- Department of Entomology, National Chung Hsing University, Taichung, Taiwan
Urbanization may lead to changes in assemblage and result in shifts in trait distribution from natural habitats to highly urbanized habitats. The shift in functional traits can affect ecosystem functions in urban areas. This study explored the foraging period of ants over 72 h and determined the relationship between the behavioral, morphological, and physiological traits of local foragers ants and environmental conditions in urban and forest sites. In addition, this study examined the ants’ ecosystem functions and compared it with that of their forest counterparts. Our results revealed that the foraging period of ants (i.e., Cardiocondyla sp.1, Monomorium chinense, Paratrechina longicornis, Pheidole megacephala, and Solenopsis sp.1) in urban areas peaked between 0900 and 1500 and that of some ants (i.e., Carebara diversa, P. megacephala, Pheidole fervens, Plagiolepis longwang, and Nylanderia sp.1) in forest areas was constant over time. For urban ants, a weak correlation was observed between foraging period and body size traits (i.e., Weber’s length and head width). This finding indicates that the major factor underlying the change in the foraging period might not be related to body size. Rather, the change may be attributed to synchronization between food availability and human activity (waste disposal; i.e., between 0900 and 1800). The shift in the functional traits of ants affects ecosystem functions in urban areas. In urban areas, although only one predatory ant species (P. megacephala) was sampled, its activity density was high. Most of these individuals were active during the daytime, indicating that the predatory behavior of ants in the novel urban environment has decreased temporally and is limited to the daytime. Urban ants tended to choose smaller food particles, whereas forest ants preferred larger food particles and had a twofold higher food removal rate.
Introduction
Natural forests and agricultural land are being converted into urban landscapes at an unprecedented rate worldwide (Gu et al., 2021; The World Bank, 2022). The resulting urban landscape is dominated by human-made structures, with only a few green spaces (Weeks, 2010). Unfortunately, habitat-specialist species are unlikely to thrive in such environments (Peng et al., 2020; Rocha and Fellowes, 2020). These widespread modifications to the landscape can result in changes to the assemblage and biotic homogenization as well as shifts in trait distribution from natural to highly urbanized habitats (Groffman et al., 2014). In general, urbanization tends to select smaller arthropods for survival, except for winged arthropods, which require dispersal across a fragmented habitat in urban areas (McIntyre, 2000; Kotze et al., 2011; Merckx et al., 2018a; Merckx et al., 2018b; Magura et al., 2020). However, for ants, not all species are equally affected by urbanization, as some forest ants with larger body-size are highly adaptable to the harsh urban environment (Peng et al., 2020; Rajesh et al., 2022).
Soil temperature in urban areas is typically around 5°C higher than that in forest areas. Coupled with low soil moisture and increased light disturbance, the urban environment can be harsh for arthropod survival (Van Nuland and Whitlow, 2014). The thermal tolerance of ants is often associated with Weber’s length (WL) or mesosomal length, with large-bodied ants being more tolerant to critical temperatures (Cerdá and Retana, 2000; Baudier et al., 2015; Baudier and O’Donnell, 2018). In addition, leg length is associated with ant locomotion, with longer legs allowing for faster movement (Weiser and Kaspari, 1999). Some studies have showed that large-bodied or long-legged ants can survive more favorably in a hot environment by lifting their bodies to protect themselves from heat radiated from the ground during foraging (Angilletta et al., 2007; Yilmaz et al., 2019). However, there is a mixed result as Liu et al. (2019) did not detect a significant positive association between Weber’s length of urban-dwelling ants and surface soil temperature in urban areas, suggesting the large-bodied ants are not necessarily prevalent in hot urban environments. Alternatively, some small-bodied urban arthropods can survive during land transformation by undergoing physiological and behavioral adaptation (Bolger et al., 2000; Egerer et al., 2017).
Variations in foraging pattern in hot urban environment are partly influenced by thermal tolerance of ant species. Studies showed that urban ants are more tolerant to elevated temperatures and took 20% longer time to lose mobility at high temperature compared to forest counterparts (Angilletta et al., 2007; Diamond et al., 2017). The increased heat tolerance is mainly driven by rapid evolutionary change in ants. For instance, the shift of thermal tolerance in urban acorn ants may evolve within 20 generations (Diamond et al., 2017).
In hot environments, some ants modify their foraging behaviors by restricting their foraging activity to specific times of the day, such as changing their foraging period from diurnal to nocturnal activity or altering their activity patterns from continuous to bimodal, to overcome the adverse effects of elevated temperature, (Heatwole and Harrington, 1989; Heatwole and Muir, 1989; Heatwole and Muir, 1991). However, other ants may be forced to forage close to their heat tolerance limits to avoid predation pressure (Wehner et al., 1992) or to dominate food sources (Cerda, 2001). Optimal foraging theory provides a conceptual framework to determine the cost of foraging in a high-risk environment. This theory states that natural selection favors foraging strategies that balance the benefits of obtaining food, including energy and nutrients, with the cost of acquiring it, including energy expenditure and mortality risk associated with foraging. This concept is pervasive among social insects as individual foragers may search for resources in a high-risk environment to benefit the entire colony (Nonacs and Dill, 1990). To date, studies that combine morphological, behavioral, and physiological traits to examine ant responses in urban context remain relatively scarce.
While ants are increasingly recognized for their ecological importance, many studies have focused on the impact of urbanization on fine-scale heterogeneity across an urban habitat mosaic and microhabitats (Pacheco and Vasconcelos, 2007; Clarke et al., 2008; Vepsäläinen et al., 2008; Ivanov and Keiper, 2010; Uno et al., 2010; Savage et al., 2015; Liu et al., 2019; Nooten et al., 2019). However, the previous studies have primarily emphasized species composition and richness, which may not be sufficient for investigating the impact of urbanization. This is because species loss during urbanization may be compensated by other species with similar functions. Ecosystem processes may appear to be insensitive to changes in biodiversity (Naeem and Wright, 2003). This functional redundancy resulting from local ant community homogenization and shifts in the morphological, physiological, or behavioral traits of urban ant communities can affect ecosystem functions in urbanized areas. Therefore, a better understanding of these relationships is paramount to predict changes in ecosystem functions in urban areas. Despite this, few studies have investigated the relationship between the functional traits of urban ant and their delivery of ecosystem services.
In this study, we examined the effect of urbanization on the functional diversity of ants in urban and forest areas. Additionally, we investigated the foraging pattern of ants over 72 h and examined the relationship between traits and environmental conditions in urban and forest sites. The extended observation period allowed us to gain insights into whether the ant community changes its foraging behavior over time. We hypothesized that the functional traits of ants foraging in urban sites would respond differently compared to those ants foraging in forest areas. We specifically focused on morphological, behavioral, and physiological traits and examined whether they would respond to elevated temperature, decreased humidity, and increased light intensity in urban areas. In this study, we also chose to study Pheidole megacephala, a species that exhibited different foraging periods between urban and forest sites, to determine whether it had different thermal tolerance between the two sites.
For ecosystem functions, this study examined putative predation activity of ants in relation to environmental variation in urban and forest study sites by examining their interocular distance (ID) and eye size; ID and eye size are surrogates for predacious ants (Silva and Brandão, 2014). Predacious ants commonly have smaller eyes or a shorter ID than do non-predacious ants (Weiser and Kaspari, 2006; Sosiak and Barden, 2021). We also examined whether urbanization affects the food particle size preference and biomass removal rate of ants over time, using six different sizes of food particles sampling. The concept of food particle size selection originally referred to the matching of forager and seed sizes in the forest ecosystem (Pfeiffer et al., 2006). We hypothesized that urbanization would alter the food particle size preference and biomass removal rate of ant assemblages over time.
Materials and methods
Study site
The study site was located in Taichung City, which experiences a subtropical climate, with a monthly average temperature of 17.0°C–28.9°C, a monthly average relative humidity of 75%, and an annual average precipitation of 1762.8 mm (data from the Central Weather Bureau Taiwan, collected from 1991 to 2020). This study was conducted in two land-use types—urban and forest areas—located along Taichung Basin up to Touke Hill in Taiping, Taichung City (24°08′N, 120°45′E–120°42′E).
A total of four sites in the urban area with different landscape characteristics were chosen: U4 (24°09′N, 120°42′E), U3 (24°08′N, 120°43′E), U2 (24°09′N, 120°44′E), and U1 (24°08′N, 120°44′E; Figures 1A, B). U4 and U3 sites are urban parks consisting of recreational structures, bushes, and trees that promote biodiversity in the urban ecosystem (Liu et al., 2019; Figure 1C). The U2 site is a lawn located next to a residential premise (Figure 1D). The U1 site is adjacent to a forest boundary that is composed of both natural vegetation and plantation (Figure 1E). Three sites were selected in the forest area: F3 (24°08′N, 120°45′E), F2 (24°08′N, 120°44′E), and F1 (24°08′N, 120°44′E; Figure 1F). Forest plots are characterized as secondary forests with understory covers, leaf litter, and various woody plant types that form stratified vegetation. These plots are marked as protected areas by the Forestry Bureau of Taiwan and have minimal development (paths for hiking). Each study site was set at least 300-m apart to ensure independence between sites. At each study site, plots where more ants were recovered in a previous study (Tsai, 2019) were selected for observing the 72-h activity pattern and performing experiments to determine the food particle size preference.
