- 1Institute of Vertebrate Biology, Czech Academy of Sciences, Brno, Czechia
- 2Department of Botany and Zoology, Faculty of Science, Masaryk University, Brno, Czechia
- 3Department of Ecology and Vertebrate Zoology, University of Łódź, Łódź, Poland
The origin, incidence, and consequences of reproductive senescence vary greatly across the tree of life. In vertebrates, research on reproductive senescence has been mainly focused on mammals and birds, demonstrating that its variation is largely linked to critical life history traits, such as growth patterns, juvenile, and adult mortality, and reproductive strategy. Fishes represent half of the vertebrate taxonomic diversity and display remarkable variation in life history. Based on a thorough literature review, we summarize current evidence on reproductive senescence in ray-finned fishes (Actinopterygii). While survival and physiological senescence are acknowledged in fish, their potential age-related reproductive decline has often been disregarded due to the prevalence of indeterminate growth. We demonstrate that age-related reproductive decline is reported across fish phylogeny, environments, and traits. An important point of our review is that the incidence of reproductive senescence in a species depends on both the number of studies for that species and the coverage of its maximum lifespan by the study. Reproductive senescence was documented for one-third of the studied fish species, with females suffering an age-related decline in reproductive traits less often than males or both parents combined. Neither parental care nor migratory strategy corresponded with the occurrence of reproductive senescence in fish. The traits that were affected by reproductive senescence most often were sex-specific, with pre-mating and mating categories of traits declining in females and sperm quality and quantity in males. We also demonstrate that reproductive senescence can be buffered by indeterminate growth. We provide rich evidence of reproductive senescence across ray-finned fishes, but we highlight the need for better data on age-related reproduction in fishes.
1 Introduction
Individual survival and offspring recruitment are two fundamental parameters of population dynamics. It is therefore important to understand age-related changes in mortality and in reproductive success, especially in exploited populations and populations facing environmental changes (Venturelli et al., 2012). At the individual level, it is the physiological decline that affects the prospect of survival and the potential decrease in the quality and quantity of offspring, which leads to senescence (Shefferson et al., 2017). The onset and rate of reproductive senescence clearly affect individual fitness (Bouwhuis et al., 2012; Austad and Finch, 2022), although, they are traditionally considered secondary aspects of overall senescence (Comfort, 1954). The organismal diversity in the rate of actuarial (demographic) senescence received particular attention (Jones et al., 2014), but a robust framework for specific analyses of the pace and shape of reproductive parameters has only recently been developed (Baudisch and Stott, 2019). The most comprehensive data on organismal age-related reproductive decline have so far come from avian and mammalian studies (Ricklefs et al., 2003; Lemaître and Gaillard, 2017; Lemaître et al., 2020; Vágási et al., 2021). The understanding of reproductive senescence across major clades of vertebrates is largely incomplete (Finch and Holmes, 2010; Nussey et al., 2013; Jones et al., 2014; Ivimey-Cook and Moorad, 2020), and existing gaps prevent the identification of potential ecological and evolutionary covariates and factors associated with reproductive senescence. This is a pressing problem as some lineages show a markedly higher rate of senescence than others (Ricklefs, 2010).
In this study, we address reproductive senescence in fishes, a group characterized by indeterminate growth and fecundity tightly related to body size (similar to non-avian reptiles; Hoekstra et al., 2020). Fishes are therefore an attractive target to search for evolutionary correlates of negligible senescence (Vaupel et al., 2004; Roper et al., 2021). As older fish become even larger, their reproductive output increases (Woodhead, 1998; Reznick et al., 2002), often disproportionately to their body size (Barneche et al., 2018). Indeed, the importance of large old females is well recognized in fish-stock assessments (Green, 2008). Ray-finned fishes (Actinopterygii) represent half of all vertebrate species (34,800 out of 70,000; IUCN, 2021; Froese and Pauly, 2022), which is reflected in their incredible life history and ecological diversity (Helfman et al., 2009). Fishes exhibit almost any mode of reproduction expressed across vertebrates (Wootton and Smith, 2015). Post-reproductive lifespan, typical for certain mammalian species (Cohen, 2004), is unexpectedly also reported in live-bearing fishes (Poeciliidae; Krumholz, 1948; Reznick et al., 2006). On the other hand, Pacific salmons (Oncorhynchus spp.; Crespi and Teo, 2002; Morbey et al., 2005) are an emblematic group known for a “big bang” reproduction, semelparity. The semelparous fish reproduce only once in a lifetime after which they die, and they are not restricted to salmonids (e.g., a galaxiid Galaxiella pusilla; Pen et al., 1993). Fishes contain both one of the earliest reproducing (Nothobranchius furzeri) and the longest reproductively active vertebrate species (Sebastes aleutianus; Vrtílek et al., 2018; Kolora et al., 2021). Overall, ray-finned fishes offer an opportunity to study multiple perspectives of life-history evolution and reproductive senescence, in particular.
Reproduction imposes a physiological cost that intensifies with age [hypothesized by Orton (1929) in Kelley (1962) and empirically studied by Craig (1977)]. This leads to a lowered allocation to reproduction, including skipping spawning in some years, to mitigate the costs that might increase the risk of mortality. Several studies on captive individuals indicated that reproductive senescence is prevalent in fish (Krumholz, 1948; Rasquin and Hafter, 1951; Woodhead, 1974a,b). The pathophysiological changes are in many aspects similar to mammals (Rasquin and Hafter, 1951; Patnaik et al., 1994). Reproductive senescence may express through various traits linked to offspring production, from mating behavior through gonad malfunction to offspring viability (Lemaître and Gaillard, 2017; Monaghan and Metcalfe, 2019). Our current knowledge of reproductive senescence from fish is, however, limited and fragmented (Finch and Holmes, 2010). The existing reviews on fish senescence (Craig, 1985; Patnaik et al., 1994; Woodhead, 1998; Reznick et al., 2002) are already outdated as the evidence that fish experience reproductive senescence is accumulating both from captive (Gasparini et al., 2019; Žák and Reichard, 2021) and wild populations (Karjalainen et al., 2016; Benoît et al., 2018). In this review, we aim to investigate published literature records for data on reproductive senescence across fish species with the overarching question “What evidence do we currently have for an age-related reproductive decline in ray-finned fishes?” We further explored three hypotheses on the occurrence of senescence in fish.
While fish were often considered immortal (thanks to their indeterminate growth) in the first half of the 20th century (Bidder, 1932), Comfort (1961), and Woodhead (1974a,b) demonstrated senescence in small ornamental fish. Given our growing understanding of the effect of indeterminate growth on reproductive senescence across vertebrates (Reinke et al., 2022), we hypothesized that, with more recent research, we will observe an increase in the proportion of studies reporting reproductive senescence in fishes.
Migration requires additional resources and increases the risks, and migratory birds and mammals show a faster pace of life than non-migratory species (Soriano-Redondo et al., 2020). We predicted that migrating strategy of a population will incur a higher probability of finding senescence in fish reproductive traits.
In addition, we consider an association between parental care and the occurrence of reproductive senescence. Parental care extends contact between the offspring and its parent(s) and exerts significant costs on the caring parent (Clutton-Brock et al., 1989). It, therefore, has the potential to increase the negative impacts of senescence on the next generation. We predicted that species providing parental care will therefore be more likely to show reproductive senescence.
2 Methods
The present literature review is focused on examining evidence for reproductive senescence in fish. We defined three selection criteria for assessing an article to be suitable for our review. The article should be on ray-finned fish (Actinopterygii), which implies that we should exclude studies on sharks, rays, lampreys, etc. The second criteria was that the age of the fish should be determined by reading growth rings from otoliths, scales, fin rays, or bones, or through a known identity of the individuals (e.g., by marking or rearing them in captivity). We, therefore, excluded studies that assigned age using population body size distribution, for example, because variation in individual growth trajectory may bias correct age identification. The last criterion was that the study should look at a reproductive trait in relationship with the age of the fish. Overall, we aimed at an expanded selection of articles, including those that do not directly focus on reproductive senescence, to obtain a wider perspective on the relationship of reproduction with age and body size across fishes. A reproductive trait was any trait associated with offspring production, that is, related to mating ability, gonads, egg, or sperm amount and quality, fertilization, number and performance of the offspring, and parental care (see below for details). Only studies on adult fish were thus included in our database for review. We preregistered the review at Open Science Forum.1
On 28 September 2021, we performed two searches for literature on fish reproductive senescence, one on the Web of Science (WOS)2 and the other on Scopus.3 We used string representing our three criteria related to reproduction, age effect, and fish:
(reproduc* OR fertil* OR fecund* OR egg* OR breed* OR “offspring” OR hatch* OR parent*) AND (senesc* OR “ageing” OR “aging” OR “age” OR old*) AND (fish OR teleost* OR actinopteryg* OR pisc*).
