- Institute of Systematic and Evolutionary Botany, University of Zurich, Zurich, Switzerland
Volatile organic compounds are of great importance for communication within biological systems. For the experimental investigation of the functions of volatiles, methods for experimental manipulation are needed. Based on scent-release methods from pheromone research, we describe a simple and cheap method for scent manipulation using silicone rubber (i.e. a silicone septum). Volatile compounds are applied to the septum by soaking the septa for 1 h in a solvent/volatile solution. After removal of the septum from the solution and a drying period of 1 h to allow for evaporation of the solvent, the silicone emits the volatiles at a continuously decreasing rate for a minimum of 24 h. In this study, we measure the variability of the emission and quantify the emission of 22 common floral scent compounds at four different time points and in four different soaking concentrations. Our results show that for the same compound and soaking concentration, variability of volatile emission was low, showing the method leads to repeatable emission rates and can be fine-tuned to the desired emission rate. We provide a calculation tool based on linear regression to allow an experimenter to calculate soaking concentration for each of the 22 compounds to achieve a desirable emission from the septa, as well as to estimate the emission rate of a volatile from the septa after a given time.
Introduction
Volatile organic compounds (VOCs) are a large group of chemicals with a low boiling point and thus high vapor pressure and variable degrees of volatility. These volatiles play important roles in chemical communication within many biological systems. They act as synomones, kairomones, pheromones, or chemical defense compounds in a huge range of organisms (Birch and Haynes, 1982; Schiestl, 2010; Wyatt, 2014; Raguso, 2020). Despite a lot of research on the functions of volatiles in the last decades, much still needs to be learned. For volatiles, manipulative experiments are demanding because of the obvious difficulties in applying or removing compounds that evaporate constantly. Recently, protocols for gene silencing have been developed and successfully used in planta to remove specific volatiles from blends (Kessler et al., 2008, 2015). These approaches are powerful as they leave the biological matrix unchanged; however, they are technically demanding, expensive, and only applicable in a few model systems. Also, genetically manipulated plants cannot be assayed in the field in many countries, and in cases where such experiments are legal, labor-intensive flower emasculation may be needed to avoid the spread of mutant genotypes (Kessler et al., 2008). Other, more simple methods present volatiles dissolved in mineral oil or other solvents during bioassays (Shuttleworth and Johnson, 2010; Campbell et al., 2016). This method has the disadvantage that volatiles are emitted in a blend together with the solvent, making interactive effects between volatiles and solvent compounds unavoidable. Mineral oil consists of a blend of alkanes, which are known to serve manifold function in chemical communication, for example, sex pheromones or cast recognition signals in many insects (Howard, 1993; Ayasse et al., 2001), and thus should not be used in bioassays with insects. Applying diluted volatiles to filter paper or directly to flowers and testing those after the solvent has evaporated (Larue et al., 2016) comes with the problem of rapidly evaporating scent compounds, leading to strong changes in volatile concentrations during the test period. The use of nonadsorbing materials such as plastic beads for bioassays comes with similar problems of rapid evaporation and is only advisable for low-volatile compounds (Schiestl and Peakall, 2005; Vereecken et al., 2007).
A simple but efficient way of releasing semiochemicals in an experimental setup is to apply the compounds to a silicone rubber carrier (e.g., a gas chromatography septum), which slowly releases the VOCs to the ambient air. In pheromone research, septa of natural rubber are often used to release pheromone compounds for bioassay and application of pheromones in agricultural fields (Butler and McDonough, 1979; Baker et al., 1980; Heath et al., 1986). The silicone rubber we use in this study is a highly inert, stable, and resistant polymer consisting of a silicone (or polysiloxane) backbone, contrasting it to natural rubber which has a carbon backbone. Using silicone rubber as a carrier for volatile compounds has several advantages over alternative methods, such as an aerosol sprayer or sol-gel polymers (El-Sayed et al., 1999; Zada et al., 2009), as it is cheap, small size, and easy to use. Because septa are dried after soaking, volatiles can be assayed without any solvent being present, which is a great advantage over methods involving low-volatile solvents, such as mineral oil. Scent-releasing silicone septa have already successfully been used for many experiments, proving its versatility for many biological questions (see Discussion for more details).
