- Applied Ecology and Conservation Lab, Programa de Pós-Graduação em Ecologia e Conservação da Biodiversidade, Universidade Estadual de Santa Cruz, Ilhéus, Brazil
Biodiversity is already experiencing the effects of climate change through range expansion, retraction, or relocation, potentializing negative effects of other threats. Future projections already indicate richness reduction and composition modifications of bird communities due to global warming, which may disrupt the provision of key ecological services to ecosystem maintenance. Here, we systematically review the effect of morphological, ecological, and geographical traits on the amount of future suitable area for birds worldwide. Specifically, we tested whether body mass, diet, habitat type, movement pattern, range size, and biogeographic realm affect birds' suitable area. Our search returned 75 studies that modeled the effects of climate change on 1,991 bird species. Our analyses included 1,661 species belonging to 128 families, representing 83% of the total, for which we were able to acquire all the six traits. The proportion of birds' suitable area was affected by range size, body mass, habitat type, and biogeographic realm, while diet and movement pattern showed lower relative importance and were not included in our final model. Contrary to expectations, the proportion of birds' suitable area was negatively related to range size, which may be explained by higher climatic stability predicted in certain areas that harbor species with restricted distribution. In contrast, we observed that birds presenting higher body mass will show an increase of the proportion of suitable area in the future. This is expected due to the high exposure of smaller birds to environmental changes and their difficulty to keep thermoregulation. Our results also indicated a low proportion of suitable area to forest-dependent birds, which is in accordance with their higher vulnerability due to specific requirements for reproduction and feeding. Finally, the proportion of suitable area was low for birds from Oceania, which is expected since the region encompasses small islands isolated from continents, preventing their species from reaching new suitable areas. Our study highlights that different traits should be considered when assessing extinction risk of species based on future projections, helping to improve bird conservation, especially the most vulnerable to climate change.
Introduction
As a result of alterations in temperature and precipitation, species distributions may shift (i.e., expansion, retraction, or relocation) to seek better climatic conditions (Bellard et al., 2012; Lenoir and Svenning, 2015). Even when a species is not directly affected by climate change, it can suffer indirectly due to its interaction with species that will lose or relocate their habitat (Thomas, 2010). In this context, several studies discuss the potential impacts of climate change on biodiversity (e.g., Pearman et al., 2011; Newbold, 2018; Hidasi-Neto et al., 2019). Several studies have reported that our planet will become warmer and drier in the next decades (e.g., Drobinski et al., 2020; Almazroui et al., 2021), which will cause an increase in the species extinction risk (Bateman et al., 2020). For instance, the number of mammal species are predicted to decrease under future scenarios, including in highly diverse regions (Hidasi-Neto et al., 2019). A similar pattern is observed for anuran and birds, whose species richness are expected to be lower in future climatic scenarios (Menéndez-Guerrero et al., 2020; Prieto-Torres et al., 2020). Additionally, climate change may lead to savannization of tropical forests (Franchito et al., 2012) and consequently the impoverishment of communities due to species replacement (i.e., extinction of specialists and expansion of generalists), as predicted for mammals (Hidasi-Neto et al., 2019; Sales et al., 2020). Because of these changes in species' suitable areas, diversity patterns (i.e., species richness and community composition) are expected to change with global warming (Mota et al., 2022), what may disrupt key ecological functions, including those provided by birds (Sekercioglu, 2006).
Avian species are among the best known organisms, they are globally distributed and sensitive to environmental modifications, making them a potential study group to evaluate climate change impacts on biodiversity (Sekercioglu et al., 2012; Bregman et al., 2014). Additionally, birds contribute to several key ecological services, including seed dispersal, pollination, and insect control, thus providing the maintenance of natural and artificial environments (Sekercioglu, 2006; Morante-Filho and Faria, 2017; Blount et al., 2021). However, those services may be at risk due to the redistribution of species' suitable area resulting from climate change (e.g., Nowak et al., 2019). For instance, large seeds depend on large dispersers to carry them away from the parent tree, thus, the loss of frugivores such as toucans and guans may exert evolutionary pressure toward smaller seeds (Galetti et al., 2013; Sales et al., 2021). Climate-driven extinctions and few colonization are also predicted to occur in plant-hummingbird networks from North America and lowland South America communities (Sonne et al., 2022). Furthermore, high temperature and low rainfall in future climatic scenarios are expected to decrease taxonomic, phylogenetic, and functional diversity of bird species, leading to a restructuring of species assemblages around the world (Prieto-Torres et al., 2021; Stewart et al., 2022).
