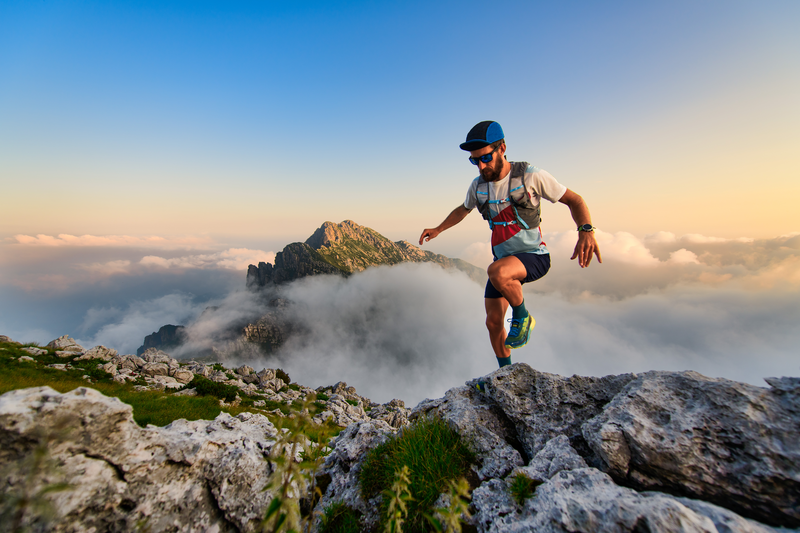
95% of researchers rate our articles as excellent or good
Learn more about the work of our research integrity team to safeguard the quality of each article we publish.
Find out more
POLICY AND PRACTICE REVIEWS article
Front. Ecol. Evol. , 17 February 2023
Sec. Ecophysiology
Volume 11 - 2023 | https://doi.org/10.3389/fevo.2023.1062441
This article is part of the Research Topic Insect Pollinators in the Anthropocene: How Multiple Environmental Stressors Are Shaping Pollinator Health View all 11 articles
The risk of poisoning bees by sprayed pesticides depends on the attractiveness of plants and environmental and climatic factors. Thus, to protect bees from pesticide intoxication, an usual exemption to pesticide regulations allows for spraying on blooming flowers with insecticides or acaricides when no bees are foraging on crops. Nevertheless, decision-making criteria for farmers to assess the absence of bees on their crops remain under debate. To fill this gap, we present here a review of the literature and an analysis of weather conditions and environmental factors that affect the presence of bees on flowering crops that may be treated with pesticides, with the objective of proposing to farmers a series of decision-making criteria on how and when to treat. We conclude that the criteria commonly considered, such as ambient temperature, crop attractiveness, or distance from field edges, cannot guarantee the absence of forager exposure during pesticide sprays. Nocturnal sprays of pesticides on crops would be the most effective action to help farmers avoid unintentional acute poisoning of bees.
Before agricultural use, pesticides that protect cultivated plants against harmful organisms (e.g., insects and fungus) or undesired weeds, must be evaluated and approved by regulatory authorities. In the current regulatory framework of unintentional exposure to non-target organisms, such as bee pollinators, the effects of pesticides are assessed by standard tests (EPPO, 2010; EFSA, 2012). However, pesticide threats to bees persist despite regulatory efforts (Decourtye et al., 2019; Mancini et al., 2019; Sgolastra et al., 2020). Thus, additional direct measures applied to bees were initiated to protect these non-target species from poisoning when farmers use pesticides. For instance, French regulations stipulate that insecticides or acaricides bearing the « Bee label » have an exemption that allows for their application on flowering plants, provided that foraging bees are absent from the treated crops (JORF n°0271).1 In Europe, the regulations specify that pesticide sprays not be applied during crop flowering or during foraging periods (Directive 2009/128/EC on Sustainable Use of Pesticides and related National Action Plans from 28 member states of the EU). When regulations prohibit the spraying of pesticides during the period of foraging activity of bees, the decision-making criteria to help farmers avoid unintentional poisoning of bees on crops remain unknown. This article aims to present an overview of the current knowledge on bee-crop interactions in order to clarify the decisions needed before the spraying of pesticides on flowering plants. In the first part, we recalled that regulations must protect a wide diversity of bee species that have different sensitivities to pesticides. In the second part, we assessed the limits of the current regulations to protect bees from pesticides by reviewing the exposure of bees to residues under real conditions. In the third part, based on the field data collected by the consortium of French apidologists, we analyzed the environmental and climatic conditions that would guarantee the absence of bees on agricultural crops. Finally in the fourth part, we investigated whether considering attractiveness of crops the exposure of bees to pesticides can be mitigated.
The two first parts set the scene and explain how we identified the two research questions which we then proceed to answer in the two following parts: (i) which factors related to the environment, the season and the climate best determine the foraging activity of bees and (ii) can we consider the attractiveness of cultivated plants as a criterion to help farmers in their decision? To address these questions, we have created a consortium of French apidologists who have developed their answers based on (i) previously published works and (ii) the analysis of their own datasets for which the methods have already been published. The answers will contribute to decision-making criteria for the use of pesticides on flowering plants in crops.
While flies, beetles and butterflies take part in the pollination of cultivated flowering plants (Rader et al., 2016), bees represent the most important group of insect pollinators (Kevan and Baker, 1983). In collecting the pollen and nectar of flowers to feed themselves, and to feed their progeny, they passively transfer pollen between flowers, a phenomenon to which their pollen-harvesting morphology (pollen baskets and brushes) and feather-shaped hairs contribute (Michener, 2007). The biological and ecological traits of about 2000 bee species known in Europe (20.000 in the world) are very diverse in terms of social organization (even though most are solitary), feeding specializations (certain harvest their pollen from a single plant species, others are more generalist), mobility (from a few hundred meters to more than 10 km) and reproductive habitat type (even if the majority are ground nesting). While this wide diversity of bee species may be affected by pesticide use, the Western honey bee Apis mellifera L. is the only species to date considered in the evaluation of toxicity before marketing pesticides (SANCO, 2002). Beyond approval tests for marketing, information about the median lethal dose (LD50) of pesticide active ingredients are mainly available for honey bees, sometimes for bumble bees (Bombus spp.), and much less frequently for other wild pollinators (Lewis et al., 2016; Lewis and Tzilivakis, 2019; Yasrebi-de-Kom et al., 2019). Moreover, wild solitary bees may be more sensitive than honey bees or other large bees (e.g., bumble bee species) to the effects of pesticides (Rundlöf et al., 2015; Azpiazu et al., 2021). For a given active ingredient, toxicity level can strongly vary between species. For instance, the median LD50 by contact for thiamethoxam is 0.28 μg.bee−1 for bumble bees and 0.024 μg.bee−1 for honey bees, while it’s only 0.004 μg.bee−1 for solitary bees (Lewis et al., 2016; Lewis and Tzilivakis, 2019). Consequently, the application conditions of pesticides should consider the diversity of bees.
