- 1Key Laboratory of Geographical Processes and Ecological Security in Changbai Mountains, Ministry of Education, School of Geographical Sciences, Northeast Normal University, Changchun, China
- 2State Environmental Protection Key Laboratory of Wetland Ecology and Vegetation Restoration, Institute for Peat and Mire Research, Northeast Normal University, Changchun, China
- 3School of Geographic Sciences, Hunan Normal University, Changsha, China
- 4Center for Ecological Forecasting and Global Change, College of Forestry, Northwest A&F University, Yangling, China
- 5Jilin Provincial Key Laboratory for Wetland Ecological Processes and Environmental Change in the Changbai Mountains, Changchun, China
- 6Department of Biology Sciences, Institute of Environment Sciences, University of Quebec at Montreal, Montreal, QC, Canada
Atmospheric deposition of nitrogen (N) and phosphorus (P) far exceeding the pre-industrial levels have the potential to change carbon (C) dynamics in northern peatlands. However, the responses of soil C concentration and organo-chemical composition to different rates and durations of nutrient enrichment are still unclear. Here, we compared the short- (3 years) and long-term (10 years) effects of N and P fertilizations on the physicochemical properties of peat and porewater in a bog-fen complex in northern China. Our results showed that the short-term fertilization increased Sphagnum moss cover, while the expansion of vascular plants was observed owing to the long-term fertilization. The preserved soil C did not vary considerably after the short- and long-term fertilizations. The harsh soil conditions may impede the decomposition of organic matters by soil microorganisms during the short-term fertilization. For the long-term fertilization, the input of high-phenolic litters owing to vascular plant expansion likely exerted an important control on soil C dynamics. These processes constrained the variation in soil C concentrations when the addition rate and cumulative amount of external N and P increased, which will advance our understanding and prediction of the resilience of soil C storage to imbalanced nutrient enrichment of N and P in northern peatlands.
Introduction
Peatlands are important carbon (C) sinks which store 500–700 Gt of soil organic C, but only comprise about 3% of the land surface (Gorham, 1991; Yu et al., 2010). These massive C sequestrations result from the imbalance between production and decomposition under waterlogged, acid and nutrient-deficient conditions (Schothorst, 1977; Rydin and Jeglum, 2013). Nutrient deposition may have unforeseen effects on global peatlands, for example increase soil C release, leading to a catastrophic impact on future climate (Dash et al., 2020; Wang et al., 2021). Unfortunately, significant increase in atmospheric deposition of reactive nitrogen (N) and phosphorus (P) have been observed due to fertilization, mining, and fossil fuel combustion in recent decades (Rice and Herman, 2012; Tipping et al., 2014; Ackerman et al., 2019). Furthermore, considering the possibly faster increase of N input than P input, the imbalance of N and P enrichment was expected to deteriorate (Peñuelas et al., 2013), which may change plant community composition and present a substantial threat to soil C stock (Moore et al., 2019; Schlesinger and Bernhardt, 2020).
Numerous nutrient manipulation experiments have been established in global peatlands to gain insight into the effects of nutrient enrichment on soil C decomposition, but these studies usually produced different or even contrasting results (e.g., Bubier et al., 2007; Li et al., 2019; Moore et al., 2019). These discrepancies might result from various biotic controls associated with shift in plant community compositions on soil C mineralization. For example, long-term fertilization might stimulate vascular plant growth at the expense of moss (Moore et al., 2019; Schlesinger and Bernhardt, 2020), and this change may have complex effects on preserved soil C.
As for the negative effect, the expansion of vascular plants, especially by the extended root network, would raise peat surface, and further contribute to the hummock-hollow microtopography to ameliorate waterlogged stress (Moore et al., 2002; Schlesinger and Bernhardt, 2020). Subsequently, this may further enhance ecosystem respiration and change nutrient storage and enzyme activity (Larmola et al., 2013; Li et al., 2020). This vascular plant-mediated effect could be reflected not only in peat quality, but also in porewater chemistry (Moore et al., 2019). For example, the concentrations of dissolved N and P in porewater were positively related to proteins or protein precursors exuded by plant roots and microorganisms but negatively related to the “capacity of the ‘filter”’ function of Sphagnum moss (Limpens et al., 2004; Chiwa et al., 2016; Moore et al., 2019).
As for the positive effect, a series of cascading effects caused by increased vascular plant growth might retard organic matter decomposition and obscure microbial response to nutrient inputs (Li et al., 2019; Fenner and Freeman, 2020; Wang et al., 2021). Microbial decomposers might be much less responsive to the enrichment of external nutrients in shrub-dominated peatlands (e.g., Wang et al., 2015; Fenner and Freeman, 2020), because the improved litter quality and increased tannin production, associated with shift in the dominance of vascular plants, could effectively bind protein and inhibit enzyme activity by oxidative degradation and biotoxicity (Freeman et al., 2001; Wang et al., 2015). This was supported by a long-term decomposition experiment conducted by Fenner and Freeman (2020), in which the water-soluble phenolics leached from wood trunks inhibited both the extracellular and intracellular metabolism and lowered nutrient mobilization. Besides, vascular plants could strongly compete with soil microorganisms for available nutrients through extended root and releasing allelopathic compounds (van Breemen, 1995; Schlesinger and Bernhardt, 2020). In both cases, because the activities of microbial decomposers were inhibited by protein-binding phenolic compounds from the litters of vascular plants, the expansion of vascular plants may eventually counteract the negative effects of nutrient enrichment on soil C stock (Freeman et al., 2001; Wang et al., 2015; Fenner and Freeman, 2020). The above-mentioned confounding effects make it difficult for the results of current studies to reach a consensus (Rousk et al., 2013; Wang et al., 2015). Therefore, the interactive effect of N and P enrichment on soil C decomposition is still under-investigated, especially in northern peatlands where to the shift in plant community composition occurs under cumulative nutrient fertilization.
The absorption bands of Fourier transform infrared (FTIR) spectroscopy can indicate the composition of major peat chemical moieties, such as polysaccharides, lignin, phenolics, and carboxylates (Biester et al., 2014; Moore et al., 2019; Drollinger et al., 2020). With regard to the peak intensity ratios, the relative contributions of refractory organic compounds increased compared to labile polysaccharides can be identified as an increase in the degree of organic matter decomposition, considering that the liberated monomers from carbohydrates are easily assimilated by microorganisms or oxidized to generate metabolic energy (Niemeyer et al., 1992; Cocozza et al., 2003; Broder et al., 2012). In this study, we evaluated whether and how the interrelated chemical properties of peat and porewater and plant community composition were changed by N and P enrichment that ultimately affected C storage under short- (3 years) and long-term fertilization (10 years) at Hani peatland, northeastern China. Specifically, we quantified the total concentrations of C, N, P, and metals in peat, and the concentrations of dissolved C, N, and P in porewater, as well as four humification indices of surface peat via FTIR spectroscopic analysis. We intended to test the following two hypotheses: (1) in Sphagnum moss-dominated plots, the strong changes in chemical moieties in peat and/or porewater due to short-term fertilization could threaten the reservoir of soil organic C and (2) the increased phenolics input due to the loss of Sphagnum and the expansion of vascular plants would protect soil C stock during long-term N and P additions.
Materials and methods
Study site and fertilization experiments
This study was conducted at Hani peatland (42°13′N, 126°31′E) in Changbai Mountains, northeastern China. Hani peatland is a bog-fen complex with a mean annual precipitation of 757–930 mm and a mean annual temperature of 2.5–3.6°C (Bu et al., 2011). The average peat depth in the area is 4 m with a maximum depth of 9.6 m (Qiao, 1993; Bu et al., 2011). The short- (since 2018) and long-term (since 2007) N and P fertilization experiments were both established on large hummocks in the open bog region of Hani peatland. A factorial design was used with three levels of N addition (0, 5, and 10 g N m–2 year–1; i.e., 0N, 5N, 10N) and in combination with P addition (0, 0.5, and 10 g P m–2 year–1; i.e., 0P, 0.5P, 1P). There were four replicates for each treatment combination, and the size of fertilized plot was 1.2 m × 1.2 m for the short-term experiment and 0.8 m × 0.8 m for the long-term experiment. In total, there were 72 plots (9 treatment combinations × 4 replicates × 2 fertilization experiments). Nitrogen and P was applied as ammonium nitrate (NH4NO3) and sodium dihydrogen phosphate (NaH2PO4⋅2H2O), respectively, and the control plots were supplied with deionized water. The dominant shrubs are Betyla ovalifolia Rupr., Rhododendron parvifolium Adams, and Potentilla fruticose Linn., and the dominant herbaceous plants are Carex lasiocarpa Ehrh., Eriophorum polystachion L., and Smilacina japonica A. Gray. The underlying moss layer is dominated by Sphagnum palustre Linn. and Polytrichum commune Linn. ex Hedw.
Vegetation measurements
We used point intercept method to determine the abundances of Sphagnum moss and vascular plants in the fertilized plots (Larmola et al., 2013). Briefly, a customized stain-steel frame (60 cm × 60 cm) with 61 grid points was used for the measurement. The number of times (i.e., “hits”) were recorded when the leaves of vascular plants at different height or Sphagnum capitula on the ground contacted a carbon fiber rod (2 mm in diameter) over the grid points. Sphagnum moss abundance (i.e., cover) was calculated by the total number of hits divided by 61. Vascular plant abundance (not identical to plant cover) was approximated by the total number of hits per unit area (i.e., hits m–2).