Although several studies have detected the pervasive effects of climate change on birds, it is still poorly understood how morphological, ecological and geographical traits can modulate this impact. For instance, birds presenting a low body mass may have high metabolic costs to keep thermoregulation, while larger ones may use metabolically more efficient mechanisms of evaporative heat loss (Smith et al., 2017). In addition, frugivorous birds from a Brazilian tropical forest are predicted to experience higher suitable area contractions compared to other trophic guilds under climate change (Miranda et al., 2019). Birds presenting restricted distributions are also predicted to be more vulnerable to global warming experiencing large suitable area contractions, while widespread species may be unaffected given that good capacity of dispersion, colonization, and maintenance of populations over large areas (Kunin and Gaston, 1997; Estrada et al., 2016; Cohen et al., 2020). Although recent studies attempt to evaluate the traits mediating birds' response to climate change, there is still no consensus. For instance, Pacifici et al. (2017) observed negative responses of birds from high altitudes, while Ortega et al. (2019) found the opposite pattern. In addition, both studies tested the effect of body mass on birds' response to climate change, but no significant relationship was found. Therefore, further investigations are needed to fully understand the impact of different traits on birds under global warming to help improve future projections and the conservation of this key species group to ecosystem functioning.
Here, we used morphological, ecological, and geographical traits obtained from Tobias et al. (2022) and Olson et al. (2001) to evaluate whether these features influence the amount of birds' suitable area in the future. Specifically, we tested whether body mass, diet, habitat type, movement pattern, range size, and biogeographic realm affect the amount of suitable area for birds registered in the literature. We expect that the amount of birds' suitable area in future scenarios will be positively related to range size and body mass, with species presenting restricted distributions and lower body mass losing more suitable area due to their already limited habitat and higher exposure to environmental variations, respectively. Additionally, we expect that forest dependent species, with specialized diet (e.g., frugivorous), and those from Oceania will suffer more contractions of suitable area compared to their counterparts due to specific requirements and lower habitat availability within isolated regions, respectively.
Materials and methods
We conducted a global literature search in the Web of Science (www.webofknowledge.com) and SCOPUS (www.scopus.com) on the first of March 2022, using the terms (in the study title, abstract, and keywords): “bird*” OR “avian” OR “avifauna” AND “climatic chang*” OR “climate chang*” OR “global warm*” OR “climate warm*” OR “changing climate” AND “distribut*” OR “suitab*” OR “niche model*” OR “scenario*” OR “range shift*”. After combining the pools of results and removing duplicates, we screened the title and abstracts from the remaining articles, removing any article that did not mention future climatic scenarios and birds' range size. We then rejected studies that did not report individually changes in suitability area of species (Figure 1).
Our literature search resulted in a final sample of 75 eligible studies (Supplementary Table 1) which were reviewed. For each study, we documented the following information: bird species name, climatic suitable area in baseline scenario, and the percentage of suitable area change or climatic suitable area in future scenarios regardless of the modeling approach used (e.g., algorithm, year, and emission scenario). Because few studies evaluate dispersion capacity, we registered only the suitable area in full dispersion scenarios which were available in all eligible studies, thus maximizing the observation number. Using the literature, we obtained the following information for each bird species: body mass, habitat type, movement pattern, diet, range size, and biogeographic realm (Olson et al., 2001; Tobias et al., 2022). Traits description are given in Supplementary Table 1. We choose these traits because they have already been used in other studies and are important in predicting bird extinction risk under climate change (e.g., Simmons et al., 2004; Machado and Loyola, 2013; Pacifici et al., 2017; Ortega et al., 2019). We used the range size of the known distribution of the species when the study did not provide the climatic suitable area in the baseline scenario. Finally, we standardized all area measures to square kilometers, given that few studies reported the suitable area of species as number of pixels.
We calculated the proportion of birds' suitable area in the future for each species and study, dividing the total amount of suitable area in the future scenario by the total area in the baseline scenario. Then, we applied square root transformation to this proportion in order to normalize our response variable. Since none of the predictor variables showed correlation higher than 0.36 that could justify its removal, we proceed to model building with all the six traits. We performed generalized linear mixed models using the glmmTMB package (Brooks et al., 2017) to model the proportion of suitable area of all bird species as a function of habitat type, movement pattern, body mass, range size, diet, and biogeographic realm. We used species as a random factor to control for those that appear in multiple studies. Afterward, we calculated all possible models, from the null to the global model, using the “dredge” function of the MuMIn package (Barton, 2022), and selected the best model based on the lowest Akaike information criterion corrected for small samples (AICc). Then, we used the best model to estimate marginal means of the traits using the packages emmeans (Lenth, 2022) and ggeffects (Lüdecke, 2018) to generate the graphics of isolated effects of each variable. All analyses were conducted in R software (Core Team, 2022).
Results
The 75 studies selected here modeled the future distribution of 1991 birds belonging to 129 families, with Tyrannidae as the most representative (n = 122). Most of the studies were conducted in Asia (31%) and Europe (28%), while less studies were carried out in Oceania (8%) and Africa (14%; Figure 2). We eliminated 330 species from our analysis because we could not obtain the traits for them, resulting in a final dataset with 1,661 species (83% of the total).
