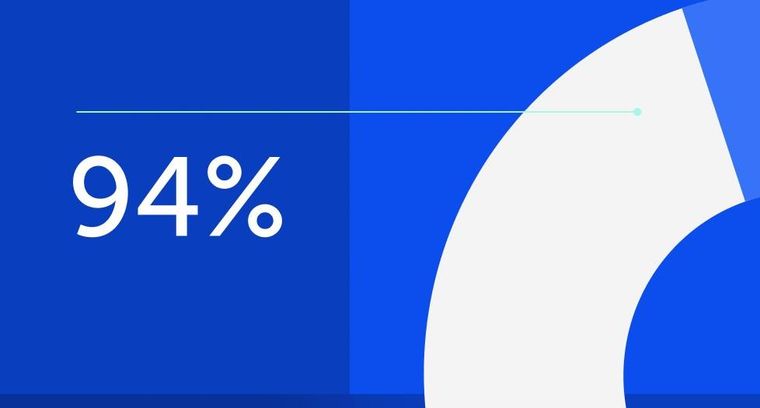
94% of researchers rate our articles as excellent or good
Learn more about the work of our research integrity team to safeguard the quality of each article we publish.
Find out more
ORIGINAL RESEARCH article
Front. Ecol. Evol., 12 August 2022
Sec. Conservation and Restoration Ecology
Volume 10 - 2022 | https://doi.org/10.3389/fevo.2022.956903
This article is part of the Research TopicDisturbance, Resilience and Restoration of WetlandsView all 20 articles
The ice cover in winter as a physical barrier and duration would profoundly impact on changes in endogenous metal loading, migration, and transformation. Although a gradual reduction in duration and occurrence of lake ice cover in boreal lake ecosystems has been confirmed, little attention to the ice-covered period is received compared to open water studies. In this study, novel-developed diffusive gradients in thin films (DGT, ZrO-Chelex) probes were deployed to obtain the in-situ and high-resolution information on metals (Cu, Zn, Pb, Mn, Cd, Cr, and As) at the sediment-water interface (SWI) in a seasonal ice-covered lake, Chagan Lake. In addition, “source-sink” characteristics of each metal related to their endogenous release were determined based on Fick’s first law. Concentrations of labile metals at the SWI demonstrated significant spatial heterogeneity, peaking exactly below the SWI. Compared with other similar studies, concentrations of Pb (0.55 μg/L), Cr (0.58 μg/L), and As (2.4 μg/L) were a little higher even under-ice than that in other freshwater rivers and lakes, indicating potential pollution due to the agricultural intensification and petroleum extraction. The apparent diffusive fluxes suggested that sediments acted as a sink for Pb (−0.01 mg m–2 day– 1), Cr (−2.37 mg m–2 day–1), and Cd (−0.1 mg m–2 day–1), diffusing from the overlying water into the sediment, while Cu (0.12 mg m–2 day–1), Zn (0.75 mg m–2 day–1), Mn (15.89 mg m–2 day–1), and As (2.12 mg m–2 day–1) as a source from sediments into the overlying water. Dissolved oxygen was the principal factor (79.5%, P = 0.032), determining the variation of the available metals at the SWI. As the urgent need for research focused on under-ice ecosystem dynamics, this study addressed the previously unknown behavior of the labile metals at the SWI and provided a unique perspective for the lake management during the ice-cover periods when external nutrient input was cut off.
More than half of the world’s lakes (>50 million) are periodically frozen, and partially or fully covered with ice (Hampton et al., 2017). During the ice period, the ice cover significantly impacts the concentrations, migration, and transformation of metals due to the transportation differences between ice and water, and the elimination of wave action, which mixes the oxygen and contaminant (Ozersky et al., 2021). Besides, when the aqueous solutions are frozen, hydrophobic and hydrophilic compounds are known to become spontaneously segregated at grain boundaries of ice during the phase transition, leading to relatively higher concentrations of ions and metals in the water beneath the ice (Heger et al., 2006). Due to global warming, the duration and extent of lake ice cover in winter are predicted to decrease in many temperate lakes (Kamari et al., 2018). Therefore, it is vital to understand the biogeochemical dynamics of nutrients and contaminants underneath the ice (Hampton et al., 2017; Ozersky et al., 2021).
The accumulation of heavy metals in lake sediments poses a severe risk to benthic and aquatic biota and ecosystem function, ultimately, affecting human health through the food chain (Gall et al., 2015; Green and Planchart, 2018). The sediment-water interface (SWI) is one of the most important interfaces in the critical zone, and the micro-reactions and environmental changes of physical, chemical, and biological reactions dominate with a complex impact on the state and migration-transformation behavior of metals near the interface (Harper et al., 1998; Gall et al., 2015; Fan, 2019). Almost all the pollution and ecological problems are associated with aquatic environments at the SWI (Fan, 2019). Traditional sediment chemistry studies were mainly based on ex-situ measurements, which cannot reflect the actual information on heavy metals’ physical and chemical characteristics (Ding et al., 2010; Ren et al., 2015). It has been verified that the diffusive gradients in thin films (DGT) is an ideal tool for the in situ evaluation of metal bioavailability in sediments (Menegario et al., 2017). Previous studies indicated that under anaerobic conditions, the concentrations of liable metals by DGT perform considerable apparent diffusion from sediment on the low dissolved oxygen (DO) condition (Ren et al., 2015; Yuan et al., 2020). Their surface binding characteristics with different solid phases (i.e., Fe/Mn oxides and organic matter) and metal binding regulation are influenced by the pH, redox potentials, and pore water composition.
