- 1Unité Physiologie Moléculaire et Adaptation, UMRMuséum National d'Histoire Naturelle, CNRS, CP32, Paris, France
- 2Behavioural Ecology & Ecophysiology Group, Department of Biology, University of Antwerp, Universiteitsplein, Wilrijk, Belgium
- 3Littoral Environnement et Sociétés, UMR, CNRS – La Rochelle Université, La Rochelle, France
- 4Institut Universitaire de France (IUF), Paris, France
- 5Centre d'Etudes Biologiques de Chizé (CEBC), UMR, CNRS/La Rochelle Université, La Rochelle, France
- 6Department of Ecological and Biological Science, Tuscia University, Largo dell’Università s.n.c., Viterbo, Italy
Although infectious disease outbreaks represent a serious threat for wildlife population viability, the environmental factors that underlie such outbreaks are poorly investigated. The French Guiana breeding population of Magnificent frigatebird Fregata magnificens is subjected to recurrent episodes of chicks’ mortality likely caused by a viral disease. We hypothesized that high mercury (Hg) concentrations may be responsible for the emergence of clinical signs. We therefore investigated whether healthy and sick chicks show different Hg concentrations in blood. Because the essential element selenium (Se) may be highly depleted during Hg poisoning, we further experimentally tested whether an increased intake of dietary Se has an effect on blood levels of Hg, increases circulating Se, and improves the oxidative status of chicks. Finally, we compared the results of this experiment with a previous food supplementation experiment. Our results show similar Hg concentrations between healthy and sick chicks with visible clinical signs of the disease. Se concentrations were significantly depleted in sick chicks. Se concentrations increased while Hg concentrations simultaneously decreased in chicks that naturally recovered from the disease. Both the Se and fish supplementation experiments significantly increased Se concentrations in blood, while Hg levels were only modestly affected. Providing food to chicks appeared to have greater benefits than only supplementing chicks with Se pills as, although food supplementation had an impact on blood Se similar to that of supplementation with Se pills, it also reduced the vulnerability of chicks to the viral disease, possibly by reducing nutritional stress and providing essential nutrients.
Introduction
Over the past years, there has been growing recognition of the risks associated with infectious disease outbreaks. Anthropogenic activities alter the environment and represent major factors for increased transmission of pathogens and emergence of new diseases (Sabin et al., 2020). When human activities deplete biodiversity, ecosystem services are impaired and pathogens are more likely to emerge (Cunningham et al., 2017). To date, outbreaks of infectious pathogens are occurring at an unprecedented rate (Fisher et al., 2012; Baker et al., 2022), but the mechanisms underlying such events are poorly investigated although they represent a serious threat for wildlife population viability.
One documented example of such outbreaks occurs on the French natural reserve of Grand Connétable, a small rocky island located near the coasts of French Guiana (South America). The site hosts several seabird species and approximately 1,300 reproductive pairs of Magnificent frigatebird Fregata magnificens (Sebastiano et al., 2017a). Since 2005, the population of frigatebirds is subjected to massive mortality episodes estimated between 85 and 95% of chicks that occur annually (Sebastiano et al., 2017b,c). The disease – most likely associated with herpesvirus activity (de Thoisy et al., 2009; Sebastiano et al., 2018) – is characterized by the appearance of skin crusts on the neck and wings of affected birds, which rapidly spread all over the chicks’ body, resulting in low chances of recovery (about 15%; Sebastiano et al., 2019). Chicks with visible clinical signs of the disease exhibit changes in blood-based oxidative status markers (Sebastiano et al., 2017b), high inflammation levels (Sebastiano et al., 2017c), and lower immune defences (Sebastiano et al., 2018) as compared to healthy chicks. Further experimental work on this population of frigatebirds showed that an experimental supplementation with resveratrol, a powerful antioxidant with antiviral proprieties (Abba et al., 2015), increased circulating antioxidants, limited the generation of oxidative damage, and stimulated the immune response (Sebastiano et al., 2018), but did not influence the chicks’ probability to develop clinical signs or to recover/survive (Sebastiano et al., 2018).
The appearance of clinical signs in wild animals may indicate that they are undergoing stress. Exposure to environmental stressors can alter wildlife immune function (Stephanie et al., 2016), but it remains unclear how this translates into changes in patterns of infectious diseases (Martin, 2009; Blaustein et al., 2012; Stephanie et al., 2016). In French Guiana, one potential environmental stressor frigatebirds may be exposed to is nutritional stress. After the dramatic decline of the local shrimp fishery activities - highly beneficial for opportunistic feeders like frigatebirds due to the associated discards - field observations suggested that adult frigatebirds were struggling to feed their chicks (Martinet and Blanchard, 2009), and recent experimental work seems to support this food limitation hypothesis (Sebastiano et al., 2019). Another potential environmental stressor is local mercury (Hg) contamination (Sebastiano et al., 2016, 2017a). A recent review pointed out how Hg impacts on almost every aspect of avian physiology (Whitney and Cristol, 2017). Given the high affinity between Hg and selenium (Se), one way through which Hg causes physiological stress is by binding to selenoproteins, thus compromising their synthesis and biological functions in oxidoreductase (Ralston and Raymond, 2010). Similarly, Hg can decrease the availability of Se (Ralston and Raymond, 2010), whose deficiency has been specifically linked with increased susceptibility to diseases (Gomez et al., 2002; Wang et al., 2009), likely due to the important regulatory role played by Se in the immune response (Hawkes et al., 2001; Hoffmann and Berry, 2008). The underlying mechanisms of disease outbreaks in this population have not yet been investigated nor identified so far, and we lack direct evidence that the occurrence of clinical signs may be related to Hg exposure or Se deficiency.
In this study, we hypothesized that exposure to Hg potentially contributes to the occurrence of clinical signs in this population. We quantified the concentrations of Hg and Se in healthy (without visible clinical signs) and sick chicks (with visible clinical signs of the disease). If exposure to Hg is associated with disease occurrence in this species, we predicted a difference between healthy and sick chicks in Hg concentrations in blood. Similarly, if Hg reduces the bioavailability of Se, we predicted a deficiency of Se in chicks exposed to higher Hg levels. Because Se decreases Hg toxicity in birds (Ikemoto et al., 2004) and other animal species (Ikemoto et al., 2004; Sørmo et al., 2011; Polak-Juszczak and Robak, 2015), we experimentally investigated the effect of dietary Se on circulating levels of Se and Hg. Se is also utilized as a cofactor by the antioxidant enzyme selenium-dependent glutathione peroxidase (Beckett and Arthur, 2005). We therefore further analyzed the effect of dietary Se on the oxidative status of chicks because prior work showed that they were associated with the occurrence of clinical signs and progress of the disease in our species (Sebastiano et al., 2017b). Finally – because prior experimental work suggested that frigatebird chicks may also be undergoing nutritional stress (Sebastiano et al., 2019) – we hypothesized that the combination of high Hg concentrations (Sebastiano et al., 2017a) and nutritional stress may be responsible for the emergence of clinical signs. If the occurrence of clinical signs of the disease is mainly tied to food shortage, we predicted that - by comparing the results from the Se supplementation experiment with the results from a previous food supplementation experiment (Sebastiano et al., 2019) – extra food would have a greater effect than Se alone on the progress of the disease and the physiological status of chicks.