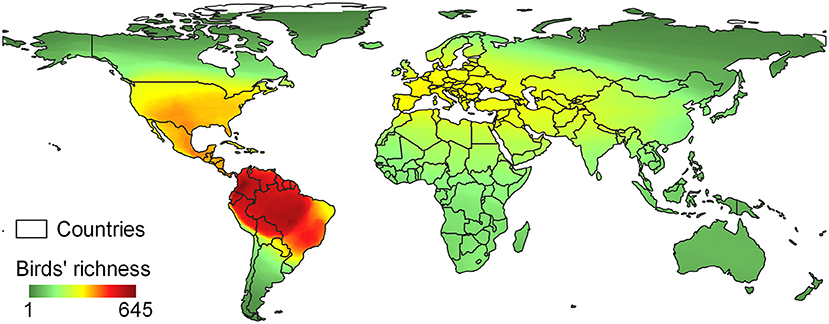
Figure 2. Map of birds' richness based on the distribution of the species included in our analysis among the eligible studies (n = 75).
We built a total of 64 models with AICc ranging from 8,232 to 8,179 and the best one included four predictor variables with relative importance higher than 0.5 (Supplementary Table 1): realm (w = 1), range size (w = 1), habitat type (w = 0.89), and body mass (w = 0.54). These variables showed significant effect on the proportion of birds' suitable area in the future.
We found a negative relationship between the proportion of birds' suitable area in the future and the baseline range size with suitable area loss increasing as the range size increases (β = −0.027, p = < 0.000; Figure 3A). The opposite pattern was observed for body mass, with the proportion of suitable area in the future increasing together with body mass (β = 0.021, p = < 0.009; Figure 3B).
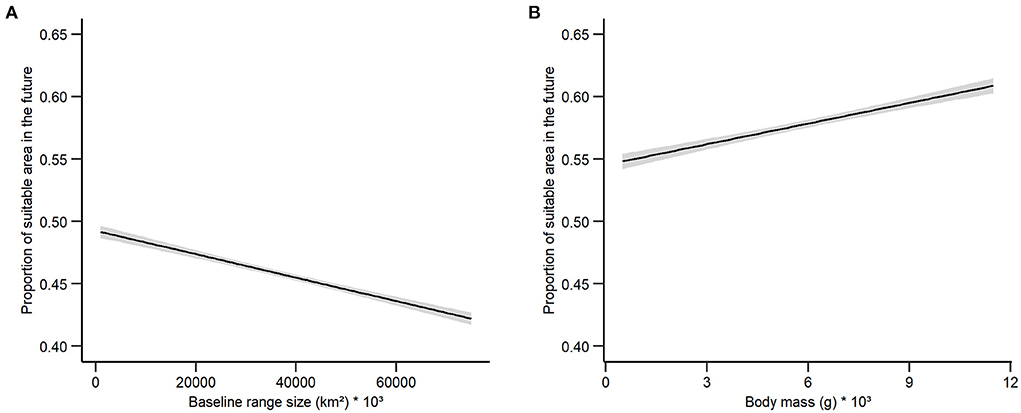
Figure 3. Relationship between the proportion of birds' suitable area in the future and (A) baseline range size, and (B) body mass. The gray area around the line indicates 95% confidence interval.
We found that the proportion of birds' suitable area differ among habitat type driven mostly by forest species, which present the lower average proportion of suitable area (0.73 ± 0.03), and species of woodland (0.95 ± 0.06) and shrubland (0.92 ± 0.05). Birds associated with riverine habitats showed a small gain of suitable area, indicated by values above 1, although the variation was one of the highest (1.02 ± 0.15; Figure 4A).
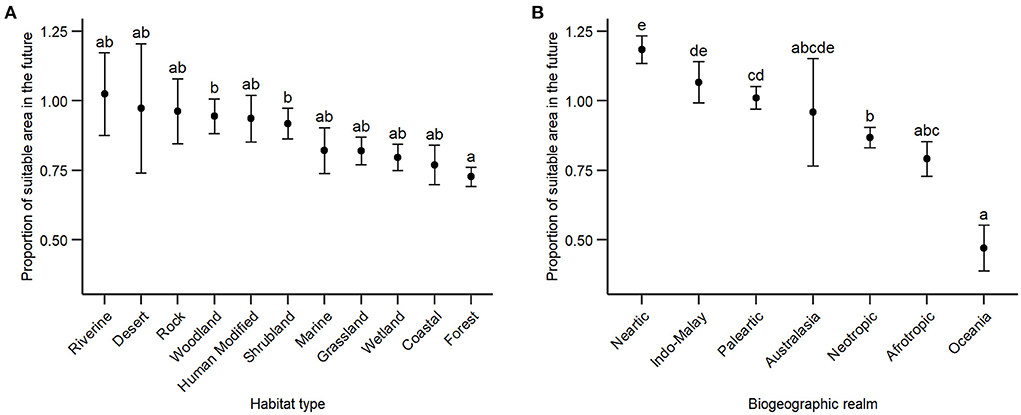
Figure 4. Relationship between the average (black dots) proportion of birds' suitable area and (A) habitat type, and (B) biogeographic realm. Values below 1 in y-axis represent suitable area loss. Different letters above the standard error of the mean indicate significant difference between two or more factors.
