pumila–A. altissima and pure R. pseudoacacia forest were analyzed. Other broad-leaved forests are not included, and the performance of R. pseudoacacia in mingled coniferous and broad-leaved forest is unknown. The research area in this paper is flat and has the same density. It is necessary to analyze and verify different site conditions and different planting densities.
The data analyzed in this study is subject to the following licenses/restrictions: The data presented in this study are available on request from the corresponding author. Requests to access these datasets should be directed to ZB, YmFpemtAY3VnYi5lZHUuY24=.
ZS and MC: conceptualization. ZS and SL: methodology and investigation. ZS: validation, formal analysis, writing—original draft preparation, and writing—review and editing. ZB and DG: data curation. ZS and XM: visualization. ZB: project administration and funding acquisition. All authors have read and agreed to the published version of the manuscript.
This study was funded by the National Natural Science Foundation of China (Nos. U1810107 and 41701607) and Shanxi basic research program (No. 202103021223129).
We thank Zhongke Bai, Donggang Guo, and Shuai Li for their suggestions during the preparation of the manuscript.
The authors declare that the research was conducted in the absence of any commercial or financial relationships that could be construed as a potential conflict of interest.
All claims expressed in this article are solely those of the authors and do not necessarily represent those of their affiliated organizations, or those of the publisher, the editors and the reviewers. Any product that may be evaluated in this article, or claim that may be made by its manufacturer, is not guaranteed or endorsed by the publisher.
Ahirwal, J., Maiti, S. K., and Satyanarayana Reddy, M. (2017). Development of carbon, nitrogen and phosphate stocks of reclaimed coal mine soil within 8 years after forestation with Prosopis juliflora (Sw.) Dc. Catena 156, 42–50. doi: 10.1016/j.catena.2017.03.019
Alarcón, R., Hernández-Plaza, E., Navarrete, L., Sánchez, M. J., Escudero, A., Hernanz, J. L., et al. (2018). Effects of no-tillage and non-inversion tillage on weed community diversity and crop yield over nine years in a Mediterranean cereal-legume cropland. Soil Tillage Res. 179, 54–62. doi: 10.1016/j.still.2018.01.014
An, P., Li, X., Zheng, Y., Eneji, A. E., Qiman, Y., Zheng, M., et al. (2014). Distribution of plant species and species-soil relationship in the east central gurbantunggut desert China. J. Geogr. Sci. 25, 101–112. doi: 10.1007/s11442-015-1156-0
Ashok, S., Behera, M. D., Tewari, H. R., and Jana, C. (2022). Developing ecotourism sustainability maximization (ESM) model: A safe minimum standard for climate change mitigation in the Indian Himalayas. Environ. Monit. Assess. 194:914. doi: 10.1007/s10661-022-10548-0
Bier, V. A., and de Souza, E. G. (2017). Interpolation selection index for delineation of thematic maps. Comput. Electron. Agric. 136, 202–209. doi: 10.1016/j.compag.2017.03.008
Call, L. J., and Nilsen, E. T. (2003). Analysis of spatial patterns and spatial association between the invasive tree-of-heaven (Ailanthus altissima) and the native black locust (Robinia pseudoacacia). Am. Midl. Nat. 150, 1–14. doi: 10.1674/0003-0031(2003)150[0001:AOSPAS]2.0.CO;2
Dong, J., Meng, L., Bian, Z., and Fang, A. (2019). Investigating the characteristics, evolution and restoration modes of mining area ecosystems. Pol. J. Environ. Stud. 28, 3539–3549. doi: 10.15244/pjoes/97390
Dutta, R. K., and Agrawal, M. (2003). Restoration of opencast coal mine spoil by planting exotic tree species: A case study in dry tropical region. Ecol. Eng. 21, 143–151. doi: 10.1016/j.ecoleng.2003.10.002
Fu, X., Ma, M., Jiang, P., and Quan, Y. (2016). Spatiotemporal vegetation dynamics and their influence factors at a large coal-fired power plant in Xilinhot, inner Mongolia. Int. J. Sustain. Dev. World Ecol. 24, 433–438. doi: 10.1080/13504509.2016.1273265
Han, Q., Ding, Y., and Peng, S. (2021). Sustainable and cost-effective vegetation restoration framework under climate change. For. Ecol. Manag. 496:119436. doi: 10.1016/j.foreco.2021.119436
