- College of Animal Science and Technology, Northeast Agricultural University, Harbin, China
This study aimed to evaluate the relationship between temperature (T), relative humidity (RH), and temperature and humidity index (THI), milk yield (MY), rumination time (RT), and activity (AT) of dairy cows in different parities under low temperature and high humidity (LTHH). In this study, the number of samples each day was determined by all healthy cows in the barn with parity and days in milk (DIM) within 5 and 305, respectively. The box plot method was used for screening and removing outliers of dairy cow indicators after classification according to parity and DIM. To remove the effect of DIM on MY, a bivariate regression model was used to standardize the MY in milk yield index (MYI). The best bivariate regression model based on the lowest Akaike information criterion was used to analyze the relationship between behavioral parameters, MYI, and microclimate indicators for each parity. In the barn with the microclimate at a low temperature above 0°C, high RH was negatively correlated with MYI in primiparous and multiparous cows but positively correlated with AT in primiparous and multiparous cows and RT in multiparous cows (p < 0.05), so RH was a significant factor related to MYI, RT, and AT of cows. The 2-day lagged daily average T and THI were correlated with MYI in primiparous cows (p < 0.05). The inflection point value of 71.9 between AT and RH in the multiparity as the upper limit of RH was beneficial for improving comfort and MY in all parity dairy cows. Compared with MYI and RT, AT had a higher R2 with a microclimate indicator, so it could be used as a better indicator for assessing the LTHH. Comparing the R2 of multiparous cows to T (R2 = 0.0807) and THI (R2 = 0.1247), primiparous cows had higher R2 in MYI to T (R2 = 0.2833) and THI (R2 = 0.3008). Therefore, primiparous cows were more susceptible to T and THI. The inflection point values for MYI to T and THI were greater in primiparous cows than in multiparous cows, indicating that primiparous cows had a smaller tolerance range to T and THI than multiparous cows. Thus, parity should be considered when studying the relationship between MY, T, and THI under LTHH.
1. Introduction
There is a growing interest in dairy cow welfare (von Keyserlingk et al., 2013; Tucker et al., 2021), which is closely related to the environment. As a major factor in the indoor barn environment, climate affects the welfare level of dairy cows (Honig et al., 2012; Polsky and von Keyserlingk, 2017). Previous studies have shown that heat stress occurs in cows when the temperature and humidity index (THI) of the environment is above 72 and that milk yield (MY) decreases by 0.2 kg for a unit increase in THI (Ravagnolo et al., 2000; West, 2003). In heat stress, cows will spend more time standing, less time activity (Cook et al., 2007; Allen et al., 2015; Polsky and von Keyserlingk, 2017), and less time rumination with increasing temperature (T; Blackshaw and Blackshaw, 1994). The comfortable ambient T for dairy cows is between 5 and 15°C (Hahn, 1999; Kadzere et al., 2002). Due to the extremely low T in northeastern China for 6 months, large intensive farms often use fully enclosed housing management to keep warm. However, this management leads to the humidity in the barn being difficult to discharge thus increasing humidity. High humidity conditions weaken the dairy cow’s fur insulation, resulting in quicker heat loss (Angrecka and Herbut, 2015). Therefore, T alone is insufficient to assess the effect of housing microclimate on dairy cows (Degen and Young, 1993), and humidity should be considered. Low-temperature and high-humidity (LTHH) microclimate in the barn may cause cows to exceed their comfort zone, thus negatively impacting welfare.
Animal behavior can reflect the condition of the environment, and this behavioral performance helps evaluate the level of animal welfare (Cook et al., 2005; Godyn et al., 2013; Hoffmann et al., 2020). There is evidence that dairy cows adjust their productivity and behavior to microclimate conditions (Angrecka and Herbut, 2016; de Sousa et al., 2021). The lying time of dairy cows can reflect their welfare level to some extent (Tucker et al., 2021), and therefore has been used as an indicator to evaluate cow welfare in several studies (Fisher et al., 2003; Tucker et al., 2003; Schütz and Cox, 2014). Wet surfaces reduce lying time (Tucker et al., 2007; Schütz et al., 2019), thus, this may result in a corresponding change in activity time (AT) for dairy cows housed in winter. The rumination time (RT) is mainly related to diet composition (Beauchemin, 2018). However, the reduction in RT may also be related to the stress that the dairy cow is experiencing (Paudyal, 2021). The MY can be interpreted to be a direct welfare indicator (Polsky and von Keyserlingk, 2017). Therefore, MY, RT, and AT changes can reflect dairy cows’ climatic environment and welfare level. The smart collar and milking robot accurately monitor dairy cows’ daily RT, AT, and MY, making obtaining behavioral indicators non-invasive and non-stressful (Schirmann et al., 2009; Burfeind et al., 2011).
The temperature and humidity index is used to assess changes in the T and humidity of the environment and is commonly used in studies of heat stress in dairy cows (Bernabucci et al., 2014; Menta et al., 2022). Also, Li et al. (2021) used it to describe the climate’s humidity and coldness in a free-stall barn (indoor). Angrecka and Herbut (2015) used the wind chill temperature (WCT) index to measure the effect of cold stress on dairy cows in a free-stall barn (indoor). However, T and humidity are important factors for the microclimate of a fully enclosed barn in cold conditions (Buonomano et al., 2017).
To our knowledge, little information is available evaluating the effect of LTHH conditions on MY and behavioral indicators of lactating dairy cows. This study aimed to evaluate the relationship between T, humidity, and THI and MY, RT, and AT of dairy cows in different parities under LTHH conditions to understand the effects of LTHH on dairy cow welfare and to provide a reference for comfortable management of LTHH environments and early development of automatic early warning systems. We hypothesized that dairy cows’ MY, RT, and AT are related to T, humidity, and THI, but different parities respond differently to T, humidity, and THI.
2. Materials and methods
2.1. Farm
The experiment was performed under an experimental license from Northeast Agricultural University, Harbin, China. All experimental procedures were conducted in accordance with the principles and responsibilities outlined in the university’s guidelines for animal research. This study was conducted on a commercial farm in Heihe City in northeastern China (49°0′6 ″N, 126°2′24 ″E), with a cold-temperate continental monsoon climate. The barn was fully enclosed with a half-bell tower roof. The middle of the barn was a feeding path and there were four pens in the barn.
2.2. Animals and management
A total of 854 healthy Holstein cows were used for the study. Each day, the number of samples included in the study was determined by all healthy cows in the barn with parity and DIM within 5 and 305, respectively. The average parity was 3 ± 1 (mean ± SD). The dairy cows were housed in a free-stall barn with sand bedding and milked two times daily (0600 and 1800 h). Dairy cows were fed a TMR to meet or exceed dietary nutritional requirements (NRC, 2001) and drank freely at all times.
2.3. Sensors and dataset
A fully automated temperature and humidity recorder (YDBS, China) was used to record the temperature and RH in the barn. Three temperature and humidity recorders were evenly distributed and installed in the barn, approximately 1.5 m high from the ground. Each recorder measured and recorded data once every 2 h (a total of 12 data records from 0000 to 2400 h every day). The recording was from 1 January 2021 to 30 April 2021. The formula reported by Kendall et al. (2008) was used to calculate the THI:
Where T (°C) is the temperature and RH (%) is the relative humidity. This formula was chosen because it has been used previously in animal trials conducted in a continental climate (Schüller et al., 2014; Shock et al., 2016). T, RH, and THI recorded by three recorders every day were averaged to obtain the daily average of T, RH, and THI.