We found that the proportion of birds' suitable area differ among biogeographic realm driven mostly by species from Oceania, which present the lower average proportion of suitable area (0.47 ± 0.08), and species from Neartic, which present a small increase in the proportion of suitable area (1.18 ± 0.05). The Neotropical realm showed intermediate values, with a small reduction in the average proportion of suitable area (0.87 ± 0.04; Figure 4B).
Discussion
Here, we evaluate for the first time the effect of morphological, ecological, and geographical traits on the amount of future suitable area for birds worldwide. The most important mediators of species' response to climate change were range size, body mass, habitat type, and geographic realm, while diet and movement pattern showed lower predictive power and were not included in our final model.
Unexpectedly, the proportion of birds' suitable area decreased with increasing baseline range size, indicating that species with restricted distributions may lose less suitable area compared to widely distributed species. At first glance, it may seem contradictory given that climate conditions could completely change within a small area (Clark, 1985; Wilbanks and Kates, 1999), however, large distributions can potentially lose more area. The lower impact of climate change on restricted distributed birds suggests that these species may be located in regions with more stable climate (Borges and Loyola, 2020). In fact, we observed a positive relationship between range size and climate stability, measured by the difference in the annual mean temperature of future and baseline scenarios, supporting our result (Supplementary Figure 1). For instance, Crax blumenbachii had an average distribution of 18,420km2 located where the annual mean temperature in the future will increase only 1°C and is still predicted to lose 55% of its suitable area. Otherwise, Myiothlypis mesoleuca, another forest resident species, presented a larger average distribution (508,800 km2) and suitable area loss (74%), however, the region where this species is located will experience a temperature increase of 2.36°C. Additionally, a strong effect of stable climate was observed on endemic birds from China, resulting in higher species richness (Feng et al., 2020). Stable climate is also related to endemism hotspots that corresponds to areas with small paleoclimatic fluctuations (Harrison and Noss, 2017).
According to expectations, the proportion of birds' suitable area was positively related to body mass with smaller species predicted to suffer higher contractions in their suitable area compared to large species. This can be explained by high metabolic costs to keep thermoregulation in smaller species, while larger ones may use metabolically more efficient mechanisms of evaporative heat loss (Smith et al., 2017). For instance, the body mass of Crax fasciolata and Euphonia violacea are 2,600 and 15 g, respectively, both have similar range size and are forest frugivorous, but the smaller species are predicted to lose almost three times more suitable area than the larger one. It is corroborated by studies with birds from arid-zones that shows negative impact on fitness of smaller species and high tolerance to heat stress in larger species (du Plessis et al., 2012; Whitfield et al., 2015). Similar to our results, Mason et al. (2019) found a positive relationship between climate suitability trend and body mass for birds from the United States, which may be attributed to the presence of large bodied species.
Contractions in birds' suitable area are predicted for species associated with all habitat types, except for riverine, but higher losses are expected to forest species, corroborating our hypothesis. Forest-dependent birds require specific conditions such as several large frugivores that need of tree holes and fleshy-fruited trees for reproduction and feeding (Malanotte et al., 2019; Yatsiuk and Wesołowski, 2020), while the generalist habits of non-forest species allows species to obtain a wider range of resources in different types of habitats, including in disturbed environments (Vázquez-Reyes et al., 2022). For instance, large suitable area losses are predicted for forest dependent birds from Amazonia compared to species associated with open lands in future climate scenarios (Miranda et al., 2019). In fact, at least 29 forest birds are predicted to lose all suitable areas currently available in the next decades (Miranda et al., 2019). Similarly, forest birds in Madagascar are also expected to experience contractions in their total range areas and species such as Oriolia bernieri may not find suitable areas in future scenarios (Andriamasimanana and Cameron, 2013). Furthermore, it should be mentioned that even species predicted to expand their distributions are not safe, and their persistence may be limited by deforestation (Morante-Filho et al., 2021), suggesting that climate and land-use change can act synergistically and contribute to the impoverishment of birds communities (Northrup et al., 2019). For instance, although the endangered forest bird Polyplectron katsumatae is predicted to gain suitable area (11.8%), it has been facing habitat loss and hunting since the 1950s (IUCN, 2022).
Our findings support a larger reduction in the proportion of birds' suitable area from Oceania compared to other biogeographic realms. Oceania encompasses several small islands isolated from other continents, in addition to its particular conditions that prevent species from pursuing new suitable areas outside this region. For instance, we observed that birds from Oceania are predicted to lose an average of 80% of their suitable area, including species that will not find suitable area in the future such as Oreomystis bairdi and Loxops caeruleirostris, both forest-dependent species (Fortini et al., 2015; Paxton et al., 2016). Birds from other islands also suffer with drastic contractions in their suitable areas and common species may become rare with warming temperatures (Ko et al., 2012). In fact, the high vulnerability within islands is known due to the specific species interactions, isolation, and low habitat availability besides holding critically endangered species already threatened by other causes not related to climate (Whittaker and Fernández-Palacios, 2007; Kumar and Tehrany, 2017).
