- 1Chemical Ecology Unit of Department of Plant Protection Biology, Swedish University of Agricultural Sciences, Alnarp, Sweden
- 2Department of Chemistry, Mkwawa University College of Education (MUCE), Iringa, Tanzania
As pests of fruits and vegetables, ovipositing tephritid fruit flies are infamous for their frugivory. Yet, adult tephritids have remained saprophytic in their feeding behavior, as they require decomposing, protein rich media for sexual maturation and oogenesis. Drosophilid fruit flies, in contrast, are saprophytic both during oviposition and feeding. Here we compared the sensory and behavioral responses of two tephritid (Bactrocera dorsalis and Ceratitis capitata) and two drosophilid species (Drosophila melanogaster and Drosophila suzukii) to differentially aged cultures of the yeast Saccharomyces cerevisiae. We assessed convergence and divergence in the detection of and behavioral response to these attractive substrates, and how these might be linked to the roles of the substrates for the different taxa. The headspace shifted substantially as broth cultures transitioned from active (1-day) to inactive (8- and 15-days). Interestingly, Drosophila flies were significantly attracted to actively fermenting 1-day old yeast cultures, whereas the preference shifted to older cultures for the tephritids. Bactrocera dorsalis flies preferred inactive, lysing cultures (8- and 15-days old). We identified compounds from the 1- to 8-days old broth cultures that elicited antennal responses in each species. Synthetic blends composed of antennally active compounds evoked similar behavioral responses as broth cultures. Similarly, the attractiveness of less attractive broth cultures (1- and 8-days old for drosophilids and tephritids, respectively) could be augmented by adding volatiles of the more attractive cultures. The results show that the volatile profiles of fermenting substrates evolve quantitatively and qualitatively, and that fly species key into volatile blends that indicate suitability of the substrates for their purposes. For drosophilids early arrival at fermenting substrates confers a competitive advantage to offspring. In contrast, for tephritid the concentration and availability of protein is facilitated by older, lysed yeast cultures. The data from this comparative study are also instrumental in the development of novel lures for these pests.
Introduction
For many insects, nitrogen is a scarce source, and hence most insect species are attracted to decomposing substrates. Often rich in protein, these substrates offer food and oviposition sites, and are frequently associated with sexual maturation. Decomposing sources are very diverse, and most insects display defined preferences for particular substrates and the microbes associated with these. In fact, a great number of close mutualistic associations between insects and microbes have been described (Fogleman et al., 1981; Becher et al., 2018; Madden et al., 2018; Stefanini, 2018), with the insects profiting from microbial breakdown, protein rich substrates, and microbes benefitting from insects as vehicles of transportation to new substrates (Ganter, 1988; Reuter et al., 2007).
The fly families Drosophilidae and Tephritidae, both generically called fruit flies, are particularly strongly associated with microbes (Lam and Howell, 2015; Deutscher et al., 2016). However, the two taxa differ in the role decomposing resources play in their life history. In Drosophila species, microbial substrates serve as breeding, feeding and mating substrates (Heed et al., 1976; Begon and Shorrocks, 1978). In Tephritidae, oviposition in decomposing substrates is ancestral (Prokopy et al., 1999), but most extant taxa use these substrates for adult feeding, sexual maturation, and oogenesis, while oviposition takes place in intact fruits and vegetables. The preferred stage of decomposition for feeding may also differ, although this has not been directly compared. Whereas drosophilids are particularly attracted to active microbial cultures (Becher et al., 2012), tephritid fruit flies are known to be attracted to lysed yeast cells, brewery waste, and old cultures that generically contain autolysis cells and freely available proteins, amino acids, polypeptides, and vitamins (Hagen and Finney, 1950; Drew et al., 1983; Meats and Kelly, 2008). Accordingly, early tephritid attractants contained volatiles typically associated with autolysis, such as ammonia and amines (Vargas and Prokopy, 2006; Epsky et al., 2014).
Of all microbe-insect interactions, associations with yeasts are among the most reported. Saccharomyces cerevisiae is particularly well studied, possibly because of the close link of S. cerevisiae with humans and early observations of fly attraction to these. Yeasts and yeast substrates are indeed highly nutritious and are sufficient for larval development and adult nutritional needs (Hagen and Finney, 1950; Chang, 2009; Fanson and Taylor, 2012; Walder et al., 2014), and strong associations between insects and yeasts have been described numerous times (Blackwell, 2017; Madden et al., 2018; Stefanini, 2018). In some cases, these relationships are specific. For example, larvae of the cactophilic Drosophila, Drosophila mojavensis, is known to selectively feed on the yeast Pichia cactophila, which metabolizes toxic fatty acids (Fogleman et al., 1981), but also more generic associations have been frequently described for drosophilids (e.g., Broderick and Lemaitre, 2012; Hamby et al., 2012), though such reports are few for Tephritidae (Deutscher et al., 2016).
Given the importance of yeasts, fruit flies have evolved an acute sensitivity to volatiles associated with them. Indeed, since long, yeast cultures and their (by) products have been used to lace baits for attract-and-kill formulations for flies (Baker et al., 1945, Epsky et al., 2014). Characteristic blends of volatile organic compounds are released from early stages of active fermentation to lysing cultures, and many of these are known to generically attract insects (Ljunggren et al., 2019), and particularly Drosophilidae (Stökl et al., 2010; Becher et al., 2012; Davis et al., 2013) and Tephritidae (Biasazin et al., 2018). In Drosophila melanogaster, active yeast cultures alone are sufficient to initiate upwind flight, whereas attraction to undamaged fruits is limited (Becher et al., 2012).
Although yeast cultures are generally attractive to drosophilids and tephritids, the preferred age of yeast culture may differ, along with the roles these substrates play in the fly’s life history. Active yeast cultures are highly attractive to D. melanogaster from very early stages of fermentation (Schiabor et al., 2014). This is linked to the fact that early arrival and reproduction increases survival of offspring, as with time conspecific and heterospecific competition increases (Shorrocks and Bingley, 1994). In addition, fermenting substrates are often ephemeral and early arrival thus increase the chance of successfully completing the larval stage (Rosewell et al., 1990; Shorrocks et al., 1990; Hodge et al., 1996). In contrast, older cultures and lysed cells facilitate imbibing substrate of and absorption of nutrients by the midgut, which suits Tephritidae. In this study we investigated whether drosophilid and tephritid fruit flies are differentially attracted to various stages of S. cerevisiae yeast cultures, whether these differences are reflected in the volatile profile and sensory responses and discussed how differences in preference link to different roles such cultures play in the flies’ life histories.
Materials and methods
Insects
Drosophila melanogaster Dalby-HL strain (Ruebenbauer et al., 2008) and Drosophila suzukii originated from infested fruits in Italy and maintained at the Swedish University of Agricultural Science (SLU), Alnarp (Rehermann et al., 2021) were reared on Bloomington standard cornmeal diet. Three to five days old flies were used for experiments. Colonies of two tephritid species, B. dorsalis and C. capitata were started from pupae provided by the International Atomic Energy Agency (IAEA, Vienna, Austria). Larvae were reared on a carrot-based diet (Biasazin et al., 2018). Adult flies were kept in bugdorm cages (L32.5 × W32.5 × H32.5 cm, Mega View Science Co., Ltd., Taiwan) with mesh sizes (96 um × 26/680 um) opening and fed with a mixture of 3:1 sugar: yeast and were provided with water-soaked cotton balls on a 9 cm plastic petri dish. Females were separated upon emergence and virgin females (4–5 days old) were used for experiments. Both Drosophilidae and Tephritidae flies were reared at 25°C, 50–65% RH and 12:12 h light: dark photoperiod.
Yeast strain and culture
Baker’s yeast (S. cerevisiae) (Kronans Jäst, Jästbolaget AB) was used for this study. One gram of the yeast cells was mixed with 3 ml of deionized water and 10 μl was streaked on agar plates and left for 48 h. When colonies were observed, a single colony was transferred to a 50 ml broth of minimal media (MM) containing 10% glucose. Minimal media was selected as it has no odorants that may interfere with the yeast production (Becher et al., 2018). Equal amounts 25 μl of the yeast-broth was then transferred to 4 ml MM and aged for 1, 8, and 15 days under controlled climatic conditions, on an oscillating incubator at 260 rpm and 25°C until needed for behavior, electrophysiology, and mass spectrometry. This procedure was followed throughout the experiments.