In addition to work carried out in the United States (Mullin et al., 2010) and in the rest of Europe (Ghini et al., 2004; Bernal et al., 2010), the research shows that honey bees, honey, pollen and wax are regularly contaminated by pesticide residues (Chauzat et al., 2006, 2009; Lambert et al., 2013; Calatayud-Vernich et al., 2018; Tosi et al., 2018; Friedle et al., 2021). These works describe, in particular, considerable contamination by fungicides, insecticides and acaricides in the bees, as well as in the pollen and honey that they consume. The latter may be used by growers to protect their crops and by beekeepers to fight against the ectoparasitic mite Varroa destructor, a major thread for the honey bee colonies. The potential presence of synthetic anti-Varroa acaricide residues in the beeswax of the hive has driven the development of alternative management methods to fight against Varroa (organics acids, essential oils, genetic selection). The work of Lambert et al. (2013) showed that 72% of honey bees sampled (n = 141), 58% of pollen (n = 128) and 95% of honey (n = 14) collected contained at least one pesticide residue in western France. More precisely, five different fungicides were detected in the bees and nine in the pollen and honey. For the insecticides, 11 different residues were detected in the bees, whereas 10 or 15 were found in the pollen and honey, respectively. This interesting study showed that honey bees are regularly exposed to insecticides and the high concentrations measured for certain substances (for example, permethrin: 48 μg/kg per bee) suggest direct exposure to the bees during their foraging activity.
The recent improvement in the sensitivity of chemical analysis methods allows for the detection of multiple substances. These advances allowed for the demonstration that the bees are simultaneously exposed to multiple pesticide residues. For example, Lambert et al. (2013) describe that, on average, honey bees are contaminated by 1.4 pesticides and the most contaminated bees contain up to 6 different pesticide residues. In the United States, extensive contamination was observed, on average, as 2.5 pesticide residues were found per honey bee sample and 25 pesticide residues in the most contaminated sample (Mullin et al., 2010). In France, the food supply of honey bees is also contaminated by multiple residues, as evidenced by studies conducted by regional beekeeping development organizations: 25% of the pollen collected by foragers is contaminated by at least 5 pesticide residues (Vidau, 2015; Table 1).
Table 1. Results of chemical analysis of 165 pollen samples collected in five French regions (Vidau, 2015).
The frequent co-exposure of bees to pesticides, but also to other stress factors (for example, pathogens/pathogenic agents, nutritional deficiencies; Goulson et al., 2015) led the European Authority of Food Safety (EFSA) to recommend that the evaluation procedure for the toxicity of a phytosanitary product, before being marketed, should integrate tests that measure the effect of chemical co-exposure with another compound (ANSES, 2015; EFSA, 2019).
This section illustrates, with examples, that current regulations are not sufficient to protect bees from pesticide exposure. In field conditions, bees are often exposed to mixtures of pesticides, which when applied individually have no toxicity, but might induce negative effects when tested as a cocktail (Prado et al., 2019). We propose that clarifying the criteria to ensure the absence of foragers on flowering crops would reduce this risk.
Bees are commonly exposed to multiple pesticide residues everyday, in particular when they forage on treated crops. One question remains in order to prevent such direct exposures: what factors related to the environment, the season, and the climate, determine bee foraging on crops? Various factors could affect bee foraging activity. Ambient temperature is the meteorological factor most frequently cited in the literature on bee foraging activity. Relative atmospheric humidity shows less of an effect on foraging activity than temperature on honey bee workers’ foraging activity and survival (Joshi and Joshi, 2010; Abou-Shaara et al., 2012, 2017). Overall, honey bees need to maintain their thoracic temperature within a certain range: 31–32°C during foraging (Heinrich, 1979). Beyond the honey bee, each bee species has specific ambient temperature thresholds (min. and max.) to maintain the body temperature and withstand the necessary energy costs (Stone, 1994). To do so, the body mass is a determining factor in insect thermoregulation (Heinrich, 1979; Stone et al., 1988; Herrera, 1990). The body temperature is greatly influenced by wind speeds and even a small increase in wind speed can result in a lowering of this parameter (Digby, 1955; Church, 1960). Hennessy et al. (2020) found an increase in wind speed of just 2.75 m/s resulted in a 37% decrease in floral visits. It is commonly accepted that more foragers are observed at wind speeds below 4 m/s (Rollin et al., 2013). In this article, we do not address in further depth the interest of wind speed as a criterion to reduce the risk of spraying pesticides, because these sprays are forbidden in the case of strong winds.
Foraging activity of honey bees (number of flights per time unit) takes place within a wide range of temperatures, from 10 to 40°C (Abou-Shaara, 2014). Clarke and Robert (2018) found that 78% of the observed variations in honey bee activity was explained by variations in temperature and solar radiation related to cloud cover. The temperature threshold below which the honey bee can no longer forage varies according to the source: 6°C according to Tan et al. (2012), 7°C from Heinrich (1979), 9°C from Burrill and Dietz (1981), 12°C from Danka et al. (2006), and 16°C in tropical areas according to Joshi and Joshi (2010). Tan et al. (2012) observed an optimal foraging activity at around 20°C. For Burrill and Dietz (1981), the 9°C threshold, below which the honey bee does not fly, was independent of luminosity.
Corbet et al. (1993) studied the effect of climate on the foraging activity of different species of social bees: Apis mellifera and 5 species of bumble bees (Bombus lapidarius, B. terrestris, B. lucorum, B. pascuorum et B. hortorum). Corbet et al. (1993) and more recently Sanderson et al. (2015) and Clarke and Robert (2018) showed that the ambient temperature and the level of radiation were positively correlated with the foraging activity of these social bee species. These results were confirmed in Portugal and in the United Kingdom on phacelia and on flowering shrubs. Workers of A. mellifera and B. lapidarius start foraging at lower temperatures than workers of B. terrestris, B. lucorum, B. pascuorun and B. horotorum. Compared to the Western honey bee, most species of bumble bee are indeed known for having flying activity at lower ambient temperatures (Lundberg, 1980; Stone and Willmer, 1989; Corbet et al., 1993).
