- 1Department of Comparative Immunobiology, Zoological Institute, Christian-Albrechts University, Kiel, Germany
- 2Department of Evolutionary Ecology and Genetics, Zoological Institute, Christian-Albrechts University, Kiel, Germany
Some small animals migrate with the help of other, more mobile animals (phoresy) to leave short-lived and resource-poor habitats. The nematode Caenorhabditis elegans lives in ephemeral habitats such as compost, but has also been found associated with various potential invertebrate vectors. Little research has been done to determine if C. elegans is directly attracted to these invertebrates. To determine whether C. elegans is attracted to compounds and volatile odorants of invertebrates, we conducted chemotaxis experiments with the isopods Porcellio scaber, Oniscus asellus, and Armadillidium sp. and with Lithobius sp. myriapods, Drosophila melanogaster fruit flies, and Arion sp. slugs as representatives of natural vectors. Because phoresy is an important escape strategy in nature, especially for dauer larvae of C. elegans, we examined the attraction of the natural C. elegans isolate MY2079 in addition to the laboratory-adapted strain N2 at the dauer and L4 stage. We found that DMSO washing solution of Lithobius sp. and the odor of live D. melanogaster attracted C. elegans N2 L4 larvae. Surprisingly, the natural isolate MY2079 was not attracted to any invertebrate during either the dauer or L4 life stages and both C. elegans strains were repelled by various compounds from O. asellus, P. scaber, Armadillidium sp., Lithobius sp., and Arion sp. feces. We hypothesize that this is due to defense chemicals released by the invertebrates. Although compounds from Lithobius sp. and D. melanogaster odorants were mildly attractive, the lack of attraction to most invertebrates suggests a predominantly opportunistic association between C. elegans and invertebrate vectors.
Introduction
For many small animals, the ability to travel longer distances is severely limited. However, migrating longer distances and thus settling in a new environment may offer advantages: Competition for food and mating partners, as well as pressure from predators, parasites, and pathogens, may be lower. In addition, mating outside of their own population can help organisms increase their genetic diversity (Lacy, 1987; Gustafson et al., 2017). Especially for animals in short-lived habitats, migration is essential to avoid limited resource availability and improve survival (Reid et al., 2018). To travel longer distances some animals therefore use other, more mobile animals as a means of transportation. One example are oribatid mites, which use a variety of hosts including beetles, birds, and mammals for migration (Dusbabek and Bukva, 1991; Krivolutsky and Lebedeva, 2004; Knee et al., 2013). This type of temporary interaction for the purpose of dispersal is called phoresy (Farish and Axtell, 1971).
It is often unclear whether the association between small invertebrates and their vectors is purely incidental or whether the invertebrates actively search a vector. Odorants or chemicals released into the environment may play a crucial role in finding a host. Snails can track their mating partners using water- or air-borne pheromones, and mucus trails (Ng et al., 2013). A particular larval stage of the mite Myianoetus muscarum is attracted to a volatile substance produced by the pupa of the fly Muscina stabulans. The mite larvae gather at the anterior end of the pupa where the fly hatches and thus position themselves to be dispersed with the hatching fly (Greenberg and Carpenter, 1960). Entomopathogenic nematodes are attracted to volatile components of insect feces and odors emitted by live insects (Schmidt and All, 1979; Dillman et al., 2012).
The nematode Caenorhabditis elegans is often found in microbe-rich, short-lived habitats such as compost and other decomposing plant material (Schulenburg and Félix, 2017). In these habitats, C. elegans is frequently found in association with invertebrates, e.g., snails, slugs, isopods, and myriapods (Barrière and Félix, 2005; Caswell-Chen et al., 2005; Cutter, 2006; Kiontke and Sudhaus, 2006; Félix and Braendle, 2010; Félix and Duveau, 2012; Petersen et al., 2015a). These associations are assumed to be part of an escape strategy to migrate to new habitats if conditions deteriorate. Thus, migration using invertebrates allows C. elegans to travel comparatively long distances and establish populations in new locations.
It is known that C. elegans responds to chemical signals from food bacteria and pathogens (Pastan and Perlman, 1971; Ward, 1973; Andrew and Nicholas, 1976; Grewal and Wright, 1992; Schulenburg and Müller, 2004), but little research has been done to determine whether C. elegans can sense potential invertebrate vectors and move toward them (Dillman et al., 2012; Lee et al., 2017; Archer et al., 2020). This would be a prerequisite for C. elegans-initiated migration using invertebrates in response to food shortages or overpopulation. Previous studies suggest that C. elegans and the terrestrial isopod Porcellio scaber likely interact opportunistically (Archer et al., 2020). Information on the nature of interactions with other migration partners is scarce (Dillman et al., 2012; Lee et al., 2012, 2017).
The aim of the current study was to investigate if the interaction between C. elegans and invertebrates of different taxa representative of potential natural migration partners is based on C. elegans perception of a chemical signal from the invertebrates. We tested the chemotactic response of C. elegans toward different extracts of compounds and odorants from the isopod species Porcellio scaber, Oniscus asellus, and Armadillidium sp.; Lithobius sp. myriapods, the fruit fly Drosophila melanogaster and Arion sp. slugs. We included two C. elegans strains, the laboratory-adapted strain N2 and the natural strain MY2079, to account for the potentially higher importance of an escape strategy for free-living strains. To test whether attraction is stage-specific, we tested dauer larvae, which are often associated with invertebrates (Félix and Braendle, 2010), and fourth instar (L4) larvae. Caenorhabditis elegans N2 L4 larvae were attracted to Lithobius sp. myriapods washed in dimethyl sulfoxide (DMSO) and odor of live D. melanogaster while P. scaber, O. asellus, Armadillidium sp., and Arion sp. had no attractive effect. Surprisingly, the natural C. elegans strain MY2079 was not attracted to chemical compounds or odorants of any of the tested invertebrates. Both N2 and MY2079 were repelled by some chemical compounds or odorants. Based on these results, C. elegans appears to be attracted to some compounds, but most of the associations between C. elegans and the invertebrates studied are likely opportunistic rather than directed.
Materials and methods
Nematodes, invertebrates, and bacterial strains
All experiments were performed with the standard laboratory C. elegans strain N2 and the natural C. elegans isolate MY2079. N2 was originally obtained from the Caenorhabditis Genetics Center (CGC) and MY2079 was isolated from compost in the Botanical Garden in Kiel, Germany (Petersen et al., 2015b). All worms were maintained on nematode growth medium (NGM) plates with Escherichia coli strain OP50 as food following standard procedures (Stiernagle, 2006). Caenorhabditis elegans strains were bleached and kept for approximately 48 h on OP50 at 20°C to obtain L4 larvae or for 2 to 3 weeks at 25°C to obtain dauer larvae. All worms were washed from the plates freshly for every experiment.
Isopods, myriapods, and slugs for the experiments were caught from nature in Kiel, Germany (Supplementary Figures 1A–H). The isopod species Porcellio scaber, Oniscus asellus, and Armadillidium sp. originated from a garden plot and were mostly found below old bricks. Myriapods and slugs originated from the same compost as the natural C. elegans strain MY2079. Myriapods were sampled below tree trunks or tree bark stored close to compost. Isopods and myriapods were either directly used in experiments or kept for up to 4 days in plastic boxes with wet tissue paper and thick tree branches and leaves from their natural habitat. The wet tissue paper was replaced daily. Arion sp. slugs were kept individually for one night in boxes containing plant material from their natural habitat to reduce adhering debris.