Physicochemical properties of peat and porewater
Peat samples (20 g of fresh peat) at 0–5 cm from the long-term and short-term fertilized plots were collected in August 2017 and 2020, respectively. Living mosses were removed and the remaining peat samples were stored at 4°C prior to analyses within seven days. A subset of fresh peat samples was dried at 65°C and ground with a ball mill (MM400, Retsch GmbH, Haan, Germany). Total carbon (TC) and total nitrogen (TN) concentrations were measured on an elemental analyzer (vario MACRO cube, elementar, Germany). The remaining samples of the ground peat were digested in concentrated sulfuric acid and hydrogen peroxide with selenium and lithium sulfate as catalysts (Parkinson and Allen, 2008). Total phosphorus (TP) concentration of samples from the long-term fertilized plots was determined colorimetrically using the ammonium molybdate-ascorbic acid method (Murphy and Riley, 1962) on a continuous flow analyzer (San + +, Skalar Analytical B.V., Breda, Netherlands). Total P concentration of samples from the short-term fertilized plots was determined by the malachite green method against calibration standard (Ohno and Zibilske, 1991) on a full-wavelength microplate reader (Multiskan GO, Thermo Fisher, Finland). Potassium (K), calcium (Ca), and magnesium (Mg) concentrations were measured on an atomic absorption spectrophotometer (AA-7003A, EWAI company, China), with the calibration standards of potassium chloride, calcium carbonate, and magnesium sheet, respectively. The data of TC, TN, and TP concentrations in the long-term fertilized plots were derived from our previous study (Li et al., 2019).
In August 2020, we collected porewater samples (∼30 mL) from the short- and long-term fertilized plots with MacroRhizons tubes (Rhizosphere Research Products B.V., Wageningen, Netherlands). Concentration of dissolved organic carbon (DOC) was measured on a TOC analyzer (TOC-L, SHIMADZU, Japan). The concentrations of NH4+-N, NO3–-N, and PO43– [i.e., dissolved inorganic phosphorus (DIP)] in porewater were measured on a continuous flow analyzer (San + +, Skalar Analytical B.V., Breda, Netherlands). The concentration of dissolved inorganic nitrogen (DIN) was calculated as the sum of NH4+-N and NO3–-N concentrations. The remaining porewater samples were then digested with potassium persulfate at 120°C for 1 h to quantify the total dissolved N and P concentrations (TDN and TDP). The concentration of dissolved organic nitrogen (DON) was calculated as the difference between the concentrations of TDN and DIN. Similarly, the concentration of dissolved organic phosphorus (DOP) was calculated as the difference between the concentrations of TDP and DIP.
Organic chemistry analysis
The organo-chemical composition of dried peat samples was measured on a FTIR spectrometer (Vetex70, BRUKER, Germany) with absorption mode. Specifically, 2 mg of ground and oven-dried peat sample was mixed with 200 mg of potassium bromide, and then pressed to translucent pellets. The range of the wavenumber was 4,000 to 400 cm–1 with a resolution of 2 cm–1 and 16 scans per sample were collected. The background of potassium bromide was removed from the spectra, and then atmospheric compensation and baseline correction was performed (see section “statistical analyses”). To determine the degree of humification of peat samples, spectra peaks around the following wavenumbers were recorded: 1,090 cm–1 (polysaccharide), 1,420 cm–1 (carboxylates and humic acids), 1,510 cm–1 (lignin and phenolics), 1,630 cm–1 (mainly aromatics), and 1,720 cm–1 (carboxylates–acids) (Niemeyer et al., 1992; Moore et al., 2019; Drollinger et al., 2020). Humification indices were calculated as the ratios of the absorption at corresponding wavenumbers, i.e., 1,420/1,090, 1,510/1,090, 1,630/1,090, and 1,720/1,090 (Niemeyer et al., 1992; Cocozza et al., 2003; Broder et al., 2012). The decreases in these humification indices were considered to reflect the enhanced degree of organic matter decomposition (Niemeyer et al., 1992; Broder et al., 2012).
Statistical analyses
Statistical analyses were performed using R 4.0.4 (R Core Team, 2021). Data normality and the homogeneity of variance were examined with Shapiro–Wilk tests and Levene’s tests, respectively. Two-way ANOVAs were used to test the fertilization effect on plant abundance, physicochemical properties, and humification indices using the addition rates of N, P, and their combination as fixed factors for the short- and long-term fertilization experiments separately. Post hoc multiple comparisons were conducted using Tukey’s test at the significance level of P < 0.05. Two-sample t-test was used to compare the results of plant abundance, physicochemical properties, and humification indices between the short- and long-term fertilization under the same N or P addition rate. The ir (Teickner, 2022) and irpeat (Teickner and Hodgkins, 2022) packages in R were used to process the spectra of FTIR, including baseline correction, normalization, and calculation of humification indices. Spearman’s correlation analysis was conducted to detect the relationships between plant species abundance and physicochemical properties of peat and porewater. Hierarchical partitioning was employed to assess the contribution of physicochemical properties of peat and porewater on organic matter decomposition as indicated by four humification indices using the rdacca.hp package in R (Lai et al., 2022).
Result
Peat and porewater chemistry
We did not observe any significant change in TC concentration in peat under neither fertilization experiment (Table 1). For the short-term fertilization, without significant N by P interaction, N and P additions significantly increased TN and TP concentrations, respectively. However, for the long-term fertilization, the effect of N and P interaction on nutrient concentrations was significant. Specifically, under the same rate of P addition, N addition increased TN (P < 0.001) and TP concentrations (P = 0.034); under the same rate of N addition, P addition increased TP concentration (Table 1). In combination with the highest rate of N addition (i.e., 10 g N m–2 year–1), P addition ultimately increased TN concentration by 54% (P < 0.001). Short-term fertilization had little effect on K, Ca, and Mg concentrations, while long-term N addition significantly decreased K concentration (P < 0.001; Table 1). Compared to the short-term fertilization, long-term N and/or P additions significantly decreased Ca and Mg concentrations.
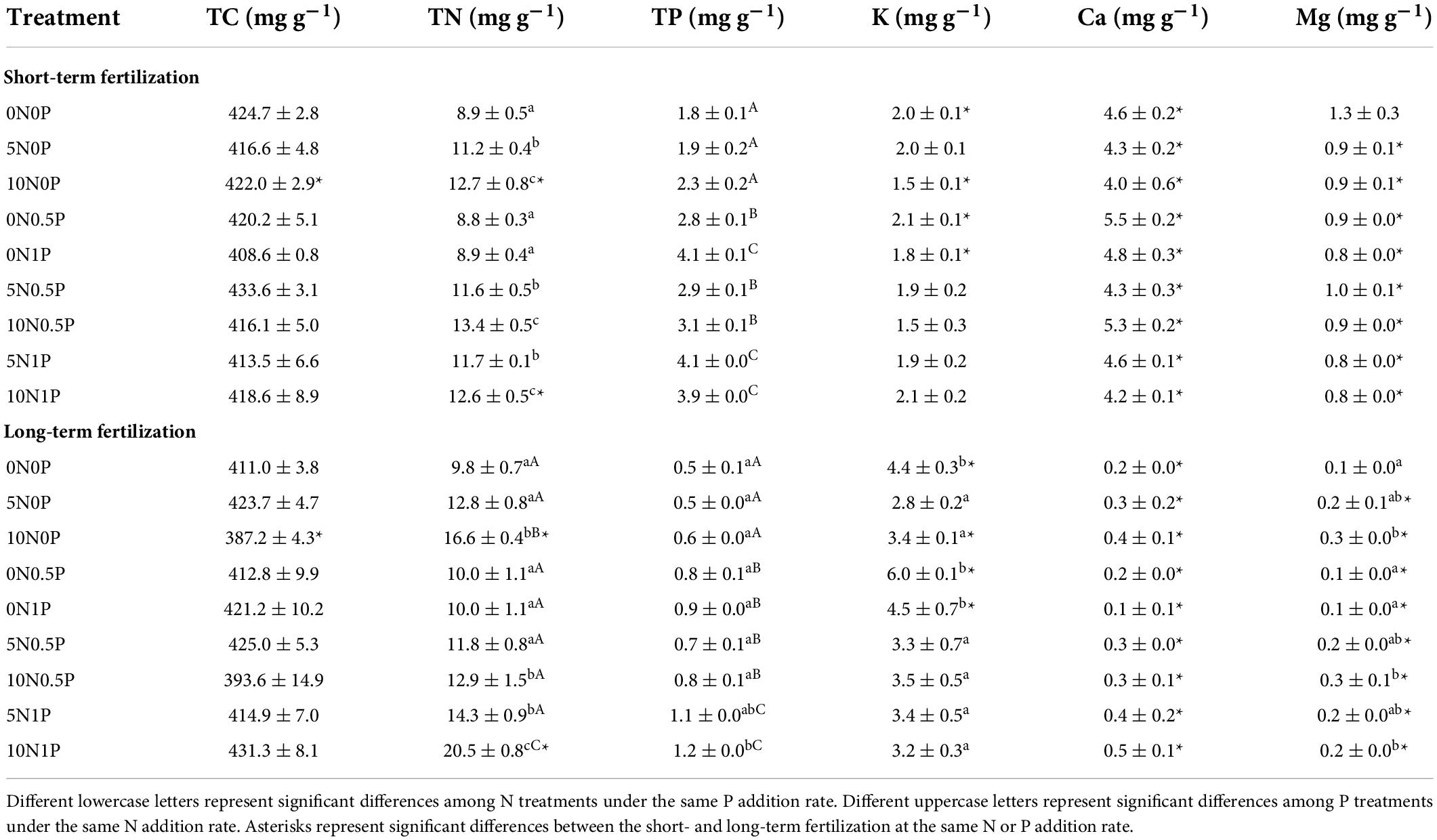
Table 1. Peat chemical properties under different treatments in the short- and long-term fertilizations.