Materials and methods
Sample collection
Fieldwork was carried out from 2015 to 2017 on Grand Connétable island, a protected area located off the Atlantic coast of South America (French Guiana, 4°49′30 N; 51°56′00 W). Most frigatebird pairs in this colony start breeding between the end of November and the beginning of December. Consequently, all chicks were approximately of the same age (~4 months old) when the experiment was carried out (see also Statistical Analysis section). A total of 93 chicks without visible clinical signs (22 in 2015, 26 in 2016, and 45 in 2016) and 101 chicks showing visible clinical signs (22 in 2015, 34 in 2016, and 45 in 2016) were randomly chosen at different sites of the island and captured at the nest by hand. Visible clinical signs of the disease include crusts on the head and the body, hyperkeratosis on eyes and the consequent thickening of the cornea (de Thoisy et al., 2009). Within a few minutes after capture, 2 ml of blood was collected from the brachial vein using a heparinized syringe and a 25G needle.
Samples were placed in a cooler and centrifuged in the field within less than 3 h to separate plasma and red blood cells. Both plasma and red blood cells were kept in dry ice until the end of the field work and, when at the laboratory, were kept in a − 80°C freezer until laboratory analyses. Birds were also ringed with an aluminum ring for individual recognition, weighted with a Pesola spring balance to the nearest 20 g (except for chicks sampled in 2015), and the length of the skull was measured with a 300 mm Caliper (from the tip of the beak to the back of the head) to control for the age of the chicks. In 2017, a food supplementation experiment (for which results are already published in Sebastiano et al., 2019) and a Se-supplementation experiment (the present study) were simultaneously carried out. Briefly, chicks received either a pill of Se, fish, or were not supplemented (control group). During the experimental period, Se pills (each pill containing 200 μg of L-Selenomethionine, the organic form of Se) were provided nine times while fish (Atlantic horse mackerels Trachurus trachurus, non-local and eviscerated, thus very low in Hg concentrations) was provided by direct feeding six times within 16 days, to match the frigatebird feeding rate. At the end of the experiments, a second sample of blood was taken and body mass and skull length were measured again. Pictures taken before the start and at the end of the experiment were scored and used to classify the chicks based on severity of visible clinical signs (“no signs - birds with absence of visible clinical signs”; “mild - birds that clearly showed clinical signs of the disease”; and “severe - birds deeply affected by the disease as highly covered with visible clinical signs”), as described in Sebastiano et al. (2019). This also enabled to detect birds that changed group over the progress of the disease (which matches a change in the visible clinical signs), thus to identify: (a) birds that never showed the appearance of clinical signs, hereafter “always healthy”; (b) birds that showed the appearance of clinical signs, hereafter “new sick”; (c) birds that had an improvement of visible clinical signs, hereafter “improved”; and (d) birds that did not change their status, hereafter “always sick”). Details on the supplementation experiments and the classification of birds based on visible clinical signs can be found in Sebastiano et al. (2019) and in the Supplementary information.
Laboratory analyses
The analysis of trace element concentrations was carried out by the Littoral Environnement et Sociétés (LIENSs) laboratory at La Rochelle Université (France). Total Hg was quantified with an Altec Advanced Mercury Analyzer AMA 254 spectrophotometer while Se was analyzed using a Varian Vista-Pro ICP-OES, as described in Bustamante et al. (2008). Prior and after freeze-drying, blood samples were weighed to determine the percentage of water in blood, and aliquots ranging from 5 to 10 mg were analyzed for quality assessment, as described in Bustamante et al. (2008). Certified Reference Materials (CRM; dogfish liver DOLT-5, NRCC, and lobster hepatopancreas TORT-3, NRCC) were treated and analyzed in the same way as the samples. Results were in good agreement with the certified values, and the standard deviations were low, proving good repeatability of the method. The results for CRMs displayed recoveries of the elements ranging from 87 to 103% (n = 30). All the results for trace elements are presented in absolute concentrations in μg g−1 dry weight (dw.). Hg was measured in samples from 2015 to 2017 while Se levels were measured only in 2016 and 2017.
All analyses of metrics of oxidative status were done using established protocols for vertebrates. High-performance liquid chromatography (HPLC) with electrochemical detection (Reversed-Phase HPLC of Shimadzu, Hai Zhong Lu, Shanghai) was used to quantify reduced glutathione (GSH) concentrations in red blood cells, following Sinha et al. (2014), and concentrations were expressed as μmol/g of fresh weight. Glutathione peroxidase (GPx) activity was determined in red blood cells by measuring the decrease in NADPH absorbance at 340 nm using a spectrophotometric method (Drotar et al., 1985), and was expressed as μmol NADPH/mg protein per minute.
Statistical analyses
Although chicks were approximately of the same age, their body mass highly fluctuates over time depending on feeding events frequency and timing. For instance, chicks may be fed even after 3 days of fasting, with an amount of food that can be up to 300/400 grams (personal observation). Therefore, chicks of similar age may strongly differ in their body weight if measured shortly after their received food from their parents. Therefore, we decided not to include it into the statistical analyses, but instead we used skull length as a proxy of the age into statistical models when possible.
Linear models were used to investigate Hg differences (pooled samples from 2015 to 2017, n = 194) and Se (pooled samples from 2016 and 2017, n = 150) among years or between the health status categories of chicks (with or without clinical signs), or among groups separated based on the severity of clinical signs (separated linear models among chicks with no signs, mild signs, and severe clinical signs). Hg and Se were included as dependent variables while the year of study and the health status of chicks were included as predictors. Skull length was not included in the models for Hg as it was not measured in 2015 chicks. Hg and Se were log10 transformed when testing years and groups differences.
Generalized linear models (GLMs) with a binomial error distribution (0 = survives, 1 = dies) were used to test whether pre-existing levels of Se and Hg predict survival probabilities of chicks (used as predictors in the model). These models were run on control individuals (non-treated birds, as the treatment could have modified the progress of the disease) from the year 2016 and 2017 (for which we had complete data). Hg and Se were log10 transformed when using generalized linear models. Skull length was included in GLMs to correct for the age of the chicks.
Linear mixed models were used to specifically test whether either the Se or the food supplementation experiments generated a significant change in Hg or Se levels, and in physiological markers of antioxidant defenses, between the first and the second sampling period (before and after the experiments). A three-way interaction (experimental period * health status * experimental group) was thus used to test among groups differences (control, Se, or fish administered groups, further divided between chicks with and without clinical signs of the disease). The skull length was included as a covariate to correct for the age of the chicks, while the ID of the chicks was used as a random factor. Hg, Se, and GPx valued were not transformed while GSH concentrations were log10 transformed when used in linear mixed models to achieve homoscedasticity and normality of residuals. Three outliers were excluded from the model on GPx as they were highly influential in the model, and exceed the mean ± 3 times the standard deviation.
Linear mixed models were further used to specifically test whether Se and Hg concentrations showed a change from the first to the second sampling period in chicks divided based on the progress of the disease (always healthy, get sick, always sick, improved; as described in detail in Sebastiano et al. (2019). These models were only run on control chicks (chicks that were not included in the experiments). The skull length was included as a covariate to correct for the age of the chicks, while the ID of the chicks was included as a random factor.
All data transformation and violation of models’ assumptions are reported throughout the manuscript. Statistical significance was set to α = 0.05. All statistical analyses were performed using R version 3.6.1.
Results
Year and group differences at sampling 1
There were no significant differences in Hg concentrations among the 3 years of study (F = 2.50, p = 0.08), although there was a tendency for Hg to be higher in 2015 than 2017 (t = 2.2, p = 0.07; Supplementary Figure S1a). Healthy and sick chicks had similar concentrations of Hg (F = 0.98, p = 0.32; Supplementary Figure S1b), even when chicks were divided among chicks with no, mild, or severe clinical signs (F = 0.70, p = 0.50; Supplementary Figure S1c).