The Drosophila melanogaster laboratory strain w[1118] was grown on standard yeast/cornmeal/agar medium at 25°C until day 4–6 of adulthood as previously described (Rahn et al., 2013; Li et al., 2015). Flies were starved in a sterile, empty fly container for 16 h at 25°C prior to the experiment with a piece of wet paper to prevent desiccation.
Invertebrate washes and extractions
Washes and extracts were prepared freshly for each replicate. One isopod, one Lithobius sp. or five D. melanogaster were transferred to a sterile 1.5-ml microcentrifuge tube. Subsequently, 180 μl sterile deionized water, DMSO or ethanol were added to isopods, 250 μl to Lithobius sp. and 120 μl to D. melanogaster. Washes were obtained from invertebrates washed in the solvent with the tube rotated on an orbital rotator for 30 min. Extracts were produced from washed invertebrates ground in the solvent using a sterile pestle.
Slug feces was collected from slugs kept for 1 day in a box and placed in a 1.5-ml microcentrifuge tube. After stirring, 1 mg feces was transferred to a new tube with 180 μl deionized water, DMSO, or ethanol, homogenized with a sterile pestle, briefly centrifuged and the liquid phase transferred to a new tube. All washes and extracts were used undiluted.
Chemotaxis assays
We tested the chemotactic response of C. elegans to wash supernatants (washes) and invertebrates ground in deionized water, DMSO, or ethanol (extracts). All experiments were performed on 6-cm NGM plates if not stated otherwise. A letter code was used to prevent any observer bias. Petri dishes were divided into quadrants as described previously to obtain an alternating pattern of test and control to avoid any bias that might result from the initial placement of the worms (Margie et al., 2013). A circle with a diameter of 1 cm marked the center of the plate (Supplementary Figure 1I). Sodium azide (0.5 M) diluted 1:3 in all test and control liquids was used as anesthetic. Immediately after preparation of the washes and extracts, 3 μl were pipetted to opposing quadrants of the plate and 3 μl of the corresponding solvent without extract or wash to the two remaining quadrants in-between. The general response of worms to chemical stimuli was tested using 3 μl of isoamyl alcohol diluted to 10−3 in ethanol (attractant) or undiluted 1-octanol (repellent) as the test compound. Plates were left for 2 h to allow all liquids to sink into the agar. Finally, 30–250 C. elegans L4 or dauer larvae were added to the center, a number that allows fast processing of the assay plates, is sufficient to limit the influence of individual worms and prevents overcrowding.
Slug mucus was stamped directly from the foot of a living slug onto one side of a 9-cm agar plate. The other side of the plate remained blank. 30–250 L4 or dauer larvae were pipetted to the center of the plate.
After 1 h, worms in the test and control quadrants or halves were scored. Worms remaining in the plate center were ignored and only replicates in which at least 10 worms left the center were considered. A choice index was calculated by subtracting the number of worms in the control quadrants (or halves) from the number of worms in test quadrants (or halves) and dividing the result by the total number of worms on the plate. A positive choice index indicates the choice of the test compound (attraction), a negative choice index indicates the choice of the control (repulsion) and a choice index around zero indicates equal choice of both.
Chemotaxis gas assay
The assays were performed on unseeded 6-cm NGM plates. The center was marked with a circle of 1 cm in diameter and plates were divided into test and control halves each halve with a drilled hole in the lid. One isopod, one Lithobius sp., or five D. melanogaster were placed in a sterile 1-ml pipette tip with a 3-mm opening. The tip was placed in the lid of one halve and an identical tip without invertebrate in the other halve. All tips were sealed at the top with a heat-sterilized 8-mm cigarette filter and a piece of heat-sterilized mesh in the tip prevented escape of Lithobius sp. and D. melanogaster. The gas exchange was not actively promoted but tested using 20 μl of isoamyl alcohol diluted to 10−3 in ethanol (attractant) or undiluted 1-octanol (repellent) on filter paper in a pipette tip as the test odorant. Additionally, the controls were used to test the general response of worms to air-borne stimuli.
30–250 C. elegans L4 or dauer larvae were added to the plate center. After 1 h at room temperature, the tips were removed and worms on each halve were scored. A choice index was calculated as described before for the chemotaxis assays.
Statistics
The experiments were performed as time-independent, biological replicates for each combination of nematode strain, nematode developmental stage, and invertebrate. Mean choice indices were reported. One-sample Wilcoxon-Signed rank tests (Wilcoxon, 1945) with false discovery rate correction (Benjamini and Hochberg, 1995) for multiple testing were performed to assess differences between a choice index of zero and the corresponding choice index of the nematode strain, developmental stage, and solvent. All statistics were performed using R-Studio (version 1.4.1717) and are presented in Supplementary Tables 1–3 and Supplementary File 1. The raw data can be found in Supplementary Tables 4–7. Composite figures were created in Inkscape (version 1.1) and Affinity Photo (version 1.10.5).
Results
Caenorhabditis elegans L4 and dauer larvae are not attracted to compounds washed from isopods or fruit flies, but N2 L4 larvae are attracted to compounds washed from myriapods
Caenorhabditis elegans’ search for hosts used for migration may potentially be facilitated by chemical signals delivered by the host to the environment. The ability to emit chemical signals is known from various invertebrates, on which C. elegans has been repeatedly found. Snails are known to release substances with their mucus that enable other snails to follow their tracks (Ng et al., 2013). Isopods such as Armadillidium vulgare use chemical signals to attract mating partners (Beauché and Richard, 2013). These chemical signals used for intra-specific communication could guide C. elegans to suitable migration partners. To test whether invertebrate secretions are attractive to C. elegans, we used wash solutions of three isopod species, as well as of myriapods, and fruit flies as potential attractants in chemotaxis assays. We found that L4 C. elegans N2 were attracted to Lithobius sp. wash in DMSO (p = 0.026), but not to wash in deionized water and ethanol. In contrast, N2 dauer larvae and neither stage of MY2079 showed attractive or repulsive behavior toward Lithobius sp. wash (Figure 1, Supplementary Tables 1, 4). Caenorhabditis elegans MY2079 dauer larvae were repelled by O. asellus wash in deionized water (p = 0.042), while L4 MY2079 and both N2 stages showed no chemotactic response to O. asellus wash. Washes of P. scaber, Armadillidium sp., and D. melanogaster did neither attract nor repel any C. elegans. Both C. elegans strains were attracted to the control attractant isoamyl alcohol and repelled by the control repellent 1-octanol as L4 larvae (Supplementary Figure 2, Supplementary Tables 1, 4). However, there was a high variability in the response of dauer larvae to both the attraction and repellent controls.
