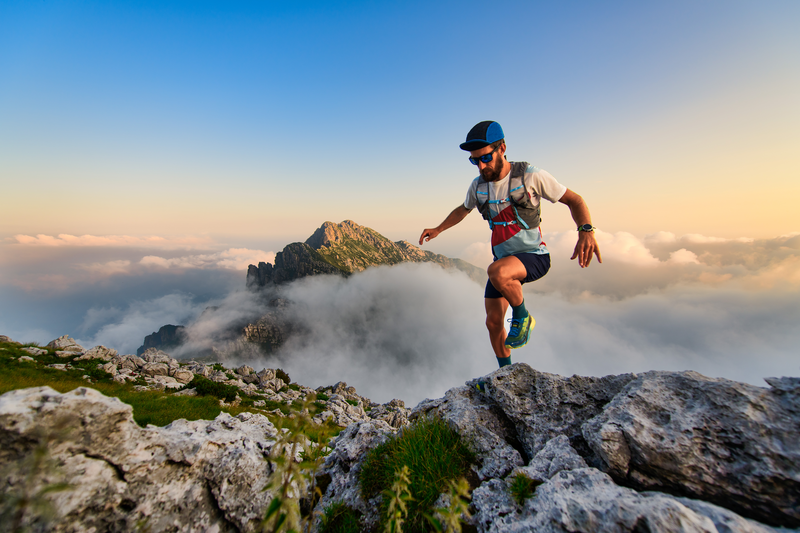
94% of researchers rate our articles as excellent or good
Learn more about the work of our research integrity team to safeguard the quality of each article we publish.
Find out more
ORIGINAL RESEARCH article
Front. Ecol. Evol. , 07 February 2023
Sec. Conservation and Restoration Ecology
Volume 11 - 2023 | https://doi.org/10.3389/fevo.2023.1007743
This article is part of the Research Topic Amphibian and Reptile Road Ecology View all 12 articles
Roadways are among the most widespread and disruptive anthropogenic land use features that influence the behavior and movement of wildlife. Negative impacts of roadways have been well documented, but the behavioral impact of roadways on smaller, cryptic species has yet to be thoroughly examined. Using a novel integration of radio telemetry and tri-axial accelerometry, we evaluated the effects of roadways on the movement behavior of 26 adult Timber Rattlesnakes (Crotalus horridus) at coarse and fine time scales in central Georgia between June 2020 and November 2021. To interpret the effect of roads at a coarse time scale, we modeled the effect of mean annual distance to roadways (DTR) on annual measures of movement and space use by C. horridus using both radio telemetry and accelerometry derived metrics (RT and ACT metrics). To explore the fine-scale impact of roadways, we quantified RT and ACT metrics during confirmed road interactions (i.e., instances when individual snakes crossed a road or encountered a road but did not cross) and compared these instances to the RT and ACT metrics calculated across the remainder of the active season within this subset of snakes. Relating the annual RT and ACT metrics to DTR revealed no significant associations at a coarse time scale. However, the evaluation of C. horridus movement behavior during punctuated road encounters revealed that snakes increased RT and ACT metrics during the road interactions compared to metrics calculated across the remainder of the active season. This might indicate that the abundance of contiguous habitat adjacent to roadways at our study site is serving as an adequate buffer to any long-term shifts in movement behavior, but the potential hidden cost of increasing movement when snakes encounter roads could have negative implications for populations that encounter roads more frequently, even in the absence of significant direct road mortality. Overall, integrating radio telemetry and accelerometry and adopting a scale-dependent approach to quantifying movement allowed for a more detailed evaluation of the response of C. horridus to roadways. This approach holds promise for detecting and interpreting previously overlooked short-term alterations in snake movement behavior with potentially significant fitness consequences.
In an increasingly human-modified world, it is essential to understand how organisms respond to anthropogenic activities and disturbances. Among the multitude of human effects on natural landscapes, the historic and ongoing construction of roadways is viewed as a leading disruptor of wildlife behavior and ecology globally (Oxley et al., 1974; Lovallo and Anderson, 1996; Trombulak and Frissell, 2000; Underhill and Angold, 2000; Spellerberg, 2002; Forman et al., 2003; Jaeger et al., 2005; Reynolds-Hogland and Mitchell, 2007; Fahrig and Rytwinski, 2009). Roads negatively impact wildlife most directly through vehicle-induced mortality (Ashley and Robinson, 1996; Shepard et al., 2008a; Quintero-Ángel et al., 2012; Rytwinski and Fahrig, 2012), but sub-lethal effects of roads have also been well documented. For example, by fragmenting habitat and acting as potential barriers to movement, roadways can limit gene flow and reduce population viability (Lodé, 2000; Keller and Largiader, 2003; Shine et al., 2004; Shepard et al., 2008b; Clark et al., 2010; Holderegger and Di Giulio, 2010). Additionally, the increased human presence, vehicular traffic, and noise associated with roads can lead to maladaptive behaviors and reduced fitness for individuals (Ware et al., 2015; Ng et al., 2019; Zhou et al., 2020). The extensive edge habitat associated with roadways can also attract some wildlife, creating a classic ecological trap for those species drawn to edges for foraging, nesting, or thermoregulating (Cowardin et al., 1985; Aresco, 2005; Mata et al., 2017). Ultimately, the alteration of behavior is at the core of wildlife-road interactions (Lomas et al., 2019), with variations in patterns of animal movement and space use in relation to roadways being a strong indicator of the relative risk that these features pose to individual species and populations.
Animals move through their environments in efforts to locate and acquire critical resources such as food, water, and mating partners, while minimizing potentially harmful encounters with predators, competitors, or unfavorable environmental conditions (Nathan et al., 2008). Accordingly, disrupting an animal’s movement behavior can have significant fitness effects. However, numerous intrinsic and extrinsic factors simultaneously influence movement decisions, making it difficult to pinpoint the mechanistic roles of each individual driver. Among these, an individual’s sex, age, and motivational state (e.g., behavioral season) have all been demonstrated to influence movement patterns, and, consequently, are important considerations when exploring the effects of individual extrinsic factors, such as roadways, on movement patterns (Waldron et al., 2006; Petersen et al., 2019). Unsurprisingly, species-specific behavioral responses to roadways can vary tremendously, ranging from avoidance (Thurber et al., 1994; Rondinini and Doncaster, 2002; Andrews et al., 2008; McGregor et al., 2008; Hibbitts et al., 2017) to strong attractance (Mumme et al., 2000; Aresco, 2005). For predators, the increase in concentrations of prey adjacent to roads has been suggested to attract some species and alter their patterns of space use (Barrientos and Bolonio, 2009; Leblond et al., 2013; Ruiz-Capillas et al., 2013). Road surface, road width, and traffic volume are also important factors that can produce variation within and between species (Ware et al., 2015; Hibbitts et al., 2017; Ng et al., 2019). Even further, the spatial and temporal scale at which movement is measured can have pronounced impacts on inferred responses, especially if an individual’s interactions with roadways are infrequent, which might be the case for those species that tend to avoid roads (Shepard et al., 2008b; Hibbitts et al., 2017). Clearly, careful selection of study organisms and suitable methods for quantifying movement is required for a detailed and accurate understanding of species-specific responses.
Despite the aforementioned importance of evaluating the relationships between roads, intrinsic variables, and movement behavior across a diversity of wildlife, such explorations are limited by the available methods for monitoring movement and space use in nature. For many small-bodied and secretive taxa, such as snakes, these constraints are amplified, as collecting movement data across a large sample of individuals and over meaningful time frames has been historically challenging or impossible. As such, manipulative experiments and predictive simulations with longitudinal datasets have represented important tools for evaluating the impact of roads on snake movement behavior. From the few manipulative studies conducted, researchers found that road crossing propensity differed between snake taxa with smaller bodied species exhibiting higher road avoidance than larger species (Andrews and Gibbons, 2005). They also found that pit vipers (Crotalinae) crossed roads more slowly compared to other species (Colubridae) (Andrews and Gibbons, 2005). Garter Snakes (Thamnophis sirtalis parietalis) also displayed avoidance behavior when placed along roads, and the ability of mate-searching males to follow female pheromone trails decreased across the road surface compared to surrounding habitat (Shine et al., 2004). Additionally, road crossing probabilities of Brown Treesnakes (Boiga irregularis) were found to decrease with increasing road magnitude but increased with increasing body size and humidity (Siers et al., 2016). Road avoidance behavior was also revealed through predictive simulations with longitudinal datasets. Eastern Massasauga Rattlesnakes (Sistrurus catenatus), Eastern Hognose Snakes (Heterodon platyrhinos) and Brown Treesnakes (Boiga irregularis) were found to cross roads significantly less frequently than expected (Shepard et al., 2008b; Robson and Blouin-Demers, 2013; Siers et al., 2014), and further, H. platyrhinos avoided crossing paved but not unpaved roads (Robson and Blouin-Demers, 2013). Although both the manipulative studies and predictive simulations report informative findings on the movement response of snakes to roadways, considerable paucity remains in our understanding of how individual species respond behaviorally to roads in a natural setting and how these responses might vary relative to key factors such as an individual’s sex or motivational state, or the spatial and temporal scales considered.