As for porewater chemistry, the concentrations of dissolved nutrients only showed weak responses to N and/or P additions in both experiments (Table 2). Specifically, the short- or long-term fertilization did not show significant effect on DOC concentration (Table 2). Nitrogen addition significantly increased the concentrations of NH4+-N (short-term, P = 0.017; long-term, P < 0.001) and DON (short-term, P = 0.045; long-term, P = 0.038; Table 2). In particular, the long-term N addition significantly increased DON concentration compared to the short-term fertilization. We did not observe significant change in dissolved P concentrations (i.e., DIP and DOP) in neither experiment (Table 2).
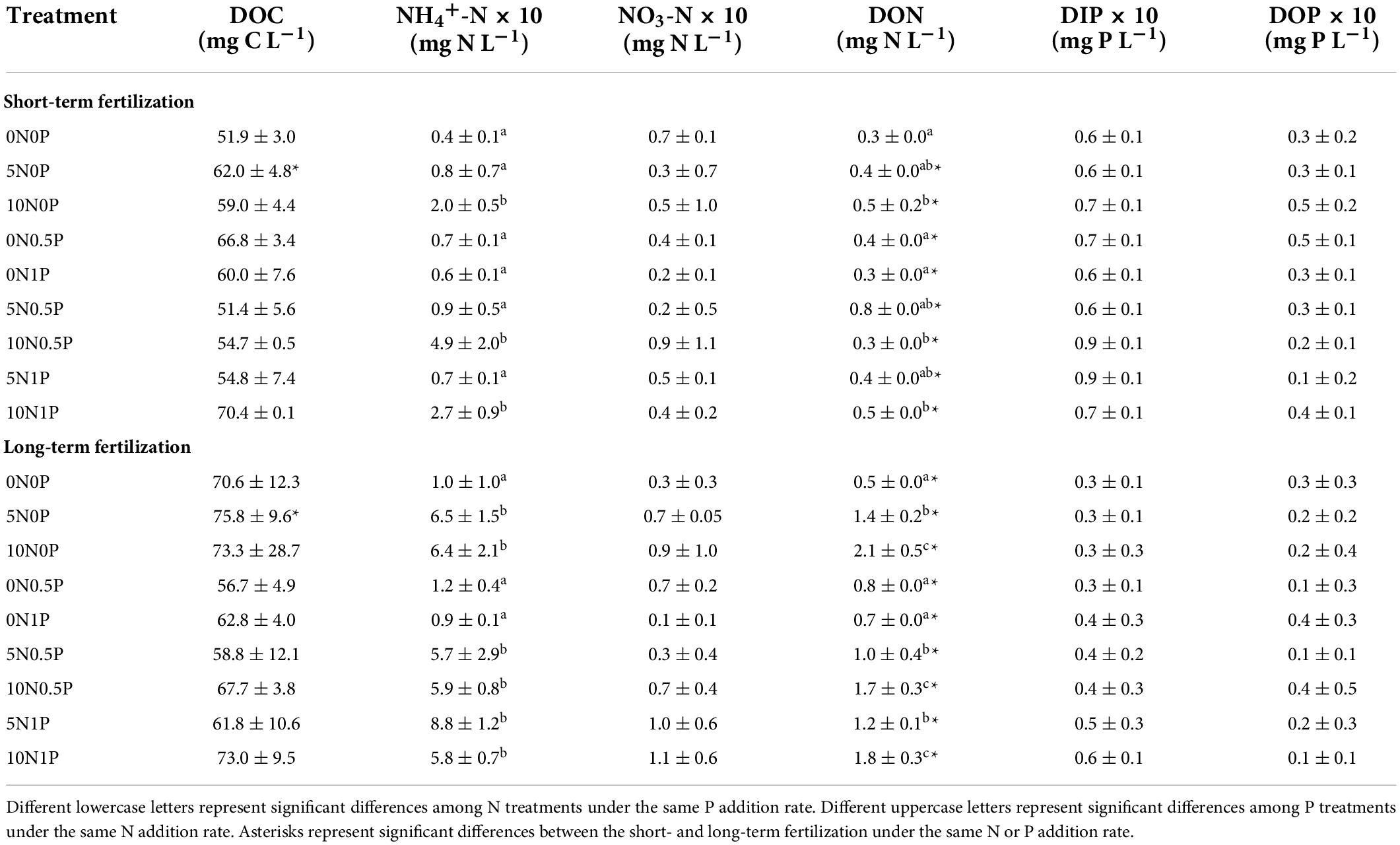
Table 2. Porewater chemical properties under different treatments in the short- and long-term fertilizations.
Variation in plant abundance
For the short-term fertilization, the abundance of Sphagnum moss was increased by 25–70% compared to the control (i.e., 0N0P treatment; Figure 1A). The total abundance of vascular plants was substantially reduced by N-only addition and the combination of N and P additions (Figure 1B). Strong and positive correlation between Sphagnum moss abundance and TP concentration was observed after short-term fertilization (Spearman’s ρ = 0.55, P < 0.001; Figure 2A). For the long-term fertilization, the abundance of Sphagnum moss was decreased by 21–55%, while the abundance of vascular plants was increased by 0.4–28% (Figures 1C, D). Results of Spearman’s correlation analysis indicated that the abundance of vascular plants was significantly and negatively correlated with Sphagnum moss abundance (ρ = −0.39, P = 0.013), and significantly and positively correlated with TP concentration under the long-term fertilization (ρ = 0.48, P = 0.014; Figure 2C).
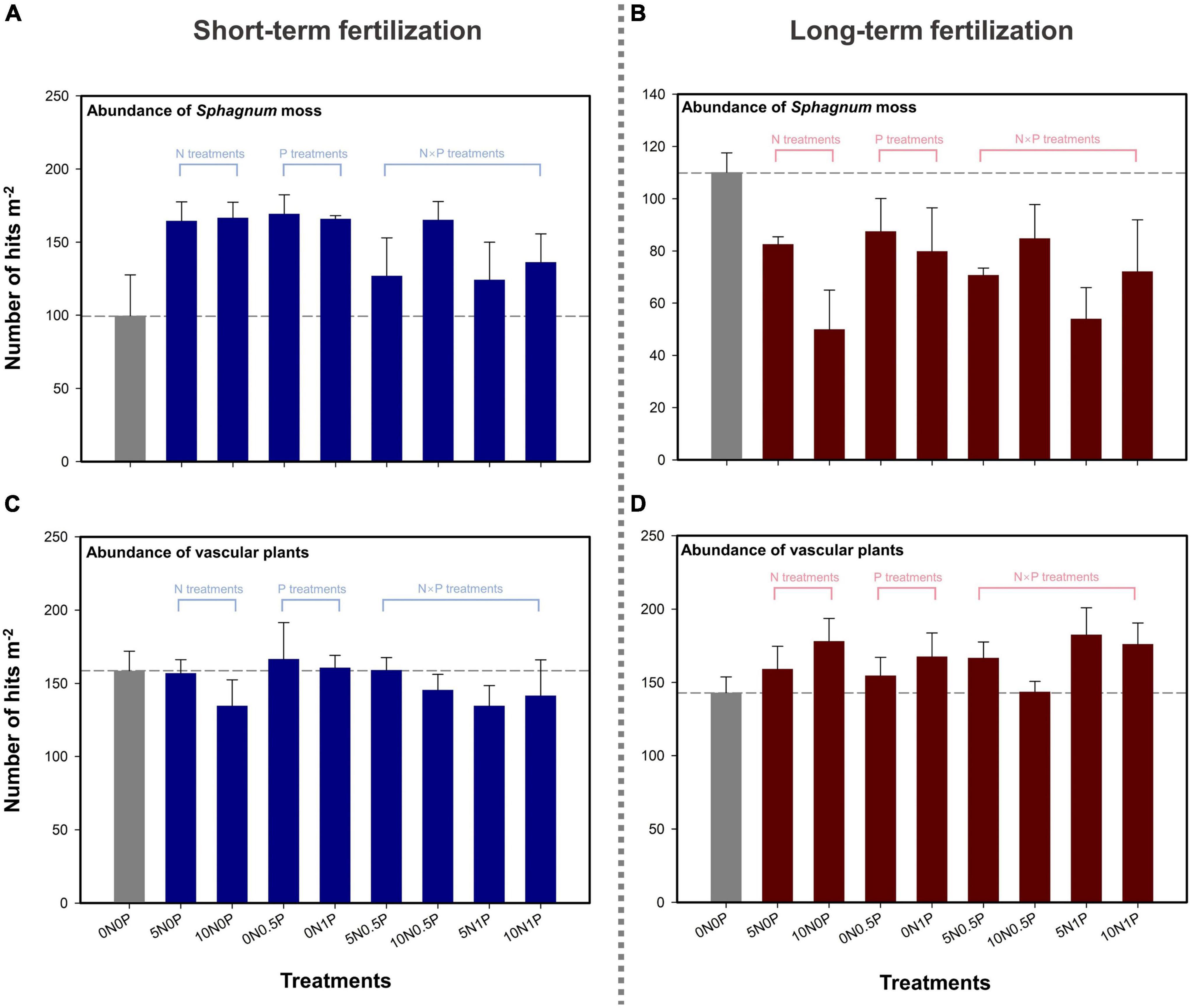
Figure 1. Abundance of Sphagnum moss (A,C) and vascular plants (B,D) in response to the short- and long-term fertilizations.