Olfactometer assay
A 6-choice olfactory setup modified from Biasazin et al. (2019) was used for the behavioral experiments. The setup was made up of a cubic glass arena (45 cm × 45 cm × 45 cm) covered with a glass plate with six circular 50 mm holes, fitted with inverted conical glass funnels of 45 mm top and 4 mm bottom holes through which flies could pass, the glass funnel was surrounded by a fly collection cylinder of 50 mm diameter × 40 mm high, and on top of which was a similarly sized odor placing cylinder, a glass plate with a 2 mm hole separates the upper and lower cylinders. On the very top, covering the odor placing cylinder was a glass plate cover with 6 holes (5 mm) receiving clean odor (Supplementary Figure 3). A 0.5 l/min air flow was received through the holes of the top glass cover. The airflow was regulated using a pump (Elite 802, Hagen Ltd, UK and airflow meters (Model ExK Kitola Instrument Oy, Muurame, Finland). The airstream was filtered and humidified through two wash bottles containing activated charcoal and distilled water. Teflon tubing was used throughout. The top light source (daylight lamp, Photo studio CFL 45 W, 5000 K) was diffracted using an opaque white plexiglass panel. The whole setup was covered with white fabric to avoid visual disturbance by external movements. Treatments (1 ml of differentially aged yeasts and MM as a control or 100 μl of synthetic blends and paraffin oil as a control) were transferred to a 5 ml cup (Nolato Cerbo AB, Sweden) and placed in each of the odor holding cylinders. Treatment cups and glass chambers were rotated between experiments to avoid effects of cross contamination and position. Thirty flies were released in the arena and the number of flies that chose either of the treatments in the six chambers were recorded after 30 min. Fifteen replicates were made for each species. All glass surfaces of the setup were thoroughly cleansed with 96% ethanol and water after a single run, following drying overnight for next experiments.
Electrophysiology
Flies were immobilized in a 200 μl micropipette tip with the antennae exposed. Capillaries filled with Beadle-Ephrussi ringer solutions (7.5 g Nacl, 0.35 g Kcl, 0.29 g Cacl2 dissolved in 1 L of distilled water) were used to create conduction between the electrodes and the insect antenna. The glass capillary attached to the reference electrode was inserted into the head of the fly, and the glass capillary on the recording electrode was connected to the tip of the antennae. The recording electrode was connected to a pre-amplifier probe and then to a high impedance GC amplifier interface box (IDAC-2; Syntech, Kirchgarten, Germany). The temperature program of the GC oven was set to start at 40°C and held for 1 min, increasing by 10°C min–1 to 250°C and held for 1 min. The inlet was in splitless mode with temperature of 250°C. The headspace sample was carried by H2 through a polar DB-WAX capillary column (60 m × 0.25 mm i.d., 0.25 μm film thickness, USD608325H Agilent Technologies Inc.). The effluent from the GC was split 1:1 between the flame ionization detector (FID) and the EAD. The column capillary for the EAD passed through a Gerstel olfactory detection port-2 transfer line tracking the GC oven temperature into a glass tube (30 cm × 8 mm), where it was mixed with charcoal filtered and humidified air at a flow rate of 1.5 l min–1 and passed over the fly’s antenna. EAD peaks were analyzed using syntech data acquisition system software (GcEad 2012 v1.2.4, syntech, Germany).
Odor sampling and identification
Odor sampling was performed using SPME (solid phase microextraction) fibers coated with DVB/CAR/PDMS, Merck KGaA, Darmstadt, Germany). Sampling was done for 10 min using 1 ml of yeast-broth in a 20 ml vial (Precision Thread Headspace-Vials 75.5×, Genetec, Västra Frölunda, Sweden) fitted with a PTFE-lined septa. Before sampling the SPME fibers were conditioned for 10 min. After sampling the SPME fibers were injected to either the inlet (250°C) of a Gas Chromatography coupled Mass Spectrometry (GC-MS, Agilent 7890B GC and 5977A MS, Agilent Technologies Inc., Palo Alto, CA, USA) or a Gas Chromatography coupled with Electroantennography (GC-EAD). Both the GC-MS and the GC-EAD used a polar DB-Wax capillary column (60 m × 0.25 mm i.d., 0.25 um film thickness, USD608325H Agilent Technologies Inc.), with helium as carrier gas in the GC-MS and hydrogen in the GC-EAD. The temperature programs of the GC-MS were as per the GC-EAD system described above. Identification and quantification of compounds were conducted using chemstation software (Agilent technologies). Compounds were initially identified by mass spectra searching of the libraries; Nist20, Alnarp 11 and Wiley’s 275. The identification was supported by comparing the retention indices generated by running standard mixtures of alkane (C6-C20) under the same conditions as the samples and comparing the identity of the compounds Kovats retention indices obtained from electrophysiology and the GC-MS with published sources. Antenna active compounds were further confirmed by injecting synthetic compounds. Quantification was performed using 10 ng/μl solutions of three esters (pentyl acetate, ethyl hexanoate and ethyl octanoate) for compounds that elute early (before 8 min), in the middle (until 12 min), and late (after 13 min), respectively.
Synthetic blends
Based on the antennal responses of drosophilids and tephritids two major blends (base blends) were formulated (Table 1). One of the major blends contained ethyl acetate, 3-methylbutan-1-ol and ethanol which were compounds commonly shared across Tephritidae antennal responses, whereas the second major blend was formulated based on compounds commonly shared across Drosophilidae antennal responses and it was composed of acetoin, acetic acid, and ethyl acetate. Specific blends for each species of fruit flies were also formulated by adding uniquely detected compounds from the 1- to 8-days old cultures to the major blends (Base + 1-day or Base + 8-day). For B. dorsalis, Base + 1-day was composed of the base blend for tephritidae combined with ethyl nonanoate and 3-methylbutyl acetate, whereas Base + 8-day was composed of the tephritidae base blend combined with 2-phenylethanol (Table 1). For C. capitata Base + 1-day refers to the tephritid base blend combined with ethyl hexanoate, whereas Base + 8-day refers to the tephritidae blend combined with butane 2,3-dione. For D. melanogaster and D. suzukii Base + 1-day refers to the drosophilid base blend combined with ethyl octanoate and ethyl hexanoate, whereas Base + 8-day refers to the drosophilid base blend combined with ethyl 2-hydroxypropanoate for D. melanogaster and the drosophilid base blend combined with ethanol and butane 2,3-dione for D. suzukii. These blends were compared with an active culture (1-day old) with 8-days or 1-day old unique compounds added to it (Table 1). All compounds except for ethanol and 3-methylbutan-1-ol were formulated in 1:1 ratio. Ethanol and 3-methylbutan-1-ol were formulated in an 11:3 ratio. The compounds were diluted 10,000 (10–4) folds for behavioral assays. The compounds used had >98% purity and were purchased from Merck (Darmstadt, Germany).
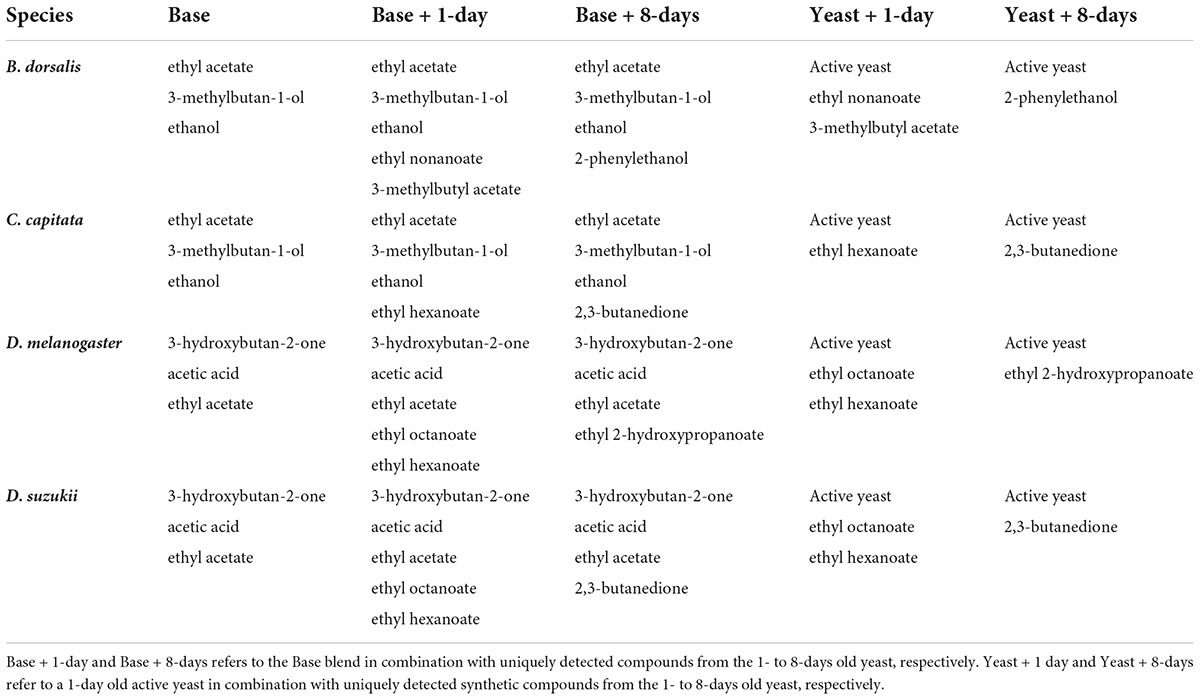
Table 1. Synthetic mixes of compounds used in the behavioral assay with and without an active yeast.
Data analysis
The number of fruit flies caught by different treatments in the 6-choice assay were analyzed using generalized linear models. The data was fitted with a poisson distribution and tested for over and underdispersion using AER (Kleiber and Zeileis, 2008), if the model was underdispersed a gaussian distribution was used instead. The data was never found to be overdispersed and hence no negative binomial was used. For each of the four species, 30 flies were released per experiment and replicated 15 times. The effects of the different treatments were tested using estimated marginal means using package emmeans (Lenth, 2022).