Historically, using radio telemetry to monitor a subset of individuals and estimating the total extent of space used (i.e., home range size) has represented the best approach for quantifying the long-term behavioral responses of snakes to roadways and other prominent landscape features (Clark et al., 2010; Kapfer et al., 2010; Row et al., 2012; Ettling et al., 2013; Anguiano and Diffendorfer, 2015). However, the estimation of an animal’s home range size and movement distances largely ignores the fine-scale temporal dimensions of animal movement, as individuals can potentially alter the frequency and duration of movements independent of spatial shifts. The recent explosion of biologging technologies has afforded researchers with an expanded toolkit for measuring the movement behavior of organisms in the field. Among these, accelerometer dataloggers are becoming increasingly popular in field studies of wildlife behavior (Brown et al., 2013; Wilmers et al., 2015). When paired with advanced machine learning techniques, accurate and automated classification of discrete behaviors can be achieved using animal-borne accelerometer data. However, until very recently, accelerometers and other biologger applications were mostly restricted to larger terrestrial mammals, large birds, and aquatic vertebrates (Brown et al., 2013). The validation of accelerometer monitoring of movement behavior in rattlesnakes has now set the stage for applied extensions of these techniques in hypothesis-testing frameworks (DeSantis et al., 2020). Herein, we report on an integration of radio telemetry and accelerometry allowing for the evaluation of movement responses by Timber Rattlesnakes (Crotalus horridus) to roadways in the Piedmont ecoregion of Georgia, USA, where remaining C. horridus populations are suspected to be in decline (Jenkins et al., 2021). We aimed to evaluate the influence of roads on the spatial movement patterns and daily movement durations of C. horridus at both a coarse time scale (annual and seasonal) and fine time scale (during isolated road interactions) while also considering the potential effects of sex and behavioral season (non-mating, mating) in these relationships. Preliminary observations of telemetered C. horridus seemingly decreasing movement in close proximity to roadways suggested that roadways may be acting as a semipermeable barrier to movement. As such, we hypothesized that snakes that are, on average, closer to roads will constrict their movements and thus exhibit smaller home range and movement estimates. Further, we hypothesized that snakes would decrease movement during isolated road encounters compared to time periods in which they were not encountering roads.
The field study site is located in Putnam County, Georgia, within the Cedar Creek Wildlife Management Area (CCWMA) and Oconee National Forest (ONF) (centered on N 33°14′16.33” W 83°30′48.24″). The ONF is mixed use, with a combination of public and private land. The habitat is characteristic of the Piedmont ecoregion in Georgia, consisting mainly of upland Loblolly Pine (Pinus taeda) and riparian hardwood forest and a mixture of the two where they adjoin. A key feature of the study site is the high levels of habitat heterogeneity therein, with clear-cut fields, uneven stand ages, residential properties, and an abundance of paved and unpaved roads all present within the study area. The paved roads present at the site are moderately trafficked and are eight meters wide. Hillsboro Road and Stanfordville Road, the paved roads that somewhat serve as the northern and eastern borders of our study area, respectively, have an approximate traffic volume of 122 vehicles per day (Georgia Department of Transportation, 2022). Highway 212 largely acts as the southern border of our study site and has a traffic volume of approximately 1,226 vehicles per day (Georgia Department of Transportation, 2022). The unpaved, dirt and gravel roads at the site vary between approximately six and eight meters wide. These roads are low-use with estimated traffic volumes not typically exceeding 20 vehicles per day (personal observation). The traffic volumes likely increase during popular hunting seasons (late fall to winter/early spring), but these times do not substantially overlap with the snakes’ active season.
Timber Rattlesnakes (C. horridus) are heavy-bodied pit vipers historically distributed throughout eastern North America (Martin et al., 2008). They are forest specialists and ambush predators, with rodents and lagomorphs making up the majority of their diet (Martin et al., 2021). As a result of local environmental pressures and the genetic distance between populations (Bushar et al., 1998), considerable size variation is noted throughout the range of C. horridus, but male-biased sexual size dimorphism is ubiquitous (Stengle et al., 2021). Although locally abundant at our study site, C. horridus have a patchy distribution throughout the Piedmont ecoregion of Georgia (Jenkins et al., 2021). Much of what is known of C. horridus ecology and behavior is from studies conducted on populations in the northern portion of their distribution, with a relative dearth of research from the southern regions. Populations in the north have seen declines due to road mortality, reduced gene flow, and disease, which are likely threats to populations in the south, as well (Petersen and Sealy, 2021). Life history traits of C. horridus (i.e., long lived, long gestation periods, habitat specialists) make them especially vulnerable to the lethal and sub-lethal effects of roads (Fahrig, 2007), so much that roads are identified as one of the leading threats to range-wide population viability (Petersen and Sealy, 2021).
Between June 2020 and November 2021, we employed radio telemetry and accelerometry monitoring of 26 adult Timber Rattlesnakes (RT – Female: N = 14, Male: N = 12; ACT – Female: N = 10, Male: N = 8; Tipton, 2022). One female was radiotracked during the study period but was not included in statistical analyses due to being confirmed as gravid. One male was lost as a result of suspected road mortality or transmitter failure as the snake remained within close proximity of a roadway for a month (8 relocations) before we lost the signal. This male only contributed data to our RT spatial analyses as the ACT was not retrieved. Additional snakes that were equipped with ACTs contributed to the RT analyses but not the ACT analyses due to ACT malfunctions (two females, one male). One additional male was lost to suspected transmitter failure, and another male was lost to suspected predation shortly after monitoring began; neither were included in RT or ACT analyses.
Radio transmitters (Holohil Systems Ltd., Model SB-2 T) and ACTs (Technosmart Europe srl., AXY-5) were coupled, internally implanted (Reinert and Cundall, 1982), and securely sutured to a rib (Hardy and Greene, 1999, 2000) in the same position and orientation for all individuals. Implants (RT = 5 g; ACT = 10 g) comprised ≤3% of each individual’s body mass at the time of implantation (Table 1). RTs had a battery life of 10 months, and ACTs had 1 Gb of storage capacity allowing for approximately 10 months of continuous, low frequency (1 Hz) recording. Rattlesnakes were released at the original site of the capture within 3 days of implantation of the RT-ACT device and were relocated every 3–4 days during the active season (March–November) and biweekly during the inactive season (December–February) to collect RT derived spatial movement data. Detailed behavioral observations were recorded during relocations along with environmental conditions, habitat type, and geographic coordinates with a hand-held GPS (Garmin Oregon 700, accuracy ≤5 m).
Table 1. Snake ID, sex, Snout-Vent Length (SVL), weight, RT monitoring durations, and ACT monitoring durations for all Crotalus horridus monitored.
We applied the ACT data processing protocols developed and validated for rattlesnakes (C. atrox) by DeSantis et al. (2020) to our remotely collected ACT data in C. horridus. During each RT data collection trip (every 3–4 days), rattlesnakes were observed for visual field validation of ACT signals corresponding to our two broad behavioral categories, “immobile” and “moving” (Le Roux et al., 2017; Barwick et al., 2018; DeSantis et al., 2020). The behavioral category “moving” was characterized by outstretched, full body motion, while “immobile” was defined as a lack of full body motion regardless of posture. Detailed and timestamped notes along with video recordings were taken during observations of ACT-equipped individuals in the field. These notes and videos were used as a reference during the manual annotation of behaviors within ACT datasets. These time-matched observations of behavior in the field represented the training and testing data for the ensemble learning model.