We obtained 12,617 and 16,685 results from WOS and Scopus, respectively (29,302 in total). We downloaded bibliographical data for the articles, including the title and abstract, and merged the two datasets into a single xls file. We screened the collected articles in two steps: to identify suitable studies and then to extract relevant data for age-related reproductive traits from these studies.
In the first step, we screened titles and abstracts of the articles and only kept those that were on Actinopterygii and measured the effect of age on some reproductive traits. The excluded studies were labeled as “off-topic” (not on fish biology), “not age” (age not properly determined or on subadult stages), or “repage missing” (age was recorded but no reproductive trait was followed). For the original collection of articles, see the doi: 10.6084/m9.figshare.20200337. We provide an overview of the screening steps and the number of articles in a PRISMA flowchart (Moher et al., 2009), as given in Supplementary File 1.
We performed a second, thorough search in full-texts of the pre-selected articles (1,249 after duplicates were removed), particularly their Sections “Methods” and “Results.” At this stage, reviews were searched for an additional primary source of data (we screened 17 additional primary articles detected in reviews, which made the total number of articles 29,319). We were not able to recover the full text for six articles, and these were eventually removed from the final database. In the second screening, we aimed to confirm that our three selection criteria were indeed met and we extracted study-, species-, and trait-specific information from each article. In addition to the already obtained bibliographical data, we searched for details on the study environment and setup, species, age, and sex of the fish, and most importantly for information on the reproductive trait and its relationship with age and body size. Full annotation of our final database is available in Supplementary File 2, so we provide only a brief overview here.
The environmental factors extracted were climate (category according to general climate zones–tropical, subtropical, temperate, or polar) and type of aquatic habitat (freshwater, brackish, or marine). We noted the study setup as whether it was captive or wild and whether authors targeted specific age classes (targeted study) or investigated the available age distribution (observational study). We assigned the currently accepted Latin name to the study species (according to FishBase, Froese and Pauly, 2022) with its taxonomic position (according to Fish Tree of Life, Rabosky et al., 2018). We also searched for species with the maximum known lifespan and mode of parental care (viviparous, biparental, maternal or paternal guarding, and no care or unknown) in FishBase (Froese and Pauly, 2022). We then noted using the age-determination method from the study, the minimum and maximum age of the fish, their sex (female, male, or both for parental traits), whether they were sampled alive or dead, and whether the study population was migratory or not. For each record of reproductive trait, we included its general description and grouped traits into trait types given the reproduction sequence with “pre-mating and mating,” “gamete quality,” “gamete number,” “gonads,” “pre-hatching,” or “post-hatching” categories. We assessed the direction of the age effect in relation to reproductive success (i.e., positive or negative age effect rather than positive or negative correlations), whether age was continuous or categorical variable, what test was used, and whether the effect size was measured. We similarly proceeded to the relationship between the reproductive trait and body size. In addition to that, we noted body size variable (e.g., somatic weight or standard length) and its goodness-of-fit relative to age (typically r or R2). At the end, we had to drop one article where the effect of age on reproductive traits was tested, but the direction was unknown. The final dataset can be found by following the doi: 10.6084/m9.figshare.20200337.
We identified indications of reproductive senescence based on a negative or bell-shaped relationship between age and a reproductive trait. Not all studies statistically tested the relationship between age and reproductive traits. Some contained only verbal statements about the relationship. In others, we had to infer the direction from mean values for age classes or plotted data. The direction of the relationship between age and a reproductive trait was therefore based on our interpretation of the study results, be it a statistical test, figure, or a plain verbal claim. We interpret the evidence for reproductive senescence across a combination of parameters mainly using pivot tables. We summarize the proportion of the records with reproductive senescence together with the overall number of records of the age–reproduction relationship. The general patterns are similar between studies from the subset containing statistically analyzed data and the entire collection. We, therefore, present the results from all studies collected. For the results with only the records that were statistically tested in the primary literature, refer to Supplementary File 3.
We tested the effect of publication year on the occurrence of reproductive senescence in a study-specific manner. All studies that contained at least one record of a negative or bell-shaped relationship between a reproductive trait and age were taken as positive cases (1, reproductive senescence present) and those without any evidence for negative or bell-shaped relationship were scored as negative (0). For species, we tested the effect of research attention and the presence of parental care on the probability of reporting reproductive senescence in a similar manner. Reproductive senescence in a species was a binomial response variable (0, if no study found reproductive senescence in that species or 1 for species with a record of reproductive senescence). The number of studies per species or the presence of parental care (“yes” for viviparity or parental guarding and “no” for no care, species with unavailable information were removed) were the explanatory variables. We tested the effect of the publication year, the effect of the number of studies in a species, and the species’ parental care on finding reproductive senescence using three different binomial generalized linear models (GLM).
We further analyzed the coverage of species-specific lifespan by maximum age in studies from our database. For each record, we calculated the lifespan index as a ratio between the study’s maximum age and the documented species’ lifespan from FishBase (Froese and Pauly, 2022). We then tested the effect of the study setup (captive vs. wild) on lifespan index distribution using a t-test. In addition to that, we tested the relationship between lifespan index and the occurrence of reproductive senescence using a binomial generalized mixed-effects model (GLMM) from package ‘lme4’ v.1.1-27 by Bates et al. (2015). Reproductive senescence in a record was a response variable (coded 0 or 1 as above), lifespan index was an explanatory variable, and we included study ID as a random effect (there were multiple records from some studies). Statistical analyses were performed in R v.4.0.5 (R Development Core Team, 2021).
3 Results
3.1 Overview of records of fish age-reproduction relationships
The initial search yielded 29,319 articles. After screening and removing the duplicates, 409 studies conformed with our three-selection criteria, namely being ray-finned fish (Actinopterygii), measuring age, and recording at least one reproductive trait. We extracted 1,078 individual records of reproductive traits related to age across 258 fish species belonging to at least 35 taxonomic orders (Figure 1). The species with the most commonly retrieved relationship between age and a reproductive trait were rainbow trout (Oncorhynchus mykiss), common carp (Cyprinus carpio), zebrafish (Danio rerio), and vendace (Coregonus albula), all with approximately 4% share of the entire dataset. The most represented taxonomic orders were Cypriniformes, Salmoniformes, and Perciformes with 24, 16, and 16% shares, respectively.
