- 1Reneco International Wildlife Consultants LLC, Abu Dhabi, United Arab Emirates
- 2Biogéosciences, UMR 6282 CNRS, Université de Bourgogne Franche-Comté, Dijon, France
- 3Centre d’Ecologie et des Sciences de la Conservation, UMR 7204 MNHN CNRS-UPMC, Museum National d’Histoire Naturelle, Paris, France
Age-dependent reduction in reproductive success can arise due to multiple factors including a deterioration of reproductive physiology. Senescing males have been shown to produce ejaculates with poor sperm quality, which impinges on male reproductive success. In addition to individual age, gamete age can also affect male reproductive success. Accordingly, variance in male reproductive success can be due to pre-meiotic (referring to individual age) and post-meiotic senescence (sperm age). Here, we tested whether male senescence and sperm cell aging have additive or interactive effects on male reproductive success in a bird with a promiscuous mating system, the North African houbara bustard. To assess the effect of pre-meiotic aging, we compared male reproductive success between two age classes (3-6- and 12–16-year-old). To infer the effect of post-meiotic aging, male ejaculates were collected at three-time intervals following a common initial collection (day 1, 5, and 10). Therefore, day 1 ejaculates are supposed to contain younger sperm than day 5 and 10 ejaculates. Following controlled artificial inseminations, reproductive success was assessed using three fitness-linked traits (hatching success, chick growth rate and survival). In addition to reproductive output, we also assessed whether pre- and post-meiotic aging affected a wide range of sperm and ejaculate traits. In agreement with previous reports, we found that males in the older age class produced less sperm with poorer motility compared to young individuals. However, contrary to the prediction, we found that ejaculates collected at day 5 and 10 tended to have better sperm traits such as motility and velocity. The results on sperm traits were generally mirrored in the effect on reproductive success since young males produced offspring that grew faster and had better survival during the first month of life, and eggs fertilized by sperm collected at day 5 had the highest hatching success. In any of the models, there was evidence for interactive effects of male and sperm age. Overall, these results confirm the role of pre-meiotic aging on male reproductive success. The lack of evidence for sperm aging could come from the experimental design but might also reflect the pattern of mating frequency in a species with a lek-based mating system.
Introduction
Senescence is the progressive decline of physiological functions with age, resulting in increased mortality risk (actuarial senescence) and decreased reproductive output (reproductive senescence) (Monaghan et al., 2008). While evidence in supporting senescence to be a widespread phenomenon in nature has been accumulating during the last decade (Nussey et al., 2013), the study of male reproductive senescence has been restricted to a narrower range of species (Lemaître and Gaillard, 2017). Despite this limited taxonomic spectrum, current evidence in humans and captive species of mammals, fish, birds and insects suggests that male age can have profound effects on several traits defining ejaculate and sperm quality (Gasparini et al., 2010; Johnson et al., 2015; Carreira et al., 2017; Herrera-Cruz et al., 2018; Colasante et al., 2019; Fricke and Koppik, 2019; Farooq et al., 2020). For instance, old males have been reported to produce lower ejaculate volume with impaired antioxidant defenses and containing sperm with reduced motility and velocity (Wolf et al., 2000; Sartorius and Nieschlag, 2010; Noguera et al., 2012; Gunes et al., 2016). In addition to these phenotypic changes, old males are also likely to accumulate mutations in the germline due to the continuous cell divisions that are inherent to spermatogenesis (Kong et al., 2012; Thomas et al., 2018). Finally, epigenetic changes of the germline were shown to occur during aging (Immler, 2018; Evans et al., 2019). The combined effect of phenotypic and (epi)genetic changes of sperm due to male age can therefore compromise reproductive success, both by reducing the probability to fertilize the ovum and increasing the risk of embryo/offspring mortality (Hale et al., 2008; Kim et al., 2011; Fay et al., 2016; Lippens et al., 2017; Ruhmann et al., 2018).
Besides these pre-meiotic (organismal) effects of aging, cellular senescence (post-meiotic senescence) can also contribute to determine male reproductive success. Sperm cells are particularly vulnerable to the environmental conditions they are exposed to, and susceptible to oxidative damage (Reinhardt, 2007). Sperm cells are prone to oxidative damage because they (i) produce high amount of reactive oxygen species (ROS) due to their high number of mitochondria and intense metabolic activity, (ii) have limited anti-oxidant defenses due to their reduced cytosol, (iii) do not have known mechanisms of DNA repair, and (iv) have membranes that are rich in unsaturated fatty acids which increase ROS production via oxidative carbonyls (Aitken, 2020). After their production during spermatogenesis, spermatozoa are stored in male ducts (epididymis and vas deferens) and after mating they can also be stored in the female reproductive tract in birds (Blesbois and Brillard, 2007). During this period, both in male and female sperm storage, oxidative damage was shown to compromise sperm motility, viability, and DNA integrity in studied species (reviewed by Tarin, 2000); thus, sperm aging can alter the capacity to fertilize the ovum or impair offspring health as shown by White et al. (2008) in kittiwakes (Rissa trydactila). For instance, prolonged sperm storage in male reproductive tract was also associated with decreased velocity and impaired fertilization in the guppy (Poecilia reticulata) (Gasparini et al., 2014, 2017), reduced viability and increased DNA fragmentation in zebrafish (Danio rerio) (Cattelan and Gasparini, 2021) and decreased fertilization, embryo development, hatching and chick condition in kittiwakes (Rissa trydactila) (White et al., 2008). Similarly, sperm aging within the female reproductive tract has been shown to have negative effects on fertility, embryonic development, and hatching rate success in poultry (Nalbandov and Card, 1943; Lodge et al., 1971).
While available evidence convincingly supports the role of both pre- and post-meiotic senescence as important drivers of male reproductive success, a question that has received little attention is whether they have additive or interactive effects on reproductive output (Pizzari et al., 2008). There are several reasons to expect that pre-meiotic senescence might amplify the effect of sperm age. For instance, as the antioxidant defense system decreases with age, sperm produced by old males might be more vulnerable to oxidative damage during the storage period. In agreement with this hypothesis, sperm of old rats are more vulnerable to both in vivo and in vitro oxidative challenge than sperm from young rats (Zubkova and Robaire, 2006). Such interactive effects of pre- and post-meiotic senescence might have important consequences on male reproductive success, and particularly so in species experiencing strong post-copulatory sexual selection (Birkhead and Pizzari, 2002). Indeed, when females mate with multiple males during the same reproductive bout, sperm compete for ovum fertilization. In this case, when old individuals compete with young males, they might fail to fertilize the ovum because of a faster rate of sperm senescence. The aim of the present study is to investigate whether pre- and post-meiotic senescence have additive or interactive effects on male reproductive success of the North African houbara bustard (Chlamydotis undulata undulata). An interactive effect between pre- and post-meiotic senescence would indicate that any effect of sperm age on sperm quality and/or reproductive success depends on male age. Previous work conducted on this species has shown that male age negatively affects ejaculate quality, hatching success and offspring growth, survival and sperm production (Preston et al., 2011, 2015; Vuarin et al., 2021). Old males also suffer from reduced fertilization success during competitive interactions with young males, even when males of both age classes provide equal number of sperm of similar motility to the insemination (Vuarin et al., 2019a). In addition to this, female houbara bustards can store sperm for weeks, as shown by females being able to lay fertile eggs weeks after being inseminated, and approximately 70% of chicks are sired by the last male used for insemination (L. Lesobre, personal observation). Thus, the houbara bustard appears to be a good candidate to explore the contribution of pre- and post-meiotic senescence on male reproductive success. To this purpose, we artificially inseminated female houbara bustards with sperm collected from old and young males. For each age class, we also collected sperm at different intervals with respect to a common initial sperm collection. A longer interval between the initial collection and the collection used to inseminate the female therefore indicates prolonged sperm storage and therefore older sperm. This experimental design allowed us to assess the effect of male and sperm age on several sperm traits (viability, motility, velocity, morphology, and ROS production) and reproductive success (hatching success, chick growth rate and survival). If pre- and post-meiotic senescence have synergistic effects, we predicted statistically significant interactions between male and sperm age on sperm quality and reproductive output.
Material and methods
General procedure and experimental groups
All birds used in this study are part of the Emirates Center for Wildlife Propagation (ECWP), a conservation breeding program located in eastern Morocco, aiming at reinforcing natural populations of the North African houbara bustard (Lacroix, 2003). This program entirely relies on artificial inseminations (Saint Jalme et al., 1994). Males and females on this experiment were born in captivity, are part of ECWP breeders flock and have been used routinely for semen collection and artificial inseminations in the previous seasons. Briefly, semen was routinely collected using a dummy female presented to males. Once the male mounted the dummy and started copulating, a glass dish was used to collect the ejaculate by pressing it gently on the male cloaca during ejaculation. The semen was immediately transferred to a 2 mL cryotube, maintained at room temperature. Ejaculates were directly assessed for motility (using a mass motility index ranging between 0 and 5, see Vuarin et al., 2019a for details), volume and sperm concentration, and diluted (1:1) in Lake 7.1 (Lake and Ravie, 1984).
For the present experiment, a 20 μl aliquot was diluted in 180 μL DPBS-BSA (1.5%) and set aside for flow cytometry analyses (see below), while the remaining was used to inseminate females. We used semen from 307 males belonging to two age classes: 154 young males (mean ± SD = 4.14 ± 1.09, min-max = 3–6 years), and 153 old males (mean ± SD = 13.58 ± 1.61, min-max = 12–16 years). Age categories were chosen based on previous work and senescing patterns (Preston et al., 2011; Vuarin et al., 2019a). These birds were subsequently allocated to three groups (evenly with respect to male age), corresponding to three intervals of sperm collections. Specifically, an initial semen collection was performed (day 0) and then for each group the collections were repeated after 1, 5, or 10 days. All birds experienced the 3 collection intervals, with a rest period of 12 days between cycles (Supplementary Data and Supplementary Figure 1).
Semen analysis
Flow cytometry analysis was performed using a Guava easycyte 5HT (IMV, L‘Aigle, France) flow cytometer equipped with a 150-mW blue argon laser (488 nm) and 3 color photomultipliers green (525/30 nm), yellow (583/26 nm) and red (695/50 nm). For each sample 10,000 events were counted in the sperm population gate. Data were analyzed using the IMV Easycyte plus software (IMV, L‘Aigle, France).
