- 1Key Laboratory of Bio-Resources and Eco-Environment of Ministry of Education, College of Life Sciences, Sichuan University, Chengdu, China
- 2Observation and Research Station of Sichuan Province of Fish Resources and Environment in Upper Reaches of the Yangtze River, College of Life Sciences, Sichuan University, Chengdu, China
- 3Chengdu Jena Environmental Protection Technology Company, Chengdu, China
The Datong and Xiaotong rivers are karst tributaries in the upper Yangtze River drainage and represent considerable habitat diversity that contributes to high fish diversity and rich fishery resources in the two rivers. During the few decades, fish resources have abruptly declined due to overfishing, water pollution and habitat degradation. In order to further strengthen conservation and restoration of fish resources and provide scientific support for fishery management in these rivers, the temporal and spatial dynamics of fish assemblage in the Datong and Xiaotong rivers were investigated in the present study. A total of 45 species were captured at 30 sample sites during four surveys conducted from March 2016 to January 2017. Species diversity and richness both increased from upstream to downstream in the two rivers. The fish assemblages significantly differed between river reaches, while did not differ in significantly between 4 months. The spatial variations in the abundance of Rhynchocypris oxycephalus, Zacco platypus, Triplophysa bleekeri, Sinogastromyzon szechuanensis, Platysmacheilus nudiventris, Sinibrama taeniatus, Saurogobio gymnocheilus, Pseudorasbora parva, Pseudobagrus truncates and Opsariichthys bidens were considered to contribute most to the spatial pattern of fish assemblages. The lack of a temporal pattern of the fish assemblage in the rivers might be attributed to ecological habits of short-distance migration and diversified mesohabitats with riffles and pools for fish spawning, feeding or overwintering within a very short river range. Our results suggest the spatio-temporal dynamics of fish assemblages is highly adapted to the habitat characteristics in the two karst rivers. We recommend that fish conservation of these tributaries be focused on the mesohabitats, particularly the many pools and riffles.
Introduction
Understanding of spatial and temporal dynamics of fish assemblages is fundamental to interpreting those factors that influence the structuring of fish populations (Silvano et al., 2000). Those variations may occur in fish composition at a scale as small as a microhabitat or as large as a basin and may occur in days or as long as decades (Schlosser, 1982; Adams et al., 2004; Nazeer et al., 2016; Wang et al., 2019). Studying the patterns of such variations has been the major focus of freshwater ecology (Rahel and Hubert, 1991; Covich, 2006). As a high trophic level organism in the aquatic ecosystem, the community structure of fish not only affects ecological functions such as nutrient cycling and food web structure, but also reflects variations in the habitat environment (Kraft et al., 2007; Ives et al., 2019; Fráguas and Pompeu, 2021). Therefore, ascertaining the patterns of the fish assemblages and associated environments is of great significance to the conservation of fish diversity and effective river management (Naiman and Latterell, 2005; Rice, 2005; Erõs et al., 2010).
In terms of spatial distribution, an upstream-downstream gradient in freshwater fish assemblage structure has been extensively studied in freshwater ecosystems (Schlosser, 1982; Poff and Allan, 1995; Liu et al., 2021). Under natural conditions, species distribution within drainages can be explained by the variability in the environment along the flow regime, especially in water temperature or hydro-morphology, creating distinct fish assemblages across the upstream-downstream gradient (Rahel and Hubert, 1991; Ibarra et al., 2005). The difference in fish composition is due to fish diversity constantly changing in response to local habitat diversity. Such upstream-downstream environment-driven changes in species assemblages are consistent with several of the predictions of the River Continuum Concept (Vannote et al., 1980). For example, Wang et al. (2019) found that fish abundance, richness and Shannon-Wiener diversity all increased from upstream to downstream. Liu et al. (2021) found that the Chishui River in China showed natural and continuous changes in geomorphologic structure along its longitudinal gradient, as there are no geographic discontinuities in the mainstream.
In terms of temporal distribution, seasonal changes in fish composition often provide information on the factors regulating immigration and emigration of assemblages (Adams et al., 2004). The seasonal precipitation and runoff in a subtropical monsoon climate region promote the change of seasonal hydrochemistry and directly affect the species richness and distribution (Melcher et al., 2012; He et al., 2017). Fishes will inhabit different habitats of the ecosystem across seasons, depending on their feeding and reproductive strategies as well as on biotic (e.g., avoiding predation) and abiotic influences (suitable environment conditions) (Fernandes, 1997; Rodriguez and Lewis, 1997; Lowe-McConnell, 1999). Moreover, seasonal migrations in potamodromous fish are critical for completing life cycles, particularly in accessing spawning and nursery habitats and refugia (Fraley et al., 2016). River fishes also have evolved several life cycle adaptations to improve their resilience against stochastic disturbances, such as high fecundity, multiple spawning, batch-spawning, a protracted annual spawning season, and long life-time fecundity with multi-cyclic spawning (Wolter et al., 2015). Therefore, they are well adapted to environmental variations driven by hydro-morphological processes in seasons.