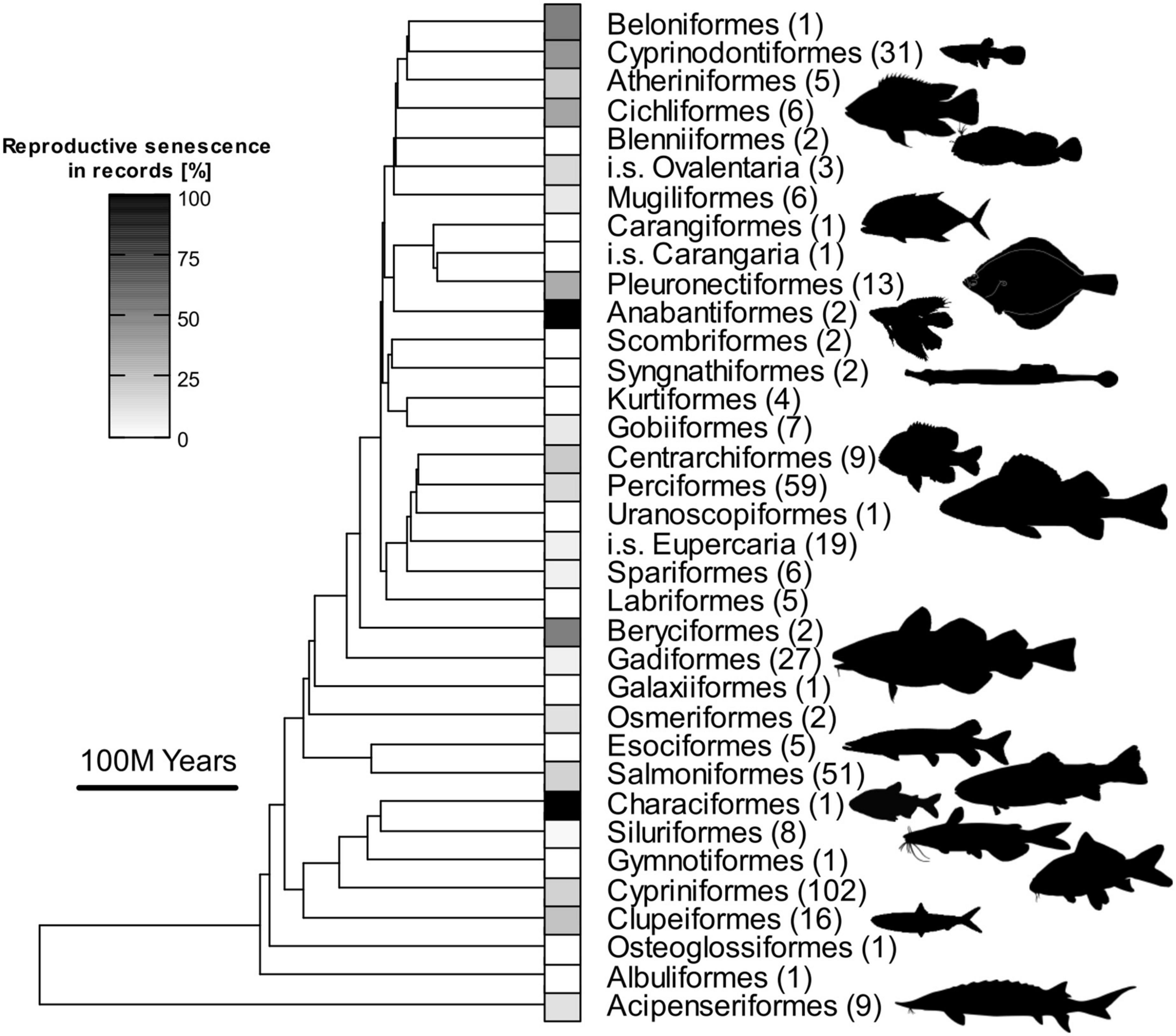
Figure 1. Records of reproductive senescence across the phylogeny of ray-finned fish (Actinopterygii). More intense black at the tip square points illustrates a higher proportion of negative or bell-shaped relationships between a reproductive trait and age among all the age-reproduction relationships (proportion of reproductive senescence records). The numbers in brackets show the total number of studies for each fish order. Fish silhouettes come from phylopic.org and fishualize R package (https://CRAN.R-project.org/package=fishualize). Please note that only the orders with a record of age effect on reproduction are presented.
With respect to the environment, records on age-related reproduction came mostly from temperate freshwaters (38%), followed by subtropical freshwaters (22%), and temperate marine environments (17%) (Table 1). Our database of reproductive parameters demonstrated a strong sex-biased distribution in data on the relationships between age and a reproductive trait (i.e., irrespective of a reproductive senescence pattern). Most (74%) of the age-related reproductive traits were measured in females, 20% were from males, and 5% were from both sexes. Most records (65%) were from wild populations and 35% were obtained in captivity. The most-recorded reproductive trait was female gamete quantity (34%), followed by female gamete quality (14%) (Table 2).
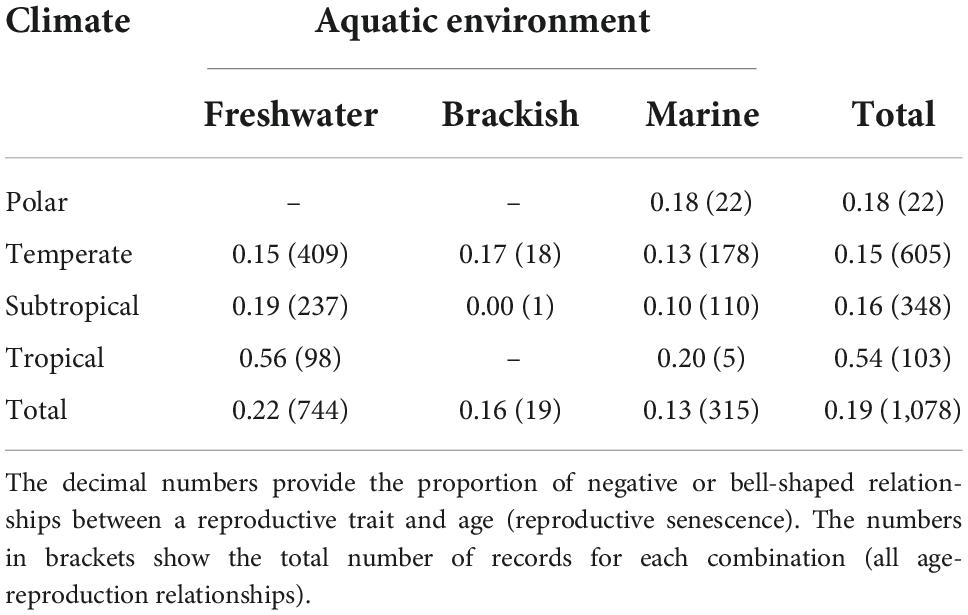
Table 1. Distribution of the reproductive senescence records across different climatic and aquatic environments.
3.2 Evidence for reproductive senescence
A negative or bell-shaped relationship between age and a reproductive trait was considered to be a sign of reproductive senescence. In general, we found reproductive senescence in 26% of the suitable studies (106 of 409 studies) and a total of 19% of age-reproductive trait records (205 of all 1,078 records). In the section below, we detail individual factors that might have an effect on the incidence of reproductive senescence in fish.
3.2.1 The year when the study was published
During the 20th century, the existence of reproductive senescence in fish gradually gained recognition. We tested whether the number of articles reporting reproductive senescence increased over time. However, the effect of publication year on the proportion of studies with a record of reproductive senescence was not apparent (binomial GLM: df = 407, z = 1.01, P = 0.313).
3.2.2 Study environment and age manipulation
The type of climate and the aquatic environment were not associated with the incidence of reproductive senescence, with approximately 20% of the records in each category (Table 1). The only exception was tropical freshwaters, where the incidence of reproductive senescence was particularly high (56%) (Table 1). This is most likely due to the tropical freshwater species, such as D. rerio, Poecilia reticulata, or N. furzeri, being often studied specifically for senescence in the laboratory. The records from captive studies were more likely to report reproductive senescence than studies from the wild populations (31 vs. 13%). This was more important for the separation of the records than the contrast between observational and targeted studies. A total of 26% of the records from targeted studies (that manipulated age distribution) demonstrated reproductive senescence, while 16% of age-reproductive trait records showed signs of senescence in observational studies. This suggests that wild fish populations are much less likely to exhibit measurable reproductive senescence compared to captive populations, without much influence of age distribution manipulation.
3.2.3 Migration
We predicted that migratory strategy is more likely to be associated with a negative age effect on reproductive performance than non-migratory. Only 16% of the records of reproductive traits, however, showed a decline with age in migratory populations compared with 20% in non-migratory populations.
3.2.4 Parental care
The presence of viviparity or parental guarding did not explain variation in the occurrence of reproductive senescence among fish species (binomial GLM: df = 242, z = 0.52, P = 0.601). The mode of parental care (viviparity, parental guarding, or no care) does not increase the proportion of senescence in records related to fish mating or post-fertilization traits (pre- or post-hatching) in either sex (Supplementary File 4). The same pattern was apparent for gamete quality, quantity, and gonad-related traits (data not shown).
3.2.5 Type of reproductive trait
In females, the highest proportion of records with reproductive senescence belongs to the “pre-mating and mating” category (51%), such as breeding frequency or proportion of reproductively active females. In males, the quality and quantity of sperm are the main traits indicating reproductive senescence (41 and 39%, respectively). Where the age of both parents was recorded, both pre- and post-hatching categories of offspring traits demonstrated a relatively high proportion of senescence effects as well (i.e., one-third of the records, refer to Table 2). Senescence thus seems to affect different types of reproductive traits between sexes.
3.2.6 Maximum age coverage (lifespan index)
To analyze species-specific lifespan coverage by the maximum age in our database, we collected data on species lifespan for 745 of all age-reproduction records (69%). The lifespan index (ratio between the study’s maximum age and species’ lifespan) was higher in studies of wild populations compared to captive studies (study-specific t-test: t57 = 2.70, P = 0.009; Figure 2A). Records with higher lifespan indexes were more likely to report reproductive senescence (binomial GLMM test: N = 745, z = 2.04, P = 0.042; Figure 2B). This means that records from the wild populations and with reproductive senescence were collected from individuals of older age (closer to the known species’ maximum age).
