- 1Sanya Nanfan Research Institute of Hainan University, Sanya, China
- 2Key Laboratory of Germplasm Resources Biology of Tropical Special Ornamental Plants of Hainan Province, College of Forestry, Hainan University, Haikou, China
- 3Institute of Plant Protection, Hainan Academy of Agricultural Sciences (Research Center of Quality Safety and Standards for Agricultural Products of Hainan Academy of Agricultural Sciences), Haikou, China
Climate is a key factor affecting the potential distribution of insects, and the host is another important constraint for the distribution of pests. To elucidate changes in the potential distribution of Beauveria bassiana under climate change scenarios, this paper used the data of two different greenhouse gas (GHG) emission scenarios (RCP2.6, RCP8.5) to predict the potential distribution of B. bassiana and its typical host, Bactrocera dorsalis (Hendel), based on the MaxEnt model. Then, the potential distribution of B. bassiana and B. dorsalis (Hendel) was compared, and their suitable growth area’s change and expansion trend under two different GHG emission scenarios were mastered. The results of this study show that the potential distribution area of B. bassiana will increase by 2,050 under the RCP8.5 climate scenario, mainly in central Europe and southwestern Asia, with an increased area of 3.28 × 105 km2. However, under the climate scenario of RCP2.6, the potential distribution area for B. bassiana decreased by 2.0 × 105 km2, mainly in North America. This study will provide a theoretical basis for the control of B. dorsalis (Hendel) with B. bassiana.
Introduction
Due to international trade and travel, the invasion of agricultural pests is frequent and serious in many countries (McCullough et al., 2006; Douma et al., 2016; Lee et al., 2016). Ecological risk assessment of invasive species includes using the historical distribution and infection information of species in the local area to determine risk and making preventive measures before the invasion and outbreak (Lovett et al., 2016; Martínez-Abraín and Jiménez, 2019). Species distribution models (SDMs), based on data of pest occurrence places and related bioclimatic variables, are a common method used to describe or predict potential distribution areas for risk analysis (Lantschner et al., 2019; Méndez-Vázquez et al., 2019; Early et al., 2022). Among these, the maximum entropy (MaxEnt) model is a widely used and easily available method for predicting species distribution with higher accuracy than other models. When using MaxEnt to predict the potential distribution of species, environmental factors such as temperature and rainfall are considered to be the most important factors (Elith et al., 2011; Silva et al., 2014; Zhang et al., 2016).
Beauveria bassiana is one of the most widely studied and applied entomopathogenic fungi in the field of biological control of pests, with more than 360 species. It has the advantages of high toxicity, wide application, and no environmental pollution (Mascarin and Jaronski, 2016; Liu et al., 2021; Tomson et al., 2021). It has been widely used to control agricultural pests such as Bactrocera dorsalis (Hendel), Tetranychus urticae (Koch), and Bemisia tabaci (Gennadius) (Zibaee et al., 2013; Liu et al., 2017; Guo et al., 2021). In a study on the biological control of the B. dorsalis (Hendel), it was found that the death rate of B. dorsalis (Hendel) in direct contact with the conidia suspension of B. bassiana was between 64 and 93%, which confirmed that B. bassiana can be transmitted among individual flies (Tora and Azerefegn, 2021). B. bassiana B6 spore suspension has a weak lethal effect on nymphs of B. dorsalis (Hendel) in soil, and the mortality rate is below 10%. However, when a certain concentration of B. bassiana spore suspension (1 × 108 spores/mL) was sprayed into the cages, 100% of the adults are killed, which indicates that B. bassiana spores are effective against adults (Zhang et al., 2010; Pan et al., 2014). Similarly, it is reported that in the soil treated with B. bassiana, 83% of the larvae of the B. dorsalis (Hendel) died in the process of soil culture (Ugwu and Nwaokolo, 2020). These studies have shown that B. bassiana has a good control effect on B. dorsalis (Hendel).
The ability of the fungus to parasitize is affected by several factors, of which temperature and humidity are especially important for the germination of B. bassiana spores (Zimmermann, 2007; Labbé et al., 2009; Jackson et al., 2010; Li et al., 2011; Gonzalez et al., 2016). B. bassiana spores can’t survive above 50°C, but completely inactivate after several months at 21°C, and they can be stored at 8°C for at least 1 year (Meyling and Eilenberg, 2007). In addition, temperature and humidity are not the only key factors of B. bassiana spore germination, but also play a key role in the growth and development of this pest. Some scholars have found that at a certain relative humidity (from 25 to 70%), the emergence success rate of egg and adult black soldier flies, Hermetia illucens (L.) increases with the increase of relative humidity, while the development time shortens with the increase of relative humidity (Holmes et al., 2012). In a study on the influence of temperature on the development of B. dorsalis (Hendel), it was found that B. dorsalis (Hendel) successfully developed from the egg stage to the adult stage at all tested temperatures except the lowest (13.0 and 14.4°C) (Samayoa et al., 2018). The optimum temperatures for the egg, larval, pupal, and egg-to-pupal stages were 20.7, 21.8, 21.1, and 22.4°C, respectively (Motswagole et al., 2019). In an external environment above 47 or below 7°C, adults and larvae of B. dorsalis (Hendel) can’t survive, and the survival rate of B. dorsalis (Hendel) at all developmental stages decreased with the increase of these two extremes of temperature and the prolongation of their duration (Wei et al., 2015). After a short period of high temperature (45°C) treatment, Zeugodacus cucurbitae (Coquillett) can adapt to extreme conditions by changing their protein expression, as evidenced by an acceleration of ovarian development, leading to earlier egg-laying. But when the number of high-temperature treatments increases, egg-laying behavior is inhibited (Walstad et al., 1970; Pham et al., 2010; Dara et al., 2015; Zhou, 2016; Lee et al., 2017; Zeng et al., 2018).