A total of 40 descriptive statistics were computed for the magnitude of acceleration, defined as , and across the x (surge; forward and backward motion), y (sway; side-to-side motion), and z (heave; upward and downward motion) ACT axes. These descriptive statistics were calculated to summarize acceleration data and identify relationships between ACT signals and behavioral category. The following features were computed for overall dynamic acceleration and acceleration along each individual axis (): mean, standard deviation, interquartile range, maximum, minimum, mean of the difference between consecutive points, standard deviation of the difference between consecutive points, interquartile range of the difference between consecutive points, maximum of the difference between consecutive points, and minimum of the difference between consecutive points. These summary stats were initially calculated to be “rolling” over varying temporal window sizes (4, 5, 10, 30, and 60-s period basis) to identify the optimal scale at which to classify activity with machine learning models. Summary statistics were ranked using ReliefF (Farzaneh, 2022) feature selection for the classification of immobile and moving behaviors.
An AdaBoost ensemble learning algorithm (Rokach, 2010) was implemented using the fitcensemble function in MATLAB (2019). The AdaBoost algorithm learner was set to have a maximum number of splits of 512 and a minimum leaf size of five. Classification performance was evaluated using a fivefold cross validation technique (Kononenko, 1994). The initial dataset was split into five subsets of equal size and five iterations were performed. Within this technique at each iteration, four of the subsets were used to train the algorithm and the remaining one was used as a test set. This process was repeated five times, each time changing the training and test subsets until all subsets have been used as a test. Performance values are computed for the test set across each iteration. Following model training and testing, the validated model was applied to the full field-collected datasets for automated behavioral classification. Using these behavioral predictions, continuous activity budgets (time classified as moving vs. immobile) were produced enabling quantification of real-time movement durations across various time scales (daily, weekly, monthly) for each individual.
Spatial aspects of rattlesnake movement were quantified for each individual within the non-mating (March–July) and mating (August–November) seasons using the following radio telemetry derived metrics: Meters Per Day (MPD), calculated as the sum of the straight-line distances between successive relocation points for an individual divided by the total number of days monitored, Distance Per Movement (DPM), calculated as the mean straight-line distance between relocation points that were ≥ 5 m apart, Minimum Movement Frequency (MMF), calculated as the number of movements (≥5 m) made by an individual out of N relocations in a specifically defined time period, and Motion Variance (MV) extracted from individual dynamic Brownian Bridge Motion Models. Motion Variance estimated the variance among displacement distances within a moving window of nine radio telemetry relocations (i.e., 1 month increments of spatial data). Higher MV values indicate more variable or abnormal movement relative to the sampling duration considered (Kranstauber et al., 2012; Silva et al., 2018). Annual and seasonal home range measurements were calculated for each individual with commonly used home range estimators, including the 100% Minimum Convex Polygon (MCP) (Row and Blouin-Demers, 2006) and 95 and 50% fixed-kernel Utilization Distributions (UD) with the plug-in bandwidth matrix (Bauder et al., 2015, 2016). The plug-in bandwidth matrix for the UD home ranges was chosen because it has been demonstrated to be robust to variation in sampling rate and duration (Bauder et al., 2015). RT movement metrics and home range sizes were calculated in R using the adehabitat, adehabitatHR, and adehabitatLT packages (R Core Team, 2022).
Temporal aspects of rattlesnake movement were quantified for each individual annually as well as within the non-mating and mating seasons. Accelerometry derived movement metrics included mean time spent moving per 24 h (Mov24) and mean number of movement bouts (periods of movement ≥60-s) per 24 h (Bout24). In order to evaluate the effects of roadways on RT and ACT movement metrics, ArcGIS was used to calculate the Euclidean distance (m) to the nearest roadway for each relocation point. The mean distance to roadway (DTR) for each individual was quantified both annually and within the non-mating and mating season.
Linear Mixed Effects (LME) models were employed to test for the effects of sex, behavioral season, and DTR on movement and space use patterns. Response variables (RT and ACT movement metrics and home range estimations) were modeled with separate LME models to evaluate significant effects and interactions. Rattlesnake ID was modeled as a random effect to control for non-independence of data across time. Fixed effects included sex (male, female), behavioral season (non-mating, mating), and DTR. Our RT response variables included MPD, DPM, MMF, MV, 100% MCP, 95% UD, and 50% UD and the ACT response variables included Mov24 and Bout24. Appropriate transformations for each response variable were determined and employed in the model. This resulted in the following set of response variable transformations: MPD (log base 10), DPM (log base 10), 100% MCP (log base 10), 95% UD (log base 10), 50% UD (square root), Mov24 (log base 10). Model fit was evaluated with marginal and conditional pseudo-R2 measures. Marginal pseudo-R2 describes the proportion of model variance attributed to the fixed effects, while conditional pseudo-R2 describes the proportion of model variance attributed to both fixed and random effects. The emmeans package in R (Lenth, 2022) was used for post hoc evaluation of relationships between variables when significant main effects were detected in the LME models.
Along with evaluating the effect of roads on coarser scale measures of movement (annual and seasonal RT and ACT metrics), we also evaluated the movement response of snakes to roadways during confirmed road encounters. We investigated this direct movement response of C. horridus to roads by identifying snakes within our sample with confirmed road interactions during the monitoring period (i.e., instances when individual snakes either crossed a road or encountered a road but did not cross and retreated). To evaluate whether the movement behavior of these specific individuals differed during these brief events relative to periods when they were not encountering roadways, we calculated RT movement metrics (MPD, DPM) and ACT movement metrics (Mov24, Bout24) across the four relocations (2 weeks) preceding each road encounter for comparison with the same metrics calculated from all remaining relocations within the datasets for this subset of individuals. We opted for a fixed temporal window for the fine-scale movement analysis to standardize the amount of data contributed across individuals. We selected four relocations, or 2 weeks for ACT data, as it represented the mean and median value for the number of relocations (range: 1–9 consecutive relocations within 25 m of roadway) associated with each of the 17 road encounters observed during the monitoring period. These RT and ACT metrics were then pooled across all snakes that interacted with roads into their respective categories (road interaction and no road interaction) for analysis. Non-parametric Mann–Whitney U tests were conducted to compare the mean RT and ACT movement metrics (MPD, DPM; Mov24, Bout24) between the road interaction and no road interaction categories.
Individual rattlesnakes were radiotracked for durations ranging from 35 to 503 days (mean ± SD = 237 ± 169) between June 2020 and November 2021 for a cumulative total of 1,437 telemetry relocations (mean ± SD = 60 ± 40; Table 1). Male (N = 14) and female (N = 17) annual movement and space use measures were calculated and condensed into behavioral season (non-mating, mating) for analyses. In total, 47 RT snake-season data points (17 non-mating, 30 mating) were accumulated. The mean annual MPD (±SD) for males was 31.5 ± 15.9 and 18.7 ± 7.00 m for females. The mean annual DPM for males was 123.1 ± 58.6 and 76.0 ± 24.0 m for females. Mean annual MMF for males was 0.79 ± 0.12 and 0.81 ± 0.11 for females. The mean annual MV for males was 2.62 ± 2.39 and 0.92 ± 0.75 for females. Mean annual 95% UD for males was 44.0 ± 33.0 ha and 23.5 ± 15.2 ha for females. The mean annual 50% UD for males was 6.55 ± 6.4 ha and 3.59 ± 3.43 ha for females. Mean annual 100% MCP for males was 28.2 ± 25.4 and 13.5 ± 10.1 for females (Figure 1).
Figure 1. Annual minimum, median, mean (white stars), and maximum values, and the interquartile ranges of male and female Crotalus horridus RT and ACT metrics. RT derived movement measures (A–D) include Motion Variance (MV), Distance Per Movement (DPM), Meters Per Day (MPD), and Minimum Movement Frequency (MMF). RT derived space use measures (E–G) include 100% Minimum Convex Polygons home range (MCP), 95% fixed kernel Utilization Distribution home range (95% UD) and 50% fixed kernel Utilization Distribution core use area (50% UD). ACT derived movement measures (H,I) include the mean time spent moving per 24 h (Mov24) and the mean number of movement bouts per 24 h (Bout24). Asterisks denote significant differences (p < 0.05).