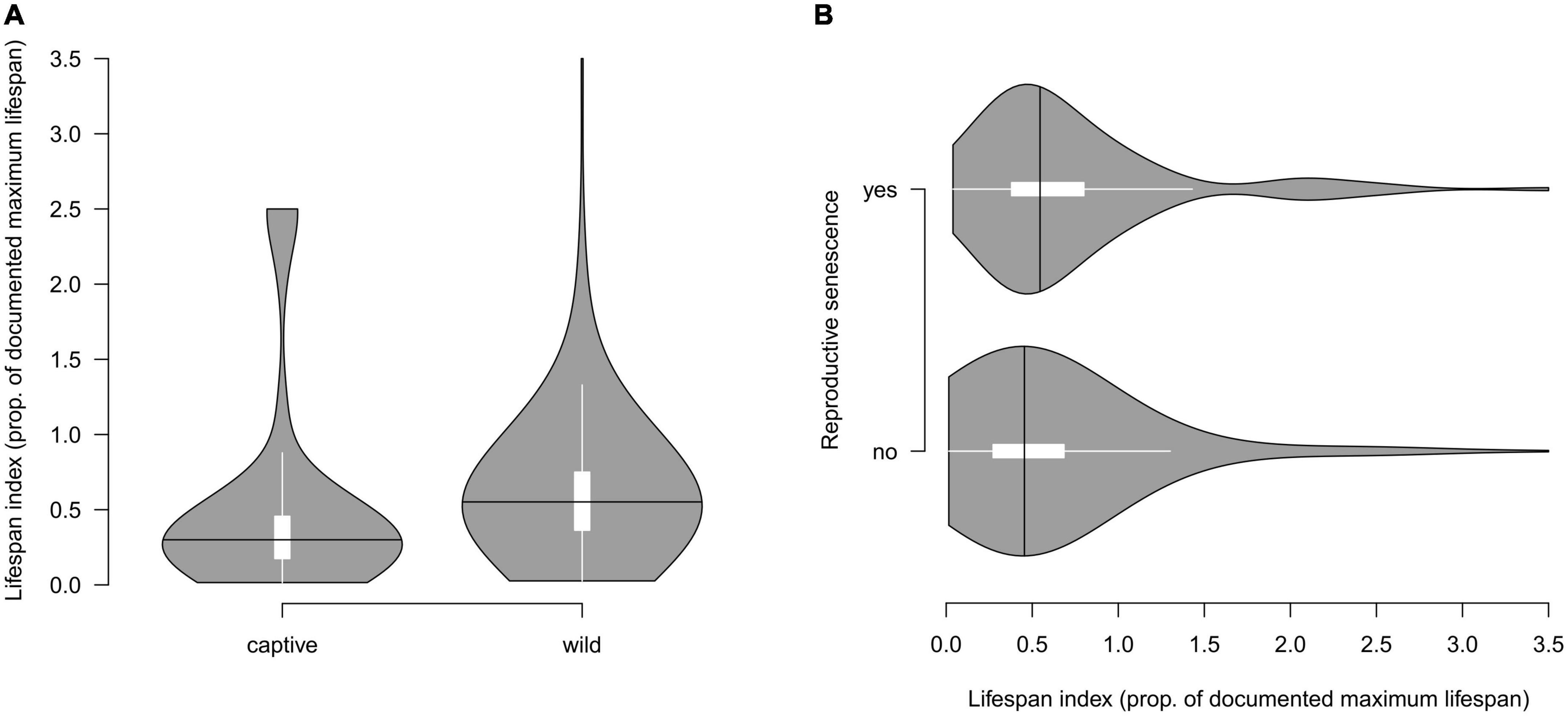
Figure 2. The coverage of species-specific maximum lifespans. The lifespan index is a ratio between the study’s maximum age and documented species’ lifespan. (A) Distribution of lifespan index in records from captive and wild populations. (B) Relationship between lifespan index and confirmed reproductive senescence. The width of the violin plots illustrates the density of the data points, the white inset box represents the interquartile range and the black crossing line indicates the median of the lifespan index. Note that the age reported in some studies exceeded the maximum known age for the species (retrieved from FishBase, Froese and Pauly, 2022).
3.2.7 Body size confounding the effect of age
Older fish individuals are also larger due to their indeterminate growth. This may bias the analysis of the age effect on reproductive traits if this relationship is not properly accounted for (e.g., with ANCOVA). As the ANCOVA and models combining age and size were relatively uncommon in the collected studies (158 records, 15% of all), we decided to directly compare the effects of these two variables in the records of reproductive traits. The effect of body size on a reproductive trait was measured along with age for 527 records (49% of our dataset). In 48 records of reproductive senescence, 31% of body size effects were negative or bell-shaped. For the records without reproductive senescence (479), 96% had either no or a positive effect of body size on the reproductive trait.
In addition to this, we retrieved goodness-of-fit measures of both age and body size for 304 records. Only 21 of those were for records of reproductive senescence, where the body size variable was a superior fit compared to the age for 16 records of reproductive traits (76%) and an inferior fit for 5 records (24%). For the records without clear signs of reproductive senescence, body size was generally (74%) a better fit to the reproductive traits data than age. Overall, the two subset analyses suggest that body size has indeed often a stronger explanatory power in fish reproductive trait variation than the age effect.
3.3 Species focus
We found signs of reproductive senescence in at least one study for 31% of species from our article collection. Relatively, more studied species had a higher probability to provide evidence of reproductive senescence because the likelihood to find a record of reproductive senescence for the particular species increased with the number of studies (binomial GLM: df = 256, z = 3.87, P < 0.001).
3.3.1 Frequent species
In the four most frequently studied species (together 16% of all records of age-reproductive trait relationships), reproductive senescence appeared more often (30%) than in the remaining, less commonly studied species (17%; the overall average being 19%). Among these four most-represented species, there was no consistent pattern in reproductive senescence in the trait categories that yielded at least 5 age-reproduction records. The reproductive traits that showed sufficient representation were mainly related to female ovaries, egg number, and egg quality (together 47% of all age–reproduction records in the four species). Rainbow trout (O. mykiss) females showed reproductive senescence of 20% in gamete number and 14% in post-hatching records, but none in the gamete quality. Vendace (C. albula) females exhibited reproductive senescence of 40% in gonad-related traits, 38% in egg number, and 19% in egg quality records. In contrast, females of common carp (C. carpio) did not show any signs of reproductive senescence in gonad-related traits, egg number, or egg quality. Zebrafish (D. rerio) was represented mainly by male traits and showed a larger prevalence of reproductive senescence with 83% in pre-mating and mating, 57% in sperm number, 67% in sperm quality, and 29% in pre-hatching traits records. The pre-hatching traits (such as fertilization rate or embryo survival) were also recorded for both parents in zebrafish and they showed a higher incidence of reproductive senescence compared to males-only records (50% of the pre-hatching trait records).
4 Discussion
We assembled studies providing evidence for reproductive senescence in fishes across various phylogenetic lineages, contexts, and traits. The collected records span from demographic aspects of reproduction in wild populations (Carter et al., 2014; Benoît et al., 2018) to laboratory studies directly focused on reproductive senescence (Woodhead, 1974a; Žák and Reichard, 2021). Different fish species represent a particularly diverse evolutionary clade that includes some exceptionally long-lived species. They can thus provide insights into the evolution of aging (Kolora et al., 2021) and perhaps contribute to the understanding of how to curb its negative effects on our own species (Woodhead, 1998). Being a long-lived species does not necessarily imply escaping senescence, at least not at the physiological or reproductive level. Although there are indeed examples of long-lived fish species reproducing relatively more successfully when growing older (Berkeley et al., 2004), our review demonstrates this is not a universal rule across ray-finned fish.
4.1 Comparative context of reproductive senescence in ray-finned fish
We found that reproductive senescence in fish is taxonomically widespread. We considered either a bell-shaped or a negative relationship with age as a sign of reproductive senescence. More than 30% of the fish species exhibited some form of reproductive senescence, and the variation across the phylogeny was large. Senescence has been recorded in all age-related reproductive traits observed in labyrinth fishes [Anabantiformes, two studies by Woodhead, 1974a,b] and was also relatively common in tooth carps (Cyprinodontiformes). On the other hand, the frequency of reproductive senescence was very low in cods (Gadiformes) and catfishes (Siluriformes), despite them being well-represented in the collection of age-related reproductive traits. Within taxonomic orders, the variation was also high. In Cypriniformes, zebrafish display a high incidence of reproductive senescence, while reproductive senescence was reported at a very low frequency in the common carp. Across fish species, parental care, and migratory strategy did not increase the occurrence of reproductive senescence.