In this study, we used environmental data from two different GHG emission scenarios (RCP2.6 and RCP8.5) based on the MaxEnt ecological model and selected B. dorsalis (Hendel) as the host of B. bassiana to investigate the distribution and trends in the suitable areas of B. bassiana and B. dorsalis (Hendel). This study provides a theoretical basis for pest control by pathogenic fungi.
Materials and methods
Sources of geographic distribution data
By collecting and reading a large number of published academic papers and visiting GBIF1 to obtain the geographic coordinate data of B. bassiana and B. dorsalis (Hendel). To avoid overfitting, we first delete the coordinate data falling on the sea surface, and then we create a 2 km × 2 km grid, and the data in the same grid only retains one. Finally, we screened out the geographic distribution data of 310 B. dorsalis (Hendel) and 525 B. bassiana. The geographic coordinate data of historical places are saved in “.CSV” format.
Climate data acquisition and screening
The current 19 bioclimatic variables data used in this study were obtained from WorldClim2 (Table 1), which was released in January 2020, spanning the period from 1,970 to 2,000, and its accuracy was 2.5 arc-minutes. MaxEnt 3.4.4 was used to analyze the correlation between environmental factors, and the contribution of environmental factors was ranked, and then SPSS 26.0 was used to analyze the correlation between environmental factors. When the absolute value of the correlation between two ecological factors was greater than or equal to 0.8, only one representative environmental factor will be kept (Lobo, 2016). At last, eight environmental factors (Bio2 Bio10, Bio11, Bio13, Bio14, Bio15, Bio18, Bio19) and nine environmental factors (Bio2, Bio4, Bio5, Bio6, Bio12, Bio14, Bio15, Bio18, Bio19) of B. dorsalis (Hendel) and B. bassiana bioclimatic variables were selected (Li et al., 2022; Table 1). In this study, environmental data from two GHG emission scenarios, RCP2.6, and RCP8.5, were selected to project the future climate suitability areas for the B. bassiana and B. dorsalis (Hendel) under future climate conditions. These bioclimatic variables ran in the model were selected from Coupled Model Intercomparison Project Phase 6 (CMIP6), and their accuracy was 2.5 arc-minutes.
Model construction and evaluation
Construction of the model
At first, we imputed the filtered current environmental data (1,970–2,000) into MaxEnt. We then input the geographical distribution data of the B. bassiana and B. dorsalis (Hendel) into the model separately, and randomly selected 75% of the data as the training set for the experiments. The remaining 25% coordinate data were used as the test set, which is repeated 10 times, and the remaining parameters are used as the default values of the software. The simulation data obtained above were input into ArcMap 10.6 to visualize the simulation results. By using the Spatial Analyst option of the Arc toolbox in the software, the raster files were reclassified using the reclassification tool, and the distribution area was set to four gradients (Welch and Harwood, 2014): unsuitable areas (0–0.2), low suitable areas (0.2– 0.4), moderately suitable areas (0.4–0.6), and high suitable areas (0.6–0.1).
Evaluation of the model
In this study, the area under the receiver operating characteristic curve (AUC) was used as a measure of the accuracy of the model prediction. The interval range is 0.5–0.1, 0.5 corresponds to a completely random prediction, 0.5–0.7 indicates that the accuracy of the prediction result is poor, and 0.7–0.9 indicates that the accuracy of the prediction result is moderate. When the prediction result is > 0.9, it shows that the prediction result has higher accuracy (Barry and Elith, 2006).
Result and analysis
The precision of the model prediction results was assessed
The AUC values of each prediction result were above 0.90 at the end of the model operation (Table 2), indicating a high degree of accuracy of the prediction result.
Distribution of suitable areas of Beauveria bassiana and Bactrocera dorsalis (Hendel) under different climatic conditions
The distribution of the two habitats under current climatic conditions
Under current climatic conditions (1,970–2,000), the highly suitable areas of B. dorsalis (Hendel) are mainly distributed in Asian regions, such as Africa and southern coastal areas of China, and sporadically in African regions, with a total area of 1.30 × 106 km2 (Table 3), accounting for 0.89% of the global land area (Figure 1). The moderately and low suitable areas are mainly distributed in central Africa and South America, with a total area of 1.65 × 107 km2. The potential global distribution of B. bassiana is concentrated between 30°N–60°N and 0°–60°S, with a total area of 2.0 × 107 km2. The highly suitable areas of B. bassiana are mainly distributed in the European region and sporadically in the American region, with a total area of 2.38 × 106 km2 (Table 4). The moderately suitable areas include the southeastern part of South America and North America, the central to the eastern part of Europe, and the coastal areas of Asia, with a total area of 6.0 × 106 km2. The low suitability areas of B. bassiana are mainly distributed in three regions, Africa, Oceania, and Asia. The prediction results showed that the contribution rate of Bio13 (Precipitation in the wettest month) to the model prediction of B. dorsalis (Hendel) accounted for 66.6%, indicating that Bio13 played a key role in the distribution of B. dorsalis (Hendel).