There was no difference between 2016 and 2017 in Se concentrations (F = 1.18, p = 0.28; Supplementary Figure S2a). Healthy and sick birds had different blood Se concentrations (F = 31.64, p < 0.001), with Se being significantly higher in healthy than sick chicks (t = 5.52, p < 0.001; Supplementary Figure S2b). Similar results were obtained when birds were divided based on the severity of clinical signs (F = 15.91, p < 0.001), as birds with no clinical signs had significantly higher Se levels than both groups with mild or severe clinical signs (both t > 4.32, both p < 0.001; Supplementary Figure S2c).
Pre-treatment levels of either Hg or Se did not predict survival probabilities. Chicks that survived had similar levels of Hg or Se to chicks that died, even when the same models were run only considering sick chicks (all z values < 1.36, all p > 0.17).
Finally, 6 out of 15 (40%) healthy chicks administered with Se pills showed the appearance of clinical signs over the course of the experiment.
Effect of the experiments on blood concentrations of Hg and Se
The food-supplementation experiment influenced blood Hg concentrations (significant three-way interaction; F = 5.14, p < 0.01; Table 1). Hg significantly increased in sick chicks administered with fish (t = −5.75, p < 0.001; Supplementary Table S1; Figure 1).
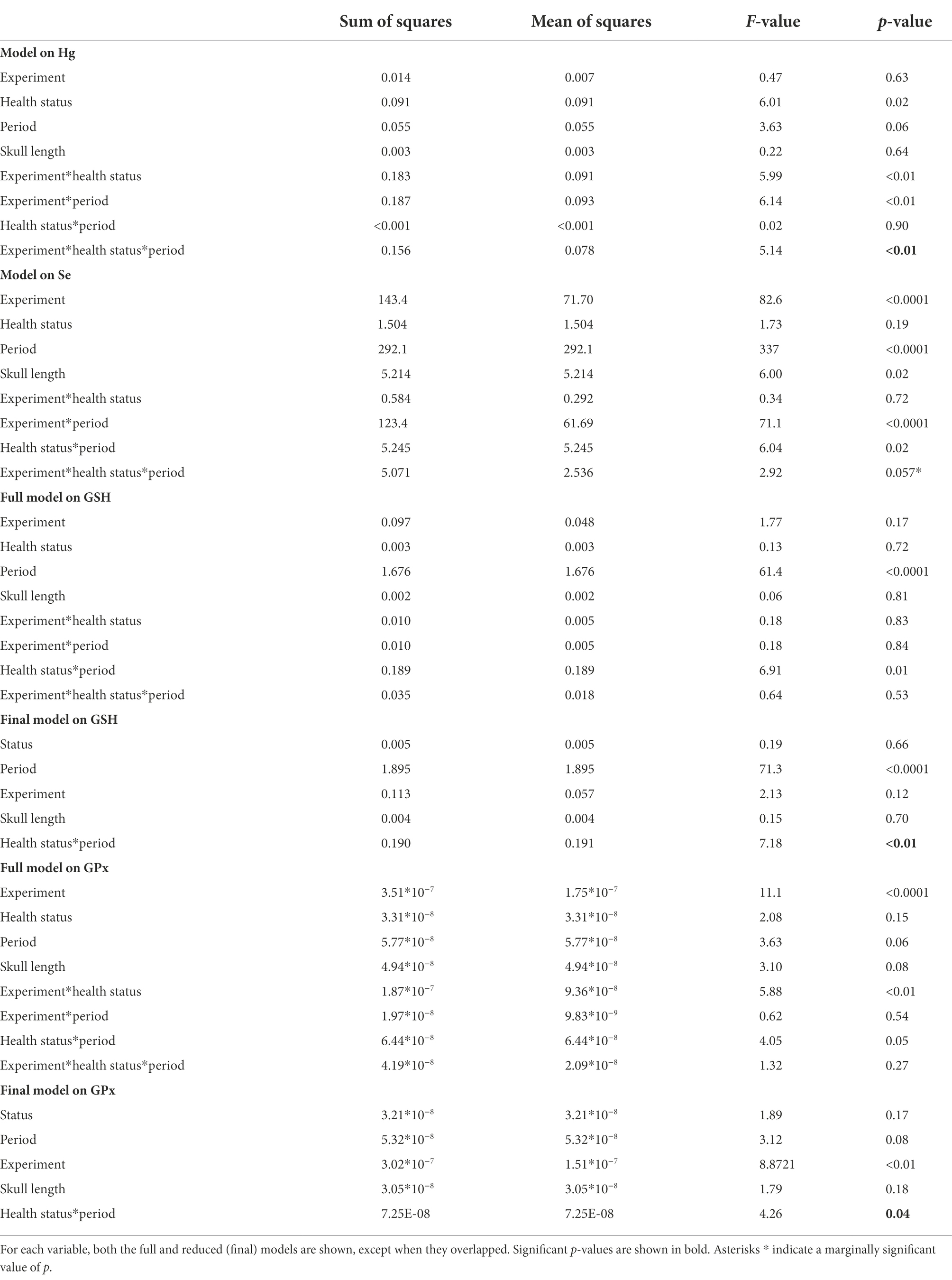
Table 1. Linear mixed models among groups divided based on the health status, the treatment received, and the experimental period.
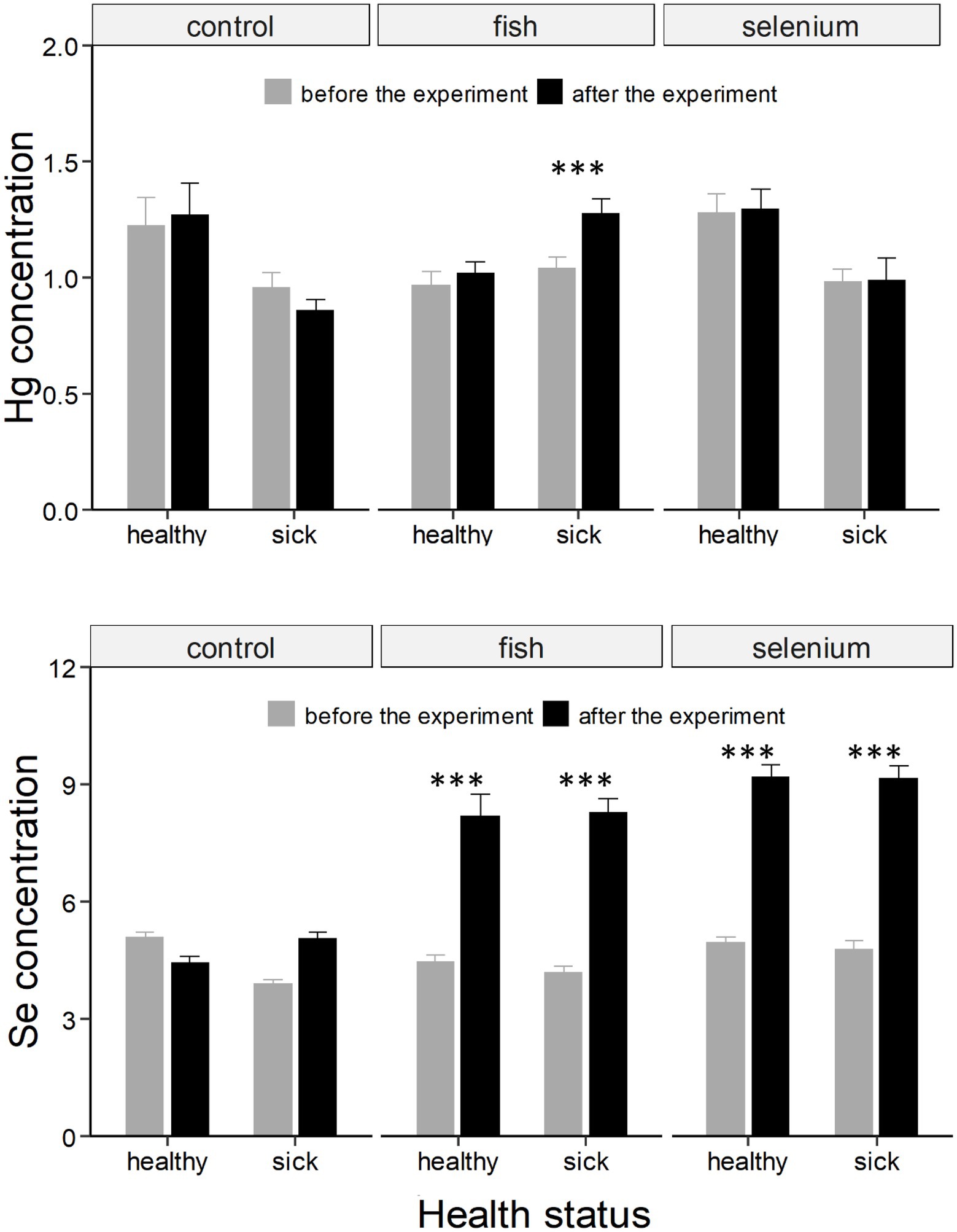
Figure 1. Hg and Se concentrations (expressed as μg g−1 dry weight ± SE) in chicks divided based on the health status and experimental group (control, fish supplemented, or Se supplemented). Asterisks * indicate a significant change in concentrations from “before the experiment” (grey column) to “after the experiment” (black column). ***, indicates a value of p < 0.001.