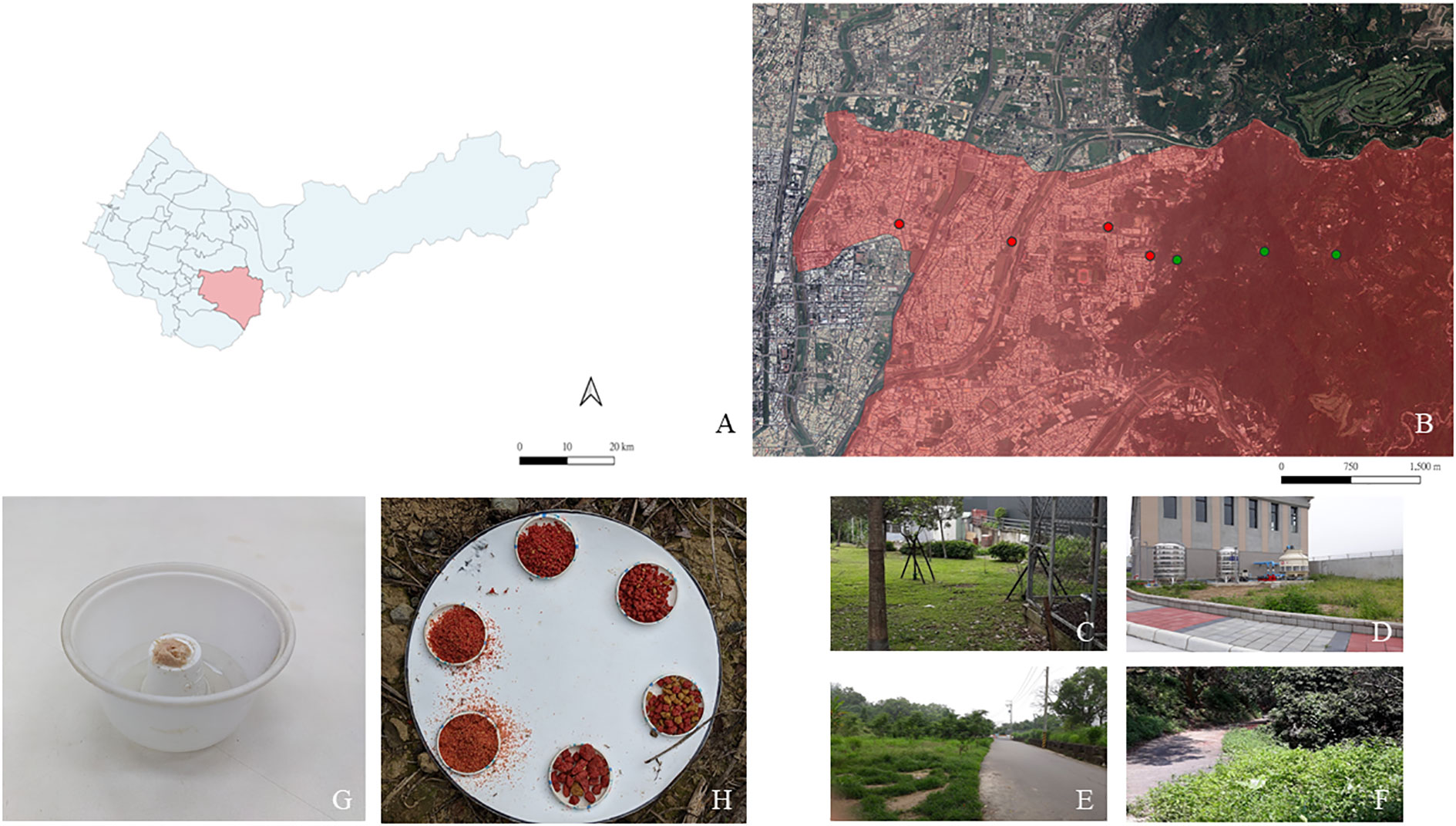
Figure 1 Map of (A) Taichung City. The study was conducted in Taiping District (B) where four sampling points were set in an urban area (red dots) and three sampling points were set in a forest area (green dots). Pitfall trap design of the foraging activity experiment. A large plastic bowl containing ethylene glycol and tuna-in-oil was placed on the top of a small plastic cup (C). Design of the bait station for performing the experiment to determine the particle size preference; six sizes of dog food particles were randomly placed on the plate (D). U4 and U3 are urban parks with recreational facilities (E). U2 is a lawn located next to a residential premise (F). U1 is located at a sharp forest boundary (G). F1–F3 are forest sites with minimal development (H).
Environmental variables
Environmental variables, namely soil moisture, soil surface temperature, and light intensity, were measured at each site within a 1-m radius of where the baited pitfall trap was placed. Soil surface temperature was measured using a pocket digital thermometer (Hi Sun Instrument Co., Ltd., Taoyuan, Taiwan). To measure soil moisture, the 5-m top soil was collected using a 100-cm3 soil core. The soil sample was weighed and then dried for 3 days to determine the water content. Light intensity was measured using a luxmeter (Lutron, LX-1102, Taiwan).
Activity pattern
The experiments for observing the 72-h activity pattern were conducted in urban and forest sites from March 26 to 28, 2019, and from May 11 to 13, 2019, respectively; a study had determined that ants were active in foraging during these periods (Lin et al., 2016). To examine the diurnal activity pattern of ants, we sampled ants by using a baited pitfall trap for three consecutive days. A baited pitfall trap consists of one fluon-coated round plastic bowl (15 cm in diameter and 7 cm in depth) and a plastic cup (4 cm in diameter and 4.2 cm in depth) placed upside down in the plastic bowl. A plastic plate served as shield to protect the bait from being disturbed. Plastic bowls were half-filled with ethylene glycol solution (ethylene glycol:water = 1:2). Tuna was placed on the top of the plastic cup as a lure to attract ground-dwelling ants (Figure 1G). Two baited pitfall traps were used at each study site to collect the maximum number of ants. The experiment was started at midnight (00:00), and ants were collected at 3-h intervals. The ant specimens were preserved in 75% alcohol until they were identified. The ant specimens were identified to the species level on the basis of identification keys for ant fauna in Taiwan (Lin, 1998).
Morphological traits
For each study site, 10 individuals of each species were identified using a Leica S8APO stereo microscope (Leica Microsystems GmbH, Wetzlar, Germany). In dimorphic or polymorphic species, only minor workers were used because major workers are generally depauperate in a colony. A total of 25 morphological traits were measured (Supplementary Table 1).
Thermal tolerance
To investigate the difference in the thermal tolerance of foragers between the urban and forest sites, we determined the critical thermal maximum (CTmax) of the dominant species P. megacephala, which accounted for 22.49% and 18.19% of total ant activity density in the urban and forest sites, respectively. A colony with 31 minor workers from the urban sites and three colonies with 24 minor workers from the forest sites were examined. To maintain test ants in natural physiological conditions, the ants were acclimated under ambient conditions (25°C and 80% relative humidity) in an incubator (CL-180H, Long Sheng Co., Ltd., Taiwan) for 2 h prior to testing. The ants were provided ad libitum access to water resources and harborage. The CTmax was determined using the protocol employed in previous studies (Kaspari et al., 2015; Bujan and Kaspari, 2017). Briefly, the test ants were individually placed in a 1.5-mL vial, and the entrance of the vial was blocked with cotton to prevent the ants from escaping. The vials were then transferred to a water bath machine (Firstek model B401L, Firstek Scientific Co., Ltd., Taiwan) and kept at 25°C for 2 min for acclimatization. After acclimatization, the temperature was gradually increased by 1°C every 2 min until it reached 35°C. Subsequently, the ramping rate was changed to increase by 1°C every 5 min. The condition of the test ants was evaluated before changing temperature. The ants were recorded as immobilized when they could not move themselves to the right position when flipped.
Particle size preference
We examined the particle size preference of the ants by following the method reported by Hooper-Bùi et al. (2002) with slight modifications. Dog food (RT-Mart Chicken Flavor Dog Food, Hsinchu, Taiwan), which served as lure, was ground and then run through different sieves (standard sieve size nos. 5, 7, 12, 20, 30, and 40), resulting in six sizes: <5 mesh (>4.00 mm), 5–7 mesh (4.00–2.83 mm), 7–12 mesh (2.83–1.68 mm), 12–20 mesh (1.68–0.84 mm), 20–30 mesh (0.84–0.59 mm), and >30 mesh (<0.42 mm). The ground food particles were placed into the bait station (a paper plate with an 18-cm diameter) containing six small pans (4 cm in diameter) filled with the different particle sizes of dog food (i.e., approximately 2 g of food particles per pan; Figure 1H). Each bait station was covered by a plastic plate to prevent disturbance (e.g., rainfall, wind blowing, and animals capable of flipping the bait station) from the environment. The experiment was conducted from 0900 to 1700 at the urban (i.e., U1–U4) and forest sites (i.e., F1–F3), which was the peak of foraging time for most ant species, as indicated by the result of the aforementioned experiment. The experiment was conducted bimonthly for 12 months from December 2019 to December 2020. Baits were examined for ant activity at 30-min intervals. New food was replaced once it was completely removed. The food particles were dried, and the weight of the remaining food particles was measured at the end of the experiment. Moreover, the presence of ants and ant bodies collected from baits was examined.
Data analysis
For each landscape type, the activity density of ants (the rate that an individual taxon moves through the environment) and ant species recovered from baited traps (urban: eight traps; forest: six traps) at a specific time was pooled. For the morphological traits, principal component analysis (PCA) was performed to determine trait distribution in both the urban and forest sites. In addition, PCA was conducted to reduce the dimensionality of morphological datasets, where only the most explainable trait according to eigenvalue was used for further analysis. Each selected functional trait from the urban and forest sites was weighted by species activity density to eliminate bias from biomass and was then pooled in accordance with the landscape type prior to analysis. Welch’s t test was performed to compare each functional trait between the urban and forest sites.
Functional diversity indices represent the dynamics of species function in ecosystems (Petchey and Gaston, 2006). We selected five functional traits that reflect the ecological functions of ants: WL, head width (HW), ID, mid-tibia length (MTL), and mandible length (ML). All the traits, except for WL, were divided by WL to standardize trait values (trait values/WL) to generate functional diversity indices. To compare functional diversity between the urban and forest sites, we examined the three aspects of trait distribution, namely functional richness (FR), functional evenness (FE), and functional divergence (FD), on the basis of the Euclidean distance of each trait in hyperspace by using the package FD (Laliberté and Legendre, 2010) in R. FR indices measure how much of the niche space is occupied by the species present; FE indices measure whether mean species traits are distributed regularly within the occupied trait space, while FD indices measure the variance of the species’ functions and the position of their clusters in trait space (Schleuter et al., 2010). These indices were used to determine the relationship between the activity density and functional traits of species that were linked to their ecosystem functions (Villéger et al., 2008; Cadotte et al., 2011).
Morphological traits predictably respond to the foraging period of ground-dwelling ants under the effect of urbanization (Weiser and Kaspari, 1999; Kaspari and Weiser, 2007). Data on morphological traits, environmental variables, and ant activity density were subjected to fourth-corner analysis to examine the response of traits to environmental variations (Dray and Legendre, 2008). The fourth-corner analysis performed in this study was based on the method used in a previous study (Brown et al., 2014). A multivariate generalized linear model was employed using the package “mvabund” (Wang et al., 2021) in R, with the environmental variables, species activity density, and their interaction terms used as explanatory variables; functional traits were used as response variables in the model.