Hellmann, C., Sutter, R., Rascher, K. G., Máguas, C., Correia, O., and Werner, C. (2011). Impact of an exotic N2-fixing Acacia on composition and N status of a native Mediterranean community. Acta Oecol. 37, 43–50. doi: 10.1016/j.actao.2010.11.005
Hou, X.-Y., Liu, S.-L., Cheng, F.-Y., Zhang, Y.-Q., Dong, S.-K., Su, X.-K., et al. (2019). Vegetation community composition along disturbance gradients of four typical open-pit mines in Yunnan province of southwest China. Land Degrad. Dev. 30, 437–447. doi: 10.1002/ldr.3234
Hou, X., Liu, S., Zhao, S., Dong, S., Sun, Y., and Beazley, R. (2019). The alpine meadow around the mining areas on the Qinghai-Tibetan plateau will degenerate as a result of the change of dominant species under the disturbance of open-pit mining. Environ. Pollut. 254:113111. doi: 10.1016/j.envpol.2019.113111
Hui, J., Bai, Z., Ye, B., and Wang, Z. (2021). Remote sensing monitoring and evaluation of vegetation restoration in grassland mining areas—a case study of the Shengli mining area in Xilinhot city, China. Land 10:743. doi: 10.3390/land10070743
Jiao, J., Zhang, Z., Bai, W., Jia, Y., and Wang, N. (2012). Assessing the ecological success of restoration by afforestation on the Chinese Loess Plateau. Restor. Ecol. 20, 240–249. doi: 10.1111/j.1526-100X.2010.00756.x
Kelty, M. J. (2006). The role of species mixtures in plantation forestry. For. Ecol. Manag. 233, 195–204. doi: 10.1016/j.foreco.2006.05.011
Laliberté, E., Turner, B. L., Costes, T., Pearse, S. J., Wyrwoll, K.-H., Zemunik, G., et al. (2012). Experimental assessment of nutrient limitation along a 2-million-year dune chronosequence in the south-western Australia biodiversity hotspot. J. Ecol. 100, 631–642. doi: 10.1111/j.1365-2745.2012.01962.x
Lei, H., Peng, Z., Yigang, H., and Yang, Z. (2016). Vegetation and soil restoration in refuse dumps from open pit coal mines. Ecol. Eng. 94, 638–646. doi: 10.3390/ijerph17061975
Li, G., Sun, S., Han, J., Yan, J., Liu, W., Wei, Y., et al. (2019). Impacts of Chinese grain for green program and climate change on vegetation in the Loess Plateau during 1982-2015. Sci. Total Environ. 660, 177–187.
Liu, X., Yang, T., Wang, Q., Huang, F., and Li, L. (2018). Dynamics of soil carbon and nitrogen stocks after afforestation in arid and semi-arid regions: A meta-analysis. Sci. Total Environ. 618, 1658–1664. doi: 10.1016/j.scitotenv.2017.10.009
Liu, X., Zhou, W., and Bai, Z. (2016). Vegetation coverage change and stability in large open-pit coal mine dumps in China during 1990–2015. Ecol. Eng. 95, 447–451. doi: 10.1016/j.ecoleng.2016.06.051
Ma, Z., Yan, N., Wu, B., Stein, A., Zhu, W., and Zeng, H. (2019). Variation in actual evapotranspiration following changes in climate and vegetation cover during an ecological restoration period (2000-2015) in the Loess Plateau, China. Sci. Total Environ. 689, 534–545. doi: 10.1016/j.scitotenv.2019.06.155
Nicolescu, V.-N., Rédei, K., Mason, W. L., Vor, T., Pöetzelsberger, E., Bastien, J.-C., et al. (2020). Ecology, growth and management of black locust (Robinia pseudoacacia L.), a non-native species integrated into European forests. J. For. Res. 31, 1081–1101. doi: 10.1007/s11676-020-01116-8
Noumi, Z. (2015). Effects of exotic and endogenous shrubs on understory vegetation and soil nutrients in the south of Tunisia. J. Arid Land 7, 481–487. doi: 10.1007/s40333-015-0047-7
Paine, C. E. T., Marthews, T. R., Vogt, D. R., Purves, D., Rees, M., Hector, A., et al. (2012). How to fit nonlinear plant growth models and calculate growth rates: An update for ecologists. Methods Ecol. Evol. 3, 245–256. doi: 10.1111/j.2041-210X.2011.00155.x
Qin, J., Xi, W., Rahmlow, A., Kong, H., Zhang, Z., and Shangguan, Z. (2016). Effects of forest plantation types on leaf traits of Ulmus pumila and Robinia pseudoacacia on the Loess Plateau, China. Ecol. Eng. 97, 416–425. doi: 10.1016/j.ecoleng.2016.10.038
Shi, Z. Y., Bai, Z. K., Guo, D. G., and Chen, M. J. (2021). Develop a soil quality index to study the results of black locust on soil quality below different allocation patterns. Land 10:785. doi: 10.3390/land10080785
Shoo, L. P., Freebody, K., Kanowski, J., and Catterall, C. P. (2016). Slow recovery of tropical old-field rainforest regrowth and the value and limitations of active restoration. Conserv. Biol. 30, 121–132. doi: 10.1111/cobi.12606
Song, D. Q. (2004). The basic conception and mathematic methods of biodiversity. J. Jinling Inst. Technol. 2, 1–4.