The Chagan Lake (CL) is the representative lake in the cold regions of northeast China, which acts as an essential ecological barrier against desertification (Bu et al., 2009; Liu et al., 2021). Research suggests that aquacultural development has dynamically increased the metal concentrations in the CL (Xie, 2021). However, most studies on metals currently focus on open water season, while studies in the ice-covered period have often been neglected or assumed to be less important (Hampton et al., 2017; Kamari et al., 2018). There is still a significant research gap in the understanding and estimation of stocks and fluxes of metals under ice cover. In this study, the ZrO-Chelex DGT technique was employed for the first time to obtain accurate information regarding the spatial distributions of labile Cu, Zn, Pd, Mn, Cd, Cr, and As at the SWI of CL during the ice-covered period. Due to redox conditions under ice cover, we hypothesized that considerable concentrations and release of labile metal would be found at the SWI due to redox conditions under ice cover, even though the cold temperature would significantly affect water quality in the following spring. This study would provide a brand-new insight into the control of internal metal loading during ice-covered periods when external input was cut off.
As the largest inland lake in Jilin Province, the CL is located west of Songnen Plain, Northeast China. It covers an area of 372 km2, holding 5.98 × 108 m3 of water. The average and maximum depths of the CL are 1.52 and 6 m. The CL is located in a semi-arid and sub-humid region with a long-term annual average temperature of 5.5°C. The duration of the ice cover is approximately 4 months, from late November to next March. The CL plays a vital role in the regional economic and cultural heritage and is well-known for winter fishing. The CL receives the discharge from Daan and Qianguo irrigation districts. Therefore, a substantial amount of N, P, and heavy metals flow into the lake with irrigation discharge.
An ice drill was used to prepare holes with a diameter of 50 cm on the surface of the CL on December 20th, 2020, when the winds over the lake were light. Six cores (Table 1) with 20 cm overlying water were collected using a gravity corer (φ90 mm × 500 mm) and then covered with a rubber plug on both ends. Water temperature, DO, pH, conductivity, and chlorophyll a were measured by a portable multi-probe (YSI 650 MDS, United States). The concentrations of DOC were determined by Xpert-TOC/TN. Surface sediment samples were collected using a grab sampler and then placed in acid-washed plastic bags. Then the samples were transported carefully to the laboratory and stored at −18°C for further analysis.
The sediment cores were stabilized for 12 h to ensure the equilibrium at the interface between the sediment and the water. The DGT device involves a device consisting of four components: a ZrO-Chelex binding gel, a diffusive gel, a membrane filter, and a fixed shell (2.5 cm × 18 cm). The ZrO-Chelex binding gel was prepared using the method described by Xu D. et al. (2012). The diffusive gel was prepared from polyacrylamide using the method published by Zhang and Davison (2002). Specifically, the ZrO-Chelex binding gel is on the top, and the diffusive gel is sandwiched between a Whatman membrane filter with a pore size of 0.45 μm, and the three layers were stacked on the base of the fixed shell. The assembled device was immersed in a 0.01 mol L–1 NaCl solution, to which N2 was added continuously for 16 h before use.
The ZrO-Chelex DGT devices would simultaneously measure multiple labile cations and oxyanions, and were provided by EasySensor Ltd (Nanjing, China)1. During the application, the ZrO-Chelex DGT device was slowly inserted into the sediment cores for 72 h. Water temperature was simultaneously measured three times at each site during the deployment. The SWI was marked after being withdrawn and then washed with deionized water to remove the sediment particles. A ceramic cutter was then used to cut the binding gel at 2 mm intervals. The gel samples were transferred to a 1.5 mL PE bottle with 1.8 mL 1 mol L–1 HNO3 for 24 h. Quality control was ensured by analyzing duplicate samples with a precision of three replicates of 15% (relative standard deviation). All the concentrations of metals and solutions were analyzed using an Agilent 7700 inductively coupled plasma mass spectrometer.
The concentrations of (CDGT) of each metal (μg/L) are calculated using:
where M is the amount of the target analyte accumulated in the DGT device (μg); Δg is the total thickness of the diffusive gel and the membrane filter (cm); D is the diffusion coefficient of the target metal in the diffusive gel (cm2 s–1); A is the area of the window of the DGT device (cm2); T is the time that the system was allowed to stand still (s).