Individual rattlesnakes were monitored via accelerometry for durations ranging from 57 to 450 days (mean ± SD = 233 ± 130) for a total of 2,791 recording days between June 2020 and November 2021 (Table 1). Male (N = 4) and female (N = 7) annual movement durations were calculated and condensed into behavioral season (non-mating, mating) for analyses. In total, 25 ACT snake-season data points (12 non-mating, 13 mating) were accumulated. The mean annual Mov24 (±SD) for males was 0.78 ± 0.35 h and 0.70 ± 0.27 h for females. Mean annual Bout24 for males was 11.2 ± 3.2 bouts and 10.2 ± 2.9 bouts for females.
The optimal window size for classifying immobile and moving behaviors was determined to be 60 s. The initial validation dataset contained a total of 828 60-s (13.80 h) observations of moving and immobile behaviors. Six hundred and sixty of the 60 s observations were of immobile behavior (11 h) and 168 of the 60 s observations were of moving behavior (2.80 h). At a 60 s window size, the model accurately classified 98.79% of both immobile and moving behaviors. The model classified the immobile behaviors with a precision of 98.95%, a recall of 99.55%, an F-score of 99.24%, and a specificity of 95.83%. The model classified moving behavior within the field datasets with a precision of 98.17%, a recall of 95.83%, an F-score of 96.99%, and a specificity of 99.55%.
Preliminary LME models included sex, season, and distance to road (DTR) as fixed effects, and our spatial response variables included MPD, DPM, MMF, MV, 100% MCP, 95% UD, and 50% UD. Limited within-season sample sizes resulted in a failure to converge across most models when including snake ID as a random effect. As a result, we calculated annual RT metrics, and the final spatial models include only sex and DTR as fixed effects.
In the annual LME models with RT response variables, there was a significant main effect of sex on MPD [Effect Size (ES) = 0.83 ± 0.39 SE, p = 0.04; Table 2] detected, with males moving more per day than females. Post hoc estimated marginal means pairwise comparisons also detected a significant difference in mean annual MPD between males and females (t18.4 = −2.467, p = 0.0237). There was no significant effect of DTR on MPD. The marginal and conditional pseudo-R2 measures of fit were 0.24 and 0.44 (Table 3). A significant main effect of sex was detected on DPM (ES = 0.74 ± 0.33 SE, p = 0.04), with males moving further per movement compared to monitored females. Post hoc estimated marginal means pairwise comparisons also detected a significant difference in mean annual DPM between males and females (t18.8 = −2.694, p = 0.0144). There was no main effect of DTR on DPM. The marginal and conditional pseudo-R2 measures of fit were 0.27 and 0.52. No significant main effect of sex or DTR was detected on MMF. The marginal and conditional pseudo-R2 measures of fit were 0.003 and 0.46. A significant main effect of sex was detected on MV (ES = 2.33 ± 0.82 SE, p = 0.009), with males having a higher measure of MV compared to monitored females. Post hoc estimated marginal means pairwise comparisons also detected a significant difference in mean annual MV between males and females (t17.6 = −2.613, p = 0.0178). There was no main effect of DTR on MV. The marginal and conditional pseudo-R2 measures of fit were 0.29 and 0.39. There was no significant main effect of sex or DTR on 100% MCP. The marginal and conditional pseudo-R2 measures of fit were 0.12 and 0.51. A significant main effect of sex was detected on 95% UD (ES = 1.58 ± 0.69 SE, p = 0.03) with male home range sizes being larger in comparison to females, but the estimated marginal means post hoc pairwise comparison of male and female 95% UD did not detect a significant difference (t18.7 = −1.561, p = 0.1352). The marginal and conditional pseudo-R2 measures of fit were 0.19 and 0.46. No significant main effect of sex or DTR was detected on 50% UD (Table 2). The marginal and conditional pseudo-R2 measures of fit were 0.11 and 0.28 (Table 3).
Table 2. Coefficients, standard error, and p-values for individual RT derived annual movement and space use model parameters.
Limited within-season and within-sex sample sizes resulted in a failure to converge across our ACT movement models. As a result, we calculated annual ACT movement metrics (Mov24 and Bout24) for our full sample of ACT-monitored snakes (males and females pooled) in order to evaluate the relationship between DTR and ACT derived movement durations. In the annual LME models with ACT response variables, there was no significant main effect of DTR detected on Mov24 or Bout24 for this pooled sample (Table 4). The marginal and conditional pseudo-R2 measures of fit for the Mov24 and Bout24 models were 0.0003 and 0.53 and 0.016 and 0.44, respectively (Table 5).
Table 4. Coefficients, standard error, and p-values for ACT derived annual movement model parameters.
Out of our full RT and ACT monitored sample, nine individuals (RT: five females, four males; ACT: three females, two males) were observed interacting with roads by either a confirmed crossing event or approaching within 25 m of a road and retreating. There were a total of 17 confirmed road interactions resulting in 15 road crossings and two instances of a snake approaching a road and retreating. The majority of confirmed road encounters resulted in a crossing event (88%).
There was a significant difference detected between the mean Mov24 for the two categories, road interaction and no road interaction (W = 23,516, r = 0.10, p = 0.007), with Mov24 (mean ± SD = 1.04 ± 1.6) during the road interactions being significantly higher than Mov24 (mean ± SD = 0.65 ± 0.98) when snakes were not interacting with a road (Table 6 and Figure 2). There was also a significant difference detected between the two categories for MPD (W = 6,313, r = 0.14, p = 0.007), with MPD during the road interactions (mean ± SD = 83.0 ± 71.8) being significantly higher than MPD when snakes were not interacting with a road (mean ± SD = 67.3 ± 95.7). No significant difference was detected in DPM or Bout24 between the road interaction and no road interaction categories (Table 6 and Figure 2).
Table 6. Mann–Whitney U test comparing response variables (MPD, DPM, Mov24, and Bout24) between road interactions and no road interactions.
Figure 2. Minimum, median, and maximum values and the interquartile range of road interactions and no road interactions in Crotalus horridus RT and ACT movement metrics. The white stars on the graphs represent the mean of each category. RT derived movement measures (A,B) include Distance Per Movement (DPM) and Meters Per Day (MPD). ACT derived movement measures (C,D) include the mean time spent moving per 24 h (Mov24) and the mean number of movement bouts per 24 h (Bout24). Asterisks denote significant differences (p < 0.05).
Historically, behavioral field monitoring of small and secretive animals has been methodologically challenging, limiting the ability to make comprehensive evaluations of the effects of anthropogenic landscape features, such as roadways, on movement patterns. With the recently validated RT-ACT framework for use in pit vipers, we sought to better understand the behavioral response of Timber Rattlesnakes (Crotalus horridus) to roadways. Based on preliminary observations, we hypothesized that roadways would constrain RT and ACT movement metrics at both a coarse and fine time scale, but our results did not support this prediction. The evaluation of RT metrics and ACT metrics at a coarse, annual time scale did not reveal any significant effect of the annual mean distance to roadways. However, a fine-scale analysis comparing the RT and ACT movement metrics during confirmed road interactions to metrics across all remaining relocations revealed a significant increase in movement distances and durations by C. horridus during these brief encounters with roadways. Although the individuals included in our sample did not interact with roads as frequently as expected given the high concentration of roadways at the site (Figure 3), this hidden fine-scale variation in movement could carry significant behavioral implications in populations where individuals more frequently interact with roads. These results also feature the importance of considering multiple time scales when evaluating the behavioral response in question and demonstrate the utility of accelerometry in longitudinal studies of pit viper movement behavior.
Figure 3. Map of the study site within Cedar Creek Wildlife Management Area in Putnam County, GA. Paved roads are outlined in purple, and unpaved, dirt roads are outlined in turquoise. Color coded points indicate the RT locations of each individual snake. 100% Minimum Convex Polygons depict home ranges for all individuals included in the study from 2020 to 2021.
Mean DTR did not affect our RT and ACT response variables when controlling for sex, indicating that at an annual time scale, the distance to roads does not significantly contribute to variation in individual movement patterns. Similar studies have found that human disturbance does not significantly change the spatial movement patterns of snakes (Shepard et al., 2008b; Lomas et al., 2019; Carrasco-Harris et al., 2020), likely due to the presence of contiguous habitat adjacent to the disturbance (Nordberg et al., 2021). Although roads are prominent features at our study site, there is sufficient unfragmented habitat alongside of the roads that likely serves as a buffer to any long-term shifts in movement behavior and space use of C. horridus (Figure 3). Additionally, the life history traits associated with habitat specialists, such as C. horridus (i.e., adapted to contiguous habitat with abundant cover), could result in a reduced boundary response given the relatively low encounter rate with habitat boundaries, such as roads (Fahrig, 2007). This could explain the lack of an annual spatial response in our dataset, given that roads at our site do not appear to be acting as impermeable barriers to movement, as 15 of 17 road interactions resulted in confirmed road crossing events. The low frequency of road interactions observed within our monitored sample of snakes, despite many individuals occupying home ranges in relatively close proximity to roadways (mean DTR = 342 m; see Table 1), also corresponds with previous observations of general road avoidance by snakes (Shine et al., 2004; Andrews et al., 2008; Shepard et al., 2008b; Robson and Blouin-Demers, 2013).