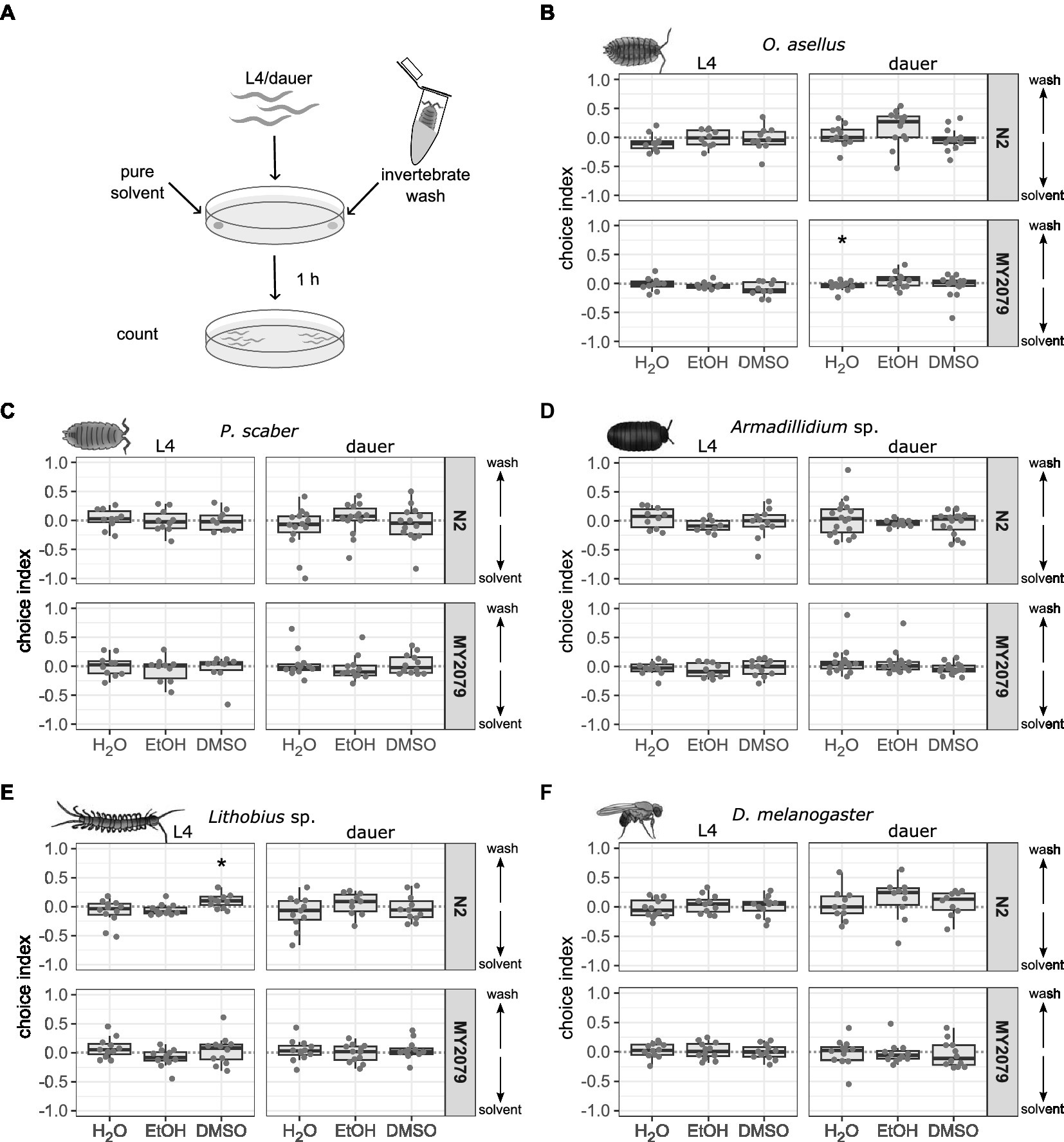
Figure 1. Caenorhabditis elegans strains N2 and MY2079 do not respond to most invertebrate washes with three different solvents. (A) Caenorhabditis elegans strains N2 and MY2079 were grown on OP50 and used as L4 or dauer larvae to evaluate their chemotactic response toward washes of potential invertebrate migration partners in three different solvents. A positive choice index indicates attraction to the invertebrate wash from (B) Oniscus asellus (n = 10–17), (C) Porcellio scaber (n = 10–16), (D) Armadillidium sp. (n = 10–20), (E) Lithobius sp. (n = 10–15), and (F) Drosophila melanogaster (n = 11–12). A negative choice index indicates repulsion from the invertebrate wash, a choice index of 0 indicates equal choice of both sides. For comparison of choice indices with 0, the Wilcoxon signed-rank test with false discovery rate correction for multiple testing was applied. Data are presented as boxplots with the median as a thick horizontal line, the interquartile range as box, the whiskers as vertical lines, and each replicate depicted by a dot. Significance is designated to *p < 0.05. From left to right each panel shows boxes representing deionized water (H2O), ethanol (EtOH), or dimethyl sulfoxide (DMSO) washes (with 30–250 worms per replicate).
Caenorhabditis elegans L4 and dauer larvae are not attracted to compounds extracted from diverse ground invertebrates
Washing dissolves only the compounds that adhere to the outside of the invertebrates. The concentration of these washed compounds might be too small to trigger a chemotactic response in C. elegans due to dilution with the solvents. Other compounds could be located inside the invertebrates, for example, in ingested food or gut content. To extract potentially attractive compounds from the inside of invertebrates, whole O. asellus, P. scaber, Armadillidium sp., Lithobius sp., and D. melanogaster were first washed in deionized water, ethanol or DMSO and then ground with a sterile pestle (Figure 2A). Caenorhabditis elegans N2 and MY2079 were not attracted to any of the ground invertebrates in either as L4 or dauer larvae (Figures 2B–F, Supplementary Tables 2, 5). In contrast, some invertebrate extracts had a repellent effect. L4 C. elegans N2 were repelled from P. scaber in deionized water (p = 0.044) and DMSO (p = 0.004), Armadillidium sp. in deionized water (p = 0.017) and ethanol (p = 0.005), and Lithobius sp. in deionized water (p = 0.005). N2 dauer larvae were neither attracted nor repelled by any invertebrate. L4 C. elegans MY2079 were repelled by O. asellus in deionized water (p = 0.016), P. scaber in deionized water (p = 0.018) and DMSO (p = 0.033), Armadillidium sp. in deionized water (p = 0.022), ethanol (p = 0.047), and DMSO (p = 0.024), and Lithobius sp. in ethanol (p = 0.034). MY2079 dauer larvae were repelled by O. asellus in ethanol (p = 0.014). L4 larvae of C. elegans N2 and MY2079 were attracted to the control attractant isoamyl alcohol and repelled by the control repellent 1-octanol (Supplementary Figure 3, Supplementary Tables 2, 5). However, dauer larvae of both C. elegans strains reacted variable to the attraction and repellent controls.