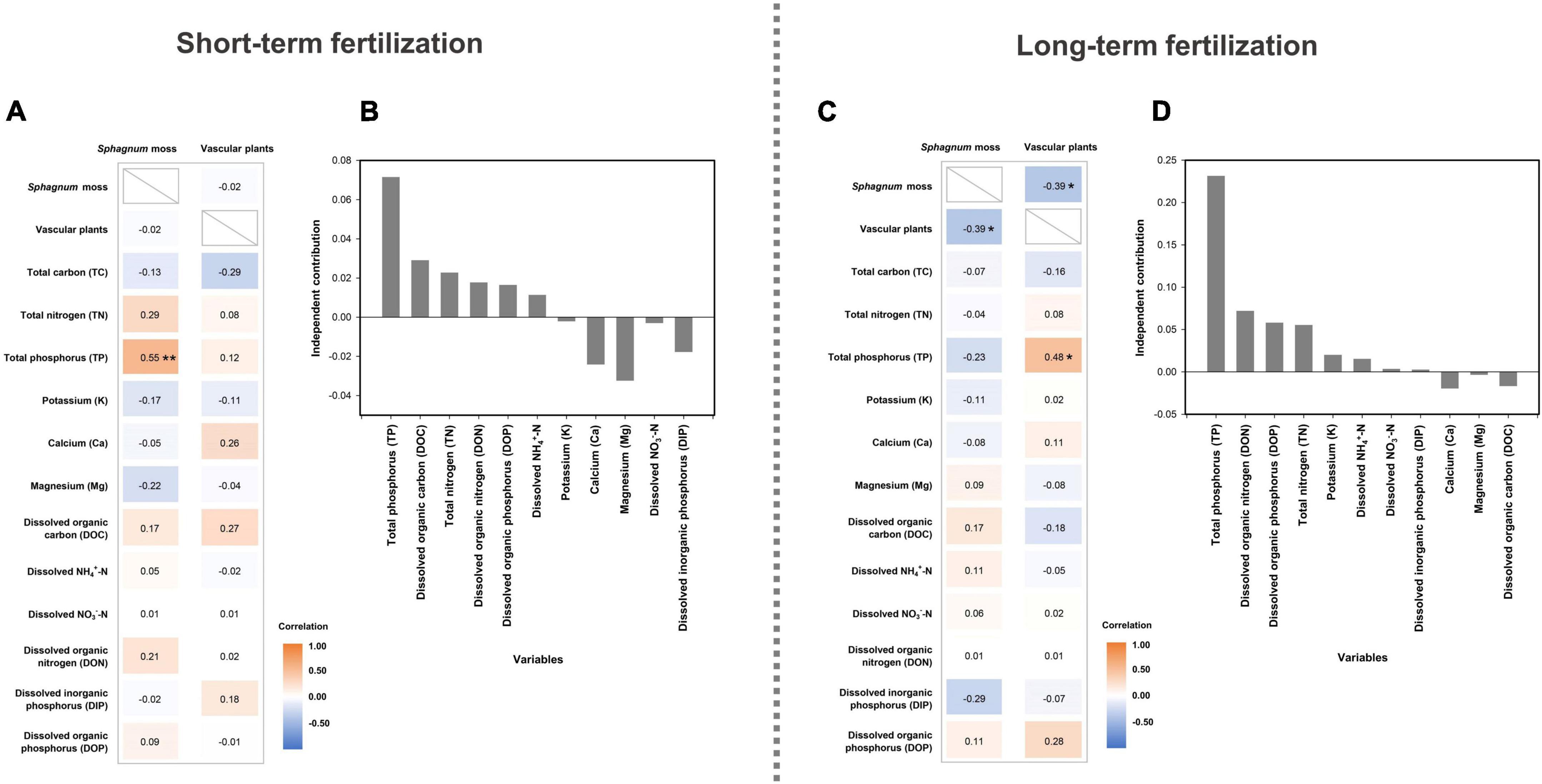
Figure 2. Spearman’s correlations between plant species abundance and physicochemical properties of peat and porewater under the short- (A) and long-term fertilizations (C), and the hierarchical partitioning between physicochemical properties of peat and porewater and the humification indices under the short- (B) and long-term fertilizations (D). Asterisks represent significant correlations.
Humification indices and the contributions of physicochemical properties
The additions of N and P did not remarkably increase the degree decomposition of organic matter as indicated by the humification indices (Figure 3). For the short-term fertilization, N and P additions weakly increased the absorbances of refractory components of the organic compounds (i.e., carboxylates and humic acids at 1,420 cm–1; lignin and phenolics at 1,510 cm–1; aromatics at 1,630 cm–1; carboxylates–acids at 1,720 cm–1) and labile polysaccharide moieties (1,090 cm–1; Figure 3A). The humification indices did not change significantly after N and/or P additions (Figures 3B–E). For the long-term fertilization, N and/or P additions, especially the combination of N and P additions, increased the absorbances of refractory components of organic compounds relative to the control (Figure 3F). Two of the humification indices (i.e., 1,510/1,090 and 1,630/1,090) were significantly increased in the long-term fertilized plots (Figures 3H,I). Specifically, N addition increased the ratio of 1,510/1,090 under the same rate of P addition, and this ratio also increased with P addition under the same rate of N addition (Figure 3H). However, the effect of N addition on the ratio of 1,630/1,090 depended on P addition rates (i.e., interactive effect). That is, the ratio of 1,630/1,090 was significantly increased by N addition only under the largest rate of P addition (i.e., 1 g P m–2 year–1). In combination with 5N treatment, the largest rate of P addition significantly increased the ratio of 1,630/1,090 (Figure 3I).
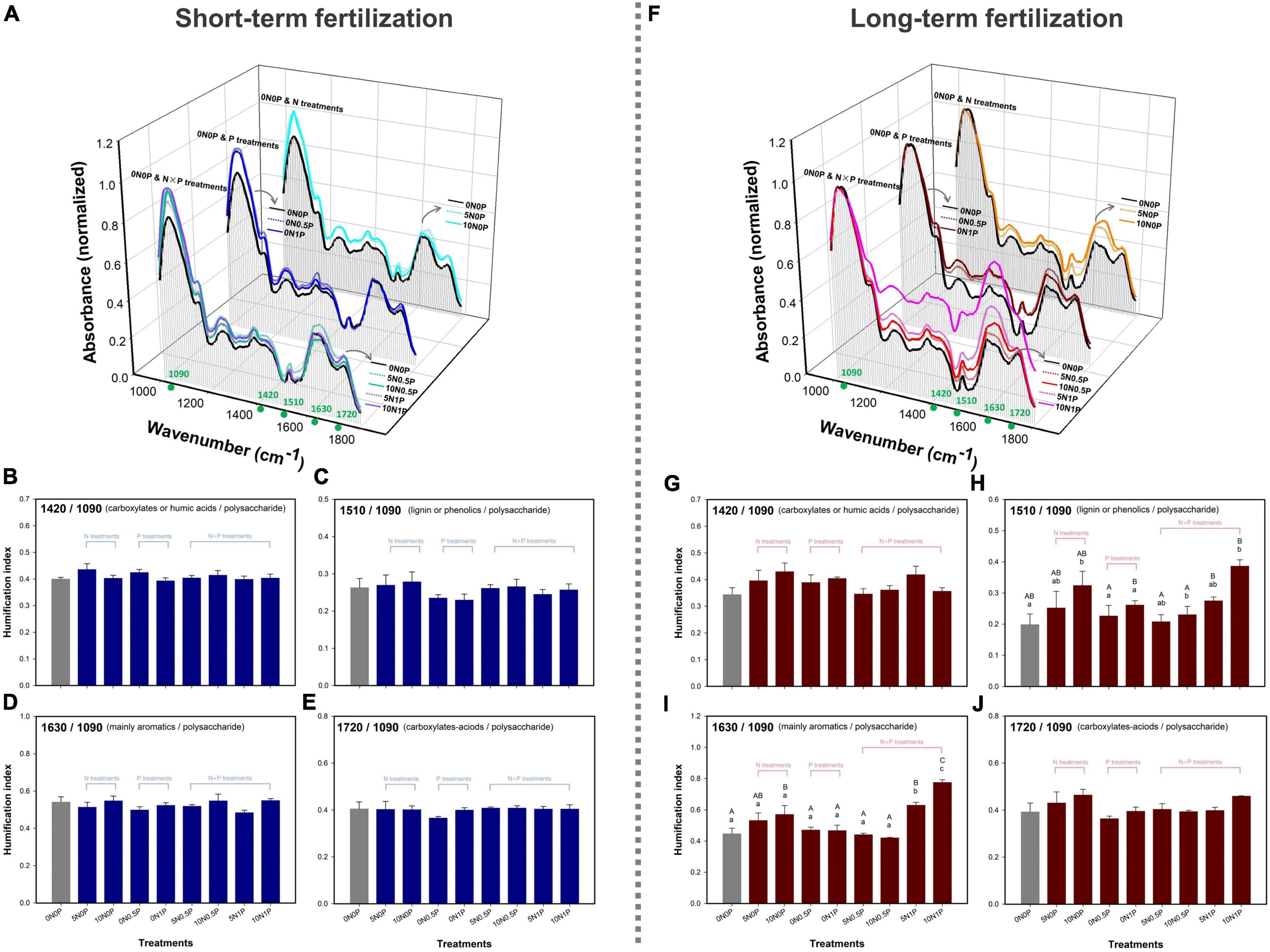
Figure 3. Fourier-transform infrared (FTIR) spectra under the short- (A) and long-term fertilizations (F), and the humification indices under the short- (B–E) and long-term fertilizations (G–J). Different lowercase letters represent significant differences among N treatments under the same P addition rate in the long-term fertilization. Different uppercase letters represent significant differences among P treatments under the same N addition rate in the long-term fertilization.