In this study, we provide data on emission rates of 22 common floral scent compounds (Table 1) from commercially available silicon septa, at different concentrations and at different time points. Based on this data, we provide a calculation tool based on linear regression that allows the calculation of soaking concentration and emission rates after a given time, for all 22 compounds investigated. We did not test different solvents or different temperatures (all values are for 20°C), despite these parameters may influence evaporation rates. Thus, despite our tool may not provide exact evaporation rates for any experimental parameters, we hope that our data can serve as a workable protocol for some experiments or provide a starting point for researchers that may wish to fine-tune the methodological parameters of their experimental needs.
Materials and methods
As septa, we used gray gas chromatography (GC) inlet silicone rubber septa (GR-2), with a 5-mm diameter (Supelco, Bellefonte PA, USA, product no. 20712). Twenty-two volatiles were quantified for their emission rates from these septa by headspace sorption followed by GC-MS analysis. For soaking septa, each compound was prepared in four concentrations: 1, 10, 100, and 1,000 μg ml−1, in dichloromethane (highperformance liquid chromatography (HPLC) grade) as solvent. For an initial assessment of the variability of the emission rates, five silicone rubber septa, and afterward for the main experiment, two to three septa were soaked in each solution for 1 h in 4 ml glass vials at 20°C. After soaking, the septa were removed from the solution and air-dried in a fume hood on a metal grid for 1 h (at 20 ± 1°C). Emission of volatiles from septa was measured directly after drying (0), as well as 1, 4, and 24 h after drying. To do so, one septum was positioned on a metal grid in a glass cylinder (Figure 1, diameter 30 mm), which was closed with a polytetrafluoroethylene (PTFE) clasp. Volatiles were collected for 30 min from inside the glass cylinder via a glass tube attached to the cylinder (Figure 1) using glass tubes (external diameter: 4 mm; inner diameter 2.3 mm) filled with 20 mg Tenax® TA (60/80 mesh; Supelco) attached to a vacuum pump (type DC06/04/20F, Fürgut, Tannheim, Germany). The incoming air was cleaned with a charcoal filter and fed into the upper arm of the glass cylinder, representing a largely closed system (push–pull system) of airflow at a rate of 200 ml min−1. Glass vials were previously coated with Sigmacote? siliconizing reagent (Sigma-Aldrich Co. LLC) followed by washing with acetone and water, and oven dried at 60 °C for several hours before use. Septa were stored at room temperature (20 ± 1°C) for the total duration of the experiment.
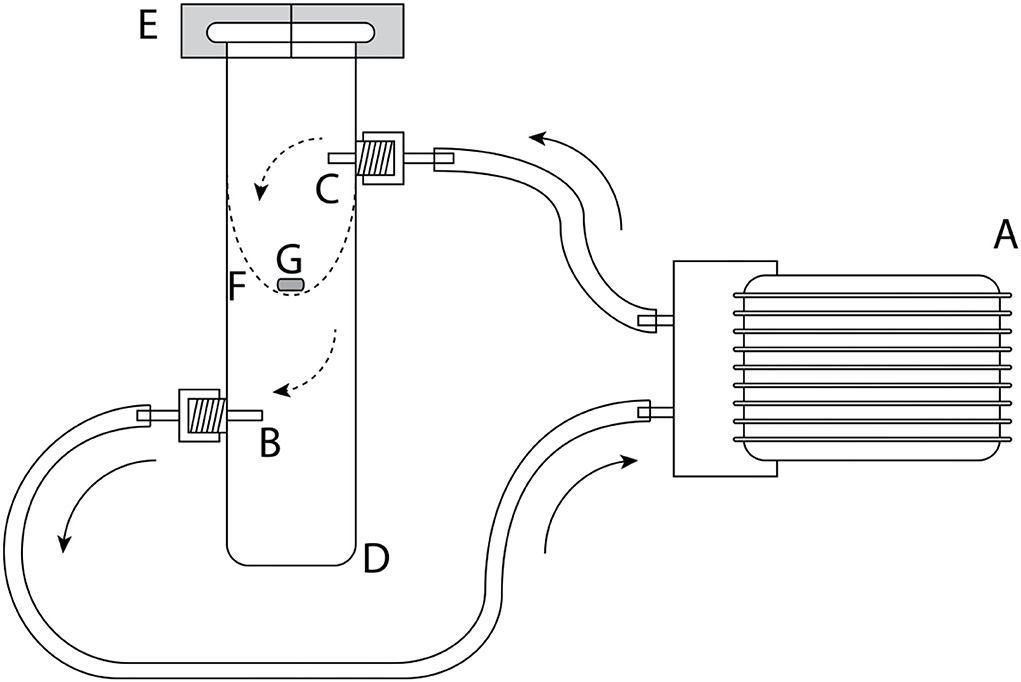
Figure 1. Experimental setup for measuring emission rates for different volatile organic compounds. (A) Airpump, (B) Tenax® TA filled glass tube, (C) air filter filled with activated charcoal, (D) glass cylinder, (E) polytetrafluoroethylene (PTFE) clasps, (F) metal grid for holding, and the (G) silicone septa. Arrows indicate the direction of airflow.