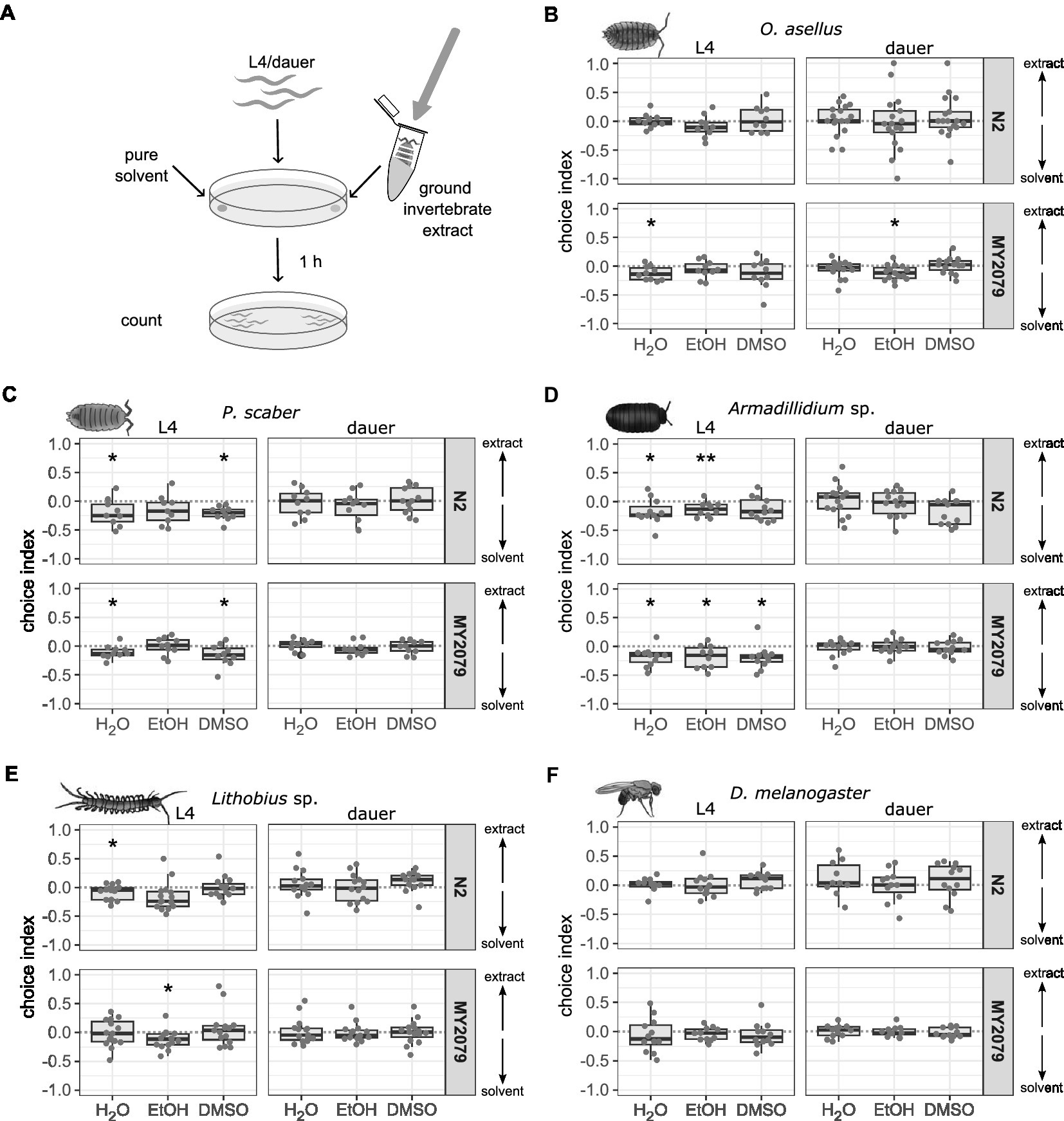
Figure 2. Caenorhabditis elegans strains N2 and MY2079 are not attracted to extracts from ground invertebrates in three solvents. (A) Caenorhabditis elegans strains N2 and MY2079 were grown on OP50 and used as L4 or dauer larvae to evaluate their chemotactic response toward extracts obtained from invertebrates ground in three different solvents. A positive choice index indicates attraction to the extract from (B) Oniscus asellus (n = 9–17), (C) Porcellio scaber (n = 9–14), (D) Armadillidium sp. (n = 11–18), (E) Lithobius sp. (n = 13–15), or (F) Drosophila melanogaster (n = 11–12). A negative choice index indicates repulsion from the invertebrate (solvent), a choice index of 0 indicates equal choice of both sides. For comparison of choice indices with 0, the Wilcoxon signed-rank test with false discovery rate correction for multiple testing was applied. Data are presented as boxplots with the median as a thick horizontal line, the interquartile range as box, the whiskers as vertical lines, and each replicate depicted by a dot. From left to right each panel shows boxes representing deionized water (H2O), ethanol (EtOH), and dimethyl sulfoxide (DMSO) extracts (with 30–250 worms per replicate). Significance is designated to the following scale: **p < 0.01, *p < 0.05.
Caenorhabditis elegans L4 and dauer larvae do not respond to Arion sp. mucus and are not attracted to Arion sp. feces
Slugs and snails have been repeatedly identified as vectors of C. elegans (Petersen et al., 2015a; Schulenburg and Félix, 2017). Since mucus plays a role in communication between snails and their conspecifics (Ng et al., 2013; Vong et al., 2019) and is therefore also a potential source of attraction for C. elegans, the attraction of mucus from the foot of Arion sp. was tested as potential attractant in the chemotaxis assay. We found that Arion sp. mucus did neither attract nor repel C. elegans (Figure 3A, Supplementary Tables 2, 6). Caenorhabditis elegans has often been isolated from the slug gut and both C. elegans and potential food bacteria can survive passage through the slug gut and be found in slug feces (Petersen et al., 2015a; Schulenburg and Félix, 2017; Pees et al., 2021). Therefore, we tested the chemotactic attraction towards slug feces next. Neither L4 nor dauer larvae of C. elegans N2 and MY2079 were attracted to Arion sp. feces (Figure 3B, Supplementary Tables 2, 6). L4 C. elegans N2 were repelled from feces in ethanol (p = 0.034). As seen before, both C. elegans strains were attracted to the control attractant isoamyl alcohol and repelled by the control repellent 1-octanol as L4 larvae (Supplementary Figure 3, Supplementary Tables 2, 6). However, the response of dauer larvae to the attraction and repellent controls was highly variable.