Viability
Sperm viability (% live out of 10,000 cells) was assessed using Easy Kit viability (IMV, L‘Aigle, France), based on propidium iodine (PI) and SYBR-14 dyes. PI enters broken plasma membrane and binds to nucleic acids provoking the emission of 617 nm red fluorescence (Gillan et al., 2005). SYBR-14 dye is membrane permeable and stains only living sperm emitting 520 nm green fluorescence when binding to nucleic acids (Garner et al., 1994). Kit’s 96 wells plate were loaded with 180 μL of PBS/1.5% BSA and 20 μL of diluted semen (1:10 in PBS/1.5% BSA). Negative control was performed diluting the sample in distilled water instead of PBS to promote membrane damage. Samples were analyzed after 10 min of incubation in the dark at room temperature.
Oxidative stress
Intracellular sperm oxidative stress was measured in live cells using a combination of Dihydroethidium (DHE, 2 mM in dimethyl sulfoxide (DMSO), Sigma-Aldrich, St Louis, USA), which emits 606 nm orange fluorescence when reacting with superoxide anions O2–, a ROS (Zhao et al., 2005), and SYBR-14 (Sigma-Aldrich) to measure percentage of living and oxidized sperm cells (out of 10,000 cells). In a 96 well plate 180 μL of PBS/1.5% BSA, 20 μL of diluted semen (1:10 in PBS/BSA), 1 μL of SYBR-14 (Sigma-Aldrich, St Louis, USA, 1 mM stock solution, 20 μM in DMSO work solution) and 2 μL of DHE (2 mM in DMSO) were added. Negative control was performed incubating sperm with hydrogen peroxide for 15 min at 40°C prior to analysis. Samples were analyzed after 15 min of incubation in the dark, at room temperature.
Motility
In addition to the initial mass motility index, sperm motility was further evaluated with Sperm Class Analyzer software (SCA, Microptics, Barcelona, Spain). Four microliters of diluted semen (1:10 in PBS/BSA 1.5%) were placed on a Makler chamber under a negative phase contrast microscope (Olympus BX41, 10 × objective) linked to the computer by a camera. A maximum of five fields were analyzed to reach 350 sperm cells, 25 frames on each field were evaluated. Percentage of motile sperm and velocity [curvilinear velocity VCL (μm/s)] were recorded. VCL was selected among other parameters as it refers to the velocity of the sperm real trajectory.
Morphology
To evaluate sperm morphology, semen was fixed by adding 10 μL of 4% buffered paraformaldehyde to 20μL of semen diluted in PBS/BSA 1.5% (1:10). Then, 6 μl of this solution were placed on a slide with a coverslip (wet mount) and observed under a phase contrast microscope (Olympus BX41, objective ×100, oil immersion). For each sample 100 sperm cells were classified according to 8 categories (Bloom, 1973; Alkan et al., 2002): acrosome loss, abnormal head, swollen head, midpiece defects, tail defects, cytoplasmic droplet, teratogenic sperm (double head or tail) and normal.
Artificial insemination, egg laying, and chick rearing
A total of 310 captive bred females were inseminated only with one of either 95 young males (N = 177 females, mean female age ± SD = 10 ± 2.4, min-max = 4–13 years) or with 74 old males (N = 133 females, mean female age ± SD = 10.48 ± 2.45, min-max = 3–13 years), from the three semen collection intervals. Females were inseminated with doses containing on average 26.10 million sperms (±16.22), an average inseminated volume of 86.18 μL (± 30.29), and a mass motility index > 3.5. The experimental inseminations were performed between the first and the second clutch with no previous insemination during the season, to guarantee the storage tubules were empty and no further insemination were performed before the experiment related eggs were collected.
For each inseminated female, we aimed at collecting the first four eggs laid corresponding approximatively to 2 clutches. The eggs were collected after a single homospermatic insemination. However, not all females laid 4 eggs. Overall, 599 eggs were laid, and more specifically 44 females laid one egg, 246 laid 2 eggs, 17, 3 eggs and 3 laid 4 eggs. Upon laying, eggs were collected and artificially incubated. 54 eggs showed defects and hence were not incubated, resulting in a total of 545 incubated eggs. Eggs were candled at day 8 to check embryo development. When no signs of fertility were detected, they were opened to discriminate early embryonic death from non-fertilized eggs. The percent of successfully hatched eggs (hatching success) was recorded. Body mass of hatchlings was measured daily (to the nearest of 1 g) from day 2 to 60 post-hatching. Any mortality occurring during this period was also recorded. Chicks were reared and manually fed in cages until 35 days and after transferred to outdoor aviaries.
Statistical analyses
Statistical analyses were performed using R 3.6.0. To investigate the effect of male and sperm age (approximated by the day of ejaculate collection) on sperm quality, we ran linear mixed-effect models [nlme package, (Pinheiro et al., 2021)] or generalized linear mixed-effect models [glmmTMB package, (Brooks et al., 2017)]. These models included male age, the day of collection and their interaction as fixed effects. Moreover, to account for seasonal variation in sperm quality, the date of each ejaculate collection (designated hereafter as “date”), was also included in the models as a polynomial term of degree three since visual inspection showed a non-linear relationship with the response variables. Male identity was included as a random effect to account for dependency between samples collected from the same male at different time of the season.
The effect of male and sperm age on hatching success was assessed using a generalized linear mixed-effect model. For offspring survival, we restricted our analysis to the first 35 days of life since, according to the breeding protocol, chicks are transferred to different breeding facilities and conditions might interfere or mask the genuine effects of father age and sperm age. However, for reference, analyses up to 60 days are reported in Supplementary Figure 2 and Supplementary Tables 4, 5). Chicks’ survival was modeled using the mixed effects Cox proportional hazards models (coxme package, Therneau, 2012). Offspring growth rate up to day 60 was modeled using a linear mixed-effect model. Preston et al. (2015) showed an effect of mother age on hatching success, therefore we decided to include it in these models as a quadratic fixed effect term in addition to male and sperm age, and hatching date (to correct for possible variation across the season). For hatching success and survival models, female and male identities were used as crossed intercept random effects to account for dependency between siblings, while the female and individual identities were used as nested intercept random effects in the offspring growth rate model to account for dependencies between siblings and repeated measures of the same chick. Finally, the model on offspring growth rate also included offspring age (and squared age), offspring sex, and the interactions between male and offspring age as additional fixed effects, to account for any potential difference in growth rate between sexes and father age groups.
Model families were chosen based on the type of response variable and its distribution. Stepwise backward elimination approach based on the corrected Akaike Information Criterion (Mazerolle, 2006) was used to select the best parsimonious model (the model with the lowest AICc) (Arnold, 2010; Aho et al., 2017). The model assumptions regarding the normality of residuals, homoscedasticity, and independency were checked. In case of assumption violation, data transformation, within-group variability and/or autocorrelation modeling were performed. The marginal effects plots based on the models were generated using the ggeffects R package (Lüdecke, 2018). Differences in sample size across models are due to missing values. More details about the model building and selection are given in Supplementary material.
Ethical statement
The breeding program runs under the approval of the Moroccan Ministry of Agriculture and a mandate independent veterinarian (mandate number: 534–98), providing control of the wellbeing of the birds. Onsite veterinary facilities ensure the best possible care for sick or injured birds by a team of expert veterinarians, and standards from sanitary authorities are regularly controlled.
Results
Ejaculate attributes and sperm traits
Both male and sperm age affected volume and sperm number in the ejaculate. Young males produced ejaculates with larger volume and higher number of sperm (Table 1 and Figures 1A,B). Ejaculates collected after a longer interval (day 5 and 10) from the common collection also had larger volume and more sperm (Table 1 and Figures 1A,B), suggesting that the sperm population kept accumulating with time. The interaction between male and sperm age (day of collection) was not significant and was not retained in the selected most parsimonious model, neither for ejaculate volume nor for number of sperm in the ejaculate.
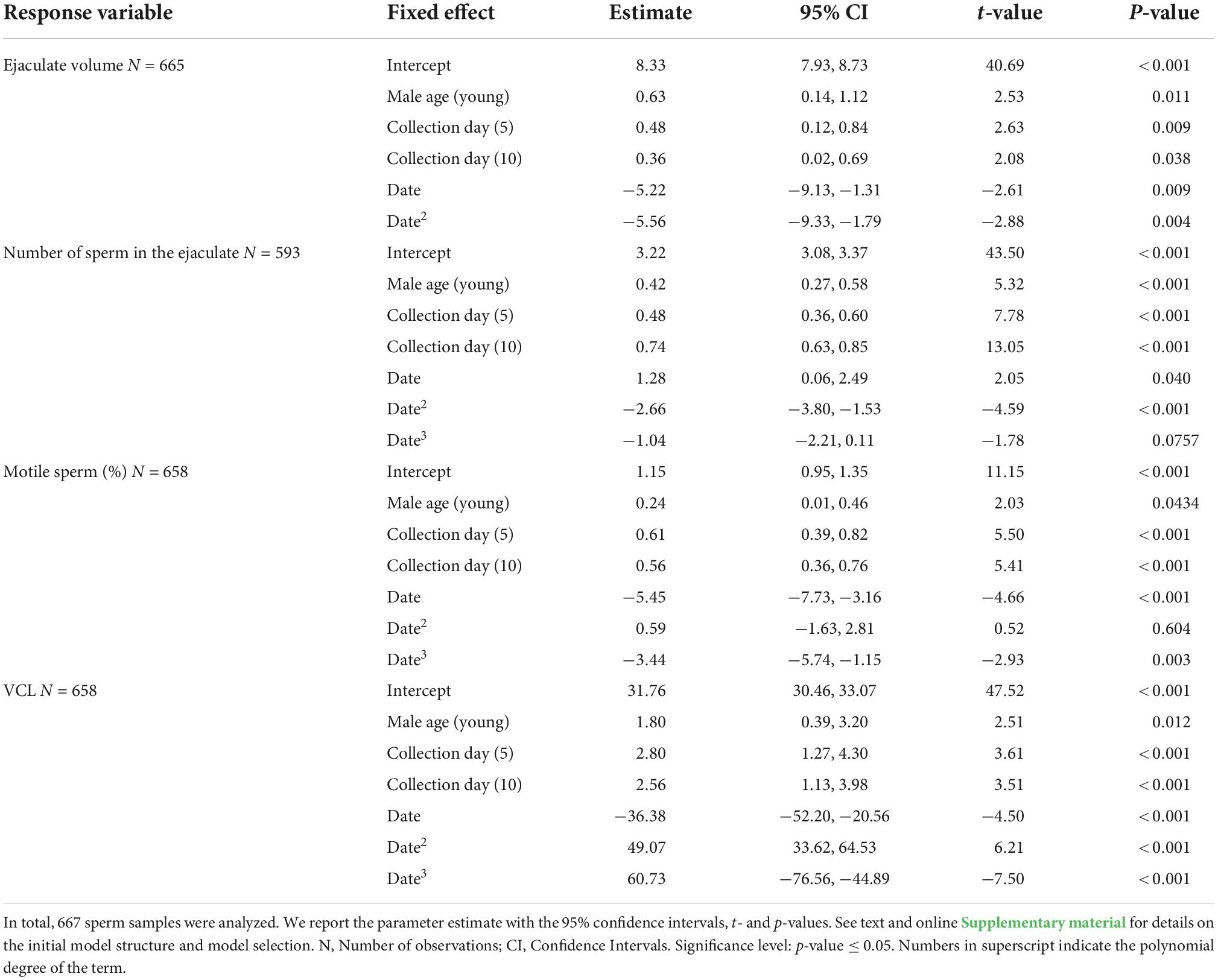
Table 1. Selected parsimonious linear mixed models exploring the effect of male and sperm age on the ejaculate volume, the number of sperm in the ejaculate, the percentage of motile sperm (on the logit scale), and the curvilinear velocity (VCL).