Changes in food particle size preference in different seasons were examined using two-way analysis of variance (ANOVA); months and food particle sizes were both considered as explanatory variables, and the amount of food removed was used as a response variable. The amount of dog food removed over 12 months was arranged in a chronological order and pooled to determine the overall preference. In terms of the functional diversity indices, data from three sites in the forest area and four sites in the urban area were pooled to evaluate specific ecosystem functions in both the land-use types. All figures were illustrated using the package “ggplot2” in R (Wickham, 2016). All analyses were conducted using R project (R Core Team, 2020).
Results
Local environment
Temperature was higher in the urban sites (daytime temperature: 31.2°C and nighttime temperature: 27.7°C) than in the forest sites (daytime temperature: 26.6°C and nighttime temperature: 22.7°C). Moreover, temperature in the urban and forest sites peaked at approximately 0900 and 1200, respectively. The soil water content was higher in the forest sites than in the urban sites and declined over time in both the sites; it peaked from 0000 to 0300 and was constantly low from 0600 to 2100. The light intensity pattern during daytime was similar between the urban and forest sites. However, at night, light intensity was slightly higher in the urban sites than in the forest sites (Supplementary Figure 1).
Activity pattern of ants
A total of 6652 ants and 24 species were collected, of which 5643 ants and 21 species were collected from the forest sites and 1009 ants and 7 species were collected from the urban sites (Figure 2). The proportions of the M. chinense and P. megacephala were the highest in the urban sites, accounting for 69.0% and 22.4% of the collected ants, respectively. The foraging activity of the ants in the urban sites peaked from 0900 to 1500, accounting for 87.9% of overall activity density. Other three ant species, namely Cardiocondyla sp.1, Paratrechina longicornis, and Solenopsis sp.1, were also observed during this period. In the forest sites, the proportions of the genera Carebara and Pheidole were the highest in the forest sites, accounting for 72.9% and 18.1% of the collected ants, respectively. The activities of Carebara diversa, P. megacephala, Pheidole fervens, Plagiolepis longwang, and Nylanderia sp.1 were observed throughout the experimental period; other ant species exclusively foraged during the daytime (Figure 2).
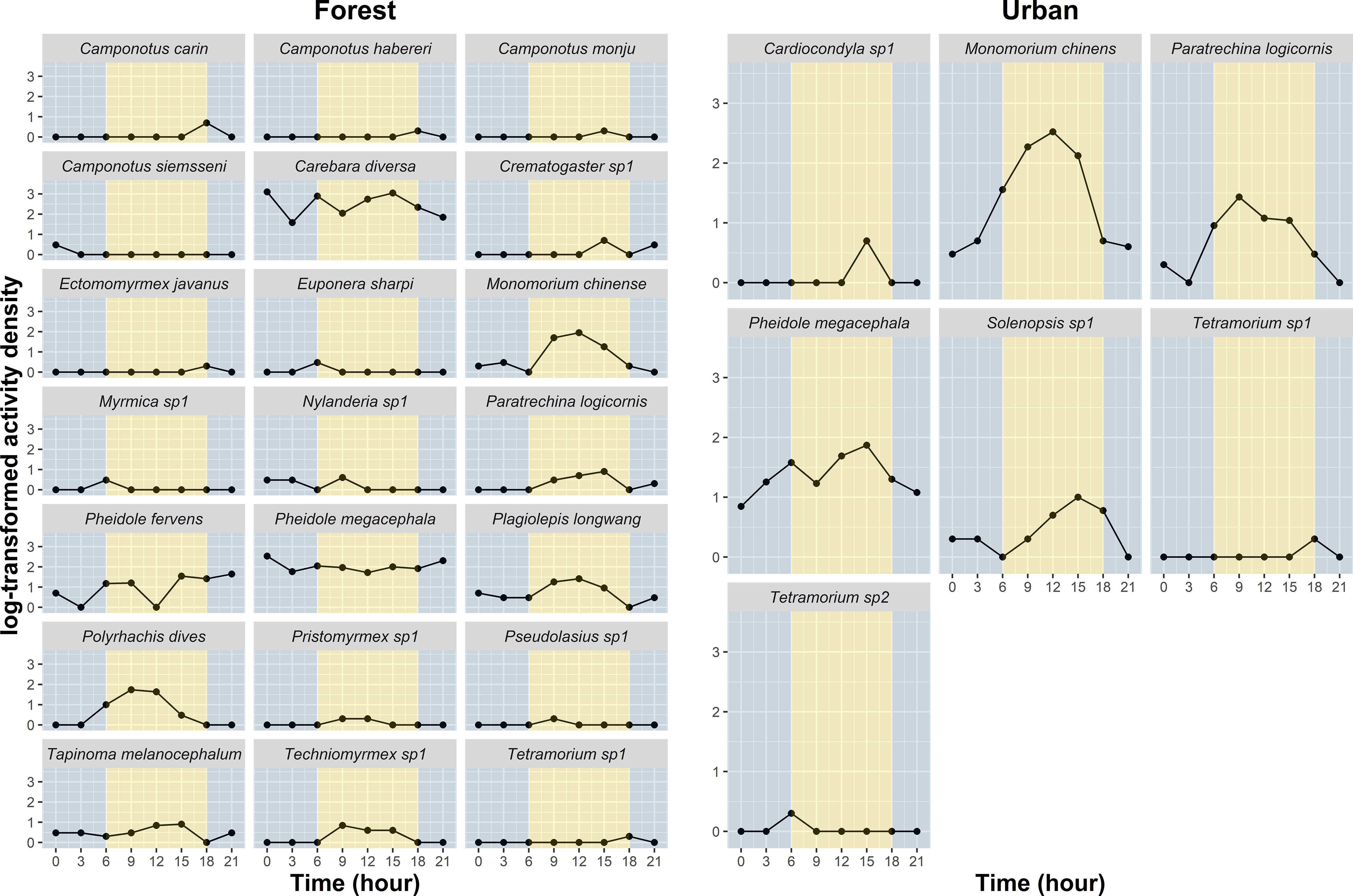
Figure 2 Foraging period of ants in the forest and urban sites. The foraging periods of Cardiocondyla sp.1, Monomorium chinense, Paratrechina longicornis, Pheidole megacephala, and Solenopsis sp.1 were observed during the daytime in urban sites. By contrast, in the forest sites, the activities of Carebara diversa, Pheidole megacephala, Pheidole fervens, Plagiolepis longwang, and Nylanderia sp.1 were observed throughout the experimental period; other ant species exclusively foraged during the daytime. Grey background indicates nighttime; Yellow background indicates daytime.
Functional diversity
According to the findings of PCA, WL, MTL, ML, ID, HW, and eye height (EH) were selected because of their relatively high scores in the first PC (Principal component; PC1), which explained 66.3% of total variance (WL = −0.301, MTL = −0.283, ML = −0.282, ID = −0.246, HW = −0.274, and EH = −0.287; Table 1). In particular, PC1 partially explained the higher values of the selected traits of some ants, particularly those present in the forest sites (Figure 3). PC1 was largely correlated to the size of species, and the major component was mainly described by MTL, WL, EH, and HL, indicating that the ants in the forest sites tended to be larger in terms of their overall size. PC2 was highly related to survival strategy traits, such as the presence of a spiny body or long legs. The functional traits of the ants in the urban sites were a subset of those in the forest sites. Compared with the ants in the forest sites, those in the urban sites exhibited narrower trait distribution on the basis of their 95% confidence intervals, indicating the homogenization of traits in the urban sites (Figure 3). Although no significant difference was noted in each trait between the sites (WL: t = −1.4699, df = 17.946, p = 0.1589; MTL: t = −1.3124, df = 16.928, p = 0.2068; ML: t = −1.175, df = 16.781, p = 0.2564; ID: t = −1.073, df = 18.289, p = 0.2972; HW: t = −1.3307, df = 18.672, p = 0.1993; EH: t = −1.0826, df = 16.959, p = 0.2941; Figure 4A), the ants in the forest sites had larger functional traits; no difference in shrinkage was observed between the urban and forest sites.
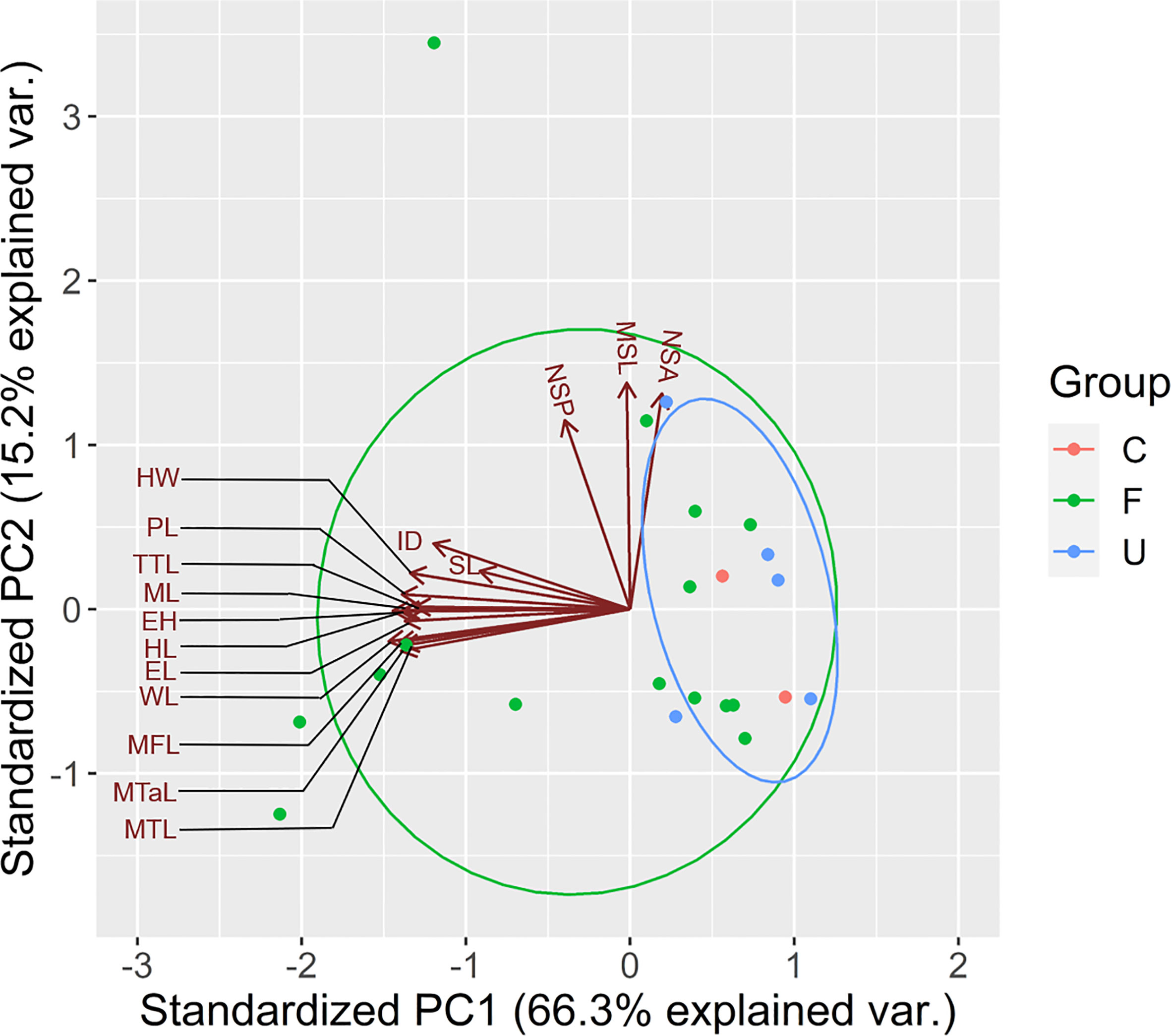
Figure 3 Trait distribution in urban and forest areas. U represents the urban area. F represents the forest area. C represents common species appearing in both areas. The best explanatory variables selected are listed in Table 1. Representations of trait abbreviations are listed in Supplementary Data Table 1.