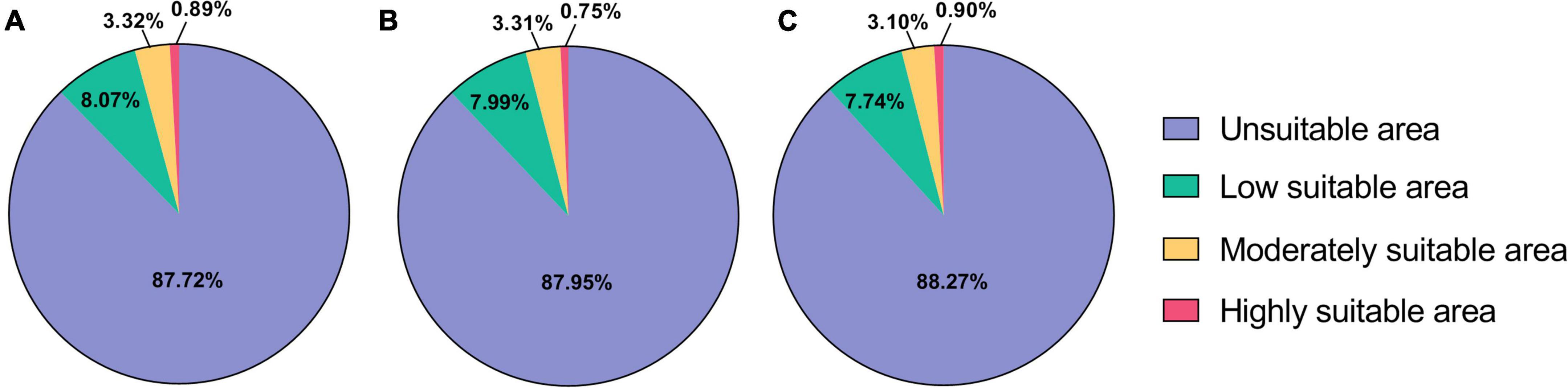
Figure 1. Distribution of predicted suitable areas of Bactrocera dorsalis (Hendel). (A) Suitable areas of Bactrocera dorsalis (Hendel) under current climatic conditions. (B) Suitable areas of Bactrocera dorsalis (Hendel) under RCP2.6 climatic conditions. (C) Suitable areas of Bactrocera dorsalis (Hendel) under RCP8.5 climatic conditions.
A comparison of the distribution of the suitable areas under the same climatic reveals some differences between the two species in distribution, most notably in Europe, where large areas are suitable for B. bassiana, but not for B. dorsalis (Hendel). However, in other areas, there was a large area overlap between the two suitable areas, and the total area of B. bassiana suitable areas is much larger than that of B. dorsalis (Hendel), which indicates that B. bassiana is more adaptable to the environment than B. dorsalis (Hendel), which provides the conditions for the use of B. bassiana to control of B. dorsalis (Hendel) in all areas. The prediction results showed that Bio6 (Lowest temperature in the coldest month) and Bio12 (Average annual precipitation) have a total contribution rate of 65.3%, which indicates that low temperature and annual precipitation have decisive effects on the survival of B. bassiana (Figure 2).
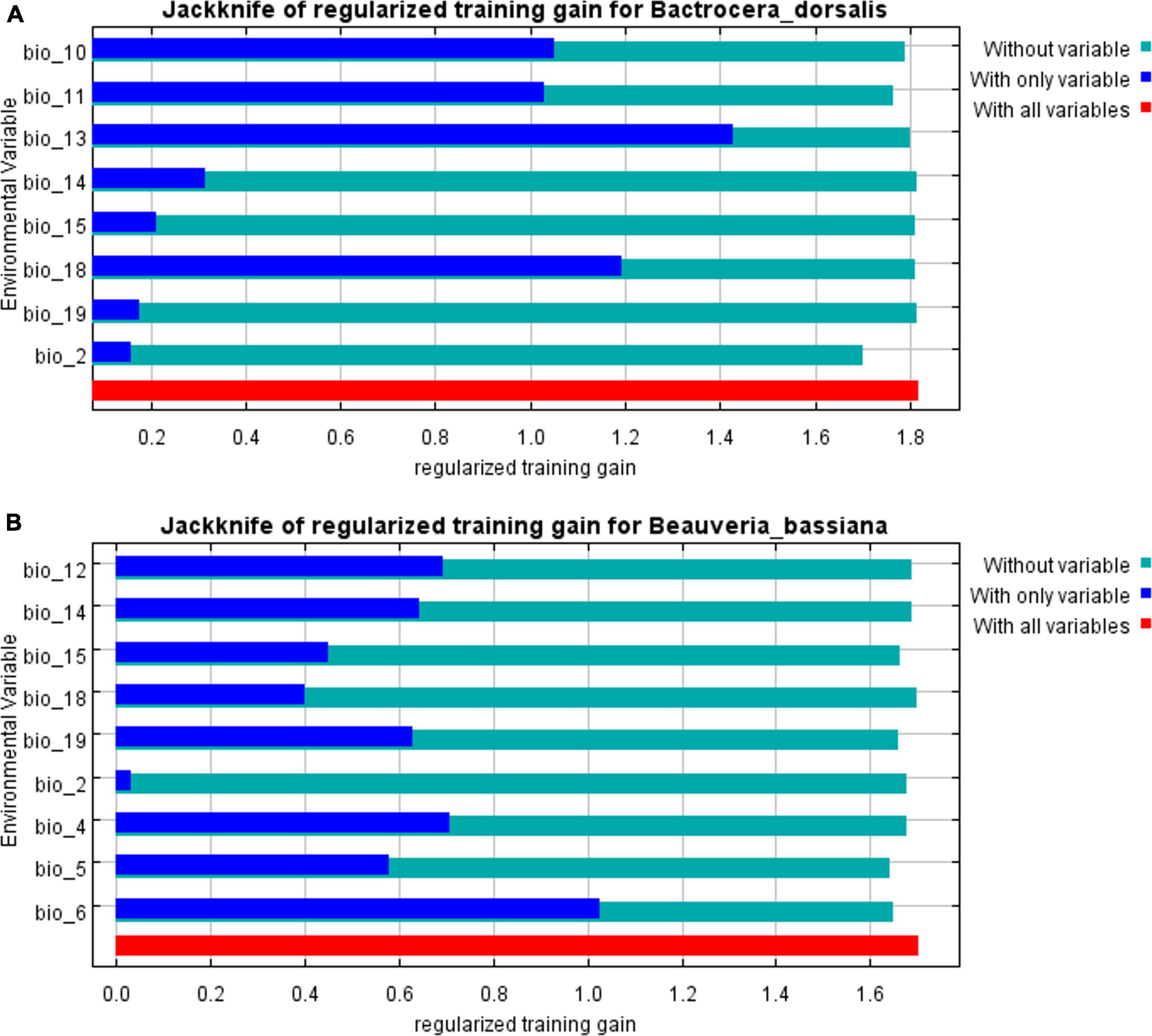
Figure 2. The results of the Jackknife test of Beauveria bassiana and its host. (A) Jackknife test of Bactrocera dorsalis (Hendel). (B) Jackknife test of Beauveria bassiana.