Some studies investigated the effects of multiple environmental variables on the foraging activity of honey bees. For instance, Burrill and Dietz (1981) showed correlations between foraging activity and temperature and solar radiation, two parameters that change inversely with relative humidity and atmospheric pressure. Overall, the optimal range of temperature for the foraging activity of the honey bee species (Apis cerana, A. dorsata and A. mellifera) ranges from 21.0 to 33.5°C (Usha and Devi, 2020). While foraging activity increases in a linear manner with temperature between 13 and 23°C, independently of the luminosity, other authors showed a quadratic effect of temperature on the foraging activity in the same Apis species (Danka et al., 2006; Abou-Shaara et al., 2012). In particular, the foraging activity increases with temperature up to 24°C and then decreases up to 30°C. A study on oilseed rape (Brassica napus) showed that between 27 and 45°C, the density of honey bees decreased as the temperature increased (Blažytė-Čereškienė et al., 2010). This inhibitory effect of rising temperatures would be particularly observed in pollen foragers (Cooper and Schaffer, 1985). It was also observed that the foraging activity of non-Apis pollinators (i.e., non-Apis bees and Syrphid flies) decreases with temperature, especially above 33°C (Usha and Devi, 2020).
New statistical analyses of the dataset of Rollin et al. (2013, 2019) show a significant nonlinear influence of temperature on the presence and abundance of bees in the sampled sites (red curves: Figure 1). In addition, the intensity of this effect varies as a function of the bee group considered (i.e., honey bees, bumble bees, and solitary bees) and the sampling period (April, May–June, July, September). In this study, field inventories of bee species (honey bees, bumble bees and solitary bees) were undertaken on different flowering plant covers and crops at the Plaine and Val de Sèvre (ZAPVS) research site, in the Poitou-Charentes region of France. Collections were conducted at an ambient temperature of ≥16°C and wind speed of ≤15 km/h (Rollin et al., 2013, 2019). The abundance of honey bee foragers (and of all bees) measured on flowers decreased at the highest temperatures. This effect seems less obvious for the bumble bees and solitary bees. The results from a study on the solitary bee species Anthophora pauperata follow the same trend. Increasing ambient temperature up to 25–30°C at midday did not lead to a reduction in foraging activity (Stone et al., 1999). On the other hand, a study on foraging by the bumble bee Bombus terrestris conducted in the greenhouse at high temperatures showed that the intensity of colony exit rates and the foraging activity of workers peaked at 25.7°C (during the morning). However, at an average temperature of 32.3°C, foraging activity decreased significantly by 70% and colony entrance traffic by 40% (Kwon and Saeed, 2003).
Figure 1. Abundance of bees on blooming plants as a function of ambient temperature. The x-axis is expressed in °C. The graphs show the abundance of all bees (A), managed bees (B), bumble bees (C) and wild bees other than bumblebees (D). The points illustrate abundance data and the red curve illustrates the result of the mathematical model linking abundance data to temperature (polynomial function; Rollin et al., 2013).
Devillers et al. (2004) used co-inertia analyses to look for statistical links between data on hive exit activity, recorded using an electronic counter, and temperature, overall sunlight, humidity, wind, and rain in the Rhone-Alpes region (France) between July and September. The existence of a co-structure between hive exit activity over 24 h and the temperature or overall sunlight was clearly established.
In the ZAPVS research site, the flying activity of thousands of honey bee workers was recorded using Radio Frequency Identification (RFID) from April to August. This life-long monitoring of bees records two bee life history traits, namely the number of exits per day and the duration of these trips (Requier et al., 2020). Similar to the previous study, flight activity (reflected by these two life traits) was significantly affected by the temperature and time of year (month). Exit activity is positively correlated with daily average temperature (Figure 2). But surprisingly, the exit activity recorded in September was not affected by temperature, i.e., the number of exits did not changed whether it was 12°C or 22°C. This month also showed a lesser effect of temperature on the duration of worker trips. One explanation of this activity pattern could be that this time of year (i.e., fall in September) was particularly dedicated to food reserve storage by the colonies in preparation for over-wintering. Interestingly, the daily flight activity of honey bees varied across the season (Figure 3). June is the period with longer daily activity, likely due to the fact that this month has a longer duration of daily sunlight. Another explanation relates to the foraging effort. Indeed, June is the period of food shortage for bees in such an intensive farming system (Requier et al., 2015, 2017; Timberlake et al., 2019), potentially affecting the need to increase the daily activity of foraging.
Figure 2. Mathematical model that predicts the number of honey bee exits from the hive according to ambient temperature. The curves depict the results of linear models, all significant, established based on recordings of RFID transponder-labelled workers (n = 1,330 bees in total; Requier, 2013). (A) Number of exits by bee and by day. (B) Average duration of trips by bee and by day in second. The amplitude of daily temperatures recorded in April, May, June, July, August, and September were, respectively, 10.2–20.7, 12.9–27.6, 13.7–27.6, 15.1–26.1, 13.3–26.1, and 12.0–22.3°C. The number of recorded bees per month ranged from 90 to 308.
Figure 3. Honey bee exit activity from the hive by time of day. The circles depict the 24 h of a day during which we recorded trips of RFID transponder-labeled workers (n = 1,330 bees in total introduced at the beginning of every month between April and September by cohort of 90–308 individuals). The circle shows the maximum exit activity recorded per hour in the month (baseline from which the exit rate at other hours was calculated). The hours are those recorded by the RFID device- embedded computer (Requier, 2013).
If ambient temperature is the most frequently cited criterion, the literature shows that it is difficult to determine a threshold to protect all bee species from pesticide spraying.
Another question concerns the relationship between bee species and crops. Wildlife inventories carried out from April to August over 3 years (2010–2012) in the ZAPVS research site consisted of capturing and identifying to species around 30,000 bees foraging on flowers and on more than 800 plant covers (Rollin et al., 2013). The results revealed clear differences in the use of floral resources between groups of bees during the flowering of rapeseed and sunflower. Honey bees, and to a lesser extent, bumble bees, prefer to forage the flowers of rapeseed and sunflower over the wildflowers in the meadows and roadsides, whereas the opposite is true for solitary bees (Figure 4). Solitary bees were observed in greater numbers on wildflowers in meadows and edges, than in the rapeseed and sunflower fields. The diversity of bees on rapeseed measured 4 times less than that on the natural, herbaceous flora (Rollin et al., 2015). In contrast, bumble bees were more present on the flowers of oilseed crops than the other floral covers, but less markedly than the honey bees.
Figure 4. Average number of foraging bees according to position in the rapeseed plot. The bars represent the standard error. B: border, 5: at 5 m from the border, C: center of the field plot (method described in Le Féon, 2010).
The wild bees use the crops as a food resource, like honey bees, but also as a nesting resource. In fact, certain ground-nesting bee species may nest in the soil within the crops (Shuler et al., 2005; Esther and Roulston, 2009). Nesting in the crop fields should be considered, because around 80% of solitary bees are ground-nesting species (Antoine and Forrest, 2021).