All dairy cows enrolled in the study were fitted with a smart collar sensor (SCR, Israel) measuring activity with a 3-axis accelerometer and rumination with a microphone and microprocessor. The SCR system was validated by Schirmann et al. (2009) and Ambriz-Vilchis et al. (2015). The SCR recorder calculated and summarized the data every 2 h and finally reported the total AT and RT data from 0 to 24 h every day to SCR DataFlow™ II System software as the daily AT (units/d) and RT (min/d). The recording was from 1 January 2021 to 30 April 2021. The milk hall was equipped with milking machines (SCR, Israel) that can measure the MY of each dairy cow from the milking parlor by infrared and upload data to the SCR DataFlow™ II System. The daily MY of each dairy cow was the sum of two milkings. Then, the daily MY of all cows included in the study every day was averaged as the daily MY. The recording was from 1 January 2021 to 30 April 2021.
2.4. Data processing and statistical analysis
The MY, RT, and AT were acquired from the SCR DataFlow™ II System. Data for T and RH were exported into an Excel spreadsheet (Microsoft Corp., Redmond, WA). The JMP Pro 16 (SAS Institute, NC) is the data processing and statistical analysis software used in this experiment.
The boxplot method can truly represent the data distribution and ensure that the results of identifying outliers were more objective. Dairy cows were grouped according to parity (1–5) and DIM (1–305 days). The boxplot method of JMP Pro 16 was used to mark the outliers of MY, RT, and AT in each group and remove them. The range of outliers defined in the boxplot was less than QL - 1.5 IQR or greater than QU + 1.5 IQR (QL: lower quartile, indicating that a quarter of the observed values in each group are smaller than it; QU: upper quartile, indicating that a quarter of the observed values in each group are larger than it; IQR: interquartile spacing, indicating the difference between the upper quartile QU and the lower quartile QL).
To avoid the influence of DIM and parities on MY, MYI was used to evaluate the MY of each dairy cow. The MY was grouped according to parity and DIM, i.e. MY with the same parity and the same DIM were in the same group, and then the maximum milk yield of the same group was selected as the dependent variable in the bivariate regression model. Models were compared using R2, and models that best explained the milk variations in DIM were chosen based on the highest R2 value. The standard MY of the same DIM was calculated according to the fitting function of the best model. The formula of MYI is
where DMY is daily MY, SMY is standard MY, and MMY is maximum milk yield.
To evaluate the relationship between T, RH, and THI and MYI, AT, and RT, daily average T, RH, and THI corresponded to daily MY, AT, and RT as the input variables of the model. In addition to considering the relationship between the current day and MYI, the relationship between measures 1, 2, and 3 days before the current day and MYI was determined (Collier et al., 1981; West et al., 2003). These relationships were termed the lag effects, which consider the environmental effects that occurred 1, 2, or 3 days before the day in which milk yield was measured by the fit curve of JMP Pro 16 and fitting the following linear mixed-effects model:
where Y is a measurement of dependent variables (daily MYI, RT, and AT), k is the order of the polynomial, β0 is the intercept, βj represents the estimated coefficients of the fixed effect of daily average T, RH, and THI, and ε represents the random residual effect.
Models were compared using AIC, and models that best explained the day-to-day variations in MYI, RT, and AT were chosen based on the lowest Akaike information criterion (Cook et al., 2005) and then determined whether it was relevant based on the significant threshold level (p < 0.05).
3. Results
Since the data (MY, AT, and RT) recorded by the system on 23 April 2021 was considered an outlier, all the data for that day was removed. To make the results representative, we removed the data records corresponding to the daily average T higher than 15°C (21 and 22 April 2022). The distribution of all dairy cows by MYI, RT, and AT is shown in Table 1, and the barn by T, RH, and THI is shown in Table 2 and Figure 1.
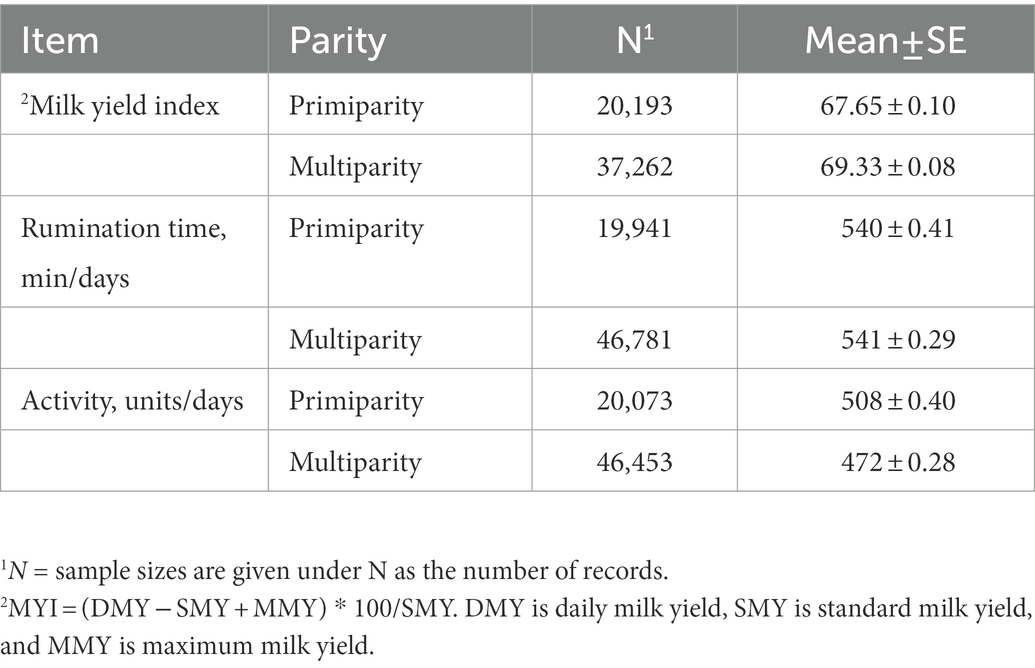
Table 1. Descriptive statistics of the milk yield index, rumination time, and activity in relation to lactation number.
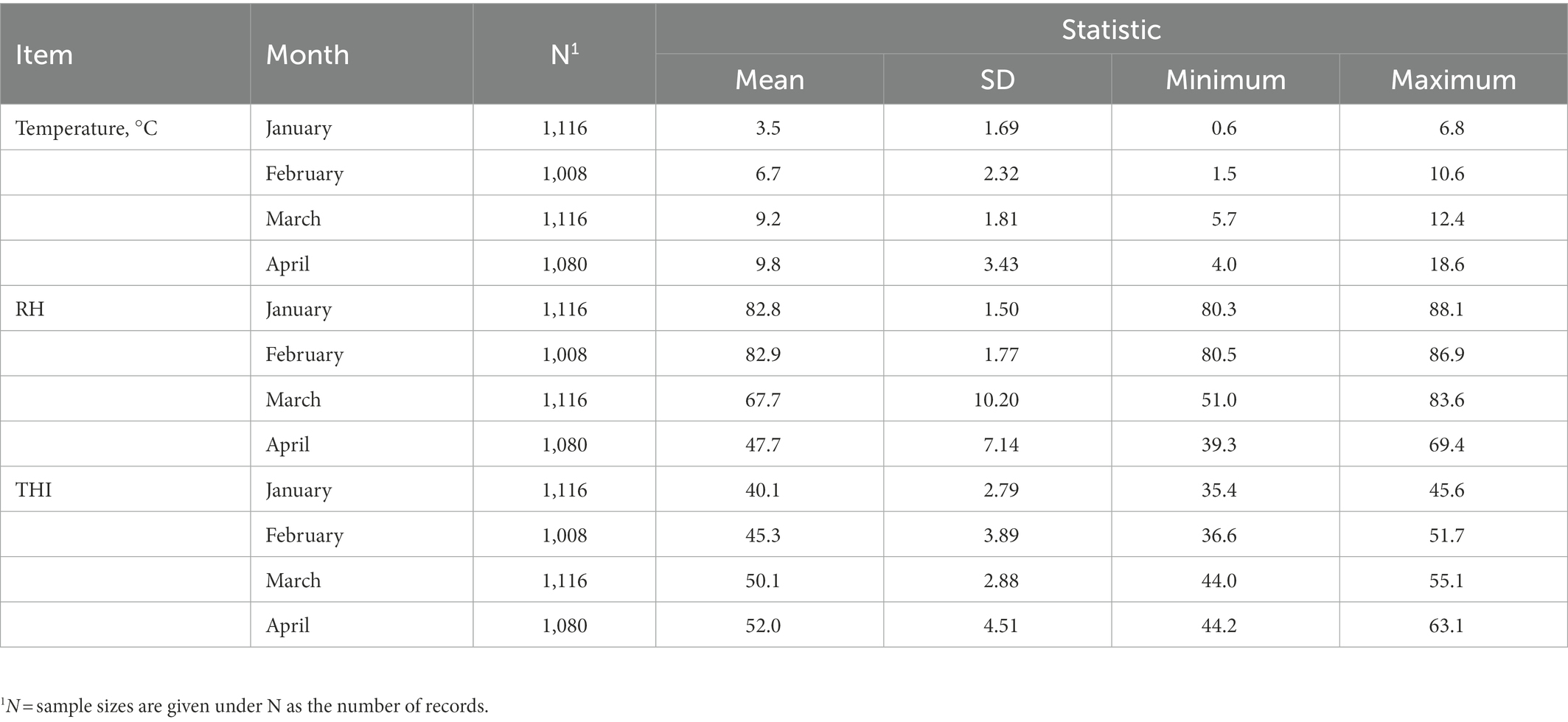
Table 2. Descriptive statistics for temperature and relative humidity in relation to month number recorded by fully automated temperature and humidity recorder (1 January 2021 to 30 April 2021) and for temperature–humidity index (THI) calculated from temperature and relative humidity data using Equations (1).