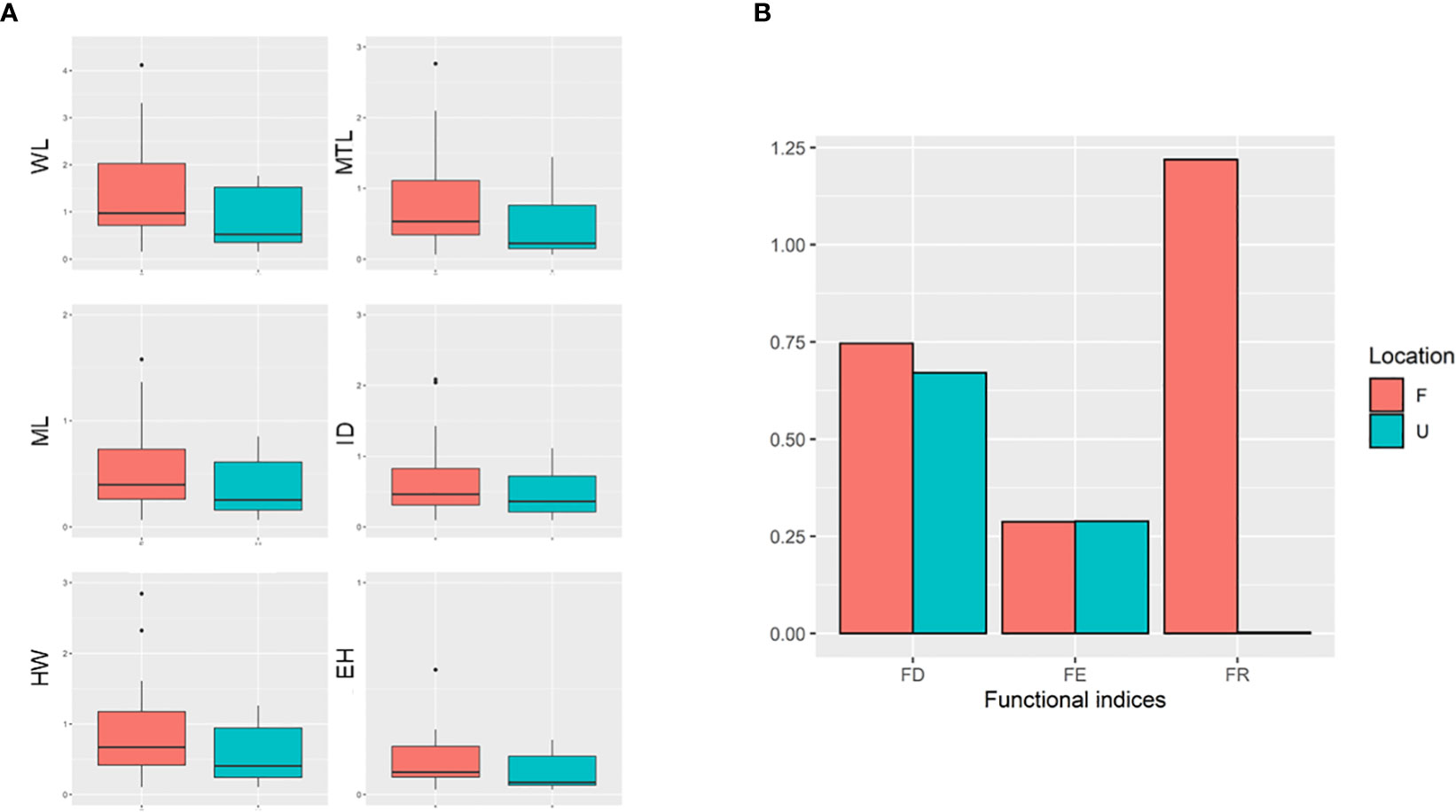
Figure 4 (A) Distribution of abundance-weighted selected functional traits. (B) Three functional indices among two areas (FD, functional divergence; FE, functional evenness; FR, functional richness). U represents the urban area. F represents the forest area.
In terms of the functional diversity indices, FE and FD did not differ between the urban and forest sites (urban: FE = 0.288, FD = 0.670; forest: FE = 0.287, FD = 0.746). However, a substantial difference in FR was noted between the urban and forest sites (urban: FR = 0.002; forest: FR = 1.218; Figure 4B).
Foraging and trait–environment relationship
The explanatory power of the fourth-corner analysis in both the forest and urban sites was 0.83 and 0.94, respectively (Table 2). Two models for the different landscapes both significantly rejected the null hypothesis (F value for the forest sites = 11.19 and F value for the urban sites = 12.73), indicating that the two models had good fit instead of being intercept-only models. The result of the fourth-corner analysis revealed that ID was positively correlated with temperature and humidity (Figure 5; Table 2). However, ID was positively associated with light intensity in the urban sites but not in the forest sites. In the urban sites, a weak association was observed between the environmental variables and size-related (HW and WL) and foraging speed traits (MTL). By contrast, ant species with a narrower HW and shorter MTL tended to forage in the forest sites during the hot daytime. In the urban sites, ants with a shorter mandible were observed when temperature increased irrespective of the phase of the day and the level of humidity. In the forest sites, ML was not associated with temperature but was inversely associated with light intensity. EH exhibited a negative relationship with light intensity in both the forest and urban sites (Figure 5; Table 2).
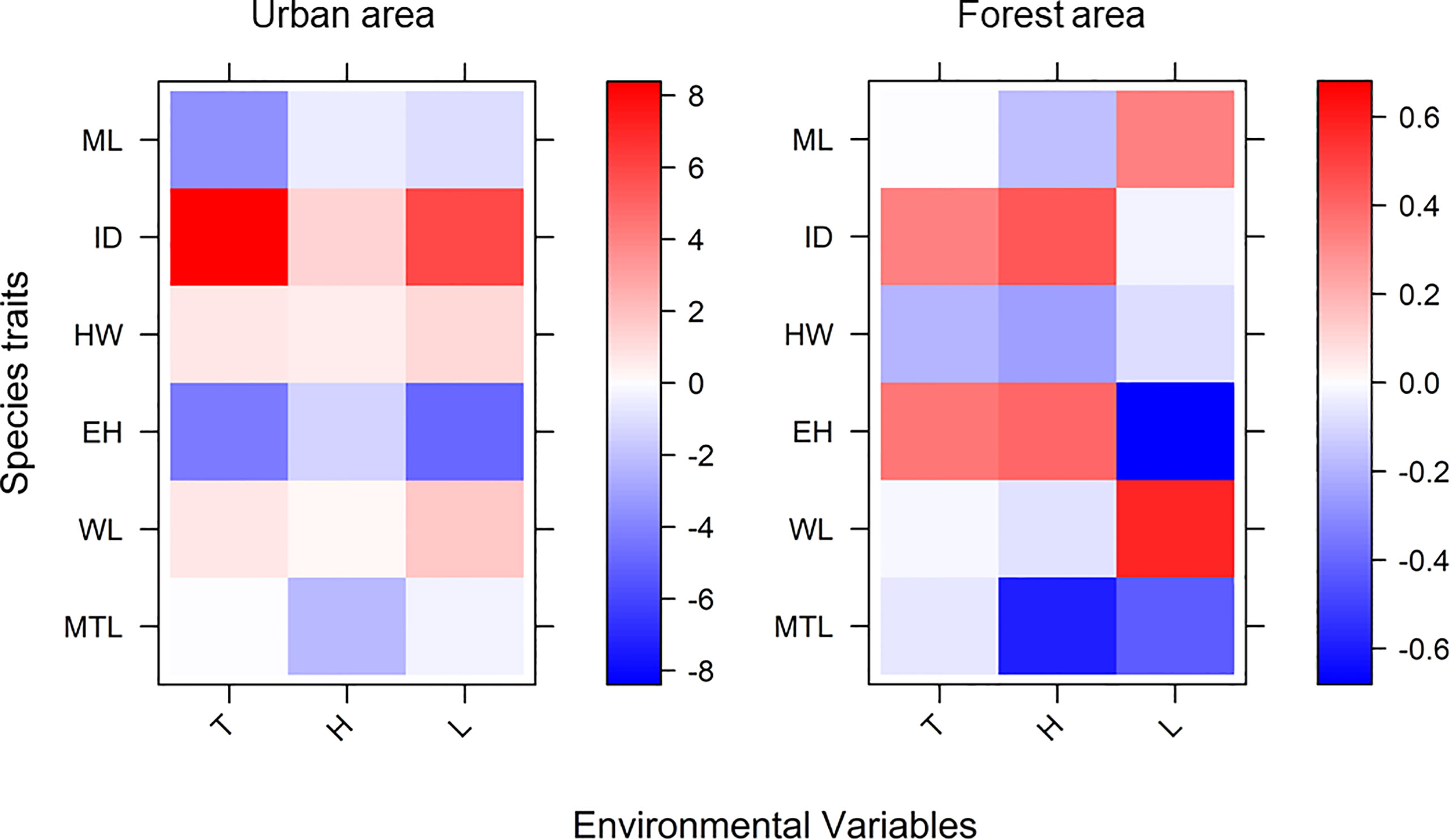
Figure 5 Result of fourth-corner analysis. Coefficients of results were transformed into a heatmap to visualize the positive or negative association between environmental factors and functional traits (T, temperature; H, humidity; and L, light intensity). The explanatory power of fourth-corner analysis in both the forest and urban sites was 0.83 and 0.94, respectively.