Both the food and Se supplementation experiments affected blood Se concentrations even if the three-way interaction was only marginally significant (F = 2.92, p = 0.057; Table 1), likely because Se strongly increased in all four experimental groups independently of the health status of chicks (all t > 8.12, all p < 0.001; Supplementary Table S1; Figure 1).
Effect of the experiments on oxidative status markers
The concentration of GSH showed an overall decline from the first to the second experimental period (Figure 2). There was a significant interaction between the health status and the expermental period (F = 7.18, p < 0.01; Table 1) showing that the drop in GSH concentrations was more pronounced in healthy than sick chicks (t = 7.47, p < 0.001 and t = 4.34, p < 0.001, respectively; Supplementary Table S1; Figure 2).
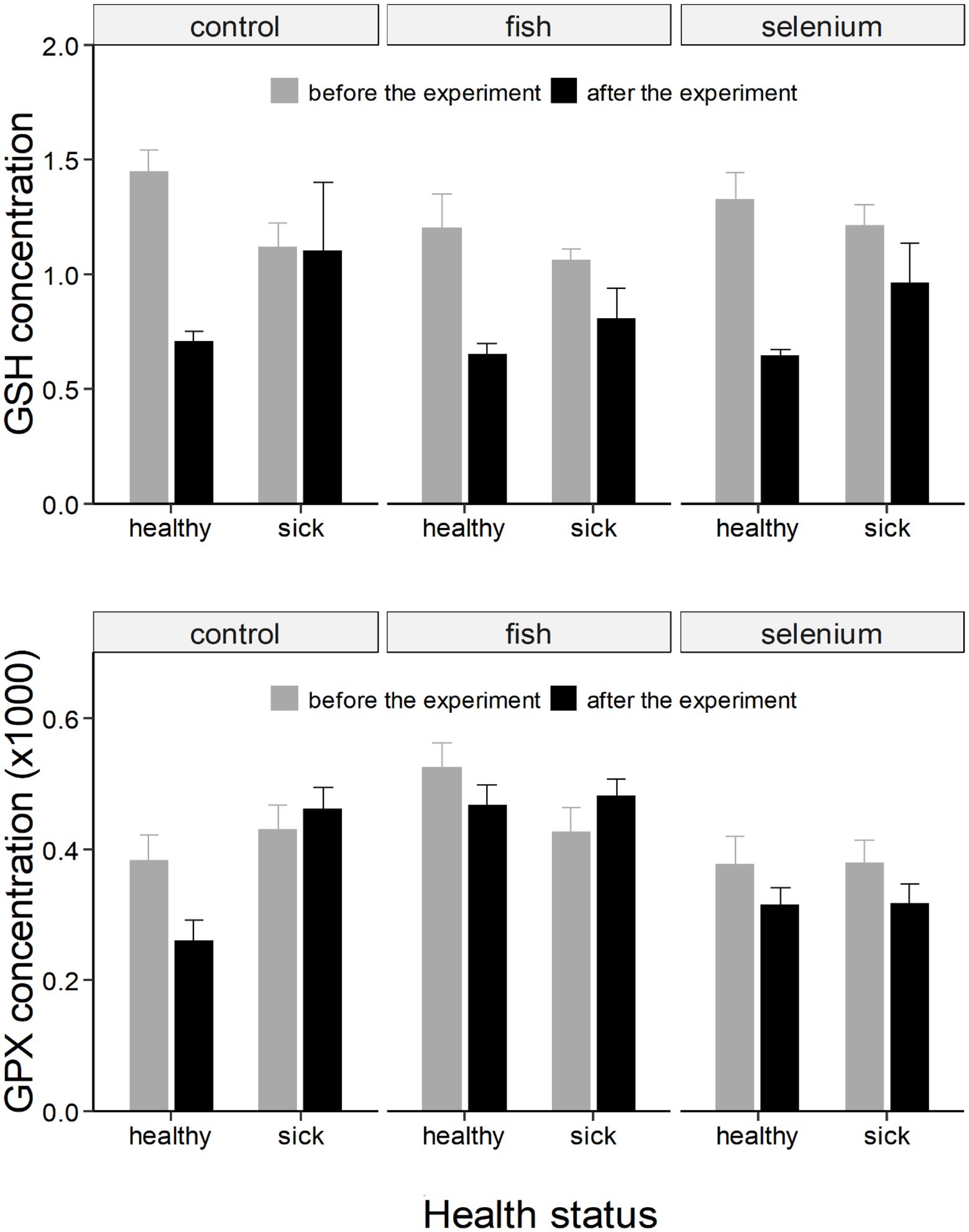
Figure 2. GSH (expressed as as μmol/g of fresh weight) and GPx (expressed as μmol NADPH/mg protein per minute) concentrations (± SE) in chicks divided based on the health status and experimental group (control, fish supplemented, or Se supplemented). Concentrations are further divided and represented as “before the experiment” (grey column) and “after the experiment” (black column). GPx concentrations were multiplied by 1,000 for an optimal graphic representation.
Similarly, there was a significant interaction between the health status and the experimental period when considering GPx (F = 4.26, p < 0.05; Table 1). The concentration of GPx decreased in healthy chicks (t = 2.56, p = 0.06; Supplementary Table S1; Figure 2) but did not change in sick chicks (t = 0.22, p = 0.99; Supplementary Table S1; Figure 2) from the first to the second experimental period.
Hg and Se concentrations during the progress of the disease
Control chicks divided based on the progress of the disease showed a different change in Hg and Se concentrations from the first to the second experimental period (F = 4.29, p < 0.01 and F = 10.11, p < 0.001, respectively; Table 2). Specifically, chicks that improved had a marginally significant decrease in Hg concentrations (t = 3.18, p = 0.05, Supplementary Table S2; Figure 3), and a strong increase in Se concentrations (t = −3.67, p = 0.01, Supplementary Table S2; Figure 3).