The absence of an effect of DTR on the annual RT and ACT movement metrics of C. horridus could also, in part, be explained by strong site fidelity (Marshall et al., 2020; Nordberg et al., 2021). Pit vipers have been documented repeatedly returning to overwintering sites and foraging locations, often across multiple active seasons (Pattishall and Cundall, 2008; Nordberg et al., 2021). In such cases, individuals might travel through disturbed landscapes or cross roads to return to sites used previously, presumably because the benefits of returning to these known sites outweigh the costs of searching for new ones. In our case, two monitored snakes were observed crossing roads while moving to and from their overwintering sites, but neither individual crossed a roadway at any other point during the active season, supporting the possibility that C. horridus might endure the risk of crossing roads to return to favorable foraging and overwintering areas used previously. Also, the road surface type, traffic volume, and traffic noise have been found to influence how snakes interact with roads (Robson and Blouin-Demers, 2013). We could not statistically account for these effects in our study, as snakes most often interacted with low traffic, low noise, unpaved roads as opposed to the higher traffic, higher noise, paved roads present at the site. Further sampling could reveal how different road surfaces and traffic volumes might impact C. horridus movement behavior in this population and elsewhere.
Intrinsic factors, such as sex and motivational state, have pronounced effects on the movement patterns of pit vipers (Waldron et al., 2006; DeSantis et al., 2019; Emerson et al., 2022) and therefore are a key consideration when investigating the influence of roadways on C. horridus movement. For pit vipers, elevated male movement is thought to be the primary determinant of mate location and success, and this is thought to drive significant increases in movement and space use by males during the mating season (Waldron et al., 2006; Petersen et al., 2019). Given this male-search based mating system and observations made by previous authors on C. horridus elsewhere in their distribution, we expected males to generally display elevated measures of movement and space use relative to females (both within seasons and when data are pooled across seasons). As a result of insufficient within-sex and within-season sample sizes, we were unable to statistically evaluate the effect of sex on our annual ACT derived movement metrics or the effect of behavioral season on the annual RT and ACT metrics. However, the annual RT LME models showed that the expected between-sex differences were expressed across several of our RT derived spatial measures of movement and space use. The small effect sizes within our RT LME models that produced statistically significant p-values (MPD, DPM, MV and 95% UD) were likely a result of the high variance within our sample when subdividing by sex. Given the abundance of evidence supporting similar sex-specific patterns in other populations of C. horridus and congeners (Waldron et al., 2006; Putman et al., 2013; Mata-Silva et al., 2018; Petersen et al., 2019), we are confident that this main effect of sex aligns with general patterns at the population-level for this site. Although males generally had higher measures of movement compared to females, neither were significantly affected by the proximity to roadways. Additionally, the marginal R2 values for RT and ACT LME models were consistently much lower than the conditional R2 values indicating that the relationships tested are being heavily influenced by the random effect, snake ID. Clearly, further RT and ACT sampling is needed to better understand the influence of sex and behavioral season on movement in this population. Specifically, it seems especially important to explore whether motivational state (mate-searching vs. foraging) is associated with additional variation in the effect of roads on movement behavior.
Although no main effects of DTR were detected for the annual RT and ACT metrics, extracting specific instances when snakes interacted with roads (i.e., when a snake either crossed a road or encountered a road and retreated) allowed for a fine-scale evaluation of variation in movement distances (RT) and durations (ACT). Contrary to our hypothesis, this approach revealed that individuals significantly increased the mean time spent moving per 24 h (Mov24) and the mean distance moved per day (MPD) within the brief periods of time (four relocations, or 2 weeks) containing road interactions. This difference was not reflected in the daily number of movement bouts (Bout24) or the mean distance traveled per movement (DPM). Considering the transient nature and low frequency of these road interactions among our sampled snakes, it is unsurprising that this fine-scale impact of roadways was not maintained at the coarse, annual time scale. Although statistically significant effects of roadways were detected at this resolution, small effect sizes for MPD (r = 0.14) and Mov24 (r = 0.10) were likely a result of the low frequency of road interaction observations relative to the remainder of the active season, along with high variance in our response variables across those relatively few observations. However, the magnitude of the movement increases observed could still hold biological significance (60.00% increase in Mov24 and 23.14% increase in MPD during road interactions) and highlight the importance of considering the time scale at which movement behavior is evaluated, as relevant variation could be overlooked if the chosen scale is too fine or too coarse. Given that rates of food intake and resulting body condition is a direct predictor of survival and fitness for ambush predators with low energetic requirements (Wasko and Sasa, 2012; Glaudas and Alexander, 2017), the possible energetic costs of increased movement distances and durations during instances when a snake encounters a road could have cascading negative impacts on fitness. Although direct mortality is ultimately the most deleterious fitness consequence associated with frequent road encounters, the sub-lethal consequences of increased movement during road interactions might be amplified in populations where individuals more frequently encounter roads, as chronic increases in time spent moving could lead to excess energy expenditure and a reduction in time spent foraging. When taken along with the increased risks of direct mortality and reductions in genetic diversity for populations exposed to higher road densities and traffic (Clark et al., 2010), this potentially hidden energetic cost from elevated movement during road encounters is an additional factor to consider in snake-road interaction studies. For C. horridus, specifically, this also further justifies the recognition of roads as the most prominent threat to populations range-wide (Petersen and Sealy, 2021).
We can only speculate on what might be driving this fine-scale elevation in movement duration and distance among individuals interacting with roads. A possible explanation is that the snakes are displaying an avoidance response by moving back and forth along the road edge prior to attempting to cross. This avoidance behavior could be a response to the road itself or to the open space with little ground or canopy cover created by the road. In previous studies, snakes have been documented to avoid roads (Shine et al., 2004; Andrews et al., 2008; Shepard et al., 2008b; Robson and Blouin-Demers, 2013), and the low frequency of confirmed road interactions during our study period, despite many of the monitored snakes being originally captured on or near roads, supports this hypothesis. Also, there were a low number of road interactions overall, and the snakes that did interact with roads moved away immediately following most road interactions. This does not support the hypothesis that the increased movement of C. horridus during road interactions is a result of being attracted to the edge habitat created by roadways (Cowardin et al., 1985; Aresco, 2005; Mata et al., 2017). There is also evidence indicating that snakes are less effective at tracing chemosensory cues across road surfaces relative to the natural landscape (Shine et al., 2004). It is possible that the elevated Mov24 and MPD signal is indicative of indecisiveness when encountering an unusual landscape feature within which they might not be able to effectively perceive the chemical environment. If this is the case, this result would have significant consequences for mate-searching male rattlesnakes in this population and warrants future investigation on the impacts of roads while more effectively accounting for the role of sex and behavioral season. In any case, the fine-scale variation in movement patterns of C. horridus revealed in this study emphasizes the value of a scale-dependent analytical approach and demonstrates the utility of the recently validated RT-ACT framework in fine-scale behavioral monitoring of pit vipers.