Electrophysiological data were collected and responses in each run were annotated using the software GC-EAD 2012 (Ver. 1.2.4. Syntech Inc. Kirchzarten, Germany). To be able to compare response profiles across runs and between species, responses were normalized as per (Biasazin et al., 2019), by expressing individual responses as a fraction of the average response to all compounds.
The amount in ng/μl of volatiles in the yeast was determined by calculating the ratio of peak areas in the samples with known injected amounts of (10 ng) of pentyl acetate, ethyl hexanoate and ethyl octanoate, which were compounds also present in the yeast volatilome. The mean difference between the amount in ng/μl of GC-EAD active compounds (n = 16) were analyzed using one-way anova followed by a TukeyHSD post-hoc comparison.
The average peak areas of the volatiles emanated from 16 injections of the three developmental ages (1-, 8-, and 15-days) of the yeast were visualized in a heatmap with a dendrogram separating the variables based on Jaccard dissimilarities indices using the package vegan (Oksanen et al., 2022), the same dissimilarity indices were used for non-metric multidimensional scaling (NMDS) to further analyze the volatilome. All analysis, visualizations and statistics were performed using (R Core Team, 2020).
Results
Headspace profile of yeast cultures of different age
Gas Chromatography -MS profiles revealed both qualitative as well as quantitative differences in the chemical profiles of differentially aged yeast culture. Volatiles of the active (1-day old) yeast were out grouped from the older cultures (8- and 15-days) both in heatmap 3 visualization using Euclidean distance matrix (Figure 1) and non-metric multidimensional analysis (NMDS) using Jaccard distance matrix (Supplementary Figure 2). The quantitative differences were more discernible than the qualitative differences (Figure 1 and Supplementary Figure 4). That is, 86% of the compounds present in the headspace of active yeast culture (1-day old) volatiles, were also found in older yeast cultures (8- and 15-days). Out of the 48 compounds in the 8-days yeast culture 56% were present in 1-day and 60% in 15-days yeast culture. Yet the absolute amounts and ratios differed with the age of the yeast.
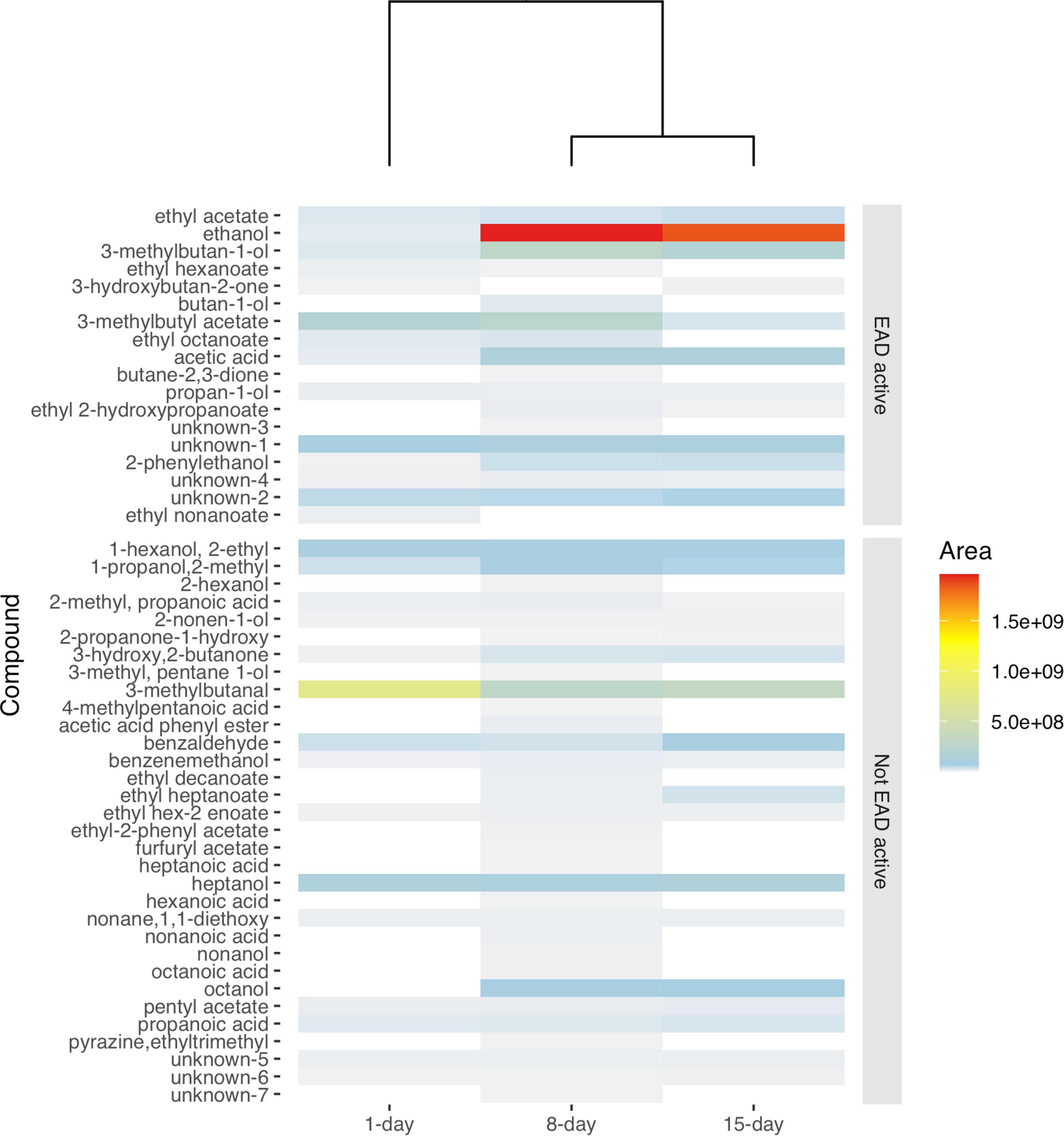
Figure 1. Heatmap showing the average peak area of volatile organic compounds emanating from 16 injections of yeast culture aged for 1-, 8-, and 15-days. Note that volatiles emanated from 8 to 15-days old yeast cultures are grouped together separated from a 1-day old yeast. EAD active compounds are arranged on top of the heatmap. The legend bar indicates the relationship between the averaged peak area values and intensity of colors from low (0) to high (1.5e + 09) with a dendrogram reflecting dissimilarities based on Euclidean distances. “unknown” compounds were those for which a spectrum query in the MS library did not return reliable candidate compounds.
As the yeast cultures became older, more, and higher amounts of acids and alcohols were observed in the headspace (Figure 1 and Supplementary Table 1). For example, in the antennally active compounds, the amounts of acetic acid, ethanol, 2-phenylethanol and 3-methylbutan-1-ol (Figure 2), were lower in 1-day compared to 8-days old media (p < 0.001). The amount of 1-butanol-3-methyl acetate was significantly lower in 15-days old yeast volatiles (P < 0.001). Subsequent TukeyHSD analysis revealed no significant differences between the 8 and 15-days old yeast volatiles in the amounts of acetic acid, ethanol, 2-phenylethanol and 3-methylbutan-1-ol. There was also no significant difference in the amounts of 1-butanol-3-methyl acetate between 1 and 8-days old yeast volatiles (p > 0.05). The amount of ethyl acetate and propan-1-ol in the three developmental ages of the yeast were not significantly different (p > 0.05) in both cases. Ethyl hexanoate and ethyl octanoate were not detected in volatilome of the 15-days old yeast culture and ethyl 2-hydroxypropanoate was not detected in volatilome of the 1-day old yeast culture. Comparison between the two ages revealed a significant difference in the amounts of ethyl hexanoate (p < 0.001) and ethyl 2-hydroxypropanoate (p = 0.03), but not in the amounts of ethyl octanoate (p = 0.19). Since some compounds, such as ethyl nonanoate and two unidentified compounds, elicited antennal response only from active (1-day old) yeast culture (Figures 2, 3), and compounds such as butan-1-ol and butane 2,3-dione elicited antennal response only from the 8-days old yeast culture (Figures 2, 3), no statistical comparison was performed for these compounds.