Changes in the suitable areas of both under future climate conditions
Based on environmental data from two future GHG emission scenarios, the results of this study predicted the suitable areas of B. bassiana, and the results are shown in the following figure (Figure 3). The results show that the total area of the habitat of B. bassiana increases under the two scenarios, RCP2.6 and RCP8.5. However, under RCP2.6, the moderate and high suitable areas of B. bassiana decreased, and the decrease of suitable areas of B. bassiana was less than the change of suitable areas of B. dorsalis (Hendel), indicating that B. bassiana is more adaptable to its environment than B. dorsalis (Hendel). In the RCP8.5 scenario, there is a more significant increase in the size of the B. bassiana suitable areas, with an additional area of 1.33 × 106 km2, when compared with the predicted B. bassiana suitable areas map for the current climate scenario, shows an expansion of the B. bassiana suitable areas from near the equator to higher latitudes, suggesting that climate change is causing the higher latitude environment to reach a level of suitable for the fungus. This indicates that climate change is causing the environment at higher latitudes to meet the conditions suitable for the fungus to live, and B. bassiana spores can germinate and grow in these areas. Under the RCP8.5 climate scenario, there is a trend toward a reduction in the size of the habitat for the B. dorsalis (Hendel), which will help to control the damage and spread of the B. dorsalis (Hendel).
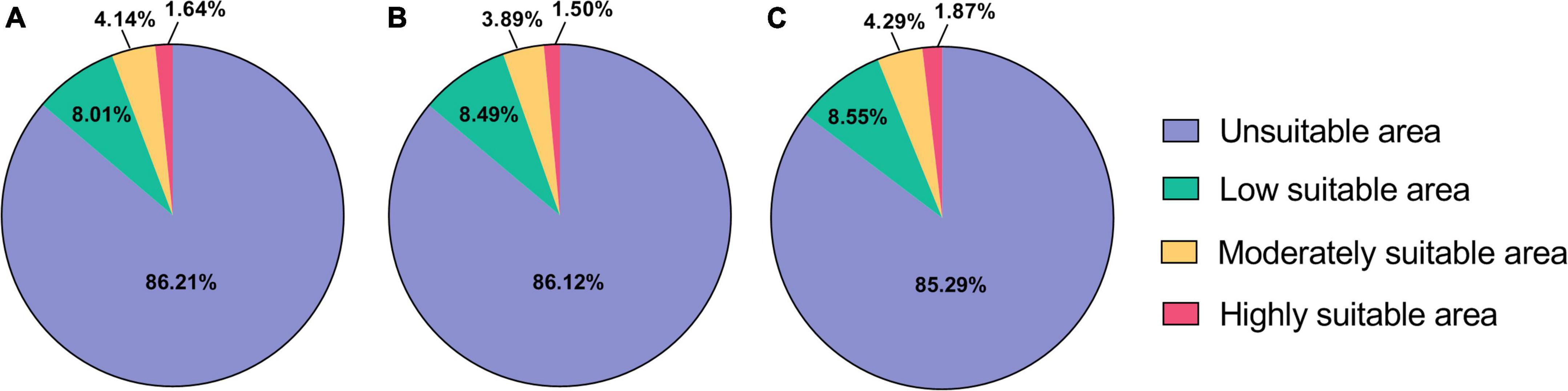
Figure 3. Distribution of predicted suitable areas of Beauveria bassiana. (A) Suitable areas of Beauveria bassiana under current climatic conditions. (B) Suitable areas of Beauveria bassiana under RCP2.6 climatic conditions. (C) Suitable areas of Beauveria bassiana under RCP8.5 climatic conditions.
Discussion
In recent years, a large amount of GHG emissions into the atmosphere have led to the rise of the global average temperatures, which creates conditions for the invasion and expansion of pests (Boggs, 2016; Marshall et al., 2020; Colares et al., 2021). Due to global climate change, these threats may increase in many countries located in tropical areas (Ou et al., 2021). This finding is consistent with our prediction of the distribution of the suitable areas of the B. dorsalis (Hendel) under the current climate conditions. MaxEnt is a correlation model, which generates predictions according to the statistical relationships between occurrence patterns and environmental data (Soares et al., 2021).
It has been found that these two models may produce different results due to different spatial resolutions and bioclimatic variables (Stephens et al., 2007; De Villiers et al., 2015). SDMs can predict the changes in the potential habitat of a known geographical distribution species over time through climate changes, thus guiding pest control. The three most commonly used SDMs are CLIMEX, DIVA-GIS, and MaxEnt. They operate according to different principles and require different data (Gonzalez et al., 2016; Rana et al., 2017; Wang et al., 2019). In this study, we tried to use only a small part of geographical data about the historical position of the B. dorsalis (Hendel) to predict the potential range of B. dorsalis (Hendel), and obtained results highly similar to the original result, indicating a situation with only a small amount of data, the MaxEnt model can also accurately predict insect range (Sultana et al., 2017). The predicted results were compared with the suitable areas for B. dorsalis (Hendel) under the same climate scenario, and it was found that the suitable areas for B. bassiana and B. dorsalis (Hendel) overlap greatly, and the suitable areas for B. dorsalis (Hendel) B. bassiana is much larger than B. dorsalis (Hendel), suggesting that B. bassiana can adapt to more environmental types than B. dorsalis (Hendel). In this study, it was found that in Asia, where B. dorsalis (Hendel) is highly suitable for living, is not the high suitable area for B. bassiana. It may be because the high temperatures in these areas hinder the normal germination of B. bassiana spores, and thus preventing them from surviving in this area. There is a large area of high suitable areas for B. bassiana with high adaptability in high latitudes of in Europe, which indicates that the temperatures in these areas are more suitable for growth and spore germination of B. bassiana. In conclusion, the current and future climate conditions are suitable for the normal survival of B. dorsalis (Hendel), and also for the survival of B. bassiana, which indicates that B. bassiana can adapt to the environment better than B. dorsalis (Hendel).