Negative effects of roads on vertebrate taxa have been widely documented (Ashley and Robinson, 1996; Shepard et al., 2008b; Clark et al., 2010), but the behavioral mechanisms mediating wildlife-road interactions have received far less attention, especially for small and secretive species such as most snakes. This study is among the first systematic uses of accelerometry for quantifying the movement behavior of snakes in the field, and the first to leverage RT-ACT monitoring for detailed evaluation of the scale-dependent behavioral responses of snakes to roadways. Annual, coarse scale RT and ACT measures of movement and space-use did not reveal differences in spatial movement patterns or daily movement durations in relation to mean distance from roads, likely as a result of the large and unfragmented patches of habitat adjacent to roads at our study site. However, quantifying RT and ACT movement metrics at a much finer time scale uncovered a significant increase in movement distances and durations by C. horridus during the rare instances when they did encounter roads. This finding has appreciable conservation implications for all C. horridus populations. In addition to the widely referenced negative effects of road mortality on C. horridus and many other snake species (Andrews et al., 2008; Clark et al., 2010; Petersen and Sealy, 2021), this potential hidden energetic cost incurred during road encounters could have significant fitness effects, especially for those populations occurring in habitats more highly fragmented by roads. While we were unable to consider the effects of road surface type and traffic volume in this response due to limited sample sizes, it is notable that 15 of the 17 road interactions included in the fine-scale analysis involved low-traffic, unpaved roads, perhaps indicating that C. horridus were more likely to interact with low-use unpaved roads than the relatively higher-trafficked paved roads in the study area. This highlights the importance of considering the effects of low-traffic roads on snake behavior even in the absence of prominent road mortality. Ultimately, further sampling is required to refine our understanding of the effects of different types of roads on the movement behavior of C. horridus, specifically to better account for the roles of sex, season, and motivational state. Nevertheless, our results highlight the value of this novel integration of radio telemetry and accelerometry for quantifying snake movement behavior across multiple time scales, particularly in attempts to capture subtle or fleeting instances of behavior with potentially important fitness consequences.
The raw data supporting the conclusions of this article will be made available by the authors, without undue reservation.
The animal study was reviewed and approved by the Institutional Animal Care and Use Committee of Georgia College & State University (protocol number 2020-D). The work was permitted by the Georgia Department of Natural Resources (Scientific Collecting Permit #1001056844).
The project was designed by AT and DD. AT contributed to data collection and analyses and led manuscript preparation. DD contributed to data collection and analyses and critically revised the manuscript. JV-D contributed to the accelerometer data processing, model validation, and application, and critically revised the manuscript. All authors contributed to the article and approved the submitted version.
This research was provided by the GCSU Department of Biological and Environmental Sciences, GCSU College of Science, and the Herpetologists’ League E. E. Williams Research Grant.
We would like to thank Liz Caldwell, Daniel Sollenberger, Zach Nichols, Cliff Rushton, David Lutz, and Paul Watson for providing study site access and logistical support for this research. We would also like to thank Reagan Thornton, Conor Evans, and Morgan Thompson for their assistance with field data collection. Finally, we thank Christine Mutiti for assistance with spatial data processing and Katie Stumpf and Matthew Milnes for their helpful feedback on early versions of this manuscript. Content in this manuscript appeared previously online in author AT’s thesis (Tipton, 2022).
The authors declare that the research was conducted in the absence of any commercial or financial relationships that could be construed as a potential conflict of interest.
All claims expressed in this article are solely those of the authors and do not necessarily represent those of their affiliated organizations, or those of the publisher, the editors and the reviewers. Any product that may be evaluated in this article, or claim that may be made by its manufacturer, is not guaranteed or endorsed by the publisher.
Andrews, K. M., and Gibbons, J. W. (2005). How do highways influence snake movement? Behavioral responses to roads and vehicles. Copeia 2005, 772–782. doi: 10.1643/0045-8511(2005)005[0772:HDHISM]2.0.CO;2
Andrews, K. M., Gibbons, J. W., Jochimsen, D. M., and Mitchell, J. (2008). Ecological effects of roads on amphibians and reptiles: a literature review. Herp. Cons. 3, 121–143.
Anguiano, M. P., and Diffendorfer, J. E. (2015). Effects of fragmentation on the spatial ecology of the California kingsnake (Lampropeltis californiae). J. Herpetol. 49, 420–427. doi: 10.1670/13-014
Aresco, M. J. (2005). The effect of sex-specific terrestrial movements and roads on the sex ratio of freshwater turtles. Biol. Conserv. 123, 37–44. doi: 10.1016/j.biocon.2004.10.006
Ashley, E. P., and Robinson, J. T. (1996). Road mortality of amphibians, reptiles and other wildlife on the long point causeway, Lake Erie, Ontario. Can. Field-Nat. 110, 403–412.
Barrientos, R., and Bolonio, L. (2009). The presence of rabbits adjacent to roads increases polecat road mortality. Biodivers. Conserv. 18, 405–418. doi: 10.1007/s10531-008-9499-9
Barwick, J., Lamb, D. W., Dobos, R., Welch, M., and Trotter, M. (2018). Categorising sheep activity using a tri-axial accelerometer. Comput. Electron. Agric. 145, 289–297. doi: 10.1016/j.compag.2018.01.007
Bauder, J. M., Breininger, D. R., Bolt, M. R., Legare, M. L., Jenkins, C. L., and McGarigal, K. (2015). The role of the bandwidth matrix in influencing kernel home range estimates for snakes using VHF telemetry data. Wildl. Res. 42, 437–453. doi: 10.1071/WR14233
Bauder, J. M., Breininger, D. R., Bolt, M. R., Legare, M. L., Jenkins, C. L., Rothermel, B. B., et al. (2016). Seasonal variation in eastern indigo snake (Drymarchon couperi) movement patterns and space use in peninsular Florida at multiple temporal scales. Herpetologica 72, 214–226. doi: 10.1655/Herpetologica-D-15-00039.1
Brown, D. D., Kays, R., Wikelski, M., Wilson, R., and Klimley, A. (2013). Observing the unwatchable through acceleration logging of animal behavior. Anim. Biotelemetry 1:20. doi: 10.1186/2050-3385-1-20
Bushar, L. M., Reinert, H. K., and Gelbert, L. (1998). Genetic variation and gene flow within and between local populations of the timber rattlesnake, Crotalus horridus. Copeia 1998, 411–422. doi: 10.2307/1447435
Carrasco-Harris, M. F., Bowman, D., Reichling, S., and Cole, J. A. (2020). Spatial ecology of copperhead snakes (Agkistrodon contortrix) in response to urban park trails. J. Urban Ecol. 6:juaa007. doi: 10.1093/jue/juaa007
Clark, R. W., Brown, W. S., Stechert, R., and Zamudio, K. R. (2010). Roads, interrupted dispersal, and genetic diversity in timber rattlesnakes. Conserv. Biol. 24, 1059–1069. doi: 10.1111/j.1523-1739.2009.01439.x
Cowardin, L. M., Gilmer, D. S., and Shaiffer, C. W. (1985). Mallard recruitment in the agricultural environment of North Dakota. Wildl. Monogr. 92, 3–37.
DeSantis, D. L., Mata-Silva, V., Johnson, J. D., and Wagler, A. E. (2020). Integrative framework for long-term activity monitoring of small and secretive animals: validation with a cryptic pitviper. Front. Ecol. Evol. 8:169. doi: 10.3389/fevo.2020.00169
DeSantis, D. L., Wagler, A. E., Mata-Silva, V., and Johnson, J. D. (2019). Effects of human-made resource hotspots on seasonal spatial strategies by a desert pitviper. Sci. Rep. 9:16690. doi: 10.1038/s41598-019-52957-1
Emerson, J. D., DeSantis, D. L., Mata-Silva, V., Wagler, A. E., and Johnson, J. D. (2022). Movement, home range size, and habitat use of eastern black-tailed rattlesnakes (Crotalus ornatus) in the northern Chihuahuan Desert. Herpetologica 78, 110–118. doi: 10.1655/Herpetologica-D-21-00009
Ettling, J. A., Aghasyan, L. A., Aghasyan, A. L., and Parker, P. G. (2013). Spatial ecology of Armenian vipers, Montivipera raddei, in a human-modified landscape. Copeia 2013, 64–71. doi: 10.1643/CE-11-172
Fahrig, L. (2007). Non-optimal animal movement in human-altered landscapes. Funct. Ecol. 21, 1003–1015. doi: 10.1111/j.1365-2435.2007.01326.x
Fahrig, L., and Rytwinski, T. (2009). Effects of roads on animal abundance: an empirical review and synthesis. Ecol. Soc. 14:21. doi: 10.5751/ES-02815-140121
Farzaneh, M. (2022). Relief Feature Selection Algorithm, MATLAB Central File Exchange. Available at: https://www.mathworks.com/matlabcentral/fileexchange/74220-relief-feature-selection-algorithm (Accessed April 6, 2022)
Forman, R. T. T., Sperling, D., Bissonette, J. A., Clevenger, A. P., Cutshall, C. D., Dale, V. H., et al. (2003). Road Ecology. Washington, DC: Island Press.