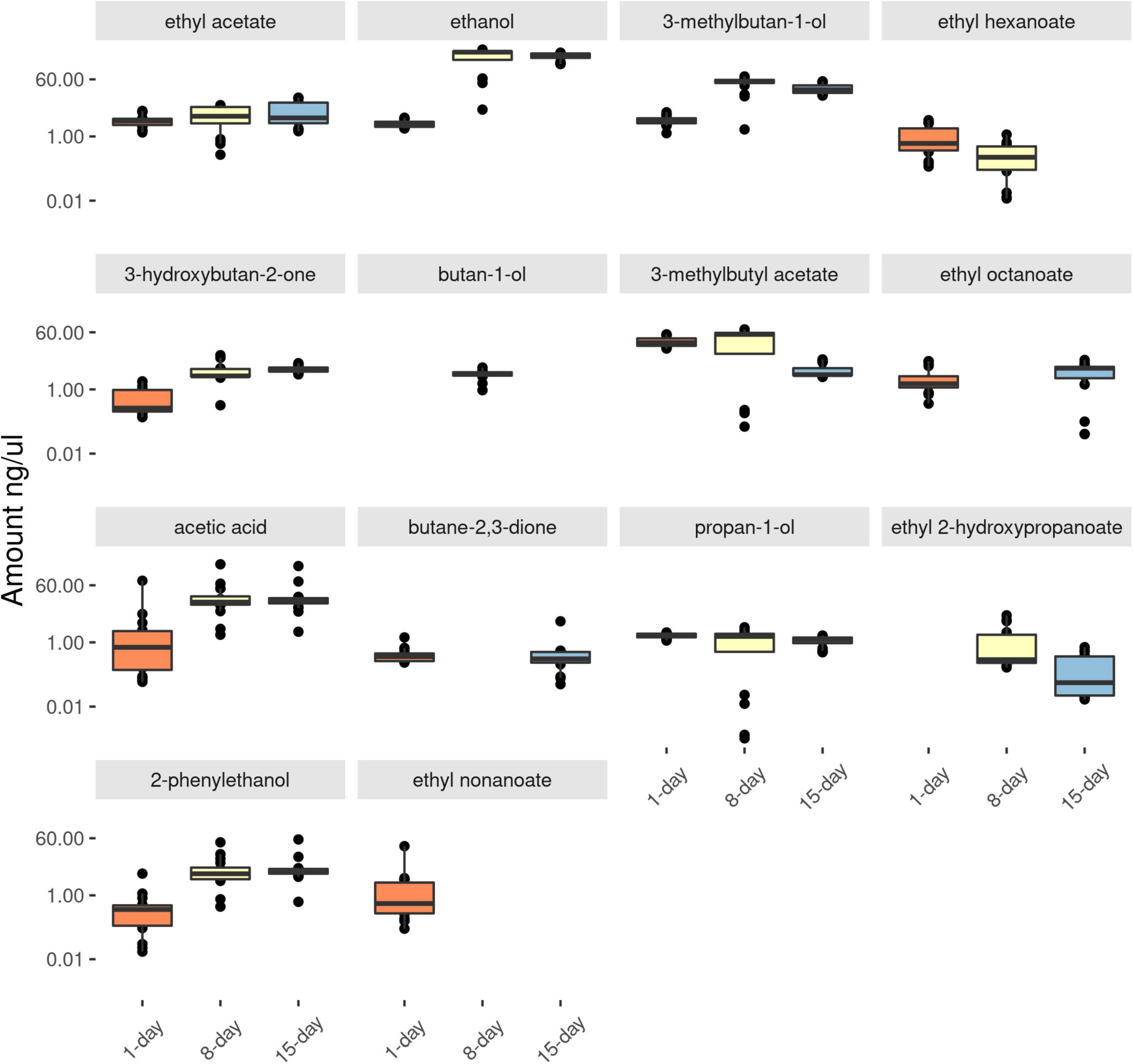
Figure 2. Log transformed value of the amount in ng/μl of GC-EAD active compounds identified from differentially aged (for 1-, 8-, and 15-days) yeast cultures. Number of replicates = 16. Boxplots represent the lower whisker, first quartile, the median, the third quartile and the upper whisker with a 95% confidence interval.
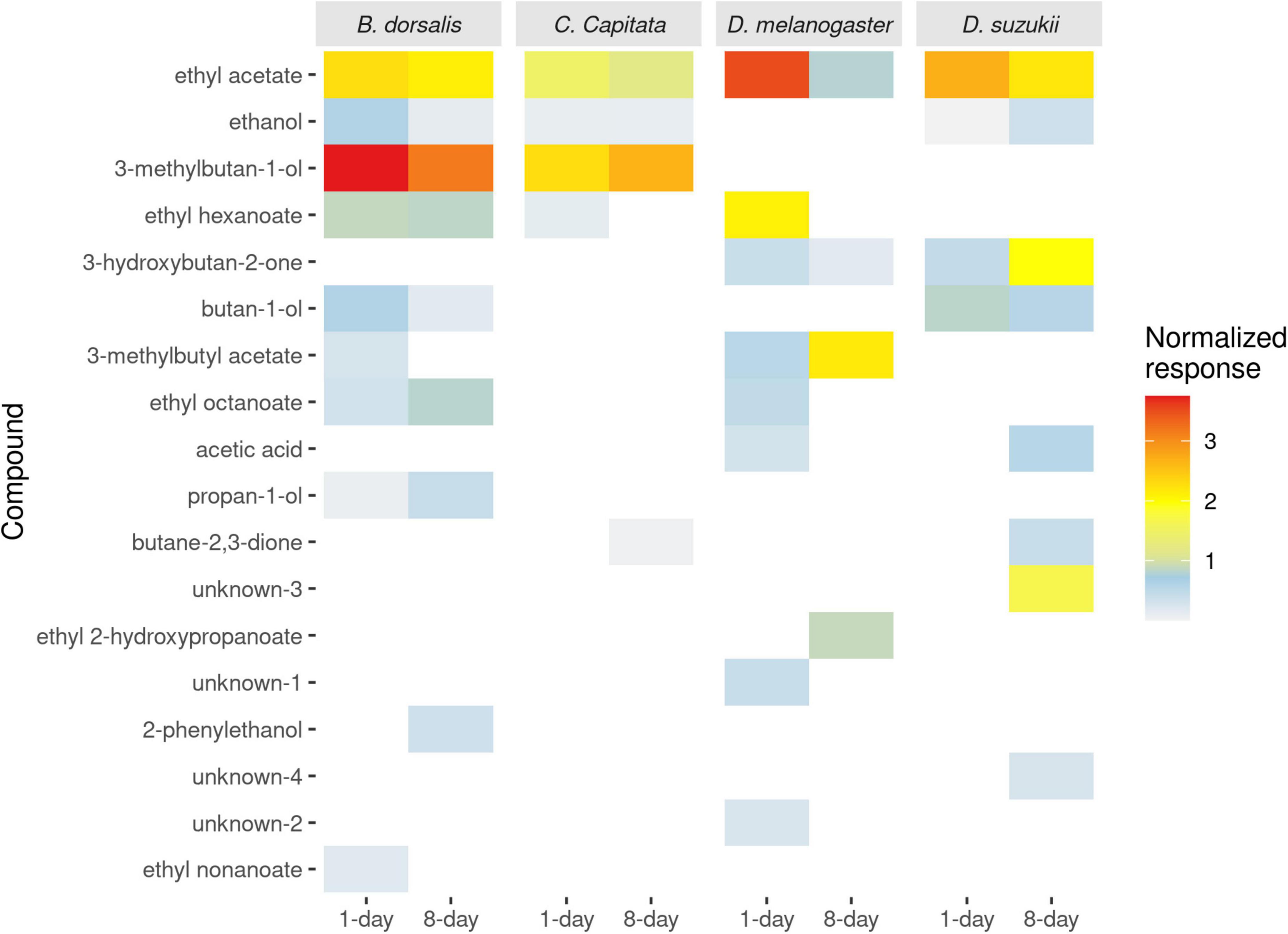
Figure 3. The average normalized antennal responses of two drosophila species (Drosophila melanogaster and Drosophila suzukii) and two tephritid species (Bactrocera dorsalis and Ceratitis capitata) to volatile compounds from differentially aged yeast culture (1- and 8-days old yeast cultures).
Attraction of flies to yeast culture
In a 6-choice olfactory setup, D. melanogaster and B. dorsalis fruit flies manifested distinctive preferences for differentially aged yeast culture. Although all developmental ages were significantly attractive compared to uninoculated minimal media (p < 0.001), distinctive preferences were observed for the different ages of the cultures. While D. melanogaster was more attracted to 1-day old yeast cultures, B. dorsalis preferred 8- and 15-day yeast cultures (Figure 4). There was no significant difference in preference of flies between 8- and 15-day old cultures (p = 0.923 for B. dorsalis and p = 0.955 for D. melanogaster). Like that of D. melanogaster, D. suzukii flies were significantly more attracted to active (1-day old) yeast culture compared to older (8- and 15-day) yeast cultures (p < 0.0001). There was no significant difference between older yeast cultures (8- and 15-day old) in D. suzukii (p = 0.999, Figure 4). However, C. capitata differed in that it preferred the 15-day old culture less than the 8-day old culture (p = 0.027). In addition, 1- and 8-day old cultures were equally attractive (p = 0.225).