In the ecosystems, the degree to which pest populations is controlled or regulated by natural enemies depends on their ability to adapt to the local environment. An important step to successfully introduce natural enemy is to evaluate their suitable areas (Fargues et al., 1997; Uma Devi et al., 2008; Amobonye et al., 2020). In addition to climatic variables, factors that limit the potential geographical distribution of pathogenic fungi include host, species competition, natural enemies, soil type, geographical features, natural, and geographical obstacles and human activities (Kannan and Rao, 2006; Cheng et al., 2017; Zhang et al., 2018; Clarke et al., 2019). Relevant research shows that B. bassiana is the most widespread pathogenic fungus in agricultural land, and it can easily colonize in different plant hosts, such as corn, wheat, tobacco, and melon (Greenfield et al., 2016; Mckinnon et al., 2018; Mahmood et al., 2019). The above studies show that B. bassiana can colonize on the leaves of many plants. Combined with the purpose of this research, it is possible to control B. dorsalis (Hendel) by inoculating B. bassiana on plant leaves.
In this paper, B. dorsalis (Hendel) was used as the host of B. bassiana to study the suitable areas of B. bassiana. The results show that the area of the habitat of B. dorsalis (Hendel) increases by 3.31 × 105 km2 by 2,050 under the RCP2.6 scenario, and increases by 7.92 × 105 km2 under the RCP8.5 scenario. When studying the impact of climate change on the potential global geographical distribution of the B. dorsalis (Hendel), it was found that under future climate conditions, the suitable area of B. dorsalis (Hendel) in the northern hemisphere is expected to expand northward, and the suitable area in the southern hemisphere is expected to expand southward (Qin et al., 2019). Under the RCP8.5 scenario, both the suitable area and habitat suitability of B. dorsalis (Hendel) are projected to increase, with the climatically suitable area in North America projected to increase by 1.52 × 105 km2 in 2,050. The above conclusions are consistent with the findings of this paper, and the differences of suitable regional changes may be caused by the selection of bioclimatic variables and data processing methods. We also found that in the prediction results, the distribution probability of B. dorsalis (Hendel) reached a peak when Bio13 (Precipitation in the wettest month) was around 1,200 mm, and that of B. bassiana reached a peak when Bio12 (Average annual precipitation) was at 7,000–8,000 mm. This shows that B. bassiana has a great demand for precipitation, which will increase the difficulty of using B. bassiana to control B. dorsalis (Hendel) in arid areas.
In this study, the potential distribution areas of B. bassiana and B. dorsalis (Hendel) were predicted by using the MaxEnt model, and the expected results were achieved. However, the model can’t combine the data of the two species’ bioclimatic variable for statistical analysis, and the final result is slightly different from the actual result, which is also the place where the model needs to be optimized.
Data availability statement
The original contributions presented in this study are included in the article/supplementary material, further inquiries can be directed to the corresponding author.
Author contributions
AW, SP, YL, XY, and SZ participated in the study design and analysis of the manuscript. JJ, JL, SY, and RZ participated in the study design and helped to draft the manuscript. Supervision and financial support by SZ, revised and processed. All authors have read and approved the final manuscript.
Funding
This study was supported by Hainan Major Science and Technology Project (ZDKJ201901). Hainan Province Science and Technology Special Fund (ZDYF2022XDNY163).
Conflict of interest
The authors declare that the research was conducted in the absence of any commercial or financial relationships that could be construed as a potential conflict of interest.
Publisher’s note
All claims expressed in this article are solely those of the authors and do not necessarily represent those of their affiliated organizations, or those of the publisher, the editors and the reviewers. Any product that may be evaluated in this article, or claim that may be made by its manufacturer, is not guaranteed or endorsed by the publisher.