In our study we evidenced that the effect of climate change on the suitable areas for birds is modulated by range size, body mass, habitat type, and geographic realm. Although many studied species will experience contractions in their distributions, other birds are predicted to gain suitable area in the future, such as species from the Neartic realm and those associated with riverine habitat. However, our findings possibly underestimate range shifts since we did not include dispersion capacity of species (e.g., intermediate dispersion) and land-use change in our analysis, which we highly recommend in future studies as species may not be able to reach climatic suitable areas further apart or because these areas may become unsuitable due to habitat loss (see Ramirez-Villegas et al., 2014; Newbold, 2018; Prieto-Torres et al., 2020). Moreover, almost half of birds worldwide are already experiencing population decline due to other causes that may be worsened by climate change (Northrup et al., 2019; IUCN, 2022), and taking into account morphological, ecological, and geographical traits should help to assess species risk of extinction based on future projections (Pacifici et al., 2017). Finally, by knowing which traits make the species more vulnerable to climate change (i.e., high suitable area loss), we can maximize conservation efforts and better direct resources to prevent the impoverishment of bird communities.
Data availability statement
The datasets presented in this study can be found in online repositories. The names of the repository/repositories and accession number(s) can be found below: https://doi.org/10.6084/m9.figshare.c.5951910.
Author contributions
FMMM conducted the literature search, data analysis, and wrote the manuscript. All authors designed the study, contributed to manuscript revisions, read, and approved the final version.
Acknowledgments
We thank the Fundação de Amparo à Pesquisa do Estado da Bahia (FAPESB) for the PhD scholarship. NMH thanks the Coordenação de Aperfeiçoamento de Pessoal de Nível Superior (CAPES) for the post-doc fellowship. The manuscript benefited from constructive comments by André F. A. de Andrade, Diego Hoffmann, and Fábio J. A. Borges.
Conflict of interest
The authors declare that the research was conducted in the absence of any commercial or financial relationships that could be construed as a potential conflict of interest.
Publisher's note
All claims expressed in this article are solely those of the authors and do not necessarily represent those of their affiliated organizations, or those of the publisher, the editors and the reviewers. Any product that may be evaluated in this article, or claim that may be made by its manufacturer, is not guaranteed or endorsed by the publisher.
Supplementary material
The Supplementary Material for this article can be found online at: https://www.frontiersin.org/articles/10.3389/fevo.2022.987204/full#supplementary-material
References
Almazroui, M., Ashfaq, M., Islam, M. N., Rashid, I. U., Kamil, S., Abid, M. A., et al. (2021). Assessment of CMIP6 Performance and Projected Temperature and Precipitation Changes Over South America. Earth Syst. Environ. 5, 155–183. doi: 10.1007/s41748-021-00233-6
Andriamasimanana, R. H., and Cameron, A. (2013). Predicting the impacts of climate change on the distribution of threatened forest-restricted birds in Madagascar. Ecology and Evolution 3, 763–769. doi: 10.1002/ece3.497
Barton, K. (2022). MuMIn: Multi-Model Inference. R package version 1, 46.0. Available online at: https://CRAN.R-project.org/package=MuMIn (accessed July 5, 2022).
Bateman, B. L., Taylor, L., Wilsey, C., Wu, J., LeBaron, G. S., and Langham, G. (2020). Risk to North American birds from climate change-related threats. Conserv. Sci. Pract. 2, 1–15. doi: 10.1111/csp2.243
Bellard, C., Bertelsmeier, C., Leadley, P., Thuiller, W., and Courchamp, F. (2012). Impacts of climate change on the future of biodiversity. Ecol. Lett. 15, 365–377. doi: 10.1111/j.1461-0248.2011.01736.x
Blount, J. D., Horns, J. J., Kittelberger, K. D., Neate-Clegg, M. H. C., and Sekercioglu, Ç. H. (2021). Avian Use of Agricultural Areas as Migration Stopover Sites: A Review of Crop Management Practices and Ecological Correlates. Frontiers in Ecology and Evolution 9. Available at: https://www.frontiersin.org/articles/10.3389/fevo.2021.650641 (accessed July 4, 2022). doi: 10.3389/fevo.2021.650641
Borges, F. J. A., and Loyola, R. (2020). Climate and land-use change refugia for Brazilian Cerrado birds. Perspectives in Ecology and Conservation 18, 109–115. doi: 10.1016/j.pecon.2020.04.002
Bregman, T. P., Sekercioglu, C. H., and Tobias, J. A. (2014). Global patterns and predictors of bird species responses to forest fragmentation: Implications for ecosystem function and conservation. Biological Conservation 169, 372–383. doi: 10.1016/j.biocon.2013.11.024
Brooks, M. E., Kristensen, K., van Benthem, K. J., Magnusson, A., Berg, C. W., Nielsen, A., et al. (2017). glmmTMB Balances Speed and Flexibility Among Packages for Zero-inflated Generalized Linear Mixed Modeling. The R Journal 9, 378–400. doi: 10.32614/RJ-2017-066
Cohen, J. M., Fink, D., and Zuckerberg, B. (2020). Avian responses to extreme weather across functional traits and temporal scales. Global Change Biology 26, 4240–4250. doi: 10.1111/gcb.15133
Core Team, R. (2022). R: A language and environment for statistical computing. R Foundation for Statistical Computing, Vienna, Austria. Available online at: https://www.R-project.org (accessed July 5, 2022).