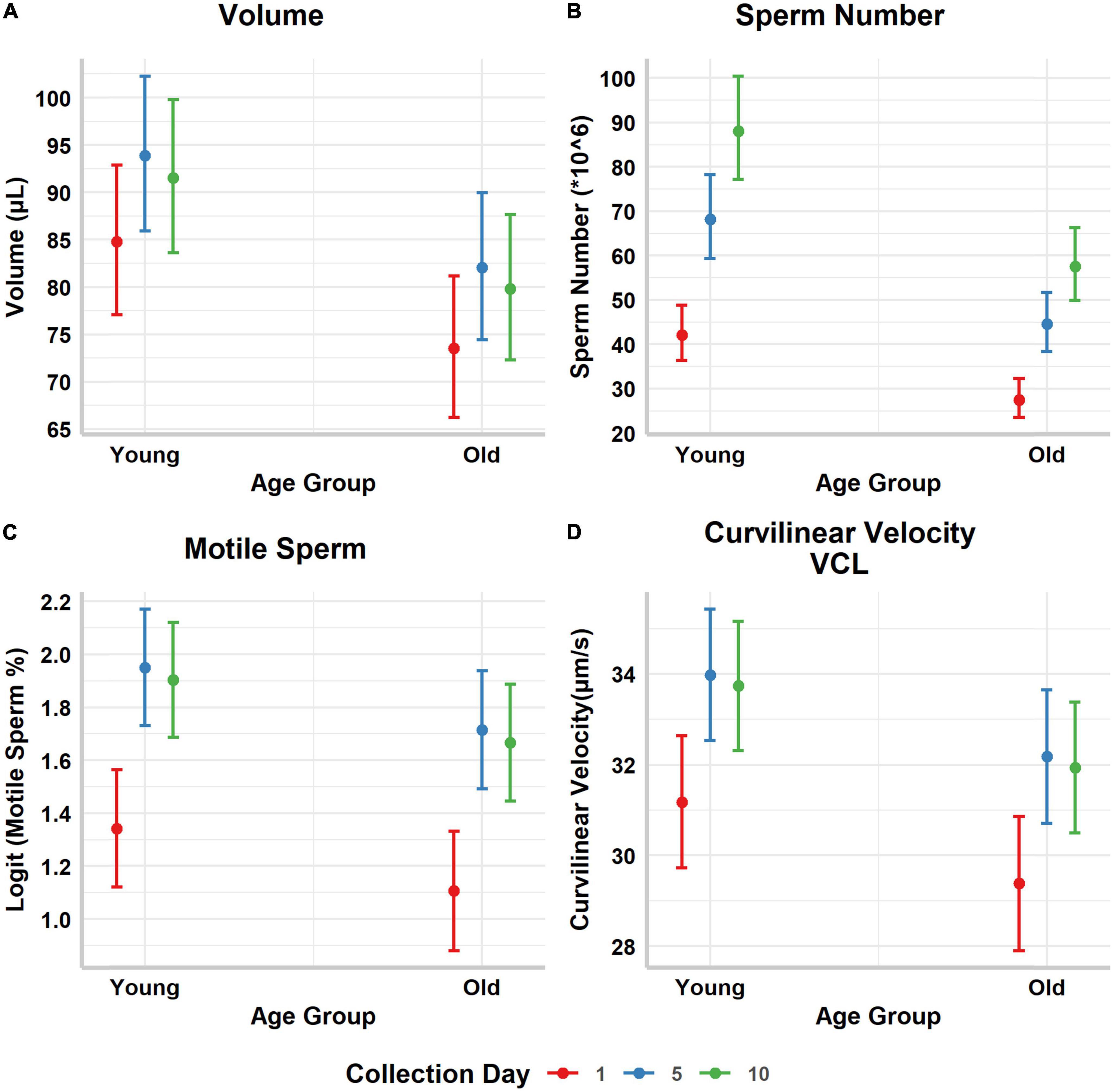
Figure 1. Predicted marginal means and their corresponding 95% confidence intervals of the (A) ejaculate volume (μL), (B) number of sperm in the ejaculate (×106), (C) percentage of motile sperm (on the logit scale), and (D) curvilinear velocity, per male (old vs. young) and sperm age. Collection day refers to the day sperm was collected after a common initial collection, and it is used as a proxy of sperm age.
Young males also had a higher proportion of motile sperm in the ejaculate compared to old males (Table 1 and Figure 1C), and higher sperm curvilinear velocity (VCL) (Table 1 and Figure 1D). As for ejaculate volume and number of sperm, both proportion of motile sperm and sperm velocity were higher in ejaculates collected at day 5 and 10 (Table 1 and Figures 1C,D).
Male age was not retained in the most parsimonious selected models for the percentage of sperm with normal morphology, the percentage of dead sperm, and the percentage of DHE positive sperm per ejaculate (Table 2 and Figure 2). However, the percentage of sperm with normal morphology increased as the collection interval increased (Table 2). Similarly, the percentage of dead sperm per ejaculate was lower when ejaculates were collected after the longest interval from the initial collection (Table 2 and Figure 2A). However, the percentage of DHE positive sperm (a measure of oxidative stress) increased with day of collection, suggesting higher oxidative stress in sperm with prolonged storage time (Table 2 and Figure 2B).
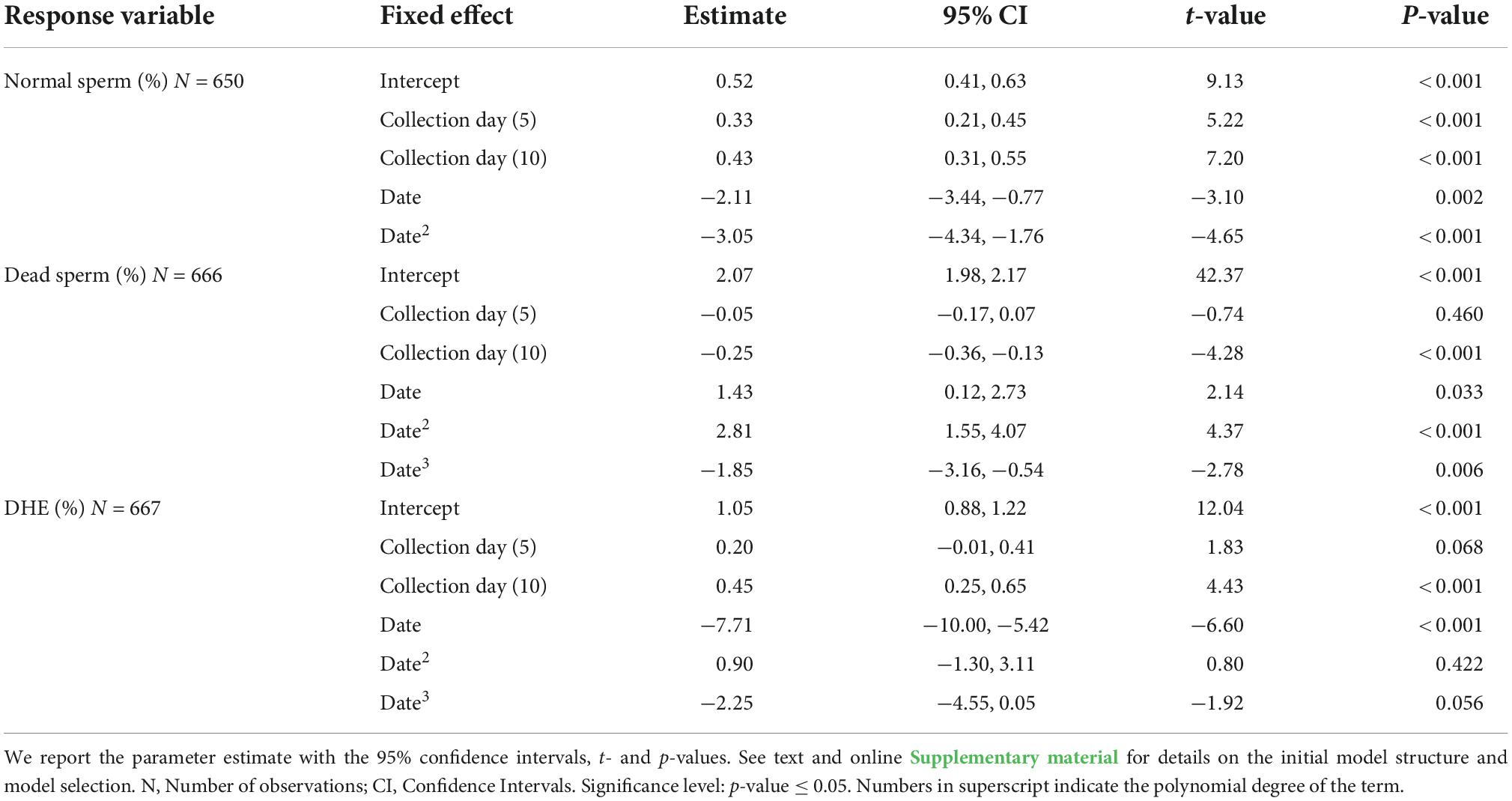
Table 2. Selected parsimonious linear mixed models exploring the effect of male and sperm age on the percentage of normal, dead, and DHE positive sperm.
