- 1Institute of Marine Sciences – OKEANOS, University of the Azores, Horta, Portugal
- 2IMAR – Instituto do Mar, Departamento de Oceanografia e Pescas, University of the Azores, Horta, Portugal
- 3Ocean Ecosystem Change and Conservation, University of Victoria, Victoria, VIC, Canada
- 4National Oceanography Centre, Southampton, United Kingdom
- 5Life Sciences Department, Natural History Museum, London, United Kingdom
- 6Oberösterreichische Landes-Kultur GmbH, Geowissenschafltiche Sammlungen, Leonding, Austria
- 7CIBIO – Centro de Investigação em Biodiversidade e Recursos Genéticos, InBIO Laboratório Associado, Ponta Delgada, Portugal
- 8Institute of Marine Sciences (ICM-CSIC), Barcelona, Spain
- 9Department of Natural History, University Museum of Bergen, University of Bergen, Bergen, Norway
- 10CIIMAR – Interdisciplinary Centre of Marine and Environmental Research, University of Porto, Matosinhos, Portugal
- 11Department of Biological Sciences, University of Bergen, Bergen, Norway
- 12Guy Harvey Oceanographic Research Center, Department of Marine and Environmental Sciences, Nova Southeastern University, Dania Beach, FL, United States
- 13CESAM – Centre for Environmental and Marine Studies and Biology Department, University of Aveiro, Aveiro, Portugal
- 14Department of Fisheries and Oceans Canada, St. John’s, NL, Canada
- 15Shirshov Institute of Oceanology, Russian Academy of Sciences, Moscow, Russia
- 16Veterinary Practitioner, Biarritz, France
Trait-based approaches that complement taxonomy-based studies have increased in popularity among the scientific community over the last decades. The collection of biological and ecological characteristics of species (i.e., traits) provides insight into species and ecosystem vulnerability to environmental and anthropogenic changes, as well as ecosystem functioning. Here, we present the FUN Azores trait database, describe our approach, evaluate its scope, compare it to other marine trait databases, and explore the spatial distribution of its traits with “functional maps.” While most of the available trait databases to date contain essential information to understand the functional diversity of a taxonomic or functional group, our ecosystem-based approach provides a comprehensive assessment of diverse fauna (i.e., meio-, macro-, and megafauna) from benthic and pelagic environments in the Azores Marine Park; including ridges, seamounts, hydrothermal vents, and the overlying water column. We used a collaborative approach involving 30 researchers with different expertise to develop the FUN Azores database, which contains compiled data on 14 traits representing morphological, behavioral, and life history characteristics for 1,210 species across 10 phyla. The “functional maps” show a distinct distribution of the two most common size classes, suggesting different communities with different functionalities. The following traits had the best scoring coverage (i.e., >95% of the species scored): maximum body size, body form, skeleton material, feeding structure, motility, environmental position, substratum affinity, distribution, and depth range; while traits related to species behavior (e.g., sociability or aggregation tendencies) and life history (e.g., developmental mechanism) had lower scoring coverage, highlighting the need for further research to fill these knowledge gaps. We found a larger number of species in the benthic compared to the pelagic environment and differing species composition between areas within the Azores Marine Park resulting from varying biodiversity, ecosystem types, sampling effort, and methodologies used. The FUN Azores database will foster and facilitate trait-based approaches in the area, develop a framework for expansion of cross-ecosystem and cross-taxa trait databases elsewhere, and improve our ecological understanding of the Azores Marine Park and its conservation requirements.
1. Introduction
A trait is a well-defined, measurable characteristic of a species representing its morphology, physiology, phenology, life history, or behavior, as well as its performance in an ecosystem (McGill et al., 2006; Violle, 2007; Cadotte et al., 2011). Functional traits describe species influence on ecosystem function (i.e., effect traits) or species response to environmental change (i.e., response traits; Díaz et al., 2013). Offering an alternative essential unit to community analyses, traits can be used to answer new ecological questions on global change science, resource management, and conservation. Traits have been referred to as “common currency” across taxa and ultimately ecosystem types and serve as a bridge between species diversity and ecosystem function and services (McGill et al., 2006; Violle et al., 2014; Gómez-Gras et al., 2021; Martini et al., 2021). These advantages explain an increasing tendency over the last decades to use trait-based approaches in ecological studies and to compile trait databases.
Trait-based approaches are an important component of conservation, which complement species diversity measures. For instance, Stuart-Smith et al. (2013) revealed diversity hotspots among shallow-water reef fishes using functional diversity indices based on traits. Moreover, monitoring the recovery of a number of reef communities following protection allowed to detect positive effects when the assessment included trait-based approaches and no effects when focusing solely on species diversity metrics (Coleman et al., 2015). Other studies based on trait vulnerability to environmental change have also predicted the risk of reduction in key ecosystem functions (e.g., habitat provisioning: Gómez-Gras et al., 2021).
The application of trait-based approaches to address key ecological questions and aid the prioritization processes for management and conservation, has prompted the publication of an increasing number of trait databases, which, in the marine environment, can be habitat-specific (e.g., deep-sea hydrothermal vents – sFDVent: Chapman et al., 2019), region-specific (The Arctic Traits database: Degen and Faulwetter, 2019; FishMed: Albouy et al., 2015; a trait collection of marine fish species from North Atlantic and Northeast Pacific continental shelf seas: Beukhof et al., 2019; and SAMT – South Australian Macrobenthic Traits: Lam-Gordillo et al., 2020), trait-specific (e.g., macrofaunal densities and biomass – BenBioDen: Stratmann et al., 2020), or taxon-specific (Polytraits: Faulwetter et al., 2014; The Coral Traits Database: Madin et al., 2016, 2017; and copepods: Brun et al., 2017). The selection of relevant traits from the extensive list of those that can be measured, as well as the collecting of trait information based on appropriate expertise, are often time-consuming tasks that benefit from a collaborative approach (Costello et al., 2015; Chapman et al., 2019). In our study, we contribute to the list of shallow-water and deep-sea trait databases with the FUN Azores database, a collaborative effort, which functionally characterizes ridge, seamount, hydrothermal vent ecosystems from the Azores Marine Park, also known as the Marine Park of the Azores. Following the definition of Marine Protected Areas by the IUCN (1988; i.e., “Any area of intertidal or subtidal terrain, together with its overlying water and associated flora, fauna, historical, and cultural features, which has been reserved by law or other effective means to protect part or all of the enclosed environment”) the Azores Marine Park (Calado et al., 2011), and therefore, the FUN Azores database, includes benthic, benthopelagic, and pelagic species.
The unique environmental conditions found on ridges, seamounts, and hydrothermal vents (e.g., abrupt topography, current acceleration, upwellings and tide amplification, and overall increased food and nutrient supply) may result in hotspots of marine diversity (Morato and Pauly, 2004; Morato et al., 2010). In fact, seamounts and ridges act as feeding grounds for top predators, including large fish, marine birds, and cetaceans (Morato et al., 2008; Tobeña et al., 2016), and support high densities and biomasses of benthic suspension and filter feeders, such as corals and sponges, that provide habitat for many demersal fish populations (Porteiro et al., 2013). Unique species occur at hydrothermal vents, where chemosynthetically derived primary production supports high biomasses of mostly benthic invertebrates and some fishes (Van Dover et al., 2018; Boschen-Rose and Colaço, 2021).