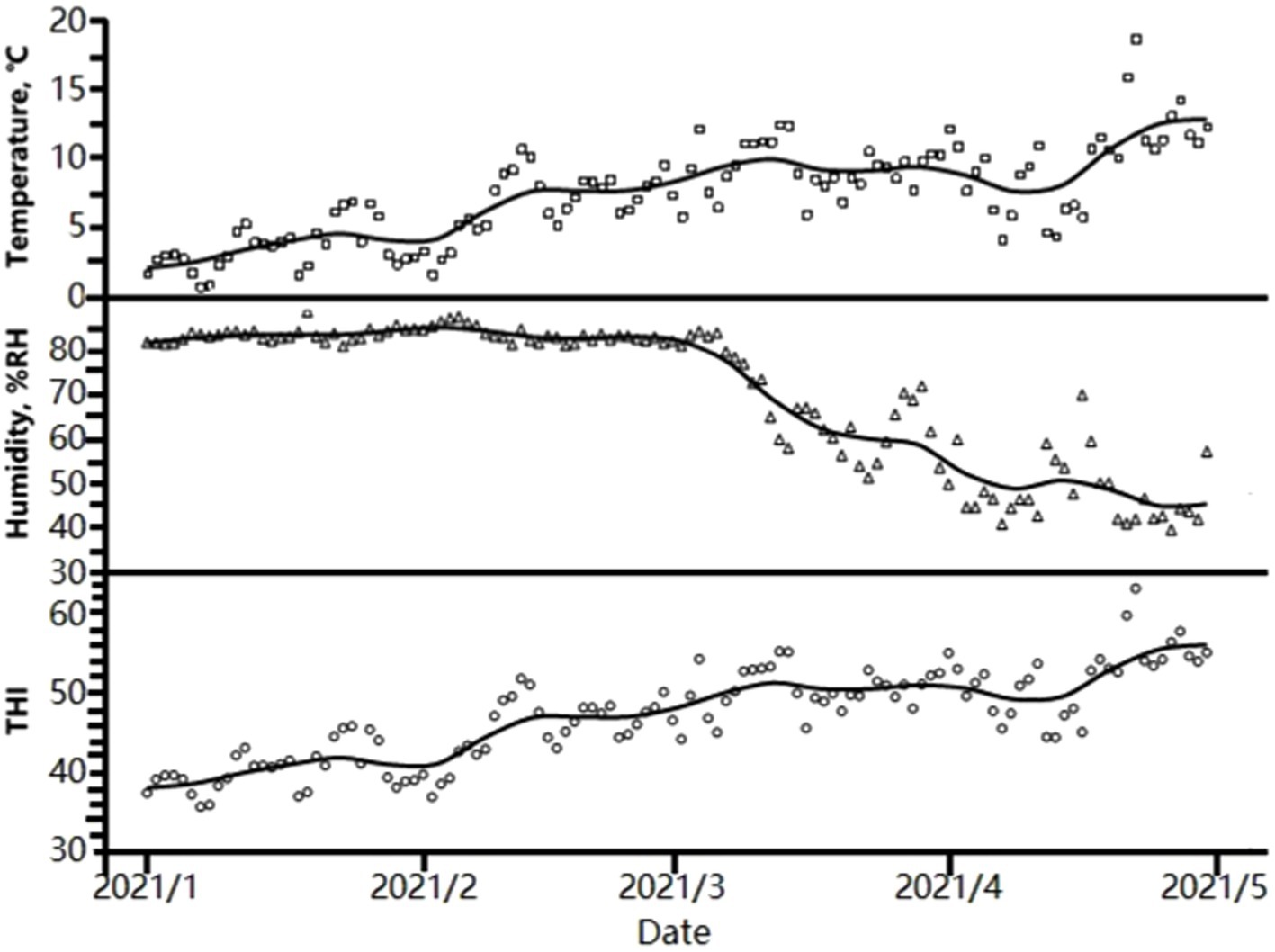
Figure 1. Mean daily temperature, relative humidity, and temperature–humidity index (THI) in the barn of the research farm, Heihe, China, during the study period (January 2021–April 2021).
3.1. The relationship between MYI and T, RH, and THI
Figure 2 shows that both primiparous and multiparous cows are related to T (p < 0.0001 and p = 0.0083, respectively; Figures 2A,D, respectively). However, the MY of primiparous cows was related to the 2-d lagged daily average T. The R2 was lower for multiparous cows (R2 = 0.0807, Figure 2D). MYI of the primiparity decreased as the T increased from 0.6 to 8.6 (Figure 2A). However, the MYI of the multiparity decreased with increasing T from 0.6 to 5.2 (Figure 2D). Thus, the different T ranges indicated a difference in the appropriate T range (from the inflection point value to the upper limit of the comfort zone temperature) for the primiparous and multiparous cows.