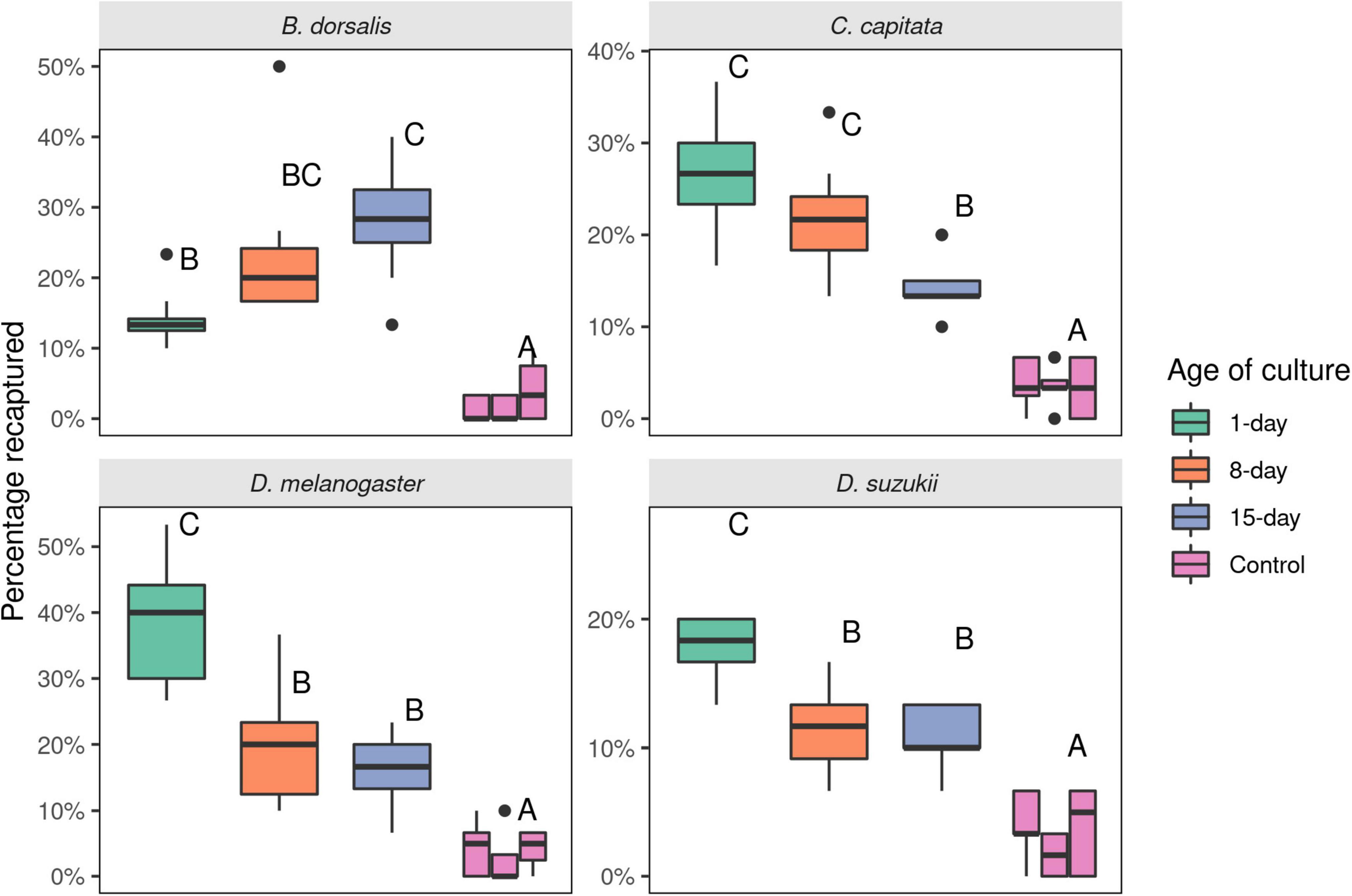
Figure 4. Number of Bactrocera dorsalis, Ceratitis capitata, Drosophila melanogaster, and Drosophila suzukii flies attracted to 1-, 8-, and 15-days old cultures of the yeast Saccharomyces cerevisiae in a 6-choice olfactometer assay. The control (minimal media) was placed in between each yeast treatment. Thirty flies were released per experiment and eight replicates were made for each species. Different letters represent statistical differences. Boxplots show the median, the first and third quartile, while the lower and upper whiskers indicate the 95% confidence interval.
Electrophysiology
Since only 3 compounds (ethyl acetate, ethanol and 3-methylbutan-ol) from the 15-day old yeast cultures induced antennal responses that were already present in the 8-day culture, they were not included in the graphs. Electrophysiological responses of fruit flies to the 1- and 8-days old yeast volatiles were analyzed. In total, 18 compounds elicited antennal response in either of the four species of fruit flies tested, out of which 14 compounds were identified (Figure 3). Mass spectra is provided for the 4 unknown compounds (Supplementary Figure 5). Ethyl acetate, which is found in active and old yeast culture, is the only compound that elicited antennal response in all fruit flies.
Bactrocera dorsalis responded to 10 of the 18 compounds, 9 from 1- to 8 from 8-days old cultures. Whereas ethyl nonanoate and 3-methylbutyl acetate elicited visible responses in B. dorsalis antennae only from 1-day old cultures, 2-phenylethanol elicited detectable responses only from 8-days old cultures. That is, B. dorsalis antennae detected 7 compounds shared between the two development ages of the yeast (Figure 3), although ratios of these compounds differed significantly between 1- and 8-days old cultures.
Ceratitis capitata responded to only 5 of the 18 compounds, with 4 responses to volatiles from 1-day old cultures as well as 8-days old cultures. While ethyl hexanoate elicited responses in C. capitata only from 1-day old cultures, butane 2,3-dione elicited responses only from 8-days old cultures, leaving three compounds shared between the two yeast cultures (Figure 3).
Drosophila melanogaster responded to 9 out of the 18 compounds. Eight were detected in the headspace of 1-day old and 4 in 8-days old headspace. Ethyl octanoate, ethyl hexanoate and two of the unknown compounds (unknown-01 and unknown-02) elicited response in D. melanogaster only from 1-day old yeast, whereas ethyl 2-hydroxypropanoate elicited response only from 8-days old yeast. Four of the 9 compounds were commonly detected among the two substrates (Figure 3).
Drosophila suzukii responded to 8 out of the 18 compounds, 3 compounds were detected from 1- to 8 from 8-days old yeast. Two of the unknowns (unknown-3 and unknown-4) and 3-hydroxybutan-2-one elicited response in D. suzukii only from 8-days old yeast. Four compounds were commonly detected among the two substrates (Figure 3).
3-methylbutyl acetate, ethanol and ethyl acetate were commonly detected in Tephritidae, whereas acetic acid, ethyl acetate and 3-hydroxybutan-2-one were commonly detected in Drosophilidae. Synthetic blends of these shared antennally active compounds in combination with uniquely detected compounds from each species were used for further behavioral analysis.
Behavior with synthetic compounds
Compounds that evoked distinctive antennal responses contributed to the differential attractiveness of 1- and 8-days old yeast culture. Adding uniquely detected compounds found in active (1-day old) or aged (8-days old) volatiles to the base blend restored attraction, despite variable responses between different species of fruit flies.
For B. dorsalis, adding uniquely detected compounds from the 8-days old yeast volatiles to active cultures (1-day old) was as attractive as uniquely detected compounds from the 8-days yeast volatile added to the base blend (p = 0.99). The base blend with or without 1-day unique compounds was less attractive, with no difference between the two (Figure 5, p = 0.67). The minimal media was not attractive (p < 0.001).
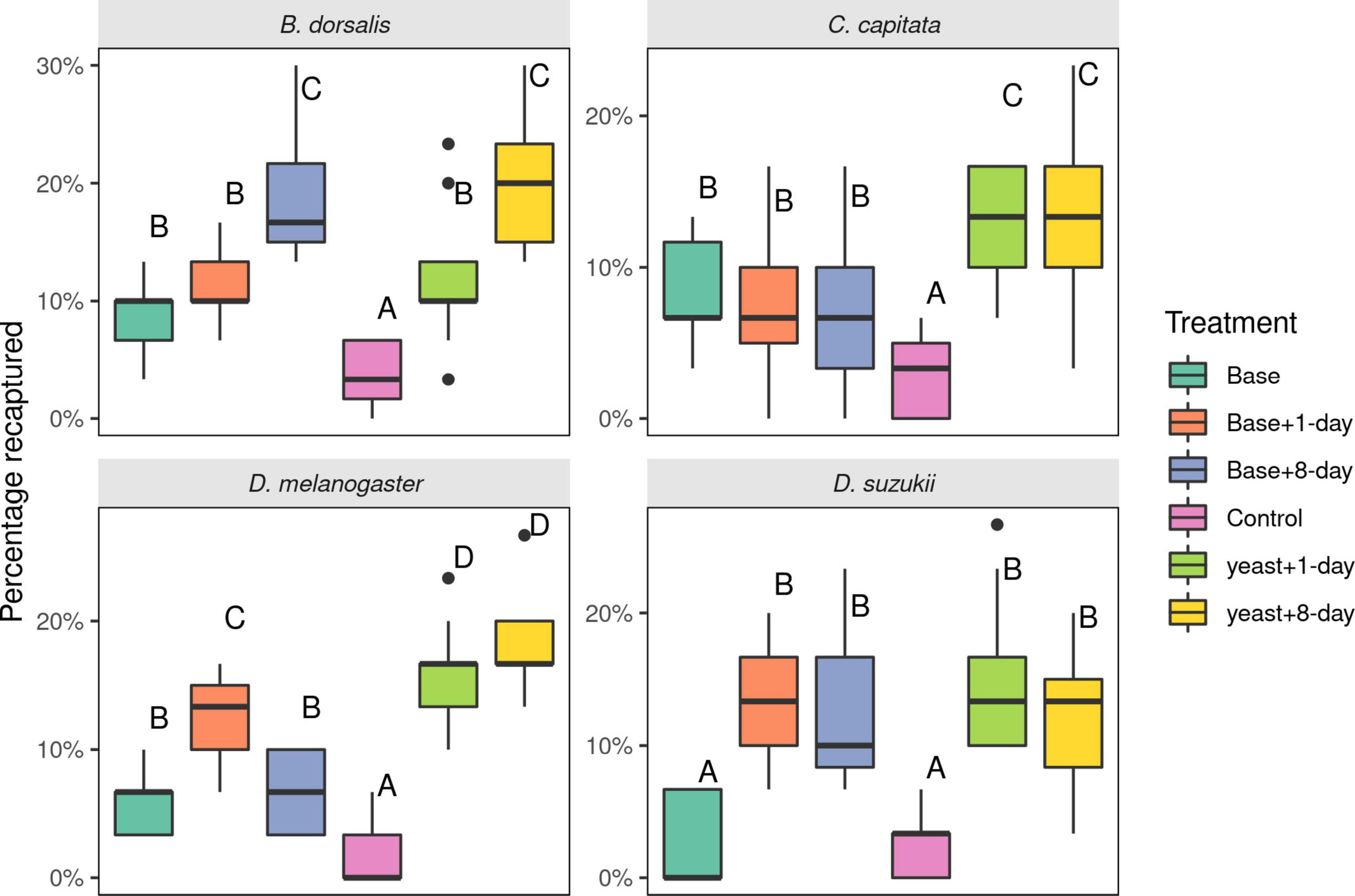
Figure 5. Number of Drosophilidae and Tephritidae fruit flies attracted to synthetic mixes of compounds with and without actively fermenting yeast. The blend compositions are described in Table 1. Thirty flies were released per experiment and eight replicates were made for each species. Different letters indicate statistical differences. Boxplots show the median, the first and third quartile, while the lower and upper whiskers indicate the 95% confidence interval.