In addition to this deterministic effect of the spatial and temporal variability on assemblages, stream fish assemblages are influenced by both natural factors and anthropogenic disturbances (Vannote et al., 1980; Allan, 2004; Kennard et al., 2006). Natural factors include hydro-morphology characteristics, climate, riparian land use and water environmental conditions like salinity, turbidity and temperature that vary in time, largely due to seasonal variations in freshwater input (Cyrus and Blaber, 1987; Barletta-Bergan et al., 2002; Barletta et al., 2003, 2005). Complex factors were identified as anthropogenic disturbances for fish assemblages such as dams, pollution, overfishing and land erosion (Stanford and Ward, 2001). Such stress may cause a decline in fish stocks, habitat destruction, and biodiversity degradation. In addition, biological factors such as predation and competition also directly or indirectly affect the composition of fish (Ostrand and Wilde, 2002).
The Datong River and Xiaotong River originate in Shanxi Province, and flow through the northeast of Sichuan Province, China. The Datong and Xiaotong rivers are tributaries of the upper Qujiang River, a tributary of the Yangtze River. The two rivers are mountain rivers in a karst geological area with many rock cavities and swallets, and alternating riffles and pools (Qi, 2018) that provide very important habitats for feeding, breeding and overwintering of aquatic animals. The two rivers’ elevations range from 374 to 608 m. Wide and twisting rivers with numerous riffle and pool alternations, huge and small drop-offs, and slightly brisk flow at the riffle and rather calm flow at the pool characterize the area. Mostly sand and gravel make up the river substrate. The region receives a lot of rain each year as a result of the subtropical monsoon climate, and the riversides are covered in lush vegetation. The vegetation in the region consists of subtropical evergreen broadleaf forests, subtropical deciduous broadleaf forests, montane scrub and grasses, which contribute to the protection of soil and water. There are about 57 fish species belonging to four orders, 13 families in the two rivers, according to a recent investigation (Liang et al., 2021). In consideration of the natural aquatic ecological environment and high fish biodiversity, most areas of the Datong and Xiaotong rivers were designated as the “Sichuan Nuoshuihe Precious Aquatic Animal National Nature Reserve” in 2012 (Liang et al., 2021).
However, the ecological environment in the Datong and Xiaotong rivers has been changing due to anthropogenic activities in recent decades, which contributed to the declines of fish resources and diversities in these two tributaries (Liang et al., 2021). Overfishing is one of the major factors attributed to the decline of fish resources in the Datong and Xiaotong rivers before the national nature reserve was established. At the same time, the discharge of urban domestic sewage, coal mining in the headwater area, and the development of tourism had a great impact on water quality. The construction of two dams (Jiuyuxi dam on the lower Datong River and Shiniuzui dam on the lower Xiaotong River) were also regarded as important anthropogenic factors affecting hydrological regimes. Dams and their associated impoundment posed a significant threat to aquatic biodiversity by altering water flow regimes, fragmenting habitats, modifying channel morphology and riverbed structure (Dudgeon, 2000). In addition, sand excavation in the tributaries has destroyed several important fish habitats, such as spawning and feeding grounds (Liang et al., 2021). Nevertheless, the contemporary and long-term effects of anthropogenic activities on distribution and abundance of native fish in the Datong and Xiaotong rivers remains poorly understood.
We conducted fish resource surveys during 2016∼2017 in the Datong and Xiaotong rivers. The main objectives were: (1) to comprehensively understand the characteristics of the spatial and temporal dynamics of fish assemblage structure in the two karst streams (Xiaotong and Datong rivers), and (2) to provide basic data for conservation and management of fish resources.
Materials and methods
Field sampling
The fish resources in two tributaries, Datong River (DR) and Xiaotong River (XR) of the upper Tongjiang River, a tributary of the Qujiang River, were surveyed in March, May, September, 2016 and January, 2017. A total of 30 sampling sites were set with 15 sites on XR (S1∼S15) and 15 sites on DR (S16∼S30). The sampling sites were classified into seven reaches, which were NT (S1 to S6), QYK (S7 to S10), FY (S11 to S15) in XR and LHK (S16 to S19), TX (S20 to S23), CP (S24 to S26), WS (S27 to S30) in DR (Figure 1).