Footnotes
References
Amobonye, A., Bhagwat, P. K., Pandey, A., Singh, S., and Santhosh, P. (2020). Biotechnological potential of Beauveria bassiana. as a source of novel biocatalysts and metabolite. Crit. Rev. Biotechnol. 40, 1019–1034. doi: 10.1080/07388551.2020.1805403
Barry, S., and Elith, J. (2006). Error and uncertainty in habitat models. J. Appl. Ecol. 43, 413–423. doi: 10.1111/j.1365-2664.2006.01136.x
Boggs, C. L. (2016). The fingerprints of global climate change on insect populations. Curr. Opin. Insect. Sci. 17, 69–73. doi: 10.1016/j.cois.2016.07.004
Cheng, D., Guo, Z. J., Riegler, M., Xi, Z. Y., Liang, G. W., and Xu, Y. J. (2017). Gut symbiont enhances insecticide resistance in a significant pest, the oriental fruit fly Bactrocera dorsalis (Hendel). Microbiome 5, 1–12. doi: 10.1186/s40168-017-0236-z
Clarke, A. R., Li, Z. H., Qin, Y. J., Zhao, Z. H., and Schutze, M. K. (2019). Bactrocera dorsalis (Hendel) (Diptera: Tephritidae) is not invasive through Asia: it’s been there all along. J. Appl. Entomol. 143, 797–801. doi: 10.1111/jen.12649
Colares, C., Roza, A. S., Mermudes, J. R. M., Silveira, L. F. L., and Macedo, M. V. D. (2021). Elevational specialization and the monitoring of the effects of climate change in insects: beetles in a Brazilian rainforest mountain. Ecol. Indic. 120:106888. doi: 10.1016/j.ecolind.2020.106888
Dara, S. K., Dara, S. R., and Dara, S. S. (2015). Endophytic colonization and pest management potential of Beauveria bassiana in strawberries. J. Berry Res. 3, 203–211. doi: 10.3233/JBR-130058
De Villiers, M., Hattingh, V., Kriticos, D. J., Brunel, S., Vayssières, J. F., Sinzogan, A., et al. (2015). The potential distribution of Bactrocera dorsalis: considering phenology and irrigation patterns. Bull 1, 1–15. doi: 10.1017/S0007485315000693
Douma, J. C., Pautasso, M., Venette, R. C., Robinet, C., Hemerik, L., Mourits, M. C. M., et al. (2016). Pathway models for analysing and managing the introduction of alien pests an overview and categorization. Ecol. Eng. 339, 58–67. doi: 10.1016/j.ecolmodel.2016.08.009
Early, R., Rwomushana, I., Chipabika, G., and Day, R. (2022). Comparing, evaluating and combining statistical species distribution models and CLIMEX to forecast the distributions of emerging crop pests. Pest Manag. 78, 671–683. doi: 10.1002/ps.6677
Elith, J., Phillips, S. J., Hastie, T., Dudík, M., Chee, Y. E., and Yates, C. J. (2011). A statistical explanation of MaxEnt for ecologists. Divers. Distrib. 17, 43–57. doi: 10.1111/j.1472-4642.2010.00725.x
Fargues, J., Goettel, M. S., Smits, N., Ouedraogo, A., and Rougier, M. (1997). Effect of temperature on vegetative growth of Beauveria bassiana isolates from different origins. Mycologia 89, 383–392. doi: 10.2307/3761032
Gonzalez, F., Tkaczuk, C., Dinu, M. M., Fiedler, V. S., Zchori, F. E., and Messelink, G. J. (2016). New opportunities for the integration of microorganisms into biological pest control systems in greenhouse crops. J. Pest Sci. 89, 295–311. doi: 10.1007/s10340-016-0751-x
Greenfield, M., Gómez-Jiménez, M. I., Ortiz, V., Vega, F. E., Kramer, M., and Parsa, S. (2016). Beauveria bassiana and Metarhizium anisopliae endophytically colonize cassava roots following soil drench inoculation. Biol. Control 95, 40–48. doi: 10.1016/j.biocontrol.2016.01.002
Guo, F., Zhao, R. N., Li, T. M., Wu, X. S., Tian, Y., and Chen, W. L. (2021). Preliminary study on contact toxicity and oviposition deterrent effect of six plant ethanol extracts against Bactrocera dorsalis Hendel adults. Plant Quarantine 35, 39–43.
Holmes, L. A., Vanlaerhoven, S. L., and Tomberlin, J. K. (2012). Relative humidity effects on the life history of Hermetia illucens (Diptera: Stratiomyidae). Environment 41, 971–978. doi: 10.1603/EN12054
Jackson, M. A., Dunlap, C. A., and Jaronski, S. T. (2010). Ecological considerations in producing and formulating fungal entomopathogens for use in insect biocontrol. Biocontrol. 55, 129–145. doi: 10.1007/s10526-009-9240-y
Kannan, M., and Rao, N. V. (2006). Ecological studies on mango fruit fly. Bactrocera dorsalis (Hendel). Plant Prot. Sci. 14, 340–342.
Labbé, R. M., Gillespie, D. R., Cloutier, C., and Brodeur, J. (2009). Compatibility of an entomopathogenic fungus with a predator and a parasitoid in the biological control of greenhouse whitefly. Biocontrol. Sci. Techn. 19, 429–446. doi: 10.1080/09583150902803229
Lantschner, M. V., de la Vega, G., and Corley, J. (2019). Modelling the establishment, spread and distribution shifts of pests. Int. J. Pest Manage 65, 187–189. doi: 10.1080/09670874.2019.1575490
Lee, S. J., Kim, S., Kim, J. C., Lee, M. R., Hossain, M. S., Shin, T. S., et al. (2017). Entomopathogenic Beauveria bassiana granules to control soil-dwelling stage of western flower thrips, Frankliniella occidentalis (Thysanoptera: Thripidae). Biocontrol 62, 639–648. doi: 10.1007/s10526-017-9818-8
Lee, W., Lee, Y., Kim, S., Lee, J. H., Lee, H., Lee, S., et al. (2016). Current status of exotic insect pests in Korea: comparing border interception and incursion during 1996-2014. J. Asia Pac. Entomol. 19, 1095–1101. doi: 10.1016/j.aspen.2016.09.003
Li, H., Huang, D., and Wang, Z. (2011). Potential of Beauveria bassiana for biological control of Apriona germari. Front. Agric. China 5:666–670. doi: 10.1007/s11703-011-1130-2
Li, Z. J., Liu, Y. M., and Zeng, H. (2022). Application of the MaxEnt model in improving the accuracy of ecological red line identification: a case study of Zhanjiang, China. Ecol. Indic. 137:108767. doi: 10.1016/j.ecolind.2022.108767
Liu, J., Ling, Z. Q., Wang, J. J., Xiang, T. T., Xu, L., Gu, C. X., et al. (2021). In vitro transcriptomes analysis identifies some special genes involved in pathogenicity difference of the Beauveria bassiana against different insect hosts. Microb. Pathog. 154:104824. doi: 10.1016/j.micpath.2021.104824
Liu, X. X., Wang, X. R., Wang, Y. Y., Huang, J. B., Chen, J. Y., and Zeng, L. (2017). Biological characteristics of highly virulent strains of Beauveria bassiana for Dendrolimus punctatus. J. Southern Agriculture 48, 1019–1023.