Volatile compounds included in the experiment were chosen from a list of floral volatiles of the genus Lithophragma (Saxifragaceae), representing a rich blend of floral volatiles (Friberg et al., 2013). For details of the compounds (Table 1).
VOC analysis
For the analysis of volatiles, gas chromatography with mass selective detection (GC-MSD) was used. Samples were injected into a GC (Agilent 6890 N; Agilent Technologies, Santa Clara, CA, USA) using a Gerstel Thermal Desorption Unit (TDU; Gerstel, Mühlheim, Germany) with a Gerstel Cooled Injection System (CIS-4). Although we used injection by thermodesorption, solvent-based injection methods should be equally suitable. For the thermal desorption of the trapped volatiles, the TDU containing the Tenax® TA filters was heated from 30°C to 240 °C at a rate of 60°C min−1 and held at a final temperature for 5 min in solvent venting desorption mode (delay time: 0.50 min; initial time: 1.00 min; Peltier cooling with UPC plus; TDU transfer temp: 300°C; transfer temp mode: fixed). The CIS was used in solvent vent mode (vent pressure: 7.6 psi; vent time: 0.01 min; purge flow: 60.0 ml/min; purge time: 1.51 min) and was cooled with liquid nitrogen to −150°C for cryo-trapping of eluting compounds from the filters in the TDU. For the introduction of the sample onto the column, the CIS was heated to 250°C at a rate of 12°C s−1, and the final temperature was held for 3 min. The GC was equipped with an Agilent HP-5 column (0.25 mm ID, 0.25 μm film thickness, 15 m length), and helium was used as carrier gas at a flow rate of 2 ml min−1. The GC oven program was 1 min isothermal at 50°C, then 10°C min−1 to 250°C and finally 6 min at 250°C. Compound determination and quantification were completed using a mass selective detector (Agilent MSD 5975). Compounds were identified by comparing the spectra obtained from the samples with those from a reference collection (National Institute of Standards and Technology (NIST) mass spectral library). Agilent MSD ChemStation (version E.02.02.1431, Agilent Technologies, Inc.) was used for quantitation. For the quantitation database, calibration samples for all studied compounds at three different amounts (1, 10, and 100 ng) dissolved in dichloromethane were analyzed on the GC-MSD system using the same thermal desorption setup as with the volatile samples.
Statistical analysis
For all compounds, soaking concentrations, and time points after drying, we calculated the coefficient of variation in emission rate by dividing the standard deviation by the mean. We used linear regression to test for the association between soaking concentration and emission rate at time point 0 (after solvent evaporation, i.e., 1 h after removal of septa from the solvent), as well as the association between emission rate and time for all of the soaking concentrations. For the latter association, volatile emission showed an exponential decay with time, thus, ln transformed values were used for linear regressions.
Results
We document all emission rates from septa for all compounds in Supplementary Table S1 (all values) and Supplementary Table S2 (mean values per soaking concentration). The variability in emission within compounds was low, especially for higher soaking concentrations (Figure 2, Supplementary Table S2). For example, for benzaldehyde, coefficients of variation in scent emission across time points were 0.31, 0.08, 0.10, and 0.16 for soaking concentrations 1, 10, 100, and 1,000 μg ml−1, respectively (Supplementary Table S2). The compound with the lowest mass, benzaldehyde (106 g/mol) had a high initial emission of 1.7 μg/l (20.8 μg/h), and the one with the highest mass, benzyl butyrate (178 g/mol) showed a comparably low emission of 0.3 μg/l (3.0 μg/h) at the highest concentration used. Some compounds, especially those with higher boiling points (~ above 510 K), showed an unexpected temporal volatile emission pattern for the strongest concentrations used. Those compounds (e.g., eugenol) showed a marked initial increase in the emission rate, with the expected decay in emission occurring only after the first few hours (Supplementary Table S2).
