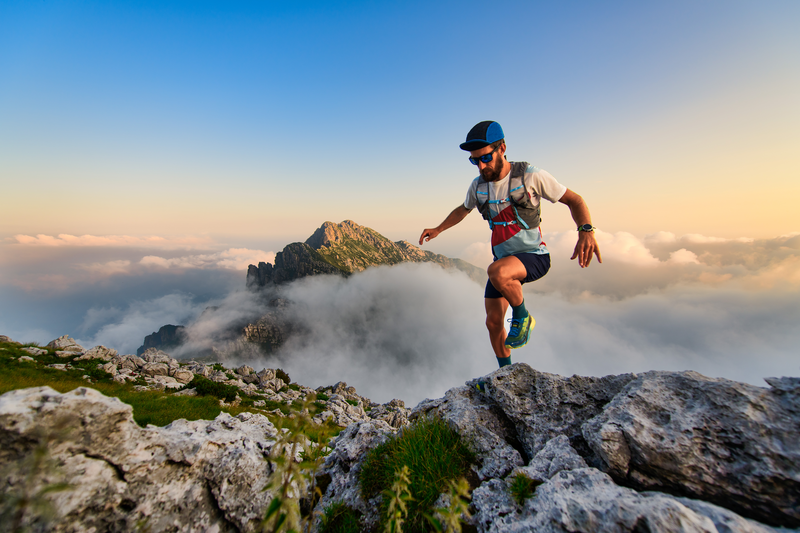
94% of researchers rate our articles as excellent or good
Learn more about the work of our research integrity team to safeguard the quality of each article we publish.
Find out more
ORIGINAL RESEARCH article
Front. Ecol. Evol. , 23 February 2023
Sec. Paleoecology
Volume 11 - 2023 | https://doi.org/10.3389/fevo.2023.1020919
This article is part of the Research Topic Textile Fiber Pollution from Source to Sink View all 6 articles
Microplastic pollution is ubiquitous, with textiles being a major source of one of the dominant microplastic types—microfibres. Microfibres have been discovered in the aquatic environment and marine biota, demonstrating direct infiltration in the environment. However, the impact of non-plastic microfibres has been overlooked until recently despite their prevalence and the ecotoxicological risk posed by chemical dyes and finishes used during processing. During an expedition from Lamu to Zanzibar (East Africa), a citizen science strategy was employed to innovate, educate and influence microfibre pollution reform through the Flipflopi project, a circular economy effort to stop the use of single-use plastic. Simple sampling methods were developed to replace costly equipment, which local citizens could use to partake in the collection and sampling of surface water samples from the previously understudied Kenyan and Tanzanian coast. To maintain the reliability of samples and to minimise contamination, a forensic science strategy was embedded throughout the methodology of the study, collection and analysis of the samples. A total of 2,403 microfibres from 37 sites were recovered and fully characterised with 55% found to be of natural origin, 8% regenerated cellulosic and 37% synthetic microfibres. Natural microfibres were in higher abundance in 33 of the 37 sampled sites. Congruent with recent studies, these findings further support the need for greater understanding of the anthropogenic impact of natural microfibres.
The ubiquitous nature of microplastics in the form of microfibres is widely acknowledged, with textiles being a major source (Pirc et al., 2016; Barrows et al., 2018; De Falco et al., 2019, 2020; Acharya et al., 2021). Microfibres refer to natural (e.g. cotton), synthetic (e.g. polyester) and regenerated cellulose (e.g. viscose) fibrous materials having a diameter less than 50 μm, length ranging from 1 μm to 5 mm and length-to-diameter ratio of more than 100 (Liu et al., 2019). They are ubiquitous in both aquatic and terrestrial environments and have been found to be more abundant than other forms of microparticles such as fragments, films, pellets, spheres or foams (Acharya et al., 2021; Kashiwabara et al., 2021; Li et al., 2021). The ubiquity of microfibres stems from the fact that they shed easily from textiles (De Wael et al., 2010; Skokan et al., 2020) and that global textile fibre production has skyrocketed over time. A 2021 report showed that in the last two decades, global fibre production has increased from 58 million tonnes in 2000 to 109 million tonnes in 2020 (Textile Exchange, 2021). Microfibres are released from clothing during laundering (De Falco et al., 2019, 2020; Lant et al., 2020), drying (Kapp and Miller, 2020; Lant et al., 2022) and normal wear (De Falco et al., 2020; Sheridan et al., 2020). These eventually end up in the air (Dris et al., 2017), on land (Zubris and Richards, 2005) and in aquatic environments mainly through wastewater treatment plants, run offs and atmospheric deposition (Browne, 2015).
Forensic scientists have used textile fibres (microfibres) to solve crimes for decades (Frank and Sobol, 1990) as garments shed their fibres easily on to other surfaces (Pounds and Smalldon, 1975). In addition to being readily shed from garments, individual fibres are mobile in the air (Moore et al., 1986; Sheridan et al., 2020). As a result, they can be used to link people, objects and environments (Grieve, 2002). Due to the invaluable role they play in criminal investigations, the process of sampling, recovery, examination and analysis are thoroughly scrutinised to ensure that any fibre evidence presented in a court of law is robust and reliable (Grieve, 2000; Robertson et al., 2017). Thus, central to forensic fibre examinations is the adoption of strict examination protocols geared towards preserving the integrity of the evidence and minimising contamination. Indeed, across the world, laboratories are expected to be accredited to international standards, e.g. ISO and ASTM (ISO, 2017; ASTM E2228-19, 2019) before they can undertake forensic fibre examinations. The fibre examination process includes the use of appropriate PPE, cleaning and/or ‘blanking’ of workstations prior to and after use, the use of stereomicroscopes when recovering fibres to minimise contamination and/or loss, and the use of unique identification systems for tracking individual recovered fibres pending further analysis. In addition to preserving evidence in the state it was discovered, reliable classification and identification of both the fibre itself and their dyes stuffs is crucial to discriminate between sometimes 1000’s of seemingly similar fibres. Relatively simple, non-destructive techniques (microscopic) are prioritised over more complex and/or destructive methods (e.g. FTIR, SEM, py-GC–MS). Natural or regenerated cellulosic fibres are accurately and quickly identified using a rigorous identification procedure primarily using microscopic techniques. Synthetic fibres are classified using specialist microscopy techniques (Polarising Light Microscopy) with mFTIR used only to determine exact polymer information (e.g. Nylon 6 v Nylon 6,6). Thus, applying well-established, purpose-built forensic science processes to environmental studies involving microfibres will yield fast, accurate and reliable results by (1) minimising contamination and (2) ensuring the accurate classification/identification of all fibre types (Woodall et al., 2015; Gwinnett and Miller, 2021).