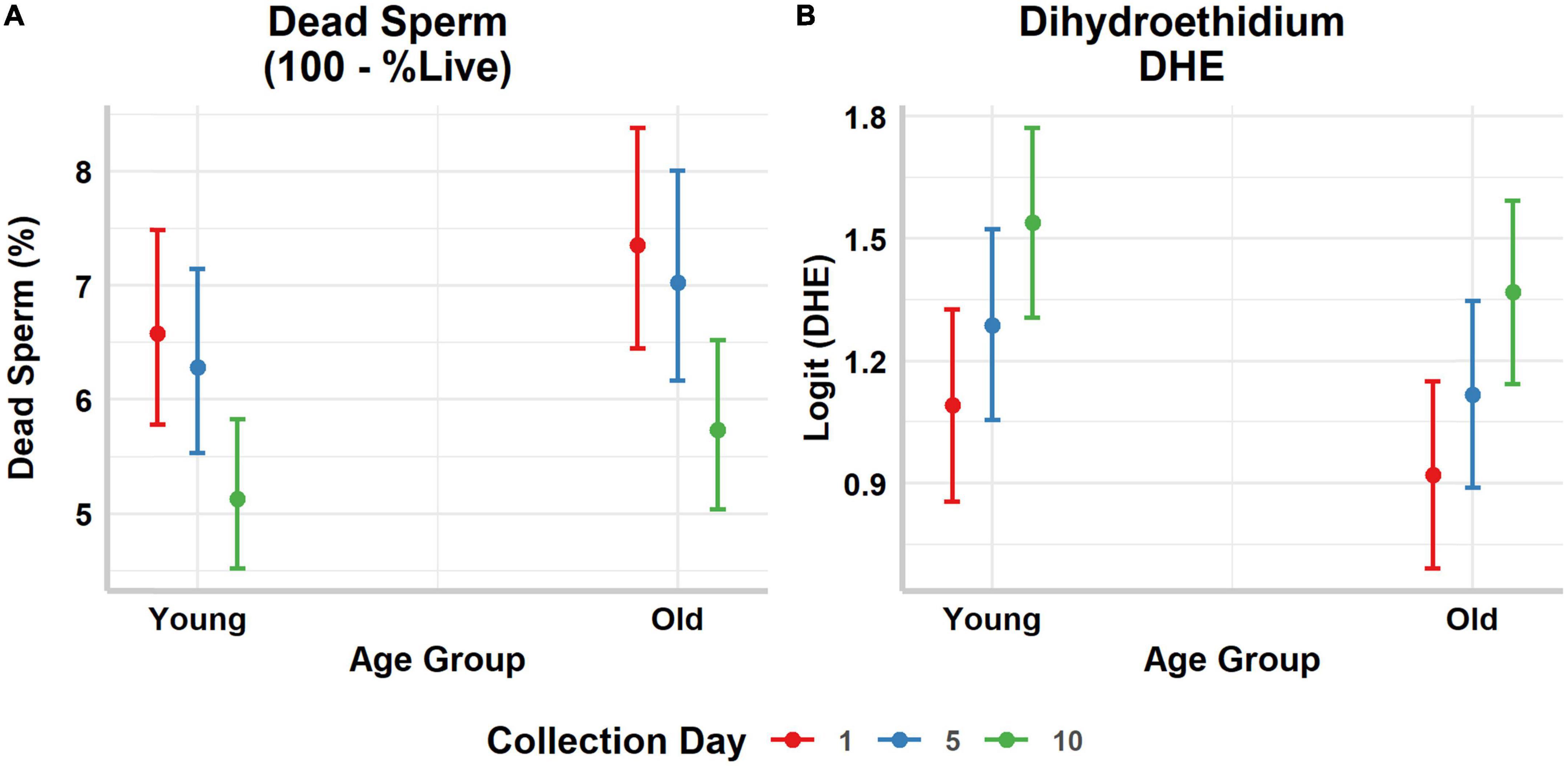
Figure 2. Predicted marginal means and their corresponding 95% confidence intervals of the (A) percentage of dead sperm, (B) and the percentage of DHE positive sperm (on the logit scale), per male (old vs. young) and sperm age. Collection day refers to the day sperm was collected after a common initial collection, and it is used as a proxy of sperm age.
Reproductive success
Over the 545 eggs that were incubated, 393 hatched (72.11%). Hatching success did not differ according to male age (however, older females laid eggs with lower hatching success) (Table 3 and Figure 3A). Females inseminated with samples collected at day 5 had the highest hatching success (Table 3 and Figure 3A). The interaction between male and sperm age was not significant and not retained in the most parsimonious model. The delay between insemination and egg laying was similar for females inseminated with ejaculates collected at the 3 different intervals (Supplementary Data and Supplementary Table 2; Day 1 mean ± SD = 6.97 ± 2.23 days, Day 5 mean ± SD = 7.88 ± 2.17 days, Day 10 mean ± SD = 7.62 ± 2.01 days).
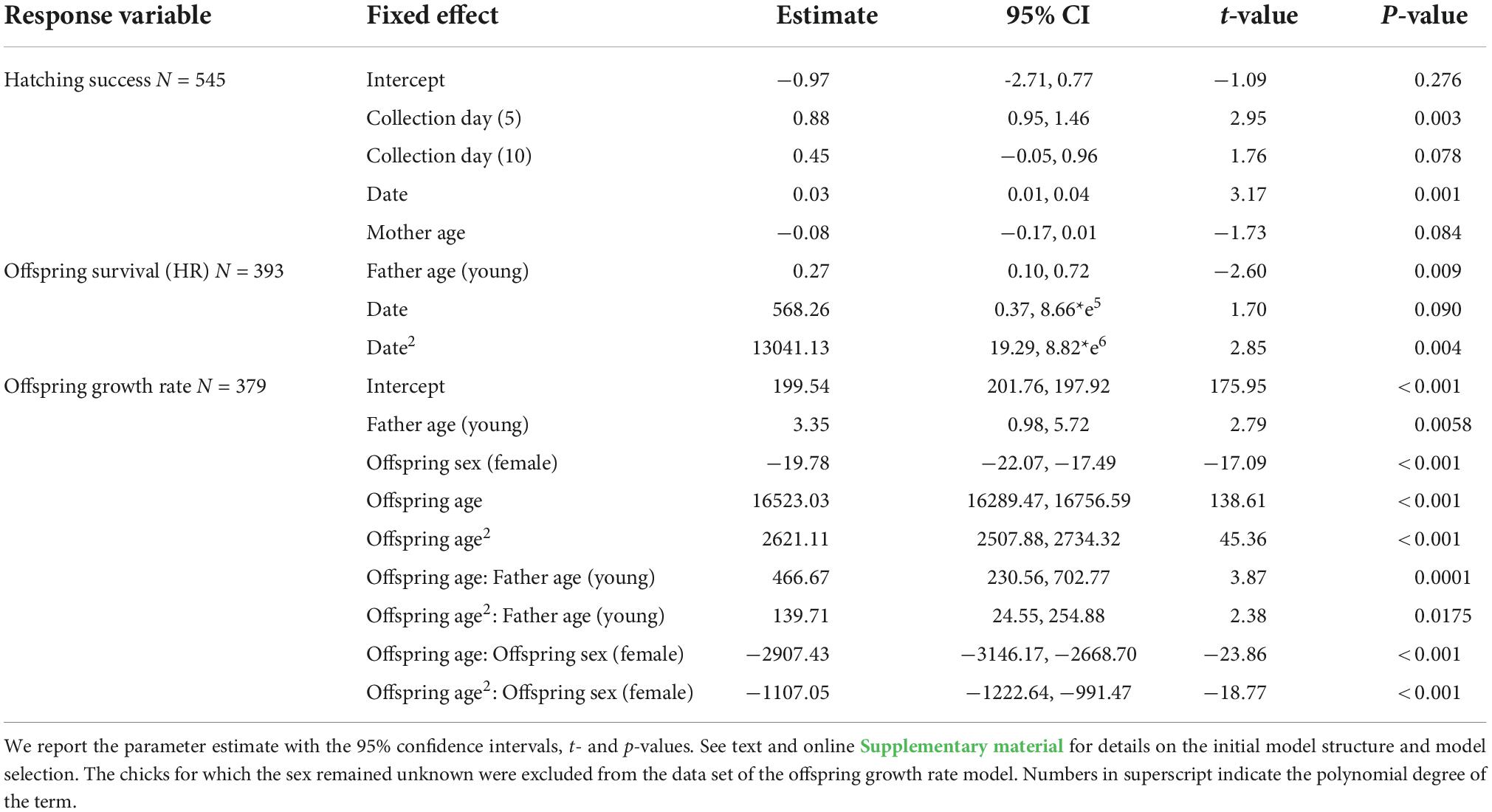
Table 3. Selected parsimonious generalized mixed linear models exploring the effect of male and sperm age on hatching success, offspring survival and growth rate.