The net exchange flux F of each metal at the SWI can be estimated based on Fick’s first law:
where F is the diffusive flux at the SWI (mg m–2 day–1); Fs is the diffusive flux from the sediment; Fw is the diffusive flux from the overlying water; ∂cs/∂xs is the concentration gradient in the sediment; ∂cw/∂xw is the concentration gradient in the overlying water; x is the depth of the sediment (cm); c is the ion concentration (mg L–1); Ds is the diffusion coefficient of each metal in the sediment, which can be calculated based on the molecular diffusion coefficient in water Dw and the surface sediment porosity φ.
In this study, the diffusive flux patterns were produced using Microsoft Excel. SPSS Statistics 23.0 was used to analyze variance (ANOVA) and independent-samples t-tests. RStudio 6.5 was used to analyze correlation analysis. In addition, the RStudio 6.5 and Canoco 5 were used to perform redundancy analysis (RDA) to determine the factors influencing the metal profiles during the ice-covered period.
The DOC of the CL under ice in winter ranged from 10.29 to 27.42 mg/L (mean: 13.23 ± 7.06 mg/L); DO from 10.15 to 11.96 mg/L (mean: 11.41 ± 0.67 mg/L); ORP from 8.4 to 35.5 mV (mean: 27.55 ± 10.82 mV); Sal from 0.61 to 0.92 psu (mean: 0.70 ± 0.11 psu); TDS from 797 to 1,178 mg/L (mean: 910.17 ± 138.76 mg/L); Turbidity from 853.54 to 1,307.04 FNU (mean: 1,198.75 ± 177 FNU); and pH from 6.75 to 7.42 (mean: 6.96 ± 0.25). The mean values of DO and pH of CG2 were slightly lower than other sites (Table 2).
Concentrations of liable Cu ranged from 0.0005 to 1.99 μg/L (mean: 0.18 μg/L); Zn from 4.43 to 18.81 μg/L (mean: 8.06 μg/L); Pb from 0.28 to 23.61 μg/L (mean: 0.55 μg/L); Cd from 0.06 to 0.29 μg/L (mean: 0.09 μg/L); Cr from 0.11 to 16.18 μg/L (mean: 0.58 μg/L); and As from 0.14 to 7.13 μg/L (mean: 2.4 μg/L). The Mn concentration ranged from 0.46 to 72.51 μg/L, the highest compared to the other metals (Figure 1). In the profile, the concentrations of all metals initially increased and then decreased with increasing depth peaking exactly below the water-sediment interface. The concentrations of Mn and Pb were considerably higher at site CG2 than at any other sampling site.
There were significant differences between different sampling sites in the concentrations of Mn (P < 0.001), Cu (P < 0.001), Zn (P < 0.001), and Pb (P < 0.001) by ANONA analysis, as well as insignificant differences between the concentrations of Cd, Cr, and As at the SWI (from −20 mm to 20 mm) of CL during the ice-covered period.
Correlation analysis revealed that the CDGT value for labile Cu was extremely significantly positively correlated with that of labile Zn (R = 0.78, P < 0.001) and Pb (R = 0.75, P < 0.001; Figure 2). The CDGT value for Zn was significantly positively correlated with Pb (R = 0.77, P < 0.001). The CDGT for Mn was significantly negatively correlated with Cu, Zn, and Pb values. There were no significant correlations between the CDGT values for Cr and As and that of any other metals (Figure 2).
The apparent diffusive fluxes of liable Cu ranged from −0.12 to 0.26 mg m–2 day–1 (mean: 0.12 mg m–2 day–1); Zn from −4.53 to 6.76 mg m–2 day–1 (mean: 0.51 mg m–2 day–1); Pb from −0.11 to 0.11 mg m–2 day–1 (mean: −0.01 mg m–2 day–1); Cd from −0.34 to 0.02 mg m–2 day–1 (mean: −0.10 mg m–2 day–1); Cr from −18.51 to 3.85 mg m–2 day–1 (mean: −2.37 mg m–2 day–1) and As from −3.47 to 5.14 mg m–2 day–1 (mean: 2.13 mg m–2 day–1; Figure 3). Concentrations of liable Mn ranged from 0.32 to 50.1 mg m–2 day–1, the highest among the other metals. Positive apparent diffusive fluxes were observed at most sampling sites for Cu, Mn, and As, indicating the upward movement from the sediment to the overlying water. Negative apparent diffusive fluxes were observed at most sampling sites for Zn, Pb, Cr, and Cd, with sediment as a sink.
Figure 3. The vertical distributions of labile Cu, Zn, Pb, Mn, Cd, Cr, and As at the sediment-water interface during the ice-covered period of Chagan Lake.
The RDA analysis indicates that the DO explains 79.5% (P = 0.032) of the variation in the whole profile, and the RDA1 axis explains 91.05% of the variation in the SWI (Figure 4).
Figure 4. Correlation analysis between metals at the sediment-water interface of Chagan Lake during the ice-covered period. **Represents P < 0.01, ***represents P < 0.001.