Studies carried out over the years in the forensic field have consistently found natural fibres to be more prevalent in the environment e.g. on outdoor surfaces (Grieve and Biermann, 1997), in human hair (Palmer and Oliver, 2004) and more recently on parapets (Eng and Koh, 2022). Studies elsewhere evidence the abundance of anthropogenic natural microfibres. For example, a study of soiled consumer wash loads from 79 households in the United Kingdom, released an average of 114 ± 66.8 mg microfibres per kg fabric with microfibres of natural origin being dominant (Lant et al., 2020). A more recent study comparing the release of microfibres from the same number of cotton and polyester T-shirts laundered under the same conditions showed that the release of cotton microfibres was significantly higher both down the drain and into the air through tumble dryers (Lant et al., 2022). Nonetheless, until recently (Stanton et al., 2019), limited attention had been given to the presence of natural microfibres in environmental pollution studies despite an increasing number (Miller et al., 2017) resulting in likely underestimation of microfibres and invariably, their impact (De Falco et al., 2020). The focus on synthetic microfibres is in part a result of the broader plastic pollution conversation and often cited as the inherent threat they pose due to their inability to degrade easily (Rebelein et al., 2021). The recovery of a cotton waistcoat after 133 years of deep ocean ship wreck (Chen and Jakes, 2001) may be an indication that natural microfibres are not as readily biodegradable as has been suggested, although preservation may be due to the high pressure and low temperature conditions.
The Indian Ocean is the third largest of the five oceans of the world and makes up about 20% of the Earth’s water surface. Knowledge about anthropogenic microfibres contained therein would therefore be relevant in understanding global microfibre pollution. So far, several studies have been carried out at different regions that make up this ocean’s capacity. Extrapolation of data from a study of seamount sediments of its Southwestern region estimates that 4 billion fibres per km2 was present in the ocean (Woodall et al., 2014). Within its Eastern region, an atmospheric deposition study showed the abundance of natural fibres with cotton occurring the most (Wang et al., 2020). However, there is a paucity of studies regarding anthropogenic microfibres in the African region of the Indian Ocean. A recent review noted that only three studies were found that had investigated anthropogenic microfibres on the African continent making it the least studied continent on the planet (Athey and Erdle, 2021). Despite data showing the prevalence of microfibres in creeks, lakes and zooplankton (Kosore et al., 2018; Kerubo et al., 2020, 2021; Jeevanandam et al., 2022), these studies have concentrated on methodologies that do not account for the presence of natural microfibres, which serves only to further widen the knowledge gap and misunderstanding.
In Africa, education and awareness (Khan et al., 2018; Migwi et al., 2020) are critical if the global microplastic pollution challenge is to be resolved. In 2019, The Flipflopi was built; the world’s first recycled plastic sailing dhow. The Flipflopi’s sailing expeditions across regions in East Africa (The Flipflopi Project, 2019) raises awareness of plastic pollution through the training and education of local citizens. A recent review (Alimi et al., 2021) recommended that collaborations be made between researchers in Africa and international laboratories to address the issue of robust research methodology bearing in mind the high cost of laboratory instruments. Their data showed that more than 50% of the published studies in microplastic pollution relied on visual identification of microplastic particles. However, most studies in this field rely on sophisticated, and often expensive sampling equipment (Hidalgo-Ruz et al., 2012; Campanale et al., 2020) conducted by skilled, trained scientists. To overcome this, simple and easy to use instrumentation could be developed in the absence of more sophisticated instrumentation and /or to involve citizen scientists who lack the skills to operate such instrumentation. The added benefits of involving local citizens in sample collection includes increased awareness, faster data collection and coverage of a wider geographical area in a relatively short time.
In the present study, samples from 37 different locations were collected from 24th January 2019 through to 6th February 2019 during the Flipflopi’s sailing expedition across 500 km of the Indian Ocean from Lamu to Zanzibar (Figure 1). Details of the sampled locations can be accessed via the link provided https://tinyurl.com/flipflopimap and the summary table in the Supplementary Table S1. The extent of microfibre pollution on surface waters was investigated by applying forensic science principles and involving local citizens. A systematic approach was taken to objectively identify all microfibres using microscopy and/or spectroscopy addressing one of the challenges earlier noted.
Figure 1. Map showing snapshot of 37 sampling locations from Lamu (Kenya) to Zanzibar (Tanzania) with arrow showing direction of expedition.
A bespoke, simple, inexpensive and easy to operate sampling device for the filtering of collected water was constructed from a coffee AeroPress® bought online. To ensure any microfibres present in the sampled water were collected, nylon filters replaced the regular paper filters usually used. The nylon filters were made from a 50 μm nylon mesh sheet (Plastok Associates Ltd., United Kingdom) that were laser cut into 50 mm diameter discs using a laser (Epilog Laser Legend 36EXT, United Kingdom). The nylon filter was placed on top of the AeroPress® metal filter. The AeroPress® was then connected to a 1,000 mm flexible High-Density Polyethylene (HDPE) plastic tube (80 Ø mm) with an aperture held open by rigid wide plastic tube to facilitate easy transfer of ocean water (Figure 2).
At each sampling site, a 2 L plastic jug was used to draw ocean water from the surface down to a maximum depth of 100 mm below the surface. The water was then poured through the tube and passed through the filter back into the ocean. This process was repeated three times sampling a total of 6 L of water. Once all the water had been filtered through the AeroPress® sampler, the nylon mesh filter containing any collected debris was removed from the device using tweezers and placed into a sealed zip lock labelled bag ready for transfer back to the United Kingdom. To sample at the next site, the sampling device was rinsed with bottled water that was stored onboard and a new filter inserted ready for collection. This process was repeated for each of the 37 sampling sites.
All samples (remaining within their sealed plastic bags) were returned to the United Kingdom where they were allowed to thoroughly dry in a fume cupboard. Once dry, each filter was removed from its plastic bag and transferred to a clean Petri dish (with lid) to prevent contamination from airborne fibres. The Petri dishes containing the filters were examined under a low power microscope (10x–40x, Leica S6E, Germany) to confirm the presence of microfibres before further sampling was conducted. Due to the difficulty of locating colourless microfibres, only coloured microfibres were recovered. Microfibres were observed in all samples.
The Petri dish lid was then removed and microfibres were individually removed with forceps and transferred to a glass slide loaded with Phytohistol mountant made in Northumbria University Forensic lab (see Supplementary Information for details) and covered with a glass coverslip. The entire process was conducted whilst under the microscope. This fibre recovery procedure, designed to minimise the possibility of contamination, is a standard accredited procedure used by forensic scientists worldwide (ASTM E2228-19, 2019). Using an Olympus CX22 microscope coupled with Euromex camera with Image Focus 4.0 software (J.B Microscopes Limited, United Kingdom), images of the recovered microfibres on the slides were taken. These images were electronically stitched together and printed on A3 sheet, providing a clear overview of each fibre’s location. Each recovered fibre was individually, and consecutively, numbered by site and sample number such that each individual microfibre could be traced back to the location from which it was obtained.