In mammals or birds, reproductive senescence is much more prevalent. Similar reviews focusing on female reproduction found that 68% of mammalian and 61% of avian species exhibited some form of age-related decline (Lemaître et al., 2020; Vágási et al., 2021). Although there are rare studies on reproductive senescence from reptile females (Hoekstra et al., 2020), a broader overview of the occurrence of reproductive senescence across vertebrates is not available. The lower prevalence of reproductive senescence in fish compared to mammals or birds is not surprising given the indeterminate growth and body size-dependent fecundity in fish. Mammalian females invest relatively more energy in reproduction for an extended period of time compared to a typical female fish. Mammalian offspring depend completely on milk provision from their mothers with lactation being the most energy-demanding phase of raising the offspring (Clutton-Brock et al., 1989; Speakman, 2008). The high allocation that mammals and birds put into parental care likely increases the potential for age-related reproductive decline, and behavioral and bodily deterioration also reduces their ability to gather sufficient nutrition toward older ages. The parental care hypothesis was not, however, supported by our analysis. We did not find a relationship between fish viviparity or parental guarding and the occurrence of reproductive senescence. Another important aspect of mammalian reproduction is that females possess a fixed pool of oocytes that deteriorate throughout their lives, unlike fish species which produce new eggs continuously (Lubzens et al., 2010). This may also increase the likelihood of a reproductive decline in mammals compared to fish. We would like to emphasize that reproductive senescence is much more widespread across fish than generally assumed, and we found cases of reproductive senescence in species that serve as textbook examples of non-senescent lineages (e.g., Sebastes alutus, de Bruin et al., 2004). In addition to that, the absence of reproductive senescence in certain lineages may stem from data deficiency rather than being a genuine aspect of their life history, and we elaborate on that in the below section.
4.2 Sex-related aspect of fish reproductive senescence
The distribution of records on age-related reproductive traits was markedly skewed toward females in our database. It is a common problem across studies on age-related reproduction in vertebrates that data from males are under-represented (Nussey et al., 2013; Lemaître and Gaillard, 2017; Archer et al., 2022). Reproductive senescence was, however, more frequently recorded for males or both parents combined rather than for females (30 and 33% compared to 15%). This is perhaps because most female-related studies focused on fecundity (Table 2), a trait tightly associated with body size in fish (Wootton and Smith, 2015). Female fish usually employ different life-history strategies compared to males. For example, females mature later and at a larger size, they grow slower but for a longer period of time, and they live longer than males (Woodhead, 1998; Wootton and Smith, 2015). The sexes also differ in their strategy of energy allocation into reproduction (Wootton and Smith, 2015). It is, therefore, reasonable to expect sex-specific differences in age-related reproductive decline as reported, for example, in red deer (Cervus elaphus), where males suffer from a steeper decline in annual fecundity than females (Nussey et al., 2009).
Female and male reproductive senescence also differed in the most frequently affected traits, a finding corroborating previous data across vertebrates (Tompkins and Anderson, 2019). In females, pre-mating and mating traits, such as inter-brood interval or regular reproduction, were most prone to age-related decline. This is likely a consequence of energetic or physiological limitations toward the end of life, where females reduce the number of reproductive events (instead of, for example, the number of eggs). Inter-brood interval extends in older mammalian females as well (see Karniski et al., 2018). While females may generally prefer to lay more of smaller eggs (Einum and Fleming, 2000), the selection should favor offspring quality over quantity with increasing female age (Moorad and Nussey, 2016). Both egg-related traits (quantity and quality) exhibited very low occurrence of reproductive senescence in our database. While they did not analyze any data for fish, Ivimey-Cook and Moorad (2020) found a negative effect of maternal age on offspring survival in wild mammals, but positive in wild birds. Our analyses do not provide any support for the role of parental care in reproductive senescence, as neither viviparity nor guarding of the offspring was associated with the increase in its incidence.
In male fish, the amount and quality of sperm declined with age more often than other reproductive traits. Sperm-related senescence also occurs in birds (sperm motility: Møller et al., 2009; DNA damage: Velando et al., 2011; sperm number: Cornwallis et al., 2014) and some mammals (Lemaître and Gaillard, 2017). These patterns can be surprising because the amount and quality of sperm directly affect male reproductive success (Stoltz and Neff, 2006). Although some general patterns for senescence in the types of reproductive traits emerged from our database, the high diversity of traits (more than 260 different reproductive traits collected) prevented us from performing a thorough quantitative analysis of the collected records on age-related reproduction.
4.3 Reproductive senescence in free-living and captive fish
Studies that were designed in captivity showed a markedly higher proportion of reproductive senescence records compared with those performed on fish populations in the wild. There is no overall concordance across animal studies comparing senescence between captivity and the wild. Some taxa, such as mammals or squamates, survive better in captivity (Scharf et al., 2015; Tidière et al., 2016) and also have lower rates of senescence (Lemaître et al., 2013), while others, such as turtles or antler flies, show mixed results (Kawasaki et al., 2008; da Silva et al., 2022). Captive mammal males often show signs of age-related decline in sperm traits (Lemaître and Gaillard, 2017), but comparative data from the wild are very rare (but see Curren et al., 2013). In fish, studies on age-related reproduction from the wild had unexpectedly better coverage of species-specific maximum age than studies from captivity. This may be because some of the captive studies were performed on commercial stocks, where keeping individuals for an extended period (until reproductive decline) is unlikely desirable (Green, 2008). A captive setting, on the other hand, offers better control over multiple factors compared to research conducted in the field. Captive stocks are also protected from natural stressors that may result in the selective disappearance of senescent individuals from the population. Assuming a strong link between physiological and reproductive senescence, as reported in birds and mammals (Ricklefs et al., 2003), senescent individuals are more likely to succumb to predation or disease in the wild (Martin and Festa-Bianchet, 2011; Bouwhuis et al., 2012; Hämäläinen et al., 2014). This makes them considerably under-represented in older age cohorts, and selective disappearance consequently masks the negative relationship between physiological or reproductive conditions and age at the population level (Maklakov et al., 2015). A higher survival rate and ability to track individual fish thus probably increased the probability of captive studies to record reproductive decline as the fish aged.
4.4 Under-represented old cohorts
It is obvious that some fish species maintain high reproductive performance until advanced age (at least compared to human lifespan), but may display signs of reproductive senescence. The fecundity of the oldest (48 years old) examined giant grenadiers (Albatrossia pectoralis) was superior to younger females (Rodgveller et al., 2010). Similarly to that, females of rougheye rockfish (S. aleutianus) possess fully functional ovaries with no signs of atresia until the age of at least 80 years. Their ovary mass, however, declines at an advanced age (de Bruin et al., 2004). Among shorter-lived species, captive male platyfish (Xiphophorus maculatus) kept their reproductive potential and the levels of reproductive hormones to the oldest ages recorded (5 years), but high fibrosis in their testes was apparent (Schreibman et al., 1983). This suggests that focusing on a single trait may ignore the age-related decline in others and underappreciate reproductive senescence. According to our database, there is no record of reproductive senescence for 70% of the studied fish species. The patchiness of evidence for reproductive senescence may have arisen from the lack of high-quality demographic data. In particular, poor coverage of maximum lifespan in age-reproduction investigations is obvious for fishes. Few studies from our database actually sampled fish populations toward the maximum known lifespan. The studies considerably under-represented older age cohorts because three-quarters of the records were from individuals that did not even reach 75% of the known species’ maximum lifespan. This especially applies to long-lived species, such as the giant grenadier or the rougheye rockfish mentioned above with a maximum lifespan of 56 and 205 years, respectively (data on maximum age comes from Froese and Pauly, 2022). Across the studies from our database, a higher lifespan index (ratio between the study’s maximum age and species’ known lifespan) was associated with a higher likelihood of recording reproductive senescence. Sampling toward the maximum lifespan is a natural requirement for the proper estimation of the impact of reproductive senescence. This is, however, complicated for the long-lived fish species by data availability in the first place and the ability to accurately determine their age in the wild (Craig, 1985). The insufficiency of high-quality demographic data thus prevents us from making a more robust general conclusion about reproductive senescence in ray-finned fish.