Results of hierarchical partitioning analysis indicated that TP concentration was the most important factor influencing the variations in humification indices both under the short- and long-term fertilizations (Figures 2B,D). For the short-term fertilization, the full model R2 of environmental variables was 0.09, indicating that peat and porewater chemical properties only had weak relationships with humification indices (Figure 2B). Specifically, TP concentration explained the largest proportion of variations in humification indices (R2 = 0.07), followed by DOC (R2 = 0.03) and TN concentrations (R2 = 0.02). For the long-term fertilization, the full model R2 of environmental variables was 0.42. TP concentration was the most important factor in explaining the variations in humification indices (R2 = 0.23), followed by DON (R2 = 0.07) and DOP concentrations (R2 = 0.06; Figure 2D).
Discussion
Changes in plant abundance and environmental variables
We found divergent results with regard to the responses of plant abundance to the short- and long-term fertilizations (Figure 1). During the short-term fertilization, the abundance of Sphagnum moss was increased by 25–70% after N and/or P additions (Figure 1A). It is not surprising to observe a positive effect of inorganic N and/or P inputs on the growth of Sphagnum moss at a low cumulative amount, considering that plant growth in peatlands is often constrained by limited N and/or P availabilities (Leon et al., 2019; Dash et al., 2020). This increase of Sphagnum moss abundance under short-term fertilization has been observed in many previous studies (e.g., Limpens et al., 2004; Bubier et al., 2007; Leon et al., 2019). Meanwhile, the positive correlations between Sphagnum moss abundance and TN and TP concentrations (Figure 2A) may indicate N and P co-limitation as observed in other peatlands (e.g., Cusell et al., 2014; Wang et al., 2016).
Long-term fertilization has shifted the dominant plant functional type from being Sphagnum moss to vascular plants (Figures 1C,D). Specifically, the increase in vascular plant abundance and decline in Sphagnum moss abundance were observed after the additions of N, P and their combinations (Figures 1C,D). Namely, the effects of nutrient addition on plant growth and community composition were dependent on the cumulative amount (i.e., rate and duration) of N and P additions (Larmola et al., 2013). Wieder et al. (2019) has documented that 5-year N addition (25 kg N ha–1 year–1) inhibited the growth of Sphagnum fuscum, while increased the net primary productivity of shrubs and a tree species (Picea mariana). Li et al. (2018) observed that the productivity of S. palustre was the largest when the rate of P addition reached 2 kg P ha–1 year–1, whereas any further increase in the addition rate inhibited its growth. Overall, the expansion of vascular plants owing to long-term nutrient enrichment has become a more commonly observed phenomenon in northern peatlands (e.g., Kool and Heijmans, 2009; Wang et al., 2016; Moore et al., 2019).
This shift in plant community composition may be induced by a variety of factors, including nutrient toxicity, shading effect, and microtopography disturbance (Heijmans et al., 2001; Pouliot et al., 2011; Larmola et al., 2013). In the early stage of fertilization, N and/or P additions stimulated Sphagnum moss growth by improving nutrient-poor condition (Leon et al., 2019). Subsequently, with cumulative nutrient input, Sphagnum moss began to lose the filtering capacity and suffered from the competition of vascular plants which had access to enriched N and P availabilities in peat (Bragazza et al., 2005; Larmola et al., 2013). This possible toxic effect owing to nutrient addition drove plant species change with loss of Sphagnum moss, and increased the abundance of vascular plants (Larmola et al., 2013). Furthermore, during the long-term fertilization, the increased vascular plant biomass and litter accumulation would further inhibit Sphagnum moss growth via shading effect (Heijmans et al., 2001; Nordbakken et al., 2003). As observed by Limpens and Berendse (2003), about 53% shading was close to the tolerance limit of Sphagnum moss. As the height and root biomass of vascular plants increased after long-term fertilization, the difference in microtopography were enhanced by physically reinforced “hummockification” (Pouliot et al., 2011). This change of microtopography further resulted in a lowering of water table by augmenting evapotranspiration (Diamond et al., 2020). Aside from the water stress, the increasing abundance of vascular plants would also reduce the accessibility of Sphagnum moss to light and nutrient, which further retarded its growth (Pouliot et al., 2011; Wieder et al., 2019). This competition between Sphagnum moss and vascular plants was confirmed by the significantly negative relationship between their abundances under the long-term fertilization (Figure 2C).
The changes of peat properties were owing to the combined effects of nutrient addition and plant growth (Moore et al., 2019). It was not surprising to observe the increase of TN and TP concentrations after N and P additions (Table 1). We did not find significant interaction between N and P after 3-year fertilization, likely because of limited duration of nutrient addition (Kellera et al., 2005). Interestingly, TP concentration was positively correlated with the abundance of Sphagnum moss under the short-term fertilization and the abundance of vascular plants under the long-term fertilization, indicating that P may play a more important role than N in controlling plant growth at Hani peatland (Li et al., 2019). Compared to the short-term fertilization, K concentration significantly increased and that of Ca and Mg significantly decreased after the long-term fertilization (Table 1). The much smaller concentrations of Ca and Mg in the long-term fertilized plots relative to the short-term ones might be caused by the larger plant uptake owing to favored growth of vascular plants under the long-term fertilization (Moore et al., 2019). The increase in K concentration could be mainly attributed to high leaching velocity with litter decomposition of vascular plants under the long-term fertilization (Bragazza et al., 2007).
Porewater chemistry was not only associated with external nutrient input, but also regulated by the metabolism of soil microorganisms and plants (Moore et al., 2019). Our results disclosed that DON was the dominant dissolved N form, followed by NH4+-N and NO3–-N (Table 2), which was in line with many previous studies (e.g., Chiwa et al., 2016; Moore et al., 2019). In particular, we found that N addition significantly led to the build-up of DON and NH4+-N under both the short- and long-term fertilizations. It is possible that large rate or long-term N addition can enhance microbial activity and plant growth, and stimulate the secretion of secondary metabolites (e.g., amino acids; Bragazza and Limpens, 2004). Moreover, we observed a slight increase in DIN concentration after the long-term fertilization, which may be related to the decline in the capacity of Sphagnum moss as a natural N “filter” owing to the decrease in abundance. However, dissolved P concentration did not change significantly (Table 2). The dissolved nutrients in porewater could be easily assimilated by microorganisms or plants in the form of monomers (Dugan et al., 2011). If a certain type of nutrient limitation in peatlands was deteriorated, the corresponding dissolved nutrients in porewater should be preferentially assimilated by microorganisms and plants (Dugan et al., 2011; Schlesinger and Bernhardt, 2020). Consequently, considering that P might be more limited than N in northern peatlands (e.g., Dimitrov et al., 2014; Hill et al., 2014), the dissolved P in porewater was rapidly assimilated, and thus no significant change in its concentration was observed after nutrient addition.
Weak response of soil carbon
Overall, based on the results of peat and porewater chemistry, TC and DOC concentrations and organic matter composition did not vary considerably neither in our short-term fertilized plots on Sphagnum moss-dominated hummocks, nor on the hummocks where vascular plants expanded after long-term N and/or P enrichment. These results contrasted with our first hypothesis that N and P additions would significantly change the composition of soil chemical moieties and compromise soil C storage during the short-term fertilization (Tables 1, 2). However, they partly supported our second hypothesis that the flourish of vascular plants at the expense of Sphagnum moss eventually protected soil C from being lost during the long-term fertilization.