Microfibres, once recovered from the filters and mounted on glass slides, were analysed using bright-field microscopy (×100–400) (Comparison Microscope, Leica DMR, Germany), followed by, if necessary, Polarising Light Microscopy (PLM) (Leica DM2700P, Germany). Both are standard, accredited methods for the characterisation and identification of textile fibres and have been used in forensic fibres studies (Palmer et al., 2015; Eng and Koh, 2022). Bright-field microscopy allows fibre discrimination of natural and man-made fibres (e.g. cotton, wool and viscose) based on their characteristic morphological features, whereas PLM is particularly useful for the identification and discrimination of synthetic microfibres (e.g. acrylic, nylon and polyester) through the exploitation of their optical properties. It is beyond the scope of this paper to explain how PLM can be used for the identification of fibre types. For those interested, we would recommend the introductory text by Greaves and Saville (1995).
The limited sample preparation, in situ analysis, speed and accuracy of microfibre characterisation by microscopic methods makes this the preferred sequence for the identification of fibre type. When further confirmation was required either due to damage or being heavily dyed or features not being clear, further analysis was carried out using an mFTIR (Perkin Elmer Frontier connected to spotlight 150i Microscope). Measurement was taken in transmittance mode with wavelength of 4,000–500 cm−1, resolution of 4 cm−1 and accumulation of 32 scans. Spectra of unknown fibres were compared with spectra contained in the internal textile fibre spectral libraries. The spectral library was created using known, authenticated textile fibre collection donated to Northumbria University from The Forensic Science Service (United Kingdom). A correlation value of >0.5 was considered an acceptable match in addition to visual examination of the spectral images. Following analysis, the microscopic fibre colour and generic fibre type was determined. Fibre classification of microfibre types found in this study is shown in Table 1.
To prevent contamination of the samples from microfibres in the environment, strict laboratory protocols were followed during the microfibre recovery process. Clean, new, white cotton laboratory coats, composed of colourless cotton fibres, were worn since colourless fibres were excluded from examination. Post-drying, samples were contained within clean, closed, petri dishes. Laboratory benches and lab equipment were thoroughly cleaned with Vikron prior to and after use, and in between samples. Following cleaning, the benches were lined with fresh clean brown paper. All fibre observation and recovery were performed under a stereomicroscope in order to minimise contamination, as any superfluous fibres would be noted and discarded. The samples remained covered anytime the sample was not actively being examined.
Kruskal–Wallis Test and Mann–Whitney U tests were used to determine statistically significant differences between natural, synthetic and regenerated fibres. All statistical calculations and graphs were conducted using Microsoft Excel™ for Microsoft 365 Version 2,205.
A total of 2,403 fibres were recovered across 37 sampled locations (Figure 3). Nineteen fibres were either lost or damaged during sample preparation, two had very poor-quality spectra and could not be identified, resulting in a total of 2,382 fibres being fully identified. This translates into an average of 10.73 ± 1.99 fibres L−1 across the 37 sampled locations. Location 28 (see Supplementary Table S1 details of location) had the highest concentration at 58.67 ± 6.23 fibres L−1, whilst the location with the least concentration of fibres was location 31 with 1.33 ± 0.17 fibres L−1.
Figure 3. Concentration of fibre types identified across 37 sampled locations along the Kenyan coast.
The recovered microfibres were first broadly classified into three categories namely, natural, synthetic or regenerated cellulosic (semi-synthetic) using bright-field microscopy, before ‘synthetic’ fibres were explicitly identified using PLM. A total of nine different fibre types were identified namely acrylic, cotton, nylon, polyester, polypropylene, polyethylene, vegetable, viscose and wool (Table 1). Of the three broad categories, natural fibres were of greatest abundance (55%), followed by synthetic fibres (37%) with the least being regenerated cellulosic microfibres (8%) (Figures 4, 5). The regenerated cellulosic category was represented by only one type of fibre, viscose. Natural fibres had the highest abundance in 33 of the 37 sampled sites. Using the Kruskal–Wallis test, statistically significant differences were found between fibre categories and their distribution across the sampled locations (H = 52.82, value of p < 0.00001 and α = 0.05). Further analysis using the Mann–Whitney Test indicated that natural fibres were statistically significantly more abundant than both synthetic and regenerated fibres (Z-Score = 3.346, value of p = 0.001 and α = 0.05) and (Z-Score = 6.573, value of p < 0.00001 and α = 0.05), respectively. Synthetic fibres were found to be significantly more abundant in distribution compared to regenerated fibres (Z-Score = −5.059, value of p < 0.00001 and α = 0.05). The natural fibre type with the highest occurrence was cotton, with a mean of 5.24 ± 0.95 fibres L−1 (n = 37 locations). Polyester was the highest occurring synthetic fibre across the 37 sampled locations, with a mean of 2.93 ± 0.63 fibres L−1. The least occurring fibre overall was polyethylene with a mean concentration of 0.01 ± 0.01 fibres L−1 (Figure 6).
Figure 5. Concentrations of natural, synthetic and regenerated cellulosic fibres distributed across the 37 sampled locations. *Statistically significant difference at α = 0.05.
Owing to the difficulty of observing colourless fibres, only coloured fibres were recovered and characterised in this study. Blue and black/grey were the highest occurring colours at 39.2 and 29.4%, respectively, whilst the least abundant colour was purple with a percentage composition of 0.5% (Figure 7). Red microfibres were also commonly found in 86% of the locations. When combined, blue, black/grey and red, made up approximately 78% of the microfibres recovered in each location. Blue fibres occurred highest in location 18 (67%), whilst black/grey was the highest in location 5 (68%). It was observed that some colours were more associated with certain fibre groups especially with respect to the relatively rarer colours. For example, yellow fibres were composed of approximately 79% natural, 14% synthetic and 7% regenerated fibres, whereas orange is composed of approximately 67% synthetic, 19% natural and 14% regenerated, compared with blue microfibres composed of approximately 40% natural, 56% a synthetic and 5% regenerated microfibres.
The sampling sites were grouped according to geographic regions and their human population sizes (Table 2). The relationship between fibre concentration and population size was explored. No clear trend was observed, supported by the absence of any statistical significance (p = 0.067 and α = 0.05). Attempt was also made to determine whether there was a relationship between fibre concentration and the distance of sampled locations from land. See supplementary information for more detail. A negative correlation was observed between fibre concentration and distance from land, with fibre concentration being high closer to land. However, this relationship was not found to be statistically significant (p = 0.32 and α = 0.05) and (p = 0.18 and α = 0.05) either when data were untreated or log-transformed, respectively. The correlation of concentration of specific fibre types to the distance of the sampled location to nearest land showed similar results between polyester and wool (see Supplementary Document).