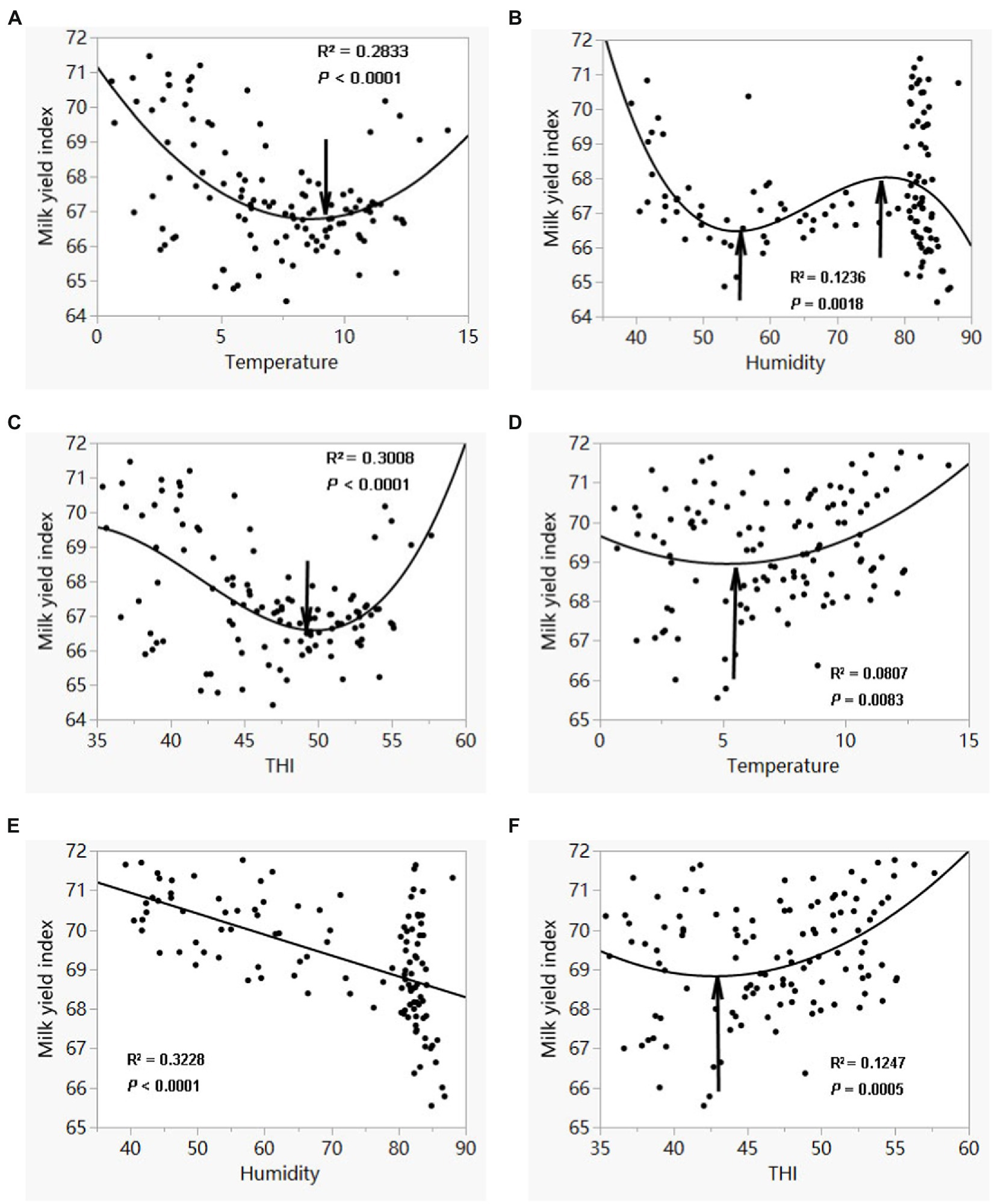
Figure 2. The relationship between milk yield index and (A) temperature (the 2-day lagged daily average temperature), (B) humidity, and (C) THI (the 2-day lagged daily average THI) of the primiparous dairy cows. The relationship between milk yield index and (D) temperature, (E) humidity, and (F) THI of the multiparous dairy cows.
Both primiparous and multiparous cows were related to RH (p = 0.0018 and p < 0.0001, respectively; Figures 2B,E, respectively). MYI tended to decrease with RH increasing when RH was in the range of 77.5 to 88 for primiparity (Figure 2B). However, the relationship between MYI and RH took on a negative linear correlation trend for the multiparity throughout the test period (Figure 2E). Overall, RH had different effects on the MY of primiparous and multiparous cows.
Figure 2 shows that both primiparous and multiparous cows are related to THI (p < 0.0001 and p = 0.0005 respectively; Figures 2C,F, respectively). However, the MY of primiparous cows was related to the 2-d lagged daily average THI. MYI of the primiparity decreased with increasing THI when THI was 35.4 to 49.8 (Figure 2C). Similarly, the MYI of the multiparity decreased with increasing THI from 35.4 to 42.8 (Figure 2F). Therefore, the different THI ranges indicated a difference in the appropriate THI range for the primiparous and multiparous cows. In conclusion, the MY of the primiparous and multiparous cows responded differently to T, RH, and THI under LTHH conditions.
3.2. The relationship between RT and T, RH, and THI
Figure 3 shows that the rumination of primiparous cows was related to T (Figure 3A), RH (Figure 3B), and THI (Figure 3C), with p-values ranging from 0.0754 to 0.1109. For multiparous cows, the RT was not related to T and THI (p = 0.0877 and p = 0.0621, respectively; Figures 3D,F). However, the rumination time of multiparous cows was related to RH (p = 0.0021), and when RH was in the range of 74.9 to 88, the rumination time of multiparous cows increased with increasing RH (Figure 3E).
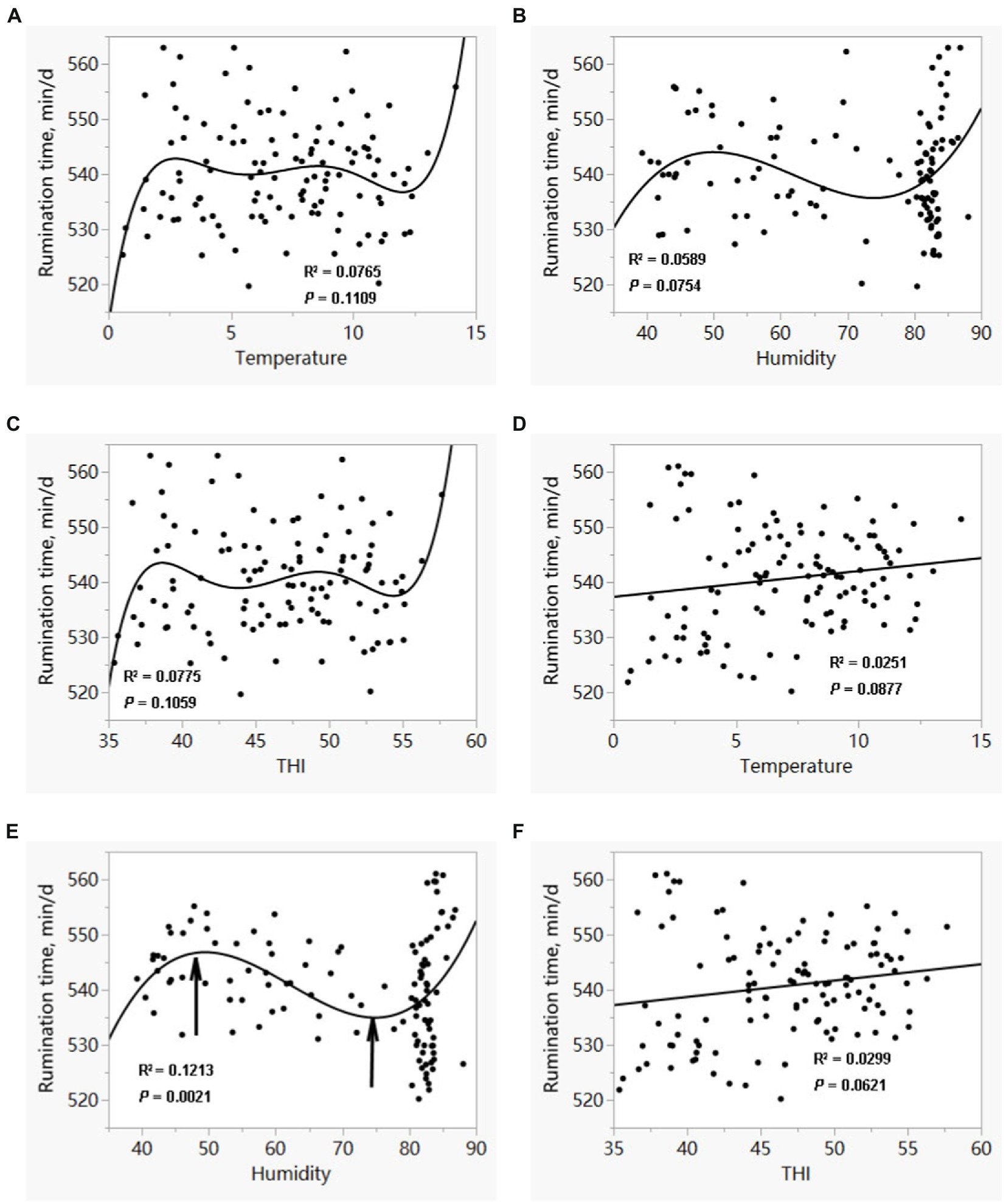
Figure 3. The relationship between rumination time and (A) temperature, (B) humidity, and (C) THI of the primiparous dairy cows. The relationship between milk yield index and (D) temperature, (E) humidity, and (F) THI of the multiparous dairy cows.