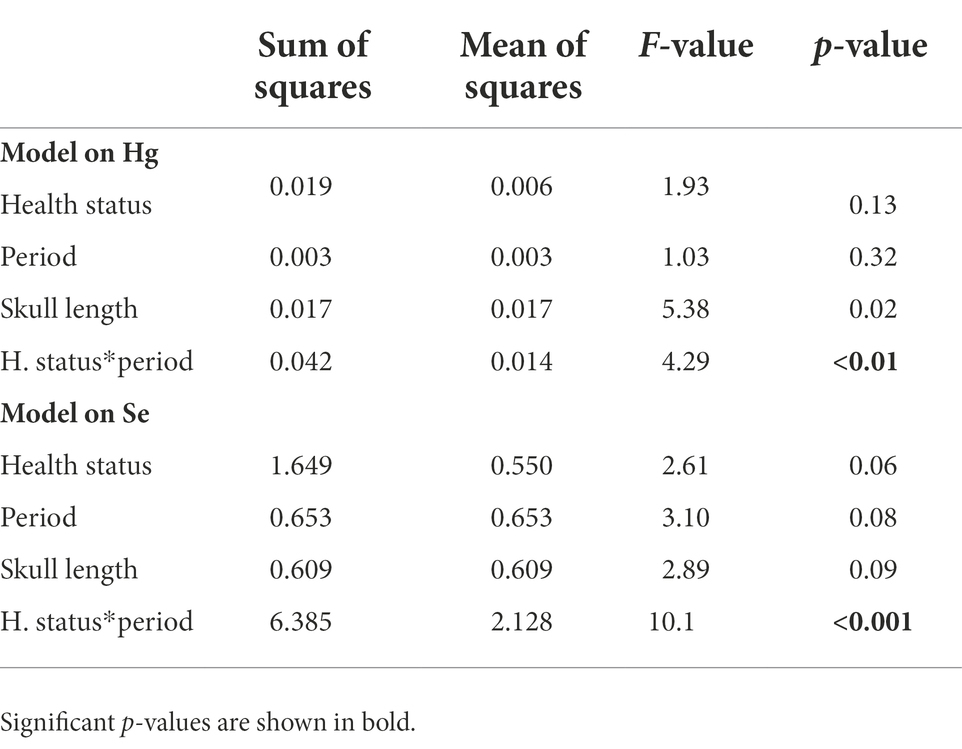
Table 2. Results of the linear mixed models run on chicks divided based on the progress of the disease (their change in status from the first to the second sampling period).
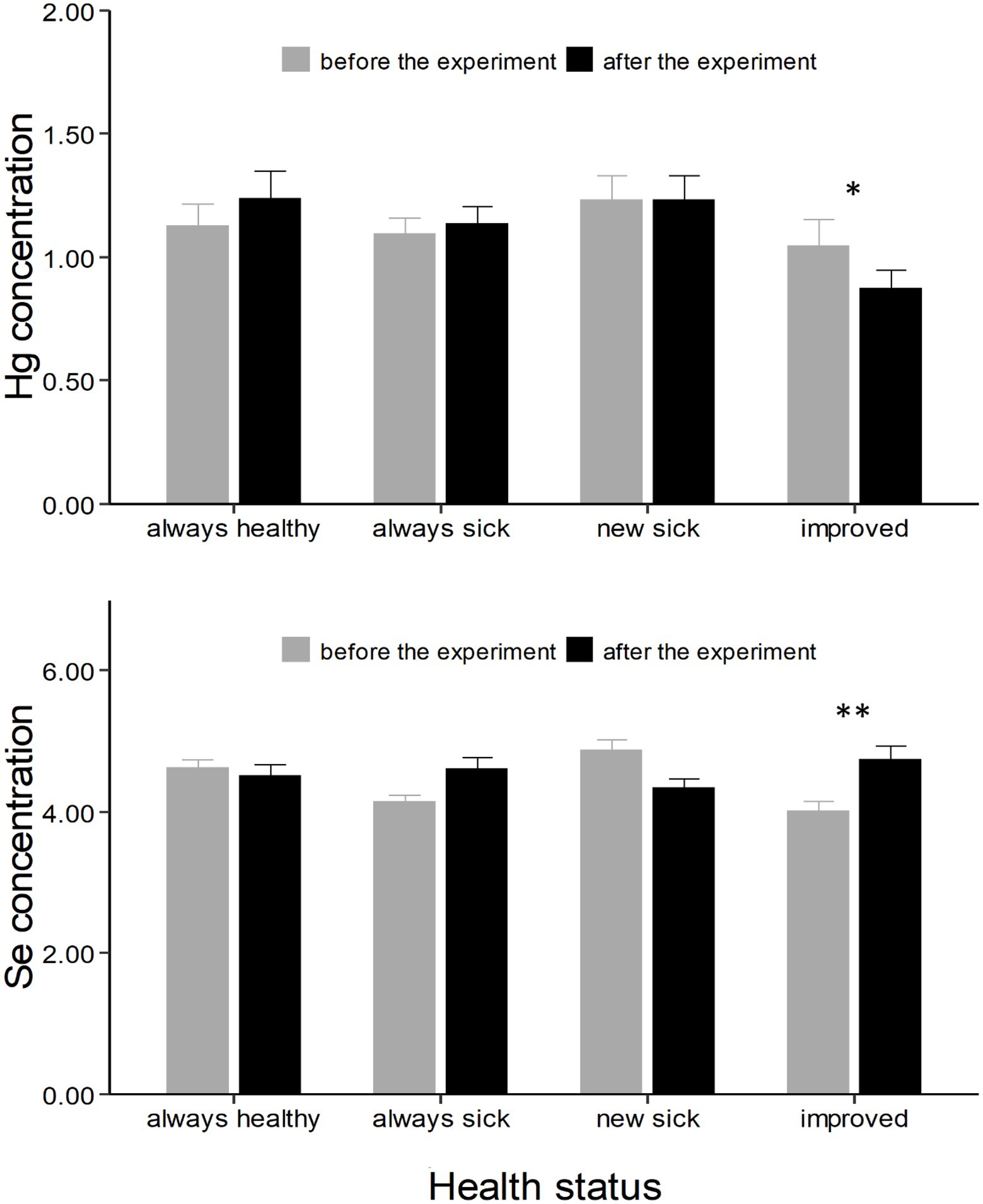
Figure 3. Hg and Se concentrations (expressed as μg g−1 dry weight ± SE) in chicks divided based on the progress of the disease (always healthy, always sick, new sick, and improved). Asterisks * indicate a significant change in concentrations from “before the experiment” (grey column) to “after the experiment” (black column). *, ** indicates a value of p < 0.05 and p < 0.01 respectively.
Discussion
Our study is the first to experimentally investigate the role of dietary Se on Hg concentrations and the physiological status of a wild vertebrate facing a viral disease. We found a significant difference in Se levels between chicks with and without clinical signs, which may suggest heterogeneity in the way chicks receive food from their parents. In contrast, we did not find any difference in Hg concentrations among chicks with or without clinical signs of the disease. The experimental part of our study further showed that administration of Se pills increased circulating Se in blood, but did not affect Hg nor the physiology of chicks.