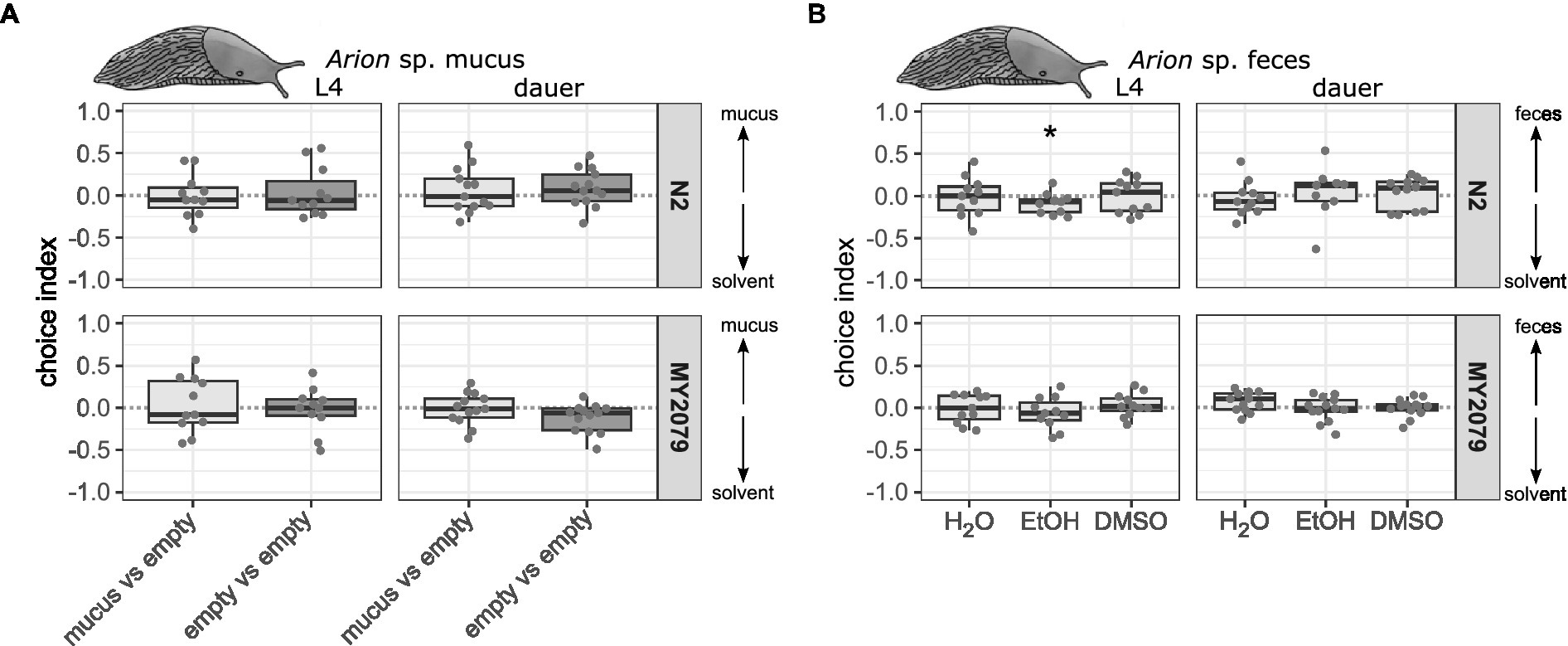
Figure 3. Caenorhabditis elegans strains N2 and MY2079 do not respond to Arion sp. mucus and L4 N2 is repelled by Arion sp. feces in ethanol. Chemotactic response of C. elegans N2 and MY2079 L4 and dauer larvae toward (A), slug mucus applied directly from the Arion sp. foot compared to an empty plates side (n = 11–13) and (B), slug feces in three different solvents compared to the corresponding control solvent (n = 9–13). A positive choice index indicates attraction to slug mucus or slug feces, a negative choice index indicates repulsion from slug mucus or slug feces, a choice index of 0 indicates equal choice of both sides. For comparison of choice indices with 0, the Wilcoxon signed-rank test with false discovery rate correction for multiple testing was applied. Data are presented as boxplots with the median as a thick horizontal line, the interquartile range as box, the whiskers as vertical lines, and each replicate depicted by a dot. Significance is designated to *p < 0.05. (B) From left to right each panel shows boxes representing deionized water (H2O), ethanol (EtOH), and dimethyl sulfoxide (DMSO) feces extracts (with 30–250 worms per replicate).
Caenorhabditis elegans L4 and dauer larvae are not attracted to invertebrate odorants
Entomopathogenic nematodes are attracted to volatile components of insect feces and odors emitted by live insects (Schmidt and All, 1979; Dillman et al., 2012). Carbon dioxide (CO2) has been identified as an essential host cue for entomopathogenic nematodes (Dillman et al., 2012). Therefore, gaseous components of odorants secreted by invertebrate hosts could play a role as attractants for C. elegans. We tested this hypothesis in simple gas chemotaxis assays using live isopods, myriapods, and fruit flies. L4 C. elegans N2 were attracted to D. melanogaster (p = 0.006), but not to any other invertebrate odorant (Figure 4, Supplementary Tables 3, 7). N2 dauer larvae were not attracted to or repulsed by any invertebrate odorant. L4 and dauer larvae of C. elegans MY2079 were not attracted to or repulsed by any invertebrate odorant. Both C. elegans strains were attracted to odor of the control attractant isoamyl alcohol in as L4 larvae (Supplementary Figure 4, Supplementary Tables 3, 7). L4 larvae of N2 were repelled by the odor of the control repellent 1-octanol while C. elegans MY2079 L4 larvae showed variability in response to 1-octanol odor. Dauer larvae of both C. elegans strains showed a high variability in the response to both the attraction and repellent controls.
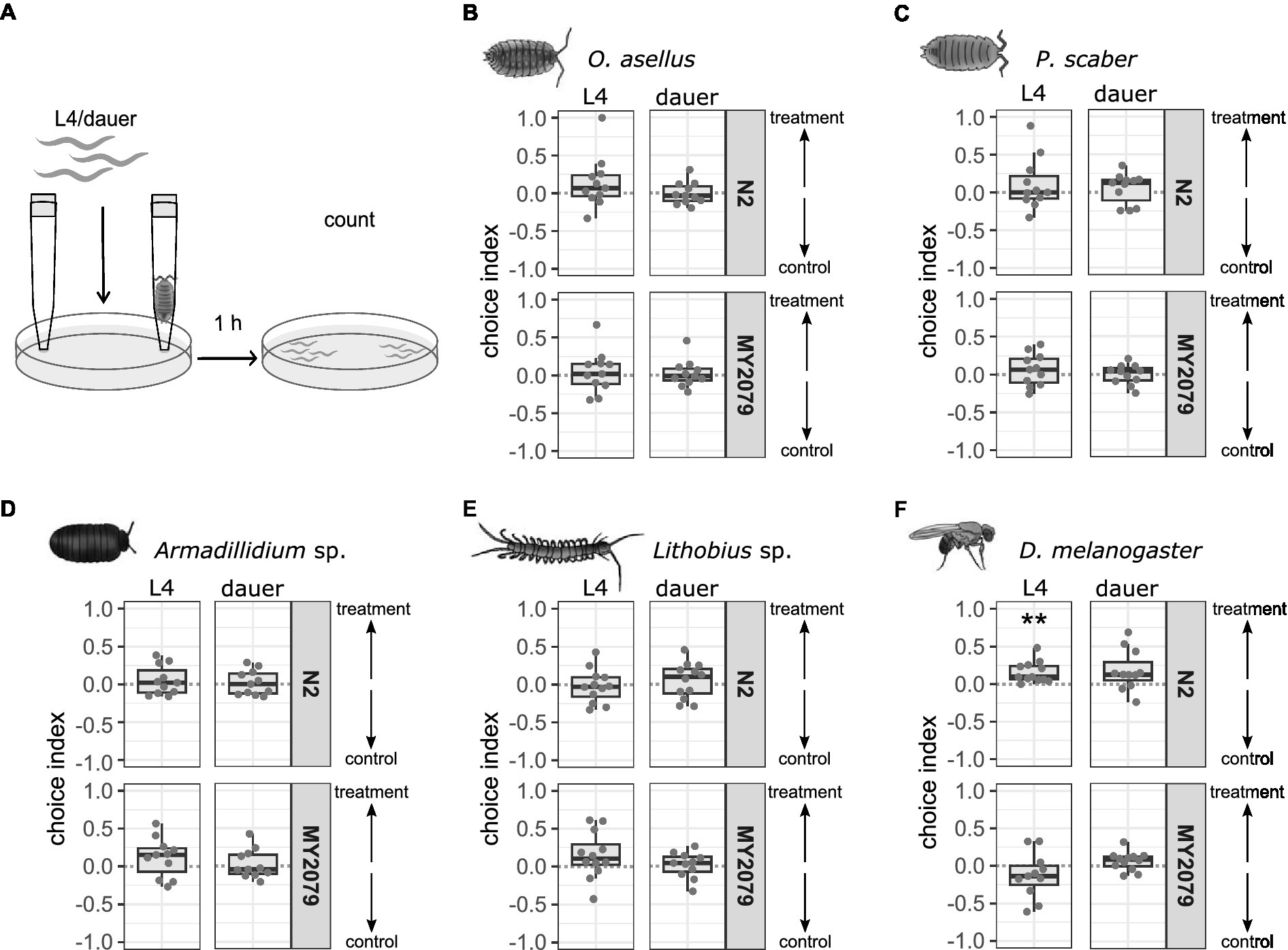
Figure 4. Caenorhabditis elegans N2 is attracted to Drosophila melanogaster odorant at L4 stage, but C. elegans does not respond to any other invertebrate odorant. (A) The attraction of C. elegans to invertebrate odorants was determined using live invertebrates fixated in pipette tips at the lid of the assay plates. A positive choice index indicates attraction to the odor of (B) Oniscus asellus (n = 11), (C) Porcellio scaber (n = 11), (D) Armadillidium sp. (n = 11), (E) Lithobius sp. (n = 11–12), or (F) D. melanogaster (n = 11–12; treatment), a negative choice index indicates repulsion from the invertebrate (control), a choice index of 0 indicates equal choice of both sides. For comparison of choice indices with 0, the Wilcoxon signed-rank test with false discovery rate (FDR) correction for multiple testing was applied. Data are presented as boxplots with the median as a thick horizontal line, the interquartile range as box, the whiskers as vertical lines, and each replicate depicted by a dot. Significance is designated to **p < 0.01 (with 30–250 worms per replicate).