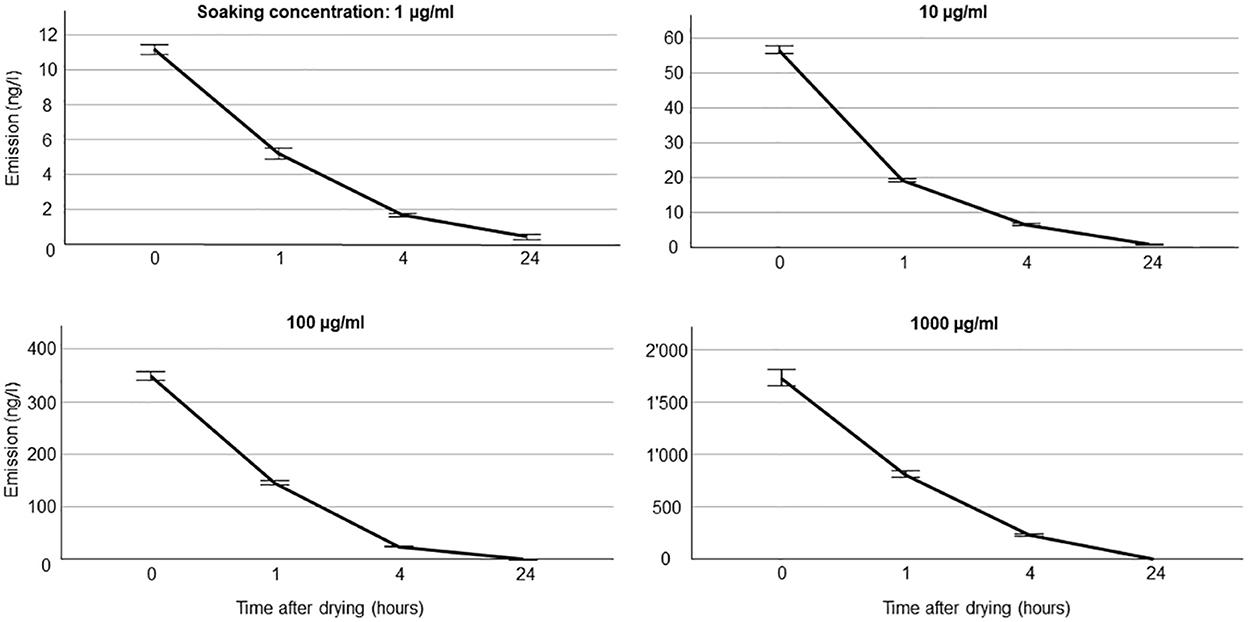
Figure 2. Volatile emission rate from silicone septa for benzaldehyde. Five trials were done for each soaking concentration and time points after drying. For volatile collection, a sampling rate of 200 ml min−1 (=12 l/h) was used, thus, to convert units into ng/h, amounts should be multiplied by 12.
Our statistical analysis with linear regression showed that both the soaking concentration and emission at t0 (after drying of the septa) and the emission rate and time after the start of the experiment had a highly significant association, and a linear association explained most of the variance, with most R2 above 0.9 (Supplementary Tables S3, S4). For two compounds, methyl 2-methoxybenzoate and benzyl butyrate, the association between emission rate and time was not significant (Supplementary Table S4). These results suggest highly predictable volatile emissions with given soaking concentrations and time after soaking for most of the tested compounds in this study. To enable researchers to find appropriate soaking concentrations for their desired emission rates, and to let them assess the emission after a given amount of time, we have built a calculation tool allowing to assess these parameters, based on our regression analyses (Supplementary Table S1).