Ceratitis capitata flies were most attracted to active 1-day old cultures with uniquely detected compounds from both 1- and 8-days cultures, with no statistical difference between the two (p = 0.975). Flies were also attracted to the base blends with or without addition of unique compounds (with no difference between them, p = 0.99). All treatments were more attractive compared to the control (minimal media, p < 0.05).
Drosophila melanogaster was highly attracted to 1-day old unique volatiles added to the base blend compared to volatiles of 8-days old medium added to the base blend (p < 0.001). D. melanogaster was equally attracted to the active yeast culture with unique compounds from 1- to 8-days old yeast cultures (p = 0.433). The base blend alone or with 8-day unique compounds was marginally attractive with no difference between the two (p = 0.98). All treatments were more attractive than minimal media (p < 0.005).
Drosophila suzukii did not uniquely detect volatiles from the 1-day old yeast cultures. Instead, compounds that elicited unique responses in D. melanogaster from 1-day old cultures (ethyl octanoate and ethyl hexanoate) were added, given the reportedly large overlap in olfactory sensitivities between the species. Adding uniquely detected compounds to either the base blend or the active yeast rendered blends equally attractive to D. suzukii flies (Figure 5). Attraction of D. suzukii flies to the base blend was not statistically different from that of minimal media (p = 0.99).
Discussion
Fermenting yeasts release organic volatile compounds that attract insects. Insect taxa differ, however, in which of these volatiles induce sensory and behavioral responses (Phelan and Lin, 1991; Cha et al., 2012; Babcock et al., 2019; Larsson Herrera et al., 2020; Đurović et al., 2021). How the age of yeast cultures contributes to observed variation is obscure. However, it is well known that with age cultures begin to lyse (Babayan and Bezrukov, 1985) and the odor profile changes along with that (Figure 1). In this study, we examined how organic volatile profile changes with the developmental ages of the yeast broth cultures and how fruit flies of different families (drosophilidae and tephritidae) react to the changes in volatile profile over time. We found that, while the headspace of yeast cultures was generally attractive at all ages for all species, actively fermenting 1-day old yeast cultures were found to be more attractive to drosophilids (D. melanogaster and D. suzukii). In contrast, the tephritids tested were equally or more attracted to older yeast cultures. Further, adding volatile components from the attractive yeast substrate to the less attractive substrate restored attraction of B. dorsalis and D. melanogaster fruit flies.
Yeasts chemically communicate with insects. This supports their dispersal by insects, it in return provides nutritional services to the insect that are important for sexual maturation and ovary development. The attraction of fruit flies toward yeast substrates is divided spatially and temporally (Atkinson and Shorrocks, 1981). Drosophilids benefit from early arriving, (Shorrocks and Bingley, 1994), whereas tephritids benefit more from lysed yeast cells (Vargas and Prokopy, 2006). In this study we found that the development age of the yeast influenced its volatile profile (Figure 1), which in turn differentially affected the behavior of fruit flies, drosophilids being more attracted to the early, actively fermenting substrates (Figure 4). Besides the need for early arrival for drosophilids, the differential attraction may also reflect differences in the life histories and dietary needs of drosophilids and tephritids. While drosophilids can ingest dry yeast cells (Shihata and Mrak, 1951), the labeller filtering mechanism of tephritids do not allow that, substrates as large as yeast must first be liquefied by regurgitating liquid from the crop (Vijaysegaran et al., 1997), a process which facilitates lysis.
Acidity of substrates plays an important role in the survival of insects. In D. melanogaster acetic acid is a strong tastant and the fly’s response to this compound is dependent on the fly’s physiological state, whether flies are hungry or well-fed (Devineni et al., 2019). For flies that are well-fed, acetic acid is perceived as a toxin indicator and it becomes repellent, whereas for hungry fruit flies, acetic acid is perceived as a reliable source of sugar and becomes very attractive (Devineni et al., 2019; Rimal et al., 2019). Further, at higher acetic acid concentration repulsion overrules attraction of D. melanogaster (Joseph et al., 2009), and acetic acid tolerance of D. suzukii is much lower than D. melanogaster (Durkin et al., 2021). In this study, more acids were observed as the yeast culture got older, specifically acetic acid, which was detected by antennae of both Drosophila flies was found to be abundant in the 8- and 15-days old yeast cultures (Figure 2). The higher amount of acetic acid in older yeast volatiles (Figures 1, 2) could have been a reason for why drosophilids preferred active yeast culture (1-day old) compared to (8- and 15-days) older yeast cultures (Figures 3, 4).
Stress due to weak carboxylic acids such as acetic acid and alcohols are known to induce yeast cell death and hence autolysis (Pinto et al., 1989; Jing et al., 2018). Autolysis yeast cells are known to attract Tephritidae fruit flies including B. dorsalis and Z. cucurbitae (Vargas and Prokopy, 2006). In a field trial, autolysis cells have outperformed baker’s yeast in capturing B. occipitalis and B. philippinensis flies (Tomambo, 2011). These results are consistent with our findings where B. dorsalis flies were more attracted to older yeast cultures compared to active yeast cultures (Figure 4). Further, the older cultures were abundant not only in acetic acid but also alcohols including ethanol, 3-methylbutan-1-ol and 2-phenylethanol, which are compounds that can potentially stress the yeast cell and cause cell death and autolysis. C. capitata was also attracted to older yeast culture (8-days old), but the bias was not as strong as in B. dorsalis. A recent study by Grout and Stephen (2021) reported that autolysis yeast cells were not suitable for trapping C. capitata flies, confirming our observation that C. capitata flies were less attracted toward the 15-days old yeast culture (Figure 3).
Curiously, in our study 15-days old yeast cultures were as attractive as the 8-days old yeast for B. dorsalis (Figure 4), despite the larger number of compounds that elicited antennal responses in the latter. This apparent discrepancy may be due to certain compounds being present in too low amounts to induce visible EAD responses. Indeed, our analyses show that the headspace of 15-days old media contained some antennally active compounds in much reduced quantities, perhaps too low to elicit visible antennal responses in our EAD setup. The decline in quantity of some ester compounds including ethyl hexanoate and ethyl nonanoate or absence of ethyl octanoate from 15-days older yeast cultures may have contributed to such discrepancies (Figures 1, 2). Nitrogenous compounds such as ammonia and amines, that may have further contributed to the differential attraction of drosophilids and tephritids, are not detectable with conventional GC (Namieśnik et al., 2003; Hartonen et al., 2018). Although a powerful tool in mapping out the evolutionary ecology of olfactomes and their translation into attractants (Biasazin et al., 2019), GC-EAD has some blind spots which require further research.
Fruit flies are attracted to fermentation and protein sources, which provide essential nutrients for sexual maturation (Drew and Yuval, 1999). Although food baits are used actively in fruit fly detection surveillance and monitoring programs, these are not target specific and not as effective as male lures (Epsky et al., 2014). This signifies the need to develop selective and more attractive lures that can be used for monitoring and control of fruit flies. The 14 compounds that elicited antennal responses in this study could contribute to selective lure development. The antennally active compounds are dominated by esters (6 out of 14) and followed by alcohols (5 out of 14) for which fruit flies are known to be attracted to as these are either associated with microbes, which are indicators of feeding substrates (Bueno et al., 2019), or fruits that host fruit flies (Biasazin et al., 2014). In this study, using antennally active compounds, we show that species selective blends can be formulated from generically attractive yeast cultures, this supports findings of Larsson Herrera et al. (2020), who showed that through tweaking the composition and ratio of microbial volatiles, lures could be formulated that attracted the grapevine moth, Lobesia botrana. Studies such as the current can help in tweaking blend compositions to increase both the attractiveness and selectivity of synthetic lures.