Nutritional resources, such as nectar, pollen, guttation drops, honeydew, and water, are found in cultivated fields and can be harvested by bees. To our knowledge, the use of the latter three resources by the bee has never been sufficiently studied to assess their use compared to the variability of factors such as the type of plant cultivated, the weather, the time of day, or the season.
For instance, in France, the ITSAP institute database2, based on specialized expertise, yields a honey bee attractivity index for almost 50 crops, cover crops or mixture for honey-fallows. This database attributes three scores: weakly or not attractive (1), somewhat attractive (2), and very attractive (3). But this approach veers far from the reality since crop visits by bees show a variability that these qualitative values miss. Incidentally the quantitative data available on the nectar-producing potential of a plant species (i.e., the expected value of honey production for a given plant species in kilograms per unit of surface area) sufficiently convey this variability (Ion et al., 2018). For the cultivated species, the available databases show wide variability in their estimations:
- for oilseed rape (Brassica napus L.), the minimum noted is 67.5 kg/ha and the maximum is 325 kg/ha, a factor of approximately 5 between the two estimated values (Pierre et al., 1999; Ion et al., 2012). Baude et al. (2016) indicated an average of nectar sugar content of 394 µg/flower/day, with a standard deviation of 301;
- for pear (Pyrus communis L.), a species grown in different regions (flowering in March–April), bibliographic sources show nectar-producing potential ranging by a factor of 9 depending on the author – from 6 to 50 kg/ha, from Koltowski (2006) and Janssens et al. (2006), respectively;
- concerning cornflower (Cyanus segetum L.), a volunteer, crop-associated Asteraceae that flowers in June, its honey-producing potential is estimated between 60 kg/ha and 350 kg/ha (Koltowski, 2006), a difference of a factor of 6.
This variability can be explained by the method used, but also by the health and developmental state of the bees and environmental, agronomic (agricultural practices, crop variety), and pedoclimatic conditions (Ion et al., 2018). Another major limit of the indicator is that it does not integrate visits to plants by the bees for pollen. Additionally, the visit of crops by bees depends on other flowering plants available nearby (Henry et al., 2012; Rollin et al., 2013). A plant with an attractiveness labeled as medium will be better visited in the absence of alternative flowers.
We previously saw that honey bees visit corn plots for the pollen of the farmed crops, but also use the weeds found in the crop. The ranking of cultivated plants, by their probable visitation by honey bees, has its limits, given that a farmed field, that produces no nectar nor pollen, can host attractive wild plant species. For example, the pollen of annual mercury (Mercurialis annua L.), a weed often observed in corn (Zea mays L.) crops, is consistently found in forager pollen pellets in the summer (up to 15% of the supply). But bees gather pollen from spontaneous plants in a variety of crops, not just corn (Bretagnolle and Gaba, 2015). This use is surprising in terms of the diversity of plants used, in frequency, and in the amount of pollen brought back to the hive. As such, 96 different pollens were catalogued in the samples from the ECOBEE long-term bee colony monitoring (Odoux et al., 2014) representing more than 30% of pollen yields during the corn flowering season (Requier et al., 2015).
A crop of cereal straw, having little interest in and of itself, will likely be visited by bee foragers if it contains weeds such as cornflower (Centaurea cyanus L.) or poppy (Papaver rhoeas L.). Poppy pollen can represent, in intensive cereal farming systems, 10% of the biomass of pollen harvested by honey bee colonies from April to September, making it the second-most harvested pollen after maize pollen (Requier, 2013; Requier et al., 2015). But the importance of the resources offered by weeds in crop fields is not reserved to intensive cereal farming systems. In July 2013, multiple pollen samplings were undertaken in an observation study during the lavender nectar flow using pollen traps (n = 5) and following eight apiaries in the Provence Drôme region (France). Palynological analysis of each sample revealed that the majority of pollen does not belong to the cultivated plants but to semi-natural (or volunteer) flora found in the inter-row areas. More precisely, the species of the genus Asteraceae (40%) and the herb plantain (Plantago spp.; 15%) are the most used floral species by the bees in a landscape that is primarily composed of vineyards (Vitis vinifera L.), cereal and lavender (Lavendula spp.). This pollen supply proved to be contaminated by 40 pesticide residues. Thus, taking into account the nature of the recovered pesticide residues and the application treatment calendars used during the month of July in this region, these observations suggest that the bees are overwhelmingly exposed to pesticides applied on the grapevines that contaminate the wildflowers traditionally found in the inter-row areas and on the field edges.
The spatial distribution of foragers within the farmed plots is a frequently asked question by farmers. Measuring the foraging of honey bees along a distance gradient from the border of a field to its center, Sáez et al. (2012) found a reduction of 25% in the number of visits on capitulum inflorescences between 1 and 100 m. The density of the foragers is up to three times lower in the central rows of corn crop field than in the border rows (Thibord et al., 2015). But an analysis of these data calculating the number of foragers present on the surfaces shows that the center (surface subtracted from those corresponding to 8 m from border rows) of 10, 50, and 100 ha corn crop fields could host 75, 88, and 91% of observed, respectively. This result is also found in oilseed rape where the total abundance of pollinators, including the Western honey bee, syrphids and bumble bees (but not solitary bees), is greater in the interior of the fields than at the edges (Figure 4). The spatial distribution of bumble bee foragers favoring the center of the oilseed rape fields observed in France (research site: Pleine-Fougères, Bretagne) was confirmed by results obtained in Denmark (Calabuig, 2000); in this latter study, on the other hand, solitary bees were more abundant at the edges of the field. This result was confirmed in a study in France in which the distance to the field edge had a negative effect on the abundance of solitary bees such as the andrenids and Nomada species in oilseed rape fields (Bailey et al., 2014). These authors highlight a significant effect of the intertegular distance (the distance separating the base of the two wings of the bee) on the spatial distribution of different species of bees within the oilseed rape fields. This morphological trait measurement reflects the size of the individual and corresponds to an estimation of their flight capacity (Greenleaf et al., 2007). Larger bees (such as bumble bees) have a tendency to fly further into the middle of the rapeseed fields than smaller bees (such as Andrena and Nomada).
Whereas in many countries the spraying of insecticides and acaricides on flowering plants or during the foraging activity of bees is regulated, it is necessary to identify the decision-making criteria that could be used by farmers to protect bees. One option would be to choose a criterion depending on the crop type. For this, data are available for attributing a degree of visitation by the honey bee to cultivated plants (attractivity score, melliferous, or polliferous, potential). Offering crop-specific recommendations for pesticide use under all conditions has limits, for the following reasons:
- the degree of crop visitation has not been documented for wild bees as a whole;
- multiple factors (other than those related to the bee itself) will modulate bee visitation (crop variety, pedoclimate, cultivation practices, health status of the bees, presence of other flowers, pollinator diversity and bioagressors);
- the natural flora present in or near the farmed plots also represent a mode of pesticide exposure for the bees, managed or wild;
- concerning wild bees, they must have access to perennial nesting sites in agricultural landscapes, both for ground-nesting species (with appropriate undisturbed soil) and above ground-nesting species (e.g., with ligneous structures for stem-nesting species or leaf-cutter bees).