Discussion
We studied the attraction of C. elegans to secreted compounds and volatile odorants of representatives of natural hosts. Our results indicate that C. elegans is not attracted to secreted compounds and volatile odorants of invertebrates, with the exception of Lithobius sp. wash and D. melanogaster odorants that were slightly attractive to N2 L4 larvae. The attraction was only observed towards Lithobius sp. DMSO wash and not towards ground animals or Lithobius sp. odorants indicating that (a) soluble attractive compounds are likely located at the body surface of Lithobius sp. and (b) the attracting compound is likely insoluble or slightly soluble in water and ethanol. DMSO is an efficient solvent, and some compounds may be more soluble in DMSO than in water or ethanol (Clark et al., 2008; Alastruey-Izquierdo et al., 2012), hinting to a dose-dependent attraction towards compounds in Lithobius sp. wash. However, a dose-dependent attraction of C. elegans to the isopod P. scaber was not detected (Archer et al., 2020). Drosophila occurs in the natural habitat of C. elegans and can disperse nictating C. elegans dauer larvae, but not non-dauer stages, under laboratory conditions (Lee et al., 2012). In contrast, N2 L4 larvae but not dauer larvae were attracted to live D. melanogaster odorants in our experiments indicating that more than only nictation behavior may be involved in C. elegans dispersal.
No other extracted invertebrate compound or odorant attracted C. elegans. These results were unexpected. In particular, we expected that some invertebrates would have an attracting effect for the natural C. elegans isolate MY2079, as a change of location is likely important for survival in the wild. Although this natural strain has been shown to be generally capable of chemotaxis (Petersen et al., 2021), it was surprisingly not attracted to any invertebrate. Similarly, Archer et al. found no attraction of C. elegans N2 and a wild isolate to the isopod P. scaber (Archer et al., 2020). Overall, our results suggest that migration of C. elegans by invertebrates is opportunistic rather than C. elegans being attracted to invertebrates.
All isopods, myriapods, and slugs used in this study were collected in the wild. The altered environment of the laboratory may have resulted in a change in the chemical signals emitted and the subsequent chemoattraction of C. elegans. Furthermore, the sampling sites for isopods and myriapods were poor in decaying plant material and may have resulted in a microbe-poor environment. This could play a role if the chemical signals emitted by invertebrates are influenced by factors from the natural environment, e.g., microbial products could contribute to odorants, leading to microbially mediated olfactory communication (Carthey et al., 2018).
It has been reported that C. elegans dauer larvae respond neutral or repulsive to odorants of potential hosts such as mole crickets, earwigs, flatheaded borers, pill bugs, and slugs, whereas parasitic nematodes from the genera Heterorhabditis, Steinernema, and Oscheius are attracted to some or all the mentioned hosts (Dillman et al., 2012). Similarly, extracts of ground invertebrates repeatedly had a repellent effect in our study. We hypothesize that this is due to the release of chemical defenses stored in the invertebrates or produced in response to the stressful washing procedure. Production, storage, and release of chemical defenses is known to occur in many invertebrates. Herbivorous insects such as the horseradish flea beetle selectively accumulate plant toxins in the hemolymph to defend themselves against predators (Yang et al., 2021). The secretions of chemical defense glands located along the body of some millipedes may consist of irritants, repellents, antifeedants, or even hydrogen cyanide gas, which is lethal to some other species (Eisner et al., 1978; Shear, 2015). Additionally, microbial symbionts may contribute to the production of repellent chemicals against predators, as hypothesized for the ladybird Harmonia axyridis (Schmidtberg et al., 2019).
Responses of dauer larvae to many chemical attractants are similar to responses of non-dauer stages (Ward, 1973; Cassada and Russell, 1975). However, sometimes dauer larvae do not respond to known attractants, such as sodium ions (Na+), and lack the rapid chemotactic response to food bacteria seen in well-fed larvae and adults (Albert and Riddle, 1983). In our study dauer larvae were not attracted to any invertebrate and failed repeatedly to respond in particular to the control repellent 1-octanol, a lack of response not seen in Archer et al. (2020). This may be due to the fact that Archer et al. generated dauer larvae within 48 h through exposure to dauer pheromone in liquid medium while we placed agar plates for 2–3 weeks at 25°C. Therefore, the dauer larvae in our study were older and thus exposed longer to the continuously secreted dauer pheromone, which may have affected olfactory plasticity (Yamada et al., 2010).
In summary, our results suggest that C. elegans appears to be attracted to some invertebrate compounds, but most of the associations between C. elegans and the invertebrates studied are likely opportunistic rather than directed. The future use of different dosages of the invertebrate extracts and washes, as well as the use of solvents with a different solubility spectrum, could help to further deepen the knowledge gained. It is possible that factors other than chemical signals play a role in attraction to a host. Diverse sensory neurons enable C. elegans to sense not only chemical cues, but also to respond to physical stimuli (Goodman and Sengupta, 2019). Thus, mechanical stimuli from host movement or thermal stimuli from host body temperature could contribute to host perception. Testing attraction in response to different stimuli from potential hosts is an interesting future step.
Data availability statement
The original contributions presented in the study are included in the article/Supplementary material, further inquiries can be directed to the corresponding author.