Our previous work on frigatebirds in 2013 showed high variability in Hg concentration among chicks (ranging from 0.65 to 1.68 μg g−1; Sebastiano et al., 2016). We previously showed that other pollutants – which may represent confounding factors for the interpretation of the results (as described in Sebastiano et al., 2022b) – occur at low concentrations in the blood of frigatebirds (Sebastiano et al., 2017a), so that they should not pose a serious threat to this population. We therefore hypothesized that Hg concentrations might be one factor underlying the probability of disease outbreaks in this population. However, our results showed that chicks with or without visible clinical signs of the disease had similar Hg concentrations. On the contrary, chicks with visible clinical signs of the disease had significantly lower concentrations of Se in their blood. Because fish is the main dietary source of Se in seabirds, these results would suggest that healthy chicks might receive more food from their parents, which is not supported by our data (as the skull length was not significant in statistical analyses thus chicks were of the same body size).
It is however possible that sick birds received more Hg contaminated food, although they showed similar blood Hg concentration than healthy chicks. At high concentrations, the high affinity between Hg and Se would indeed lead to Hg binding to Se (Ralston and Raymond, 2010), with the consequent generation of mercuric selenide (HgSe), a non-toxic form in marine mammals and birds (Ikemoto et al., 2004). HgSe accumulates in muscles, kidneys, and the liver (Nigro and Leonzio, 1996; Manceau et al., 2021), thereby its formation may have reduced blood Hg concentrations in sick birds. This hypothesis would also explain why Se was lower in sick chicks, as Se would be also stored in those tissues with the excess of Hg, resulting in a deficiency of Se in the blood of sick birds. A way to test this hypothesis requires the quantification of Se and Hg in the soft tissues of both sick and healthy chicks. As birds are also able to excrete Hg into growing feathers (Dauwe et al., 2003), further investigations in smaller chicks before feathers have started growing, or the analyses of Hg in both blood and in feathers would also help to better clarify this aspect.
Previous work found that Se deficiency may selectively influence the susceptibility to viruses (experimentally tested on coxsackie viruses and herpes simplex 1; Gómez et al., 2001) and that Se-containing molecules may play a very effective role against cancer, and viral and bacterial infections (Fernandes and Gandin, 2015; Lenardão et al., 2018). Our Se supplementation experiment dramatically increased circulating Se into the blood, with Se concentrations in treated birds being almost double compared to control birds at the end of the experiment. However, higher concentrations of Se in blood were not able to decrease Hg concentrations in blood. One possible reason might be related to the growth stage of supplemented birds. If Se increases the rate at which Hg is excreted into growing feathers, reducing Hg concentrations in internal tissues, this will need to be tested in smaller chicks, as chicks included in the experiment were approximately 4 months old when we started the supplementation, thus their feathers were almost fully grown and there was no Hg remobilization from internal tissues.
Similarly to the Se supplementation experiment, supplementing chicks with extra food strongly increased the concentration of circulating Se in blood. Sick birds that received food also showed an increase in blood Hg concentrations at the end of the experiment. As described in Sebastiano et al. (2019), non-local and eviscerated fish – thus very low in Hg concentrations – was used, therefore we exclude the possibility that extra food increased Hg in blood, as also confirmed by the fact that this result was not found in healthy chicks supplemented with extra food. One possible explanation may be represented by the begging behavior of chicks. As begging calls reflect the degree of hunger in birds and modulate parental feeding rate (Klenova, 2015), healthy chicks which received extra food may have reduced their begging and therefore their intake of Hg-contaminated fish delivered by the parents.
Our results further show that neither the activity of GPx nor the concentration of GSH were influenced by the Se or the fish supplementation experiment. A previous long-term Se dietary supplementation trial in cows showed that Se content rapidly increased in the liver and plasma, while it took almost 2 months before it increased in red blood cells, suggesting that the time required for maximal assimilation is tissue-specific (Brennan et al., 2011), explaining why we did not find an effect on these markers. Specifically related to birds, experimental work on mallards Anas platyrhynchos also suggests a detrimental effect of Hg on antioxidant enzymes as GPx (Hoffman and Heinz, 1998), which may explain the observed general decrease in GPx and GSH concentrations in the present study. However, it remains unclear why this drop in GPx activity was more pronounced in healthy chicks. A possible explanation may be that healthy chicks downregulate the costly antioxidant machinery, while sick chicks keep it upregulated as it is needed to face the ongoing infection.
The relationship between the concentrations of Hg and that of Se is also relevant to describe Hg toxicity. Previous work on Guiana dolphins Sotalia guianensis in Southeastern Brazil showed that all Se was complexed as HgSe, implying that Se was not available for important biological functions (Manhães et al., 2021), and further suggesting a possible cumulative effect of Hg toxicosis and Se deficiency on the severity of morbillivirus infections in cetaceans (Manhães et al., 2021). Our results on Hg and Se concentrations seem to support this cumulative effect hypothesis (Sebastiano et al., 2022a). Indeed, when chicks were divided based on the progress of the disease, we found that chicks that improved – i.e. which exhibited a reduction in the visible clinical signs of the disease – showed an increase in Se concentrations while simultaneously showing a decrease of Hg concentrations in blood. As these results were obtained in control birds, which were not included in either experiments, the most likely hypothesis is that chicks improved because they received more food from their parents. This result would provide an explanation for the increased concentrations of Se in the blood of these chicks. As for the concomitant decrease in Hg concentrations, it is possible that by receiving more food, chicks grew faster and eliminated Hg in their feathers; or that adults shifted to a low Hg diet. As availability of food appears to be one relevant factor that can affect the probability of chicks to develop the disease or to survive, we suggest further work on this population to be refined with (i) stable isotope analyses, as the trophic position (reflected by nitrogen isotopes) and the feeding habitat (reflected by carbon isotopes) are important drivers of Hg contamination in this population (Sebastiano et al., 2017a); and (ii) tracking devices, as GPS and accelerometer data would provide fundamental insights on habitat selection, feeding behavior of adults, and the begging behavior of chicks.
Conclusion
Our multi-year correlative and experimental results suggest that food shortage might have a stronger impact than Hg toxicosis on the probability that viral outbreaks occur in our frigatebird population. This inference is corroborated by the fact that food supplementation protected all chicks from the occurrence of clinical signs (Sebastiano et al., 2019). On the contrary, Se supplementation did not protect chicks nor did it positively affect the physiological status of chicks.
Se is known to decrease Hg toxicity in various organisms, including birds (Hoffman and Heinz, 1998; Spiller, 2018). However, if chicks are also undergoing deficiency of Se in their diet due to food shortage – which increases physiological stress (Kitaysky et al., 2007) and impairs immune function (Gasparini et al., 2006) – the immune system of chick frigatebirds might remain depressed even after Se supplementation due to the ongoing nutritional stress.
Further studies are needed to clarify whether specific dietary molecules - as Se - i) increase the excretion rate of Hg into growing feathers, or ii) increase Hg deposition into other organs (e.g., liver) as mercuric selenide HgSe formations. This will provide fundamental knowledge on how birds can detoxify from Hg and if this detoxification mechanism would affect the vulnerability of chicks to the viral disease.
Data availability statement
The raw data supporting the conclusions of this article will be made available by the authors, without undue reservation.
Ethics statement
The animal study was reviewed and approved by Ethische Commissie Dierproeven — University of Antwerp.
Author contributions
MS, DC, and ME: conceptualization. MS, PB, and OC: methodology. MS: formal analysis, writing — original draft, and visualization. MS, ME, PB, OC, and DC: writing — review and editing. All authors contributed to the article and approved the submitted version.
Funding
CNRS-funded project SENTINEL (to OC), DEAL Guyane, the MNHN of Paris, the CEBC, the University of Antwerp, the FWO (Fonds Wetenschappelijk Onderzoek), the GEPOG, the CPER, the FEDER, and the King Leopold III Fund. MS was supported by a Marie Curie postdoctoral fellowship (SUPREME).