- As of today no criterion has been established that is true for all crops and bee species regarding the spatial distribution of foragers within plots. Such a criterion would null and void any decision-making treatment criterion that is based on the area to treat (for example, « do not treat x rows of crop y when flowering).
A second option that has been advanced is to employ meteorological criteria (for example, “treat if the temperature is lower than X°C”). While the temperature remains the meteorological parameter that explains a large part of the variability of foraging activity in bees, the notion of temperature threshold, beyond which this activity would be absent, is jeopardized by the significant effect of other factors related to the bees themselves (species, health, and developmental cycle) or to luminosity, wind, environment (quality, quantity, and location of resources), season (sunlight duration) and time of day (e.g., Burrill and Dietz, 1981; Woyke et al., 2003; Danka et al., 2006; Clarke and Robert, 2018). Our current knowledge is not sufficient at this time to define decision-making criteria that would combine these parameters together. For this reason, decisions based on meteorological criteria seem inappropriate to us.
French regulations stipulate that insecticides or acaricides bearing the « Bee label » have an exemption that allows for their application on flowering plants, provided that foragers are absent from the treated plots. For that, spraying only once the sun has set would significantly reduce the risk exposure of foragers to phytosanitary products. The goal of these regulations is to protect all bee species, namely managed honey bees raised by beekeepers, but also wild bees. Around 80% of bees are ground-nesting species, some of which may be affected by pesticide spraying on crops (Kim et al., 2006; Esther and Roulston, 2009), even if carried out at night. Moreover, nocturnal pollinators, which contribute to pollen transport of plants in agrosystems (Walton et al., 2020), would remain exposed to pesticides. The night spraying of pesticides will also not prevent the contamination of nectar and pollen that induces oral exposure of bees (Rortais et al., 2017) and the degradation of the quality of hive products (Mukherjee, 2009).
For some crops, the constraints on farmers related to the nocturnal spraying of pesticides on flowering plants are high: problems of safety at work, strain at work, and nuisance for the neighboring areas. Scientists need to prove the feasibility and sustainability of new practices with nocturnal application of pesticides. For that, they must test new work organizations with a research-intervention approach based on the cooperation of farmers.
Safeguarding bee biodiversity should be a priority, and thus, in the absence of less-dangerous alternatives to pesticides, the spraying of pesticides should be carried out at night, even if the modalities of acceptable application by farmers often remain to be defined.
AD: coordination of the article. OR, FR, and CR: data acquisition and development of statistics and figures. FA, CV, OR, FR, CR, and MH: proofreading and expertise. FA, CV, OR, FR, CR, and MH: list of bibliographic references. AD, FA, and MH: coordination of projects producing the data. All authors contributed to the article and approved the submitted version.
The data used in this study were financed by the French Ministry of Food and Agriculture via the funding program CASDAR (POLINOV, RESAPI, INTERAPI projects) by the France AgriMer-EU Community Program for Beekeeping 2013-2016 (French Bee Institute database and APIMODEL project).
We thank Cynthia McDonnell for the proofreading of the manuscript.
The authors declare that the research was conducted in the absence of any commercial or financial relationships that could be construed as a potential conflict of interest.
All claims expressed in this article are solely those of the authors and do not necessarily represent those of their affiliated organizations, or those of the publisher, the editors and the reviewers. Any product that may be evaluated in this article, or claim that may be made by its manufacturer, is not guaranteed or endorsed by the publisher.
Abou-Shaara, H. (2014). The foraging behaviour of honey bees, Apis mellifera: a review. Veterinarni Medicina 59, 1–10. doi: 10.17221/7240-VETMED
Abou-Shaara, H. F., Al-Ghamdi, A. A., and Mohamed, A. A. (2012). Tolerance of two honey bee races to various temperature and relative humidity gradients. Environ. Exp. Biol. 10, 133–138. doi: 10.3954/JAUE14-05.1
Abou-Shaara, H. F., Owayss, A. A., Ibrahim, Y. Y., and Basuny, N. K. (2017). A review of impacts of temperature and relative humidity on various activities of honey bees. Insect. Soc. 64, 455–463. doi: 10.1007/s00040-017-0573-8
ANSES (2015). Co-exposition des abeilles aux facteurs de stress. Avis de l’Anses - Rapport d’expertise collective. Available at: https://www.anses.fr/fr/content/avis-et-rapport-de-lanses-relatifs-aux-co-expositions-des-abeilles-aux-facteurs-de-stress
Antoine, C. M., and Forrest, J. R. (2021). Nesting habitat of ground-nesting bees: a review. Ecol. Entomol. 46, 143–159. doi: 10.1111/een.12986
Azpiazu, C., Bosch, J., Bortolotti, L., Medrzycki, P., Teper, D., Molowny-Horas, R., et al. (2021). Toxicity of the insecticide sulfoxaflor alone and in combination with the fungicide fluxapyroxad in three bee species. Sci. Rep. 11:6821. doi: 10.1038/s41598-021-86036-1
Bailey, S., Requier, F., Nusillard, B., Roberts, S. P., Potts, S. G., and Bouget, C. (2014). Distance from forest edge affects bee pollinators in oilseed rape fields. Ecology and Evolution 4, 370–380. doi: 10.1002/ece3.924
Baude, M., Kunin, W. E., Boatman, N. D., Conyers, S., Davies, N., Gillespie, M. A., et al. (2016). Historical nectar assessment reveals the fall and rise of floral resources in Britain. Nature 530, 85–88. doi: 10.1038/nature16532
Bernal, J., Garrido-Bailon, E., Del Nozal, M. J., Gonzalez-Porto, A. V., Martin-Hernandez, R., Diego, J. C., et al. (2010). Overview of pesticide residues in stored pollen and their potential effect on bee colony (Apis mellifera) losses in Spain. J. Econ. Entomol. 103, 1964–1971. doi: 10.1603/EC10235
Blažytė-Čereškienė, L., Vaitkevičienė, G., Venskutonytė, S., and Būda, V. (2010). Honey bee foraging in spring oilseed rape crops under high ambient temperature conditions. Žemdirbystė 97, 61–70.