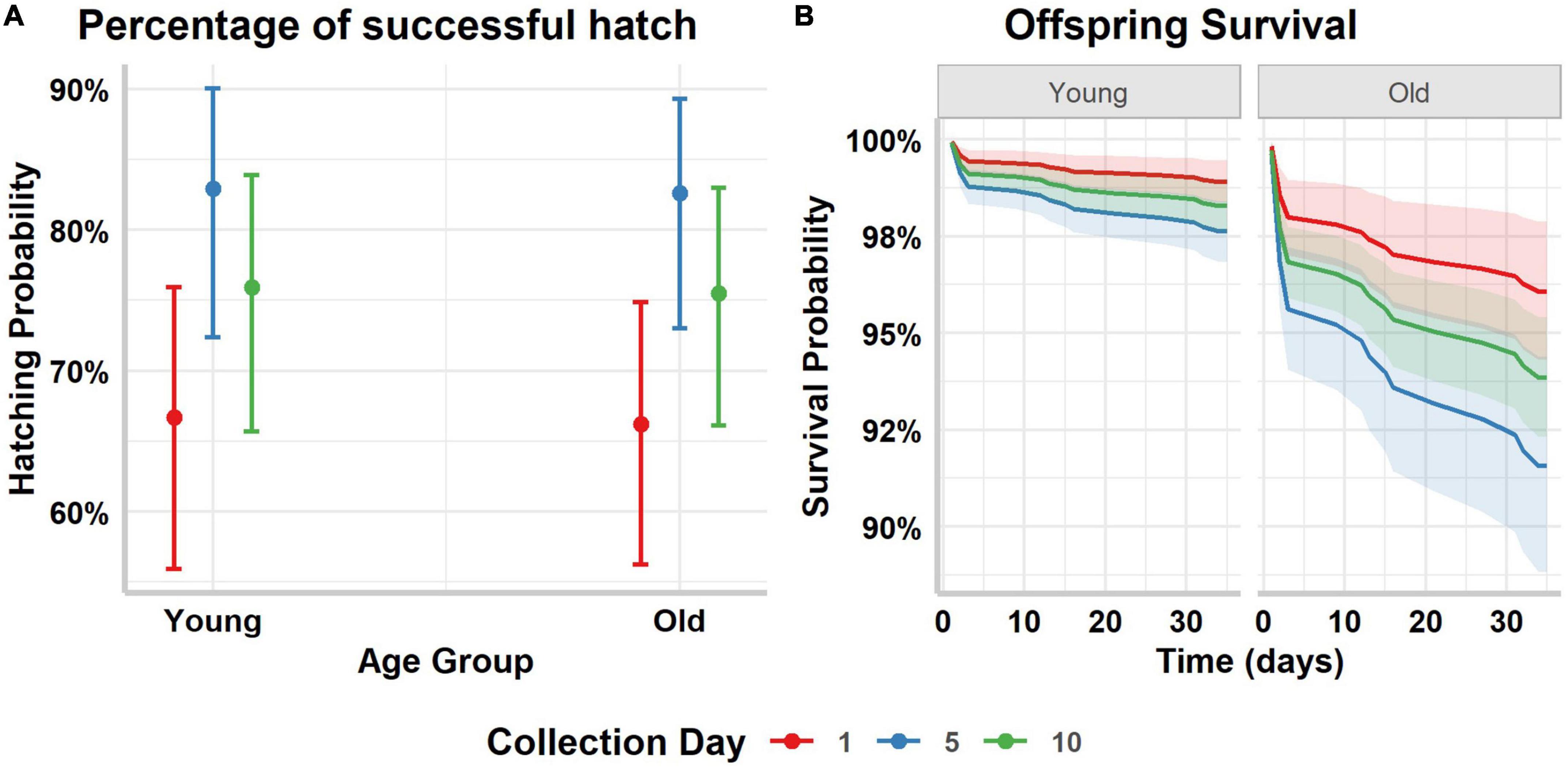
Figure 3. Predicted marginal means of the (A) hatching success and the corresponding 95% confidence intervals, (B) and chick survival during the first month of life and the corresponding standard errors, per male and sperm age. Collection day refers to the day sperm was collected after a common initial collection, and it is used as a proxy of sperm age.
Offspring sired by old males had a slower growth rate during the first 60 days compared to offspring sired by young males, as shown by a significant interaction between male and offspring age (Table 3). Offspring sired by young males gained on average 0.5 g per day more than those sired by old males, which resulted in a weight difference of 27 g at 60 days [mean weight of chicks sired by old males = 736.37 g (SE = 6.60, CI:723.44, 749.30) for females and 915.68 g (SE = 5.75, CI: 900.43, 930.23) for males; mean weight of chicks sired by young males = 763.62 g (SE = 7.61, CI:752.05, 774.59) and 942.60 g (SE = 6.84, CI:928.89, 955.67) for females and males, respectively]. Neither sperm age alone, nor the interaction between father and sperm age were significant predictors of offspring growth rate.
Twenty-one chicks out of 393 hatchlings died during the first 35 days of life. Offspring sired by young males had a better survival rate compared to offspring sired by old males (Table 3 and Figure 3B). A higher mortality rate was mainly seen in the first 3 days of chicks’ life. Neither sperm age, nor the interaction between male and sperm age affected offspring survival.
Discussion
The aim of the present study was to investigate pre- and post-meiotic senescence and their potential interaction in males of a bird species with a promiscuous mating system, the houbara bustard. In agreement with previous work conducted on the species, we found that pre-meiotic effects are important determinants of ejaculate and sperm quality, and male reproductive success. However, contrary to our prediction, we found that prolonged sperm storage did not impair sperm quality and did not reduce reproductive success, in both young and old males. Thus, we did not find any evidence suggesting that male age might accelerate post-meiotic senescence.
The deleterious effect of male age on sperm quality and reproductive success was expected as it has already been reported in the houbara bustard and other species. Ejaculate attributes such as ejaculate volume, sperm number and mass motility index have been previously shown to increase during the first years of life up to the age of 5–6 years and then gradually decline (Preston et al., 2011). Here, we confirmed these previous findings since old males (mean age of 13.58 years old) produced ejaculates with lower volume and with less sperm. We extended previous findings by using flow cytometry and computer-assisted techniques to assess several sperm traits that collectively contribute to define sperm quality. We found that young males had a higher percentage of motile sperm and sperm with higher velocity (VCL) compared to old individuals. However, we did not find evidence suggesting that male age affected other traits related to sperm quality such as the percentage of dead sperm or the percentage of DHE positive sperm in the ejaculate corresponding to the intercellular ROS production, a marker for oxidative stress. These results suggest age-specific effects on different components of sperm and ejaculate quality, similar to the observation that different phenotypic traits have different rates of age-dependent decline (e.g., Lecomte et al., 2010; Hayward et al., 2015).
In addition, to reducing ejaculate and sperm quality, father age has also been shown to affect negatively both offspring growth (Preston et al., 2015) and post-release offspring survival (Vuarin et al., 2019a) in the houbara bustard. Here, we also found that offspring sired by old males had a slightly slower growth rate during the first 60 days of life and a higher mortality rate during the first 35 days of life in captivity, indicating high fitness cost due to reproductive senescence in this species. Despite the statistical significance of the difference in growth rate between paternal age groups, its biological relevance and impact on fitness should be further investigated, especially in the wild.
Contrary to our prediction, post-meiotic aging did not impair sperm quality and reproductive success. We used a proxy of post-meiotic aging, since we compared ejaculates that were collected at different intervals with respect to a common initial collection: the longer the interval, the longer the storage period, and we assumed that a longer storage would increase the proportion of old sperm in the ejaculate. Similar reasoning was used in other studies to assess the effect of sperm age on reproductive success. For instance, White et al. (2008) equipped male kittiwakes with rings on the cloaca preventing sperm transfer during mating. Some birds wore the rings for few days, others up to 2 weeks. Once the rings were removed, birds were able to copulate again, and the longer the period of ring wearing the older the sperm transferred. Using this elegant experimental design, they found that sperm aging indeed impaired male reproductive success, in terms of hatching success and hatchling condition (White et al., 2008). In a more recent study, sperm of male zebrafish were initially collected, then males were isolated during 4, 7, or 12 days (sexual rest), after which their sperm were collected again, imposing sexual rest and consequently sperm storage in males, resulting in reduced sperm velocity (Cattelan and Gasparini, 2021). However, it should be fully acknowledged that other studies have failed to report the predicted effect of sperm age on sperm quality. For instance, Firman et al. (2015) did not find any evidence of decreased sperm quality in house mice (Mus domesticus) experiencing a 2-month sexual rest compared to sexually active males. Even within species, the effect of sperm age might be inconsistent since, contrary to Cattelan and Gasparini (2021), a previous work conducted also on zebrafish found that old (stored) sperm had higher velocity compared to freshly produced ones (Vega-Trejo et al., 2019).
Despite an experimental design similar to those adopted in previous studies, we did not find evidence for deleterious effects of sperm aging. Ejaculates collected at day 5 and 10 had higher volume and contained more sperm compared to ejaculates collected at day 1, showing that sperm accumulated in the epididymis and vas deferens over time, in the absence of mating opportunities. However, unexpectedly, the increase in sperm age resulted in a higher proportion of motile sperm in the ejaculate, higher velocity, higher proportion of sperm with normal morphology, and less dead sperm in the ejaculate. The only phenotypic trait potentially suggesting a possible decline in sperm quality with storage was the higher proportion of DHE positive sperm. However, this did not produce any deleterious effect in terms of male reproductive success since eggs fertilized with sperm after a prolonged storage had a higher hatching success and sperm age did not have any effect on chick growth rate and survival.
There are several possible explanations for these results. It is possible that our experimental design failed to produce a substantial change in the age distribution of sperm. The effect of sexual abstinence has been extensively studied in humans (Ayad et al., 2018), and recently, Okada et al. (2020) found an increase on DHE positive cells in male ejaculates with a 4-day abstinence in comparison to 1 day. They suggested that this increase would appear when sperm surpass the physiological necessary epididymal transit. The distribution of sperm age at a given time accumulated in male tract will depend on a differentiation/death process, where new sperm are produced, age and die. In the absence of sperm release (during sexual rest), the variance in sperm age will increase, with the occurrence of old and new sperm in the subsequent ejaculate. This could explain an increase in volume and sperm number along with some level of substitution of dead by viable sperm cells with time, partially explaining why the percentage of dead sperm and defective cells is improved with storage in our study. Physiological loss of sperm cells in the male tract to the cloaca and by duct cells absorption in birds is expected at some rate, however, in domestic fowls it needed more than 4 weeks of storage to observe dead sperm cells being processed by the duct structure (Tingari and Lake, 1972). Nothing is known about such process in bustards, if it happens and at which rate. With this respect, it should also be noted that the longest rest duration in our experimental design corresponds to the average duration of spermatogenesis in birds [ca 10 days in Japanese quails (Coturnix japonica), 12 days in barbary drakes, 13–15 days in domestic fowls (Jones and Lin, 1993)]. How sperm are stored in the male reproductive tract can have profound consequences on the reproductive success of males (Reinhardt, 2007). Moreover, if the number of sperm released in the ejaculate is large enough, the reproductive success might still be ensured by the young sperm present in the ejaculate. This also highlights the possible role of post-copulatory selection (including cryptic female choice) as a filter promoting younger sperm within the ejaculate to fertilize the ovum. Finally, it could be that sperm aging occurring post-mating in the female reproductive tract might obscure any effect of prolonged storage in the male reproductive tract. Particularly, this can occur if the rate of sperm aging follows a non-linear (accelerating) trend. To explore this issue, we compared the delay between insemination and egg laying (a proxy of the time sperm spent in the female reproductive tract) and found no difference between the experimental groups (the delay between insemination and egg laying being around 7 days in the three sperm age groups, see Supplementary material). This suggests that any additional effect of sperm aging in the female reproductive tract was constant across experimental groups. According to the literature sperm stays quiescent inside the females STT, losing motility and only being released for fertilization of the ovulated oocyte (Sasanami et al., 2013). Molecular exchanges between sperm membrane and STT/female tract fluids do occur, and lactic acid concentrations for example play an important role on sperm release and retention (Matsuzaki et al., 2015). Moreover, molecules of the utero vaginal junction involved in sperm storage have been identified and have shown to improve sperm viability in vitro when added to the diluent (Matsuzaki et al., 2020) but no evidence of sperm remodeling or cell maturation per se were described so far. However, once more, little is known about avian species and especially wild models.