The SWI is the boundary between the overlying water column and sediments, and this zone is easily affected by physical disturbance (e.g., temperature, currents caused by resuspension), biological processes (e.g., bioturbation generating mounds and trenches), and chemical process (Wu et al., 2014, 2016; Fan, 2019). These processes affect the migration and transformation of metals around the SWI in complex ways (e.g., oxidation, reduction, adsorption, desorption, and degradation; Voermans et al., 2017; Fan, 2019). In this study, peak concentrations of labile metals also appeared just below the SWI during the ice-covered period (Figure 5). The vertical variation of labile metals on the sediment profiles generally shows a decreasing trend with depth. Most metals in the surface sediment were reported in an active or incompletely degraded state, therefore they have a high tendency to participate in the biogeochemical process at the SWI (Ren et al., 2015; Wu et al., 2015; Liu et al., 2016). The behavior of labile metals near the SWI is significant because the dynamic chemical and biochemical processes occurring in surface sediment cause major transformations in the speciation/mobility of metals due to remobilization or sequestration from minerals/organisms (Wu et al., 2014). These processes can result in an increase in the CDGT values of the metals at the SWI. Moreover, the biological and physical disturbances increase the sediment’s porosity and resuspended sediment particles, consequently inducing the release of contaminations from the sediment to the pore water. This results in increases in the concentrations of labile metals in the pore water at the SWI (Song et al., 2005; Fan, 2019). A substantially higher average concentration was found at sampling site CG2 than at any other site (P < 0.001), indicating notable spatial heterogeneity (Figure 5).
Few studies were reported on labile metals by DGT technique during the ice-covered period in lakes in China, and it is only possible to compare our findings with the former research in Lake Xingkai (In press). Compared with the Lake Xingkai Basin, the CL had a considerably higher concentration of labile Cr (0.58 vs 0.06 μg/L), Pb (0.55 vs 0.26 μg/L), Cd (0.09 vs 0.04 μg/L), and As (2.4 vs 1.06 μg/L; Table 3). Different from Lake Xingkai Basin, located far away in the border area, the CL was extensively affected by human activity, such as petroleum extraction, the use of pesticides and fertilizers, and famous winter fishing, contributing to the accumulation of heavy metals (Zhang et al., 2008; Xie et al., 2010). Besides, there were fewer inflow rivers to the CL, thus intensifying the accumulation of metals with low flow. A much lower concentration of labile Mn (12.5 vs 366.27 μg/L) was found compared to the Lake Xingkai basin but similar to the background value of soil in Jilin Province (452.65 ppm), which might be caused by the diagenetic process (Zhao et al., 1987). Compared with world average freshwater, concentrations of most metals in CL were a little higher even in a cold season, while much lower than in the developed warm area (Lake Xuanwu), indicating potential pollution in the CL.
Similar to previous studies (Yuan et al., 2011, 2014), strong correlations were found between Cu, Zn, and Pb concentrations, while there was a significantly negative correlation between Mn and Cu, Zn and Pb (Figure 2). This might cause by Mn oxides in sediments mainly origin from natural geochemical processes (Yuan et al., 2014; Maina et al., 2019). Besides, Mn/Fe oxides have a strong capacity to adsorb metals due to the large specific surface areas and regular porous structures (Xu L. et al., 2012; Xia et al., 2020). Therefore, the reductive dissolution of iron and manganese oxides would release labile metals (e.g., Cu, Zn, and Pb), resulting in higher concentrations (Yuan et al., 2011). Moreover, previous research has shown that Mn oxides in sediment are essential oxidants for the degradation of OM (Luo et al., 2000). The notable release of dissolved Mn at the SWI of Aha Lake was a result of the participation of MnO2 in OM degradation as an oxidant (Liu, 2019). The flux estimates obtained in this study similarly revealed a marked release of dissolved Mn at the SWI of CL. This may be an essential factor leading to the significant negative correlations between the concentration of Mn and Cu, Zn and Pb.
Lake sediment can function either as an internal metal load or sink. Therefore, studies on the source-sink characteristics at the SWI are becoming a hotspot (Templeton et al., 2000; Wang et al., 2015). It is found that the diffusive fluxes of Zn, Pb, Cr, and Cd were negative, suggesting that the sediment acted as a sink for these metals. In contrast, the diffusive fluxes of Cu, Mn, and As were positive, indicating sediments as a source. During the initial stage of the ice-covered period in winter, the DO concentration in the CL’s water was still relatively high, and the SWI might still be in a continuously oxidative environment. Under these conditions, as micro- and nanoparticles, Fe/Mn oxides known for their high activity and large surface areas were important geological adsorbents for metals such as Zn, Pb, Cr, and Cd (Banks et al., 2012; Wang, 2018; Liu, 2019). The OM-bound metals are another major form of these metals in sediment (Zoumis et al., 2001; Fan et al., 2002, 2019). The OM bound with metal degradation was to be reported released into the overlying water. In lake sediment, sulfides, after sulfate reduction, also have a strong ability to bind to metal ions. This produces insoluble metal sulfides, one of the major forms of metals in sediment (Burdige, 1993; Toro et al., 2002).