Paradoxically, the same uniqueness that promotes biodiversity on ridges, seamounts, and hydrothermal vents makes these systems vulnerable to anthropogenic activities. The abundant and biomass-rich fish populations support fisheries, and their unique geology makes them one of the few underwater systems suitable for mineral extraction, although this is still in an exploratory phase (Drazen et al., 2020). Exploitation activities are occurring in the context of global climate change where increasing CO2 emissions result mostly in varying temperatures, dissolved oxygen concentrations, and pH and carbonate saturation values (Sweetman et al., 2017). Increasing temperatures are expected to reduce body size of organisms, shift species distributions, and increase their metabolism, while likely deoxygenation effects include a decrease in abundance and biomass of larger organisms (i.e., macrofauna and megafauna; Sweetman et al., 2017). Ocean acidification may reduce the abundance and diversity of calcifying species, particularly species depositing more soluble CaCO3 mineral phases such as aragonite and high-Mg calcite (Figuerola et al., 2021, 2023), while increasing their metabolic demands (Sweetman et al., 2017). Altogether, these factors result in ridges, seamounts, and hydrothermal vents being some of the most resource-rich and vulnerable ecosystems in the marine environment, which, given their remoteness and lack of fundamental biological knowledge, require special attention.
The FUN Azores trait database is based on a collaborative effort involving 30 researchers with different expertise and includes a broad range of taxa from benthic and pelagic environments to provide an ecosystem-based overview of the functional diversity associated with the ridges, seamounts, and hydrothermal vents within the Azores Marine Park, including benthic ecosystem and its overlying water column. Here, we present the step-by-step process of species and trait collection, with a description of the collaborative approach used in the species-scoring process. We finally describe and highlight the usefulness of the FUN Azores trait database for promoting trait-based approaches in the area by presenting species and trait coverage, their spatial distribution with “functional maps,” and discuss the database strengths and limitations.
2. Materials and methods
2.1. Geographic coverage: the Azores Marine Park
The Azores Marine Park is one of the largest networks of Marine Protected Areas (MPAs) within the EU and is unique in integrating Areas Beyond National Jurisdiction (Calado et al., 2011; Maestro et al., 2020). This network of 15 MPAs covers a total area of 111,393 km2, encompassing 13 MPAs that include ridges, seamount and hydrothermal vent ecosystems. One of these, MPA 10 – MARNA, is largely unexplored and therefore only the remaining 12 MPAs are considered in our study (Figure 1; Supplementary Table S1; UNEP-WCMC, 2022). Their resource-rich nature has incentivized commercial fishing, resulting in early signs of intensive exploitation in the 1980s, leading to, during the 2000s, investment in marine science (i.e., increased number of projects), monitoring of MPAs, and the implementation of protective measures for certain habitats (i.e., sponge aggregations, hydrothermal vent fields, and deep-sea coral gardens and reefs) and species (e.g., the long-lived and late-maturing orange roughy Hoplostethus atlanticus; Abecasis et al., 2015).
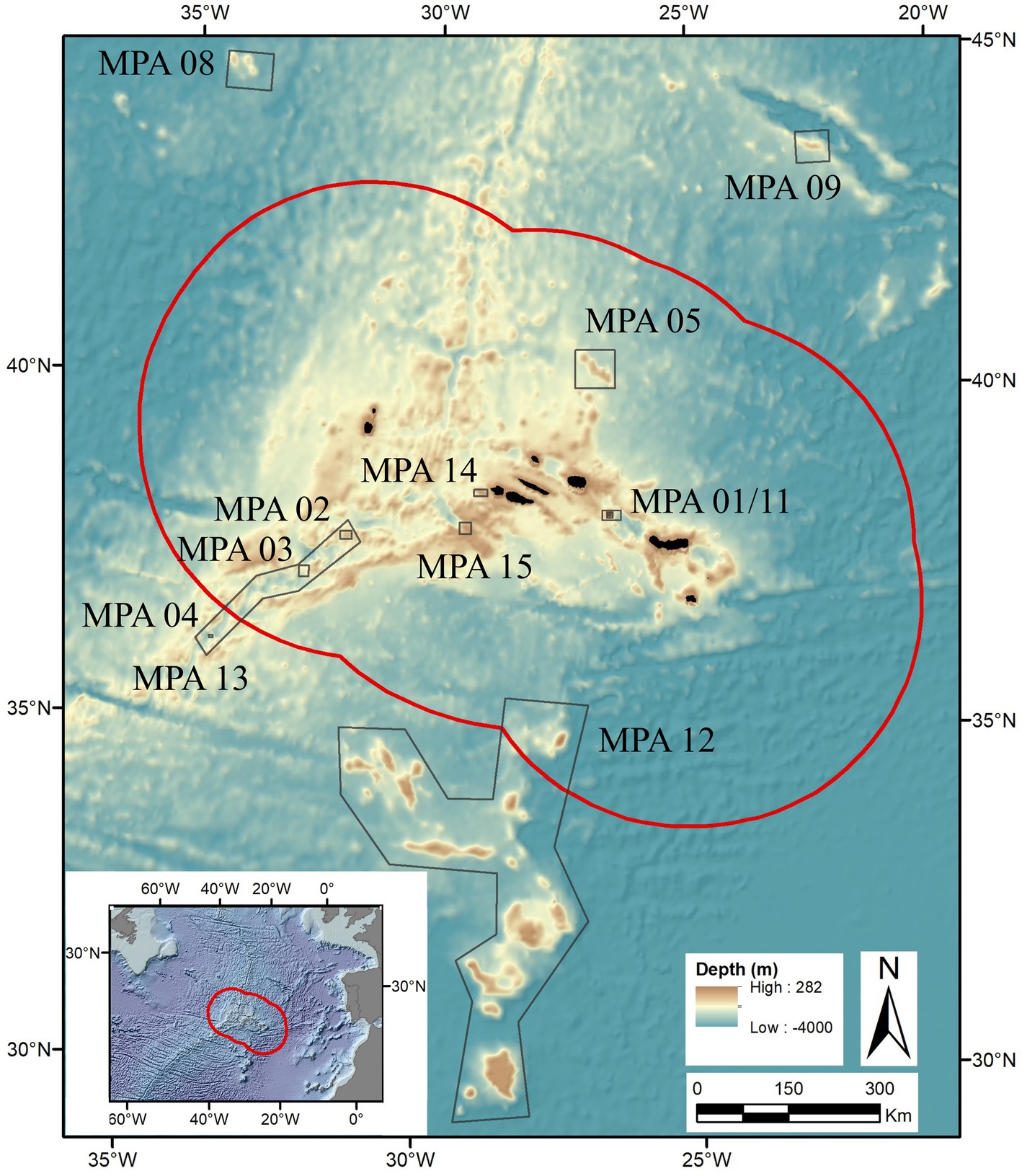
Figure 1. The 12 Marine Protected Areas (MPAs) from the Azores Marine Park included in the FUN Azores trait database.
2.2. Species records
We compiled a list of species occurring on ridges, seamounts, and hydrothermal vents within the Azores Marine Park and its overlying water column, including organisms belonging to a range of taxonomic groups. For the purposes of demonstrating key results of this study, marine species/taxa were classified into the following groups: Actinopterygii (gigaclass), Anthozoa (class), Aves (class), Bryozoa (phylum), Decapoda (order), Echinodermata (phylum), Chondrichthyes (i.e., class Elasmobranchii and class Holocephalii), Foraminifera (phylum), Hydrozoa and Scyphozoa (class), Malacostraca (class, excluding the order Decapoda), Mammalia (class), Mollusca (phylum), Ostracoda (class), Porifera (phylum), Reptilia (class), Thecostraca (class), and vermiformes (phyla Annelida and Nematoda). We sourced species records from the European Marine Observation Data Network (EMODnet; https://emodnet.ec.europa.eu/en), which includes a variety of public datasets, and we complemented this list with published studies (Supplementary Material 1). We verified the taxonomic status of each entry using the World Register of Marine Species Traits (2022), WoRMS Editorial Board (2022) and validated their occurrences within the Azores Marine Park using the OBIS Ocean Biodiversity Information System (2022). Duplicates were removed to obtain a final list of accepted and validated taxa (Supplementary Figure S1). We included taxa at the species level or genera if no representative species was already present and if the scoring could be conducted with sufficient accuracy (i.e., >75% species from that genus in the area have similar trait scores, acknowledging a low certainty score for the trait modalities scored in this way – see section 2.4).