Specifically, as for the short-term fertilization, we observed that TC and DOC concentrations did not change remarkably with N and P additions (Tables 1, 2). Similarly, changes in FTIR spectra were minimal, and we did not observe a significant effect of the short-term nutrient addition on peat organo-chemical composition as indicated by the humification indices (Figures 3A–E). The short-term nutrient addition did not dramatically change the acidic, waterlogged, and nutrient-deficient conditions, and thus the preserved C in peatlands remained relatively stable (Clymo and Hayward, 1982; Fritz et al., 2011). And this finding was also supported by the extremely low contribution of environmental variables to the humification indices. In addition, the balance between litterfall and decomposition controls soil C dynamics (Berg and Laskowski, 2006). In our study, the FTIR bands indicative of polysaccharide increased in the fertilized plots (Figure 3A), likely due to larger organic matter input instead of greater litter production of predominant Sphagnum moss in the fertilized plots relative to the control. Thus, Sphagnum moss growth may partly compensate for the positive effects of short-term nutrient enrichment on soil C release.
The shift in plant community composition could change the accumulation of high molecular weight compounds via litterfall under long-term fertilization (Moore et al., 2019). The results of FTIR spectra showed that the absorptions of refractory phenolic compounds, carboxylates, and humic acids were enhanced in the fertilized plots, accompanied by a tendency of increasing abundance of vascular plants. Such effects of nutrient addition on FTIR spectra by driving change of plant community composition have also been noted by other studies (e.g., Artz et al., 2008; Chen et al., 2018; Moore et al., 2019). However, for the long-term fertilization, we still did not observe any significant change in the concentrations of TC and DOC, and the humification indices had only slightly changed after being fertilized for 10 years. A possible explanation for this phenomenon might be that vascular plants inhibit the activity of microbial decomposers via the increase of high-phenolic litter production (Keuskamp et al., 2015; Fenner and Freeman, 2020). FTIR spectroscopic analysis demonstrated that the long-term nutrient addition increased the concentrations of aromatic compounds in peat, especially after the combination of N and P additions, as indicated by increasing peak ratios of 1510/1090 and 1630/1090 (i.e., lignin and phenolics/polysaccharide, and aromatics/polysaccharide, respectively; Figures 3H,I). The phenolics (e.g., tannin) and other aromatic components of plant litter were strongly correlated with microbial decomposition, considering that they could bind protein and retard nutrient mobilization via oxidative degradation and biotoxicity (Wang et al., 2015; Fenner and Freeman, 2020). Meanwhile, phenolics have been found to inhibit key hydrolase activities (e.g., β-1,4-glucosidase, invertase, and cellobiohydrolase) and impede nutrient cycling and decomposition (Freeman et al., 2001, 2004). This “enzymatic latch” mechanism is expected to play a key role in peatlands dominated by vascular plants (Wang et al., 2015).
Other processes associated with vascular plant expansion may also retard soil C decomposition. First, the phenolics not only directly impede microbial activity (Freeman et al., 2001; Fenner and Freeman, 2020), but could also shift the fungal community to a slow-growing strategy (Wang et al., 2021). For example, Wang et al. (2021) found that nearly 85% of fungi were fast-growing in Sphagnum-dominated habitat, while shrub-dominated habitat was dominated by slow-growing fungi (75%). The different composition of fungal community with different growing strategies led to the variation in decomposition rate, which was faster in Sphagnum-dominated peatlands than that in peatlands dominated by vascular plants (Koch, 2001; Wang et al., 2021). Second, the extensive root system helps shrub efficiently capture available N and P in soils (Lambers et al., 2006), and compared to soil microorganisms, vascular plants may have advantages of nutrient acquisition (Smith et al., 2011). The competition for nutrients between vascular plants and soil microorganisms might indirectly reduce the sensitivity of soil microorganisms to external nutrient enrichment.
In this study, we formulated a hypothetical logic frame, i.e., C storage involved complex interrelation among peat, porewater, and vegetation processes, and assessed the divergent effects of the short- and long-term fertilizations on organic matter decomposition as indicated by the humification indices. Interestingly, the physicochemical properties of peat and porewater had little effect on the humification indices in the short-term fertilization plots where Sphagnum moss-dominated. Besides, the harsh conditions for microbial decomposers, combined with the increased C return from litterfall of Sphagnum moss, likely resulted in the lack of significant changes in soil C concentrations and organo-chemical composition during the short-term fertilization (Figure 4A). For the long-term fertilization, although the contribution of environmental variables to organic matter decomposition has increased, the input of high-phenolic litters owing to the shift in plant community composition from being Sphagnum moss- to vascular plant-dominated, may ultimately inhibit microbial activity and soil C release (Figure 4B).
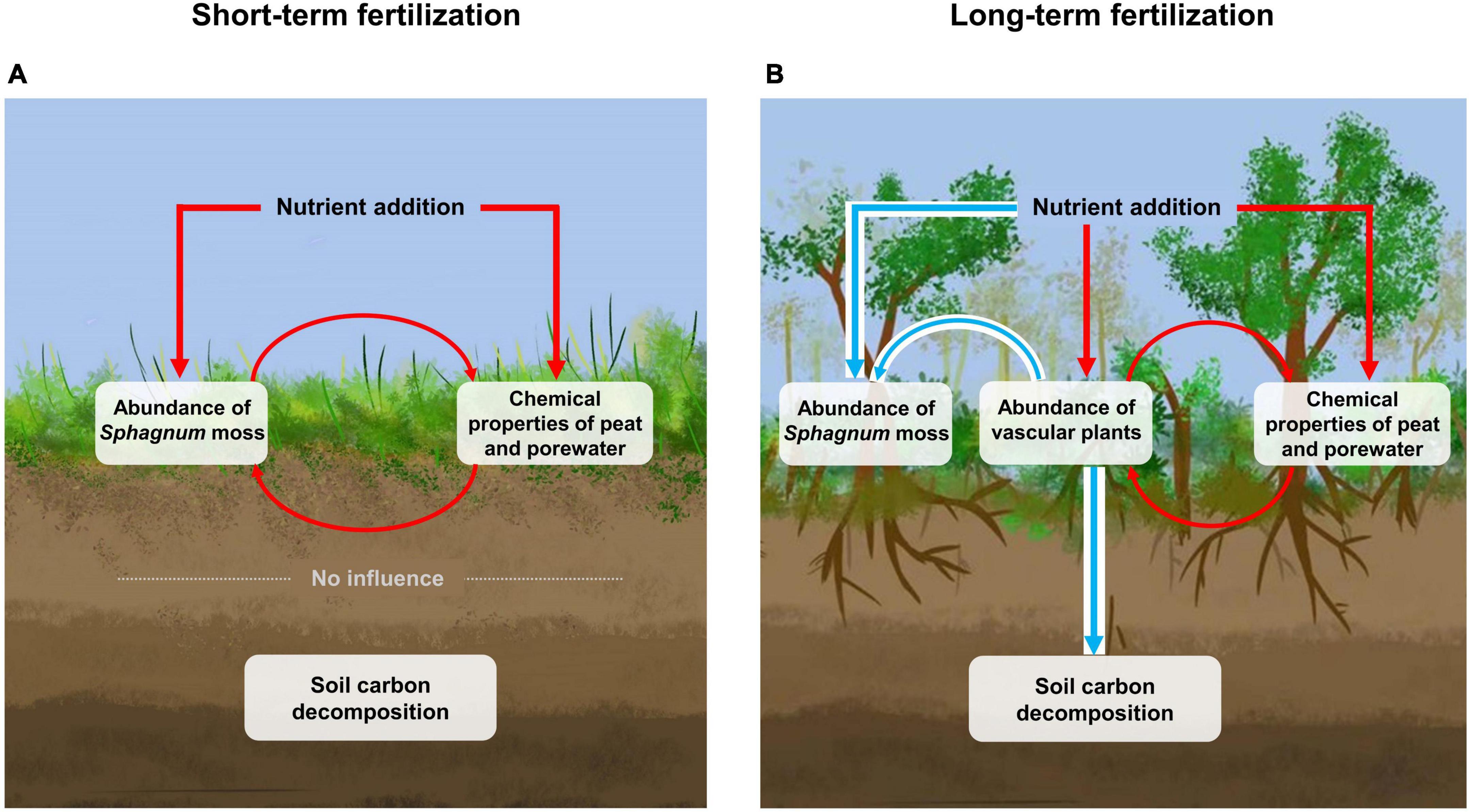
Figure 4. Schematic diagrams of the mechanisms of soil C protection in the short- (A) and long-term fertilizations (B). Red arrows indicate positive influences and blue arrows indicate negative influences.
Data availability statement
The raw data supporting the conclusions of this article will be made available by the authors upon request.
Author contributions
TL and MW: conceptualization. TL, XY, LG, CC, YS, ZL, SL, and MW: field sampling and laboratory analyses. All authors contributed to manuscript preparation.
Funding
This study was funded by the National Natural Science Foundation of China (41971118).
Conflict of interest
The authors declare that the research was conducted in the absence of any commercial or financial relationships that could be construed as a potential conflict of interest.
Publisher’s note
All claims expressed in this article are solely those of the authors and do not necessarily represent those of their affiliated organizations, or those of the publisher, the editors and the reviewers. Any product that may be evaluated in this article, or claim that may be made by its manufacturer, is not guaranteed or endorsed by the publisher.
Acknowledgments
We are grateful to Jingjing Huang, Lin Zhu, Liying Lin, and Xuying Pei for their assistance in the laboratory, and Li Zhao for the assistance of sampling in a pilot study.