Microfibre concentration found in this study ranged from 1.33 ± 0.17 fibres L−1 to 58.67 ± 6.23 fibres L−1, generally increasing as the sampling location was closer to land. A mean concentration of 10.73 ± 1.99 fibres L−1 was found across all locations. These data are comparable with results obtained in a 2017 expedition involving 617 locations across 6 oceanic basins including the Indian Ocean (Suaria et al., 2020). However, a previous study on the Indian Ocean reported a lower average (4.2 ± 1.2 particles L−1) (Barrows et al., 2018). In contrast to this present study, their work included quantification of fragments other than microfibres, although 91% were identified as microfibres including those that were clear/transparent. In the absence of a direct comparison study, it is difficult to understand the exact reasons for such differences. Differences in recorded quantities could have been due to differing methodologies (Athey and Erdle, 2021). Variability in data has been stated as a possibility when a relatively small volume of water is used for sampling (Barrows et al., 2018), as was the case in Barrows et al. study when a smaller 1 L grab was used as opposed to the 6 L used in this study.
The reported samples with a lower microfibre concentration (Barrows et al., 2018) were collected 6 years prior to those in this study and 4 years prior to the research published in 2020 (Suaria et al., 2020). Between 2013 and 2019, textile production has increased. Given it is widely accepted that textiles are a major source of anthropogenic microfibres found in the aquatic environment, higher productions of textiles will invariably translate to higher release of anthropogenic microfibres. This may be particularly true for regions of Africa due to inadequate or lack of waste control (Alimi et al., 2021).
Of the microfibres found in this study, only 37% of them were synthetic, and almost half of all natural identified as cotton fibres. This finding substantiates recent evidence (Stanton et al., 2019), speculations (Ladewig et al., 2015) and laundry experiments (Lant et al., 2020, 2022) that natural microfibres are more prevalent in the aquatic environment than their synthetic and regenerated cellulosic counterparts. Moreover, forensic science studies that aim to determine the frequency of occurrence of different fibre types in terrestrial environments, have consistently shown that natural fibres, and particularly cotton, are more prevalent compared to other fibre types (Grieve and Biermann, 1997; Cantrell et al., 2001; Eng and Koh, 2022). The findings of this and other recent environmental studies are thus congruent with that of forensic science studies; terrestrial and aquatic environments appear to be dominated by natural fibre types.
Black/grey and blue microfibres were the highest occurring colours, followed by red, and were present in all locations. In fact, when combined, black/grey, blue and red colours accounted for the majority of microfibres found. The recurring abundance of these three colours in almost all locations is in line with that of other anthropogenic studies (Nel and Froneman, 2015; Suaria et al., 2020; Li et al., 2021; Jeevanandam et al., 2022). Forensic studies of microfibres prevalence on various surfaces where textile fibres are shed such as seats (Cantrell et al., 2001), head hair (Palmer and Oliver, 2004) and on skin (Palmer and Burch, 2009) have also shown the high prevalence of these colours. These findings are unsurprising given black, blue and red clothing are a common choice of colour of textile (Fornazarič and Toroš, 2018; Sanad, 2018; Sidhu et al., 2021).
Worryingly, studies have indicated that certain aquatic organisms, such as the omnivore Amberstripe scad (Decapterus muroadsi, Ory et al., 2017 and Girella laevifrons, Mizraji et al., 2017), tend to ingest blue and red fibres, respectively, as these have the same colour as their natural diets. Research into how other forms of colourful diets such as red and green algae which are found in the Kenyan coast (Bolton et al., 2007) may also be mistaken with red and green microfibres ought to be carried out. The implication being that the more information that is available on the prevalence of various microfibre colours in different regions, a better prediction could be made on which organisms are at a higher risk as they have the tendency of mistaking these fibres with their diet. Evidence from both field and laboratory studies has shown that ingesting microfibres is hazardous (Athey and Erdle, 2021) especially because these microfibres serve as a habitat for pathogens (First Sentier MUFG and Sustainable Investment Institute, 2022).
Biodegradability of microfibres may be a factor that determines the abundance or scarcity of microfibres, and it is one solution to microfibre pollution that many fashion brands and activists are calling for. The easier it is for microfibres to biodegrade, the less risk they pose to the environment. However, the overwhelming presence of ‘natural’ microfibres across all environments, especially cotton and wool, and with examples of textiles found intact after 100’s of years, indicates that this relationship may be more complex or much less significant compared to other factors contributing to microfibre accumulation. Some authors have speculated that the prevalence of cellulosic fibres may be because of slow degradation and therefore accumulate over time since they are the oldest forms of textile (Suaria et al., 2020). However, a study into the biodegradability of cotton showed that the time it takes for cotton to biodegrade would in part depend on the finishes applied during production (Zambrano et al., 2021) and other factors such as level of exposure (Arshad et al., 2014). These finishes when released into the aquatic environment may have toxicological implications. It is important to note that faster biodegradability may translate to quicker release of toxic compounds such as chemical and dye components (Ladewig et al., 2015). Furthermore, by the time a cotton fibre has been ‘processed’ its chemical structure is no longer in its original natural state (Krässig, 1993; Kljun et al., 2011). Cellulosic fibres have been found to be more biodegradable compared to wool when subjected to similar conditions (Arshad et al., 2014). The medium where these microfibres are present apparently affects the duration of degradation. For example, a laboratory experiment has shown that wool biodegrades easier in soil compared to an aqueous medium (Muniyasamy and Patnaik, 2021). This study also showed that the rate of degradation slows down following a period of accelerated degradation. However, precise and specific laboratory conditions that demonstrate fibre biodegradation may not necessarily correlate with real life environments, as has been demonstrated here by the sheer quantity of natural fibres.
Generally, fibre concentration appeared to decrease as sample location was further away from land. However, the data was not found to be of statistical significance. This may be in part due to large inter-sample variance which was not compensated for, by analysing replicate samples (Ryan et al., 2020).