3.3. The relationship between AT and T, RH, and THI
Figure 4 shows that AT is related to T in both primiparous and multiparous cows (p < 0.0001 and p = 0.0004, respectively; Figures 4A,D, respectively) and varied in a similar pattern with T with inflection points of 5.7 and 6.1, respectively, with AT decreasing with increasing T below inflection points.
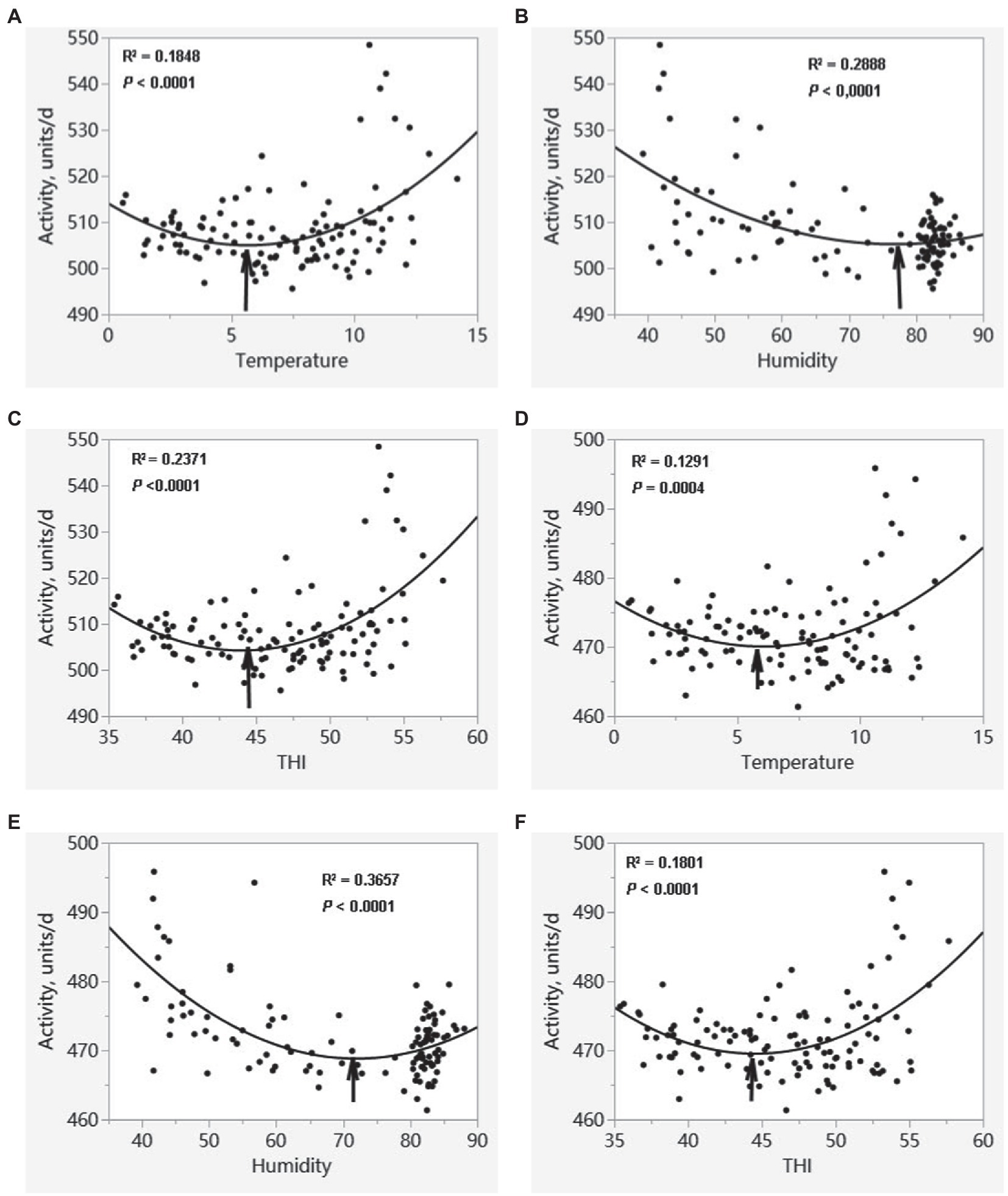
Figure 4. The relationship between activity and (A) temperature, (B) humidity, and (C) THI of the primiparous dairy cows. The relationship between milk yield index and (D) temperature, (E) humidity, and (F) THI of the multiparous dairy cows.
AT of both primiparous and multiparous cows was related to RH (p < 0.0001 and p < 0.0001, respectively; Figures 4B,E, respectively) and was more highly correlated with RH (R2 = 0.2888 and R2 = 0.3657, respectively) compared to T and THI. The RH change pattern was similar for the primiparous and multiparous cows, with RH inflection points of 76.9 and 71.9, respectively, and the AT increased with increasing RH above inflection points.
Both primiparous and multiparous cows were related to THI (p < 0.0001 and p < 0.0001, respectively; Figures 4C,F, respectively). AT of the primiparity decreased with increasing THI at THI below 44 (Figure 4C). Similarly, AT of the multiparity decreased with increasing THI at THI below 44.5 (Figure 4F). In summary, AT of the primiparity and multiparity responded similarly to T, RH, and THI under LTHH conditions.
4. Discussion
According to West (2003), a thermoneutral zone ranging between −0.5 and +20°C is acceptable for dairy cows. Although our results showed that the average daily T in the barn was above 0°C, MY, RT, and AT still varied with T, RH, and THI under LTHH conditions. This indicates that high humidity at low T was an important environmental factor affecting dairy cows’ MYI, RT, and AT. In addition, our results showed that the difference in inflection point values of MYI in T and THI between primiparity and multiparity was large and that the trend with RH was different for different parities. This suggested that different parities of dairy cows had different ranges of adaptation to T, humidity, and THI. Therefore, the same T, RH, and THI levels were not used to assess the response of dairy cows of different parities to LTHH (Hammami et al., 2013), and the parity was a factor that cannot be ignored when assessing the effect of cold and high humidity on dairy cows. Although our results showed that the R2 values (from 0.0807 to 0.1848) of the models assessing MYI with RH in primiparous cows, MYI with T and THI in multiparous cows, AT with T in primiparous and multiparous cows, and AT with THI in multiparous cows were small, the R2 value of the model by Stone et al. (2017) assessing the correlation between THI and cow lying time was 0.01 and they concluded that THI was related to lying time. This shows that the level of R2 value cannot be used to determine whether there is a correlation or not, but rather the p-value is used to determine the correlation, so our results assessing the correlation between T, RH, THI and MYI, RT, and AT under LTHH conditions are reliable.