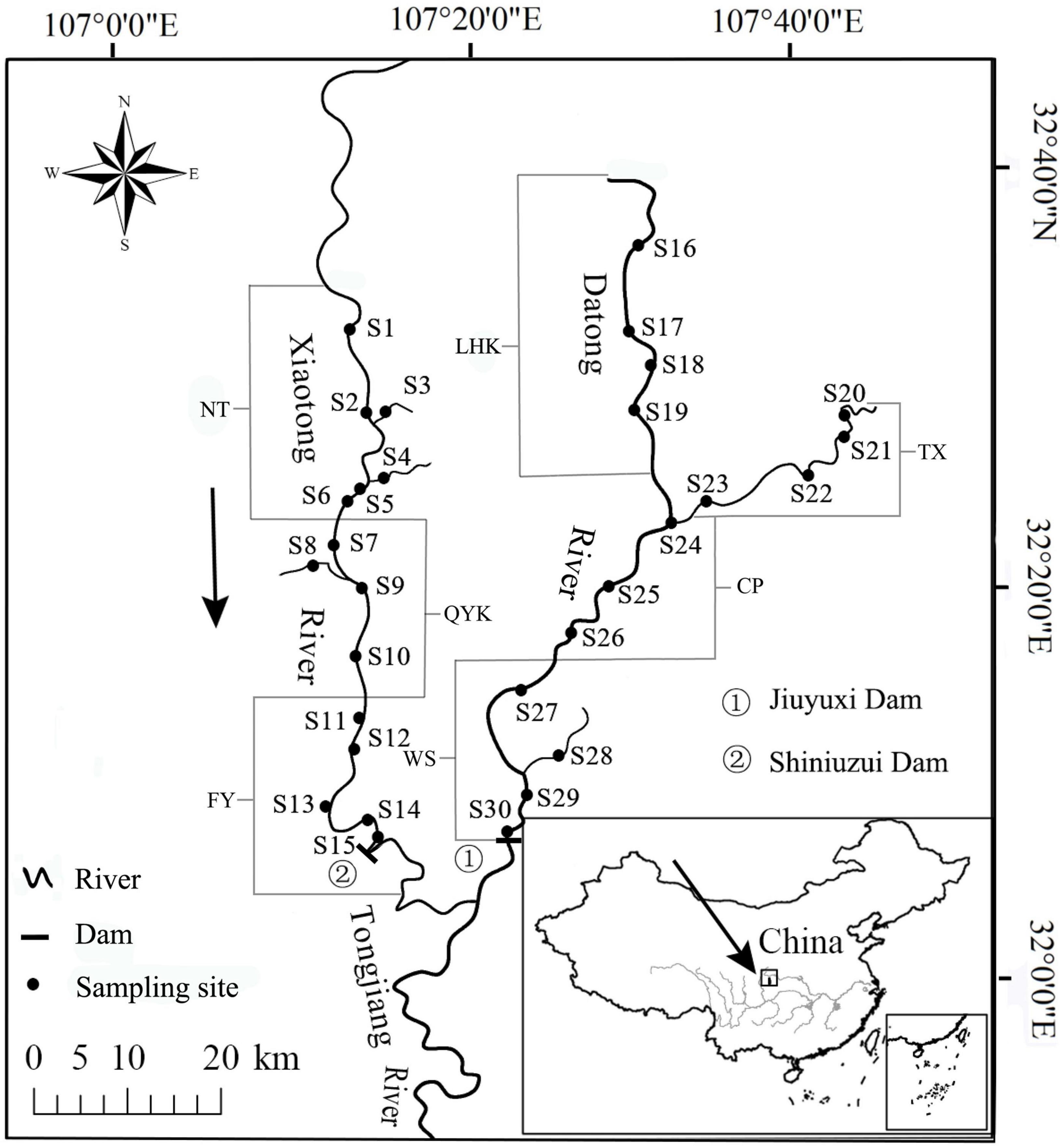
Figure 1. Water system map of the Datong and Xiaotong rivers, showing the sampling localities of fish and constructed dams on the rivers. Nuoshui Town reach is represented by NT; Qingyukou reach is represented by QYK; Fuyang reach is represented by FY; Lianghekou reach is represented by LHK; Tiexi reach is represented by TX; Changping reach is represented by CP; Washi reach is represented by WS.