Biological disturbances resuspend sediment particles and metal sulfides were exposed to water containing active aerobic organisms (Caille et al., 2003). In the presence of sulfur-oxidizing bacteria, metal sulfides were oxidized into sulfates, releasing metals bound to the sulfides (Vera et al., 2013). The oxidation of sulfides produces H+ and therefore reduces the pH at the SWI (Miao et al., 2006). The relatively high DO content and the low water pH of 6.75 during the ice-covered period indicated a weak release of Cu and As.
DO was found as the primary factor (79.5%, P = 0.032) affecting the vertical distribution of the labile metals at the SWI. In sediments, metals combine with Fe/Mn oxides and sulfides, forming insoluble oxidizable states. As the DO concentration increased, the Fe (II) and Mn (II) released due to oxidation rapidly form colloidal Fe and Mn oxides or hydroxides, which have a strong ability to adsorb metal ions and are highly susceptible to co-precipitating with metals. During the freezing period, ice cover and continuous mineralization of organic aggregates would lead to conditions of hypoxia and anoxia at the SWI. This created favorable conditions for endogenous metal release from bounded to Fe/Mn/S oxides into the pore water (Toro et al., 2002; Shao et al., 2012; Xia et al., 2020). Therefore, changes in DO concentration control the release and precipitation of metals. Moreover, metals bound to OM were a major form in sediments (Zoumis et al., 2001; Fan et al., 2002). When OM bound to metals was degraded by microorganisms, these metals were released into the water. As a principal factor affecting microorganism-induced degradation (Canfield et al., 1993), DO also substantially impacts the concentrations of metals in the water. DO dynamics with a high spatiotemporal resolution by planar optode should be obtained in further study.
Diffusive gradients in thin films technique was firstly employed to acquire high-resolution millimeter-scale in situ information regarding the labile Cu, Zn, Pb, Mn, Cd, Cr, and As at the SWI in the CL during the ice-covered period. The labile metals at the SWI of CL displayed markedly different spatial and vertical distribution patterns. Specifically, the concentration of each metal peaked exactly below the interface. Based on Fick’s first law, the diffusive fluxes revealed that the sediment acted as a sink for Zn, Pb, Cr, and Cd, while a source for Cu, Mn, and As in winter. Compared with other studies, Pb, Cr, and As concentrations under-ice were even slightly higher, indicating potentially ecological risk. In situ passive sampling techniques could help inform potential ecological or human health risks associated with metal contamination without disturbing aquatic biota. DO was identified as the primary factor affecting the vertical distribution of the labile metals at the SWI. It may be worth considering actions to take into account redox processes and control the O2 dynamics when management decision-making during remediation planning. Our results quantified the endogenous metal concentrations and diffusion fluxes across the SWI during the ice-cover period, giving insight into lake water quality management in temperate seasonal frozen lakes.
The original contributions presented in this study are included in the article/supplementary material, further inquiries can be directed to the corresponding author.
XZ and YY proposed and structured the study. QW, ZW, YzZ, QY, and SL were involved in the experiments. XZ, QW, XD, and ZW wrote the manuscript. QW, XD, and YjZ made the figure. XZ, QW, and YY reviewed and improved the English of the manuscript and made a funding acquisition. All authors participated in discussion of the research.
This research was financially supported by the Jilin Province Education Department Science and Technology Research Project (No. JJKH20210289KJ); National Natural Science Foundation of China (No. 42101071); Natural Science Foundation of Jilin Province (YDZJ202201ZYTS480); and Scientific and Technological Innovation and Entrepreneurship Project for Overseas Researchers in Jilin Province in 2021.
We would like to thank MY Sang for his help in the field work; Y. R. Wang, Z. X. Liu, Q. Y. Zhu, and D. Zhang for their preparation for the data.
The authors declare that the research was conducted in the absence of any commercial or financial relationships that could be construed as a potential conflict of interest.
All claims expressed in this article are solely those of the authors and do not necessarily represent those of their affiliated organizations, or those of the publisher, the editors and the reviewers. Any product that may be evaluated in this article, or claim that may be made by its manufacturer, is not guaranteed or endorsed by the publisher.
Banks, J., Ross, D. J., and Keough, M. J. (2012). Short-term (24 h) effects of mild and severe hypoxia (20% and 5% dissolved oxygen) on metal partitioning in highly contaminated estuarine sediments. Estuarine Coastal Shelf Sci. 99, 121–131. doi: 10.1016/j.ecss.2011.12.025
Bu, X. J., Chai, S. L., Zhang, Q. W., and Xu, X. C. (2009). The spatial distributions of elements in sediments of lake Chagan in west Jilin Province. J. Arid Land Resour. Environ. 23, 179–184.