Approximately 84% of samples were collected less than 5 km from land. To determine whether a relationship does exist, further sampling would be required, to increase the precision of the data. On the other hand, no apparent relationship was observed between fibre concentration and human population. One of the challenges faced in the analysis of this relationship was the absence of detailed population data for communities closest to the sampled locations. However, these authors (Nel et al., 2017) in their study of water and sediment samples collected in South Africa found a weak correlation between human population and fibre concentration. Moreover, they did find a high concentration of microfibres in samples collected from two harbours. Nevertheless, there is seemingly contradictory observations of relationships between microfibre concentration and distance to land and human population. Factors complicating the issue may include the manner in which people wash their clothes. Wastewater from washing machines has been noted as a major pathway for anthropogenic microfibres to the aquatic environment, but just 21% of households in Kenya use washing machines (Kunst, 2022). According to a 2017 survey, more than half of Nairobi residents wash their clothing by hand (Isamado, 2017), and dispose of the wastewater straight on the ground. The implication is that a greater proportion of the microfibres released may be retained in soil rather than the aquatic environment. The proportion that is not retained in the soil may end up in the aquatic environment as a result of runoff and wind influences. However, regardless of direct anthropogenic activity in the environment, the intricate and complicated interaction of water circulation (Kerubo et al., 2020) and atmospheric microfibre content (Finnegan et al., 2022) may have a greater impact on fibre concentration found in surface water.
Anti-contamination procedures adapted from forensic examination of fibres were followed during the examination and analysis of microfibres in the laboratory. This procedure minimises the potential for contamination from atmospheric fibres as fibre recovery is controlled by being conducted entirely with the sample in view under the microscope (following equipment and bench cleaning procedures). Contamination may have been introduced through sample collection on board and through the use of bottled water to rinse equipment. Although citizen scientists who were involved in sample collection were provided with Flipflop branded uniforms made from 100% cotton including white face caps, white or blue T-shirts and beige cotton shorts, no control materials were collected and thus their elimination as contaminants was not possible, as would usually be the case in forensic science practice. As a result, there may be an overestimation of the quantity of blue cotton fibres (beige cotton fibres will appear colourless under the microscope and thus the shorts can therefore be eliminated as a contaminant). Nonetheless, the impact of the possible overestimation of the data obtained herein may be minimal due to the variability in shades of blue indicating they have originated from multiple sources [and therefore source(s) other than the blue t-shirts]. Addressing contamination issues during the sampling stage have been re-iterated in this study (Torre et al., 2016) and more recently by Gwinnett and Miller (2021) who showed that contamination can be minimised by at least 36.9% during both sampling and processing of samples when strict quality assurance and controls are followed. Future research would benefit by prioritising this issue by providing clothing made with relatively rarer colours such as orange, purple and be mindful of the garment’s rate of shedding for those involved in sample collection (Gwinnett and Miller, 2021).
Simplicity and repeatability have been outlined as important factors to be considered when utilising a citizen science approach in (micro)plastic pollution studies (Hidalgo-Ruz et al., 2012; Rambonnet et al., 2019). To ensure the role of the volunteers was not cumbersome and complicated, a simple and inexpensive sampling device modified from a commercially available AeroPress® was designed. The use of the device required little expertise, ensuring the emphasis was placed on the experimental procedure to maintain high-quality standards and ensure collected data were reliable. The success of this approach is borne out in the scientific findings of the study. Microfibres were recovered in all samples, falling in line with broad expectations based on other published microfibre aquatic studies. The presence of one or two dominant specific colour/type(s) of microfibres, e.g. navy blue, round, semi-delustered, 18 μm ∅, polyester fibre, within and across multiple locations would have indicated a contamination source, poor sampling technique and/or poor methods. This wasn’t the case as the recovered microfibres were of a wide and random variety, within and between locations. This provided confidence that not only is the sample device suitable for this type of study but that it can be correctly operated by citizens with little to no prior knowledge or experience. It also demonstrates that citizen scientists can execute strict quality assurance protocols to ensure reliable and publishable data is collected (Rambonnet et al., 2019).
Moreover, not only did this raise awareness of microfibre pollution as the reality was made evident, but a sense of responsibility was ignited in local citizens which will hopefully change attitudes and behaviours in the future.
In a previously understudied region of Lamu to Zanzibar along the Kenyan coastline, anthropogenic microfibres were found in high concentration, similar to other regions in the Indian Ocean. Moreover, natural microfibres were found to be significantly higher in abundance than their synthetic counterparts, challenging the widely held view that ‘natural’ fibres pose no risk as they biodegrade. Greater focus is therefore needed to research factors affecting the rate of biodegradability of natural fibres and the risk they may or may not pose to aquatic life.
The methodological approach taken in this study was one adopted by well-established practices and procedures routinely used by forensic scientists for the collection, recovery, examination and analysis of textile fibres. This approach ensured that all microfibres were recovered, contamination was kept at a minimum, quantification was accurate and all microfibres were correctly classified in the most efficient way possible.
We have demonstrated in this study, that a simple, inexpensive sampling device can be effectively used by local citizens with no prior experience or knowledge in the field, and by following strict protocols produce reliable and publishable data. As well as increasing awareness though the involvement of local citizens, this approach could open up possibilities for researchers who do not have access to expensive equipment, resulting in greater and faster data collection from which we can all benefit.
The original contributions presented in the study are included in the article/Supplementary material, further inquiries can be directed to the corresponding authors.
CK-O: data curation, formal analysis, investigation, methodology, writing—original draft and writing—review and editing. UA-N and LB: data curation, methodology and writing—review and editing. VB: conceptualization, project administration, resources and writing—review and editing. FK: formal analysis, investigation, writing—original draft and writing—review and editing. MG: formal analysis, investigation and writing—review and editing. KS and SS-H: conceptualization, data curation, formal analysis, investigation, methodology, project administration, resources, supervision, writing—original draft and writing—review and editing. All authors contributed to the article and approved the submitted version.
Consumables were supplied by Northumbria University. Materials used in sample collection in Kenya were funded by The Flipflopi project.
VB was employed by the company Flipflopi Project Foundation Limited.
The remaining authors declare that the research was conducted in the absence of any commercial or financial relationships that could be construed as a potential conflict of interest.
All claims expressed in this article are solely those of the authors and do not necessarily represent those of their affiliated organizations, or those of the publisher, the editors and the reviewers. Any product that may be evaluated in this article, or claim that may be made by its manufacturer, is not guaranteed or endorsed by the publisher.
The Supplementary material for this article can be found online at: https://www.frontiersin.org/articles/10.3389/fevo.2023.1020919/full#supplementary-material
Acharya, S., Rumi, S. S., Hu, Y., and Abidi, N. (2021). Microfibers from synthetic textiles as a major source of microplastics in the environment: a review. Text. Res. J. 91, 2136–2156. doi: 10.1177/0040517521991244
Alimi, O. S., Fadare, O. O., and Okoffo, E. D. (2021). Microplastics in African ecosystems: current knowledge, abundance, associated contaminants, techniques, and research needs. Sci. Total Environ. 755:142422. doi: 10.1016/j.scitotenv.2020.142422
Arshad, K., Skrifvars, M., Vivod, V., Valh, J., and Voncina, B. (2014). Biodegradation of natural textile materials in soil. Tekstilec 57, 118–132. doi: 10.14502/Tekstilec2014.57.118-132
Athey, S. N., and Erdle, L. M. (2021). Are we underestimating anthropogenic microfiber pollution? A critical review of occurrence, methods, and reporting. Environ. Toxicol. Chem. 41, 822–837. doi: 10.1002/etc.5173
Barrows, A., Cathey, S. E., and Petersen, C. W. (2018). Marine environment microfiber contamination: global patterns and the diversity of microparticle origins. Environ. Pollut. 237, 275–284. doi: 10.1016/j.envpol.2018.02.062
BBC NEWS. (2018). Zanzibar Profile. Available at: https://www.bbc.co.uk/news/world-africa-14115176 ().