2.3. Selection of traits
We selected traits that match the following criteria: (1) increase our understanding of a species performance in its ecosystem (e.g., Maximum Body Size), (2) characterize the capacity of a species to adapt to change (e.g., Distribution), (3) characterize the ability of an organism to resist perturbation and environmental change (e.g., Skeleton Material), (4) are transferable across groups of taxa, and (5) are present in existing databases to ensure cross-ecosystem compatibility in terminology and definitions. In Table 1, we provide the selected traits and modalities within each trait category, their definition, and the rationale for trait inclusion.
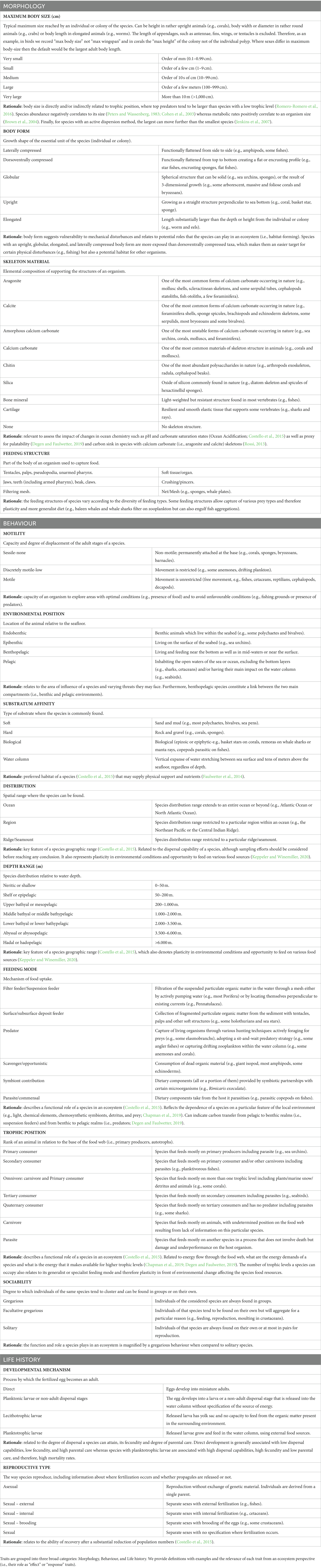
Table 1. List of species traits and modalities included in the FUN Azores database applicable to adult marine species from the Azores Marine Park.
2.4. Trait scoring
We scored traits based on the characteristics of the adult stage of each species, apart from the Developmental Mechanism trait, and according to global knowledge of the species characteristics (e.g., the scoring of the trait Maximum Body Size represent a potential maximum for a species across its range, rather than corresponding necessarily to the maximum size found in the Azores Marine Park). The resulting database, therefore, reflects “fundamental functional niches” (i.e., the potential functional space occupied by a particular species), from which we can infer potential responses to environmental heterogeneity and human-induced perturbations and, in turn, potential effects on overall ecosystem functioning. This approach may, however, result in lower predictability at highly localized spatial scales (e.g., for specific seamount areas; Cordlandwehr et al., 2013). We designed a flexible database that allows for multiple modalities for each trait (e.g., a single species can be scored both as a “Carnivore” and “Scavenger” in the Feeding mode trait) and for future updates as knowledge about the species included increases. This flexibility in trait scoring, together with the broad classification of trait modalities, encompasses the associated variability at the individual level resulting from localized abiotic and biotic interactions. Our study focuses on categorical and ordinal traits, despite continuous traits resulting in a higher quality of functional spaces (Maire et al., 2015), highlighting knowledge gaps in continuous traits for deep-sea species that result from sampling constrains (i.e., costs and accessibility).
We collected trait information from available databases, literature searches and expert knowledge (Supplementary Figure S2). Taxonomic classification was taken from the World Register of Marine Species (WoRMS; see footnote 1), species distribution from Ocean Biogeographic Information System (OBIS; see footnote 2), and depth ranges, life history, habitat, trophic ecology, species associations, morphology, and motility from other databases: FishBase (2022), SeaLifeBase (2022) TraitBank from the Encyclopedia of Life (2022), Marine Species Identification Portal (2022). and Biologic Traits Information Catalogue (BIOTIC7). We standardized the information extracted from the various databases to the stipulated definitions of FUN Azores (see Table 1). We filled in missing information with literature searches and inference from related species or from higher taxonomic levels (i.e., we selected the evolutionary closest taxon from which an observed trait value is available). Because this inference assumes that niche conservatism (i.e., tendency of a species to retain ancestral ecological characteristics; Wiens and Graham, 2005) prevails over niche partitioning (i.e., process by which competing species use the environmental resources in different ways, thereby permiting coexistence), we classified this type of scoring with low certainty as a quality measure (see paragraph below). Additionally, we consulted a total of 30 experts in different taxonomic groups to validate and complement the extracted data based on their knowledge, supported by available literature and observational data. The consultation process consisted of an agreement to the terms and conditions, provision of the database set of the appropriate taxonomic speciality, together with instructions on how to fill in the database in an excel file, and a follow-up virtual or in-person meeting to clarify the scoring system and modify the database structure accordingly if needed.
For each trait we scored, we mentioned the respective trait modality for the species, the source of information (i.e., a specific reference, an online database, and/or “Expert knowledge”), and we defined a certainty score that classified the level of confidence in the annotation. The certainty modalities are: 0-No knowledge from the trait, 1-Inferred trait, 2-Expert knowledge and observation and/or data published once, 3-Expert knowledge and observation and/or published more than once. The certainty classification represents a quality control while the traceability of information allows researchers to re-use the data with the appropriate context for specific research questions.
We error-checked initial versions of the database following a series of steps to ensure completeness and consistency of the scored data: (1) removal of duplicate entries and synonymized names, (2) confirmation of blank entries (i.e., missing information) and low certainty scores, (3) ensuring nomenclature uniformization using drop-down menus to enter the data, and (4) ensuring the biological plausibility of trait combinations (e.g., a “sessile” species cannot be found in the “water column”).
2.5. Data analyses
We mapped the distribution of the modal functional entities across all MPAs (i.e., “functional maps”) with ArcGIS Desktop (version 10.8.1.14362). We plotted the kernel density based on georeferenced records from EMODnet. For that purpose, we used the “Kernel density” tool in the Spatial Analyst ArcToolbox to calculate DENSITY with the GEODESIC method and an output cell size of 0.01.
We ran rarefaction curves with the function rarefy () {vegan} in R programing software (v.4.2.1) to compare biodiversity and sampling efforts among areas and environmental domains (i.e., Benthic, Benthopelagic, and Pelagic). The rarefaction curves that we present are based only on the data extracted from EMODnet repositories where we could find both species records and numbers of records for each species (i.e., EMODnet and literature species records).
3. Results and discussion
The FUN Azores trait database compiles relevant information to understand the functional ecology of the Azores Marine Park using an ecosystem-based approach that includes species from shallow waters to the deep sea and open ocean. This is the second contribution to deep-sea trait databases following Chapman et al. (2019) and the first one to include both chemosynthetic and non-chemosynthetic seamount and ridge ecosystems, with a broad taxonomic coverage from a wide range of organism sizes (i.e., millimeters to meters) and representing benthic and pelagic environments. Our final list contained 1,210 species distributed among 10 phyla and 14 traits classified either into morphological, behavioral, or life history categories. The data collection approach used in the construction of the FUN Azores database follows the methodology used in Chapman et al. (2019), who developed a protocol for including expert knowledge to score traits.