Each sampling was conducted along at least a 500 m length of the river’s reach and usually contained all microhabitat types (riffles, pools, torrents and back waters). Fish specimens were caught by a combination of different sampling techniques, due to the heterogeneity of the river habitats. In reaches with shallow but torrential water, specimens were sampled via a battery-powered backpack electro-fisher (16.8 V). In backwater and deep pools, gill nets with a mesh size of 1∼6 mm (20∼50 m long and 1∼2 m high) and fish cages (0.3 m × 0.25 m × 8 m, 4 mm mesh) were used to catch fish. The duration of electrofishing on each sampling site was 40∼60 min. Three gill nets and 3∼6 cages were placed on each survey location. The gill nets and cages were placed at 18:00 in the afternoon, and withdrawn at 6:00 the next morning. The sampling was approved by the Administrative Department of Nature Reserve of Sichuan Province. The collected fish individuals were identified first, then their standard lengths (SL) were measured to the nearest 0.1 mm and body weights (BW) were measured to the nearest 0.1 g immediately after sampling, and finally individuals were released into the water where captured, except for a few specimens that were anesthetized by MS-222 solution and fixed with formaldehyde solution (5∼10%) after death.
Data analysis
We used rank-abundance and occurrence frequency diagrams to exhibit species abundance (Magurran, 2004). We then grouped all the species by their relative abundance (percentage of total fish individuals) and occurrence frequency (percentage of sampling locations in which fish species occurred), then categorized them into dominating species, common species, and rare species. We classified species as dominant, common and rare according to index of relative importance (Liang et al., 2021). Based on their maximum standard body length of less than 100, 100–200, and more than 200 mm, species are categorized as small, medium, and large-sized individuals (Wang et al., 2019). Fish species diversity for each location was calculated using Shannon-Wiener diversity: H′ = −ΣPiln(Pi), Pi = Ni/N, where Ni = the total individuals of species i collected in each location; N = the total individuals collected in each location (Magurran, 1988). We used morphological features, living habits and scientific literature to determine main dietary items and then classified the species into different feeding guilds.
One-way repeated measured analysis of variance (ANOVA) was undertaken on data (Shannon-wiener diversity, species richness, evenness, Cypriniformes diversity) to detect differences among the seven reaches (Datong River: LHK, TX, CP, WS; Xiaotong River: NT, QYK, FY) and 4 months (March, May, September, and January). Non-metric multidimensional scaling (NMDS) was performed using abundance data to assess the similarity in patterns between reaches and seasons (Hadley, 2016). Prior to NMDS analysis, the abundance data were log (x+1) transformed and categorized with the Bray-Curtis similarity matrix (Clarke and Warwick, 1994). In order to examine possible seasonal and spatial differences of fish assemblage structure, species abundance was compared between months and reaches using two-way analysis of similarities (two-way ANOSIM). Finally, the similarity percentage procedure (SIMPER) was used to identify fish species contributing most to similarities between reaches within season and between seasons with reaches of the two rivers (Clarke and Warwick, 2001).
The SPSS 23.0 statistics package (SPSS Inc., Chicago, IL, USA) was used to perform one-way repeated ANOVA, and statistical significance was accepted at P < 0.05. NMDS and two-way ANOSIM analyses were performed using R software with the package “vegan” version 2.5–6 (Oksanen et al., 2022) and “ggplot2” version 3.2.1 (Wickham et al., 2016). SIMPER analysis was conducted in PEIMER version 5 (Clarke and Warwick, 2001).
Results
Fish composition
A total of 6,160 individuals belonging to 45 fish species in 35 genera, seven families, and three orders were collected from the Datong and Xiaotong rivers. Cypriniformes and Siluriformes were the most representative orders, with 32 and 11 species, respectively, and together represented more than 95% of the species. Cyprinidae was the dominant family, comprising 22 genera and 27 species, followed by Bagridae (7 species), Cobitidae (5 species), Siluridae (2 species), Serranidae (2 species), Sisoridae (1 species), and Amblycipitidae (1 species). Altogether 32 species were captured in the Datong River, and 38 species in the Xiaotong River, respectively (Table 1).