Drobinski, P., Da Silva, N, Bastin, S., Mailler, S., Muller, C., Ahrens, B., et al. (2020). How warmer and drier will the Mediterranean region be at the end of the twenty-first century? Reg. Environ. Chang. 20, 78. doi: 10.1007/s10113-020-01659-w
du Plessis, K. L., Martin, R. O., Hockey, P. A. R., Cunningham, S. J., and Ridley, A. R. (2012). The costs of keeping cool in a warming world: implications of high temperatures for foraging, thermoregulation and body condition of an arid-zone bird. Global Change Biol. 18, 3063–3070. doi: 10.1111/j.1365-2486.2012.02778.x
Estrada, A., Morales-Castilla, I., Caplat, P., and Early, R. (2016). Usefulness of species traits in predicting range shifts. Trends Ecol. Evol. 31, 190–203. doi: 10.1016/j.tree.2015.12.014
Feng, G., Huang, X., Mao, L., Wang, N., Yang, X., Wang, Y., et al. (2020). More endemic birds occur in regions with stable climate, more plant species and high altitudinal range in China. Avian Res. 11, 17. doi: 10.1186/s40657-020-00203-y
Fortini, L. B., Vorsino, A. E., Amidon, F. A., Paxton, E. H., and Jacobi, J. D. (2015). Large-scale range collapse of hawaiian forest birds under climate change and the need 21st century conservation options. PLoS ONE 10, e0140389. doi: 10.1371/journal.pone.0140389
Franchito, S. H., Rao, V. B., and Fernandez, J. P. R. (2012). Tropical land savannization: impact of global warming. Theor Appl Climatol. 109, 73–79. doi: 10.1007/s00704-011-0560-3
Galetti, M., Guevara, R., Côrtes, M. C., Fadini, R., Von Matter, S., Leite, A. B., et al. (2013). Functional Extinction of Birds Drives Rapid Evolutionary Changes in Seed Size. Science 340, 1086–1090. doi: 10.1126/science.1233774
Harrison, S., and Noss, R. (2017). Endemism hotspots are linked to stable climatic refugia. Annals of Botany 119, 207–214. doi: 10.1093/aob/mcw248
Hidasi-Neto, J., Joner, D. C., Resende, F., Monteiro, L. d. e. M., Faleiro, F. V., Loyola, R. D., et al. (2019). Climate change will drive mammal species loss and biotic homogenization in the Cerrado Biodiversity Hotspot. Persp. Ecol. Conser. 17, 57–63. doi: 10.1016/j.pecon.2019.02.001
IUCN (2022). The IUCN Red List of Threatened Species. Version 2022-1. Available online at: https://www.iucnredlist.org (accessed August 29, 2022).
Ko, C-. Y., Root, T. L., Lin, S-. H., Schneider, S. H., and Lee, P-. F. (2012). Global change projections for taiwan island birds: linking current and future distributions. Nat Conser. 2, 21–40. doi: 10.3897/natureconservation.2.2351
Kumar, L., and Tehrany, M. S. (2017). Climate change impacts on the threatened terrestrial vertebrates of the Pacific Islands. Sci Rep. 7, 5030. doi: 10.1038/s41598-017-05034-4
Kunin, W. E., and Gaston, K. J. (1997). The Biology of Rarity: Causes and Consequences of Rare–Common Differences. Germany: Springer. doi: 10.1007/978-94-011-5874-9
Lenoir, J., and Svenning, J. C. (2015). Climate-related range shifts—a global multidimensional synthesis and new research directions. Ecography 38, 15–28. doi: 10.1111/ecog.00967
Lenth, R. V. (2022). emmeans: Estimated Marginal Means, aka Least-Squares Means. R package version 1, 8.0. Available online at: https://CRAN.R-project.org/package=emmeans
Lüdecke, D. (2018). ggeffects: tidy data frames of marginal effects from regression models. J. Open Source Softw. 3, 772. doi: 10.21105/joss.00772
Machado, N., and Loyola, R. D. (2013). A comprehensive quantitative assessment of bird extinction risk in Brazil. PLoS ONE. 8, e72283. doi: 10.1371/journal.pone.0072283
Malanotte, M. L., Machado-de-Souza, T., Campos, R. P., Petkowicz, C. L. O., and Varassin, I. G. (2019). How do fruit productivity, fruit traits and dietary specialization affect the role of birds in a mutualistic network? J. Trop. Ecol. 35, 213–222. doi: 10.1017/S0266467419000178
Mason, L. R., Green, R. E., Howard, C., Stephens, P. A., Willis, S. G., Aunins, A., et al. (2019). Population responses of bird populations to climate change on two continents vary with species' ecological traits but not with direction of change in climate suitability. Clim. Change 157, 337–354. doi: 10.1007/s10584-019-02549-9