Alternative explanations for our findings refer to the process of post-testicular sperm maturation. In mammals, sperm released in the lumen of the seminiferous tubules at the end of spermatogenesis are not able to fertilize the ovum, yet. During their transit through the epididymis, sperm undergo several morphological and biochemical changes such as nuclear compaction, modification of the membrane composition, loss of cytoplasmic droplet and gain of factors necessary for oocyte binding, leading to sperm capacitation (James et al., 2020). However, in birds, the role of sperm maturation in the epididymis remains unclear. The epididymis is much shorter in birds compared to mammals and sperm transits through the epididymis faster due to the high rate of sperm production. Indeed, spermatogenesis is a much longer process in mammals compare to birds as it ranges from 35 days in the mice to 74 days in man (Bennett, 1977). Furthermore, artificial insemination with testicular sperm does produce fertile eggs in poultry which suggests that maturation in the epididymis is not a necessary condition for ovum fertilization (Munro, 1938; Howarth, 1983). However, other work has suggested that maturational changes do occur in birds as well. For instance, increasing sperm motility and velocity through epididymal transit has been reported in the domestic fowl (Munro, 1938; Ahammad et al., 2011a) and in the Japanese quail (Clulow and Jones, 1982). Similarly, in poultry, viability and fertilization capacity gradually increase from testes to vas deferentia (Ahammad et al., 2011a,b). The ejaculate is not only made of sperm cells. Indeed, after ejaculation spermatozoa are transported by seminal plasma, a very complex fluid composed of a diversity of molecules. Therefore, sperm cells interact with seminal proteins and other molecules impacting sperm physiology and fertilization outcome (Druart and de Graaf, 2018). Proteomics studies of seminal plasma in avian species have identified proteins associated with fertility in the turkey (Słowińska et al., 2017), in the chicken (Labas et al., 2015) and also with age and sperm velocity in the Red Junglefowl (Borziak et al., 2016). More recently, extracellular vesicles were found in chicken seminal plasma with different size and proteins markers between fertile and sub fertile males (Cordeiro et al., 2021). Another promising topic is the differences in miRNAs in high- and low-quality semen samples, miRNA patterns can be different depending on the male status and environmental changes, and differences between high and low motility samples were recently described in roosters (Xing et al., 2022). Further work comparing the composition of seminal plasma and the presence of different patterns of miRNAs of ejaculates between different storage time and between old and young males in the houbara bustard would be of great interest.
As for organismal aging, the rate of sperm aging is certainly not constant neither among-species nor among-individuals within species. The rate of sperm aging can be modulated by several factors (see Reinhardt, 2007 for a review). However, investment into mechanisms that limit age-associated damage does not come without cost. Therefore, natural selection could have shaped the evolution of anti-aging mechanisms according to potential fitness costs of accumulating old sperm in the ejaculate. In several bird species, females mate several times with the same male. Repeated copulations with the same male might provide freshly produced sperm for ovum fertilization. Actually, in some social species, females have been observed to eject sperm when mating occurs too early with respect to egg laying (White et al., 2008), which might represent a female strategy to avoid fertilization with old sperm. Spontaneous sperm discharge has also been suggested in males of several bird species, as shown by the presence of sperm in the cloaca of individuals sampled during the spring migration (before the mating season had started) (Quay, 1985). Sperm release might be a mechanism allowing males to get rid of sperm that had been stored for long time before reaching the breeding ground. The North African houbara bustard is a solitary bird dwelling at very low density in rocky deserts and steppes (Monnier-Corbel et al., 2022). Although males cluster in leks during the mating season, the lek sites are dispersed over much larger areas compared to classical lekking species. Therefore, the mating frequency of males is certainly very low and probably most of them only mate a handful of times (or maybe never) during the whole mating period. In species where males have reduced sexual opportunities, it is straightforward to predict the evolution of mechanisms allowing to protect stored sperm from oxidative attack. This might also apply to sperm that are stored in the female sperm storage tubules which preserve the sperm viability for weeks.
Our experimental set up involved homospermic (single male) inseminations. Accordingly, our measure of reproductive success only refers to the male capacity to fertilize eggs that hatch and produce viable offspring. Obviously, a different picture might emerge when ejaculates of different males compete for ovum fertilization. Female houbara bustards do mate with multiple males during the same reproductive bout, therefore there is scope for sperm competition to operate in this species (Lesobre et al., 2010). Under sperm competition, selection on traits promoting ovum fertilization is exacerbated (Vuarin et al., 2019b). For instance, small reduction in motility might have no consequences under homospermic mating, whereas sperm with lower motility can be easily outcompeted if females mate with multiple males. Previous work conducted on the houbara bustard has shown that when sperm of young and old males compete for ovum fertilization (during mixed sperm inseminations), young males have a substantial advantage since they have a larger paternity share compared to the 1:1 null hypothesis (Vuarin et al., 2019a). Therefore, an extension of the present work could consider performing competitive inseminations using sperm of different ages. Future work should also extend the period of sperm storage to fully assess the possible duration of sperm lifespan and perhaps increase the likelihood to observe sperm aging in this species.
Data availability statement
The original contributions presented in this study are included in the article/Supplementary material, further inquiries can be directed to the corresponding author.
Ethics statement
Ethical review and approval was not required for the animal study because the breeding program runs under the approval of the Moroccan Ministry of Agriculture and a mandate Independent Veterinarian (mandate number: 534–98), providing control of the wellbeing of the birds. Onsite veterinary facilities ensure the best possible care for sick or injured birds by a team of expert veterinarians, and standards from sanitary authorities are regularly controlled.
Author contributions
YH, MS-J, LL, GS, and JT conceived the study. LM, NR, and JT collected the data. HAH analyzed the data and prepared the tables and figures. YH and LL curated and supervised the conservation breeding program. LM and GS wrote the first draft of the manuscript. All authors edited, revised, and approved the final version.
Funding
This study was funded by the Emirates Center for Wildlife Propagation (ECWP), a program of the International Fund for Houbara Conservation (IFHC). The authors declare that this study received funding from IFHC. The funder was not involved in the study design, collection, analysis, interpretation of data, the writing of this article, or the decision to submit it for publication.
Acknowledgments
Funds and samples used in this study were provided by the International Fund for Houbara Conservation (IFHC). We are grateful to His Highness Sheikh Mohamed bin Zayed Al Nahyan, President of the United Arab Emirates and founder of the IFHC, His Highness Sheikh Theyab bin Mohamed Al Nahyan, Chairman of the IFHC, and His Excellency Mohammed Ahmed Al Bowardi, Deputy Chairman, for their support. This study was conducted under the guidance of Reneco International Wildlife Consultants LLC, a consulting company that manages the IFHC’s conservation programmes. We thank Dr. Frédéric Lacroix, Managing Director of Reneco, for his supervision, as well as all staff of Reneco who participated in data collection.
Conflict of interest
LM, HAH, YH, LL, and JT were employed by Reneco International Wildlife Consultants LLC.
The remaining authors declare that the research was conducted in the absence of any commercial or financial relationships that could be construed as a potential conflict of interest.
Publisher’s note
All claims expressed in this article are solely those of the authors and do not necessarily represent those of their affiliated organizations, or those of the publisher, the editors and the reviewers. Any product that may be evaluated in this article, or claim that may be made by its manufacturer, is not guaranteed or endorsed by the publisher.
Supplementary material
The Supplementary Material for this article can be found online at: https://www.frontiersin.org/articles/10.3389/fevo.2022.977184/full#supplementary-material
References
Ahammad, M. U., Nishino, C., Tatemoto, H., Okura, N., Kawamoto, Y., Okamoto, S., et al. (2011a). Maturational changes in motility, acrosomal proteolytic activity, and penetrability of the inner perivitelline layer of fowl sperm, during their passage through the male genital tract. Theriogenology 76, 1100–1109. doi: 10.1016/j.theriogenology.2011.05.017
Ahammad, M. U., Nishino, C., Tatemoto, H., Okura, N., Kawamoto, Y., Okamoto, S., et al. (2011b). Maturational changes in the survivability and fertility of fowl sperm during their passage through the male reproductive tract. Anim. Reprod. Sci. 128, 129–136. doi: 10.1016/j.anireprosci.2011.09.010
Aho, K., Derryberry, D., and Peterson, T. (2017). A graphical framework for model selection criteria and significance tests: Refutation, confirmation and ecology. Methods Ecol. Evol. 8, 47–56. doi: 10.1111/2041-210X.12648
Aitken, R. J. (2020). Impact of oxidative stress on male and female germ cells: Implications for fertility. Reproduction 159:R189–R201. doi: 10.1530/REP-19-0452
Alkan, S., Baran, A., Özdaş, ÖB., and Evecen, M. (2002). Morphological defects in Turkey semen. Turk. J. Vet. Anim. Sci. 26, 1087–1092.