Burdige, D. J. (1993). The biogeochemistry of manganese and iron reduction in marine sediments. Earth-Science Rev. 35, 249–284. doi: 10.1016/0012-8252(93)90040-E
Caille, N., Tiffreau, C., Leyval, C., and Morel, J. L. (2003). Solubility of metals in an anoxic sediment during prolonged aeration. Sci. Total Environ. 301, 239–250. doi: 10.1016/s0048-9697(02)00289-9
Canfield, D. E., Jørgensen, B. B., Fossing, H., Glud, R., Gundersen, J., Ramsing, N. B., et al. (1993). Pathways of organic carbon oxidation in three continental margin sediments. Mar. Geol. 113, 27–40. doi: 10.1016/0025-3227(93)90147-N
Ding, S., Xu, D., Sun, Q., Yin, H., and Zhang, C. (2010). Measurement of dissolved reactive phosphorus using the diffusive gradients in thin films technique with a high-capacity binding phase. Environ. Sci. Technol. 44, 8169–8174. doi: 10.1021/es1020873
Dragun, Z., Raspor, B., and Roje, V. (2008). The labile metal concentrations in Sava River water assessed by diffusive gradients in thin films. Chem. Speciation Bioavail. 20, 33–46. doi: 10.3184/095422908X299164
Fan, C. X. (2019). Research progress and Prospect of lake sediment water interface. Lake Sci. 31, 1191–1218.
Fan, W., Wang, W.-X., Chen, J., Li, X., and Yen, Y.-F. (2002). Cu, Ni, and Pb speciation in surface sediments from a contaminated bay of northern China. Mar. Pollut. Bull. 44, 820–826. doi: 10.1016/s0025-326x(02)00069-3
Fan, X., Ding, S., Chen, M., Gao, S., Fu, Z., Gong, M., et al. (2019). Peak chromium pollution in summer and winter caused by high mobility of chromium in sediment of a eutrophic lake: in situ evidence from high spatiotemporal sampling. Environ. Sci. Technol. 53, 4755–4764. doi: 10.1021/acs.est.8b07060
Fei, Z., Wang, Z., and Tang, Y. (2021). Characteristics and source analysis of heavy metals in Aha Lake during wet and dry periods. Earth Environ. 49, 42–50.
Foerstner, U., and Wittmann, G. T. W. (1980). Metal Pollution in the Aquatic Environment. Berlin: Springer.
Gall, J. E., Boyd, R. S., and Rajakaruna, N. (2015). Transfer of heavy metals through terrestrial food webs: a review. Environ. Monit. Assess 187:201. doi: 10.1007/s10661-015-4436-4433
Gao, L., Li, R., Liang, Z., Wu, Q., Yang, Z., Li, M., et al. (2021). Mobilization mechanisms and toxicity risk of sediment trace metals (Cu, Zn, Ni, and Pb) based on diffusive gradients in thin films: a case study in the Xizhi River basin, South China. J. Hazard. Mater. 410:124590. doi: 10.1016/j.jhazmat.2020.124590
Green, A. J., and Planchart, A. (2018). The neurological toxicity of heavy metals: a fish perspective. Comp. Biochem. Physiol. Part C Toxicol. Pharmacol. 208, 12–19. doi: 10.1016/j.cbpc.2017.11.008
Hampton, S. E., Galloway, A. W. E., Powers, S. M., Ozersky, T., Whiteford, E. J., and Xenopoulos, M. A. (2017). Ecology under lake ice. Ecol. Lett. 20, 98–111.
Harper, M. P., Davison, W., Zhang, H., and Tych, W. (1998). Kinetics of metal exchange between solids and solutions in sediments and soils interpreted from DGT measured fluxes. Geochim. Cosmochim. Acta 62, 2757–2770. doi: 10.1016/S0016-7037(98)00186-180
Heger, D., Klánová, J., and Klán, P. (2006). Enhanced protonation of cresol red in acidic aqueous solutions caused by freezing. J. Phys. Chem. B 110, 1277–1287. doi: 10.1021/jp0553683
Kamari, M., Tattari, S., Lotsari, E., Koskiaho, J., and Lloyd, C. E. M. (2018). High-frequency monitoring reveals seasonal and event-scale water quality variation in a temporally frozen river. J. Hydrol. 564, 619–639. doi: 10.1016/j.jhydrol.2018.07.037
Liu, C., Fan, C., Shen, Q., Shao, S., Zhang, L., and Zhou, Q. (2016). Effects of riverine suspended particulate matter on post-dredging metal re-contamination across the sediment-water interface. Chemosphere 144, 2329–2335. doi: 10.1016/j.chemosphere.2015.11.010
Liu, J. (2019). Adsorption, Oxidation/Reduction and Crystal Growth of Metal Ions on Typical Iron Oxide Surfaces. Doctor thesis, China: University of Chinese Academy of Sciences.