Menéndez-Guerrero, P. A., Green, D. M., and Davies, T. J. (2020). Climate change and the future restructuring of Neotropical anuran biodiversity. Ecography 43, 222–235. doi: 10.1111/ecog.04510
Miranda, L. S., Imperatriz-Fonseca, V. L., and Giannini, T. C. (2019). Climate change impact on ecosystem functions provided by birds in southeastern Amazonia. PLoS ONE 14, e0215229. doi: 10.1371/journal.pone.0215229
Morante-Filho, J. C., Benchimol, M., and Faria, D. (2021). Landscape composition is the strongest determinant of bird occupancy patterns in tropical forest patches. Landscape Ecol. 36, 105–117. doi: 10.1007/s10980-020-01121-6
Morante-Filho, J. C., and Faria, D. (2017). An Appraisal of Bird-Mediated Ecological Functions in a Changing World. Trop. Conserv. Sci. 10, 1940082917703339. doi: 10.1177/1940082917703339
Mota, F. M. M., Heming, N. M., Morante-Filho, J. C., and Talora, D. C. (2022). Climate change is expected to restructure forest frugivorous bird communities in a biodiversity hot-point within the Atlantic Forest. Divers. Distrib. 00, 1–12. doi: 10.1111/ddi.13602
Newbold, T. (2018). Future effects of climate and land-use change on terrestrial vertebrate community diversity under different scenarios. Proc. Royal Soc. B Biol. Sci. 285, 20180792. doi: 10.1098/rspb.2018.0792
Northrup, J. M., Rivers, J. W., Yang, Z., and Betts, M. G. (2019). Synergistic effects of climate and land-use change influence broad-scale avian population declines. Glob. Chang. Biol. 25, 1561–1575. doi: 10.1111/gcb.14571
Nowak, L., Kissling, W. D., Bender, I. M. A., Dehling, D. M., Töpfer, T., Böhning-Gaese, K., et al. (2019). Projecting consequences of global warming for the functional diversity of fleshy-fruited plants and frugivorous birds along a tropical elevational gradient. Div. Distrib. 25, 1362–1374. doi: 10.1111/ddi.12946
Olson, D. M., Dinerstein, E., Wikramanayake, E. D., Burgess, N. D., Powell, G. V. N., Underwood, E. C., et al. (2001). Terrestrial ecoregions of the world: a new map of life on earth: a new global map of terrestrial ecoregions provides an innovative tool for conserving biodiversity. BioScience 51, 933–938. doi: 10.1641/0006-3568(2001)051[0933:TEOTWA]2.0.CO;2
Ortega, J. C. G., Machado, N., Diniz-Filho, J. A. F., Rangel, T. F., Araújo, M. B., Loyola, R., et al. (2019). Meta-analyzing the likely cross-species responses to climate change. Ecol. Evol. 9, 11136–11144. doi: 10.1002/ece3.5617
Pacifici, M., Visconti, P., Butchart, S. H. M., Watson, J. E. M., Cassola, F. M., Rondinini, C., et al. (2017). Species' traits influenced their response to recent climate change. Nat. Clim Change 7, 205–208. doi: 10.1038/nclimate3223
Paxton, E. H., Camp, R. J., Gorresen, P. M., Crampton, L. H., Leonard, D. L., VanderWerf, E. A., et al. (2016). Collapsing avian community on a Hawaiian island. Sci. Adv. 2, e1600029. doi: 10.1126/sciadv.1600029
Pearman, P. B., Guisan, A., and Zimmermann, N. E. (2011). Impacts of climate change on Swiss biodiversity: An indicator taxa approach. Biol. Conserv. 144, 866–875. doi: 10.1016/j.biocon.2010.11.020
Prieto-Torres, D. A., Lira-Noriega, A., and Navarro-Sigüenza, A. G. (2020). Climate change promotes species loss and uneven modification of richness patterns in the avifauna associated to Neotropical seasonally dry forests. Perspect. Ecol. Conserv. 18, 19–30. doi: 10.1016/j.pecon.2020.01.002
Prieto-Torres, D. A., Sánchez-González, L. A., Ortiz-Ramírez, M. F., Ramírez-Albores, J. E., García-Trejo, E. A., Navarro-Sigüenza, A. G., et al. (2021). Climate warming affects spatio-temporal biodiversity patterns of a highly vulnerable Neotropical avifauna. Clim. Change 165, 57. doi: 10.1007/s10584-021-03091-3
Ramirez-Villegas, J., Cuesta, F., Devenish, C., Peralvo, M., Jarvis, A., Arnillas, C. A., et al. (2014). Using species distributions models for designing conservation strategies of Tropical Andean biodiversity under climate change. J. Nat. Conserv. 22, 391–404. doi: 10.1016/j.jnc.2014.03.007
Sales, L. P., Galetti, M., and Pires, M. M. (2020). Climate and land-use change will lead to a faunal “savannization” on tropical rainforests. Global Change Biol. 26, 7036–7044. doi: 10.1111/gcb.15374