3.1. Cross-ecosystem and cross-taxa compatibility
A key aspect of trait-based ecology is the use of a “common currency” (i.e., traits; Violle et al., 2014; Martini et al., 2021) that is independent of geographic region and species, facilitating comparisons across ecosystems (e.g., communities from different seamounts or chemosynthetic and heterotrophic environments) and taxa (i.e., phylogenetically distant taxa such as cetaceans and corals). To contextualize the FUN Azores database, the traits included are comparable to biological characteristics found in existing marine trait databases (Table 2) in terms of their ecological relevance and the accessibility of the information. However, comparisons become difficult with freshwater and terrestrial databases that include at least double the number of traits and have a larger proportion of numerical traits (Serra et al., 2016; Fraser, 2020), highlighting the large differences in knowledge availability between these environments.
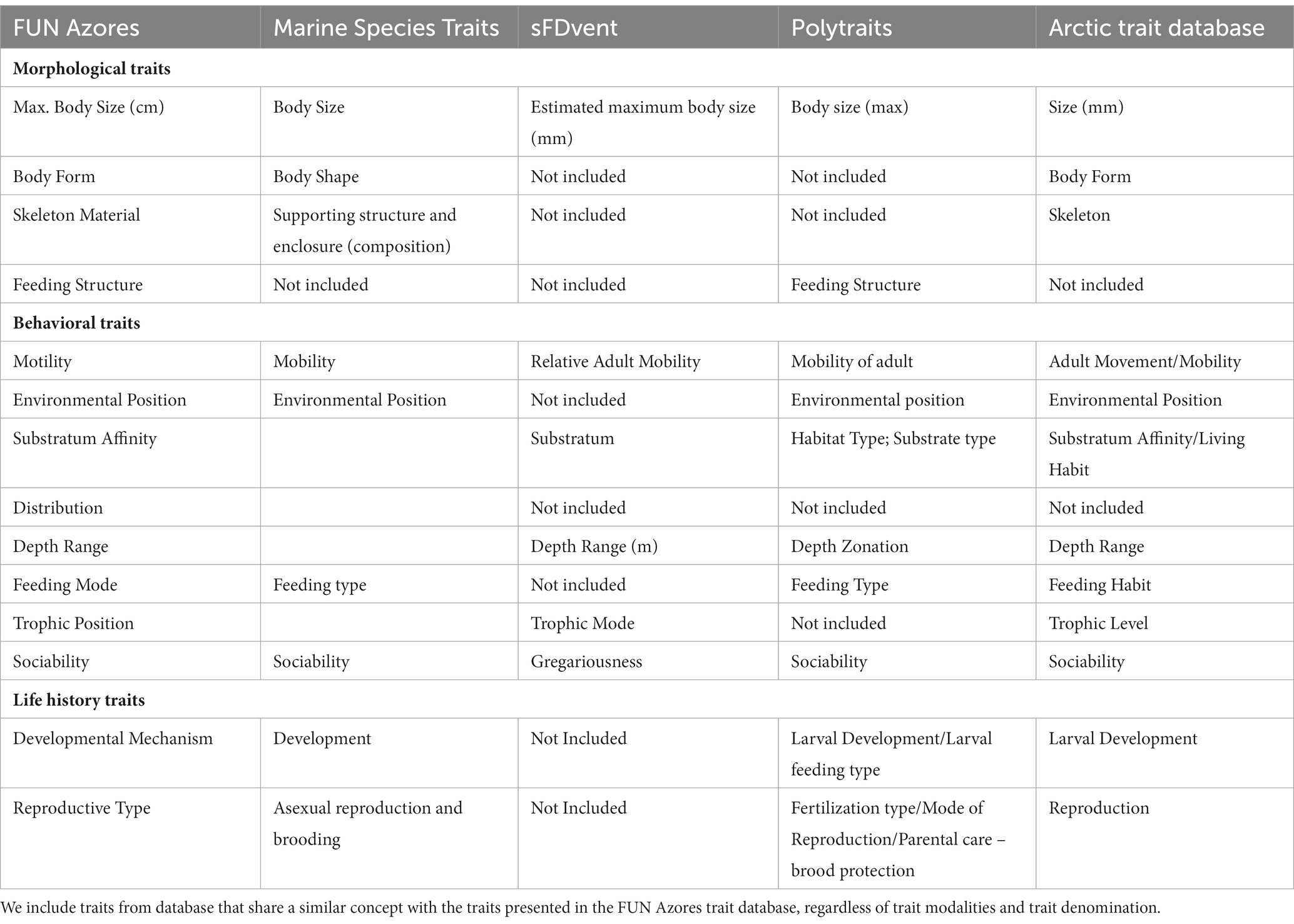
Table 2. Equivalences of the species traits used in the FUN Azores database and other relevant marine databases (i.e., Marine Species Traits, sFDVents, Polytraits, and Arctic trait database).
Costello et al. (2015) identified the following 10 traits as those considered most relevant by marine ecologists: Taxonomic classification, Environment, Geography, Depth, Substratum, Mobility, Skeleton, Diet, Body size, and Reproduction. These are all included in the FUN Azores database. Given the broader approach of FUN Azores (i.e., highly unrelated taxonomic groups and a combination of pelagic and benthic species) when compared to databases that focus on a particular taxonomic group (i.e., Polytraits: Faulwetter et al., 2014; marine copepods: Brun et al., 2017; and corals: Madin et al., 2016, 2017) or environmental compartment (i.e., benthos in the Arctic Trait Database: Degen and Faulwetter, 2019; and macrobenthos in Australia: Lam-Gordillo et al., 2020), the modalities for the same traits can differ among databases resulting in a broader classification in the FUN Azores. For example, where the FUN Azores includes three categories to describe fish morphology (i.e., “Elongated,” “Dorsoventrally compressed,” and “Laterally compressed”), fish databases will include more detailed information (e.g., “Deep,” “Short,” “Elongated,” “Fusiform”; Albouy et al., 2015), thereby increasing cross-taxon comparisons at the expense of resolution.
3.2. Trait coverage
Traits included in the FUN Azores trait database cover a wide variety of functions within an ecosystem. Some of them represent multiple ecosystem functions such as Maximum Body Size, which reflects both carbon storage and food web structure, or represent specific functions in the ecosystem, such as Developmental Mechanism, which reflects the dispersal capabilities of the early life-history stages of the species. Traits that are commonly found in the original description of a species and/or in lists of species records are scored for most taxa in FUN Azores and with a high certainty score (i.e., Maximum Body Size, Body Form, Motility, Environmental Position, Distribution, and Depth Range).
The modal functional entity within the database is a small to medium-sized species (i.e., from a few to tens of centimeters) that corresponds to the description of carnivorous epibenthic Actinopterygii inhabiting the upper bathyal (i.e., 200–1,000 m) with non-adult dispersal and sexual reproduction (Supplementary Table S2). The “functional maps” (i.e., spatial distribution of the modal functional entities; Figure 2) reveals important characteristics of the database and of the different functions across MPAs. For example, there is a noticeable heterogeneity in the distribution of the two main size classes, where small organisms in the size range 1 to 9.99 cm are more prevalent in the south (MPA12) and organisms in the size range 10 to 99.9 cm are more prevalent in the center of the area of study (MPAs 14 – Condor Bank and 15 – Princesa Alice Bank), which suggests a different community with different functionalities. Other traits have a more homogenous representation of the main modality over all MPAs, resulting from their broad spatial coverage and representation in a large number of species (i.e., “Epibenthic,” “Oceanic,” “Upper bathyal,” “Solitary”), or because the trait modality has a broad scope in order to accommodate uncertainties and lack of specific information (i.e., “Carnivore,” “Planktonic or non-adult dispersal,” and “Sexual” reproduction). A significant number of trait modalities match their spatial distribution (i.e., “Laterally compressed,” “Bone mineral” and “Aragonite,” “Jaws, teeth, plates, bills or beaks” as feeding structure, “Motile” and “Predators”). These define the vast majority of Actinopterygii and highlight the importance of this taxonomic group within the FUN Azores database. The overall distribution of the modal functional entities highlights their presence in three areas with different spatial extents (i.e., MPA 14 – Condor Bank, MPA 15 – Princesa Alice Bank, MPA 12 – Meteor Ridge), again highlighting the larger knowledge base in those areas as well as the good representation of both species and functions.