Lobo, J. M. (2016). The use of occurrence data to predict the effects of climate change on insects. Curr. Opin. Insect. Sci. 17, 62–68. doi: 10.1016/j.cois.2016.07.003
Lovett, G. M., Weiss, M., Liebhold, A. M., Holmes, T. P., Leung, B., Lambert, K. F., et al. (2016). Nonnative forest insects and pathogens in the United States: impacts and policy options. Ecol. Appl. 26, 1437–1455. doi: 10.1890/15-1176
Mahmood, Z., Steenberg, T., Mahmood, K., Labouriau, R., and Kristensen, M. (2019). Endophytic Beauveria bassiana in maize affects survival and fecundity of the aphid Sitobion avenae. Biol. Control 137:104017. doi: 10.1016/j.biocontrol.2019.104017
Marshall, K. E., Gotthard, K., and Williams, C. M. (2020). Evolutionary impacts of winter climate change on insects. Curr. Opin. Insect Sci. 41, 54–62. doi: 10.1016/j.cois.2020.06.003
Martínez-Abraín, A., and Jiménez, J. (2019). Dealing with growing forest insect pests: the role of top-down regulation. J. Appl. Ecol. 56, 2574–2576. doi: 10.1111/1365-2664.13418
Mascarin, G. M., and Jaronski, S. T. (2016). The production and uses of Beauveria bassiana as a microbial insecticide. World J. Microbiol. 32, 1–26. doi: 10.1007/s11274-016-2131-3
McCullough, D. G., Work, T. T., Cavey, J. F., Liebhold, A. M., and Marshall, D. (2006). Interceptions of nonindigenous plant pests at US ports of entry and border crossings over a 17-year period. Biol. Invasions 8, 611–630. doi: 10.1007/s10530-005-1798-4
Mckinnon, A. C., Glare, T. R., Ridgway, H. J., Mendoza-Mendoza, A., Holyoake, A., Godsoe, W. K., et al. (2018). Detection of the entomopathogenic fungus Beauveria bassiana in the rhizosphere of Wound-Stressed Zea mays plants. Front. Microbiol. 9:1161. doi: 10.3389/fmicb.2018.01161
Méndez-Vázquez, L. J., Lira-Noriega, A., Lasa-Covarrubias, R., and Cerdeira-Estradad, S. (2019). Delineation of site-specific management zones for pest control purposes: exploring precision agriculture and species distribution modeling approaches. Comput. Electron Agric. 167:105101. doi: 10.1016/j.compag.2019.105101
Meyling, N. V., and Eilenberg, J. (2007). Ecology of the entomopathogenic fungi Beauveria bassiana and Metarhizium anisopliae in temperate agroecosystems: potential for conservation biological control. Biol. Control 43, 145–155. doi: 10.1016/j.biocontrol.2007.07.007
Motswagole, R., Gotcha, N., and Nyamukondiwa, C. (2019). Thermal biology and seasonal population abundance of Bactrocera dorsalis Hendel (Diptera: Tephritidae): implications on pest management. Int. J. Insect Sci. 11, 1–9. doi: 10.1177/1179543319863417
Ou, Y. S., Qin, S. S., Chen, W. F., Yan, Y. Q., and Chen, Y. H. (2021). Prediction of potential distribution of Buddleja based on the Maxent and ARCGIS. Ecol. Sci. 40, 165–173.
Pan, Z. P., Li, D. S., Huang, S. H., and Zeng, L. (2014). Effect of two application methods of Beauveria bassiana on its controlling effect on the fruit fly, Bactrocera dorsalis. J. Econ. Entomol. 36, 102–108.
Pham, T. A., Kim, J. J., and Kim, K. (2010). Optimization of solid-state fermentation for improved conidia production of Beauveria bassiana as a Mycoinsecticide. Mycobiology 38, 137–143. doi: 10.4489/MYCO.2010.38.2.137
Qin, Y. J., Wang, C., Zhao, Z. H., Pan, X. B., and Li, Z. H. (2019). Climate change impacts on the global potential geographical distribution of the agricultural invasive pest, Bactrocera dorsalis (Hendel) (Diptera: Tephritidae). Clim. Change 155, 145–156. doi: 10.1007/s10584-019-02460-3
Rana, S. K., Rana, H. K., Ghimire, S. K., Shrestha, K. K., and Ranjitkar, S. (2017). Predicting the impact of climate change on the distribution of two threatened Himalayan medicinal plants of Liliaceae in Nepal. J. Mt. Sci. 14, 558–570. doi: 10.1007/s11629-015-3822-1
Samayoa, A. C., Choi, K. S., Wang, Y. S., Hwang, S. Y., Huang, Y. B., and Ahn, J. J. (2018). Thermal effects on the development of Bactrocera dorsalis (Hendel) (Diptera: Tephritidae) and model validation in Taiwan. Phytoparasitica 46, 365–376. doi: 10.1007/s12600-018-0674-6
Silva, D. P., Gonzalez, V. H., Melo, G. A. R., Lucia, M., Alvarez, L. J., and Marco, P. D. (2014). Seeking the flowers for the bees: integrating biotic interactions into niche models to assess the distribution of the exotic bee species Lithurgus huberi in South America. Ecol. Modell. 273, 200–209. doi: 10.1016/j.ecolmodel.2013.11.016
Soares, J. R. S., Da Silva, S. R. S., Ramos, R. S., and Picanço, M. C. (2021). Distribution and invasion risk assessments of Chrysodeixis includens (Walker, [1858]) (Lepidoptera: Noctuidae) using CLIMEX. Int. J. Biometeorol. 65, 1137–1149. doi: 10.1007/s00484-021-02094-0
Stephens, A. E. A., Kriticos, D. J., and Leriche, A. (2007). The current and future potential geographical distribution of the oriental fruit fly, Bactrocera dorsalis (Diptera: Tephritidae). Bull. Entomol. Res. 97, 369–378. doi: 10.1017/S0007485307005044
Sultana, S., Baumgartner, J. B., Dominiak, B. C., Royer, J. E., and Beaumont, L. J. (2017). Potential impacts of climate change on habitat suitability for the Queensland fruit fly. Sci. Rep. 7:13025. doi: 10.1038/s41598-017-13307-1
Tomson, M., Sahayaraj, K., Sayed, S., Elarnaouty, S. A., and Petchidurai, G. (2021). Entomotoxic proteins of Beauveria bassiana Bals. (Vuil.) and their virulence against two cotton insect pests. J. King Saud. Univ. Sci. 33:101595. doi: 10.1016/j.jksus.2021.101595
Tora, M., and Azerefegn, F. (2021). Virulence of Beauveria bassiana and Metarhizium anisopliae isolates against the oriental fruit fly Bactrocera dorsalis (Diptera: Tephritidae) hendel under laboratory conditions. Ethiopian J. Agricultural Sci. 31, 53–67.