Bretagnolle, V., and Gaba, S. (2015). Weeds for bees? A review. Agron. Sustain. Dev. 35, 891–909. doi: 10.1007/s13593-015-0302-5
Burrill, R. M., and Dietz, A. (1981). The response of honey bees to variations in solar radiation and temperature. Apidologie 12, 319–328. doi: 10.1051/apido:19810402
Calabuig, I. (2000). Solitary Bees and Bumble Bees in a Danish Agricultural Landscape. Dissertation. Denmark: University of Copenhagen, Zoological Institute.
Calatayud-Vernich, P., Calatayud, F., Simó, E., and Picó, Y. (2018). Pesticide residues in honey bees, pollen and beeswax: assessing beehive exposure. Environ. Pollut. 241, 106–114. doi: 10.1016/j.envpol.2018.05.062
Chauzat, M. P., Carpentier, P., Martel, A. C., Bougerard, S., Cougoule, N., and Porta, P. (2009). Influence of pesticide residue on honey bee (hymenoptera: Apidae) colony health in France. Environ. Entomol. 38, 514–523. doi: 10.1603/022.038.0302
Chauzat, M. P., Faucon, J. P., Martel, A. C., Lachaieze, J., Cougoule, N., and Aubert, M. (2006). A survey of pesticide residues in pollen loads collected by honey bees in France. J. Econ. Entomol. 99, 253–262. doi: 10.1093/jee/99.2.253
Church, N. S. (1960). Heat loss and the body temperatures of flying insects. II. Heat conduction within the body and its loss by radiation and convection. J. Exp. Biol. 37, 186–212. doi: 10.1242/jeb.37.1.186
Clarke, D., and Robert, D. (2018). Predictive modelling of honey bee foraging activity using local weather conditions. Apidologie 49, 386–396. doi: 10.1007/s13592-018-0565-3
Cooper, P. D., and Schaffer, W. M. (1985). Temperature regulation of honey bees (Apis mellifera) foraging in the Sonoran desert. J. Exp. Biol. 114, 1–15. doi: 10.1242/jeb.114.1.1
Corbet, S. A., Fussell, M., Ake, R., Fraser, A., Gunson, C., Savage, A., et al. (1993). Temperature and the pollinating activity of social bees. Ecol. Entomol. 18, 17–30. doi: 10.1111/j.1365-2311.1993.tb01075.x
Danka, R. G., Sylvester, H. A., and Boykin, D. (2006). Environmental influences on flight activity of USDA-ARS Russian and Italian stocks of honey bees (hymenoptera: Apidae) during almond pollination. J. Econ. Entomol. 99, 1565–1570. doi: 10.1603/0022-0493-99.5.1565
Decourtye, A., Alaux, C., Le Conte, Y., and Henry, M. (2019). Toward the protection of bees and pollination: the rise of global change science. Curr. Opin. Insect Sci. 35, 123–131. doi: 10.1016/j.cois.2019.07.008
Devillers, J., Morlot, M., Pham-Delegue, M. H., and Dore, J. C. (2004). Classification of monofloral honeys based on their quality control data. Food Chem. 86, 305–312. doi: 10.1016/j.foodchem.2003.09.029
Digby, P. S. (1955). Factors affecting the temperature excess of insects in sunshine. J. Exp. Biol. 32, 279–298. doi: 10.1242/jeb.32.2.279
EFSA (2012). Scientific opinion on the science behind the development of a risk assessment of plant protection products on bees (Apis mellifera, Bombus spp. and solitary bees). EFSA J. 10:2668. doi: 10.2903/j.efsa.2012.2668
EFSA (2019). Technical report of the public consultation on the draft ‘guidance on harmonised risk assessment methodologies for human health, animal health and ecological risk assessment of combined exposure to multiple chemicals’. EFSA J. doi: 10.2903/sp.efsa.2019.EN-1589
EPPO (2010). Side-effects on honey bees. Efficacy evaluation of plant protection products. Bull OEPP/EPPO Bull. 40, 323–331. doi: 10.1111/j.1365-2338.2010.02418.x
Esther, H. J., and Roulston, T. A. H. (2009). Wild bee abundance and pollination service in cultivated pumpkins: farm management, nesting behavior and landscape effects. J. Econ. Entomol. 102, 563–573. doi: 10.1603/029.102.0214
Friedle, C., Wallner, K., Rosenkranz, P., Martens, D., and Vetter, W. (2021). Pesticide residues in daily bee pollen samples (April–July) from an intensive agricultural region in southern Germany. Environ. Sci. Pollut. Res. 28, 22789–22803. doi: 10.1007/s11356-020-12318-2
Ghini, S., Fernandez, M., Pico, Y., Marin, R., Fini, F., Manes, J., et al. (2004). Occurrence and distribution of pesticides in the province of Bologna, Italy, using honeybees as bioindicators. Arch. Environ. Contam. Toxicol. 47, 479–488. doi: 10.1007/s00244-003-3219-y
Goulson, D., Nicholls, E., Botías, C., and Rotheray, E. L. (2015). Bee declines driven by combined stress from parasites, pesticides, and lack of flowers. Science 347:6229. doi: 10.1126/science.12559
Greenleaf, S., Williams, N., Winfree, R., and Kremen, C. (2007). Bee foraging ranges and their relationship to body size. Oecologia 153, 589–596. doi: 10.1007/s00442-007-0752-9
Heinrich, B. (1979). Thermoregulation of African and European honeybees during foraging, attack, and hive exits and returns. J. Exp. Biol. 80, 217–229. doi: 10.1242/jeb.80.1.217
Hennessy, G., Harris, C., Eaton, C., Wright, P., Jackson, E., Goulson, D., et al. (2020). Gone with the wind: effects of wind on honey bee visit rate and foraging behaviour. Anim. Behav. 161, 23–31. doi: 10.1016/j.anbehav.2019.12.018
Henry, M., Fröchen, M., Maillet-Mezeray, J., Breyne, E., Allier, F., Odoux, J.-F., et al. (2012). Spatial autocorrelation in honeybee foraging activity reveals optimal focus scale for predicting agro-environmental scheme efficiency. Ecol. Model. 225, 103–114. doi: 10.1016/j.ecolmodel.2011.11.015
Herrera, C. M. (1990). Daily patterns of pollinator activity, differential pollinating effectiveness, and floral resource availability, in a summer-flowering Mediterranean shrub. Oikos 58, 277–288. doi: 10.2307/3545218
Ion, N., Ion, V., Coman, R., and Băşa, A. G. (2012). Studies concerning nectar secretion at rapeseed (Brassica napus L. ssp. oleifera D.C.). Sci. Papers A Agron. 55, 162–169.