4.1. The relationship between MYI and T, RH, and THI
The findings of this study showed that MYI of both primiparity and multiparity was related to T, humidity, and THI, indicating that the microclimate of the barn affects MY (Vaculíková et al., 2017). However, the MYI of dairy cows in response to T, RH, and THI at LTHH varied from parity to parity. Compared to multiparous dairy cows, primiparous dairy cows had higher R2 in T and THI, suggesting that T and THI explained more variation in MYI in primiparous dairy cows than in multiparous dairy cows, and therefore primiparous dairy cows were more susceptible to the effects of T and THI. In addition, our results suggested that the inflection point of MY with T for multiparous dairy cows was 6.1, which is similar to the lower critical T of 5, considered by Vtoryi et al. (2018) as the most suitable for cow production. However, the inflection point values of MYI in relation to T and THI for primiparous dairy cows were greater than those for multiparous dairy cows, indicating that the T and THI tolerance range of primiparous cows is less than that of multiparous cows. This difference may be because primiparous dairy cows are still in the growth phase and the energy obtained from the diet must be distributed to growth (Wathes et al., 2007), thus compared to the multiparous dairy cows, the primiparous dairy cows use less energy to maintain body T balance. The MY increased with an increase in lactation (Vijayakumar et al., 2017) and an increase in the number of mammary epithelial cells (Herve et al., 2016). For high-yield dairy cows with the multiparous dairy cows (Lee and Kim, 2006), more metabolic heat is generated during milk synthesis to maintain body T balance (Marumo et al., 2022), so the multiparous dairy cows have a higher tolerance to low T than the primiparous dairy cows. In addition, primiparous cows are lighter than multiparous cows, so the ratio of surface area to volume will be slightly higher, thus predisposing them to heat loss. Interestingly, our study found that the MY of primiparous cows was related to the 2-day lagged daily average temperature and THI, but not to RH on the current day. This delayed effect may be related to a change in feeding or a delayed response to a change in the metabolic or endocrine status of the animal (Collier et al., 1981; West et al., 2003). Changes in RH may rapidly change the endocrine status of the cow thus leading to MY in relation to the daily average RH of the current day. This suggests that in dairy management, we should take immediate action to reduce the loss of MY at the time of climate change, rather than taking action after MY has decreased.
For primiparous cows, MYI decreased with increasing RH at RH greater than 77.5. Interestingly, Sharma et al. (1988) found in their study of heat stress in dairy cows that optimal conditions for MY were maximum T below 19.4°C and minimum RH between 33.4 and 78.2%. This means that in both cold and hot conditions, when the humidity in the barn exceeds about 77, it will have a negative impact on milk yield. However, a negative linear correlation existed between the MYI of multiparous cows and RH. This can be attributed to the fact that at higher RH, dairy cows transfer net energy for heat production to maintain heat balance resulting in lower MY (Brouček et al., 1991; Collier and Gebremedhin, 2015). The MY is a direct reflection of the welfare level of the dairy cow (Polsky and von Keyserlingk, 2017). Our results suggest that higher RH plays a negative role in the welfare of primiparous and prolific cows under LTHH conditions. Thus, it is important for farm managers to control the RH to maximize the productive performance of animals during the period of LTHH (Marumo et al., 2022).
Previous works assessing the effect of cold stress on MY have given conflicting views on the T at which the MY begins to decrease (Young, 1983; Brouček et al., 1991; Kadzere et al., 2002). Our results also suggested that MY was affected in a proportion of dairy cows even when the T was above 0°C. Therefore, the low critical T should be defined with caution after full consideration of the interaction of several climatic factors. In addition, our study showed that the MYI of the multiparous dairy cows at THI below 42.8 decreased with increasing THI, while Hammami et al. (2013) found that MY decreased in assessing the effect of THI on MY with increasing THI when THI is below 62. This may be because their T and humidity data were obtained from a nearby weather station, which does not accurately represent the microclimate in the barn, thus affecting the determination of the inflection point. Barn microclimate would have provided a better understanding of the effect of ambient T and humidity on dairy cow performance (Gauly et al., 2013). In conclusion, microclimate should prevail when assessing the effect of the environment on housed cows, and the role of RH on T should be considered.
4.2. The relationship between RT and T, RH, and THI
Rumination time is commonly used to assess heat stress in dairy cows and exceeding the critical threshold RT that negatively correlates with THI (Soriani et al., 2013; Moretti et al., 2017). However, our results showed that THI and T under LTHH did not correlate with RT, whereas only the RT of multiparous cows correlated with RH and increased with increasing RH. This may further suggest that the physical and chemical composition of the diet and NDF intake were the most important factors influencing RT (Beauchemin, 2018). Therefore, RT cannot be used as an assessment factor for the effect of LTHH.
4.3. The relationship between AT and T, RH, and THI
The high R2 values of AT with T, RH, and THI, compared to MYI and RT, indicated that AT was a better factor in assessing the influence of LTHH. In addition, comparing T and THI, AT had a high R2 value with RH, suggesting that humidity strongly correlated with AT under LTHH conditions and was an important factor that could not be ignored in influencing cow AT.
Primiparous and multiparous cows presented similar trends, showing higher AT at lower levels below the inflection point of T and THI and at higher levels above the inflection point of RH. Higher AT levels indicate that cows spend more time exposed to wet surfaces, leading to paws that absorb moisture and become soft, raising the risk of lameness (Borderas et al., 2004). Keeping track of changes in cows, AT provides insight into the levels of T, RH, and THI in the barn and can be useful in preventing the occurrence of lameness (Tolkamp et al., 2010), which is beneficial to the welfare of the dairy cow. In addition, higher AT levels mean that cows tend to spend less time lying down, thus reducing their comfort. This phenomenon can be explained by the fact that active cows generate more heat to maintain body T than lying cows (Tucker et al., 2007). However, we need to investigate further the variation of active and lying time with T and humidity, which will help us fully understand cows’ behavioral changes under LTHH conditions.
5. Conclusion
In the barn with the microclimate at low T above 0°C, RH correlates with MYI and AT in primiparous cows and RT in multiparous cows, so RH is a significant factor related to MYI, RT, and AT in cows. In addition, the inflection point value of 71.9 between AT and RH in the multiparous cows as the upper limit of ambient RH is beneficial for improving comfort and maintaining good performance in all parity dairy cows. AT was a better factor in assessing the impact of LTHH than MYI and RT. The vulnerability of MY to T and THI, as well as the smaller range of tolerance to T and THI in primiparous cows compared to multiparous cows, suggests that parity should be considered when studying the relationship between MY and T and THI under LTHH conditions.
Data availability statement
The raw data supporting the conclusions of this article will be made available by the authors, without undue reservation.
Ethics statement
The animal study was reviewed and approved by Northeast Agricultural University. Written informed consent was obtained from the owners for the participation of their animals in this study.
Author contributions
JS and YS conceptualized and designed the study. JS conducted animal trials, analyzed some of the data, and drafted the original manuscript. QY, XW, and YW analyzed some of the data. YS and YZ reviewed and provided critical comments on the manuscript. All authors contributed to the article and approved the submitted version.
Funding
This study was financially supported by the National Key Research and Development Program of China (2021YFD1300503-2) and by the earmarked fund for CARS36. The authors thank Northeast Agricultural University for the supporting facility.
Acknowledgments
The authors thank a Land Reclamation farm in Beian City for providing the test site.
Conflict of interest
The authors declare that the research was conducted in the absence of any commercial or financial relationships that could be construed as a potential conflict of interest.
Publisher’s note
All claims expressed in this article are solely those of the authors and do not necessarily represent those of their affiliated organizations, or those of the publisher, the editors and the reviewers. Any product that may be evaluated in this article, or claim that may be made by its manufacturer, is not guaranteed or endorsed by the publisher.