Thermal tolerance
The urban ants demonstrated a weaker response to elevated temperature, and they reached total heat stupor at 42°C. By contrast, the forest ants displayed better heat tolerance, and they reached total heat stupor at 44°C (Figure 6). The immobility of the urban ants markedly increased after 39°C, whereas that of the forest ants exhibited a stable trend with increasing temperature.
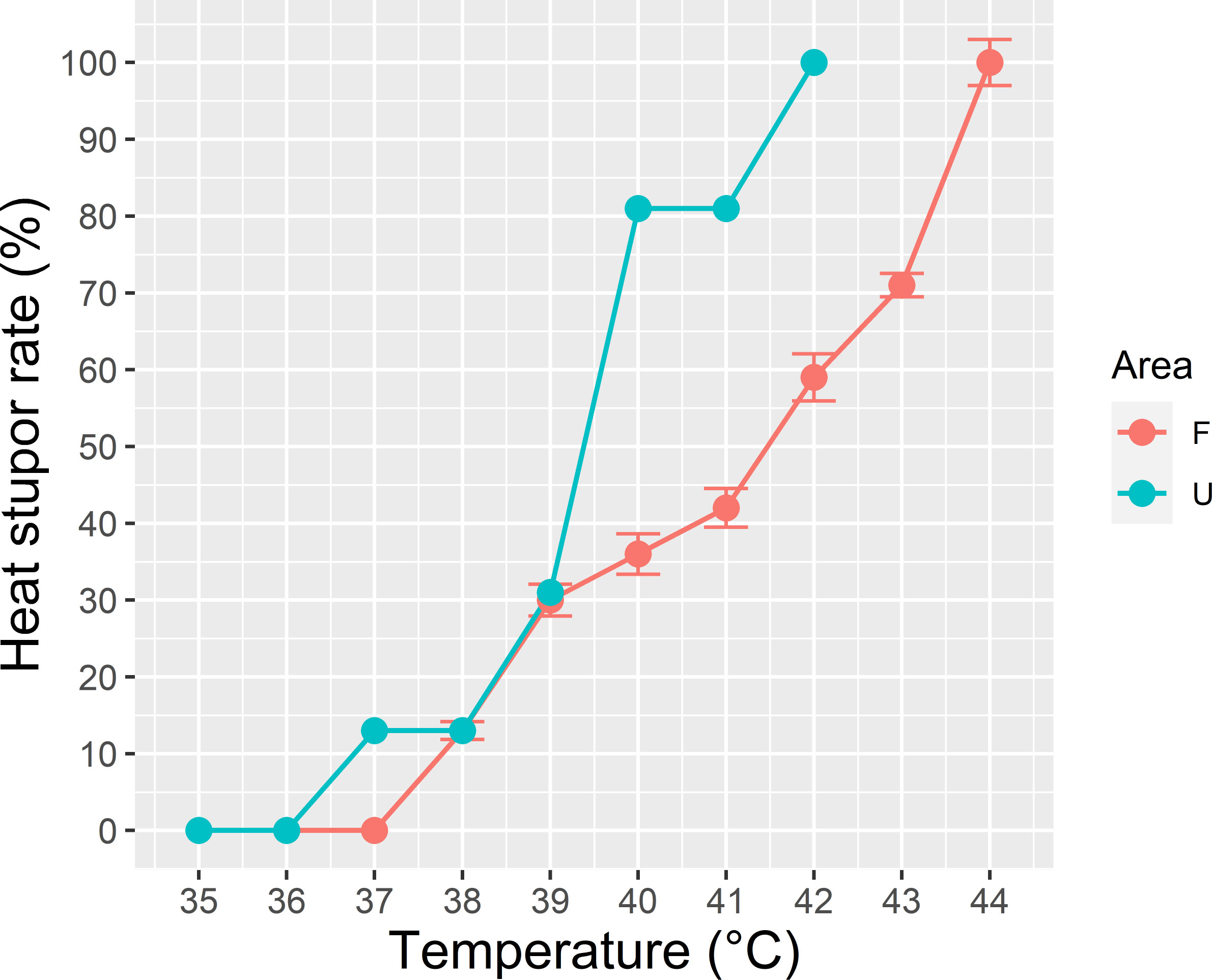
Figure 6 Critical thermal maximum (CTmax) of the dominant species Pheidole megacephala in both forest (F) and urban sites (U). Urban ants demonstrated a weaker response to elevated heat and reached the total heat stupor at 42°C, whereas forest ants displayed better heat tolerance and reached total heat stupor at 44°C.
Particle size preference
The findings of two-way ANOVA revealed that the seasonal effect of particle size preference was not significant in both the urban and forest sites (urban: F = 0.579, p = 0.9583; forest: F = 0.502, p = 0.9820; Figure 7). Overall, the amount of bait that the ants removed in the forest sites was approximately two times higher (removal weight = 80.57 g) than that removed by the ants in the urban sites (removal weight = 41.31 g). The ant species mainly involved in bait removal were C. diversa, M. chinense, P. megacephala, and P. fervens in the forest sites and Pheidole parva, M. chinense, and P. megacephala in the urban sites. A shift in food particle size preference was observed in the forest and urban sites. The ants in the urban sites tended to consume finer particles, ranging from 0.24 to 0.84 mm (t = 3.516; df = 84; p = 0.0001), whereas those in the forest sites tended to consume larger food particles, ranging from 0.84 to 1.68 mm (t = 2.5468; df = 160; p = 0.0118) (Figure 8).
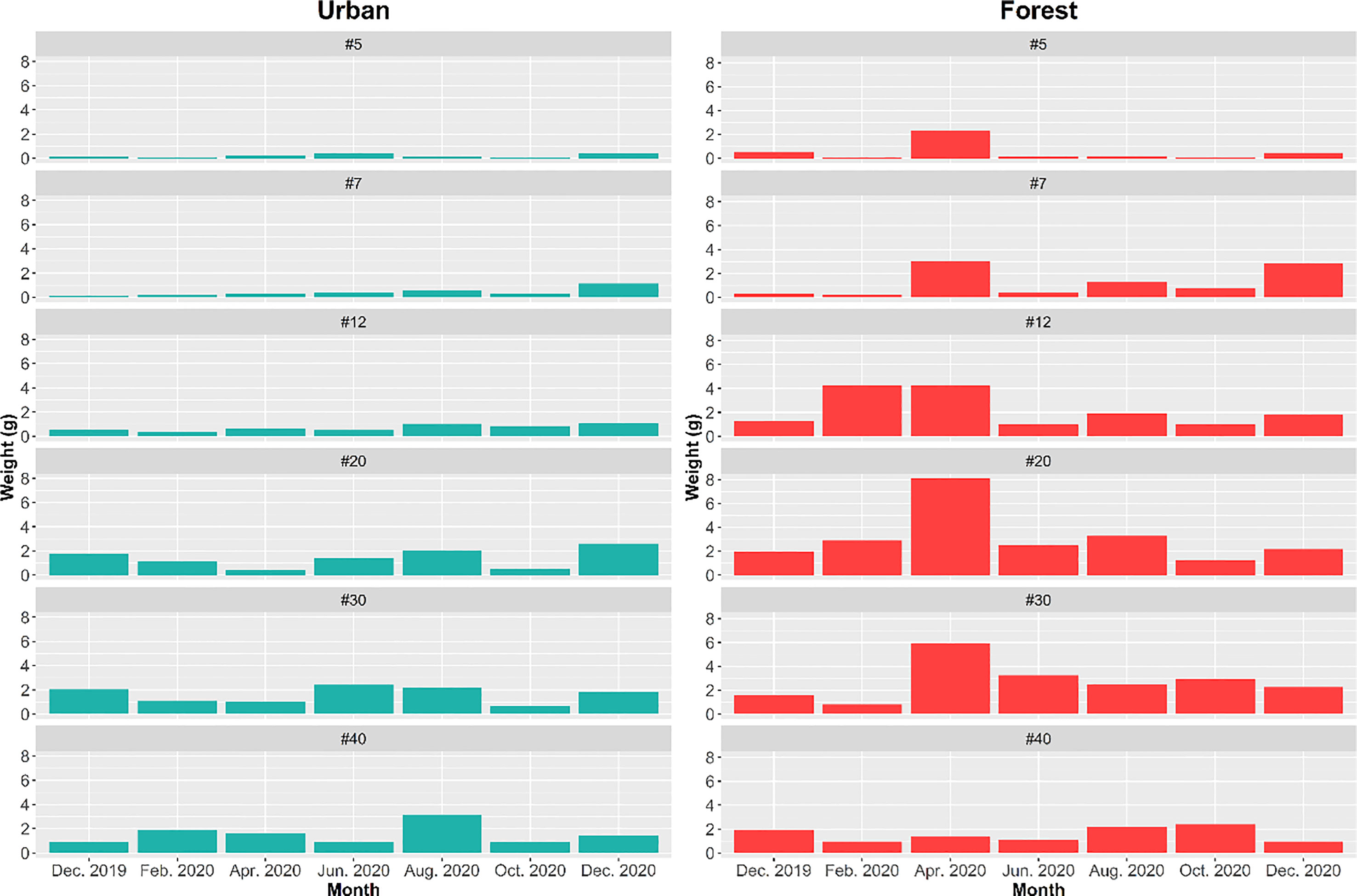
Figure 7 Weight of different sizes of food particles removed in forest and urban sites at each sampling period.