Sales, L. P., Kissling, W. D., Galetti, M., Naimi, B., and Pires, M. M. (2021). Climate change reshapes the eco-evolutionary dynamics of a Neotropical seed dispersal system. Global Ecol. Biogeogr. 30, 1129–1138. doi: 10.1111/geb.13271
Sekercioglu, C. H. (2006). Increasing awareness of avian ecological function. Trends Ecol. Evol. 21, 464–471. doi: 10.1016/j.tree.2006.05.007
Sekercioglu, Ç. H., Primack, R. B., and Wormworth, J. (2012). The effects of climate change on tropical birds. Biol. Conserv. 148, 1–18. doi: 10.1016/j.biocon.2011.10.019
Simmons, R. E., Barnard, P., Dean, W., Midgley, G. F., Thuiller, W., Hughes, G., et al. (2004). Climate change and birds: perspectives and prospects from southern Africa. Ostrich 75, 295–308. doi: 10.2989/00306520409485458
Smith, E. K., O'Neill, J. J., Gerson, A. R., McKechnie, A. E., and Wolf, B. O. (2017). Avian thermoregulation in the heat: resting metabolism, evaporative cooling and heat tolerance in Sonoran Desert songbirds. J. Exp. Biol. 220, 3290–3300. doi: 10.1242/jeb.161141
Sonne, J., Maruyama, P. K., Martín González, A. M., Rahbek, C., Bascompte, J., Dalsgaard, B., et al. (2022). Extinction, coextinction and colonization dynamics in plant–hummingbird networks under climate change. Nat. Ecol. Evol. 6, 720–729. doi: 10.1038/s41559-022-01693-3
Stewart, P. S., Voskamp, A., Santini, L., Biber, M. F., Devenish, A. J. M., Hof, C., et al. (2022). Global impacts of climate change on avian functional diversity. Ecol. Lett. 25, 673–685. doi: 10.1111/ele.13830
Thomas, C. D. (2010). Climate, climate change and range boundaries. Div. Distrib. 16, 488–495. doi: 10.1111/j.1472-4642.2010.00642.x
Tobias, J. A., Sheard, C., Pigot, A. L., Devenish, A. J. M., Yang, J., Sayol, F., et al. (2022). AVONET: morphological, ecological and geographical data for all birds. Ecol. Lett. 25, 581–597. doi: 10.1111/ele.13898
Vázquez-Reyes, L. D., Paz-Hernández, H., Godínez-Álvarez, H. O., Arizmendi, M. del C., and Navarro-Sigüenza, A. G. (2022). Trait shifts in bird communities from primary forest to human settlements in Mexican seasonal forests. Are there ruderal birds? Perspect. Ecol. Conserv. 20, 117–125. doi: 10.1016/j.pecon.2021.11.005
Whitfield, M. C., Smit, B., McKechnie, A. E., and Wolf, B. O. (2015). Avian thermoregulation in the heat: scaling of heat tolerance and evaporative cooling capacity in three southern African arid-zone passerines. J. Exp. Biol. 218, 1705–1714. doi: 10.1242/jeb.121749
Whittaker, R. J., and Fernández-Palacios, J. M. (2007). Island biogeography: ecology, evolution, and conservation. New York: Oxford University Press.
Wilbanks, T. J., and Kates, R. W. (1999). Global change in local places: how scale matters. Clim. Change 43, 601–628. doi: 10.1023/A:1005418924748
Keywords: climate change, ecological niche model (ENM), bird traits, suitability, systematic review, species distribution
Citation: Mota FMM, Heming NM, Morante-Filho JC and Talora DC (2022) Amount of bird suitable areas under climate change is modulated by morphological, ecological and geographical traits. Front. Ecol. Evol. 10:987204. doi: 10.3389/fevo.2022.987204
Received: 05 July 2022; Accepted: 08 September 2022;
Published: 28 September 2022.
Edited by:
Rafael Arruda, Federal University of Mato Grosso, BrazilReviewed by:
Ana Claudia Almeida, Universidade Federal de Mato Grosso do Sul, BrazilKamila Prado Cruz Serra Thomas, Federal University of Mato Grosso, Brazil
Copyright © 2022 Mota, Heming, Morante-Filho and Talora. This is an open-access article distributed under the terms of the Creative Commons Attribution License (CC BY). The use, distribution or reproduction in other forums is permitted, provided the original author(s) and the copyright owner(s) are credited and that the original publication in this journal is cited, in accordance with accepted academic practice. No use, distribution or reproduction is permitted which does not comply with these terms.
*Correspondence: Flávio Mariano Machado Mota, ZmxhdmlvbW9jQGdtYWlsLmNvbQ==