Data availability statement
The original contributions presented in this study are included in the article/Supplementary material, further inquiries can be directed to the corresponding author.
Author contributions
TB wrote and prepared the original draft and revised the manuscript. SH, FK, and TD reviewed and edited the manuscript. All authors conceptualized the manuscript and were involved in experimental design and data collection, and approved the final version for publication.
Funding
We thank Ekhaga Stiftelsen (2018-90, TD) and Vetenskaprådet (2019-04421, TB), and Schlumverger Foundation (FK) for providing funding.
Acknowledgments
We thank the International Atomic and Energy Agency, IAEA, in Vienna for providing tephritid colony material. We would also like to thank Ilich Figueroa for drawing the six-choice olfactometer setup.
Conflict of interest
The authors declare that the research was conducted in the absence of any commercial or financial relationships that could be construed as a potential conflict of interest.
Publisher’s note
All claims expressed in this article are solely those of the authors and do not necessarily represent those of their affiliated organizations, or those of the publisher, the editors and the reviewers. Any product that may be evaluated in this article, or claim that may be made by its manufacturer, is not guaranteed or endorsed by the publisher.
Supplementary material
The Supplementary Material for this article can be found online at: https://www.frontiersin.org/articles/10.3389/fevo.2022.999762/full#supplementary-material
References
Atkinson, W. D., and Shorrocks, B. (1981). Competition on a divided and ephemeral resource: a simulation model. J. Animal Ecol. 50:461. doi: 10.2307/4067
Babayan, T. L., and Bezrukov, M. G. (1985). Autolysis in yeasts. Acta Biotechnol. 5, 129–136. doi: 10.1515/9783112580561-004
Babcock, T., Borden, J. H., Gries, R., Carroll, C., Lafontaine, J. P., Moore, M., et al. (2019). Inter-kingdom signaling — symbiotic yeasts produce semiochemicals that attract their yellowjacket hosts. Entomol. Exp. Appl. 167, 220–230. doi: 10.1111/eea.12752
Baker, A. C., Seibert, H. C., Stone, W. E., Plummer, C. C., and Mcphail, M. (1945). A review of studies on the Mexican fruit fly and related Mexican species. Quarterly Rev. Biol. 20, 276–276. doi: 10.1086/394916
Becher, P. G., Flick, G., Rozpȩdowska, E., Schmidt, A., Hagman, A., Lebreton, S., et al. (2012). Yeast, not fruit volatiles mediate Drosophila melanogaster attraction, oviposition and development. Funct. Ecol. 26, 822–828. doi: 10.1111/j.1365-2435.2012.02006.x
Becher, P. G., Hagman, A., Verschut, V., Chakraborty, A., Rozpȩdowska, E., Lebreton, S., et al. (2018). Chemical signaling and insect attraction is a conserved trait in yeasts. Ecol. Evol. 8, 2962–2974. doi: 10.1002/ece3.3905
Begon, M., and Shorrocks, B. (1978). The feeding- and breeding-sites of Drosophila obscuraFallén andD. subobscuraCollin. J. Nat. History 12, 137–151. doi: 10.1080/00222937800770031
Biasazin, T., Chernet, H., Herrera, S., Bengtsson, M., Karlsson, M., Lemmen-Lechelt, J., et al. (2018). Detection of volatile constituents from food lures by tephritid fruit flies. Insects 9:119. doi: 10.3390/insects9030119
Biasazin, T. D., Karlsson, M. F., Hillbur, Y., Seyoum, E., and Dekker, T. (2014). Identification of host blends that attract the african invasive fruit fly. Bactrocera invadens. J. Chem. Ecol. 40, 966–976. doi: 10.1007/s10886-014-0501-6
Biasazin, T. D., Larsson Herrera, S., Kimbokota, F., and Dekker, T. (2019). Translating olfactomes into attractants: shared volatiles provide attractive bridges for polyphagy in fruit flies. Ecol. Lett. 22, 108–118. doi: 10.1111/ele.13172
Blackwell, M. (2017). “Made for each other: ascomycete yeasts and insects,” in The Fungal Kingdom, eds J. Heitman, B. J. Howlett, P. W. Crous, E. H. Stukenbrock, T. Y. James, and N. A. R. Gow (Washington, DC: ASM Press), 945–962.
Broderick, N. A., and Lemaitre, B. (2012). Gut-associated microbes of Drosophila melanogaster. Gut Microbes 3, 307–321. doi: 10.4161/gmic.19896
Bueno, E., Martin, K. R., Raguso, R. A., Mcmullen, J. G. II, Hesler, S. P., Loeb, G. M., et al. (2019). Response of wild spotted wing Drosophila (Drosophila suzukii) to microbial volatiles. J. Chem. Ecol. 46, 688–698. doi: 10.1007/s10886-019-01139-4
Cha, D. H., Adams, T., Rogg, H., and Landolt, P. J. (2012). Identification and field evaluation of fermentation volatiles from wine and vinegar that mediate attraction of spotted wing Drosophila, Drosophila suzukii. J. Chem. Ecol. 38, 1419–1431. doi: 10.1007/s10886-012-0196-5
Chang, C. L. (2009). Evaluation of yeasts and yeast products in larval and adult diets for the oriental fruit fly,Bactrocera dorsalis, and adult diets for the medfly,Ceratitis capitata, and the Melon Fly,Bactrocera curcurbitae. J. Insect. Sci. 9:23. doi: 10.1673/031.009.2301
Davis, T. S., Crippen, T. L., Hofstetter, R. W., and Tomberlin, J. K. (2013). Microbial volatile emissions as insect semiochemicals. J. Chem. Ecol. 39, 840–859. doi: 10.1007/s10886-013-0306-z
Deutscher, A. T., Reynolds, O. L., and Chapman, T. A. (2016). Yeast: an overlooked component of Bactrocera tryoni(Diptera: Tephritidae) larval gut microbiota. J. Econ. Entomol. 110, 298–300. doi: 10.1093/jee/tow262
Devineni, A. V., Sun, B., Zhukovskaya, A., and Axel, R. (2019). Acetic acid activates distinct taste pathways in Drosophila to elicit opposing, state-dependent feeding responses. eLife 8:e47677. doi: 10.7554/elife.47677
Drew, R., and Yuval, B. (1999). “The evolution of fruit fly feeding behavior,” in Fruit Flies (Tephritidae), eds M. Aluja and A. L. Norrbom (Boca Raton, FL: CRC Press), 731–749.
Drew, R. A. I., Courtice, A. C., and Teakle, D. S. (1983). Bacteria as a natural source of food for adult fruit flies (Diptera: Tephritidae). Oecologia 60, 279–284. doi: 10.1007/bf00376839
Durkin, S. M., Chakraborty, M., Abrieux, A., Lewald, K. M., Gadau, A., Svetec, N., et al. (2021). Behavioral and genomic sensory adaptations underlying the pest activity of Drosophila suzukii. Mol. Biol. Evol. 38, 2532–2546. doi: 10.1093/molbev/msab048
Đurović, G., Van Neerbos, F. A. C., Bossaert, S., Herrera-Malaver, B., Steensels, J., Arnó, J., et al. (2021). The pupal parasitoid Trichopria drosophilae is attracted to the same yeast volatiles as its adult host. J. Chem. Ecol. 47, 788–798. doi: 10.1007/s10886-021-01295-6
Epsky, N. D., Kendra, P. E., and Schnell, E. Q. (2014). “History and development of food-based attractants,” in Trapping and the Detection, Control, and Regulation of Tephritid Fruit Flies, eds T. Shelly, N. Epsky, E. B. Jang, J. Reyes-Flores, and R. Vargas (Dordrecht: Springer), 75–118.