Author contributions
CP: conceptualization, data curation, formal analysis, investigation, methodology, project administration, supervision, validation, visualization, writing—original draft, and writing—review and editing. AK: investigation. ML: resources. All authors read and approved of the final manuscript.
Funding
The work was funded by the German Science Foundation within the Collaborative Research Center CRC 1182 on Origin and Function of Metaorganisms (project A1.1 funding for CP). The funders had no role in study design, data collection and analysis, decision to publish, or preparation of the manuscript.
Acknowledgments
The authors would like to thank Heidrun Ließegang and Joyce Schrader for technical support, Lena Peters for access to her allotment and help collecting invertebrates, Susanne Petersen for access to the compost sampling site in the Botanical Garden in Kiel, and Katja Dierking for valuable feedback on the manuscript. D. melanogaster w[1118] was kindly provided by Lasse Tiedemann from the Molecular Physiology Group at Kiel University, headed by Thomas Roeder. Caenorhabditis elegans MY2079 was kindly provided by Hinrich Schulenburg of the Evolutionary Ecology and Genetics Group at Kiel University. Caenorhabditis elegans N2 was originally provided by the CGC, which is funded by the NIH Office of Research Infrastructure Programs (P40 OD010440).
Conflict of interest
The authors declare that the research was conducted in the absence of any commercial or financial relationships that could be construed as a potential conflict of interest.
Publisher’s note
All claims expressed in this article are solely those of the authors and do not necessarily represent those of their affiliated organizations, or those of the publisher, the editors and the reviewers. Any product that may be evaluated in this article, or claim that may be made by its manufacturer, is not guaranteed or endorsed by the publisher.
Supplementary material
The Supplementary material for this article can be found online at: https://www.frontiersin.org/articles/10.3389/fevo.2023.1069056/full#supplementary-material
References
Alastruey-Izquierdo, A., Gómez-López, A., Arendrup, M. C., Lass-Florl, C., Hope, W. W., Perlin, D. S., et al. (2012). Comparison of dimethyl sulfoxide and water as solvents for echinocandin susceptibility testing by the EUCAST methodology. J. Clin. Microbiol. 50, 2509–2512. doi: 10.1128/JCM.00791-12
Albert, P. S., and Riddle, D. L. (1983). Developmental alterations in sensory neuroanatomy of the Caenorhabditis elegans dauer larva. J. Comp. Neurol. 219, 461–481. doi: 10.1002/cne.902190407
Andrew, P. A., and Nicholas, W. L. (1976). Effect of bacteria on dispersal of Caenorhabditis elegans (Rhabditidae). Nematologica 22, 451–461. doi: 10.1163/187529276X00454
Archer, H., Deiparine, S., and Andersen, E. C. (2020). The nematode Caenorhabditis elegans and the terrestrial isopod Porcellio scaber likely interact opportunistically. PLoS One 15:e0235000. doi: 10.1371/journal.pone.0235000
Barrière, A., and Félix, M.-A. (2005). High local genetic diversity and low outcrossing rate in Caenorhabditis elegans natural populations. Curr. Biol. 15, 1176–1184. doi: 10.1016/j.cub.2005.06.022
Beauché, F., and Richard, F.-J. (2013). The best timing of mate search in Armadillidium vulgare (isopoda, Oniscidea). PLoS One 8:e57737. doi: 10.1371/journal.pone.0057737
Benjamini, Y., and Hochberg, Y. (1995). Controlling the false discovery rate: a practical and powerful approach to multiple testing. J. R. Stat. Soc. Series B Stat. Methodol. 57, 289–300. doi: 10.1111/j.2517-6161.1995.tb02031.x
Carthey, A. J. R., Gillings, M. R., and Blumstein, D. T. (2018). The extended genotype: microbially mediated olfactory communication. Trends Ecol. Evol. 33, 885–894. doi: 10.1016/j.tree.2018.08.010
Cassada, R. C., and Russell, R. L. (1975). The dauerlarva, a post-embryonic developmental variant of the nematode Caenorhabditis elegans. Dev. Biol. 46, 326–342. doi: 10.1016/0012-1606(75)90109-8
Caswell-Chen, E. P., Chen, J., Lewis, E. E., Douhan, G. W., Nadler, S. A., and Carey, J. R. (2005). Revising the standard wisdom of C. elegans natural history: ecology of longevity. Sci. Aging Knowl. Environ. 2005:pe30. doi: 10.1126/sageke.2005.40.pe30
Clark, T., Murray, J. S., Lane, P., and Politzer, P. (2008). Why are dimethyl sulfoxide and dimethyl sulfone such good solvents? J. Mol. Model. 14, 689–697. doi: 10.1007/s00894-008-0279-y
Cutter, A. D. (2006). Nucleotide polymorphism and linkage disequilibrium in wild populations of the partial selfer Caenorhabditis elegans. Genetics 172, 171–184. doi: 10.1534/genetics.105.048207
Dillman, A. R., Guillermin, M. L., Lee, J. H., Kim, B., Sternberg, P. W., and Hallem, E. A. (2012). Olfaction shapes host-parasite interactions in parasitic nematodes. Proc. Natl. Acad. Sci. 109, E2324–E2333. doi: 10.1073/pnas.1211436109
Dusbabek, F., and Bukva, V. (1991). Modern acarology. Presented at the International Congress of Acarology 1990, SPB Academic Publishing, České Budějovice, Czechoslovakia.
Eisner, T., Alsop, D., Hicks, K., and Meinwald, J. (1978). “Defensive secretions of millipeds” in Arthropod Venoms, Handbook of Experimental Pharmacology/Handbuch Der Experimentellen Pharmakologie. ed. S. Bettini (Berlin, Heidelberg: Springer), 41–72.
Farish, D. J., and Axtell, R. C. (1971). Phoresy redefined and examined in Macrocheles muscaedomesticae (Acarina:Macrochelidae). Acarologia 13, 16–29.
Félix, M.-A., and Braendle, C. (2010). The natural history of Caenorhabditis elegans. Curr. Biol. 20, R965–R969. doi: 10.1016/j.cub.2010.09.050
Félix, M.-A., and Duveau, F. (2012). Population dynamics and habitat sharing of natural populations of Caenorhabditis elegans and C. briggsae. BMC Biol. 10. doi: 10.1186/1741-7007-10-59
Goodman, M. B., and Sengupta, P. (2019). How Caenorhabditis elegans senses mechanical stress, temperature, and other physical stimuli. Genetics 212, 25–51. doi: 10.1534/genetics.118.300241
Greenberg, B., and Carpenter, P. D. (1960). Factors in phoretic association of a mite and fly. Science 132, 738–739. doi: 10.1126/science.132.3429.738
Grewal, P. S., and Wright, D. J. (1992). Migration of Caenorhabditis elegans (Nematoda: Rhabditidae) larvae towards bacteria and the nature of the bacterial stimulus. Fundam. Appl. Nematol. 15:8.