4.5 Role of indeterminate growth in fish reproductive senescence
Ray-finned fish species are characterized by continuous growth throughout their lives (Bidder, 1932; Charnov et al., 2001). This makes them candidates for negligible and negative senescence because large (and hence old) individuals are generally favored by natural selection (Bidder, 1932; Vaupel et al., 2004; Jones and Vaupel, 2017). This is because mortality (actuarial senescence) increases as a function of age but declines with body size (Caswell and Salguero-Gómez, 2013; Colchero and Schaible, 2014; Jones and Vaupel, 2017). In fact, body size explained variations in fish reproductive traits better than age in 75% of cases in our database. On the other hand, for records with an age-related reproductive decline in a trait, only one-third of the body size effects were negative or bell-shaped. This may be a consequence of variation in growth strategy across fish species. For example, turquoise killifish (N. furzeri) or Trinidadian guppy (P. reticulata) reaches body size limits long after maturity, and reproductive senescence occurs after cessation of their growth (Reznick et al., 2006; Žák and Reichard, 2021). Indeterminately growing fish, such as cods (Gadiformes) (Woodhead, 1998), demonstrate a very low incidence of reproductive senescence. This suggests that the negligible senescence can indeed be characteristic of some fish lineages. In reptiles, for example, it often arises that even after body size correction, the reproductive trait does not decline with age (Warner et al., 2016; Tully et al., 2020; but see Sparkman et al., 2007). Among indeterminately growing invertebrates, common woodlouse (Armadillidium vulgare) females of older ages also produce a higher number of larger offspring. Yet, the offspring of older female woodlouse have lower fitness, offsetting the increase in maternal fertility arising from the indeterminate growth (Depeux et al., 2020). The current datasets indicate that indeterminate growth itself is not sufficient to escape reproductive senescence. To provide any solid conclusion on the effect of indeterminate growth on reproductive senescence, we need that future studies on age-related reproduction properly account for the confounding effect of body size, that is, by using body size as a covariate.
4.6 Conclusion
Reproductive senescence is present and diverse across ray-finned fish species. The indeterminate growth may indeed, to some extent, buffer against the age-related reproductive decline, but growing larger is often not sufficient. The understanding of the evolution of fish reproductive senescence would definitely benefit from more empirical life-history studies with the effort put into covering the species’ lifespan and accounting for the size-related fecundity. It is important to state that our review inevitably suffers from attention bias. The studies focused on one or a few traits, and the set of focal traits varied notably among studies. The overall insight into what actually occurs during the aging of particular species is therefore still incomplete. Interspersed records of age-related reproductive decline across the fish phylogeny and the identified sampling bias indicate that reproductive senescence is prevalent in fishes. The present overview of the up-to-date published records thus offers a new, more elaborate perspective on fish life history, and we hope that our review will stimulate further research in the most neglected directions.
Author contributions
MV: conceptualization (equal), data curation (lead), formal analysis (lead), investigation (lead), methodology (lead), visualization (lead), writing – original draft (lead), and writing – review and editing (equal). JŽ: conceptualization (equal), data curation (supporting), formal analysis (supporting), investigation (equal), methodology (supporting), writing – original draft (supporting), and writing – review and editing (equal). MR: conceptualization (equal), funding acquisition (lead), investigation (supporting), methodology (supporting), resources (lead), supervision (lead), writing – original draft (supporting), and writing – review and editing (equal). All authors contributed to the article and approved the submitted version.
Funding
Funding came from a research project awarded by Czech Science Foundation (19-01781S). The funder had no role in experimental design or publication decisions.
Acknowledgments
We would like to thank the authors who made their articles available through open-access or public repositories such as ResearchGate. We appreciate the help from the authors who shared their work with us upon request, and from Atilla Arslan (Selcuk University), Wenjing Yi (Institute of Vertebrate Biology), and Ali Serhan Tarkan (Muğla Sıtkı Koçman University) who kindly shared PDFs of their local colleagues. We benefited from the services of Semantic Scholar (www.semanticscholar.org) and SciHub. The manuscript was improved, thanks to the comments from Krish Sanghvi (Oxford University), two referees, and the editor (J-FL).
Conflict of interest
The authors declare that the research was conducted in the absence of any commercial or financial relationships that could be construed as a potential conflict of interest.
Publisher’s note
All claims expressed in this article are solely those of the authors and do not necessarily represent those of their affiliated organizations, or those of the publisher, the editors and the reviewers. Any product that may be evaluated in this article, or claim that may be made by its manufacturer, is not guaranteed or endorsed by the publisher.
Supplementary material
The Supplementary Material for this article can be found online at: https://www.frontiersin.org/articles/10.3389/fevo.2022.982915/full#supplementary-material
Footnotes
References
Archer, C. R., Paniw, M., Vega-Trejo, R., and Sepil, I. (2022). A sex skew in life-history research: The problem of missing males. Proc. R. Soc. B Biol. Sci. 289:20221117. doi: 10.1098/rspb.2022.1117
Austad, S. N., and Finch, C. E. (2022). How ubiquitous is aging in vertebrates? Science 376, 1384–1385. doi: 10.1126/science.adc9442
Barneche, D. R., Robertson, D. R., White, C. R., and Marshall, D. J. (2018). Fish reproductive-energy output increases disproportionately with body size. Science 360, 642–645. doi: 10.1126/science.aao6868
Bates, D., Maechler, M., Bolker, B., and Walker, S. (2015). Fitting linear mixed-effects models using lme4. J. Stat. Softw. 67, 1–48. doi: 10.18637/jss.v067.i01
Baudisch, A., and Stott, I. (2019). A pace and shape perspective on fertility. Methods Ecol. Evol. 10, 1941–1951. doi: 10.1111/2041-210X.13289
Benoît, H. P., Swain, D. P., Hutchings, J. A., Knox, D., Doniol-Valcroze, T., and Bourne, C. M. (2018). Evidence for reproductive senescence in a broadly distributed harvested marine fish. Mar. Ecol. Prog. Ser. 592, 207–224. doi: 10.3354/meps12532
Berkeley, S. A., Chapman, C., and Sogard, S. M. (2004). Maternal age as a determinant of larval growth and survival in a marine fish, Sebastes melanops. Ecology 85, 1258–1264. doi: 10.1890/03-0706
Bouwhuis, S., Choquet, R., Sheldon, B. C., and Verhulst, S. (2012). The forms and fitness cost of senescence: Age-specific recapture, survival, reproduction, and reproductive value in a wild bird population. Am. Nat. 179, E15–E27. doi: 10.1086/663194
Carter, A. B., Russ, G. R., Tobin, A. J., Williams, A. J., Davies, C. R., and Mapstone, B. D. (2014). Spatial variation in the effects of size and age on reproductive dynamics of common coral trout Plectropomus leopardus. J. Fish Biol. 84, 1074–1098. doi: 10.1111/jfb.12346
Caswell, H., and Salguero-Gómez, R. (2013). Age, stage and senescence in plants. J. Ecol. 101, 585–595. doi: 10.1111/1365-2745.12088
Charnov, E. L., Turner, T. F., and Winemiller, K. O. (2001). Reproductive constraints and the evolution of life histories with indeterminate growth. Proc. Natl. Acad. Sci. U.S.A. 98, 9460–9464. doi: 10.1073/pnas.161294498
Clutton-Brock, T. H., Albon, S. D., and Guinness, F. E. (1989). Fitness costs of gestation and lactation in wild mammals. Nature 337:260. doi: 10.1038/337260a0
Cohen, A. A. (2004). Female post-reproductive lifespan: A general mammalian trait. Biol. Rev. Camb. Philos. Soc. 79, 733–750. doi: 10.1017/S1464793103006432
Colchero, F., and Schaible, R. (2014). Mortality as a bivariate function of age and size in indeterminate growers. Ecosphere 5:161. doi: 10.1890/ES14-00306.1
Comfort, A. (1954). Biological aspects of senescence. Biol. Rev. 29, 284–329. doi: 10.1111/j.1469-185X.1954.tb00598.x
Comfort, A. (1961). Age and reproduction in female Lebistes. Gerontologia 5, 146–149. doi: 10.1159/000211050
Cornwallis, C. K., Dean, R., and Pizzari, T. (2014). Sex-specific patterns of aging in sexual ornaments and gametes. Am. Nat. 184, E66–E78. doi: 10.1086/677385
Craig, J. F. (1977). The body composition of adult perch, Perca fluviatilis in Windermere, with reference to seasonal changes and reproduction. J. Anim. Ecol. 46, 617–632.