Georgia Department of Transportation (2022). Georgia Department of Transportation’s Traffic Analysis and Data Application. Available at: https://gdottrafficdata.drakewell.com/ (Accessed December 26, 2022)
Glaudas, X., and Alexander, G. J. (2017). Food supplementation affects the foraging ecology of a low-energy, ambush-foraging snake. Behav. Ecol. Sociobiol. 71, 1–11. doi: 10.1007/s00265-016-2239-3
Hardy, D. L. S., and Greene, H. W. (1999). Surgery on rattlesnakes in the field for implantation of transmitters. Sonoran Herpetol. 12, 25–27.
Hardy, D. L. S., and Greene, H. W. (2000). Inhalation anesthesia of rattlesnakes in the field for processing and transmitter implantation. Sonoran Herpetol. 13, 109–113.
Hibbitts, T. J., Fitzgerald, L. A., Walkup, D. K., and Ryberg, W. A. (2017). Why didn’t the lizard cross the road? Dunes sagebrush lizards exhibit road-avoidance behaviour. Wildl. Res. 44, 194–199. doi: 10.1071/WR16184
Holderegger, R., and Di Giulio, M. (2010). The genetic effects of roads: a review of empirical evidence. Basic Appl. Ecol. 11, 522–531. doi: 10.1016/j.baae.2010.06.006
Jaeger, J. A., Bowman, J., Brennan, J., Fahrig, L., Bert, D., Bouchard, J., et al. (2005). Predicting when animal populations are at risk from roads: an interactive model of road avoidance behavior. Ecol. Model. 185, 329–348. doi: 10.1016/j.ecolmodel.2004.12.015
Jenkins, C. L., Martin, W. H., Hudson, B. D., Stevenson, D. J., and Spivey, P. B. (2021). “Georgia” in The Timber Rattlesnake: Life History, Distribution, Status, and Conservation Action Plan. eds. A. R. Breisch, W. H. Martin, J. B. Sealy, C. E. Petersen, and E. Possardt (Nashville, TN: Amphibian and Reptile Conservancy, Inc.), 133–146.
Kapfer, J. M., Pekar, C. W., Reineke, D. M., Coggins, J. R., and Hay, R. (2010). Modeling the relationship between habitat preferences and home-range size: a case study on a large mobile colubrid snake from North America. J. Zool. 282, 13–20. doi: 10.1111/j.1469-7998.2010.00706.x
Keller, I., and Largiader, C. R. (2003). Recent habitat fragmentation caused by major roads leads to reduction of gene flow and loss of genetic variability in ground beetles. Proc. R. Soc. Lond. B Biol. Sci. 270, 417–423. doi: 10.1098/rspb.2002.2247
Kononenko, I. (1994). “Estimating attributes: analysis and extensions of RELIEF,” in In European Conference on Machine Learning. eds. F. Bergadano and L. De Raedt (Berlin, Heidelberg: Springer), 171–182.
Kranstauber, B., Kays, R., LaPoint, S. D., Wikelski, M., and Safi, K. (2012). A dynamic Brownian bridge movement model to estimate utilization distributions for heterogeneous animal movement. J. Anim. Ecol. 81, 738–746. doi: 10.1111/j.1365-2656.2012.01955.x
Le Roux, S. P., Marias, J., Wolhuter, R., and Niesler, T. (2017). Animal-borne behaviour classification for sheep (Dohne merino) and rhinoceros (Ceratotherium simum and Diceros bicornis). Anim. Biotelemetry 5, 1–13.
Leblond, M., Dussault, C., and Ouellet, J. P. (2013). Impacts of human disturbance on large prey species: do behavioral reactions translate to fitness consequences? PLoS One 8:e73695. doi: 10.1371/journal.pone.0073695
Lenth, R. V. (2022). Emmeans: Estimated Marginal Means, aka Least-Squares Means. Available at: https://CRAN.R-project.org/package=emmeans
Lodé, T. (2000). Effect of a motorway on mortality and isolation of wildlife populations. AMBIO J. Hum. Environ. 29, 163–166. doi: 10.1579/0044-7447-29.3.163
Lomas, E., Maida, J. R., Bishop, C. A., and Larsen, K. W. (2019). Movement ecology of northern Pacific rattlesnakes (Crotalus o. oreganus) in response to disturbance. Herpetologica 75, 153–161. doi: 10.1655/D-17-00060
Lovallo, M. J., and Anderson, E. M. (1996). Bobcat movements and home ranges relative to roads in Wisconsin. Wildl. Soc. Bull. 24, 71–76.
Marshall, B. M., Crane, M., Silva, I., Strine, C. T., Jones, M. D., Hodges, C. W., et al. (2020). No room to roam: king cobras reduce movement in agriculture. Mov. Ecol. 8, 1–14. doi: 10.1186/s40462-020-00219-5
Martin, W. H., Sealy, J. B., Petersen, C. E., and Brown, W. S. (2021). “Life history and ecological requirements” in The timber rattlesnake: Life history, distribution, status, and conservation action plan. eds. A. R. Breisch, W. H. Martin, J. B. Sealy, C. E. Petersen, and E. Possardt (Nashville, TN: Amphibian and Reptile Conservancy, Inc.), 23–57.
Martin, W. H., Stevenson, D. J., and Spivey, P. B. (2008). “Timber rattlesnake” in Amphibians and reptiles of Georgia. eds. J. B. Jensen, C. D. Camp, W. Gibbons, and M. J. Elliott (Athens, GA: University of Georgia Press), 433–436.
Mata, C., Ruiz-Capillas, P., and Malo, J. E. (2017). Small-scale alterations in carnivore activity patterns close to motorways. Eur. J. Wildl. Res. 63, 1–12. doi: 10.1007/s10344-017-1118-1
Mata-Silva, V., DeSantis, D. L., Wagler, A. E., and Johnson, J. D. (2018). Spatial ecology of rock rattlesnakes (Crotalus lepidus) in far West Texas. Herpetologica 74, 245–254. doi: 10.1655/Herpetologica-D-16-00030.1
McGregor, R. L., Bender, D. J., and Fahrig, L. (2008). Do small mammals avoid roads because of the traffic? J. Appl. Ecol. 45, 117–123. doi: 10.1111/j.1365-2664.2007.01403.x
Mumme, R. L., Schoech, S. J., Woolfenden, G. W., and Fitzpatrick, J. W. (2000). Life and death in the fast lane: demographic consequences of road mortality in the Florida scrub jay. Conserv. Biol. 14, 501–512. doi: 10.1046/j.1523-1739.2000.98370.x
Nathan, R., Getz, W. M., Revilla, E., Holyoak, M., Kadmon, R., Saltz, D., et al. (2008). A movement ecology paradigm for unifying organismal movement research. Proc. Natl. Acad. Sci. 105, 19052–19059. doi: 10.1073/pnas.0800375105
Ng, C. S., Des Brisay, P. G., and Koper, N. (2019). Chestnut-collared longspurs reduce parental care in the presence of conventional oil and gas development and roads. Anim. Behav. 148, 71–80. doi: 10.1016/j.anbehav.2018.12.001
Nordberg, E., Ashley, J., Hoekstra, A. A., Kirkpatrick, S., and Cobb, V. A. (2021). Small nature preserves do not adequately support large-ranging snakes: movement ecology and site fidelity in a fragmented rural landscape. Glob. Ecol. Conserv. 28:e01715. doi: 10.1016/j.gecco.2021.e01715
Oxley, D. J., Fenton, M. B., and Carmody, G. R. (1974). The effects of roads on populations of small mammals. J. Appl. Ecol. 11, 51–59. doi: 10.2307/2402004
Pattishall, A., and Cundall, D. (2008). Spatial biology of northern watersnakes (Nerodia sipedon) living along an urban stream. Copeia 2008, 752–762. doi: 10.1643/CH-07-228
Petersen, C. E., Goetz, S. M., Dreslik, M. J., Kleopfer, J. D., and Savitzky, A. H. (2019). Sex, mass, and monitoring effort: keys to understanding spatial ecology of timber rattlesnakes (Crotalus horridus). Herpetologica 75, 162–174. doi: 10.1655/D-18-00035
Petersen, C. E., and Sealy, J. B. (2021). “Threats and conservation actions” in The Timber Rattlesnake: Life History, Distribution, Status, and Conservation Action Plan. eds. A. R. Breisch, W. H. Martin, J. B. Sealy, C. E. Petersen, and E. Possardt (Nashville, TN: Amphibian and Reptile Conservancy, Inc.), 81–98.