References
Allen, J., Hall, L., Collier, R., and Smith, J. (2015). Effect of core body temperature, time of day, and climate conditions on behavioral patterns of lactating dairy cows experiencing mild to moderate heat stress. J. Dairy Sci. 98, 118–127. doi: 10.3168/jds.2013-7704
Ambriz-Vilchis, V., Jessop, N. S., Fawcett, R. H., Shaw, D. J., and Macrae, A. I. (2015). Comparison of rumination activity measured using rumination collars against direct visual observations and analysis of video recordings of dairy cows in commercial farm environments. J. Dairy Sci. 98, 1750–1758. doi: 10.3168/jds.2014-8565
Angrecka, S., and Herbut, P. (2015). Conditions for cold stress development in dairy cattle kept in free stall barn during severe frosts. Czeh J. Anim. Sci. 60, 81–87. doi: 10.17221/7978-cjas
Angrecka, S., and Herbut, P. (2016). Impact of barn orientation on insolation and temperature of stalls surface. Ann. Anim. Sci. 16, 887–896. doi: 10.1515/aoas-2015-0096
Beauchemin, K. A. (2018). Invited review: current perspectives on eating and rumination activity in dairy cows. J. Dairy Sci. 101, 4762–4784. doi: 10.3168/jds.2017-13706
Bernabucci, U., Biffani, S., Buggiotti, L., Vitali, A., Lacetera, N., and Nardone, A. (2014). The effects of heat stress in Italian Holstein dairy cattle. J. Dairy Sci. 97, 471–486. doi: 10.3168/jds.2013-6611
Blackshaw, J. K., and Blackshaw, A. (1994). Heat stress in cattle and the effect of shade on production and behaviour: a review. Aust. J. Exp. Agric. 34, 285–295. doi: 10.1071/EA9940285
Borderas, T. F., Pawluczuk, B., de Passillé, A. M., and Rushen, J. (2004). Claw hardness of dairy cows: relationship to water content and claw lesions. J. Dairy Sci. 87, 2085–2093. doi: 10.3168/jds.S0022-0302(04)70026-0
Brouček, J., Letkovičová, M., and Kovalčuj, K. (1991). Estimation of cold stress effect on dairy cows. Int. J. Biometeorol. 35, 29–32. doi: 10.1007/BF01040960
Buonomano, A., Montanaro, U., Palombo, A., and Santini, S. (2017). Temperature and humidity adaptive control in multi-enclosed thermal zones under unexpected external disturbances. Energ. Build. 135, 263–285. doi: 10.1016/j.enbuild.2016.11.015
Burfeind, O., Schirmann, K., von Keyserlingk, M. A. G., Veira, D. M., Weary, D. M., and Heuwieser, W. (2011). Technical note: evaluation of a system for monitoring rumination in heifers and calves. J. Dairy Sci. 94, 426–430. doi: 10.3168/jds.2010-3239
Collier, R., Eley, R., Sharma, A., Pereira, R., and Buffington, D. (1981). Shade management in subtropical environment for milk yield and composition in Holstein and Jersey cows. J. Dairy Sci. 64, 844–849. doi: 10.3168/jds.S0022-0302(81)82656-2
Collier, R. J., and Gebremedhin, K. G. (2015). Thermal biology of domestic animals. Annu. Rev. Anim. Biosci. 3, 513–532. doi: 10.1146/annurev-animal-022114-110659
Cook, N. B., Bennett, T. B., and Nordlund, K. V. (2005). Monitoring indices of cow comfort in free-stall-housed dairy herds*. J. Dairy Sci. 88, 3876–3885. doi: 10.3168/jds.S0022-0302(05)73073-3
Cook, N., Mentink, R., Bennett, T., and Burgi, K. (2007). The effect of heat stress and lameness on time budgets of lactating dairy cows. J. Dairy Sci. 90, 1674–1682. doi: 10.3168/jds.2006-634
de Sousa, K. T., Deniz, M., Vale, M. M. D., Dittrich, J. R., and Hötzel, M. J. (2021). Influence of microclimate on dairy cows’ behavior in three pasture systems during the winter in South Brazil. J. Therm. Biol. 97:102873. doi: 10.1016/j.jtherbio.2021.102873
Degen, A. A., and Young, B. A. (1993). Rate of metabolic heat production and rectal temperature of steers exposed to simulated mud and rain conditions. Can. J. Anim. Sci. 73, 207–210. doi: 10.4141/cjas93-021
Fisher, A. D., Stewart, M., Verkerk, G. A., Morrow, C. J., and Matthews, L. R. (2003). The effects of surface type on lying behaviour and stress responses of dairy cows during periodic weather-induced removal from pasture. Appl. Anim. Behav. Sci. 81, 1–11. doi: 10.1016/S0168-1591(02)00240-X
Gauly, M., Bollwein, H., Breves, G., Brügemann, K., Dänicke, S., Daş, G., et al. (2013). Future consequences and challenges for dairy cow production systems arising from climate change in Central Europe – a review. Animal 7, 843–859. doi: 10.1017/S1751731112002352
Godyn, D., Herbut, E., and Walczak, J. (2013). Infrared thermography as a method for evaluating the welfare of animals subjected to invasive procedures - a review/Termografia jako metoda oceny dobrostanu zwierzat poddanych inwazyjnym zabiegom - artykul przegladowy. Ann. Anim. Sci. 13, 423–434. doi: 10.2478/aoas-2013-0027
Hahn, L. G. (1999). Dynamic responses of cattle to thermal heat loads. J. Anim. Sci. 77, 10–20. doi: 10.2527/1997.77suppl_210x
Hammami, H., Bormann, J., Hamdi, N. M., Montaldo, H. H., and Gengler, N. (2013). Evaluation of heat stress effects on production traits and somatic cell score of Holsteins in a temperate environment. J. Dairy Sci. 96, 1844–1855. doi: 10.3168/jds.2012-5947
Herve, L., Quesnel, H., Lollivier, V., and Boutinaud, M. (2016). Regulation of cell number in the mammary gland by controlling the exfoliation process in milk in ruminants. J. Dairy Sci. 99, 854–863. doi: 10.3168/jds.2015-9964
Hoffmann, G., Herbut, P., Pinto, S., Heinicke, J., Kuhla, B., and Amon, T. (2020). Animal-related, non-invasive indicators for determining heat stress in dairy cows. Biosyst. Eng. 199, 83–96. doi: 10.1016/j.biosystemseng.2019.10.017
Honig, H., Miron, J., Lehrer, H., Jackoby, S., Zachut, M., Zinou, A., et al. (2012). Performance and welfare of high-yielding dairy cows subjected to 5 or 8 cooling sessions daily under hot and humid climate. J. Dairy Sci. 95, 3736–3742. doi: 10.3168/jds.2011-5054
Kadzere, C. T., Murphy, M. R., Silanikove, N., and Maltz, E. (2002). Heat stress in lactating dairy cows: a review. Livest. Prod. Sci. 77, 59–91. doi: 10.1016/S0301-6226(01)00330-X
Kendall, P. E., Tucker, C. B., Dalley, D. E., Clark, D. A., and Webster, J. R. (2008). Milking frequency affects the circadian body temperature rhythm in dairy cows. Livest. Sci. 117, 130–138. doi: 10.1016/j.livsci.2007.12.009
Lee, J.-Y., and Kim, I.-H. (2006). Advancing parity is associated with high milk production at the cost of body condition and increased periparturient disorders in dairy herds. J. Vet. Sci. 7, 161–166. doi: 10.4142/jvs.2006.7.2.161
Li, H., Zhang, Y., Li, R., Wu, Y., Zhang, D., Xu, H., et al. (2021). Effect of seasonal thermal stress on oxidative status, immune response and stress hormones of lactating dairy cows. Anim. Nutr. 7, 216–223. doi: 10.1016/j.aninu.2020.07.006
Marumo, J. L., Lusseau, D., Speakman, J. R., Mackie, M., and Hambly, C. (2022). Influence of environmental factors and parity on milk yield dynamics in barn-housed dairy cattle. J. Dairy Sci. 105, 1225–1241. doi: 10.3168/jds.2021-20698
Menta, P. R., Machado, V. S., Piñeiro, J. M., Thatcher, W. W., Santos, J. E. P., and Vieira-Neto, A. (2022). Heat stress during the transition period is associated with impaired production, reproduction, and survival in dairy cows. J. Dairy Sci. 105, 4474–4489. doi: 10.3168/jds.2021-21185
Moretti, R., Biffani, S., Chessa, S., and Bozzi, R. (2017). Heat stress effects on Holstein dairy cows’ rumination. Animal 11, 2320–2325. doi: 10.1017/S1751731117001173
NRC (2001). Nutrient requirements of dairy cattle. 7th rev. ed. United States National Academy of Sciences, Washington, DC.