Discussion
Using correct amounts in bioassays is important because odor concentration can change the perception of volatiles as odors (Wright et al., 2005) and thus influences the attractiveness of an odor bouquet. Our experiments showed that the silicone septa method can be used to achieve reproducible volatile emission rates, as shown by the low variability of emission, and a highly predictable change in emission rate with decreasing concentration in the soaking solution and decline in emission over time. Only a few compounds showed an unexpected increase in emission after some time, but only at the highest concentration used. We believe that this pattern of emission is an artifact of the glass vials used for scent collection. At the start of the experiment, compounds may have been adsorbed by the glass surface and thus retarded, and fewer amounts were collected than were actually emitted. After the glass surface had been saturated, the entire amount of the emitted volatile finally exited through the tube and was collected; hence, the increase in collected volatiles amount after a given retard time.
In terms of predicting emission amounts from septa, concentration in the soaking solution, and time after soaking, were very good predictors with linear and exponential regression. Our calculation tool, based on the regression analysis, may be helpful for researchers to apply soaking concentrations for desirable volatile emission amounts, and to estimate the change in emission over time. It may also serve as a starting point to determine the experimental conditions for tests with compounds that we did not test, such as sesquiterpenes, or for compound blends.
The artificial scent release method described in this study has already proven its versatility for a range of biological questions and experimental approaches in the context of plant–insect communication. Small silicone septa can be easily added to sticky traps or to real or artificial flowers (or any other items), they can also be used in an experimental maze. For example, Huber et al. (2005) and Steinebrunner et al. (2008) used scent-emitting septa placed on sticky traps to catch pollinator insects and fungi-visiting flies in the field over several days, using synthetic copies of previously identified electroantennographically (EAD) active volatiles. Knauer et al. (2018) tested the attractiveness of individual floral compounds of Biscutella laevigata (Brassicaceae) flowers for crab spiders using Y-tube bioassays with scented silicone septa in field experiments. Scent (β-ocimene) emitting septa were also directly applied to Biscutella flowers to test their attractiveness to bee pollinators. Cozzolino et al. (2015) added scented silicone septa to Silene latifolia (Caryophyllaceae) inflorescences to mimic and examine the effect of increased emission of two volatiles after an herbivore attack.
A powerful feature of the septa method is the possibility to relatively precisely adjust the amount of emitted scent, by varying the concentration of scent in the soaking solution. Knauer and Schiestl (2015) made use of this opportunity by adding different amounts of individual scent compounds to artificial flowers that held variable amounts of sugar reward in a greenhouse experiment, to create a population of honestly and dishonestly signaling flowers, and examine the learning behavior of bumblebees in response to them. Desurmont et al. (2020) used scented septa to add different amounts of floral volatile blends to herbivore-invested plants, to test the interference of variable amounts of floral volatiles with parasitoid-wasp attraction in the wind tunnel. Schiestl et al. (2014) tested the attractiveness of floral volatile blends of herbivore-infested and noninfested Brassica rapa plants to bumblebees in flight cage experiments. They used septa to mimic the low emission of infested- and the higher emission of noninfested plants. Using precise emission of a scent also allows the manipulation of natural scent variability. As an example, Salzmann et al. (2007) added uniformly scented septa to orchid inflorescences to mask their intraspecific variability in scent and to test the fitness value of natural fragrance variation, and Balbuena et al. (2022) added linalool-emitting septa to polymorphic Oenothera flowers to test the effect of linalool emission on pollinator attraction and oviposition by herbivores. Another study tested the importance of interspecies variability in two species of Silene; Waelti et al. (2008) added phenylacetaldehyde emitting from septa to the species that normally lacks this compound, to test its importance on ethological floral isolation between two species.
Overall, we feel the scented silicone septa method has great potential for research in various aspects of chemical ecology research. It allows for solvent-free testing of scent compounds, application of precise amounts, and an easy adding/removing of a scent source to any test item.
Data availability statement
The original contributions presented in the study are included in the article/Supplementary material, further inquiries can be directed to the corresponding author.
Author contributions
FH performed the experiments. FS and FH designed the experiments, analyzed the data, and wrote the manuscript. Both authors contributed to the article and approved the submitted version.
Funding
The research of FS is supported by the Swiss National Science Funds (SNF grant no. 31003A_172988).
Acknowledgments
We thank Andreas Ruckstuhl (ZHAW) and Dieter Ramseier (ETHZ) for discussing the data, methods, and previous versions of the manuscript.
Conflict of interest
The authors declare that the research was conducted in the absence of any commercial or financial relationships that could be construed as a potential conflict of interest.