Crespi, B. J., and Teo, R. (2002). Comparative phylogenetic analysis of the evolution of semelparity and life history in salmonid fishes. Evolution 56, 1008–1020. doi: 10.1554/0014-38202002056
Curren, L. J., Wedele, M. L., and Holekamp, K. E. (2013). Ejaculate quality in spotted hyenas: Intraspecific variation in relation to life-history traits. J. Mammal. 94, 90–99.
da Silva, R., Conde, D. A., Baudisch, A., and Colchero, F. (2022). Slow and negligible senescence among testudines challenges evolutionary theories of senescence. Science 376, 1466–1470. doi: 10.1126/science.abl7811
de Bruin, J. P., Gosden, R. G., Finch, C. E., and Leaman, B. M. (2004). Ovarian aging in two species of long-lived rockfish, Sebastes aleutianus and S. alutus. Biol. Reprod. 71, 1036–1042. doi: 10.1095/biolreprod.103.019919
Depeux, C., Lemaître, J.-F., Moreau, J., Dechaume-Moncharmont, F. X., Laverre, T., Pauhlac, H., et al. (2020). Reproductive senescence and parental effects in an indeterminate grower. J. Evol. Biol. 33, 1256–1264. doi: 10.1111/jeb.13667
Einum, S., and Fleming, I. A. (2000). Highly fecund mothers sacrifice offspring survival to maximize fitness. Nature 57, 565–567. doi: 10.1038/35014600
Finch, C. E., and Holmes, D. J. (2010). Ovarian aging in developmental and evolutionary contexts. Ann. N. Y. Acad. Sci. 1204, 82–94. doi: 10.1111/j.1749-6632.2010.05610.x
Froese, R., and Pauly, D. (2022). FishBase. World Wide Web electronic publication, version (02/2022). Available online at: www.fishbase.org (accessed November 14, 2022).
Gasparini, C., Devigili, A., and Pilastro, A. (2019). Sexual selection and ageing: Interplay between pre- and post-copulatory traits senescence in the guppy. Proc. R. Soc. B Biol. Sci. 286:20182873. doi: 10.1098/rspb.2018.2873
Green, B. S. (2008). Maternal effects in fish populations. Adv. Mar. Biol. 54, 1–105. doi: 10.1016/S0065-2881(08)00001-1
Hämäläinen, A., Dammhahn, M., Aujard, F., Eberle, M., Hardy, I., Kappeler, P. M., et al. (2014). Senescence or selective disappearance? Age trajectories of body mass in wild and captive populations of a small-bodied primate. Proc. R. Soc. B 281:20140830. doi: 10.1098/rspb.2014.0830
Helfman, G., Collette, B. B., Facey, D. E., and Bowen, B. W. (2009). The Diversity of Fishes: Biology, evolution, and ecology. Oxford: John Wiley & Sons, 736.
Hoekstra, L. A., Schwartz, T. S., Sparkman, A. M., Miller, D. A. W., and Bronikowski, A. M. (2020). The untapped potential of reptile biodiversity for understanding how and why animals age. Funct. Ecol. 34, 38–54. doi: 10.1111/1365-2435.13450
IUCN (2021). The IUCN red list of threatened species. Version 2021-3. Available online at: https://www.iucnredlist.org (accessed June 30, 2022).
Ivimey-Cook, E., and Moorad, J. A. (2020). The diversity of maternal-age effects upon pre-adult survival across animal species: Diversity of maternal-age effects. Proc. R. Soc. B Biol. Sci. 287:20200972. doi: 10.1098/rspb.2020.0972
Jones, O. R., and Vaupel, J. W. (2017). Senescence is not inevitable. Biogerontology 18, 965–971. doi: 10.1007/s10522-017-9727-3
Jones, O. R., Scheuerlein, A., Salguero-Gómez, R., Camarda, C. G., Schaible, R., Casper, B. B., et al. (2014). Diversity of ageing across the tree of life. Nature 505, 5–10. doi: 10.1038/nature12789
Karjalainen, J., Urpanen, O., Keskinen, T., Huuskonen, H., Sarvala, J., Valkeajärvi, P., et al. (2016). Phenotypic plasticity in growth and fecundity induced by strong population fluctuations affects reproductive traits of female fish. Ecol. Evol. 6, 779–790. doi: 10.1002/ece3.1936
Karniski, C., Krzyszczyk, E., and Mann, J. (2018). Senescence impacts reproduction and maternal investment in bottlenose dolphins. Proc. R. Soc. B Biol. Sci. 285:20181123. doi: 10.1098/rspb.2018.1123
Kawasaki, N., Brassil, C. E., Brooks, R. C., and Bonduriansky, R. (2008). Environmental effects on the expression of life span and aging: An extreme contrast between wild and captive cohorts of Telostylinus angusticollis (Diptera: Neriidae). Am. Nat. 172, 346–357. doi: 10.1086/589519
Kelley, J. W. (1962). Sexual maturity and fecundity of the largemouth bass, Micropterus salmoides (Lacépède), in Maine. Trans. Am. Fish. Soc. 91, 23–28. doi: 10.1577/1548-8659196291[23:smafot]2.0.co;2
Kolora, S. R. R., Owens, G. L., Vazquez, J. M., Stubbs, A., Chatla, K., Jainese, C., et al. (2021). Origins and evolution of extreme lifespan in Pacific Ocean rockfishes. Science 847, 1–18. doi: 10.1126/science.abg5332
Krumholz, L. A. (1948). Reproduction in the western mosquitofish, Gambusia affinis affinis (Baird & Girard), and its use in mosquito control. Ecol. Monogr. 18, 1–43.
Lemaître, J. F., Ronget, V., and Gaillard, J. M. (2020). Female reproductive senescence across mammals: A high diversity of patterns modulated by life history and mating traits. Mech. Ageing Dev. 192:111377. doi: 10.1016/j.mad.2020.111377
Lemaître, J.-F., and Gaillard, J.-M. (2017). Reproductive senescence: New perspectives in the wild. Biol. Rev. 92, 2182–2199. doi: 10.1111/brv.12328
Lemaître, J.-F., Gaillard, J.-M., Lackey, L. B., Clauss, M., and Müller, D. W. H. (2013). Comparing free-ranging and captive populations reveals intra-specific variation in aging rates in large herbivores. Exp. Gerontol. 48, 162–167. doi: 10.1016/j.exger.2012.12.004
Lubzens, E., Young, G., Bobe, J., and Cerdà, J. (2010). Oogenesis in teleosts: How eggs are formed. Gen. Comp. Endocrinol. 165, 367–389. doi: 10.1016/j.ygcen.2009.05.022
Maklakov, A. A., Rowe, L., and Friberg, U. (2015). Why organisms age: Evolution of senescence under positive pleiotropy? BioEssays 37, 802–807. doi: 10.1002/bies.201500025
Martin, J. G. A., and Festa-Bianchet, M. (2011). Age-independent and age-dependent decreases in reproduction of females. Ecol. Lett. 14, 576–581. doi: 10.1111/j.1461-0248.2011.01621.x
Moher, D., Liberati, A., Tetzlaff, J., Altman, D. G., and The PRISMA Group (2009). Preferred reporting items for systematic reviews and meta-analyses: The PRISMA statement. PLoS Med. 6:e1000097. doi: 10.1371/journal.pmed1000097
Møller, A. P., Mousseau, T. A., Rudolfsen, G., Balbontín, J., Marzal, A., Hermosell, I., et al. (2009). Senescence sperm performance in old male birds. J. Evol. Biol. 22, 334–344.
Monaghan, P., and Metcalfe, N. B. (2019). The deteriorating soma and the indispensable germline: Gamete senescence and offspring fitness. Proc. R. Soc. B Biol. Sci. 286:20192187. doi: 10.1098/rspb.2019.2187
Moorad, J. A., and Nussey, D. H. (2016). Evolution of maternal effect senescence. Proc. Natl. Acad. Sci. U.S.A. 113, 362–367. doi: 10.1073/pnas.1520494113
Morbey, Y. E., Brassil, C. E., and Hendry, A. P. (2005). Rapid senescence in Pacific salmon. Am. Nat. 166, 556–568. doi: 10.1086/491720
Nussey, D. H., Froy, H., Lemaître, J.-F., Gaillard, J.-M., and Austad, S. N. (2013). Senescence in natural populations of animals: Widespread evidence and its implications for bio-gerontology. Ageing Res. Rev. 12, 214–225. doi: 10.1016/j.arr.2012.07.004
Nussey, D. H., Kruuk, L. E., Morris, A., Clements, M. N., Pemberton, J. M., and Clutton-Brock, T. H. (2009). Inter- and intrasexual variation in aging patterns across reproductive traits in a wild red deer population. Am. Nat. 174, 342–357. doi: 10.1086/603615
Pen, L. J., Gill, H. S., Humphries, P., and Potter, I. C. (1993). Biology of the black-stripe minnow Galaxiella nigvostriata, including comparisons with the other two Galaxiella species. J. Fish Biol. 43, 847–863. doi: 10.1111/j.1095-8649.1993.tb01160.x
R Development Core Team (2021). R: A language and environment for statistical computing. Vienna: R Foundation for Statistical Computing.