Stoorvogel, J. J., Bakkenes, M., Brink, B. J. E., and Temme, A. J. A. M. (2017). To what extent did we change our soils? A global comparison of natural and current conditions. Land Degrad. Dev. 28, 1982–1991.
Sun, W., Song, X., Mu, X., Gao, P., Wang, F., and Zhao, G. (2015). Spatiotemporal vegetation cover variations associated with climate change and ecological restoration in the Loess Plateau. Agric. For. Meteorol. 20, 87–99.
Tordoff, G. M., Baker, A. J. M., and Willis, A. J. (2000). Current approaches to the revegetation and reclamation of metalliferous mine wastes. Chemosphere 41, 219–228. doi: 10.1016/s0045-6535(99)00414-2
Ussiri, D. A. N., and Lal, R. (2005). Carbon sequestration in reclaimed minesoils. Crit. Rev. Plant Sci. 24, 151–165.
Von Holle, B., Joseph, K. A., Largay, E. F., and Lohnes, R. G. (2005). Facilitations between the introduced nitrogen-fixing tree, Robinia pseudoacacia, and nonnative plant species in the glacial outwash upland ecosystem of Cape Cod, MA. Biodivers. Conserv. 15, 2197–2215.
Wagh, G. S., and Sayyed, M. R. G. (2013). Assessment of macro and micronutrients in soils from Panvel Area, Maharashtra, India. Univ. J. Environ. Res. Technol. 3, 72–78.
Yuan, Y., Zhao, Y., Gao, Y., Gao, G., Ren, Y., and Hou, F. (2022). The effect of tree species on soil organic carbon recovery in a restoration project is associated with vegetation biomass: Evidence from the pingshuo mine reclaimed ecosystem, North China. Land Degrad. Dev. 33, 3870–3881.
Zhang, B., Wu, P., Zhao, X., Wang, Y., and Gao, X. (2012). Changes in vegetation condition in areas with different gradients (1980–2010) on the Loess Plateau, China. Environ. Earth Sci. 68, 2427–2438.
Zhang, Y., Liang, W., Liao, Z., Han, Z., Xu, X., Jiao, R., et al. (2019). Effects of climate change on lake area and vegetation cover over the past 55 years in Northeast Inner Mongolia Grassland, China. Theor. Appl. Climatol. 138, 13–25.
Zhao, A., Zhang, A., Lu, C., Wang, D., Wang, H., and Liu, H. (2017). Spatiotemporal variation of vegetation coverage before and after implementation of grain for green program in Loess Plateau, China. Ecol. Eng. 104, 13–22.
Zhao, B. Q., Guo, D. G., Bai, Z. K., and Zhao, Z. Q. (2018). Community dynamics of artificial vegetation in a reclaimed spoil from a semi-arid open-cast coal mine in 2010-2015. Chin. J. Ecol. 37, 1636–1644.
Zhao, J., Huang, S., Huang, Q., Wang, H., Leng, G., Peng, J., et al. (2019). Copula-based abrupt variations detection in the relationship of seasonal vegetation-climate in the Jing River Basin, China. Remote Sens. 11:1628.
Zhao, Z., Bai, Z., Zhang, Z., Guo, D., Li, J., Xu, Z., et al. (2012). Population structure and spatial distributions patterns of 17 years old plantation in a reclaimed spoil of Pingshuo opencast mine, China. Ecol. Eng. 44, 147–151.
Keywords: open-pit mine, diversity, growth rate, synergistic effect, soil, climate
Citation: Shi Z, Bai Z, Guo D, Ma X, Chen M and Li S (2023) Synergistic effects aided the growth of black locust in reclaimed areas of semi-arid open-pit coal mines. Front. Ecol. Evol. 11:1082321. doi: 10.3389/fevo.2023.1082321
Received: 28 October 2022; Accepted: 04 January 2023;
Published: 19 January 2023.
Edited by:
Purabi Saikia, Central University of Jharkhand, IndiaReviewed by:
Sanjay Kumar, Deen Dayal Upadhyay Gorakhpur University, IndiaCopyright © 2023 Shi, Bai, Guo, Ma, Chen and Li. This is an open-access article distributed under the terms of the Creative Commons Attribution License (CC BY). The use, distribution or reproduction in other forums is permitted, provided the original author(s) and the copyright owner(s) are credited and that the original publication in this journal is cited, in accordance with accepted academic practice. No use, distribution or reproduction is permitted which does not comply with these terms.
*Correspondence: Zhongke Bai, YmFpemtAY3VnYi5lZHUuY24=
Disclaimer: All claims expressed in this article are solely those of the authors and do not necessarily represent those of their affiliated organizations, or those of the publisher, the editors and the reviewers. Any product that may be evaluated in this article or claim that may be made by its manufacturer is not guaranteed or endorsed by the publisher.
Research integrity at Frontiers
Learn more about the work of our research integrity team to safeguard the quality of each article we publish.