Publisher's note
All claims expressed in this article are solely those of the authors and do not necessarily represent those of their affiliated organizations, or those of the publisher, the editors and the reviewers. Any product that may be evaluated in this article, or claim that may be made by its manufacturer, is not guaranteed or endorsed by the publisher.
Supplementary material
The Supplementary Material for this article can be found online at: https://www.frontiersin.org/articles/10.3389/fevo.2022.958982/full#supplementary-material
References
Ayasse, M., Paxton, R. J., and Tengo, J. (2001). Mating behavior and chemical communication in the order hymenoptera. Annu. Rev. Entomol. 46, 31–78. doi: 10.1146/annurev.ento.46.1.31
Baker, T. C., Carde, R. T., and Miller, J. R. (1980). Oriental fruit moth (Lepidoptera, Tortricidae) pheromone component emission rates measured after collection by glass surface adsorption. J. Chem. Ecol. 6, 749–758. doi: 10.1007/BF00990399
Balbuena, M. S., Broadhead, G. T., Dahake, E., Barnett, A., Vergara, M., Skogen, K. A., et al. (2022). Mutualism has its limits: consequences of asymmetric interactions between a well-defended plant and its herbivorous pollinator. Philos. Trans. R. Soc. 377. doi: 10.1098/rstb.2021.0166
Butler, L. I., and McDonough, L. M. (1979). Insect sex-pjheromones - evaporation rates of acetates from natural-rubber septa. J. Chem. Ecol. 5, 825–837. doi: 10.1007/BF00986567
Campbell, D. R., Jurgens, A., and Johnson, S. D. (2016). Reproductive isolation between Zaluzianskya species: the influence of volatiles and flower orientation on hawkmoth foraging choices. New Phytol. 210:333–342. doi: 10.1111/nph.13746
Cozzolino, S., Fineschi, S., Litto, M., Scopece, G., Trunschke, J., and Schiestl, F. P. (2015). Herbivory increases fruit set in silene latifolia: a consequence of induced pollinator-attracting floral volatiles? J. Chem. Ecol. 41, 622–630. doi: 10.1007/s10886-015-0597-3
Desurmont, G. A., von Arx, M., Turlings, T. C. J., and Schiestl, F. P. (2020). Floral Odors Can Interfere With the Foraging Behavior of Parasitoids Searching for Hosts. Front. Ecol. Evolut. 8. doi: 10.3389/fevo.2020.00148
El-Sayed, A., Godde, J., and Arn, H. (1999). Sprayer for quantitative application of odor stimuli. Environ. Entomol. 28:947–953. doi: 10.1093/ee/28.6.947
Friberg, M., Schwind, C., Raguso, R. A., and Thompson, J. N. (2013). Extreme divergence in floral scent among woodland star species (Lithophragma spp.) pollinated by floral parasites. Ann. Bot. 111:539–550. doi: 10.1093/aob/mct007
Heath, R. R., Teal, P. E. A., Tumlinson, J. H., and Mengelkoch, L. J. (1986). Prediction of release ratios of multicomponent pheromones from rubber septa. Pages 2133–2143. doi: 10.1007/BF01020315
Howard, R. W. (1993). Cuticular Hydrocarbons and Chemical Communication. in Insect lipids: chemistry, biochemistry and biology, Stanley-Samuelson, D. W., and Nelson, D. R., editors. University of Nebraska Press. p. 179–226
Huber, F. K., Kaiser, R., Sauter, W., and Schiestl, F. P. (2005). Floral scent emission and pollinator attraction in two species of Gymnadenia (Orchidaceae). Oecologia. 142, 564–575. doi: 10.1007/s00442-004-1750-9
Kessler, D., Gase, K., and Baldwin, I. T. (2008). Field experiments with transformed plants reveal the sense of floral scents. Science. 321:1200–1202. doi: 10.1126/science.1160072
Kessler, D., Kallenbach, M., Diezel, C., Rothe, E., and Murdock, M. (2015). How scent and nectar influence floral antagonists and mutualists. Elife. doi: 10.7554/eLife.07641.009
Knauer, A. C., Bakhtiari, M., and Schiestl, F. P. (2018). Crab spiders impact floral-signal evolution indirectly through removal of florivores. Nat. Commun. 9. doi: 10.1038/s41467-018-03792-x