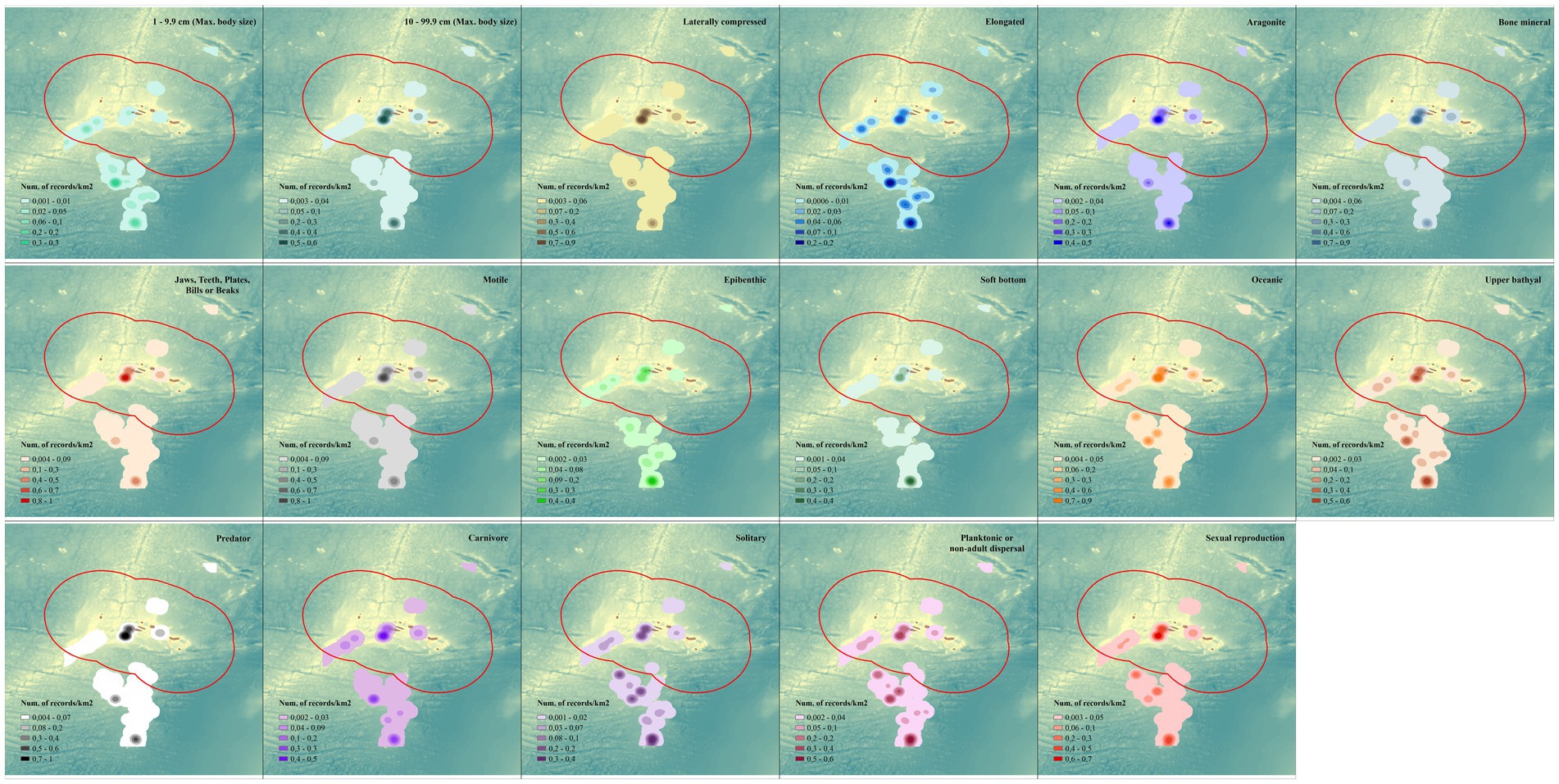
Figure 2. Kernel distribution of the modal functional entities of the FUN Azores database within each MPA of the Azores Marine Park.
Most of the available trait information was scored for species from the phylum Chordata (i.e., marine Mammalia, marine Aves, marine Reptilia, Actinopterygii, and Chondrichthyes), whereas little information exists for invertebrates (e.g., Ostracoda, vermiformes, Echinoderms; Supplementary Table S2 and Supplementary Figure S3). The specificity of the information required to score “Trophic position,” usually determined from either gut contents or biochemical analyses, complicated the scoring of this trait when compared to other measurable morphological traits such as “Feeding structure,” particularly for invertebrates. Furthermore, information relevant to behavioral and life history traits such as “Sociability” and “Larval development,” which requires detailed observations or even experimentation in the laboratory, lacked information or had low certainty scores.
Conservation efforts based on trait-based approaches frequently use only a few traits (e.g., Maximum Body Size, Motility, Distribution, and Feeding Mode; Coleman et al., 2015), which are all well-represented in the FUN Azores database (Supplementary Table S2 and Supplementary Figure S3) and represent various components of the overall functional diversity. However, our extensive review of functional diversity has also highlighted knowledge gaps for the Azores Marine Park that are important for managing Marine Protected Area networks. For example, there is a critical gap in our knowledge of traits relevant to ecosystem recovery after perturbation, disturbance, or a change in the environment (i.e., reproductive type, larval development, Supplementary Figure S3; and growth rates and life span, which are not included in the database because of scarcity of the data). There is also a general lack of information for those invertebrates that link the pelagic chordates with the benthos (e.g., copepods, which are the diet of mesopelagic fishes that undertake vertical migrations, or the habitat provisioning of certain sessile invertebrates – corals, sponges, bryozoans, etc. – for fishes and their early stages in life; Porteiro et al., 2013; Drazen et al., 2020). These data are essential for effective ecosystem-based management.
3.3. Taxonomic coverage and sampling effort
The most species-rich taxonomic groups were the Actinopterygii (n = 316; >25%) and Mollusca (n = 164; 13.5%), followed by Foraminifera (n = 120; 9.9%), Hydrozoa (n = 107; 8.8%), Anthozoa (n = 90; 7.5%), and non-decapod Malacostraca (n = 75; 6.2%).
Particularities of each MPA of the Azores Marine Park contributed to the final taxonomic coverage of the FUN Azores trait database (i.e., species composition, surface area covered, sampling effort and methodology; Supplementary Figure S4). As a result, the number of species reported in the seamount complex found in MPA 12 (Meteor Ridge – South of the Azores) by far exceeds that of the less sampled Sedlo seamount (MPA 05). Condor (MPA 14) is one of the most studied seamounts of the Azores Marine Park and is represented by a larger number of species in the FUN Azores trait database than the less visited, but with similar surface area, Altair (MPA 08) and Antialtair (MPA 09) seamounts. Most of the taxonomic groups known from Condor belong to the megafaunal size spectrum (e.g., fishes, corals, cetaceans, birds, cephalopods, sponges) and have been documented by intensive visual, video and longline fisheries surveys (Giacomello and Menezes, 2011; Porteiro et al., 2013; Silva et al., 2013). In contrast, on the Meteor Ridge to the south of the Azores (MPA 12) we have a larger representation of infauna (e.g., some foraminifera and vermiforme taxa) than on any other seamounts, as a result of the collection of numerous sediment samples during various research expeditions (Surugiu et al., 2008; Carvalho et al., 2020; Hoffman et al., 2020). Similarly, there is a low representation of Actinopterygii in MPA12 compared to all other MPAs. Perhaps the most unique fauna is that found in MPA 13, which includes hydrothermal vent species that are not found in other MPAs. These contrasts in taxonomic coverage are evident in both benthic and pelagic environments (Supplementary Figure S4).