Arnold, T. W. (2010). Uninformative parameters and model selection using akaike’s information criterion. J. Wildlife. Manage. 74, 1175–1178. doi: 10.1111/j.1937-2817.2010.tb01236.x
Ayad, B. M., Van der Horst, G., and Plessis, S. S. D. (2018). Revisiting the relationship between the ejaculatory abstinence period and semen characteristics. Int. J. Fertil. Steril. 11, 238–246. doi: 10.22074/ijfs.2018.5192
Bennett, M. D. (1977). The time and duration of meiosis. Philos. Trans. R. Soc. Lond. B 277, 201–226. doi: 10.1098/rstb.1977.0012
Birkhead, T. R., and Pizzari, T. (2002). Postcopulatory sexual selection. Nat. Rev. Genet. 3, 262–273. doi: 10.1038/nrg774
Blesbois, E., and Brillard, J. P. (2007). Specific features of in vivo and in vitro sperm storage in birds. Animal 1, 1472–1481. doi: 10.1017/S175173110700081X
Bloom, E. (1973). Ultrastructure of some characteristic sperm defects and a proposal for a new classification of the bull spermiogram. Nord. Vet. Med. 25, 383–391.
Borziak, K., Álvarez-Fernández, A., Karr, T. L., Pizzari, T., and Dorus, S. (2016). The Seminal fluid proteome of the polyandrous red junglefowl offers insights into the molecular basis of fertility, reproductive ageing and domestication. Sci. Rep. 6:35864. doi: 10.1038/srep35864
Brooks, M. E., Kristensen, K., van Benthem, K. J., Magnusson, A., Berg, C. W., Nielsen, A., et al. (2017). glmmTMB balances speed and flexibility among packages for zero-inflated generalized linear mixed modeling. R. J. 9:378. doi: 10.32614/RJ-2017-066
Carreira, J., Trevizan, J., Carvalho, I., Kipper, B., Rodrigues, L., Silva, C., et al. (2017). Does sperm quality and DNA integrity differ in cryopreserved semen samples from young, adult, and aged Nellore bulls? Basic Clin. Androl. 21:12. doi: 10.1186/s12610-017-0056-9
Cattelan, S., and Gasparini, C. (2021). Male sperm storage impairs sperm quality in the zebrafish. Sci. Rep. 11:16689. doi: 10.1038/s41598-021-94976-x
Clulow, J., and Jones, R. C. (1982). Production, transport, maturation, storage and survival of spermatozoa in the male Japanese quail, coturnix coturnix. J. Reprod. Fertil. 64, 259–266. doi: 10.1530/jrf.0.0640259
Colasante, A., Minasi, M., Scarselli, F., Casciani, V., Zazzaro, V., Ruberti, A., et al. (2019). The aging male: Relationship between male age, sperm quality and sperm DNA damage in an unselected population of 3124 men attending the fertility centre for the first time. Arch. Ital. Urol. Androl. 90, 254–259. doi: 10.4081/aiua.2018.4.254
Cordeiro, L., Lin, H., Vitorino Carvalho, A., Grasseau, I., Uzbekov, R., and Blesbois, E. (2021). First insights on seminal extracellular vesicles in chickens of contrasted fertility. Reproduction 161, 489–498. doi: 10.1530/REP-20-0462
Druart, X., and de Graaf, S. (2018). Seminal plasma proteomes and sperm fertility. Anim. Reprod. Sci. 194, 33–40. doi: 10.1016/j.anireprosci.2018.04.061
Evans, J. P., Wilson, A. J., Pilastro, A., and Garcia-Gonzalez, F. (2019). Ejaculate-mediated paternal effects: Evidence, mechanisms and evolutionary implications. Reproduction 157:R109–R126. doi: 10.1530/REP-18-0524
Farooq, U., Malecki, I., Mahmood, M., and Martin, G. (2020). Age-related declines in ejaculate quality and sperm kinematics vary among strains of Japanese Quail (Coturnix japonica). Reprod. Domest. Anim. 55, 64–73. doi: 10.1111/rda.13585
Fay, R., Barbraud, C., Delord, K., and Weimerskirch, H. (2016). Paternal but not maternal age influences early-life performance of offspring in a long-lived seabird. Proc. R. Soc. B. 283:20152318. doi: 10.1098/rspb.2015.2318
Firman, R. C., Young, F. J., Rowe, D. C., Duong, H. T., and Gasparini, C. (2015). Sexual rest and post-meiotic sperm ageing in house mice. J. Evol. Biol. 28, 1373–1382. doi: 10.1111/jeb.12661
Fricke, C., and Koppik, M. (2019). Male reproductive ageing: A tale of the whole ejaculate. Reproduction 158:R219–R229. doi: 10.1530/REP-18-0579
Garner, D. L., Johnson, L. A., Yue, S. T., Roth, B. L., and Haugland, R. P. (1994). Dual DNA staining assessment of bovine sperm viability using SYBR-14 and propidium iodide. J. Androl. 15, 620–629.
Gasparini, C., Dosselli, R., and Evans, J. P. (2017). Sperm storage by males causes changes in sperm phenotype and influences the reproductive fitness of males and their sons. Evol. Lett. 1, 16–25. doi: 10.1002/evl3.2
Gasparini, C., Kelley, J. L., and Evans, J. P. (2014). Male sperm storage compromises sperm motility in guppies. Biol. Lett. 10:20140681. doi: 10.1098/rsbl.2014.0681
Gasparini, C., Marino, I., Boschetto, C., and Pilastro, A. (2010). Effect of male age on sperm traits and sperm competition success in the guppy (Poecilia reticulata). J. Evol. Biol. 23, 124–135. doi: 10.1111/j.1420-9101.2009.01889.x
Gillan, L., Evans, G., and Maxwell, W. M. C. (2005). Flow cytometric evaluation of sperm parameters in relation to fertility potential. Theriogenology 63, 445–457. doi: 10.1016/j.theriogenology.2004.09.024
Gunes, S., Hekim, G. N. T., Arslan, M. A., and Asci, R. (2016). Effects of aging on the male reproductive system. J. Assist. Reprod. Genet. 33, 441–454. doi: 10.1007/s10815-016-0663-y
Hale, J. M., Elgar, M. A., and Jones, T. M. (2008). Sperm quantity explains age-related variation in fertilization success in the hide beetle. Ethology 114, 797–807. doi: 10.1111/j.1439-0310.2008.01534.x
Hayward, A. D., Moorad, J., Regan, C. E., Berenos, C., Pilkington, J. G., Pemberton, J. M., et al. (2015). Asynchrony of senescence among phenotypic traits in a wild mammal population. Exp. Gerontol. 71, 56–68. doi: 10.1016/j.exger.2015.08.003
Herrera-Cruz, M., Abraham, S., Nuñez-Beverido, N., Flores-Estévez, N., Reyes-Hernández, M., Alvarado, M., et al. (2018). Male age and strain affect ejaculate quality in the Mexican fruit fly. Insect Sci. 25, 703–711. doi: 10.1111/1744-7917.12446
Howarth, B. (1983). Fertilizing ability of cock spermatozoa from the testis epididymis and vas deferens following intramagnal insemination. Biol. Reprod. 28, 586–590. doi: 10.1095/biolreprod28.3.586
Immler, S. (2018). The sperm factor: Paternal impact beyond genes. Heredity 121, 239–247. doi: 10.1038/s41437-018-0111-0
James, E. R., Carrell, D. T., Aston, K. I., Jenkins, T. G., Yeste, M., and Salas-Huetos, A. (2020). The role of the epididymis and the contribution of epididymosomes to mammalian reproduction. Int. J. Mol. Sci. 21:5377. doi: 10.3390/ijms21155377
Johnson, S. L., Dunleavy, J., Gemmell, N. J., and Nakagawa, S. (2015). Consistent age-dependent declines in human semen quality: A systematic review and meta-analysis. Ageing Res. Rev. 19, 22–33. doi: 10.1016/j.arr.2014.10.007
Kim, S.-Y., Velando, A., Torres, R., and Drummond, H. (2011). Effects of recruiting age on senescence, lifespan and lifetime reproductive success in a long-lived seabird. Oecologia 166, 615–626. doi: 10.1007/s00442-011-1914-3
Kong, A., Frigge, M. L., Masson, G., Besenbacher, S., Sulem, P., Magnusson, G., et al. (2012). Rate of de novo mutations and the importance of father’s age to disease risk. Nature 488, 471–475. doi: 10.1038/nature11396
Labas, V., Grasseau, I., Cahier, K., Gargaros, A., Harichaux, G., Teixeira-Gomes, A., et al. (2015). Qualitative and quantitative peptidomic and proteomic approaches to phenotyping chicken semen. J. Proteomics 112, 313–335. doi: 10.1016/j.jprot.2014.07.024
Lacroix, F. (2003). The emirates center for wildlife propagation: developing a comprehensive strategy to secure a self-sustaining population of houbara bustards in Eastern Morocco. Houbara News 5:2.