Liu, P., Zheng, C., Wen, M., Luo, X., Wu, Z., Liu, Y., et al. (2021). Ecological risk assessment and contamination history of heavy metals in the sediments of Chagan lake. Northeast China. Water 13:894. doi: 10.3390/w13070894
Luo, S. S., Wan, G. J., and Huang, R. G. (2000). Distribution and migration characteristics of iron and manganese at the sediment water interface of Erhai Lake, Yunnan. Chongqing Environ. Sci. 6, 19–21.
Maina, C. W., Sang, J. K., Raude, J. M., and Mutua, B. M. (2019). Geochronological and spatial distribution of heavy metal contamination in sediment from Lake Naivasha. Kenya. J. Radiation Res. Appl. Sci. 12, 37–54. doi: 10.1080/16878507.2019.1593718
Menegario, A. A., Yabuki, L. N. M., Luko, K. S., Williams, P. N., and Blackburn, D. M. (2017). Use of diffusive gradient in thin films for in situ measurements: a review on the progress in chemical fractionation, speciation and bioavailability of metals in waters. Anal. Chim. Acta 983, 54–66. doi: 10.1016/j.aca.2017.06.041
Miao, S., DeLaune, R. D., and Jugsujinda, A. (2006). Influence of sediment redox conditions on release/solubility of metals and nutrients in a Louisiana Mississippi River deltaic plain freshwater lake. Sci. Total Environ. 371, 334–343. doi: 10.1016/j.scitotenv.2006.07.027
Ozersky, T., Bramburger, A. J., Elgin, A. K., Vanderploeg, H. A., Wang, J., Austin, J. A., et al. (2021). The changing face of winter: lessons and questions from the laurentian Great Lakes. J. Geophys. Res. Biogeosci. 126:e2021JG006247. doi: 10.1029/2021JG006247
Ren, J., Williams, P. N., Luo, J., Ma, H., and Wang, X. (2015). Sediment metal bioavailability in Lake Taihu, China: evaluation of sequential extraction, DGT, and PBET techniques. Environ. Sci. Pollution Res. 22, 12919–12928. doi: 10.1007/s11356-015-4565-4569
Shao, L., Xiao, H. Y., Wu, D. S., and Tang, C. G. (2012). Research progress of metals pollution from traffic sources. Earth Environ. 40, 445–459. doi: 10.14050/j.cnki.1672-9250.2012.03.016
Song, C. L., Cao, X. Y., Li, J. Q., Li, Q. M., Chen, G. Y., and Zhou, Y. Y. (2005). Contribution of phosphatase and microbial activity to endogenous load of phosphorus in lakes and its relation to eutrophication. Sci. China Series S2, 90–100.
Templeton, D. M., Ariese, F., Cornelis, R., Danielsson, L.-G., Muntau, H., van Leeuwen, H. P., et al. (2000). Guidelines for terms related to chemical speciation and fractionation of elements. definitions, structural aspects, and methodological approaches (IUPAC Recommendations 2000). Pure Appl. Chem. 72, 1453–1470. doi: 10.1351/pac200072081453
Toro, D. M. D., Mahony, J. D., Hansen, D. J., Scott, K. J., Carlson, A. R., and Ankley, G. T. (2002). Acid Volatile Sulfide Predicts the Acute Toxicity of Cadmium and Nickel in Sediments. Washington, DC: ACS Publications.
Vera, M., Schippers, A., and Sand, W. (2013). Progress in bioleaching: fundamentals and mechanisms of bacterial metal sulfide oxidation–part A. Appl. Microbiol. Biotechnol. 97, 7529–7541. doi: 10.1007/s00253-013-4954-2
Voermans, J. J., Ghisalberti, M., and Ivey, G. N. (2017). The variation of flow and turbulence across the sediment-water interface. J. Fluid Mechan. 824, 413–437. doi: 10.1017/jfm.2017.345
Wang, Y. (2018). High Resolution Simultaneous Acquisition and Microscale Migration Mechanism of Metalss at Sediment Interface. Doctor thesis, China: University of Chinese Academy of Sciences.
Wang, Y. P., Guan, Q. W., Li, C., Xu, D., and Ding, S. M. (2015). DGT in situ simultaneous analysis of available phosphorus and sulfur in sediments of Chaohu Lake. J. Environ. Sci. 35, 2512–2518. doi: 10.13671/j.hjkxxb.2014.0996
Wu, Z., Jiao, L., and Wang, S. (2016). The measurement of phosphorus, sulfide and metals in sediment of Dianchi Lake by DGT (diffusive gradients in thin films) probes. Environ. Earth Sci. 75:193. doi: 10.1007/s12665-015-4978-4972
Wu, Z., Wang, S., He, M., and Wu, F. (2015). The measurement of metals by diffusive gradients in thin films (DGT) at sediment/water interface (SWI) of bay and remobilization assessment. Environ. Earth Sci. 73, 6283–6295. doi: 10.1007/s12665-014-3851-z
Wu, Z., Wang, S., Jiao, L., and Wu, F. (2014). The simultaneous measurement of phosphorus, sulfide, and trace metals by Ferrihydrite/AgI/Chelex-100 DGT (Diffusive Gradients in Thin Films) probe at sediment/water interface (SWI) and remobilization assessment. Water Air Soil Pollution 225:2188. doi: 10.1007/s11270-014-2188-2182
Xia, J. D., Long, J. Y., Gao, Y. P., Chen, Y., Meng, J., Zhou, Y. Q., et al. (2020). Ecological risk assessment and source analysis of metals pollution in sediments of Chaohu Lake. Earth Environ. 48, 220–227. doi: 10.14050/j.cnki.1672-9250.2020.01.020
Xie, R. F. (2021). Metals Deposition Characteristics, Pollution Sources and Trend Prediction of Typical Lakes in Northern China. Doctor thesis, China: Harbin Normal University.