The pelagic environment appears to be better sampled than the benthos (Supplementary Figure S5). In most cases we can attain almost complete taxonomic coverage of pelagic species, a statement that is far from true for the benthic and benthopelagic environments, where the lower sampling effort and undersampling of habitat variability result in rarefaction curves hardly reaching an asymptote with increasing number of records from the EMODnet database. In fact, with similar sampling efforts (i.e., same Num. ind.) the benthic environment has a higher number of species in most MPAs.
4. Future research directions
We emphasize the need for collaborative initiatives and the use of data inferences to improve the completeness of databases, and a transition from categorical to ordinal and numerical traits in this and future trait databases. We expect an iterative process with future versions of FUN Azores incorporating new knowledge, thereby increasing its taxonomic range and hopefully introducing a temporal dimension, a key element that is already being included in terrestrial plant databases (Kattge et al., 2020). We particularly emphasize the need for increased observation, experimentation, and modeling in the following three areas: (1) life history traits (e.g., developmental mechanism and larval dispersal), (2) variability in sampling methodologies and, therefore, targeted species, in each of the Azores Marine Park MPAs, and (3) the benthic environment, which in the Azores Marine Park is undersampled while also being the most species-rich.
The knowledge acquired will help to enhance the effective management of one of the largest Marine Protected Area networks in Europe.
Data availability statement
The original contributions presented in the study are published in a database repository following the FAIR principles (i.e., Findable, Accessible, Interoperable, and Reusable; Wilkinson et al. 2016). This data can be found here: PANGAEA, https://doi.org/10.1594/PANGAEA.955357. Further inquiries can be directed to the corresponding author.
Author contributions
Order of authorship is as follows: (a) first author (NC-L); (b) core FUN Azores scoring by the number of species contributed to; (c) senior author (AC). All manuscript authors contributed data to the database, with NC-L, AEB, DCu, and AC designing and gathering data from existing literary and online sources as a start point and organizing contributions from international collaborators. NC-L, EFSC, and AC cleaned and processed the data. All authors checked and edited and/or approved the recommended dataset and manuscript.
Funding
This work was performed under the framework of the project FunAzores co-funded by AÇORES 2020, through the FEDER fund from the European Union.: ACORES 01-0145-FEDER-000123. Okeanos team received national funds through the FCT – Foundation for Science and Technology, I.P., under the Project UIDB/05634/2020 and UIDP/05634/2020 and through the Regional Government of the Azores through the initiative to support the Research Centers of the University of the Azores and through the project M1.1.A/REEQ.CIENTÍFICO UI&D/2021/010. AC work was supported by FCT/MCTES through national funds in the scope of the CEEC contract CEECIND/00101/2021, NC-L and DCa are also supported by the national funds through the -FCTFoundation for Science and Technology under the project UIDP/05634/2020 granted to Okeanos respectivelly. AC, EG, DCu, and MAS were co-financed by the Operational Program AZORES 2020, through the Fund 01-0145-FEDER-000140 “MarAZ Researchers: Consolidate a body of researchers in Marine Sciences in the Azores” of the European Union. BF has received funding from the postdoctoral fellowships programme Beatriu de Pinós funded by the Secretary of Universities and Research (Government of Catalonia) and by the Horizon 2020 programme of research and innovation of the European Union under the Marie Skłodowska-Curie grant agreement no 801370 (Incorporation grant 2019 BP 00183) and from the MedCalRes project Grant PID2021-125323OA-I00 funded by MCIN/AEI/10.13039/501100011033 and by ‘ERDF A way of making Europe’. LF was supported by the project SOS TubaProf (MAR-01.03.02-FEAMP-0040). TH was supported by the UK Natural Environment Research Council Climate Linked Atlantic Sector Science project (NE/R015953/1) and from the European Union’s Horizon 2020 research and innovation programme under grant agreement no. 818123 (iAtlantic). JX research is further supported by national funds through FCT Foundation for Science and Technology within the scope of UIDB/04423/2020, UIDP/04423/2020, and CEECIND/00577/2018. SPR work was supported by FCT/MCTES through national funds in the scope of the CEEC contract (CEECIND/00758/2017) and grants UIDP/50017/2020, UIDB/50017/2020, and LA/P/0094/2020 attributed to CESAM. MCS was supported by Program Stimulus of Scientific Employment (CCCIND/03346/2020) from the Fundacṃão para a Ciência e Tecnologia.
Conflict of interest
The authors declare that the research was conducted in the absence of any commercial or financial relationships that could be construed as a potential conflict of interest.
Publisher’s note
All claims expressed in this article are solely those of the authors and do not necessarily represent those of their affiliated organizations, or those of the publisher, the editors and the reviewers. Any product that may be evaluated in this article, or claim that may be made by its manufacturer, is not guaranteed or endorsed by the publisher.