Putman, B. J., Lind, C., and Taylor, E. N. (2013). Does size matter? Factors influencing the spatial ecology of northern Pacific rattlesnakes (Crotalus oreganus oreganus) in Central California. Copeia 2013, 485–492. doi: 10.1643/CE-12-048
Quintero-Ángel, A., Osorio-Dominguez, D., Vargas-Salinas, F., and Saavedra-Rodríguez, C. A. (2012). Roadkill rate of snakes in a disturbed landscape of Central Andes of Colombia. Herpetol. Notes 5, 99–105.
R Core Team (2022). R: A Language and Environment for Statistical Computing. R Foundation for Statistical Computing, Vienna, Austria. Available at: https://www.R-project.org/
Reinert, H. E., and Cundall, D. (1982). An improved surgical implantation method for radio-tracking snakes. Copeia 1982, 702–705. doi: 10.2307/1444674
Reynolds-Hogland, M. J., and Mitchell, M. S. (2007). Effects of roads on habitat quality for bears in the southern Appalachians: a long-term study. J. Mammal. 88, 1050–1061. doi: 10.1644/06-MAMM-A-072R1.1
Robson, L. E., and Blouin-Demers, G. (2013). Eastern hognose snakes (Heterodon platirhinos) avoid crossing paved roads, but not unpaved roads. Copeia 2013, 507–511. doi: 10.1643/CE-12-033
Rokach, L. (2010). Ensemble-based classifiers. Artif. Intell. Rev. 33, 1–39. doi: 10.1007/s10462-009-9124-7
Rondinini, C., and Doncaster, C. P. (2002). Roads as barriers to movement for hedgehogs. Funct. Ecol. 16, 504–509. doi: 10.1046/j.1365-2435.2002.00651.x
Row, J. R., and Blouin-Demers, G. (2006). Kernels are not accurate estimators for home-range size in herpetofauna. Copeia 2006, 797–802. doi: 10.1643/0045-8511(2006)6[797:KANAEO]2.0.CO;2
Row, J. R., Blouin-Demers, G., and Lougheed, S. C. (2012). Movements and habitat use of eastern Foxsnakes (Pantherophis gloydi) in two areas varying in size and fragmentation. J. Herpetol. 46, 94–99. doi: 10.1670/10-262
Ruiz-Capillas, P., Mata, C., and Malo, J. E. (2013). Community response of mammalian predators and their prey to motorways: implications for predator-prey dynamics. Ecosystems 16, 617–626. doi: 10.1007/s10021-013-9634-7
Rytwinski, T., and Fahrig, L. (2012). Do species life history traits explain population responses to roads? A meta-analysis. Biol. Conserv. 147, 87–98. doi: 10.1016/j.biocon.2011.11.023
Shepard, D. B., Dreslik, M. J., Jellen, B. C., and Phillips, C. A. (2008a). Reptile road mortality around an oasis in the Illinois Corn Desert with emphasis on the endangered eastern Massasauga. Copeia 2008, 2008, 350–359. doi: 10.1643/CE-06-276
Shepard, D. B., Kuhns, A. R., Dreslik, M. J., and Phillips, C. A. (2008b). Roads as barriers to animal movement in fragmented landscapes. Anim. Conserv. 11, 288–296. doi: 10.1111/j.1469-1795.2008.00183.x
Shine, R., Lemaster, M., Wall, M., Langkilde, T., and Mason, R. (2004). Why did the snake cross the road? Effects of roads on movement and location of mates by garter snakes (Thamnophis sirtalis parietalis). Ecol. Soc. 9:9. doi: 10.5751/ES-00624-090109
Siers, S. R., Reed, R. N., and Savidge, J. A. (2016). To cross or not to cross: modeling wildlife road crossings as a binary response variable with contextual predictors. Ecosphere 7:e01292. doi: 10.1002/ecs2.1292
Siers, S. R., Savidge, J. A., and Reed, R. N. (2014). Invasive Brown Treesnake movements at road edges indicate road crossing avoidance. J. Herpetol. 48, 500–505. doi: 10.1670/13-037
Silva, I., Crane, M., Suwanwaree, P., Strine, C., and Goode, M. (2018). Using dynamic Brownian bridge movement models to identify home range size and movement patterns in king cobras. PLoS One 13:e0203449. doi: 10.1371/journal.pone.0203449
Spellerberg, I. F. (2002). Ecological effects of roads. Land Reconstruction and Management, vol. 2. Science Publishers, Enfield, NH.
Stengle, A., Martin, W. H., Sealy, J. B., and Petersen, C. E. (2021). “Genetics and description” in The Timber Rattlesnake: Life History, Distribution, Status, and Conservation Action Plan. eds. A. R. Breisch, W. H. Martin, J. B. Sealy, C. E. Petersen, and E. Possardt (Nashville, TN: Amphibian and Reptile Conservancy, Inc.), 11–22.
Thurber, J. M., Peterson, R. O., Drummer, T. D., and Thomasma, S. A. (1994). Gray wolf response to refuge boundaries and roads in Alaska. Wildl. Soc. Bull. 22, 61–68.
Tipton, A. F. (2022). Integrating radio telemetry and accelerometry to evaluate the scale-dependent effects of roadways on the movement patterns of timber rattlesnakes (Crotalus horridus). Master’s thesis. Milledgeville, GA: Georgia College & State University.
Trombulak, S. C., and Frissell, C. A. (2000). Review of ecological effects of roads on terrestrial and aquatic communities. Conserv. Biol. 14, 18–30. doi: 10.1046/j.1523-1739.2000.99084.x
Underhill, J. E., and Angold, P. G. (2000). Effects of roads on wildlife in an intensively modified landscape. Environ. Rev. 8, 21–39. doi: 10.1139/a00-003
Waldron, J. L., Lanham, J. D., and Bennett, S. H. (2006). Using behaviorally-based seasons to investigate canebrake rattlesnake (Crotalus horridus) movement patterns and habitat selection. Herpetologica 62, 389–398. doi: 10.1655/0018-0831(2006)62[389:UBSTIC]2.0.CO;2
Ware, H. E., McClure, C. J., Carlisle, J. D., and Barber, J. R. (2015). A phantom road experiment reveals traffic noise is an invisible source of habitat degradation. Proc. Natl. Acad. Sci. U. S. A. 112, 12105–12109. doi: 10.1073/pnas.1504710112
Wasko, D. K., and Sasa, M. (2012). Food resources influence spatial ecology, habitat selection, and foraging behavior in an ambush-hunting snake (Viperidae: Bothrops asper): an experimental study. Zoology 115, 179–187. doi: 10.1016/j.zool.2011.10.001
Wilmers, C. C., Nickel, B., Bryce, C. M., Smith, J. A., Wheat, R. E., and Yovovich, V. (2015). The golden age of bio-logging: how animal-borne sensors are advancing the frontiers of ecology. Ecology 96, 1741–1753. doi: 10.1890/14-1401.1
Keywords: road ecology, accelerometry, machine learning, radio telemetry, home range
Citation: Tipton AF, Vázquez-Diosdado JA and DeSantis DL (2023) Scale-dependent effects of roadways on the movement behavior of a large-bodied pit viper (Crotalus horridus). Front. Ecol. Evol. 11:1007743. doi: 10.3389/fevo.2023.1007743
Received: 30 July 2022; Accepted: 18 January 2023;
Published: 07 February 2023.
Edited by:
Cheryl S. Brehme, Western Ecological Research Center (USGS), United StatesReviewed by:
Saeed Mohammadi, University of Zabol, IranCopyright © 2023 Tipton, Vázquez-Diosdado and DeSantis. This is an open-access article distributed under the terms of the Creative Commons Attribution License (CC BY). The use, distribution or reproduction in other forums is permitted, provided the original author(s) and the copyright owner(s) are credited and that the original publication in this journal is cited, in accordance with accepted academic practice. No use, distribution or reproduction is permitted which does not comply with these terms.
*Correspondence: Anna F. Tipton, ✉ YW5uYXRpcHRvbjcyQGdtYWlsLmNvbQ==
Disclaimer: All claims expressed in this article are solely those of the authors and do not necessarily represent those of their affiliated organizations, or those of the publisher, the editors and the reviewers. Any product that may be evaluated in this article or claim that may be made by its manufacturer is not guaranteed or endorsed by the publisher.
Research integrity at Frontiers
Learn more about the work of our research integrity team to safeguard the quality of each article we publish.