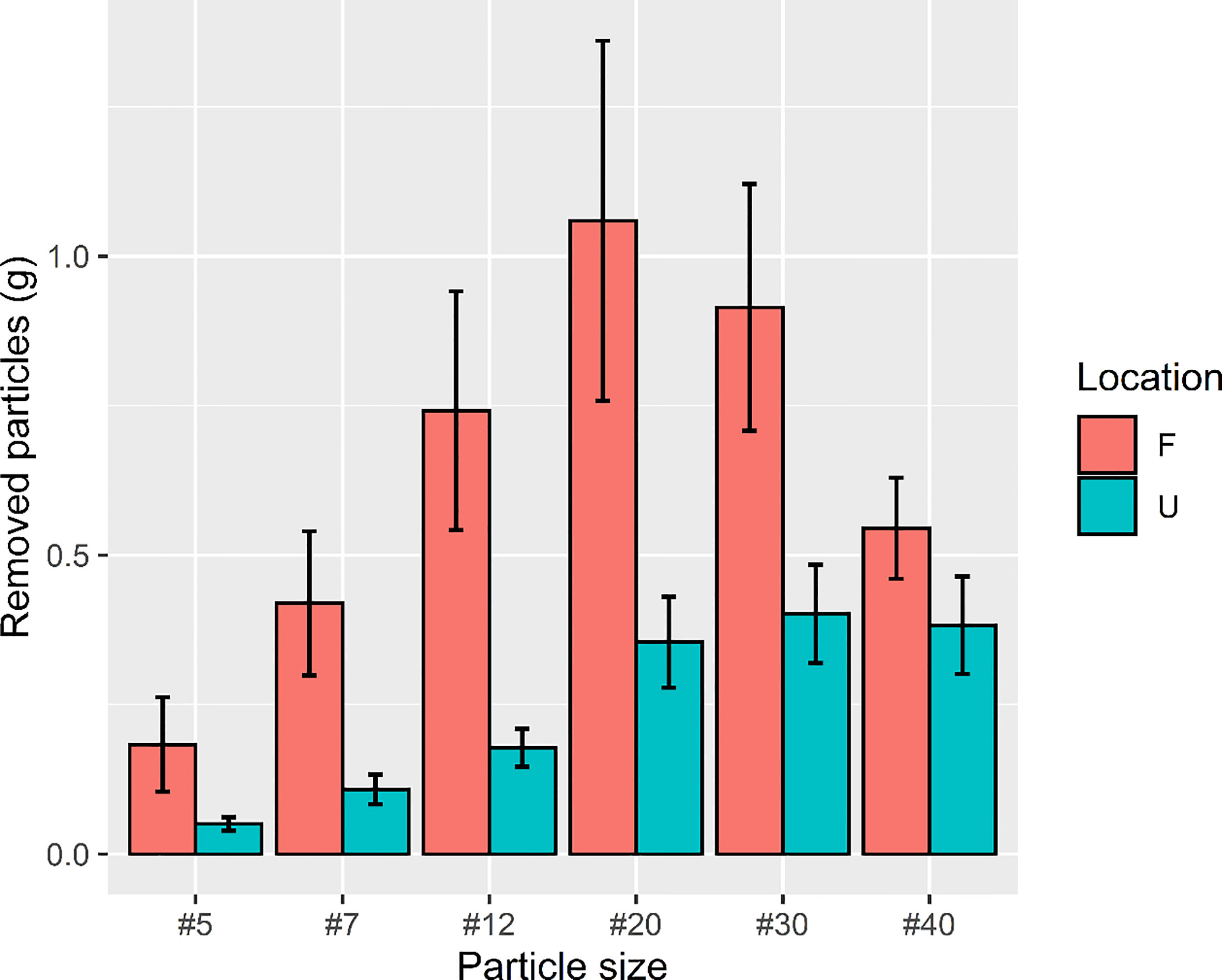
Figure 8 Total weight of food particles removed in urban and forest sites from December 2019 to December 2020. The amount of bait that ants removed in the forest site was approximately two times higher (removal weight = 80.57 g) than that in the urban site (removal weight = 41.31 g).
Discussion
The findings of the present study demonstrated a decline in the mean trait value of most urban ants. This finding suggests the presence of trait homogenization in urban ants. In addition, we noted a more significant change in the functional traits of ants affects ecosystem functions in the urban sites compared to the forest sites.
The decline in the mean size of the urban ants as observed in our study supports the finding reported by Nooten et al. (2019), who conducted an ant survey in green spaces in urban areas by selecting three habitat types representing low, medium, and high complexity. The authors observed positive associations between WL and tree density and between the cephalic index and tree biomass, indicating larger ants in high-complexity woody habitats and smaller ants in low-complexity open fields (Nooten et al., 2019). Similar decrease in mean size was also observed for other taxa, such as spiders, coleopterans, and aquatic arthropods (Merckx et al., 2018b). However, this trend was not observed in bumblebee and moth (Merckx et al., 2018a; Theodorou et al., 2021). We suggested that limited availability of resources, including food and nesting sites, in the urban environment may be responsible for the smaller and more homogenous size of urban ants. For instance, urban ants essentially rely on limited nesting resources, such as cracks and crevices (Friedrich and Philpott, 2009). Our findings regarding the ants in the forest sites agree with the hypothesized ecological function of ants: larger ant species forage during the daytime, whereas smaller ant species forage during the nighttime when the environment is cooler (Yilmaz et al., 2019; Johnson and Stahlschmidt, 2020) as similarly shown in dung beetles (Asha et al., 2021). However, it is important to note that in this study, we only sampled from four urban sites and three forest sites, which may have resulted in some large-bodied forest ants being under sampled.
The current study demonstrated that none of the examined morphological traits adequately explained the difference in the foraging period between the sites. However, other underlying strategies, such as shifting foraging time and physiological plasticity, might play role in determining when ants forage in the urban environment. For instance, urban ants might avoid food searching during the hot daytime hours (Marsh, 1985; Stringer et al., 2007). This is because foraging under elevated temperatures typically increases the energy expenditure of forager ants (Cerdá et al., 2009; Gottlieb et al., 2013). In contrast to our expectations, our findings demonstrated that the foraging period of the urban ant community peaked from 0900 to 1500, suggesting that they were active during the hot daytime, which contrasts with the activity pattern of the forest ant community. However, this finding should be interpreted with caution since the use of tuna-in-oil baits might have led to a sampling bias, with the ants preferring protein- and oil-based food.
Studies have demonstrated that many ant species that have adapted to the urban environment developed better tolerance to respond to more harsh conditions (Angilletta et al., 2007; Diamond et al., 2017; Diamond et al., 2018). However, the present study examined one of the dominant species, P. megacephala, from both the sites and observed that the CTmax of the urban ants was lower than that of the forest ants. This finding rules out the possible involvement of physiological advantages that drive the decision of the urban ant to forage during the hot daytime. The temperature at the urban sites is comparable to the critical thermal tolerance of urban ant species. Thus, it is possible that forager ants might forage under their CTmax, as has been observed in a local ant community in the Mediterranean area (Cros et al., 1997; Cerdá et al., 1998), but caution is warranted as only one colony from urban area was examined.
According to Penick et al. (2015), urban ants tend to feed on nitrogen-rich food, implying their reliance on human-made food in urban areas. Additionally, Liu et al. (2019) conducted ant surveillance in urban parks and reported that the number of urban ants was positively associated with the number of garbage bins in parks. Forager ants tend to forage during the daytime possibly because of increased human activity in urban areas from 1100 to 1800, and human food waste results in the availability of increased food resources. Forager ants forage under harsh conditions, suggesting that forager ants prefer to forage when the environment is rich in food resources during the daytime to acquire nutrients and ensure colony survival in a novel urban environment. However, such a foraging decision can be detrimental to the foragers themselves (Ydenberg and Schmid-Hempel, 1994) because they might experience a high risk of desiccation owing to high average temperature, low humidity, and extreme thermal fluctuation in the urban environment (Uno et al., 2010). This finding accords with the concept of optimal foraging theory.
The effect of the urban heat island disproportionately affects forest species and leads to the homogenization of the functional trait community that is dominated by habitat generalists. Loss of functionally diverse ants is related to the increased vulnerability of ecosystem function (Bihn et al., 2010). In the present study, we used the conventional functional trait-based approach and considered ID as an indicator of the predatory nature of ants. We chose ID instead of eye size because our model showed a significant negative correlation between eye size and light intensity. Our results revealed that compared with the ants found in the urban and forest sites during the daytime, those found during the nighttime had larger eyes that helped them receive more light. Thus, to prevent sampling bias related to the light receptivity of ants at night, we selected ID as a more relevant surrogate of predatory behavior.
Urban areas, contrary to expectations, still harbor predatory ants, particularly P. megacephala, which were mostly active during the daytime. This result differs from that obtained in the forest sites, where predatory ants were active during both day and night (e.g., C. diversa, P. megacephala, and P. fervens), as indicated by the nonsignificant relationship between ID and light intensity. This suggests that the predatory activity of ants in the urban environment may have been reduced and limited to a certain period of time. A key difference between previous studies and our study is that previous studies have estimated potential ecosystem function by examining the occurrence of a species in a given location (Brown et al., 2014; Treiber et al., 2020). Such methods may mask the effects of a shift in behavioral traits caused by urbanization (i.e., foraging period and foraging behavior) on the degree of predatory activity. By contrast, the current observations of the foraging period were obtained during both day and night for 72 h, providing a more comprehensive assessment of predatory activity of both diurnal and nocturnal ants.
Previous research demonstrated variations in food particle size removal across season in in granivorous ants (Silvestre et al., 2019). However, our results revealed no significant variations in the efficiency of ants in removing biomass across seasons in either urban or forest sites. Although no seasonal variations in food particle size preference were noted, we did observe that urban ants removed small- (<0.42 mm) to medium-sized (0.84–1.68 mm) food particles, which is consistent with their smaller body size and shorter mandible. By contrast, forest ants with increased functional diversity removed medium-sized (0.84–1.68 mm) to large-sized (1.68–2.83 mm) food particles. These results agree with the finding of a previous study that demonstrated that the decomposition or removal ability of nitrogen resources is mediated by body size, with larger ants being better biomass decomposers than smaller ants (Nooten et al., 2022). Although some smaller urban ants engaged in cooperative food transport, mass recruitment, and in situ food fragment mastication, their food removal efficiency was low than that of larger forest ants.