Lake, P. E., and Ravie, O. (1984). An exploration of cryoprotective compounds for fowl spermatozoa. Br. Poult. Sci. 25, 145–150. doi: 10.1080/13632758408454852
Lecomte, V. J., Sorci, G., Cornet, S., Jaeger, A., Faivre, B., Arnoux, E., et al. (2010). Patterns of aging in the long-lived wandering albatross. Proc. Natl. Acad. Sci. U.S.A. 107, 6370–6375. doi: 10.1073/pnas.0911181107
Lemaître, J.-F., and Gaillard, J.-M. (2017). Reproductive senescence: New perspectives in the wild. Biol. Rev. Camb. Philos. Soc. 92, 2182–2199. doi: 10.1111/brv.12328
Lesobre, L., Lacroix, F., Le Nuz, E., Hingrat, Y., Chalah, T., and Saint Jalme, M. (2010). Absence of male reproductive skew, along with high frequency of polyandry and conspecific brood parasitism in the lekking Houbara bustard Chlamydotis undulata undulata. J. Avian Biol. 41, 117–127. doi: 10.1111/j.1600-048X.2009.04794.x
Lippens, C., Faivre, B., Lechenault, C., and Sorci, G. (2017). Aging parasites produce offspring with poor fitness prospects. Biol. Lett. 13:20160888. doi: 10.1098/rsbl.2016.0888
Lodge, J. R., Fechheimer, N. S., and Jaap, R. G. (1971). The relationship of in vivo sperm storage interval to fertility and embryonic survival in the chicken. Biol. Reprod. 5, 252–257. doi: 10.1093/biolreprod/5.3.252
Lüdecke, D. (2018). ggeffects: tidy data frames of marginal effects from regression models. J. Open Source Softw. 3:772. doi: 10.21105/joss.00772
Matsuzaki, M., Mizushima, S., Dohra, H., and Sasanami, T. (2020). Expression of transferrin and albumin in the sperm-storage Tubules of Japanese quail and their possible involvement in long-term sperm storage. J. Poult. Sci. 57, 88–96. doi: 10.2141/jpsa.0190049
Matsuzaki, M., Mizushima, S., Hiyama, G., Hirohashi, N., Shiba, K., Inaba, K., et al. (2015). Lactic acid is a sperm motility inactivation factor in the sperm storage tubules. Sci. Rep. 1:17643. doi: 10.1038/srep17643
Mazerolle, M. (2006). Improving data analysis in herpetology: Using Akaike’s Information Criterion (AIC) to assess the strength of biological hypotheses. Amphib. Reptilia. 27, 169–180. doi: 10.1163/156853806777239922
Monaghan, P., Charmantier, A., Nussey, D., and Ricklefs, R. (2008). The evolutionary ecology of senescence. Funct. Ecol. 22, 371–378. doi: 10.1111/j.1365-2435.2008.01418.x
Monnier-Corbel, A., Monnet, A. C., Hingrat, Y., and Robert, A. (2022). Patterns of abundance reveal evidence of translocation and climate effects on Houbara bustard population recovery. Anim. Conserv. 25, 297–310. doi: 10.1111/acv.12738
Munro, S. S. (1938). Functional changes in fowl sperm during their passage through the excurrent ducts of the male. J. Exp. Zool. 79, 71–92. doi: 10.1002/jez.1400790106
Nalbandov, A., and Card, L. E. (1943). Effect of stale sperm on fertility and hatchability of chicken eggs. Poultry. Sci. 22, 218–226. doi: 10.3382/ps.0220218
Noguera, J. C., Dean, R., Isaksson, C., Velando, A., and Pizzari, T. (2012). Age-specific oxidative status and the expression of pre- and postcopulatory sexually selected traits in male red junglefowl, Gallus gallus. Ecol. Evol. 2, 2155–2167. doi: 10.1002/ece3.300
Nussey, D. H., Froy, H., Lemaitre, J.-F., Gaillard, J.-M., and Austad, S. N. (2013). Senescence in natural populations of animals: Widespread evidence and its implications for bio-gerontology. Ageing Res. Rev. 12, 214–225. doi: 10.1016/j.arr.2012.07.004
Okada, F. K., Andretta, R. R., and Spaine, D. M. (2020). One day is better than four days of ejaculatory abstinence for sperm function. Reprod. Fert. 1, 1–10. doi: 10.1530/RAF-20-0018
Pinheiro, J., Bates, D., DebRoy, S., Sarkar, D., and R Core Team (2021). nlme: linear and nonlinear mixed effects models. Available online at: https://cran.r-project.org/package=nlme (accessed January 10, 2021).
Pizzari, T., Dean, R., Pacey, A., Moore, H., and Bonsall, M. B. (2008). The evolutionary ecology of pre- and post-meiotic sperm senescence. Trends Ecol. Evol. 23, 131–140. doi: 10.1016/j.tree.2007.12.003
Preston, B. T., Jalme, M. S., Hingrat, Y., Lacroix, F., and Sorci, G. (2011). Sexually extravagant males age more rapidly: Sexually extravagant males age rapidly. Ecol. Lett. 14, 1017–1024. doi: 10.1111/j.1461-0248.2011.01668.x
Preston, B. T., Saint Jalme, M., Hingrat, Y., Lacroix, F., and Sorci, G. (2015). The sperm of aging male bustards retards their offspring’s development. Nat. Commun. 6:6146. doi: 10.1038/ncomms7146
Quay, W. B. (1985). Cloacal sperm in spring migrants: occurrence and interpretation. Condor 87:273. doi: 10.2307/1366894
Reinhardt, K. (2007). Evolutionary consequences of sperm cell aging. Q. Rev. Biol. 82, 375–393. doi: 10.1086/522811
Ruhmann, H., Koppik, M., Wolfner, M. F., and Fricke, C. (2018). The impact of ageing on male reproductive success in Drosophila melanogaster. Exp. Gerontol. 103, 1–10. doi: 10.1016/j.exger.2017.12.013
Saint Jalme, M. S., Gaucher, P., and Paillat, P. (1994). Artificial insemination in Houbara bustards (Chlamydotis undulata): Influence of the number of spermatozoa and insemination frequency on fertility and ability to hatch. J. Reprod. Fertil. 100, 93–103. doi: 10.1530/jrf.0.1000093
Sartorius, G. A., and Nieschlag, E. (2010). Paternal age and reproduction. Hum. Reprod. Update 16, 65–79. doi: 10.1093/humupd/dmp027
Sasanami, T., Matsuzaki, M., Mizushima, S., and Hiyama, G. (2013). Sperm storage in the female reproductive tract in birds. J. Reprod. Dev. 59, 334–338. doi: 10.1262/jrd.2013-038
Słowińska, M., Nynca, J., Arnold, G., Fröhlich, T., Jankowski, J., Kozłowski, K., et al. (2017). Proteomic identification of turkey (Meleagris gallopavo) seminal plasma proteins. Poult. Sci. 96, 3422–3435. doi: 10.3382/ps/pex132
Tarin, J. J. (2000). Consequences on offspring of abnormal function in ageing gametes. Hum. Reprod. Update 6, 532–549. doi: 10.1093/humupd/6.6.532
Therneau, T. (2012). coxme: mixed effects Cox models. R package version 2.2-3. Vienna: R Foundation for Statistical Computing.
Thomas, G. W. C., Wang, R. J., Puri, A., Harris, R. A., Raveendran, M., Hughes, D. S. T., et al. (2018). Reproductive longevity predicts mutation rates in primates. Curr. Biol. 28, 3193–3197.e5. doi: 10.1016/j.cub.2018.08.050
Tingari, M. D., and Lake, P. E. (1972). Ultrastructural evidence for resorption of spermatozoa and testicular fluid in the excurrent ducts of the testis of the domestic fowl, gallus domesticus. J. Reprod. Fertil. 31, 373–381. doi: 10.1530/jrf.0.0310373
Vega-Trejo, R., Fox, R. J., Iglesias-Carrasco, M., Head, M. L., and Jennions, M. D. (2019). The effects of male age, sperm age and mating history on ejaculate senescence. Funct. Ecol. 33, 1267–1279. doi: 10.1111/1365-2435.13305
Vuarin, P., Bouchard, A., Lesobre, L., Levêque, G., Chalah, T., Jalme, M. S., et al. (2019a). Post-copulatory sexual selection allows females to alleviate the fitness costs incurred when mating with senescing males. Proc. R. Soc. B. 286:20191675. doi: 10.1098/rspb.2019.1675
Vuarin, P., Hingrat, Y., Lesobre, L., Jalme, M. S., Lacroix, F., and Sorci, G. (2019b). Sperm competition accentuates selection on ejaculate attributes. Biol. Lett. 15:20180889. doi: 10.1098/rsbl.2018.0889
Vuarin, P., Lesobre, L., Levêque, G., Saint Jalme, M., Lacroix, F., Hingrat, Y., et al. (2021). Paternal age negatively affects sperm production of the progeny. Ecol. Lett. 24, 719–727. doi: 10.1111/ele.13696
White, J., Wagner, R. H., Helfenstein, F., Hatch, S. A., Mulard, H., Naves, L. C., et al. (2008). Multiple deleterious effects of experimentally aged sperm in a monogamous bird. Proc. Natl. Acad. Sci. U.S.A. 105, 13947–13952. doi: 10.1073/pnas.0803067105
Wolf, K. N., Wildt, D. E., Vargas, A., Marinari, P. E., Kreeger, J. S., Ottinger, M. A., et al. (2000). Age-dependent changes in sperm production, semen quality, and testicular volume in the Black-footed ferret (Mustela nigripes)1. Biol. Reprod. 63, 179–187. doi: 10.1095/biolreprod63.1.179
Xing, K., Chen, Y., Wang, L., Lv, X., Li, Z., Qi, X., et al. (2022). Epididymal mRNA and miRNA transcriptome analyses reveal important genes and miRNAs related to sperm motility in roosters. Poult. Sci. 101:101558. doi: 10.1016/j.psj.2021.101558
Zhao, H., Joseph, J., Fales, H. M., Sokoloski, E. A., Levine, R. L., Vasquez-Vivar, J., et al. (2005). Detection and characterization of the product of hydroethidine and intracellular superoxide by HPLC and limitations of fluorescence. Proc. Natl. Acad. Sci. U.S.A. 102, 5727–5732. doi: 10.1073/pnas.0501719102
Keywords: aging, chick survival, reproductive success, sperm age, sperm quality
Citation: Meunier L, Sorci G, Abi Hussein H, Hingrat Y, Rehspringer N, Saint-Jalme M, Lesobre L and Torres Carreira J (2022) Pre-but not post-meiotic senescence affects sperm quality and reproductive success in the North African houbara bustard. Front. Ecol. Evol. 10:977184. doi: 10.3389/fevo.2022.977184
Received: 24 June 2022; Accepted: 21 November 2022;
Published: 08 December 2022.
Edited by:
Jean-Francois Lemaitre, UMR 5558 Biométrie et Biologie Evolutive (LBBE), FranceReviewed by:
Teri Orr, New Mexico State University, United StatesJosé E. A. R. Marian, University of São Paulo, Brazil
Copyright © 2022 Meunier, Sorci, Abi Hussein, Hingrat, Rehspringer, Saint-Jalme, Lesobre and Torres Carreira. This is an open-access article distributed under the terms of the Creative Commons Attribution License (CC BY). The use, distribution or reproduction in other forums is permitted, provided the original author(s) and the copyright owner(s) are credited and that the original publication in this journal is cited, in accordance with accepted academic practice. No use, distribution or reproduction is permitted which does not comply with these terms.
*Correspondence: Janaina Torres Carreira, anRjYXJyZWlyYUByZW5lY28ub3Jn