Xie, X. J., Kang, J. C., Li, W. L., Wang, G. D., Yan, G. D., and Zhang, J. P. (2010). Distribution and source analysis of metalss in agricultural soil in Baoshan District of Shanghai. Environ. Sci. 31, 768–774. doi: 10.13227/j.hjkx.2010.03.010
Xu, D., Ding, S., Sun, Q., Zhong, J., Wu, W., and Jia, F. (2012). Evaluation of in situ capping with clean soils to control phosphate release from sediments. Sci. Total Environ. 438, 334–341. doi: 10.1016/j.scitotenv.2012.08.053
Xu, L., Zhang, L., Hou, X., and Xu, K. (2012). Adsorption of metals ions on two types of manganese oxides analyzed by AAS and AFS. Spectroscopy Spectral Anal. 32, 2842–2846. doi: 10.3964/j.issn.1000-0593201210-2842-2845
Yuan, H., Shen, J., Liu, E., Wang, J., and Meng, X. (2011). Assessment of nutrients and heavy metals enrichment in surface sediments from Taihu Lake, a eutrophic shallow lake in China. Environ. Geochem. Health 33, 67–81. doi: 10.1007/s10653-010-9323-9329
Yuan, H., Yin, H., Yang, Z., Yu, J., Liu, E., Li, Q., et al. (2020). Diffusion kinetic process of heavy metals in lacustrine sediment assessed under different redox conditions by DGT and DIFS model. Sci. Total Environ. 741:140418. doi: 10.1016/j.scitotenv.2020.140418
Yuan, Z., Taoran, S., Yan, Z., and Tao, Y. (2014). Spatial distribution and risk assessment of heavy metals in sediments from a hypertrophic plateau lake Dianchi. China. Environ. Monit. Assess 186, 1219–1234. doi: 10.1007/s10661-013-3451-3455
Zhang, H., and Davison, W. (2002). Performance Characteristics of Diffusion Gradients in Thin Films for the in Situ Measurement of Trace Metals in Aqueous Solution. Washington, DC: ACS Publications.
Zhang, M. K., Wang, H., and Zhang, H. M. (2008). Source identification of metalss in farmland soil of marine plain in eastern Zhejiang. J. Environ. Sci. 10, 1946–1954. doi: 10.13671/j.hjkxxb.2008.10.003
Zhao, Z. L., Dong, G. F., Li, Z. X., Zhang, H. T., Yu, S. Q., and Yu, B. (1987). Study on background values of nine heavy metals in natural soils in Jilin Province. Soil 6, 315–317.
Keywords: Chagan Lake, in-situ high resolution, diffusive fluxes, winter, sediment-water interface
Citation: Yuan Y, Wang Q, Dong X, Zhu Y, Wu Z, Yang Q, Zuo Y, Liang S, Wang C and Zhu X (2022) In situ, high-resolution evidence of metals at the sediment-water interface under ice cover in a seasonal freezing lake. Front. Ecol. Evol. 10:956903. doi: 10.3389/fevo.2022.956903
Received: 30 May 2022; Accepted: 26 July 2022;
Published: 12 August 2022.
Edited by:
He Yixin, Key Laboratory of Mountain Ecological Rehabilitation and Biological Resource Utilization, Chengdu Institute of Biology (CAS), ChinaReviewed by:
Feng Li, Institute of Subtropical Agriculture (CAS), ChinaCopyright © 2022 Yuan, Wang, Dong, Zhu, Wu, Yang, Zuo, Liang, Wang and Zhu. This is an open-access article distributed under the terms of the Creative Commons Attribution License (CC BY). The use, distribution or reproduction in other forums is permitted, provided the original author(s) and the copyright owner(s) are credited and that the original publication in this journal is cited, in accordance with accepted academic practice. No use, distribution or reproduction is permitted which does not comply with these terms.
*Correspondence: Xiaoyan Zhu, emh1eHkwNjEzQDEyNi5jb20=
Disclaimer: All claims expressed in this article are solely those of the authors and do not necessarily represent those of their affiliated organizations, or those of the publisher, the editors and the reviewers. Any product that may be evaluated in this article or claim that may be made by its manufacturer is not guaranteed or endorsed by the publisher.
Research integrity at Frontiers
Learn more about the work of our research integrity team to safeguard the quality of each article we publish.