Bolton, J., Oyieke, H., and Gwada, P. (2007). The seaweeds of Kenya: checklist, history of seaweed study, coastal environment, and analysis of seaweed diversity and biogeography. S. Afr. J. Bot. 73, 76–88. doi: 10.1016/j.sajb.2006.08.006
Browne, M. A. (2015). “Sources and pathways of microplastics to habitats” in Marine Anthropogenic Litter. eds. M. Bergmann, L. Gutow, and M. Klages (Cham: Springer), 229–244.
Campanale, C., Massarelli, C., Savino, I., Locaputo, V., and Uricchio, V. F. (2020). A detailed review study on potential effects of microplastics and additives of concern on human health. Int. J. Environ. Res. Public Health 17:1212. doi: 10.3390/ijerph17041212
Cantrell, S., Roux, C., Maynard, P., and Robertson, J. (2001). A textile fibre survey as an aid to the interpretation of fibre evidence in the Sydney region. Forensic Sci. Int. 123, 48–53. doi: 10.1016/S0379-0738(01)00520-5
Chen, R., and Jakes, K. A. (2001). Cellulolytic biodegradation of cotton fibers from a deep-ocean environment. J. Am. Inst. Conserv. 40, 91–103. doi: 10.1179/019713601806113076
De Falco, F., Cocca, M., Avella, M., and Thompson, R. C. (2020). Microfiber release to water, via laundering, and to air, via everyday use: a comparison between polyester clothing with differing textile parameters. Environ. Sci. Technol. 54, 3288–3296. doi: 10.1021/acs.est.9b06892
De Falco, F., Di Pace, E., Cocca, M., and Avella, M. (2019). The contribution of washing processes of synthetic clothes to microplastic pollution. Sci. Rep. 9, 1–11. doi: 10.1038/s41598-019-43023-x
De Wael, K., Lepot, L., Lunstroot, K., and Gason, F. (2010). Evaluation of the shedding potential of textile materials. Sci. Justice 50, 192–194. doi: 10.1016/j.scijus.2010.06.001
Dris, R., Gasperi, J., Mirande, C., Mandin, C., Guerrouache, M., Langlois, V., et al. (2017). A first overview of textile fibers, including microplastics, in indoor and outdoor environments. Environ. Pollut. 221, 453–458. doi: 10.1016/j.envpol.2016.12.013
Eng, V., and Koh, S. (2022). The population of textile fibres on parapets of high-rise housing in Singapore. Forensic Sci. Int. 336:111320. doi: 10.1016/j.forsciint.2022.111320
Finnegan, A. M. D., Süsserott, R., Gabbott, S. E., and Gouramanis, C. (2022). Man-made natural and regenerated cellulosic fibres greatly outnumber microplastic fibres in the atmosphere. Environ. Pollut. 310:119808. doi: 10.1016/j.envpol.2022.119808
First Sentier MUFG and Sustainable Investment Institute (2022). Microfibres: The Invisible Pollution from Textiles Sources, Distribution and Interventions. Available at: https://www.firstsentier-mufg-sustainability.com/content/dam/sustainabilityinstitute/assets/research/FSI-Sustainability-Investment-Institute-Report-January2022-final.pdf
Fornazarič, M., and Toroš, J. (2018). Relationship between behavioural factors and colour preferences for clothing. Tekstilec 61, 4–14. doi: 10.14502/Tekstilec2018.61.4-14
Frank, R. S., and Sobol, S. P. (1990). Fibres and their examination in forensic science. Forensic Sci. Progress 4, 41–125. doi: 10.1007/978-3-642-75186-8_3
Greaves, P. H., and Saville, B. P. (1995). Microscopy of Textile Fibres, Oxford: BIOS Scientific in Association with the Royal Microscopical Society.
Grieve, M. C. (2000). Back to the future −40 years of fibre examinations in forensic science. In Proceedings of the 40th Anniversary Meeting of the Forensic Science Society, November 1999. 40, 93–99.
Grieve, M. C. (2002). The changing face of fibre examinations in forensic science. Z Zagadnien Nauk Sadowych, z. L, 50, 155–165.
Grieve, M. C., and Biermann, T. (1997). The population of coloured textile fibres on outdoor surfaces. Sci. Justice 37, 231–239. doi: 10.1016/S1355-0306(97)72196-8
Gwinnett, C., and Miller, R. Z. (2021). Are we contaminating our samples? A preliminary study to investigate procedural contamination during field sampling and processing for microplastic and anthropogenic microparticles. Mar. Pollut. Bull. 173:113095. doi: 10.1016/j.marpolbul.2021.113095
Hidalgo-Ruz, V., Gutow, L., Thompson, R. C., and Thiel, M. (2012). Microplastics in the marine environment: a review of the methods used for identification and quantification. Environ. Sci. Technol. 46, 3060–3075. doi: 10.1021/es2031505
Isamado, (2017). Who’s washing your clothes? Available at: https://isamado.co.ke/blogpost/washing-your-clothes-in-nairobi/ ().