Supplementary material
The Supplementary material for this article can be found online at: https://www.frontiersin.org/articles/10.3389/fevo.2023.1050268/full#supplementary-material
Footnotes
References
Abecasis, R. C., Afonso, P., Colaço, A., Longnecker, N., Clifton, J., Schmidt, L., et al. (2015). Marine conservation in the Azores: evaluating marine protected area development in a remote island context. Front. Mar. Sci. 2:104. doi: 10.3389/fmars.2015.00104
Albouy, C., Lasram, F. B. R., Velez, L., Guilhaumon, F., Meynard, C. N., Boyer, S., et al. (2015). FishMed: traits, phylogeny, current and projected species distribution of mediterranean fishes, and environmental data. Ecology 96, 2312–2313. doi: 10.1890/14-2279.1
Beukhof, E., Dencker, T. S., Palomares, M. L. D., and Maureaud, A. (2019). Supplementary information to: a trait collection of marine fish species from North Atlantic and Northeast Pacific continental shelf seas. Pangaea 10:1594. doi: 10.1594/PANGAEA900866
Boschen-Rose, R. E., and Colaço, A. (2021). Northern mid-Atlantic ridge hydrothermal habitats: a systematic review of knowledge status for environmental management. Front. Mar. Sci. 8, 1–23. doi: 10.3389/fmars.2021.657358
Brown, J. H., Gillooly, J. F., Allen, A. P., Savage, V. M., and West, G. B. (2004). Toward a metabolic theory of ecology. Ecology 85, 1771–1789. doi: 10.1890/03-9000
Brun, P., Payne, M. R., and Kiørboe, T. (2017). A trait database for marine copepods. Earth. Syst. Sci. Data 9, 99–113. doi: 10.5194/essd-9-99-2017
Cadotte, M. W., Carscadden, K., and Mirotchnick, N. (2011). Beyond species: functional diversity and the maintenance of ecological processes and services. J. Appl. Ecol. 48, 1079–1087. doi: 10.1111/j.1365-2664.2011.02048.x
Calado, H., Ng, K., Lopes, C., and Paramio, L. (2011). Introducing a legal management instrument for offshore marine protected areas in the Azores-the Azores Marine Park. Environ. Sci. Pol. 14, 1175–1187. doi: 10.1016/j.envsci.2011.09.001
Carvalho, F. C., Cárdenas, P., Ríos, P., Cristobo, J., Rapp, H. T., and Xavier, J. R. (2020). Rock sponges (lithistid demospongiae) of the Northeast Atlantic seamounts, with description of ten new species. PeerJ 8, e8703–e8787. doi: 10.7717/peerj.8703
Chapman, A. S. A., Beaulieu, S. E., Colaço, A., Gebruk, A. V., Hilario, A., Kihara, T. C., et al. (2019). sFDvent: a global trait database for deep-sea hydrothermal-vent fauna. Glob. Ecol. Biogeogr. 28, 1538–1551. doi: 10.1111/geb.12975
Cohen, J. E., Jonsson, T., and Carpenter, S. R. (2003). Ecological community description using the food web, species abundance, and body size. Proc. Natl. Acad. Sci. 100, 1781–1786. doi: 10.1073/PNAS.232715699
Coleman, M. A., Bates, A. E., Stuart-Smith, R. D., Malcolm, H. A., Harasti, D., Jordan, A., et al. (2015). Functional traits reveal early responses in marine reserves following protection from fishing. Divers. Distrib. 21, 876–887. doi: 10.1111/ddi.12309
Cordlandwehr, V., Meredith, R. L., Ozinga, W. A., Bekker, R. M., Van Groenendael, J. M., and Bakker, J. P. (2013). Do plant traits retrieved from a database accurately predict on-site measurements? J. Ecol. 101, 662–670. doi: 10.1111/1365-2745.12091
Costello, M. J., Claus, S., Dekeyzer, S., Vandepitte, L., Tuama, É. Ó., Lear, D., et al. (2015). Biological and ecological traits of marine species. PeerJ 3:e1201. doi: 10.7717/peerj.1201
Degen, R., and Faulwetter, S. (2019). The Arctic traits database – a repository of Arctic benthic invertebrate traits. Earth Syst. Sci. Data 11, 301–322. doi: 10.5194/essd-11-301-2019
Díaz, S., Purvis, A., Cornelissen, J. H. C., Mace, G. M., Donoghue, M. J., Ewers, R. M., et al. (2013). Functional traits, the phylogeny of function, and ecosystem service vulnerability. Ecol. Evol. 3, 2958–2975. doi: 10.1002/ece3.601
Drazen, J. C., Smith, C. R., Gjerde, K. M., Haddock, S. H. D., Carter, G. S., Choy, C. A., et al. (2020). Opinion: midwater ecosystems must be considered when evaluating environmental risks of deep-sea mining. Proc. Natl. Acad. Sci. 117, 17455–17460. doi: 10.1073/pnas.2011914117
Encyclopedia of Life (2022). Available at: http://eol.org (Accessed January 1, 2022).
Faulwetter, S., Markantonatou, V., Pavloudi, C., Papageorgiou, N., Keklikoglou, K., Chatzinikolaou, E., et al. (2014). Polytraits: a database on biological traits of marine polychaetes. Biodivers. Data J. 2:e1024. doi: 10.3897/BDJ.2.e1024
Figuerola, B., Griffiths, H., Krzeminska, M., Piwoni-Piorewicz, A., Iglikowska, A., and Kuklinski, P. (2023). Temperature as a likely driver shaping global patterns in mineralogical composition in bryozoans: implications for marine calcifiers under global change. Ecography e06381. doi: 10.1111/ecog.06381
Figuerola, B., Hancock, A. M., Bax, N., Cummings, V. J., Downey, R., Griffiths, H. J., et al. (2021). A review and meta-analysis of potential impacts of ocean acidification on marine Calcifiers from the Southern Ocean. Front. Mar. Sci. 8:584445. doi: 10.3389/fmars.2021.584445
FishBase. (2022). World wide web electronic publication. Available at: www.fishbase.org (Accessed January 1, 2022).
Fraser, L. H. (2020). TRY—a plant trait database of databases. Glob. Chang. Biol. 26, 189–190. doi: 10.1111/gcb.14869
Giacomello, E., and Menezes, G. (Eds) (2011). CONDOR observatory for long-term study and monitoring of Azorean seamount ecosystems. Final project report. Arquivos do DOP, Série Estudos 1/2012. 261 pp + 9 annexes
Gómez-Gras, D., Linares, C., Dornelas, M., Madin, J. S., Brambilla, V., Ledoux, J. B., et al. (2021). Climate change transforms the functional identity of Mediterranean coralligenous assemblages. Ecol. Lett. 24, 1038–1051. doi: 10.1111/ele.13718
Hoffman, L., Gofas, S., and Freiwald, A. (2020). A large biodiversity of skeneimorph (Gastropoda: Vetigastropoda) species from the south Azorean seamount chain, with the description of seventeen new species. Iberus 38, 1–82.
Jenkins, D. G., Brescacin, C. R., Duxbury, C. V., Elliott, J. A., Evans, J. A., Grablow, K. R., et al. (2007). Does size matter for dispersal distance? Glob. Ecol. Biogeogr. 16, 415–425. doi: 10.1111/J.1466-8238.2007.00312.X
Kattge, J., Bönisch, G., Díaz, S., Lavorel, S., Prentice, I. C., Leadley, P., et al. (2020). TRY plant trait database – enhanced coverage and open access. Glob. Chang. Biol. 26, 119–188. doi: 10.1111/gcb.14904
Keppeler, F. W., and Winemiller, K. O. (2020). Incorporating indirect pathways in body size–trophic position relationships. Oecologia 194, 177–191. doi: 10.1007/S00442-020-04752-3/FIGURES/5
Lam-Gordillo, O., Baring, R., and Dittmann, S. (2020). Establishing the south Australian macrobenthic traits (SAMT) database: a trait classification for functional assessments. Ecol. Evol. 10, 14372–14387. doi: 10.1002/ece3.7040
Madin, J. S., Anderson, K. D., Andreasen, M. H., Bridge, T. C. L., Cairns, S. D., Connolly, S. R., et al. (2016). The coral trait database, a curated database of trait information for coral species from the global oceans. Sci. Data 3:160017. doi: 10.1038/sdata.2016.17
Madin, J. S., Anderson, K. D., Andreasen, M. H., Bridge, T. C. L., Cairns, S. D., Connolly, S. R., et al. (2017). Corrigendum: the coral trait database, a curated database of trait information for coral species from the global oceans. Sci. Data 4:170174. doi: 10.1038/sdata.2017.174
Maestro, M., Chica-Ruiz, J. A., and Pérez-Cayeiro, M. L. (2020). Analysis of marine protected area management: the Marine Park of the Azores (Portugal). Mar. Policy 119:104104. doi: 10.1016/j.marpol.2020.104104
Maire, E., Grenouillet, G., Brosse, S., and Villéger, S. (2015). How many dimensions are needed to accurately assess functional diversity? A pragmatic approach for assessing the quality of functional spaces. Glob. Ecol. Biogeogr. 24, 728–740. doi: 10.1111/geb.12299
Marine Species Identification Portal. (2022). Available at: https://linnaeus.naturalis.nl/ (Accessed January 1, 2022).
Marine Species Traits. (2022). Available at: http://www.marinespecies.org/traits (Accessed November 29, 2022).