Ugwu, J. A., and Nwaokolo, V. M. (2020). Biocidal activity of selected botanicals and Beauveria bassiana on oriental fruit fly, Bactrocera dorsalis (Diptera; Tephritidae). J. Res. For. Wildl. Environ. 12, 53–61.
Uma Devi, K., Padmavathi, J., Uma Maheswara, Rao, C., Khan, A. A. P., and Mohan, M. C. (2008). A study of host specificity in the entomopathogenic fungus Beauveria bassiana (Hypocreales, Clavicipitaceae). Biocontrol. Sci. Technol. 18, 975–989. doi: 10.1080/09583150802450451
Walstad, J. D., Anderson, R. F., and Stambaugh, W. J. (1970). Effects of environmental conditions on two species of muscardine fungi (Beauveria bassiana and Metarrhizium anisopliae). J. Invertebr. Pathol. 16, 221–226. doi: 10.1016/0022-2011(70)90063-7
Wang, Y., Wang, Z. H., Xing, H. F., Li, J. W., and Sun, S. (2019). Prediction of potential suitable distribution of Davidia involucrata Baill in China based on MaxEnt. Chinese J. Ecol. 38, 1230–1237.
Wei, D., Jia, F. X., Tian, C. B., Tian, Y., Smagghe, G., Dou, W., et al. (2015). Comparative proteomic analysis of Bactrocera dorsalis (Hendel) in response to thermal stress. J. Insect Physiol. 74, 16–24. doi: 10.1016/j.jinsphys.2015.01.012
Welch, K. D., and Harwood, J. D. (2014). Temporal dynamics of natural enemy-pest interactions in a changing environment. Biol. Control. 75, 18–27. biocontrol.2014.01.004 doi: 10.1016/j.biocontrol.2014.01.004
Zeng, B., Zhu, W. J., Fu, Y. G., and Zhou, S. H. (2018). Influence of high-temperature exposure on the mating, oviposition and thermotaxis of Bactrocera cucurbitae (Coquillet) (Diptera: Tephritidae). PLoS One 13:e0204065. journal.pone.0204065 doi: 10.1371/journal.pone.0204065
Zhang, H. L., Guo, Y. L., and Gao, B. (2016). Multi-model suitability assessment of construction land in Tsinling mountains based on the niche theory: a case study of Shangzhou, Shangluo. Geogr. Geo-Inf. Sci. 32, 83–89.
Zhang, Y. B., Gao, C. H., and Qin, H. (2018). Prediction of the suitable distribution and responses to climate change of Elaeagnus mollis in Shanxi Province, China. Chinese J. Appl. Ecol. 29, 1156–1162.
Zhang, Y. P., Huang, S. H., Li, D. S., Zhang, B. X., and Chen, M. Y. (2010). Control effect of Beauveria bassiana B6 strain on Bactrocera dorsalis (Hendel). Chinese J. Biol. Control 26, 14–18.
Zhou, S. H. (2016). Study on the Responses of Bactrocera cucurbitae to High Temperature Stress and its Molecular Basis. China: Hainan University.
Zibaee, I., Bandani, A. R., and Sendi, J. J. (2013). Pathogenicity of Beauveria bassiana to fall webworm (Hyphantria cunea) (Lepidoptera: Arctiidae) on different host plants. Plant Prot. Sci. 49, 169–176. doi: 10.17221/72/2012-PPS
Keywords: Beauveria bassiana, fungus, Bactrocera dorsalis (Hendel), potential distribution areas, MaxEnt model, biological control
Citation: Wang A, Peng S, Lian Y, Yang X, Jia J, Li J, Yang S, Zheng R and Zhou S (2022) Distribution and interaction of the suitable areas of Beauveria bassiana and Bactrocera dorsalis (Hendel). Front. Ecol. Evol. 10:990747. doi: 10.3389/fevo.2022.990747
Received: 10 July 2022; Accepted: 01 August 2022;
Published: 25 August 2022.
Edited by:
Anouschka R. Hof, Wageningen University & Research, NetherlandsReviewed by:
Rihui Yan, Tennessee State University, United StatesBingxin Wang, Wageningen University & Research, Netherlands
Copyright © 2022 Wang, Peng, Lian, Yang, Jia, Li, Yang, Zheng and Zhou. This is an open-access article distributed under the terms of the Creative Commons Attribution License (CC BY). The use, distribution or reproduction in other forums is permitted, provided the original author(s) and the copyright owner(s) are credited and that the original publication in this journal is cited, in accordance with accepted academic practice. No use, distribution or reproduction is permitted which does not comply with these terms.
*Correspondence: Shihao Zhou, zsh88200939@126.com
†These authors have contributed equally to this work