ISO. (2017). ISO/IEC 17025 – General Requirements for the Competence of Testing and Calibration Laboratories. Available at: https://www.iso.org/files/live/sites/isoorg/files/store/en/PUB100424.pdf
Jeevanandam, M., Talelign, W., Biru, A., Sakthi, J. S., Silva, J. D., Saravanan, P., et al. (2022). Evidences of microplastics in Hawassa Lake, Ethiopia: a first-hand report. Chemosphere 296:133979. doi: 10.1016/j.chemosphere.2022.133979
Kapp, K. J., and Miller, R. Z. (2020). Electric clothes dryers: an underestimated source of microfiber pollution. PLoS One 15:e0239165. doi: 10.1371/journal.pone.0239165
Kashiwabara, L. M., Kahane-Rapport, S. R., King, C., Devogelaere, M., Goldbogen, J. A., and Savoca, M. S. (2021). Microplastics and microfibers in surface waters of Monterey Bay National Marine Sanctuary, California. Mar. Pollut. Bull. 165:112148. doi: 10.1016/j.marpolbul.2021.112148
Kenya National Bureau of Statistics. (2019). 2019 Kenya Population and Housing Census. Population by County and Sub-county. Available at: https://www.knbs.or.ke/?wpdmpro=2019-kenya-population-and-housing-census-volume-i-population-by-county-and-sub-county
Kerubo, J. O., Muthumbi, A. W., Onyari, J. M., Kimani, E. N., and Robertson-Andersson, D. (2020). Microplastic pollution in the surface waters of creeks along the Kenyan coast, Western Indian Ocean (WIO). Western Indian Ocean J. Mar. Sci. 19, 75–88. doi: 10.4314/wiojms.v19i2.6
Kerubo, J., Muthumbi, A., Onyari, J., Robertson-Andersson, D., and Kimani, E. (2021). Microplastics pollution in the sediments of creeks and estuaries of Kenya, western Indian Ocean. Afr. J. Mar. Sci. 43, 337–352. doi: 10.2989/1814232X.2021.1966505
Khan, F. R., Mayoma, B. S., Biginagwa, F. J., and Syberg, K. (2018). “Microplastics in inland African waters: presence, sources, and fate” in Freshwater Microplastics. eds. M. Wagner and S. Lambert (Cham: Springer)
Kljun, A., Benians, T. A., Goubet, F., Meulewaeter, F., Knox, J. P., and Blackburn, R. S. (2011). Comparative analysis of crystallinity changes in cellulose I polymers using ATR-FTIR, X-ray diffraction, and carbohydrate-binding module probes. Biomacromolecules 12, 4121–4126. doi: 10.1021/bm201176m
Kosore, C., Ojwang, L., Maghanga, J., Kamau, J., Kimeli, A., Omukoto, J., et al. (2018). Occurrence and ingestion of microplastics by zooplankton in Kenya's marine environment: first documented evidence. Afr. J. Mar. Sci. 40, 225–234. doi: 10.2989/1814232X.2018.1492969
Krässig, H. A. (1993). Cellulose: Structure, Accessibility and Reactivity. Pennsylvania: Gordon and Breach Science Publ.
Kunst, A. (2022). Household appliances ownership in Kenya (2021). Statista. Available at: https://www.statista.com/forecasts/1191002/household-appliances-ownership-in-kenya (Accessed July 14, 2022).
Ladewig, S. M., Bao, S., and Chow, A. T. (2015). Natural fibers: a missing link to chemical pollution dispersion in aquatic environments. Environ. Sci. Technol. 49, 12609–12610. doi: 10.1021/acs.est.5b04754
Lant, N. J., Defaye, M. M., Smith, A. J., Kechi-Okafor, C., Dean, J. R., and Sheridan, K. J. (2022). The impact of fabric conditioning products and lint filter pore size on airborne microfiber pollution arising from tumble drying. PLoS One 17:e0265912. doi: 10.1371/journal.pone.0265912
Lant, N. J., Hayward, A. S., Peththawadu, M. M., Sheridan, K. J., and Dean, J. R. (2020). Microfiber release from real soiled consumer laundry and the impact of fabric care products and washing conditions. PLoS One 15:e0233332. doi: 10.1371/journal.pone.0233332
Li, Y., Zhang, Y., Chen, G., Xu, K., Gong, H., Huang, K., et al. (2021). Microplastics in surface waters and sediments from Guangdong coastal areas. Sustainability 13:2691. doi: 10.3390/su13052691
Liu, J., Yang, Y., Ding, J., Zhu, B., and Gao, W. (2019). Microfibers: a preliminary discussion on their definition and sources. Environ. Sci. Pollut. Res. Int. 26, 29497–29501. doi: 10.1007/s11356-019-06265-w
Migwi, F. K., Ogunah, J. A., and Kiratu, J. M. (2020). Occurrence and spatial distribution of microplastics in the surface waters of Lake Naivasha, Kenya. Environ. Toxicol. Chem. 39, 765–774. doi: 10.1002/etc.4677
Miller, R. Z., Watts, A. J. R., Winslow, B. O., Galloway, T. S., and Barrows, A. P. W. (2017). Mountains to the sea: river study of plastic and non-plastic microfiber pollution in the Northeast USA. Mar. Pollut. Bull. 124, 245–251. doi: 10.1016/j.marpolbul.2017.07.028
Mizraji, R., Ahrendt, C., Perez-Venegas, D., Vargas, J., Pulgar, J., Aldana, M., et al. (2017). Is the feeding type related with the content of microplastics in intertidal fish gut? Mar. Pollut. Bull. 116, 498–500. doi: 10.1016/j.marpolbul.2017.01.008
Moore, J. E., Jackson, G., and Firth, M. (1986). Movement of fibres between working areas as a result of routine examination of garments. J. For. Sci. Soc. 26, 433–440. doi: 10.1016/S0015-7368(86)72534-6
Muniyasamy, S., and Patnaik, A. (2021). Biodegradable behavior of waste wool and their recycled polyester preforms in aqueous and soil conditions. J. Renew. Mater. 9, 1661–1671. doi: 10.32604/jrm.2021.014904
Nel, H. A., and Froneman, P. W. (2015). A quantitative analysis of microplastic pollution along the south-eastern coastline of South Africa. Mar. Pollut. Bull. 101, 274–279. doi: 10.1016/j.marpolbul.2015.09.043
Nel, H. A., Hean, J. W., Noundou, X. S., and Froneman, P. W. (2017). Do microplastic loads reflect the population demographics along the southern African coastline? Mar. Pollut. Bull. 115, 115–119. doi: 10.1016/j.marpolbul.2016.11.056
Ory, N. C., Sobral, P., Ferreira, J. L., and Thiel, M. (2017). Amberstripe scad Decapterus muroadsi (Carangidae) fish ingest blue microplastics resembling their copepod prey along the coast of Rapa Nui (Easter Island) in the South Pacific subtropical gyre. Sci. Total Environ. 586, 430–437. doi: 10.1016/j.scitotenv.2017.01.175
Palmer, R., and Burch, H. J. (2009). The population, transfer and persistence of fibres on the skin of living subjects. Sci. Justice 49, 259–264. doi: 10.1016/j.scijus.2009.02.008
Palmer, R., Burnett, E., Luff, N., Wagner, C., Stinga, G., Carney, C., et al. (2015). The prevalence of two ‘commonly’ encountered synthetic target fibres within a large urban environment. Sci. Justice 55, 103–106. doi: 10.1016/j.scijus.2015.01.001