References
Ackerman, D., Millet, D. B., and Chen, X. (2019). Global estimates of inorganic nitrogen deposition across four decades. Glob. Biogeochem. Cycles 33, 100–107. doi: 10.1029/2018GB005990
Artz, R. R., Chapman, S. J., Robertson, A. J., Potts, J. M., Laggoun-Défarge, F., Gogo, S., et al. (2008). FTIR spectroscopy can be used as a screening tool for organic matter quality in regenerating cutover peatlands. Soil Biol. Biochem. 40, 515–527. doi: 10.1016/j.soilbio.2007.09.019
Berg, B., and Laskowski, R. (2006). Litter Decomposition: A Guide to Carbon and Nutrient Turnover. Amsterdam: Elservier Academic Press.
Biester, H., Knorr, K. H., Schellekens, J., Basler, A., and Hermanns, Y. M. (2014). Comparison of different methods to determine the degree of peat decomposition in peat bogs. Biogeosciences 11, 2691–2707. doi: 10.5194/bg-11-2691-2014
Bragazza, L., and Limpens, J. (2004). Dissolved organic nitrogen dominates in European bogs under increasing atmospheric N deposition. Glob. Biogeochem. Cycles 18:GH4018. doi: 10.1029/2004GB002267
Bragazza, L., Limpens, J., Gerdol, R., Grosvernier, P., Hajek, M., Hajek, T., et al. (2005). Nitrogen concentration and delta 15N signature of ombrotrophic Sphagnum mosses at different N deposition levels in Europe. Glob. Change Biol. 11, 106–114. doi: 10.1111/j.1365-2486.2004.00886.x
Bragazza, L., Siffi, C., Iacumin, P., and Gerdol, R. (2007). Mass loss and nutrient release during litter decay in peatland: the role of microbial adaptability to litter chemistry. Soil Biol. Biochem. 39, 257–267. doi: 10.1016/j.soilbio.2006.07.014
Broder, T., Blodau, C., Biester, H., and Knorr, K. H. (2012). Peat decomposition records in three pristine ombrotrophic bogs in southern Patagonia. Biogeosciences 9, 1479–1491. doi: 10.5194/bg-9-1479-2012
Bu, Z. J., Rydin, H., and Chen, X. (2011). Direct and interaction-mediated effects of environmental changes on peatland bryophytes. Oecologia 166, 555–563. doi: 10.1007/s00442-010-1880-1
Bubier, J. L., Moore, T. R., and Bledzki, L. A. (2007). Effects of nutrient addition on vegetation and carbon cycling in an ombrotrophic bog. Glob. Change Biol. 13, 1168–1186. doi: 10.1111/j.1365-2486.2007.01346.x
Chen, X., Jin, M. C., Zhang, Y. J., Hu, J. W., Gao, H. J., Chu, W. Y., et al. (2018). Nitrogen application increases abundance of recalcitrant compounds of soil organic matter: a 6-year case study. Soil Sci. 183, 169–178. doi: 10.1097/SS.0000000000000243
Chiwa, M., Sheppard, L. J., Leith, I. D., Leeson, S. R., Tang, Y. S., and Cape, J. N. (2016). Sphagnum can ‘flter’ N deposition, but effects on the plant and pore water depend on the N form. Sci. Total Environ. 559, 113–120. doi: 10.1016/j.scitotenv.2016.03.130
Clymo, R. S., and Hayward, P. M. (1982). “The ecology of Sphagnum,” in Bryophyte Ecology, ed. A. J. E. Smith (Dordrecht: Springer), 229–289.
Cocozza, C., D’Orazio, V., Miano, T. M., and Shotyk, W. (2003). Characterization of solid and aqueous phases of a peat bog profle using molecular fluorescence spectroscopy, ESR and FT-IR, and comparison with physical properties. Org. Geochem. 34, 49–60. doi: 10.1016/S0146-6380(02)00208-5
Cusell, C., Kooijman, A., and Lamers, L. P. M. (2014). Nitrogen or phosphorus limitation in rich fens? – Edaphic differences explain contrasting results in vegetation development after fertilization. Plant Soil 384, 153–168. doi: 10.1007/s11104-014-2193-7
Dash, S., Borah, S. S., and Kalamdhad, A. S. (2020). Study of the limnology of wetlands through a one-dimensional model for assessing the eutrophication levels induced by various pollution sources. Ecol. Modell. 416:108907. doi: 10.1016/j.ecolmodel.2019.108907
Diamond, J. S., McLaughlin, D. L., Slesak, R. A., and Stovall, A. (2020). Microtopography is a fundamental organizing structure of vegetation and soil chemistry in black ash wetlands. Biogeosciences 17, 901–915. doi: 10.5194/bg-17-901-2020
Dimitrov, D. D., Bhatti, J. S., and Grant, R. F. (2014). The transition zones (ecotone) between boreal forests and peatlands: ecological controls on ecosystem productivity along a transition zone between upland black spruce forest and a poor forested fen in central Saskatchewan. Ecol. Modell. 291, 96–108. doi: 10.1016/j.ecolmodel.2014.07.020
Drollinger, S., Knorr, K. H., Knierzinger, W., and Glatzel, S. (2020). Peat decomposition proxies of Alpine bogs along a degradation gradient. Geoderma 369, 114331. doi: 10.1016/j.geoderma.2020.114331
Dugan, J. E., Hubbard, D. M., Page, H. M., and Schimel, J. P. (2011). Marine macrophyte wrack inputs and dissolved nutrients in Beach Sands. Estuar. Coasts 34, 839–850. doi: 10.1007/s12237-011-9375-9
Fenner, N., and Freeman, C. (2020). Woody litter protects peat carbon stocks during drought. Nat. Climate Chang. 10, 363–369. doi: 10.1038/s41558-020-0727-y
Freeman, C., Ostle, N. J., Fenner, N., and Kang, H. (2004). A regulatory role for phenol oxidase during decomposition in peatlands. Soil Biol. Biochem. 36, 1663–1667. doi: 10.1016/j.soilbio.2004.07.012
Freeman, C., Ostle, N., and Kang, H. (2001). An enzymic ‘latch’ on a global carbon store. Nature 409:149. doi: 10.1038/35051650
Fritz, C., van Dijk, G., Smolders, A. J. P., Pancotto, V. A., and Elzenga, T. J. T. M. (2011). Nutrient additions in pristine Patagonian Sphagnum bog vegetation: can phosphorus addition alleviate (the effects of) increased nitrogen loads. Plant Biol. 14, 491–499. doi: 10.1111/j.1438-8677.2011.00527.x
Gorham, E. (1991). Northern peatlands: role in the carbon cycle and probable responses to climatic warming. Ecol. Appl. 1, 182–195. doi: 10.2307/1941811
Heijmans, M. P. D., Berendase, F., Arp, W. J., Masselink, A. K., Kless, H., de Visser, W., et al. (2001). Effects of elevated carbon dioxide and increased nitrogen deposition on bog vegetation in the Netherlands. J. Ecol. 89, 268–279. doi: 10.1046/j.1365-2745.2001.00547.x
Hill, B. H., Elonen, C. M., Jicha, T. M., Kolka, R. K., Lehto, L. L. P., Sebestyen, S. D., et al. (2014). Ecoenzymatic stoichiometry and microbial processing of organic matter in northern bogs and fens reveals a common P-limitation between peatland types. Biogeochemistry 120, 203–224. doi: 10.1007/s10533-014-9991-0
Kellera, J. K., Bridghamb, S. D., Chapin, C. T., and Iversend, C. M. (2005). Limited effects of six years of fertilization on carbon mineralization dynamics in a Minnesota fen. Soil Biol. Biochem. 37, 1197–1204. doi: 10.1016/j.soilbio.2004.11.018
Keuskamp, J. A., Feller, I. C., Laanbroek, H. J., Verhoeven, J. T. A., and Hefting, M. M. (2015). Short- and long-term effects of nutrient enrichment on microbial exoenzyme activity in mangrove peat. Soil Biol. Biochem. 81, 38–47. doi: 10.1016/j.soilbio.2014.11.003
Kool, A., and Heijmans, M. M. P. D. (2009). Dwarf shrubs are stronger competitors than graminoid species at high nutrient supply in peat bogs. Plant Ecol. 204, 125–134. doi: 10.1007/s11258-009-9574-7
Lai, J. S., Zhou, Y., Zhang, J. L., and Peres-neto, P. R. (2022). Generalizing hierarchical and variation partitioning in multiple regression and canonical analyses using the rdacca.hp R package. Methods Ecol. Evol. 13, 782–788. doi: 10.1111/2041-210X.13800
Lambers, H., Shane, M. W., Cramer, M. D., Pearse, S. J., and Veneklaas, E. J. (2006). Root structure and functioning for efficient acquisition of phosphorus: matching morphological and physiological traits. Ann. Bot. 98, 693–713. doi: 10.1093/aob/mcl114
Larmola, T., Bubier, J. L., Kobyljanec, C., Basiliko, N., Juutinen, S., Humphreys, E., et al. (2013). Vegetation feedbacks of nutrient addition lead to a weaker carbon sink in an ombrotrophic bog. Glob Change Biol. 19, 3729–3739. doi: 10.1111/gcb.12328