Ion, N., Odoux, J.-F., and Vaissière, B. E. (2018). Melliferous potential of weedy herbaceous plants in the crop fields of Romania from 1949 to 2012. J. Apicult. Sci. 62, 149–165. doi: 10.2478/jas-2018-0017
Janssens, X., Bruneau, E., and Lebrun, P. (2006). Prévision des potentialités de production de miel à l’échelle d’un rucher au moyen d’un système d’information géographique. Apido 37, 351–365. doi: 10.1051/apido:2006006
Joshi, N. C., and Joshi, P. C. (2010). Foraging behaviour of Apis spp. on apple lowers in a subtropical environment. N. Y. Sci. J. 3, 71–76.
Kevan, P. G., and Baker, H. G. (1983). Insects as flower visitors and pollinators. Annu. Rev. Entomol. 28, 407–453. doi: 10.1146/annurev.en.28.010183.002203
Kim, J., Williams, N., and Kremen, C. (2006). Effects of cultivation and proximity to natural habitat on ground-nesting native bees in California sunflower fields. J. Kansas Entomol. Soc. 79, 309–320. doi: 10.2317/0507.11.1
Koltowski, Z. (2006). Beekeeping value of sunflower plantation. International Apicultural Scientific Conference in centenary of Jan Dzierzon’s death, Pulawy, April 25–27, 169.
Kwon, Y. J., and Saeed, S. (2003). Effect of temperature on the foraging activity of Bombus terrestris L. (hymenoptera: Apidae) on greenhouse hot pepper (Capsicum annuum L.). Appl. Ent. Zool. 38, 275–280. doi: 10.1303/aez.2003.275
Lambert, O., Piroux, M., Puyo, S., Thorin, C., L’Hostis, M., and Wiest, L. (2013). Widespread occurrence of chemical residues in beehive matrices from apiaries located in different landscapes of Western France. PLoS One 8:e67007. doi: 10.1371/journal.pone.0067007
Le Féon, V. (2010). Insectes pollinisateurs dans les paysages agricoles: approche pluri-échelle du rôle des habitats semi-naturels, des pratiques agricoles et des cultures entomophiles. Thèse de doctorat, Université européenne de Bretagne, Rennes, 257 p. Available at: https://www.theses.fr/2010REN1S131
Lewis, K. A., and Tzilivakis, J. (2019). Wild bee toxicity data for pesticide risk assessments. Data 4:98. doi: 10.3390/data4030098
Lewis, K. A., Tzilivakis, J., Warner, D. J., and Green, A. (2016). An international database for pesticide risk assessments and management. Human and ecological risk assessment. Int. J. 22, 1050–1064. doi: 10.1080/10807039.2015.1133242
Lundberg, H. (1980). Effects of weather on foraging-flights of bumblebees (hymenoptera, Apidae) in a subalpine/alpine area. Ecography 3, 104–110. doi: 10.1111/j.1600-0587.1980.tb00715.x
Mancini, F., Woodcock, B. A., and Isaac, N. J. B. (2019). Agrochemicals in the wild: identifying links between pesticide use and declines of nontarget organisms. Curr. Opin. Environ. Sci. Health Environ. Poll. 11, 53–58. doi: 10.1016/j.coesh.2019.07.003
Mukherjee, I. (2009). Determination of pesticide residues in honey samples. Bull. Environ. Contam. Toxicol. 83, 818–821. doi: 10.1007/s00128-009-9772-y
Mullin, C. A., Frazier, M., Frazier, J. L., Ashcraft, S., Simonds, R., vanEngelsdorp, D., et al. (2010). High levels of miticides and agrochemicals in north American apiaries: implications for honey bee health. PLoS One 5:e9754. doi: 10.1371/journal.pone.0009754
Odoux, J. F., Aupinel, P., Gateff, S., Requier, F., Henry, M., and Bretagnolle, V. (2014). ECOBEE: a tool for long-term bee colony monitoring at landscape scale in west European intensive agrosystems. J. Apic. Res. 53, 57–66. doi: 10.3896/IBRA.1.53.1.05
Pierre, J., Mesquida, J., Marilleau, R., Pham-Delègue, M. H., and Renard, M. (1999). Nectar secretion in winter oilseed rape (Brassica napus): quantitative and qualitative variability among 71 genotypes. Plant Breeding 118, 471–476.
Prado, A., Pioz, M., Vidau, C., Requier, F., Jury, M., Crauser, D., et al. (2019). Exposure to pollen-bound pesticide mixtures induces longer-lived but less efficient honey bees. Sci. Total Environ. 650, 1250–1260. doi: 10.1016/j.scitotenv.2018.09.102
Rader, R., Bartomeus, I., Garibaldi, L. A., Garratt, M. P. D., Howlett, B. G., Winfree, R., et al. (2016). Non-bee insects are important contributors to global crop pollination. Proc. Natl. Acad. Sci. U. S. A. 113, 146–151. doi: 10.1073/pnas.1517092112
Requier, F. (2013). Dynamique spatiotemporelle des ressources florales et écologie de l’abeille domestique en usage agricole intensif. Thèse de doctorat, Université de Poitiers, 202 p. Available at: http://www.theses.fr/2013POIT2325
Requier, F., Henry, M., Decourtye, A., Brun, F., Aupinel, P., Rebaudo, F., et al. (2020). Measuring ontogenetic shifts in central-place foragers: a case study with honey bees. J. Animal Ecol. 89, 1860–1871. doi: 10.1111/1365-2656.13248
Requier, F., Odoux, J. F., Henry, M., and Bretagnolle, V. (2017). The carry-over effects of pollen shortage decrease the survival of honeybee colonies in farmlands. J. Appl. Ecol. 54, 1161–1170. doi: 10.1111/1365-2664.12836
Requier, F., Odoux, J.-F., Tamic, T., Moreau, N., Henry, M., Decourtye, A., et al. (2015). Honey bee diet in intensive farmland habitats reveals an unexpectedly high flower richness and a major role of weeds. Ecol. Appl. 25, 881–890. doi: 10.1890/14-1011.1
Rollin, O., Bretagnolle, V., Decourtye, A., Aptel, J., Michel, N., Vaissière, B. E., et al. (2013). Differences of floral resource use between honey bees and wild bees in an intensive farming system. Agric. Ecosyst. Environ. 179, 78–86. doi: 10.1016/j.agee.2013.07.007
Rollin, O., Bretagnolle, V., Fortel, L., Guilbaud, L., and Henry, M. (2015). Habitat, spatial and temporal drivers of diversity patterns in a wild bee assemblage. Biodivers. Conserv. 24, 1195–1214. doi: 10.1007/s10531-014-0852-x
Rollin, O., Pérez-Méndez, N., Bretagnolle, V., and Henry, M. (2019). Preserving habitat quality at local and landscape scales increases wild bee diversity in intensive farming systems. Agric. Ecosyst. Environ. 275, 73–80. doi: 10.1016/j.agee.2019.01.012
Rortais, A., Arnold, G., Dorne, J. L., More, S. J., Sperandio, G., Streissl, F., et al. (2017). Risk assessment of pesticides and other stressors in bees: principles, data gaps and perspectives from the European food safety authority. Sci. Total Environ. 587-588, 524–537. doi: 10.1016/j.scitotenv.2016.09.127
Rundlöf, M., Andersson, G. K. S., Bommarco, R., Fries, I., Hederstrom, V., Herbertsson, L., et al. (2015). Seed coating with a neonicotinoid insecticide negatively affects wild bees. Nature 521, 77–80. doi: 10.1038/nature14420
Sáez, A., Sabatino, M., and Aizen, M. A. (2012). Interactive effects of large- and small-scale sources of feral honey-bees for sunflower in the argentine pampas. PLoS One 7:e30968. doi: 10.1371/journal.pone.0030968
SANCO (2002). Guidance Document on Terrestrial Ecotoxicology Under Council Directive 91/414/EEC. Sanco/10329/2002 rev 2. Brussel: European Commission, Health and Consumer Protection Directorate-General.