Palmer, R., and Oliver, S. (2004). The population of coloured fibres in human head hair. Sci. Justice 44, 83–88. doi: 10.1016/S1355-0306(04)71693-7
Pirc, U., Vidmar, M., Mozer, A., and Kržan, A. (2016). Emissions of microplastic fibers from microfiber fleece during domestic washing. Environ. Sci. Pollut. Res. 23, 22206–22211. doi: 10.1007/s11356-016-7703-0
Pounds, C. A., and Smalldon, K. W. (1975). The transfer of fibres between clothing materials during simulated contacts and their persistence during wear. Part III–fibre persistence. J. Forensic Sci. Soc. 15, 29–37. doi: 10.1016/S0015-7368(75)70933-7
Rambonnet, L., Vink, S. C., Land-Zandstra, A. M., and Bosker, T. (2019). Making citizen science count: best practices and challenges of citizen science projects on plastics in aquatic environments. Mar. Pollut. Bull. 145, 271–277. doi: 10.1016/j.marpolbul.2019.05.056
Rebelein, A., Int-Veen, I., Kammann, U., and Scharsack, J. P. (2021). Microplastic fibers — underestimated threat to aquatic organisms? Sci. Total Environ. 777:146045. doi: 10.1016/j.scitotenv.2021.146045
Robertson, J., Roux, C., and Wiggins, K. G. (2017). Forensic Examination of Fibres: Third Edition. Boca Raton, FL: Taylor and Francis
Ryan, P. G., Suaria, G., Perold, V., Pierucci, A., Bornman, T. G., and Aliani, S. (2020). Sampling microfibres at the sea surface: the effects of mesh size, sample volume and water depth. Environ. Pollut. 258:113413. doi: 10.1016/j.envpol.2019.113413
Sanad, R. A. (2018). Gender-specific colours of textile and clothing products throughout history. Int. J. 6, 67–77. doi: 10.15640/ijaah.v6n1a8
Sheridan, K. J., Saltupyte, E., Palmer, R., and Gallidabino, M. D. (2020). A study on contactless airborne transfer of textile fibres between different garments in small compact semi-enclosed spaces. Forensic Sci. Int. 315:110432. doi: 10.1016/j.forsciint.2020.110432
Sidhu, N., Qualter, C., Higgs, E., and Guo, K. (2021). What colour should I wear? How clothing colour affects women's judgement of other women's body attractiveness and body size. Acta Psychol. 218:103338. doi: 10.1016/j.actpsy.2021.103338
Skokan, L., Tremblay, A., and Muehlethaler, C. (2020). Differential shedding: a study of the fiber transfer mechanisms of blended cotton and polyester textiles. Forensic Sci. Int. 308:110181. doi: 10.1016/j.forsciint.2020.110181
Stanton, T., Johnson, M., Nathanail, P., Macnaughtan, W., and Gomes, R. L. (2019). Freshwater and airborne textile fibre populations are dominated by ‘natural’, not microplastic, fibres. Sci. Total Environ. 666, 377–389. doi: 10.1016/j.scitotenv.2019.02.278
Suaria, G., Achtypi, A., Perold, V., Lee, J. R., Pierucci, A., Bornman, T. G., et al. (2020). Microfibers in oceanic surface waters: a global characterisation. Sci. Adv. 6:eaay8493. doi: 10.1126/sciadv.aay8493
Textile Exchange (2021). Preferred Fiber and Materials Market Report 2021. Available at: https://textileexchange.org/app/uploads/2021/08/Textile-Exchange_Preferred-Fiber-and-Materials-Market-Report_2021.pdf
The Flipflopi Project. (2019). Expeditions. Available at: https://www.theflipflopi.com/expeditions ().
Torre, M., Digka, N., Anastasopoulou, A., Tsangaris, C., and Mytilineou, C. (2016). Anthropogenic microfibres pollution in marine biota. A new and simple methodology to minimize airborne contamination. Mar. Pollut. Bull. 113, 55–61. doi: 10.1016/j.marpolbul.2016.07.050
Wang, X., Li, C., Liu, K., Zhu, L., Song, Z., and Li, D. (2020). Atmospheric microplastic over the South China Sea and East Indian Ocean: abundance, distribution and source. J. Hazard. Mater. 389:121846. doi: 10.1016/j.jhazmat.2019.121846
Woodall, L. C., Gwinnett, C., Packer, M., Thompson, R. C., Robinson, L. F., and Paterson, G. L. J. (2015). Using a forensic science approach to minimize environmental contamination and to identify microfibres in marine sediments. Mar. Pollut. Bull. 95, 40–46. doi: 10.1016/j.marpolbul.2015.04.044
Woodall, L. C., Sanchez-Vidal, A., Canals, M., Paterson, G. L., Coppock, R., Sleight, V., et al. (2014). The deep sea is a major sink for microplastic debris. R. Soc. Open Sci. 1:140317. doi: 10.1098/rsos.140317
Zambrano, M. C., Pawlak, J. J., Daystar, J., Ankeny, M., and Venditti, R. A. (2021). Impact of dyes and finishes on the aquatic biodegradability of cotton textile fibers and microfibers released on laundering clothes: correlations between enzyme adsorption and activity and biodegradation rates. Mar. Pollut. Bull. 165:112030. doi: 10.1016/j.marpolbul.2021.112030
Keywords: microplastics, plastic pollution, forensic science, microfibres, citizen science, Africa, natural fibres and biodegradability
Citation: KeChi-Okafor C, Khan FR, Al-Naimi U, Béguerie V, Bowen L, Gallidabino MD, Scott-Harden S and Sheridan KJ (2023) Prevalence and characterisation of microfibres along the Kenyan and Tanzanian coast. Front. Ecol. Evol. 11:1020919. doi: 10.3389/fevo.2023.1020919
Received: 16 August 2022; Accepted: 17 January 2023;
Published: 23 February 2023.
Edited by:
David Ryves, Loughborough University, United KingdomReviewed by:
Agnes Muthumbi, University of Nairobi, KenyaCopyright © 2023 KeChi-Okafor, Khan, Al-Naimi, Béguerie, Bowen, Gallidabino, Scott-Harden and Sheridan. This is an open-access article distributed under the terms of the Creative Commons Attribution License (CC BY). The use, distribution or reproduction in other forums is permitted, provided the original author(s) and the copyright owner(s) are credited and that the original publication in this journal is cited, in accordance with accepted academic practice. No use, distribution or reproduction is permitted which does not comply with these terms.
*Correspondence: Kelly J. Sheridan, ✉ a2VsbHkuc2hlcmlkYW5Abm9ydGh1bWJyaWEuYWMudWs=; Simon Scott-Harden, ✉ c2ltb24uc2NvdHQtaGFyZGVuQG5vcnRodW1icmlhLmFjLnVr
†These authors have contributed equally to this work and share last authorship
Disclaimer: All claims expressed in this article are solely those of the authors and do not necessarily represent those of their affiliated organizations, or those of the publisher, the editors and the reviewers. Any product that may be evaluated in this article or claim that may be made by its manufacturer is not guaranteed or endorsed by the publisher.
Research integrity at Frontiers
Learn more about the work of our research integrity team to safeguard the quality of each article we publish.