Acknowledgments
We thank the CNRS, DEAL Guyane, the MNHN of Paris, the CEBC, the LIENSs laboratory at University La Rochelle, the University of Antwerp, the FWO, the GEPOG, the Institut Pasteur de Guyane, and the King Leopold III Fund for funding, logistic support, and access to the Grand Connétable Nature Reserve. The authors are grateful to Carine Churlaud and Maud Brault-Favrou from the «Analyses Elémentaires» platform (LIENSs) for their support during trace element analysis. Thanks are due to the CPER (Contrat de Projet Etat-Région) and the FEDER (Fonds Européen de Développement Régional) for funding the AMA and ICPs of LIENSs laboratory. The Institut Universitaire de France (IUF) is acknowledged for its support to PB as a Senior Member. We are especially grateful to Grand Connétable reserve staff (Kévin Pineau, Alain Alcide, Jérémie Tribot) and to Jonathan Simon for their great help in the field. MS was supported by a Marie Curie postdoctoral fellowship (SUPREME). We thank the editor and two reviewers for providing valuable comments that helped us to improve the presentation of the results.
Conflict of interest
The authors declare that the research was conducted in the absence of any commercial or financial relationships that could be construed as a potential conflict of interest.
Publisher’s note
All claims expressed in this article are solely those of the authors and do not necessarily represent those of their affiliated organizations, or those of the publisher, the editors and the reviewers. Any product that may be evaluated in this article, or claim that may be made by its manufacturer, is not guaranteed or endorsed by the publisher.
Supplementary material
The Supplementary material for this article can be found online at: https://www.frontiersin.org/articles/10.3389/fevo.2022.963512/full#supplementary-material
References
Abba, Y., Hassim, H., Hamzah, H., and Noordin, M. M. (2015). Antiviral activity of resveratrol against human and animal viruses. Adv. Virol. 2015:184241. doi: 10.1155/2015/184241
Baker, R. E., Mahmud, A. S., Miller, I. F., Rajeev, M., Rasambainarivo, F., Rice, B. L., et al. (2022). Infectious disease in an era of global change. Nat. Rev. Microbiol. 20, 193–205. doi: 10.1038/s41579-021-00639-z
Beckett, G. J., and Arthur, J. R. (2005). Selenium and endocrine systems. J. Endocrinol. 184, 455–465. doi: 10.1677/joe.1.05971
Blaustein, A. R., Gervasi, S. S., Johnson, P. T. J., Hoverman, J. T., Belden, L. K., Bradley, P. W., et al. (2012). Ecophysiology meets conservation: understanding the role of disease in amphibian population declines. Philos. Trans. R. Soc. B, Biol. Sci. 367, 1688–1707. doi: 10.1098/rstb.2012.0011
Brennan, K. M., Burris, W. R., Boling, J. A., and Matthews, J. C. (2011). Selenium content in blood fractions and liver of beef heifers is greater with a mix of inorganic/organic or organic versus inorganic supplemental selenium but the time required for maximal assimilation is tissue-specific. Biol. Trace Elem. Res. 144, 504–516. doi: 10.1007/s12011-011-9069-y
Bustamante, P., González, A. F., Rocha, F., Miramand, P., and Guerra, A. (2008). Metal and metalloid concentrations in the giant squid Architeuthis dux from Iberian waters. Mar. Environ. Res. 66, 278–287. doi: 10.1016/j.marenvres.2008.04.003
Cunningham, A. A., Daszak, P., and Wood, J. L. N. (2017). One health, emerging infectious diseases and wildlife: two decades of progress? Philos. Trans. R. Soc. Lond. Ser. B Biol. Sci. 372:20160167. doi: 10.1098/rstb.2016.0167
Dauwe, T., Bervoets, L., Pinxten, R., Blust, R., and Eens, M. (2003). Variation of heavy metals within and among feathers of birds of prey: effects of molt and external contamination. Environ. Pollut. 124, 429–436. doi: 10.1016/S0269-7491(03)00044-7
de Thoisy, B., Lavergne, A., Semelin, J., Pouliquen, J. F., Blanchard, F., Hansen, E., et al. (2009). Outbreaks of disease possibly due to a natural avian herpesvirus infection in a colony of young magnificent Frigatebirds (Fregata magnificens) in French Guiana. J. Wildl. Dis. 45, 802–807. doi: 10.7589/0090-3558-45.3.802
Drotar, A., Phelps, P., and Fall, R. (1985). Evidence for glutathione peroxidase activities in cultured plant cells. Plant Sci. 42, 35–40. doi: 10.1016/0168-9452(85)90025-1
Fernandes, A. P., and Gandin, V. (2015). Selenium compounds as therapeutic agents in cancer. Biochim. Biophys. Acta Gen. Subj. 1850, 1642–1660. doi: 10.1016/j.bbagen.2014.10.008
Fisher, M. C., Henk, D. A., Briggs, C. J., Brownstein, J. S., Madoff, L. C., McCraw, S. L., et al. (2012). Emerging fungal threats to animal, plant and ecosystem health. Nature 484, 186–194. doi: 10.1038/nature10947
Gasparini, J., Roulin, A., Gill, V. A., Hatch, S. A., and Boulinier, T. (2006). In kittiwakes food availability partially explains the seasonal decline in humoral Immunocompetence. Funct. Ecol. 20, 457–463. doi: 10.1111/j.1365-2435.2006.01130.x
Gómez, R. M., Berría, M. I., and Levander, O. A. (2001). Host selenium status selectively influences susceptibility to experimental viral myocarditis. Biol. Trace Elem. Res. 80, 23–31. doi: 10.1385/BTER:80:1:23
Gomez, R. M., Solana, M. E., and Levander, O. A. (2002). Host selenium deficiency increases the severity of chronic inflammatory myopathy in Trypanosoma cruzi-inoculated mice. J. Parasitol. 88, 541–547. doi: 10.1645/0022-3395(2002)088[0541:HSDITS]2.0.CO;2
Hawkes, W. C., Kelley, D. S., and Taylor, P. C. (2001). The effects of dietary selenium on the immune system in healthy men. Biol. Trace Elem. Res. 81, 189–213. doi: 10.1385/BTER:81:3:189
Hoffman, D. J., and Heinz, G. H. (1998). Effects of mercury and selenium on glutathione metabolism and oxidative stress in mallard ducks. Environ. Toxicol. Chem. 17, 161–166. doi: 10.1002/etc.5620170204
Hoffmann, P. R., and Berry, M. J. (2008). The influence of selenium on immune responses. Mol. Nutr. Food Res. 52, 1273–1280. doi: 10.1002/mnfr.200700330
Ikemoto, T., Kunito, T., Tanaka, H., Baba, N., Miyazaki, N., and Tanabe, S. (2004). Detoxification mechanism of heavy metals in marine mammals and seabirds: interaction of selenium with mercury, silver, copper, zinc, and cadmium in liver. Arch. Environ. Contam. Toxicol. 47, 402–413. doi: 10.1007/s00244-004-3188-9
Kitaysky, A., Piatt, J., and Wingfield, J. (2007). Stress hormones link food availability and population processes in seabirds. Mar. Ecol.-Progr. Ser. 352, 245–258. doi: 10.3354/meps07074
Klenova, A. V. (2015). Chick begging calls reflect degree of hunger in three auk species (Charadriiformes: Alcidae). PLoS One 10:e0140151. doi: 10.1371/journal.pone.0140151
Lenardão, E. J., Santi, C., and Sancineto, L. (2018). “Bioactive Organoselenium compounds and therapeutic perspectives” in New Frontiers in Organoselenium compounds. eds. E. J. Lenardão, C. Santi, and L. Sancineto (Cham: Springer International Publishing), 99–143.