Knauer, A. C., and Schiestl, F. P. (2015). Bees use honest floral signals as indicators of reward when visiting flowers. Ecol. Lett. 18, 135–143. doi: 10.1111/ele.12386
Larue, A.-A. C., Raguso, R. A., and Junker, R. R. (2016). Experimental manipulation of floral scent bouquets restructures flower-visitor interactions in the field. J. Anim. Ecol. 85, 396–408. doi: 10.1111/1365-2656.12441
Raguso, R. (2020). Behavioral responses to floral scent: experimental manipulations and multimodal plant-pollinator communication. in Pichersky, N. D. E. (ed). Biology of Plant Volatiles. CRC Press, Taylor and Francis. p. 297–325. doi: 10.1201/9780429455612-19
Salzmann, C. C., Nardella, A. M., Cozzolino, S., and Schiestl, F. P. (2007). Variability in floral scent in rewarding and deceptive orchids: the signature of pollinator-imposed selection? Ann. Bot. 100:757–765. doi: 10.1093/aob/mcm161
Schiestl, F. P. (2010). The evolution of floral scent and insect chemical communication. Ecol. Lett. 13, 643–656. doi: 10.1111/j.1461-0248.2010.01451.x
Schiestl, F. P., Kirk, H., Bigler, L., Cozzolino, S., and Desurmont, G. A. (2014). Herbivory and floral signaling: phenotypic plasticity and trade-offs between reproduction and indirect defense. New Phytol. 203, 257–266. doi: 10.1111/nph.12783
Schiestl, F. P., and Peakall, R. (2005). Two orchids attract different pollinators with the same floral odour compound: ecological and evolutionary implications. Funct. Ecol. 19:674–680. doi: 10.1111/j.1365-2435.2005.01010.x
Shuttleworth, A., and Johnson, S. D. (2010). The missing stink: sulphur compounds can mediate a shift between fly and wasp pollination systems. Proc. Royal Soc. 277, 2811–2819. doi: 10.1098/rspb.2010.0491
Steinebrunner, F., Twele, R., Francke, W., Leuchtmann, A., and Schiestl, F. P. (2008). Role of odour compounds in the attraction of gamete vectors in endophytic Epichloe fungi. New Phytol. 178, 401–411. doi: 10.1111/j.1469-8137.2007.02347.x
Vereecken, N. J., Mant, J., and Schiestl, F. P. (2007). Population differentiation in female sex pheromone and male preferences in a solitary bee. Behav. Ecol. Sociobiol. 61, 811–821. doi: 10.1007/s00265-006-0312-z
Waelti, M. O., Muhlemann, J. K., Widmer, A., and Schiestl, F. P. (2008). Floral odour and reproductive isolation in two species of Silene. J. Evol. Biol. 21, 111–121. doi: 10.1111/j.1420-9101.2007.01461.x
Wright, G. A., Thomson, M. G. A., and Smith, B. H. (2005). Odour concentration affects odour identity in honeybees. Proc. Royal Soc. 272, 2417–2422. doi: 10.1098/rspb.2005.3252
Wyatt, T. D. (2014). Fight or Flight: Alarm Pheromones and Cues. Cambridge: Cambridge Univ Press. doi: 10.1017/CBO9781139030748.010
Keywords: floral scent, pollination, volatile, chemical ecology, bioassay, herbivore
Citation: Huber FK and Schiestl FP (2022) Scent releasing silicone septa: A versatile method for bioassays with volatiles. Front. Ecol. Evol. 10:958982. doi: 10.3389/fevo.2022.958982
Received: 01 June 2022; Accepted: 05 September 2022;
Published: 29 September 2022.
Edited by:
Ping Wen, Xishuangbanna Tropical Botanical Garden (CAS), ChinaReviewed by:
Robert A. Raguso, Cornell University, United StatesStefan Schulz, Technische Universitat Braunschweig, Germany
Copyright © 2022 Huber and Schiestl. This is an open-access article distributed under the terms of the Creative Commons Attribution License (CC BY). The use, distribution or reproduction in other forums is permitted, provided the original author(s) and the copyright owner(s) are credited and that the original publication in this journal is cited, in accordance with accepted academic practice. No use, distribution or reproduction is permitted which does not comply with these terms.
*Correspondence: Florian P. Schiestl, Zmxvcmlhbi5zY2hpZXN0bEBzeXN0Ym90LnV6aC5jaA==