Gustafson, K. D., Vickers, T. W., Boyce, W. M., and Ernest, H. B. (2017). A single migrant enhances the genetic diversity of an inbred puma population. R. Soc. Open Sci. 4:170115. doi: 10.1098/rsos.170115
Kiontke, K., and Sudhaus, W. (2006). Ecology of Caenorhabditis species. WormBook, 1–14. doi: 10.1895/wormbook.1.37.1
Knee, W., Forbes, M. R., and Beaulieu, F. D. R. (2013). Diversity and host use of mites (Acari: Mesostigmata, Oribatida) phoretic on bark beetles (Coleoptera: Scolytinae): global generalists, local specialists? Ann. Entomol. Soc. Am. 106:12. doi: 10.1603/AN12092
Krivolutsky, D. A., and Lebedeva, N. V. (2004). Oribatid mites (Oribatei, Acariformes) in bird feathers: non-passerines. Acta Zool. Litu. 14, 26–47. doi: 10.1080/13921657.2004.10512570
Lacy, R. C. (1987). Loss of genetic diversity from managed populations: interacting effects of drift, mutation, immigration, selection, and population subdivision. Conserv. Biol. 1, 143–158. doi: 10.1111/j.1523-1739.1987.tb00023.x
Lee, H., Choi, M., Lee, D., Kim, H.-s., Hwang, H., Kim, H., et al. (2012). Nictation, a dispersal behavior of the nematode Caenorhabditis elegans, is regulated by IL2 neurons. Nat. Neurosci. 15, 107–112. doi: 10.1038/nn.2975
Lee, D., Yang, H., Kim, J., Brady, S., Zdraljevic, S., Zamanian, M., et al. (2017). The genetic basis of natural variation in a phoretic behavior. Nat. Commun. 8:273. doi: 10.1038/s41467-017-00386-x
Li, Y., Fink, C., El-Kholy, S., and Roeder, T. (2015). The octopamine receptor octß2R is essential for ovulation and fertilization in the fruit fly Drosophila melanogaster. Arch. Insect Biochem. Physiol. 88, 168–178. doi: 10.1002/arch.21211
Margie, O., Palmer, C., and Chin-Sang, I. (2013). C. elegans chemotaxis assay. J. Vis. Exp. :e50069. doi: 10.3791/50069
Ng, T. P. T., Saltin, S. H., Davies, M. S., Johannesson, K., Stafford, R., and Williams, G. A. (2013). Snails and their trails: the multiple functions of trail-following in gastropods. Biol. Rev. 88, 683–700. doi: 10.1111/brv.12023
Pastan, I., and Perlman, R. L. (1971). Cyclic AMP in metobolism. Nat. New Biol. 229, 5–7. doi: 10.1038/newbio229005a0
Pees, B., Johnke, J., Möhl, M., Hamerich, I. K., Leippe, M., and Petersen, C. (2021). Microbes to-go: slugs as source for Caenorhabditis elegans microbiota acquisition. Environ. Microbiol. 23, 6721–6733. doi: 10.1111/1462-2920.15730
Petersen, C., Hermann, R. J., Barg, M.-C., Schalkowski, R., Dirksen, P., Barbosa, C., et al. (2015a). Travelling at a slug’s pace: possible invertebrate vectors of Caenorhabditis nematodes. BMC Ecol. 15:19. doi: 10.1186/s12898-015-0050-z
Petersen, C., Pees, B., Martínez Christophersen, C., and Leippe, M. (2021). Preconditioning with natural microbiota strain Ochrobactrum vermis MYb71 influences Caenorhabditis elegans behavior. Front. Cell. Infect. Microbiol. 11:775634. doi: 10.3389/fcimb.2021.775634
Petersen, C., Saebelfeld, M., Barbosa, C., Pees, B., Hermann, R. J., Schalkowski, R., et al. (2015b). Ten years of life in compost: temporal and spatial variation of north German Caenorhabditis elegans populations. Ecol. Evol. 5, 3250–3263. doi: 10.1002/ece3.1605
Rahn, T., Leippe, M., Roeder, T., and Fedders, H. (2013). EGFR signaling in the brain is necessary for olfactory learning in drosophila larvae. Learn. Mem. 20, 194–200. doi: 10.1101/lm.029934.112
Reid, J. M., Travis, J. M. J., Daunt, F., Burthe, S. J., Wanless, S., and Dytham, C. (2018). Population and evolutionary dynamics in spatially structured seasonally varying environments: partially migratory meta-populations. Biol. Rev. 93, 1578–1603. doi: 10.1111/brv.12409
Schmidt, J., and All, J. N. (1979). Attraction of Neoaplectana carpocapsae (Nematoda: Steinernematidae) to common excretory products of insects. Environ. Entomol. 7, 55–61.
Schmidtberg, H., Shukla, S. P., Halitschke, R., Vogel, H., and Vilcinskas, A. (2019). Symbiont-mediated chemical defense in the invasive ladybird Harmonia axyridis. Ecol. Evol. 9, 1715–1729. doi: 10.1002/ece3.4840
Schulenburg, H., and Félix, M.-A. (2017). The natural biotic environment of Caenorhabditis elegans. Genetics 206, 55–86. doi: 10.1534/genetics.116.195511
Schulenburg, H., and Müller, S. (2004). Natural variation in the response of Caenorhabditis elegans towards Bacillus thuringiensis. Parasitology 128, 433–443. doi: 10.1017/S003118200300461X
Shear, W. A. (2015). The chemical defenses of millipedes (diplopoda): biochemistry, physiology and ecology. Biochem. Syst. Ecol. 61, 78–117. doi: 10.1016/j.bse.2015.04.033
Vong, A., Ansart, A., and Dahirel, M. (2019). Dispersers are more likely to follow mucus trails in the land snail Cornu aspersum. Sci. Nat. 106:43. doi: 10.1007/s00114-019-1642-9
Ward, S. (1973). Chemotaxis by the nematode Caenorhabditis elegans: identification of attractants and analysis of the response by use of mutants. Proc. Natl. Acad. Sci. U. S. A. 70, 817–821. doi: 10.1073/pnas.70.3.817
Wilcoxon, F. (1945). Individual comparisons by ranking methods. Biom. Bull. 1, 80–83. doi: 10.2307/3001968
Yamada, K., Hirotsu, T., Matsuki, M., Butcher, R. A., Tomioka, M., Ishihara, T., et al. (2010). Olfactory plasticity is regulated by pheromonal signaling in Caenorhabditis elegans. Science 329, 1647–1650. doi: 10.1126/science.1192020
Keywords: Caenorhabditis elegans , chemotaxis, migration, invertebrates, Arion sp. slugs, Drosophila melanogaster , phoresy, isopods
Citation: Petersen C, Krahn A and Leippe M (2023) The nematode Caenorhabditis elegans and diverse potential invertebrate vectors predominantly interact opportunistically. Front. Ecol. Evol. 11:1069056. doi: 10.3389/fevo.2023.1069056
Edited by:
Jennifer M. Gleason, University of Kansas, United StatesReviewed by:
Michael A. Herman, University of Nebraska-Lincoln, United StatesJoanne Yew, University of Hawaii at Manoa, United States
Erik Andersen, Northwestern University, United States
Copyright © 2023 Petersen, Krahn and Leippe. This is an open-access article distributed under the terms of the Creative Commons Attribution License (CC BY). The use, distribution or reproduction in other forums is permitted, provided the original author(s) and the copyright owner(s) are credited and that the original publication in this journal is cited, in accordance with accepted academic practice. No use, distribution or reproduction is permitted which does not comply with these terms.
*Correspondence: Carola Petersen, ✉ cpetersen@zoologie.uni-kiel.de