Fanson, B. G., and Taylor, P. W. (2012). Additive and interactive effects of nutrient classes on longevity, reproduction, and diet consumption in the Queensland fruit fly (Bactrocera tryoni). J. Insect Physiol. 58, 327–334. doi: 10.1016/j.jinsphys.2011.11.002
Fogleman, J. C., Starmer, W. T., and Heed, W. B. (1981). Larval selectivity for yeast species by Drosophila mojavensis in natural substrates. Proc. Natl. Acad. Sci. U S A. 78, 4435–4439. doi: 10.1073/pnas.78.7.4435
Ganter, P. F. (1988). The vectoring of cactophilic yeasts by Drosophila. Oecologia 75, 400–404. doi: 10.1007/bf00376943
Grout, T. G., and Stephen, P. R. (2021). Are yeast autolysate attractants for ceratitis species (diptera: Tephritidae) in south africa more attractive and palatable than a currently used protein attractant? J. Econ. Entomol. 114, 1005–1008. doi: 10.1093/jee/toaa324
Hagen, K. S., and Finney, G. L. (1950). A food supplement for effectively increasing the fecundity of certain tephritid species. J. Econ. Entomol. 43, 735–735. doi: 10.1093/jee/43.5.735
Hamby, K. A., Hernández, A., Boundy-Mills, K., and Zalom, F. G. (2012). Associations of yeasts with spotted-wing Drosophila (Drosophila suzukii; Diptera: Drosophilidae) in cherries and raspberries. Appl. Environ. Microbiol. 78, 4869–4873. doi: 10.1128/aem.00841-12
Hartonen, K., Helin, A., Parshintsev, J., and Riekkola, M.-L. (2018). Problems caused by moisture in gas chromatographic analysis of headspace SPME samples of short-chain amines. Chromatographia 82, 307–316. doi: 10.1007/s10337-018-3641-y
Heed, W. B., Starmer, W. T., Miranda, M., Miller, M. W., and Phaff, H. J. (1976). An analysis of the yeast flora associated with cactiphilic Drosophila and their host plants in the sonoran desert and its relation to temperate and tropical associations. Ecology 57, 151–160. doi: 10.2307/1936406
Hodge, S., Arthur, W., and Mitchell, P. (1996). Effects of temporal priority on interspecific interactions and community development. Oikos 76:350. doi: 10.2307/3546207
Jing, H., Liu, H., Zhang, L., Gao, J., Song, H., and Tan, X. (2018). Ethanol induces autophagy regulated by mitochondrial ROS in Saccharomyces cerevisiae. J. Microbiol. Biotechnol. 28, 1982–1991. doi: 10.4014/jmb.1806.06014
Joseph, R. M., Devineni, A. V., King, I. F. G., and Heberlein, U. (2009). Oviposition preference for and positional avoidance of acetic acid provide a model for competing behavioral drives in Drosophila. Proc. Natl. Acad. Sci. U S A. 106, 11352–11357. doi: 10.1073/pnas.0901419106
Lam, S. S. T. H., and Howell, K. S. (2015). Drosophila-associated yeast species in vineyard ecosystems. FEMS Microbiol. Lett. 362:fnv170. doi: 10.1093/femsle/fnv170
Larsson Herrera, S., Rikk, P., Köblös, G., Szelényi, M. O., Molnár, B. P., Dekker, T., et al. (2020). Designing a species-selective lure based on microbial volatiles to target Lobesia botrana. Sci. Rep. 10:6512. doi: 10.1038/s41598-020-63088-3
Lenth, R. V. (2022). CRAN. Package Emmeans. Available online at: https://CRAN.R-project.org/package=emmeans (accessed July 24, 2022).
Ljunggren, J., Borrero-Echeverry, F., Chakraborty, A., Lindblom, T. U., Hedenström, E., Karlsson, M., et al. (2019). Yeast volatomes differentially effect larval feeding in an insect herbivore. Appl. Environ. Microbiol. 85:e01761-19. doi: 10.1128/AEM.01761-19
Madden, A. A., Epps, M. J., Fukami, T., Irwin, R. E., Sheppard, J., Sorger, D. M., et al. (2018). The ecology of insect-yeast relationships and its relevance to human industry. Proc. R. Soc. B Biol. Sci. 285:20172733. doi: 10.1098/rspb.2017.2733
Meats, A., and Kelly, G. L. (2008). Relation of constant, daily fluctuating, and ambient feeding temperature to daily and accumulated consumption of yeast autolysate and sucrose by female Queensland fruit fly. Entomol. Exp. Appl. 129, 87–95. doi: 10.1111/j.1570-7458.2008.00755.x
Namieśnik, J., Jastrzȩbska, A., and Zygmunt, B. (2003). Determination of volatile aliphatic amines in air by solid-phase microextraction coupled with gas chromatography with flame ionization detection. J. Chromatogr. A 1016, 1–9. doi: 10.1016/s0021-9673(03)01296-2
Oksanen, J., Gavin, L. S., Blanchet, F. G., Kindt, R., Legendre, P., Minchin, P. R., et al. (2022). Package “vegan”. Community Ecology Package. Package vegan. Available online at: https://CRAN.R-project.org/package=vegan (accessed July 24, 2022).
Phelan, P. L., and Lin, H. (1991). Chemical characterization of fruit and fungal volatiles attractive to dried-fruit beetle, Carpophilus hemipterus (L.) (Coleoptera: Nitidulidae). J. Chem. Ecol. 17, 1253–1272. doi: 10.1007/bf01402948
Pinto, I., Cardoso, H., Leão, C., and van Uden, N. (1989). High enthalpy and low enthalpy death in Saccharomyces cerevisiae induced by acetic acid. Biotechnol. Bioeng. 33, 1350–1352. doi: 10.1002/bit.260331019
Prokopy, R., Papaj, D., Díaz-Fleischer, F., Aluja, M., and Norrbom, A. (1999). “Evolution of fruit fly oviposition behavior,” in Fruit Flies (Tephritidae), (Boca Raton, FL: CRC Press).
R Core Team (2020). R: A Language and Environment for Statistical Computing. Vienna: R Foundation for Statistical Computing. Available online at: https://www.R-project.org/ (accessed May 24, 2022).
Rehermann, G., Spitaler, U., Sahle, K., Cossu, C. S., Donne, L. D., Bianchi, F., et al. (2021). Behavioral manipulation of Drosophila suzukii for pest control: high attraction to yeast enhances insecticide efficacy when applied on leaves. Pest. Manag. Sci. 78, 896–904. doi: 10.1002/ps.6699
Reuter, M., Bell, G., and Greig, D. (2007). Increased outbreeding in yeast in response to dispersal by an insect vector. Curr. Biol. 17, R81–R83. doi: 10.1016/j.cub.2006.11.059
Rimal, S., Sang, J., Poudel, S., Thakur, D., Montell, C., and Lee, Y. (2019). Mechanism of acetic acid gustatory repulsion in Drosophila. Cell Rep. 26, 1432–1442.e4. doi: 10.1016/j.celrep.2019.01.042
Rosewell, J., Shorrocks, B., and Edwards, K. (1990). Competition on a divided and ephemeral resource: testing the assumptions. I. aggregation. J. Animal Ecol. 59, 977. doi: 10.2307/5026
Ruebenbauer, A., Schlyter, F., Hansson, B. S., Löfstedt, C., and Larsson, M. C. (2008). Genetic variability and robustness of host odor preference in Drosophila melanogaster. Curr. Biol. 18, 1438–1443. doi: 10.1016/j.cub.2008.08.062
Schiabor, K. M., Quan, A. S., and Eisen, M. B. (2014). Saccharomyces cerevisiae mitochondria are required for optimal attractiveness to Drosophila melanogaster. PLoS One 9:e113899. doi: 10.1371/journal.pone.0113899
Shihata, A. E.-T. A., and Mrak, E. M. (1951). The fate of yeast in the digestive tract of Drosophila. Am. Nat. 85, 381–383. doi: 10.1086/281692
Shorrocks, B., and Bingley, M. (1994). Priority effects and species coexistence: experiments with fungal-breeding Drosophila. J. Animal Ecol. 63:799. doi: 10.2307/5257
Shorrocks, B., Rosewell, J., and Edwards, K. (1990). Competition on a divided and ephemeral resource: testing the assumptions. II. association. J. Animal Ecol. 59:1003. doi: 10.2307/5027
Stefanini, I. (2018). Yeast-insect associations: it takes guts. Yeast 35, 315–330. doi: 10.1002/yea.3309
Stökl, J., Strutz, A., Dafni, A., Svatos, A., Doubsky, J., Knaden, M., et al. (2010). A deceptive pollination system targeting drosophilids through olfactory mimicry of yeast. Curr. Biol. 20, 1846–1852. doi: 10.1016/j.cub.2010.09.033
Tomambo, R. D. (2011). Laboratory and Field Evaluation of Yeast-based Baits for Fruit Flies, Bactrocera occipitalis (Bezzi) and B. philippinensis Grew and Hancock (Diptera:Tephritidae). Rome: AGRIS, International Information System for the Agricultural Science and Technology.
Vargas, R. I., and Prokopy, R. (2006). Attraction and feeding responses of melon flies and oriental fruit flies (Diptera: Tephritidae) to various protein baits with and without toxicants. Proc. Hawaiian. Entomol. Soc. 38, 49–60.
Vijaysegaran, S., Walter, G. H., and Drew, R. A. I. (1997). Mouthpart structure, feeding mechanisms, and natural food sources of adult bactrocera (Diptera: Tephritidae). Ann. Entomol. Soc. Am. 90, 184–201. doi: 10.1093/aesa/90.2.184
Keywords: attraction, Drosophilidae, fermentation, Tephritidae, yeast
Citation: Biasazin TD, Herrera SL, Kimbokota F and Dekker T (2022) Diverging olfactory sensitivities to yeast volatiles reflect resource partitioning of tephritids and drosophilids. Front. Ecol. Evol. 10:999762. doi: 10.3389/fevo.2022.999762
Received: 21 July 2022; Accepted: 31 August 2022;
Published: 15 September 2022.
Edited by:
Ana Depetris Chauvin, Max Planck Institute for Chemical Ecology, GermanyReviewed by:
Ian W. Keesey, University of Nebraska-Lincoln, United StatesMaría Teresa Vera, FAZ-UNT / CONICET, Argentina
Copyright © 2022 Biasazin, Herrera, Kimbokota and Dekker. This is an open-access article distributed under the terms of the Creative Commons Attribution License (CC BY). The use, distribution or reproduction in other forums is permitted, provided the original author(s) and the copyright owner(s) are credited and that the original publication in this journal is cited, in accordance with accepted academic practice. No use, distribution or reproduction is permitted which does not comply with these terms.
*Correspondence: Tibebe Dejene Biasazin, dGliZWJlLmRlamVuZUBzbHUuc2U=