Martini, S., Larras, F., Boyé, A., Faure, E., Aberle, N., Archambault, P., et al. (2021). Functional trait-based approaches as a common framework for aquatic ecologists. Limnol. Oceanogr. 66, 965–994. doi: 10.1002/lno.11655
McGill, B., Enquist, B., Weiher, E., and Westboy, M. (2006). Rebuilding community ecology from functional traits. Trends Ecol. Evol. 21, 178–185. doi: 10.1016/j.tree.2006.02.002
Morato, T., Hoyle, S. D., Allain, V., and Nicol, S. J. (2010). Seamounts are hotspots of pelagic biodiversity in the open ocean. Proc. Natl. Acad. Sci. U. S. A. 107, 9707–9711. doi: 10.1073/pnas.0910290107
Morato, T., and Pauly, D. (2004). Seamounts: biodiversity and fisheries. Fish. Bethesda 12:78. http://www.seaaroundus.org/report/seamounts.htm
Morato, T., Varkey, D. A., Damaso, C., Machete, M., Santos, M., Prieto, R., et al. (2008). Evidence of a seamount effect on aggregating visitors. Mar. Ecol. Prog. Ser. 357, 23–32. doi: 10.3354/meps07269
OBIS Ocean biodiversity information system (2022). Paris: Intergovernmental Oceanographic Commission. Available at: https://obis.org (Accessed January 1, 2022).
Peters, R. H., and Wassenberg, K. (1983). The effect of body size on animal abundance. Oecologia 60, 89–96. doi: 10.1007/BF00379325
Porteiro, F. M., Gomes-Pereira, J. N., Pham, C. K., Tempera, F., and Santos, R. S. (2013). Distribution and habitat association of benthic fish on the Condor seamount (NE Atlantic, Azores) from in situ observations. Deep Res. Part II Top. Stud. Oceanogr. 98, 114–128. doi: 10.1016/j.dsr2.2013.09.015
Romero-Romero, S., Molina-Ramírez, A., Höfer, J., and Acuña, J. L. (2016). Body size-based trophic structure of a deep marine ecosystem. Ecology 97, 171–181. doi: 10.1890/15-0234.1
Rossi, S. (2013). The destruction of the ‘animal forests’ in the oceans: Towards an over-simplification of the benthic ecosystems. Ocean Coast. Manag. 84, 77–85. doi: 10.1016/J.OCECOAMAN.2013.07.004
SeaLifeBase. World wide web electronic publication. (2022). Available at: www.sealifebase.org (Accessed January 1, 2022).
Serra, S. R. Q., Cobo, F., Graç, M. A. S., Dolédec, S., and Feio, M. J. (2016). Synthesising the trait information of European Chironomidae (Insecta: Diptera): towards a new database. Ecol. Indic. 61, 282–292. doi: 10.1016/j.ecolind.2015.09.028
Silva, M. A., Prieto, R., Jonsen, I., Baumgartner, M. F., and Santos, R. S. (2013). North Atlantic blue and fin whales suspend their spring migration to forage in middle latitudes: building up energy reserves for the journey? PLoS One 8:e76507. doi: 10.1371/journal.pone.0076507
Stratmann, T., van Oevelen, D., Martínez Arbizu, P., Wei, C. L., Liao, J. X., Cusson, M., et al. (2020). The BenBioDen database, a global database for meio-, macro-and megabenthic biomass and densities. Sci. Data 7, 1–12. doi: 10.1038/s41597-020-0551-2
Stuart-Smith, R. D., Bates, A. E., Lefcheck, J. S., Duffy, J. E., Baker, S. C., Thomson, R. J., et al. (2013). Integrating abundance and functional traits reveals new global hotspots of fish diversity. Nature 501, 539–542. doi: 10.1038/nature12529
Surugiu, V., Dauvin, J. C., Gillet, P., and Ruellet, T. (2008). Can seamounts provide a good habitat for polychaete annelids? Example of the northeastern Atlantic seamounts. Deep. Res. Part I Oceanogr. Res. Pap. 55, 1515–1531. doi: 10.1016/j.dsr.2008.06.012
Sweetman, A. K., Thurber, A. R., Smith, C. R., Levin, L. A., Mora, C., Wei, C. L., et al. (2017). Major impacts of climate change on deep-sea benthic ecosystems. Elementa 5:1–23. doi: 10.1525/elementa.203
Tobeña, M., Prieto, R., Machete, M., and Silva, M. A. (2016). Modeling the potential distribution and richness of cetaceans in the Azores from fisheries observer program data. Front. Mar. Sci. 3:202. doi: 10.3389/fmars.2016.00202
UNEP-WCMC. (2022). Protected area profile for Portugal from the world database of protected areas. Available at: www.protectedplanet.net. (Accessed August 15, 2022)
Van Dover, C. L., Arnaud-Haond, S., Gianni, M., Helmreich, S., Huber, J. A., Jaeckel, A. L., et al. (2018). Scientific rationale and international obligations for protection of active hydrothermal vent ecosystems from Deep-Sea mining. Mar. Policy 90, 20–28. doi: 10.1016/j.marpol.2018.01.020
Violle, C. (2007). Let the concept of trait be functional! Oikos 116, 882–892. doi: 10.1111/j.0030-1299.2007.15559.x
Violle, C., Reich, P. B., Pacala, S. W., Enquist, B. J., and Kattge, J. (2014). The emergence and promise of functional biogeography. Proc. Natl. Acad. Sci. U. S. A. 111, 13690–13696. doi: 10.1073/pnas.1415442111
Wiens, J. J., and Graham, C. H. (2005). Niche conservatism: integrating evolution, ecology, and conservation biology. Annu. Rev. Ecol. Evol. Syst. 36, 519–539. doi: 10.1146/annurev.ecolsys.36.102803.095431
Wilkinson, M. D., Dumontier, M., Aalbersberg, Ij. J., Appleton, G., Axton, M., Baak, A., et al. (2016). Comment: The FAIR Guiding Principles for scientific data management and stewardship. Sci. Data 3, 1–9. doi: 10.1038/sdata.2016.18
WoRMS Editorial Board (2022). World register of marine species. Available at: https://www.marinespecies.orgatVLIZ (Accessed August 31, 2022).
Keywords: behavior, life history, seamounts and ridges, hydrothermal vents, collaborative effort, trait-based approaches, animal morphology, Marine Protected Areas
Citation: Campanyà-Llovet N, Bates AE, Cuvelier D, Giacomello E, Catarino D, Gooday AJ, Berning B, Figuerola B, Malaquias MAE, Moura CJ, Xavier JR, Sutton TT, Fauconnet L, Ramalho SP, Neves BdM, Menezes GM, Horton T, Gebruk AV, Minin K, Bried J, Molodtsova T, Silva MA, Dilman A, Kremenetskaia A, Costa EFS, Clarke J, Martins HR, Pham CK, Carreiro-Silva M and Colaço A (2023) FUN Azores: a FUNctional trait database for the meio-, macro-, and megafauna from the Azores Marine Park (Mid-Atlantic Ridge). Front. Ecol. Evol. 11:1050268. doi: 10.3389/fevo.2023.1050268
Edited by:
Peter Convey, British Antarctic Survey (BAS), United KingdomReviewed by:
Sarah Faulwetter, University of Patras, GreeceKai Horst George, Senckenberg am Meer Wilhelmshaven, Germany
Copyright © 2023 Campanyà-Llovet, Bates, Cuvelier, Giacomello, Catarino, Gooday, Berning, Figuerola, Malaquias, Moura, Xavier, Sutton, Fauconnet, Ramalho, Neves, Menezes, Horton, Gebruk, Minin, Bried, Molodtsova, Silva, Dilman, Kremenetskaia, Costa, Clarke, Martins, Pham, Carreiro-Silva and Colaço. This is an open-access article distributed under the terms of the Creative Commons Attribution License (CC BY). The use, distribution or reproduction in other forums is permitted, provided the original author(s) and the copyright owner(s) are credited and that the original publication in this journal is cited, in accordance with accepted academic practice. No use, distribution or reproduction is permitted which does not comply with these terms.
*Correspondence: Neus Campanyà-Llovet, bmV1cy5jaS5sbG92ZXRAdWFjLnB0