Sanderson, R. A., Goffe, L. A., and Leifert, C. (2015). Time-series models to quantify short-term effects of meteorological conditions on bumblebee forager activity in agricultural landscapes. Agric. For. Entomol. 17, 270–276. doi: 10.1111/afe.12102
Sgolastra, F., Medrzycki, P., Bortolotti, L., Maini, S., Porrini, C., Simon-Delso, N., et al. (2020). Bees and pesticide regulation: lessons from the neonicotinoid experience. Biol. Conserv. 241:108356:108356. doi: 10.1016/j.biocon.2019.108356
Shuler, R., Roulston, T., and Farris, G. (2005). Farming practices influence wild pollinator populations on squash and pumpkin. J. Eco. Entomol. 98, 790–795. doi: 10.1603/0022-0493-98.3.790
Stone, G. N. (1994). Activity patterns of females of the solitary bee Anthophora plumipes in relation to temperature, nectar supplies and body size. Ecol. Entomol. 19, 177–189. doi: 10.1111/j.1365-2311.1994.tb00408.x
Stone, G. N., Amos, J. N. N., Stone, T. F., Knight, R. L., Gay, H., and Parrott, F. (1988). Thermal effects on activity patterns and behavioural switching in a concourse of foragers on Stachytarpheta mutabilis in New Guinea. Oecologia 77, 56–63. doi: 10.1007/BF00380925
Stone, G. N., Gilbert, F., Willmer, P. G., Potts, S., Semida, F., and Zalat, S. (1999). Windows of opportunity and the temporal structuring of foraging activity in a desert solitary bee. Ecol. Entomol. 24, 208–221. doi: 10.1046/j.1365-2311.1999.00181.x
Stone, G. N., and Willmer, P. G. (1989). Warm-up rates and body temperatures in bees: the importance of body size, thermal regime and phylogeny. J. Exp. Biol. 147, 303–328. doi: 10.1242/jeb.147.1.303
Tan, K., Yang, M., Wang, Z., Radloff, S. E., and Pirk, C. W. W. (2012). The pheromones of laying workers in two honeybee sister species: Apis cerana and Apis mellifera. J. Comp. Physiol. A 198, 319–323. doi: 10.1007/s00359-012-0710-9
Thibord, J. B., Marques, M., Dionisi, M., and Bretagnolle, V. (2015). Quand les abeilles vont-elles dans le maïs? Phytoma La Santé des Végétaux 681, 39–43.
Timberlake, T. P., Vaughan, I. P., and Memmott, J. (2019). Phenology of farmland floral resources reveals seasonal gaps in nectar availability for bumblebees. J. Appl. Ecol. 56, 1585–1596. doi: 10.1111/1365-2664.13403
Tosi, S., Costa, C., Vesco, U., Quaglia, G., and Guido, G. (2018). A 3-year survey of Italian honey bee-collected pollen reveals widespread contamination by agricultural pesticides. Sci. Total Environ. 615, 208–218. doi: 10.1016/j.scitotenv.2017.09.226
Usha, V. K. M., and Devi, M. S. (2020). Effect of environmental factors on the foraging activities of major bee pollinators. J. Entomol. Zool. Stud. 8, 450–454.
Vidau, C. (2015). “Exposition des colonies d’abeille aux pesticides” in Actes des 3e Journées de la recherche apicole. ITSAP-Institut de l’abeille Ed., Paris. Available at: https://itsap.asso.fr/downloads/evenements/journees_de_la_recherche_api_2015__pre-programme.pdf
Walton, R. E., Sayer, C. D., Bennion, H., and Axmacher, J. C. (2020). Nocturnal pollinators strongly contribute to pollen transport of wild flowers in an agricultural landscape. Biol. Lett. 16:20190877. doi: 10.1098/rsbl.2019.0877
Woyke, J., Wilde, J., and Wilde, M. (2003). Periodic mass flights of Apis laboriosa in Nepal. Apidologie 34, 121–127. doi: 10.1051/apido:2003002
Keywords: bees, pesticides, pollination, regulation, beekeeping, pollinators
Citation: Decourtye A, Rollin O, Requier F, Allier F, Rüger C, Vidau C and Henry M (2023) Decision-making criteria for pesticide spraying considering the bees’ presence on crops to reduce their exposure risk. Front. Ecol. Evol. 11:1062441. doi: 10.3389/fevo.2023.1062441
Received: 05 October 2022; Accepted: 27 January 2023;
Published: 17 February 2023.
Edited by:
Fabio Sgolastra, University of Bologna, ItalyReviewed by:
Giovanni Tamburini, University of Bari Aldo Moro, ItalyCopyright © 2023 Decourtye, Rollin, Requier, Allier, Rüger, Vidau and Henry. This is an open-access article distributed under the terms of the Creative Commons Attribution License (CC BY). The use, distribution or reproduction in other forums is permitted, provided the original author(s) and the copyright owner(s) are credited and that the original publication in this journal is cited, in accordance with accepted academic practice. No use, distribution or reproduction is permitted which does not comply with these terms.
*Correspondence: Axel Decourtye, ✉ YXhlbC5kZWNvdXJ0eWVAaXRzYXAuYXNzby5mcg==
Disclaimer: All claims expressed in this article are solely those of the authors and do not necessarily represent those of their affiliated organizations, or those of the publisher, the editors and the reviewers. Any product that may be evaluated in this article or claim that may be made by its manufacturer is not guaranteed or endorsed by the publisher.
Research integrity at Frontiers
Learn more about the work of our research integrity team to safeguard the quality of each article we publish.