Rabosky, D. L., Chang, J., Title, P. O., Cowman, P. F., Sallan, L., Friedman, M., et al. (2018). An inverse latitudinal gradient in speciation rate for marine fishes. Nature 559, 392–395. doi: 10.1038/s41586-018-0273-1
Rasquin, P., and Hafter, E. (1951). Age changes in the testis of the teleost, Astyanax mexicanus. J. Morphol. 89, 397–407. doi: 10.1002/jmor.1050890302
Reinke, B. A., Cayuela, H., Janzen, F. J., Lemaître, J.-F., Gaillard, J.-M., Lawing, A. M., et al. (2022). Diverse aging rates in ectothermic tetrapods provide insights for the evolution of aging and longevity. Science 376, 1459–1466. doi: 10.1126/science.abm0151
Reznick, D. N., Bryant, M., and Holmes, D. (2006). The evolution of senescence and post-reproductive lifespan in guppies (Poecilia reticulata). PLoS Biol. 4:e7. doi: 10.1371/journal.pbio.0040007
Reznick, D. N., Ghalambor, C. K., and Nunney, L. (2002). The evolution of senescence in fish. Mech. Ageing Dev. 123, 773–789.
Ricklefs, R. E. (2010). Insights from comparative analyses of aging in birds and mammals. Aging Cell 9, 273–284. doi: 10.1111/j.1474-9726.2009.00542.x
Ricklefs, R. E., Scheuerlein, A., and Cohen, A. (2003). Age-related patterns of fertility in captive populations of birds and mammals. Exp. Gerontol. 38, 741–745. doi: 10.1016/S0531-5565(03)00101-3
Rodgveller, C. J., Clausen, D. M., Nagler, J. J., and Hutchinson, C. (2010). Reproductive characteristics and mortality of female giant grenadiers in the Northern Pacific ocean. Mar. Coast. Fish. 2, 73–82. doi: 10.1577/c09-028.1
Roper, M., Capdevila, P., and Salguero-Gómez, R. (2021). Senescence: Why and where selection gradients might not decline with age. Proc. R. Soc. B Biol. Sci. 288:20210851. doi: 10.1098/rspb.2021.0851
Scharf, I., Feldman, A., Novosolov, M., Pincheira-Donoso, D., Das, I., Böhm, M., et al. (2015). Late bloomers and baby boomers: Ecological drivers of longevity in squamates and the tuatara. Glob. Ecol. Biogeogr. 24, 396–405. doi: 10.1111/geb.12244
Schreibman, M. P., Margolis-Kazan, H., Bloom, J. L., and Kallman, K. D. (1983). Continued reproductive potential in aging platyfish as demonstrated by the persistence of gonadotropin, luteinizing hormone releasing hormone and spermatogenesis. Mech. Ageing Dev. 22, 105–112. doi: 10.1016/0047-6374(83)90103-3
Shefferson, R. P., Jones, O. R., and Salguero-Gómez, R. (2017). The evolution of senescence in the tree of life, eds R. P. Shefferson, O. R. Jones, and R. Salguero-Gomez (Cambridge: Cambridge University Press). doi: 10.1017/9781139939867
Soriano-Redondo, A., Gutiérrez, J. S., Hodgson, D., and Bearhop, S. (2020). Migrant birds and mammals live faster than residents. Nat. Commun. 11, 1–8. doi: 10.1038/s41467-020-19256-0
Sparkman, A. M., Arnold, S. J., and Bronikowski, A. M. (2007). An empirical test of evolutionary theories for reproductive senescence and reproductive effort in the garter snake Thamnophis elegans. Proc. R. Soc. B Biol. Sci. 274, 943–950. doi: 10.1098/rspb.2006.0072
Speakman, J. R. (2008). The physiological costs of reproduction in small mammals. Philos. Trans. R. Soc. B Biol. Sci. 363, 375–398. doi: 10.1098/rstb.2007.2145
Stoltz, J. A., and Neff, B. D. (2006). Sperm competition in a fish with external fertilization: The contribution of sperm number, speed and length. J. Evol. Biol. 19, 1873–1881. doi: 10.1111/j.1420-9101.2006.01165.x
Tidière, M., Gaillard, J. M., Berger, V., Müller, D. W. H., Lackey, L. B., Gimenez, O., et al. (2016). Comparative analyses of longevity and senescence reveal variable survival benefits of living in zoos across mammals. Sci. Rep. 6:36361. doi: 10.1038/srep36361
Tompkins, E. M., and Anderson, D. J. (2019). Sex-specific patterns of senescence in Nazca boobies linked to mating system. J. Anim. Ecol. 88, 986–1000. doi: 10.1111/1365-2656.12944
Tully, T., Le Galliard, J. F., and Baron, J. P. (2020). Micro-geographic shift between negligible and actuarial senescence in a wild snake. J. Anim. Ecol. 89, 2704–2716. doi: 10.1111/1365-2656.13317
Vágási, C. I., Vincze, O., Lemaître, J.-F., Pap, P. L., Ronget, V., and Gaillard, J.-M. (2021). Is degree of sociality associated with reproductive senescence? A comparative analysis across birds and mammals. Philos. Trans. R. Soc. B Biol. Sci. 376, 20190744. doi: 10.1098/rstb.2019.0744
Vaupel, J. W., Baudisch, A., Dölling, M., Roach, D. A., and Gampe, J. (2004). The case for negative senescence. Theor. Popul. Biol. 65, 339–351. doi: 10.1016/j.tpb.2003.12.003
Velando, A., Noguera, J. C., Drummond, H., and Torres, R. (2011). Senescent males carry premutagenic lesions in sperm. J. Evol. Biol. 24, 693–697. doi: 10.1111/j.1420-9101.2010.02201.x
Venturelli, P. A., Shuter, B. J., and Murphy, C. A. (2012). Evidence for harvest-induced maternal influences on the reproductive rates of fish populations. Proc. R. Soc. B Biol. Sci. 276, 919–924. doi: 10.1098/rspb.2008.1507
Vrtílek, M., Žák, J., Pšenička, M., and Reichard, M. (2018). Extremely rapid maturation of a wild African annual fish. Curr. Biol. 28, R822–R824. doi: 10.1016/j.cub.2018.06.031
Warner, D. A., Miller, D. A. W., Bronikowski, A. M., and Janzen, F. J. (2016). Decades of field data reveal that turtles senesce in the wild. Proc. Natl. Acad. Sci. U.S.A. 113, 6502–6507. doi: 10.1073/pnas.1600035113
Woodhead, A. D. (1974a). Ageing changes in the siamese fighting fish, Betta splendens-I. The testis. Exp. Gerontol. 9, 75–81. doi: 10.1016/0531-5565(74)90042-2
Woodhead, A. D. (1974b). Ageing changes in the siamese fighting fish, Betta splendens-II. The ovary. Exp. Gerontol. 9, 131–139. doi: 10.1016/0531-5565(74)90042-4
Woodhead, A. D. (1998). Aging, the fishy side: An appreciation of Alex Comfort’s studies. Exp. Gerontol. 33, 39–51. doi: 10.1016/S0531-5565(97)00064-8
Wootton, R. J., and Smith, C. (2015). Reproductive biology of teleost fishes. Oxford: John Wiley & Sons. doi: 10.1017/CBO9781107415324.004
Keywords: reproductive aging, fish, Actinopterygii, indeterminate growth, lifespan, fecundity, life-history evolution, negligible senescence
Citation: Vrtílek M, Žák J and Reichard M (2022) Evidence for reproductive senescence across ray-finned fishes: A review. Front. Ecol. Evol. 10:982915. doi: 10.3389/fevo.2022.982915
Received: 30 June 2022; Accepted: 23 November 2022;
Published: 15 December 2022.
Edited by:
Jean-Francois Lemaitre, UMR 5558 Biométrie et Biologie Evolutive (LBBE), FranceReviewed by:
Csongor Vágási, Babeş-Bolyai University, RomaniaVictor Ronget, Université de Lyon, France
Copyright © 2022 Vrtílek, Žák and Reichard. This is an open-access article distributed under the terms of the Creative Commons Attribution License (CC BY). The use, distribution or reproduction in other forums is permitted, provided the original author(s) and the copyright owner(s) are credited and that the original publication in this journal is cited, in accordance with accepted academic practice. No use, distribution or reproduction is permitted which does not comply with these terms.
*Correspondence: Milan Vrtílek, dnJ0aWxla0BpdmIuY3o=; Martin Reichard, cmVpY2hhcmRAaXZiLmN6