In conclusion, the present study provides important insights into how ants contribute to ecosystem functioning in a novel urban environment where there are shifts in their foraging behavior. Our results yielded three key points. First, larger ant species were filtered out from urban ecosystem, resulting in a significant homogenization of body size, as indicated by the lower FR of the urban forager ants compared to those in forest ecosystems. Second, we observed alterations in the ant foraging period during the hot daytime hours in urban areas. None of the morphological and physiological traits we measured could explain the changes in foraging behavior, there may be unexplored optimal foraging theory factors that coincide with human waste disposal activities. Third, we found that changes in the functional traits of forager ants had pronounced effects on ecosystem function, especially on predatory activity and food particle removal rate. The predatory activity of ants significantly decreased and was restricted to the hot daytime hours in urban areas. The smaller forager ants in the urban areas moved small-sized particles at a lower rate than did the larger forest ants.
Data availability statement
The original contributions presented in the study are included in the article/Supplementary Material. Further inquiries can be directed to the corresponding author.
Author contributions
Y-HC and K-BN contributed to the conception and design of the study. Y-HC conducted the experiment and performed the statistical analysis. Y-HC and K-BN wrote the first draft of the manuscript. Both authors contributed to the article and approved the submitted version.
Funding
This study was funded by the Ministry of Science and Technology, Taiwan (MOST 110-2313-B-005-025- and MOST 111-2313-B-005-022-).
Acknowledgments
We thank Ming-Hsiao Peng (NCHU) for field assistance.
Conflict of interest
The authors declare that the research was conducted in the absence of any commercial or financial relationships that could be construed as a potential conflict of interest.
Publisher’s note
All claims expressed in this article are solely those of the authors and do not necessarily represent those of their affiliated organizations, or those of the publisher, the editors and the reviewers. Any product that may be evaluated in this article, or claim that may be made by its manufacturer, is not guaranteed or endorsed by the publisher.
Supplementary material
The Supplementary Material for this article can be found online at: https://www.frontiersin.org/articles/10.3389/fevo.2023.1044485/full#supplementary-material
References
Angilletta M. J. Jr., Wilson R. S., Niehaus A. C., Sears M. W., Navas C. A., Ribeiro P. L. (2007). Urban physiology: city ants possess high heat tolerance. PloS One 2 (2), e258. doi: 10.1371/journal.pone.0000258
Asha G., Manoj K., Megha P. P., Sinu P. A. (2021). Spatiotemporal effects on dung beetle activities in island forests-home garden matrix in a tropical village landscape. Sci. Rep. 11, 17398. doi: 10.1038/s41598-021-96831-5
Baudier K. M., Mudd A. E., Erickson S. C., O’Donnell S. (2015). Microhabitat and body size effects on heat tolerance: implications for responses to climate change (army ants: Formicidae, Ecitoninae). J. Anim. Ecol. 84 (5), 1322–1330. doi: 10.1111/1365-2656.12388
Baudier K., O’Donnell S. (2018). Complex body size differences in thermal tolerance among army ant workers (Eciton burchellii parvispinum). J. Therm. Biol. 78, 277–280. doi: 10.1016/j.jtherbio.2018.10.011
Bihn J. H., Gebauer G., Brandl R. (2010). Loss of functional diversity of ant assemblages in secondary tropical forests. Ecology 91 (3), 782–792. doi: 10.1890/08-1276.1
Bolger D. T., Suarez A. V., Crooks K. R., Morrison S. A., Case T. J. (2000). Arthropods in urban habitat fragments in southern California: area, age and edge effects. Ecol. Appl. 10 (4), 1230–1248. doi: 10.1890/1051-0761(2000)010[1230:AIUHFI]2.0.CO;2
Brown A. M., Warton D. I., Andrew N. R., Binns M., Cassis G., Gibb H., et al. (2014). The fourth-corner solution - using predictive models to understand how species traits interact with the environment. Methods Ecol. Evol. 5 (4), 344–352. doi: 10.1111/2041-210x.12163
Bujan J., Kaspari M. (2017). Nutrition modifies critical thermal maximum of a dominant canopy ant. J. Insect Physiol. 102, 1–6. doi: 10.1016/j.jinsphys.2017.08.007
Cadotte M. W., Carscadden K., Mirotchnick N. (2011). Beyond species: functional diversity and the maintenance of ecological processes and services. J. Appl. Ecol. 48 (5), 1079–1087. doi: 10.1111/j.1365-2664.2011.02048.x
Cerda X. (2001). Behavioral and physiological traits to thermal stress tolerance in two Spanish desert ants. Etología 9, 15–27.
Cerdá X., Angulo E., Boulay R., Lenoir A. (2009). Individual and collective foraging decisions: a field study of worker recruitment in the gypsy ant aphaenogaster senilis. Behav. Ecol. Sociobiol. 63 (4), 551–562. doi: 10.1007/s00265-008-0690-5
Cerdá X., Retana J. (2000). Alternative strategies by thermophilic ants to cope with extreme heat: individual versus colony level traits. Oikos 89 (1), 155–163. doi: 10.1034/j.1600-0706.2000.890117.x
Cerdá X., Retana J., Cros S. (1998). Critical thermal limits in Mediterranean ant species: trade-off between mortality risk and foraging performance. Funct. Ecol. 12 (1), 45–55. doi: 10.1046/j.1365-2435.1998.00160.x
Clarke K. M., Fisher B. L., LeBuhn G. (2008). The influence of urban park characteristics on ant (Hymenoptera, formicidae) communities. Urban Ecosyst. 11 (3), 317–334. doi: 10.1007/s11252-008-0065-8
Cros S., Cerdá X., Retana J. (1997). Spatial and temporal variations in the activity patterns of Mediterranean ant communities. Écoscience 4 (3), 269–278. doi: 10.1080/11956860.1997.11682405
Diamond S. E., Chick L., Perez A., Strickler S. A., Martin R. A. (2017). Rapid evolution of ant thermal tolerance across an urban-rural temperature cline. Biol. J. Linn. Soc 121 (2), 248–257. doi: 10.1093/biolinnean/blw047
Diamond S. E., Chick L. D., Perez A., Strickler S. A., Zhao C. (2018). Evolution of plasticity in the city: urban acorn ants can better tolerate more rapid increases in environmental temperature. Conserv. Physiol. 6 (1), coy030. doi: 10.1093/conphys/coy030
Dray S., Legendre P. (2008). Testing the species traits-environmental relationships: the fourth-corner problem revisited. Ecology 89 (12), 3400–3412. doi: 10.1890/08-0349.1
Egerer M. H., Arel C., Otoshi M. D., Quistberg R. D., Bichier P., Philpott S. M. (2017). Urban arthropods respond variably to changes in landscape context and spatial scale. J. Urban Ecol. 3 (1), jux001. doi: 10.1093/jue/jux001
Friedrich R., Philpott S. M. (2009). Nest-site limitation and nesting resources of ants (Hymenoptera: formicidae) in urban green spaces. Environ. Entomol. 38 (3), 600–607. doi: 10.1603/022.038.0311
Gottlieb D., Phillips B. B., Sendova-Franks A. B., Franks N. R. (2013). Individual and social information gathering are fine-tuned to the internal state of the group. Anim. Behav. 85 (6), 1479–1484. doi: 10.1016/j.anbehav.2013.03.046
Groffman P. M., Cavender-Bares J., Bettez N. D., Grove J. M., Hall S. J., Heffernan J. B., et al. (2014). Ecological homogenization of urban USA. Front. Ecol. Environ. 12 (1), 74–81. doi: 10.1890/120374
Gu D., Andreev K., Dupre M. E. (2021). Major trends in population growth around the world. China CDC Wkly. 3 (28), 604–613. doi: 10.46234/ccdcw2021.160
Heatwole H., Harrington S. (1989). Heat tolerances of some ants and beetles from the pre-Saharan steppe of Tunisia. J. Arid Environ. 16, 69–77. doi: 10.1016/S0140-1963(18)31048-6
Heatwole H., Muir R. (1989). Seasonal and daily activity of ants in the pre-Saharan steppe of Tunisia. J. Arid Environ. 16, 49–67. doi: 10.1016/S0140-1963(18)31047-4
Heatwole H., Muir R. (1991). Foraging, abundance and biomass of ants in the pre-Saharan steppe of Tunisia. J. Arid Environ. 21, 337–350. doi: 10.1016/S0140-1963(18)30672-4
Hooper-Bùi L. M., Appel A. G., Rust M. K. (2002). Preference of food particle size among several urban ant species. J. Econ. Entomol. 95 (6), 1222–1228. doi: 10.1603/0022-0493-95.6.1222
Ivanov K., Keiper J. (2010). Ant (Hymenoptera: formicidae) diversity and community composition along sharp urban forest edges. Biodivers. Conserv. 19 (14), 3917–3933. doi: 10.1007/s10531-010-9937-3
Johnson D. J., Stahlschmidt Z. R. (2020). City limits: heat tolerance is influenced by body size and hydration state in an urban ant community. Ecol. Evol. 10 (11), 4944–4955. doi: 10.1002/ece3.6247
Kaspari M., Clay N. A., Lucas J., Yanoviak S. P., Kay A. (2015). Thermal adaptation generates a diversity of thermal limits in a rainforest ant community. Glob. Change Biol. 21 (3), 1092–1102. doi: 10.1111/gcb.12750
Kaspari M., Weiser M. D. (2007). The size-grain hypothesis: do macroarthropods see a fractal world? Ecol. Entomol. 32 (3), 279–282. doi: 10.1111/j.1365-2311.2007.00870.x
Kotze J., Venn S., Niemelä J., Spence J. (2011). Effects of urbanization on the ecology and evolution of arthropods. urban ecology, patterns, processes and applications (New York: Oxford University Press), 159–166.
Laliberté E., Legendre P. (2010). A distance-based framework for measuring functional diversity from multiple traits. Ecology 91 (1), 299–305. doi: 10.1890/08-2244.1
Lin C. (1998). Systematic and zoogeographic studies on the ant subfamily myrmicinae in Taiwan (Hymenoptera: formicidae) (Taiwan: National Taiwan University Press).