Manceau, A., Gaillot, A.-C., Glatzel, P., Cherel, Y., and Bustamante, P. (2021). In vivo formation of HgSe nanoparticles and Hg–Tetraselenolate complex from methylmercury in seabirds—implications for the Hg–Se antagonism. Environ. Sci. Technol. 55, 1515–1526. doi: 10.1021/acs.est.0c06269
Manhães, B. M. R., Santos-Neto, E. B., Tovar, L. R., Guari, E. B., Flach, L., Kasper, D., et al. (2021). Changes in mercury distribution and its body burden in delphinids affected by a morbillivirus infection: evidences of methylmercury intoxication in Guiana dolphin. Chemosphere 263:128286. doi: 10.1016/j.chemosphere.2020.128286
Martin, L. B. (2009). Stress and immunity in wild vertebrates: timing is everything. Gen. Comp. Endocrinol. 163, 70–76. doi: 10.1016/j.ygcen.2009.03.008
Martinet, V., and Blanchard, F. (2009). Fishery externalities and biodiversity: trade-offs between the viability of shrimp trawling and the conservation of frigatebirds in French Guiana. Ecol. Econ. 68, 2960–2968. doi: 10.1016/j.ecolecon.2009.06.012
Nigro, M., and Leonzio, C. (1996). Intracellular storage of mercury and selenium in different marine vertebrates. Mar. Ecol.-Progr. Ser. 135, 137–143. doi: 10.3354/meps135137
Polak-Juszczak, L., and Robak, S. (2015). Mercury toxicity and the protective role of selenium in eel, Anguilla anguilla. Environ. Sci. Pollut. Res. Int. 22, 679–688. doi: 10.1007/s11356-014-3382-x
Ralston, N. V., and Raymond, L. J. (2010). Dietary selenium's protective effects against methylmercury toxicity. Toxicology 278, 112–123. doi: 10.1016/j.tox.2010.06.004
Sabin, N. S., Calliope, A. S., Simpson, S. V., Arima, H., Ito, H., Nishimura, T., et al. (2020). Implications of human activities for (re)emerging infectious diseases, including COVID-19. J. Physiol. Anthropol. 39:29. doi: 10.1186/s40101-020-00239-5
Sebastiano, M., Bustamante, P., Costantini, D., Eulaers, I., Malarvannan, G., Mendez-Fernandez, P., et al. (2016). High levels of mercury and low levels of persistent organic pollutants in a tropical seabird in French Guiana, the magnificent frigatebird, Fregata magnificens. Environ. Pollut. 214, 384–393. doi: 10.1016/j.envpol.2016.03.070
Sebastiano, M., Bustamante, P., Eulaers, I., Malarvannan, G., Mendez-Fernandez, P., Churlaud, C., et al. (2017a). Trophic ecology drives contaminant concentrations within a tropical seabird community. Environ. Pollut. 227, 183–193. doi: 10.1016/j.envpol.2017.04.040
Sebastiano, M., Costantini, D., Eens, M., Pineau, K., Bustamante, P., and Chastel, O. (2022b). Possible interaction between exposure to environmental contaminants and nutritional stress in promoting disease occurrence in seabirds from French Guiana: a review. Reg. Environ. Chang. 22:63. doi: 10.1007/s10113-022-01914-2
Sebastiano, M., Eens, M., Abd Elgawad, H., Thoisy, B., Lacoste, V., Pineau, K., et al. (2017b). Oxidative stress biomarkers are associated with visible clinical signs of a disease in frigatebird nestlings. Sci. Rep. 7:1599. doi: 10.1038/s41598-017-01417-9
Sebastiano, M., Eens, M., Angelier, F., Pineau, K., Chastel, O., and Costantini, D. (2017c). Corticosterone, inflammation, immune status and telomere length in frigatebird nestlings facing a severe herpesvirus infection. Conser. Phys. 5:cow073-cow073. doi: 10.1093/conphys/cow073
Sebastiano, M., Eens, M., Messina, S., AbdElgawad, H., Pineau, K., Beemster, G. T. S., et al. (2018). Resveratrol supplementation reduces oxidative stress and modulates the immune response in free-living animals during a viral infection. Funct. Ecol. 32, 2509–2519. doi: 10.1111/1365-2435.13195
Sebastiano, M., Eens, M., Pineau, K., Chastel, O., and Costantini, D. (2019). Food supplementation protects magnificent frigatebird chicks against a fatal viral disease. Conserv. Lett. 12:e12630. doi: 10.1111/conl.12630
Sebastiano, M., Messina, S., Marasco, V., and Costantini, D. (2022a). Hormesis in ecotoxicological studies: a critical evolutionary perspective. Curr. Opin.Toxicol. 29, 25–30. doi: 10.1016/j.cotox.2022.01.002
Sinha, A. K., AbdElgawad, H., Giblen, T., Zinta, G., De Rop, M., Asard, H., et al. (2014). Anti-oxidative defences are modulated differentially in three freshwater teleosts in response to ammonia-induced oxidative stress. PLoS One 9:e95319. doi: 10.1371/journal.pone.0095319
Sørmo, E. G., Ciesielski, T. M., Øverjordet, I. B., Lierhagen, S., Eggen, G. S., Berg, T., et al. (2011). Selenium moderates mercury toxicity in free-ranging freshwater fish. Environ. Sci. Technol. 45, 6561–6566. doi: 10.1021/es200478b
Spiller, H. A. (2018). Rethinking mercury: the role of selenium in the pathophysiology of mercury toxicity. Clin. Toxicol. 56, 313–326. doi: 10.1080/15563650.2017.1400555
Stephanie, H., Edward, J. N., Thompson, R. C. A., and Stephanie, S. G. (2016). The relationship between physiological stress and wildlife disease: consequences for health and conservation. Wildl. Res. 43, 51–60. doi: 10.1071/WR15183
Wang, C., Wang, H., Luo, J., Hu, Y., Wei, L., Duan, M., et al. (2009). Selenium deficiency impairs host innate immune response and induces susceptibility to listeria monocytogenes infection. BMC Immunol. 10:55. doi: 10.1186/1471-2172-10-55
Keywords: seabirds, contaminants, wildlife disease, mercury, food shortage, essential nutrients
Citation: Sebastiano M, Eens M, Bustamante P, Chastel O and Costantini D (2022) Seabirds under environmental pressures: Food supplementation has a larger impact than selenium on chicks exposed to mercury and a viral disease. Front. Ecol. Evol. 10:963512. doi: 10.3389/fevo.2022.963512
Edited by:
Andrew James Jonathan MacIntosh, Kyoto University, JapanReviewed by:
Pedro Miguel Araújo, Coimbra, PortugalSalvatore Siciliano, Escola Nacional de Saúde Pública Sergio Arouca, Fundação Oswaldo Cruz (Fiocruz), Brazil
Copyright © 2022 Sebastiano, Eens, Bustamante, Chastel and Costantini. This is an open-access article distributed under the terms of the Creative Commons Attribution License (CC BY). The use, distribution or reproduction in other forums is permitted, provided the original author(s) and the copyright owner(s) are credited and that the original publication in this journal is cited, in accordance with accepted academic practice. No use, distribution or reproduction is permitted which does not comply with these terms.
*Correspondence: Manrico Sebastiano, bWFucmljby5zZWJhc3RpYW5vQHVhbnR3ZXJwZW4uYmU=