Leon, C. A., Neila-Pivet, M., Benitez-Mora, A., and Lara, L. (2019). Effect of phosphorus and nitrogen on Sphagnum regeneration and growth: an experience from Patagonia. Wetl. Escol. Manag. 27, 257–266. doi: 10.1007/s11273-019-09657-4
Li, T. T., Lei, Y., Dai, C., Yang, L. F., Li, Z. Q., and Wang, Z. X. (2018). Effects of both substrate and nitrogen and phosphorus fertilizer on Sphagnum palustre growth in subtropical high-mountain regions and implications for peatland recovery. Wetl. Ecol. Manag. 26, 651–663. doi: 10.1007/s11273-018-9598-7
Li, T., Bu, Z. J., Liu, W. Y., Zhang, M. Y., Peng, C. H., Zhu, Q. A., et al. (2019). Weakening of the ‘enzymatic latch’ mechanism following long-term fertilization in a minerotrophic peatland. Soil Biol. Biochem. 136:107528. doi: 10.1016/j.soilbio.2019.107528
Li, T., Ge, L. M., Huang, J. J., Yuan, X., Peng, C. H., Wang, S. Z., et al. (2020). Contrasting responses of soil exoenzymatic interactions and the dissociated carbon transformation to short- and long-term drainage in a minerotrophic peatland. Geoderma 377:114585. doi: 10.1016/j.geoderma.2020.114585
Limpens, J., and Berendse, F. (2003). Growth reduction of Sphagnum magellanicum subjected to high nitrogen deposition: the role of amino acid nitrogen concentration. Oecologia 135, 339–345. doi: 10.1007/s00442-003-1224-5
Limpens, J., Berendse, F., and Klees, H. (2004). How phosphorus availability affects the impact of nitrogen deposition on Sphagnum and vascular plants in bogs. Ecosystems 7, 793–804. doi: 10.1007/s10021-004-0274-9
Moore, T. R., Bubier, J. L., Frolking, S. E., Lafleur, P. M., and Roulet, N. T. (2002). Plant biomass and production and CO2 exchange in an ombrotrophic bog. J. Ecol. 90, 25–36. doi: 10.1046/j.0022-0477.2001.00633.x
Moore, T. R., Knorr, K. H., Thompson, L., Roy, C., and Bubier, J. L. (2019). The effect of long-term fertilization on peat in an ombrotrophic bog. Geoderma 343, 176–186. doi: 10.1016/j.geoderma.2019.02.034
Murphy, J., and Riley, J. P. (1962). A modified single solution method for the determination of phosphate in natural waters. Anal. Chim. Acta 26, 31–36. doi: 10.1021/ja038277x
Niemeyer, J., Chen, Y., and Bollag, J. M. (1992). Characterization of humic acids, composts, and peat by diffuse reflectance Fourier-transform infrared spectroscopy. Soil Sci. Soc. Am. J. 56, 135–140. doi: 10.2136/sssaj1992.03615995005600010021x
Nordbakken, J. F., Ohlson, M., and Hogbeng, P. (2003). Boreal bog plants: nitrogen sources and uptake of recently deposited nitrogen. Environ. Poll. 126, 191–200. doi: 10.1016/S0269-7491(03)00194-5
Ohno, T., and Zibilske, L. M. (1991). Determination of low concentrations of phosphorus in soil extracts using malachite green. Soil Sci. Soc. Am. J. 55:3. doi: 10.2136/sssaj1991.03615995005500030046x
Parkinson, J. A., and Allen, S. E. (2008). A wet oxidation procedure suitable for the determination of nitrogen and mineral nutrients in biological material. Commun. Soil Sci. Plant Anal. 6, 1–11. doi: 10.1080/00103627509366539
Peñuelas, J., Poulter, B., Sardans, J., Ciais, P., van der Velde, M., Bopp, L., et al. (2013). Human-induced nitrogen-phosphorus imbalances alter natural and managed ecosystems across the globe. Nat. Commun. 4:2934. doi: 10.1038/ncomms3934
Pouliot, R., Rochefort, L., Karofeld, E., and Mercier, C. (2011). Initiation of Sphagnum moss hummocks in bogs and the presence of vascular plants: is there a link? Acta Oecol. 37, 346–354. doi: 10.1016/j.actao.2011.04.001
Qiao, S. (1993). A preliminary study on Hani peat mire in the west part of the Changbai Mountain (in Chinese). Sci. Geogr. Sin. 13, 279–286. doi: 10.13249/j.cnki.sgs.1993.03.279
R Core Team (2021). R: A Language and Environment for Statistical Computing. Vienna: R Foundation for Statistical Computing. Available online at: https://www.R-project.org/
Rice, K. C., and Herman, J. S. (2012). Acidification of Earth: an assessment across mechanisms and scales. Appl. Geochem. 27, 1–14. doi: 10.1016/j.apgeochem.2011.09.001
Rousk, J., Smith, A. R., and Jones, D. L. (2013). Investigating the long-term legacy of drought and warming on the soil microbial community across five European shrubland ecosystems. Glob. Change Biol. 19, 3872–3884. doi: 10.1111/gcb.12338
Rydin, H., and Jeglum, J. K. (2013). The Biology of Peatlands. New York, NY: Oxford University Press.
Schlesinger, W. H., and Bernhardt, E. S. (2020). “Wetland ecosystem,” in Biogeochemistry: An Analysis of Global Change, Fourth edition, ed. A. Shapiro (Salt Lake City, UT: American Academic Press), 249–291.
Schothorst, C. J. (1977). Subsidence of low moor peat soils in the western Netherlands. Geoderma 17, 265–291. doi: 10.1016/0016-7061(77)90089-1
Smith, S. E., Jakobsen, I., Gronlund, M., and Smith, F. A. (2011). Roles of arbuscular mycorrhizas in plant phosphorus nutrition: interactions between pathways of phosphorus uptake in arbuscular mycorrhizal roots have important implications for understanding and manipulating plant phosphorus acquisition. Plant Physiol. 156, 1050–1057. doi: 10.1104/pp.111.174581
Teickner, H. (2022). ir: Functions to Handle and Preprocess Infrared Spectra. Available online at: https://zenodo.org/record/5747170 (assessed July 5, 2022).
Teickner, H., and Hodgkins, S. B. (2022). irpeat: Functions to Analyze Mid Infrared Spectra of Peat Samples. Available online at: https://github.com/henningte/irpeat (accessed July 5, 2022).
Tipping, E., Benham, S., Boyle, J., Crow, P., Davies, J., Fischer, U., et al. (2014). Atmospheric deposition of phosphorus to land and freshwater. Environ. Sci. Process. Impacts 16, 1608–1617. doi: 10.1039/c3em00641g
van Breemen, N. (1995). How Sphagnum bogs down other plants. Trends Ecol. Evol. 10, 270–275. doi: 10.1016/0169-5347(95)90007-1
Wang, H. J., Richardson, C. J., and Ho, M. C. (2015). Dual controls on carbon loss during drought in peatlands. Nat. Clim. Change 5, 584–587. doi: 10.1038/nclimate2643
Wang, H. J., Tian, J. Q., Chen, H., Ho, M. C., Vilgalys, R., Bu, Z. J., et al. (2021). Vegetation and microbes interact to preserve carbon in many wooded peatlands. Commun. Earth Environ. 2:67. doi: 10.1038/s43247-021-00136-4
Wang, M., Larmola, T., Murphy, M. T., Moore, T. R., and Bubier, J. L. (2016). Stoichiometric response of shrubs and mosses to long-term nutrient (N, P and K) addition in an ombrotrophic peatland. Plant Soil 400, 403–416. doi: 10.1007/s11104-015-2744-6
Wieder, R. K., Vitt, D. H., Vile, M. A., Graham, J. A., Hartsock, J. A., Fillingim, H., et al. (2019). Experimental nitrogen addition alters structure and function of a boreal bog: critical load and thresholds revealed. Ecol. Monogr. 89:e01371. doi: 10.1002/ecm.1371
Keywords: fen, bog, fertilization, FTIR, humification indices
Citation: Li T, Yuan X, Ge L, Cao C, Suo Y, Bu Z-J, Peng C, Song H, Liu Z, Liu S and Wang M (2022) Weak impact of nutrient enrichment on peat: Evidence from physicochemical properties. Front. Ecol. Evol. 10:973626. doi: 10.3389/fevo.2022.973626
Received: 20 June 2022; Accepted: 07 July 2022;
Published: 25 July 2022.
Edited by:
Chuanyu Gao, Northeast Institute of Geography and Agroecology (CAS), ChinaReviewed by:
Yuanchun Zou, Northeast Institute of Geography and Agroecology (CAS), ChinaXiaoming Kang, Institute of Wetland Research, Chinese Academy of Forestry, China
Copyright © 2022 Li, Yuan, Ge, Cao, Suo, Bu, Peng, Song, Liu, Liu and Wang. This is an open-access article distributed under the terms of the Creative Commons Attribution License (CC BY). The use, distribution or reproduction in other forums is permitted, provided the original author(s) and the copyright owner(s) are credited and that the original publication in this journal is cited, in accordance with accepted academic practice. No use, distribution or reproduction is permitted which does not comply with these terms.
*Correspondence: Meng Wang, bWVuZy53YW5nM0BtYWlsLm1jZ2lsbC5jYQ==