Paudyal, S. (2021). Using rumination time to manage health and reproduction in dairy cattle: a review. Vet. Q. 41, 292–300. doi: 10.1080/01652176.2021.1987581
Polsky, L., and von Keyserlingk, M. A. G. (2017). Invited review: effects of heat stress on dairy cattle welfare. J. Dairy Sci. 100, 8645–8657. doi: 10.3168/jds.2017-12651
Ravagnolo, O., Misztal, I., and Hoogenboom, G. (2000). Genetic component of heat stress in dairy cattle, development of heat index function. J. Dairy Sci. 83, 2120–2125. doi: 10.3168/jds.S0022-0302(00)75094-6
Schirmann, K., von Keyserlingk, M. A. G., Weary, D. M., Veira, D. M., and Heuwieser, W. (2009). Technical note: validation of a system for monitoring rumination in dairy cows. J. Dairy Sci. 92, 6052–6055. doi: 10.3168/jds.2009-2361
Schüller, L. K., Burfeind, O., and Heuwieser, W. (2014). Impact of heat stress on conception rate of dairy cows in the moderate climate considering different temperature–humidity index thresholds, periods relative to breeding, and heat load indices. Theriogenology 81, 1050–1057. doi: 10.1016/j.theriogenology.2014.01.029
Schütz, K. E., Cave, V. M., Cox, N. R., Huddart, F. J., and Tucker, C. B. (2019). Effects of 3 surface types on dairy cattle behavior, preference, and hygiene. J. Dairy Sci. 102, 1530–1541. doi: 10.3168/jds.2018-14792
Schütz, K. E., and Cox, N. R. (2014). Effects of short-term repeated exposure to different flooring surfaces on the behavior and physiology of dairy cattle. J. Dairy Sci. 97, 2753–2762. doi: 10.3168/jds.2013-7310
Sharma, A. K., Rodriguez, L. A., Wilcox, C. J., Collier, R. J., Bachman, K. C., and Martin, F. G. (1988). Interactions of climatic factors affecting Milk yield and composition 1. J. Dairy Sci. 71, 819–825. doi: 10.3168/jds.S0022-0302(88)79622-8
Shock, D. A., LeBlanc, S. J., Leslie, K. E., Hand, K., Godkin, M. A., Coe, J. B., et al. (2016). Studying the relationship between on-farm environmental conditions and local meteorological station data during the summer. J. Dairy Sci. 99, 2169–2179. doi: 10.3168/jds.2015-9795
Soriani, N., Panella, G., and Calamari, L. (2013). Rumination time during the summer season and its relationships with metabolic conditions and milk production. J. Dairy Sci. 96, 5082–5094. doi: 10.3168/jds.2013-6620
Stone, A. E., Jones, B. W., Becker, C. A., and Bewley, J. M. (2017). Influence of breed, milk yield, and temperature-humidity index on dairy cow lying time, neck activity, reticulorumen temperature, and rumination behavior. J. Dairy Sci. 100, 2395–2403. doi: 10.3168/jds.2016-11607
Tolkamp, B. J., Haskell, M. J., Langford, F. M., Roberts, D. J., and Morgan, C. A. (2010). Are cows more likely to lie down the longer they stand? Appl. Anim. Behav. Sci. 124, 1–10. doi: 10.1016/j.applanim.2010.02.004
Tucker, C. B., Jensen, M. B., de Passillé, A. M., Hänninen, L., and Rushen, J. (2021). Invited review: lying time and the welfare of dairy cows. J. Dairy Sci. 104, 20–46. doi: 10.3168/jds.2019-18074
Tucker, C. B., Rogers, A. R., Verkerk, G. A., Kendall, P. E., Webster, J. R., and Matthews, L. R. (2007). Effects of shelter and body condition on the behaviour and physiology of dairy cattle in winter. Appl. Anim. Behav. Sci. 105, 1–13. doi: 10.1016/j.applanim.2006.06.009
Tucker, C. B., Weary, D. M., and Fraser, D. (2003). Effects of three types of free-stall surfaces on preferences and stall usage by dairy cows. J. Dairy Sci. 86, 521–529. doi: 10.3168/jds.S0022-0302(03)73630-3
Vaculíková, M., Komzáková, I., and Chládek, G. (2017). The effect of low air temperature on behaviour and milk production in Holstein dairy cows. Acta Univ. Agric. et Silvic. Mendelianae Brun. 65, 1623–1627. doi: 10.11118/actaun201765051623
Vijayakumar, M., Park, J. H., Ki, K. S., Lim, D. H., Kim, S. B., Park, S. M., et al. (2017). The effect of lactation number, stage, length, and milking frequency on milk yield in Korean Holstein dairy cows using automatic milking system. Asian-Aust. J. Anim. Sci. 30, 1093–1098. doi: 10.5713/ajas.16.0882
von Keyserlingk, M. A. G., Martin, N. P., Kebreab, E., Knowlton, K. F., Grant, R. J., Stephenson, M., et al. (2013). Invited review: sustainability of the US dairy industry. J. Dairy Sci. 96, 5405–5425. doi: 10.3168/jds.2012-6354
Vtoryi, V., Vtoryi, S., and Ylyin, R. (2018). Investigations of temperature and humidity conditions in barn in winter.
Wathes, D. C., Cheng, Z., Bourne, N., Taylor, V. J., Coffey, M. P., and Brotherstone, S. (2007). Differences between primiparous and multiparous dairy cows in the inter-relationships between metabolic traits, milk yield and body condition score in the periparturient period. Domest. Anim. Endocrinol. 33, 203–225. doi: 10.1016/j.domaniend.2006.05.004
West, J. W. (2003). Effects of heat-stress on production in dairy cattle. J. Dairy Sci. 86, 2131–2144. doi: 10.3168/jds.S0022-0302(03)73803-X
West, J., Mullinix, B., and Bernard, J. (2003). Effects of hot, humid weather on milk temperature, dry matter intake, and milk yield of lactating dairy cows. J. Dairy Sci. 86, 232–242. doi: 10.3168/jds.S0022-0302(03)73602-9
Keywords: low temperature and high humidity, milk yield, rumination time, activity, parity
Citation: Song J, Yu Q, Wang X, Wang Y, Zhang Y and Sun Y (2023) Relationship between microclimate and cow behavior and milk yield under low-temperature and high-humidity conditions. Front. Ecol. Evol. 11:1058147. doi: 10.3389/fevo.2023.1058147
Edited by:
Benjamin Lamptey, University of Leeds, United KingdomReviewed by:
Marco Milanesi, University of Tuscia, ItalyCaleb Mensah, University of Energy and Natural Resources, Ghana
Meagan King, University of Manitoba, Canada
Copyright © 2023 Song, Yu, Wang, Wang, Zhang and Sun. This is an open-access article distributed under the terms of the Creative Commons Attribution License (CC BY). The use, distribution or reproduction in other forums is permitted, provided the original author(s) and the copyright owner(s) are credited and that the original publication in this journal is cited, in accordance with accepted academic practice. No use, distribution or reproduction is permitted which does not comply with these terms.
*Correspondence: Yonggen Zhang, zhangyonggen@neau.edu.cn; Yukun Sun,
sun_yukun@126.com
†These authors have contributed equally to this work and share first authorship