Lin S.-Y., Giusto B. D., Bain A., Chou L.-S. (2016). Variation of ant community structure on Ficus benguetensis. Taiwania 61 (1), 49–57. doi: 10.6165/tai.2016.61.49
Liu K.-L., Peng M.-H., Hung Y.-C., Neoh K.-B. (2019). Effects of park size, peri-urban forest spillover, and environmental filtering on diversity, structure, and morphology of ant assemblages in urban park. Urban Ecosyst. 22 (4), 643–656. doi: 10.1007/s11252-019-00851-z
Magura T., Ferrante M., Lövei G. L. (2020). Only habitat specialists become smaller with advancing urbanization. Glob. Ecol. Biogeogr. 29 (11), 1978–1987. doi: 10.1111/geb.13168
Marsh A. C. (1985). Microclimatic factors influencing foraging patterns and success of the thermophilic desert ant, Ocymyrmex barbiger. Insectes Soc. 32 (3), 286–296. doi: 10.1007/BF02224917
McIntyre N. E. (2000). Ecology of urban arthropods: a review and a call to action. Ann. Entomol. Soc Am. 93 (4), 825–835. doi: 10.1603/0013-8746(2000)093[0825:EOUAAR]2.0.CO;2
Merckx T., Kaiser A., Van Dyck H. (2018a). Increased body size along urbanization gradients at both community and intraspecific level in macro-moths. Glob. Change Biol. 24 (8), 3837–3848. doi: 10.1111/gcb.14151
Merckx T., Souffreau C., Kaiser A., Baardsen L. F., Backeljau T., Bonte D., et al. (2018b). Body-size shifts in aquatic and terrestrial urban communities. Nature 558 (7708), 113–116. doi: 10.1038/s41586-018-0140-0
Naeem S., Wright J. P. (2003). Disentangling biodiversity effects on ecosystem functioning: deriving solutions to a seemingly insurmountable problem. Ecol. Lett. 6 (6), 567–579. doi: 10.1046/j.1461-0248.2003.00471.x
Nonacs P., Dill L. M. (1990). Mortality risk vs. food quality trade-offs in a common currency: ant patch preferences. Ecology 71 (5), 1886–1892. doi: 10.2307/1937596
Nooten S. S., Chan K. H., Schultheiss P., Bogar T. A., Guénard B. (2022). Ant body size mediates functional performance and species interactions in carrion decomposer communities. Funct. Ecol. 36 (5), 1279–1291. doi: 10.1111/1365-2435.14039
Nooten S. S., Schultheiss P., Rowe R. C., Facey S. L., Cook J. M. (2019). Habitat complexity affects functional traits and diversity of ant assemblages in urban green spaces (Hymenoptera: formicidae). Myrmecol. News 29, 67–77. doi: 10.25849/myrmecol.news_029:067
Pacheco R., Vasconcelos H. L. (2007). Invertebrate conservation in urban areas: ants in the Brazilian cerrado. Landsc. Urban Plan. 81 (3), 193–199. doi: 10.1016/j.landurbplan.2006.11.004
Peng M.-H., Hung Y.-C., Liu K.-L., Neoh K.-B. (2020). Landscape configuration and habitat complexity shape arthropod assemblage in urban parks. Sci. Rep. 10 (1), 16043. doi: 10.1038/s41598-020-73121-0
Penick C. A., Savage A. M., Dunn R. R. (2015). Stable isotopes reveal links between human food inputs and urban ant diets. Proc. R. Soc. B. 282 (1806), 20142608. doi: 10.1098/rspb.2014.2608
Petchey O. L., Gaston K. J. (2006). Functional diversity: back to basics and looking forward. Ecol. Lett. 9, 741–758. doi: 10.1111/j.1461-0248.2006.00924.x
Pfeiffer M., Nais J., Linsenmair K. E. (2006). Worker size and seed size selection in ‘seed’-collecting ant ensembles (Hymenoptera: formicidae) in primary rain forests on Borneo. J. Trop. Ecol. 22 (6), 685–693. doi: 10.1017/S0266467406003622
Rajesh T. P., Manoj K., Prashanth Ballullaya U., Shibil V. K., Asha G., Varma S., et al. (2022). Urban tropical forest islets as hotspots of ants in general and invasive ants in particular. Sci. Rep. 12, 12003. doi: 10.1038/s41598-022-16243-x
R Core Team (2020). R: a language and environment for statistical computing (Vienna, Austria: R Foundation for Statistical Computing). Available at: http://www.r-project.org/index.html.
Rocha E. A., Fellowes M. D. E. (2020). Urbanisation alters ecological interactions: ant mutualists increase and specialist insect predators decrease on an urban gradient. Sci. Rep. 10 (1), 6406. doi: 10.1038/s41598-020-62422-z
Savage A. M., Hackett B., Guénard B., Youngsteadt E. K., Dunn R. R. (2015). Fine-scale heterogeneity across manhattan's urban habitat mosaic is associated with variation in ant composition and richness. Insect Conserv. Divers. 8 (3), 216–228. doi: 10.1111/icad.12098
Schleuter D., Daufresne M., Massol F., Argillier C. (2010). A user's guide to functional diversity indices. Ecol. Monogr. 80, 469–484. doi: 10.1890/08-2225.1
Silva R. R., Brandão C. R. F. (2014). Ecosystem-wide morphological structure of leaf-litter ant communities along a tropical latitudinal gradient. PloS One 9 (3), e93049. doi: 10.1371/journal.pone.0093049
Silvestre M., Aguilar A., Seoane J., Azcárate F. M. (2019). Responses of seed size, ant worker size, and seed removal rate to elevation in Mediterranean grasslands. Oecologia 189, 781–793. doi: 10.1007/s00442-019-04356-6
Sosiak C. E., Barden P. (2021). Multidimensional trait morphology predicts ecology across ant lineages. Funct. Ecol. 35 (1), 139–152. doi: 10.1111/1365-2435.13697
Stringer L. D., Haywood J., Lester P. J. (2007). The influence of temperature and fine-scale resource distribution on resource sharing and domination in an ant community. Ecol. Entomol. 32 (6), 732–740. doi: 10.1111/j.1365-2311.2007.00924.x
Theodorou P., Baltz L. M., Paxton R. J., Soro A. (2021). Urbanization is associated with shifts in bumblebee body size, with cascading effects on pollination. Evol. Appl. 14 (1), 53–68. doi: 10.1111/eva.13087
The World Bank. (2022). World bank data: urban population. Available at: https://data.worldbank.org/indicator/SP.URB.TOTL.IN.ZS?locations=Z4&name_desc=false (Accessed July 16, 2022).
Treiber J., von Wehrden H., Zimmermann H., Welk E., Jäger E. J., Ronnenberg K., et al. (2020). Linking large-scale and small-scale distribution patterns of steppe plant species–an example using fourth-corner analysis. Flora 263, 151553. doi: 10.1016/j.flora.2020.151553
Tsai C. Y. (2019). Diversity, community structure and morphological patterns of ground-dwelling ant in urban-rural interface. (Master’s thesis) National Chung-Hsing University, 70 pp.
Uno S., Cotton J., Philpott S. M. (2010). Diversity, abundance, and species composition of ants in urban green spaces. Urban Ecosyst. 13 (4), 425–441. doi: 10.1007/s11252-010-0136-5
Van Nuland M. E., Whitlow W. L. (2014). Temporal effects on biodiversity and composition of arthropod communities along an urban–rural gradient. Urban Ecosyst. 17 (4), 1047–1060. doi: 10.1007/s11252-014-0358-z
Vepsäläinen K., Ikonen H., Koivula M. J. (2008). The structure of ant assemblages in an urban area of Helsinki, southern Finland. Ann. Zoo. Fenn. 45 (2), 109–127. doi: 10.5735/086.045.0203
Villéger S., Mason N. W. H., Mouillot D. (2008). New multidimensional functional diversity indices for a multifaceted franework in functional ecology. Ecology 89 (8), 2290–2301. doi: 10.1890/07-1206.1
Wang Y., Naumann U., Eddelbuettel D., Wilshire J., Warton D. I. (2021). Mvabund: statistical methods for analysing multivariate abundance data, R package version 4.1.12.
Weeks J. R. (2010). “Defining urban areas,” in Remote sensing of urban and suburban areas. Eds. Rashed T., Jürgens C. (Dordrecht: Springer), 33–45. doi: 10.1007/978-1-4020-4385-7_3
Wehner R., Marsh A. C., Wehner S. (1992). Desert ants on a thermal tightrope. Nature 357, 586–587. doi: 10.1038/357586a0
Weiser M. D., Kaspari M. (1999). The size–grain hypothesis and interspecific scaling in ants. Funct. Ecol. 13, 530–538. doi: 10.1046/j.1365-2435.1999.00343.x
Weiser M. D., Kaspari M. (2006). Ecological morphospace of new world ants. Ecol. Entomol. 31 (2), 131–142. doi: 10.1111/j.0307-6946.2006.00759.x
Ydenberg R., Schmid-Hempel P. (1994). Modelling social insect foraging. Trends Ecol. Evol. 9 (12), 491–493. doi: 10.1016/0169-5347(94)90321-2
Yilmaz A. R., Chick L. D., Perez A., Strickler S. A., Vaughn S., Martin R. A., et al. (2019). Remarkable insensitivity of acorn ant morphology to temperature decouples the evolution of physiological tolerance from body size under urban heat islands. J. Therm. Biol. 85, 102426. doi: 10.1016/j.jtherbio.2019.102426
Keywords: urbanization, predatory ants, ecosystem functions, biotic homogenization, nutrient recycling
Citation: Chen Y-H and Neoh K-B (2023) Urbanization causes shifts in the functional traits and foraging activity, and alters food particle size preference and biomass removal of urban-dwelling ants. Front. Ecol. Evol. 11:1044485. doi: 10.3389/fevo.2023.1044485
Received: 14 September 2022; Accepted: 28 June 2023;
Published: 14 July 2023.
Edited by:
Francisco José Cabrero-Sañudo, Complutense University of Madrid, SpainReviewed by:
Gabriela Castaño-Meneses, National Autonomous University of Mexico, MexicoAntonio Moura, Federal University of Paraíba, Brazil
Copyright © 2023 Chen and Neoh. This is an open-access article distributed under the terms of the Creative Commons Attribution License (CC BY). The use, distribution or reproduction in other forums is permitted, provided the original author(s) and the copyright owner(s) are credited and that the original publication in this journal is cited, in accordance with accepted academic practice. No use, distribution or reproduction is permitted which does not comply with these terms.
*Correspondence: Kok-Boon Neoh, neohkokboon@nchu.edu.tw