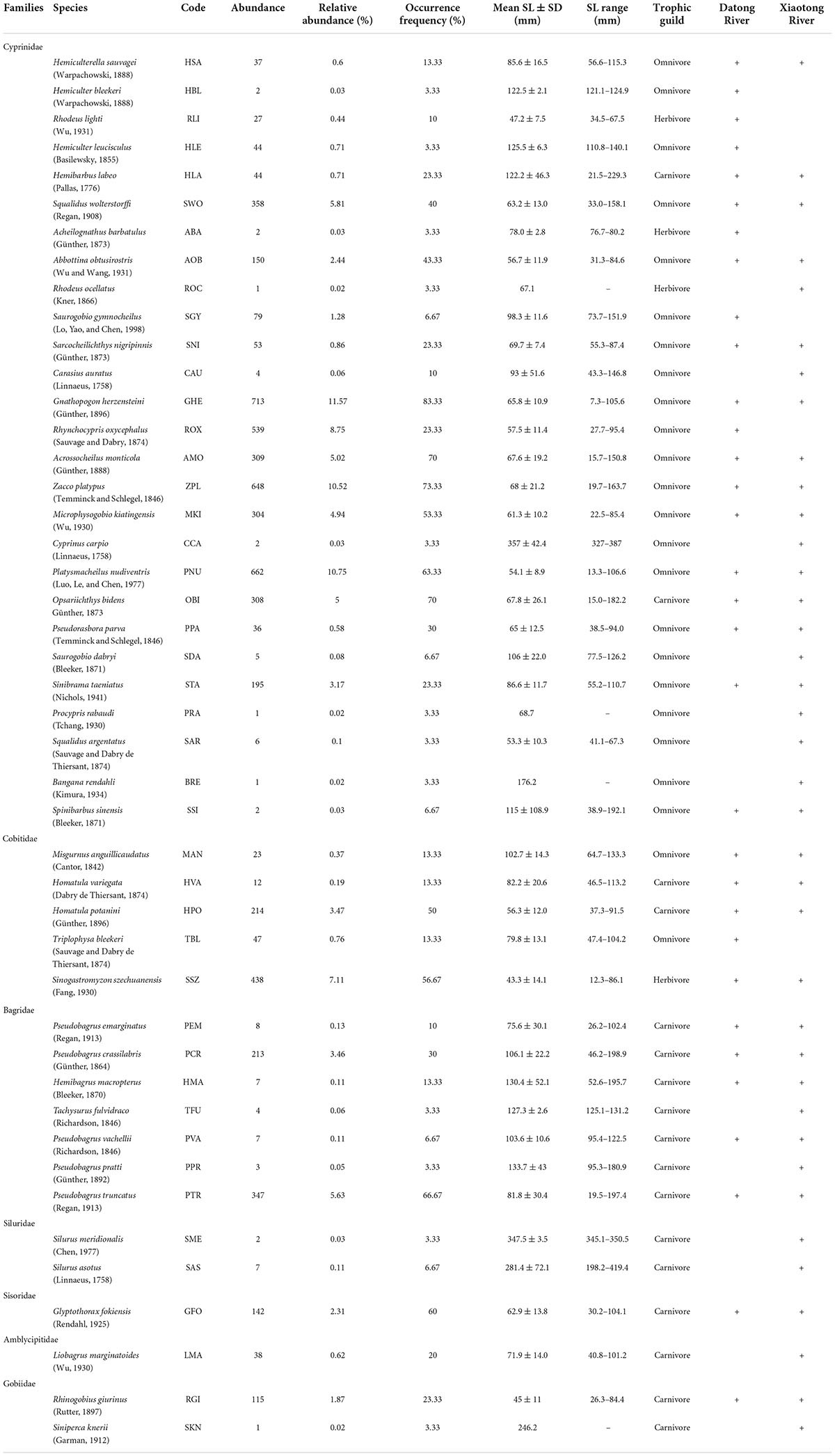
Table 1. Composition of fish species captured from Datong and Xiaotong rivers, with their species code, abundance, relative abundance, occurrence frequency, mean SL, SL range, trophic guild (TG).
A species rank-abundance and occurrence diagram indicated that the 45 species were classified into three groups, according to their relative abundance and occurrence frequency (Figure 2 and Table 1). Dominant species included G. herzensteini, P. nudiventris, Z. platypus, R. oxycephalus, S. szechuanensis, Squalidus wolterstorffi, P. truncatus, Acrossocheilus monticola, O. bidens, Microphysogobio kiatingensis and accounted for 75% of the total individuals captured (Figure 2 and Table 1). These dominant species were characterized by high relative abundance (from 4.94 to 11.57% of the total individuals captured) and high occurrence frequency (from 23.33 to 83.33% of the 30 sites). The dominant species R. oxycephalus was characterized by the highest relative abundance (8.75% of the total individuals captured) and lowest occurrence frequency (only 23.33% of the 30 sampling locations). The common species were Homatula potanini, Pseudobagrus crassilabris, S. taeniatus, Abbottina obtusirostris, Glyptothorax fokiensis and Rhinogobius giurinus, exhibiting moderate relative abundance (between 1.87 and 3.47%) and moderate occurrence frequency (from 23.33 to 60%). These common species accounted for 16.54% of the total individuals captured. The remaining 29 species were considered as rare species with a relative abundance below 1.28% and an occurrence frequency less than 23.33%, which contributed only 8.20% of the total individuals captured.
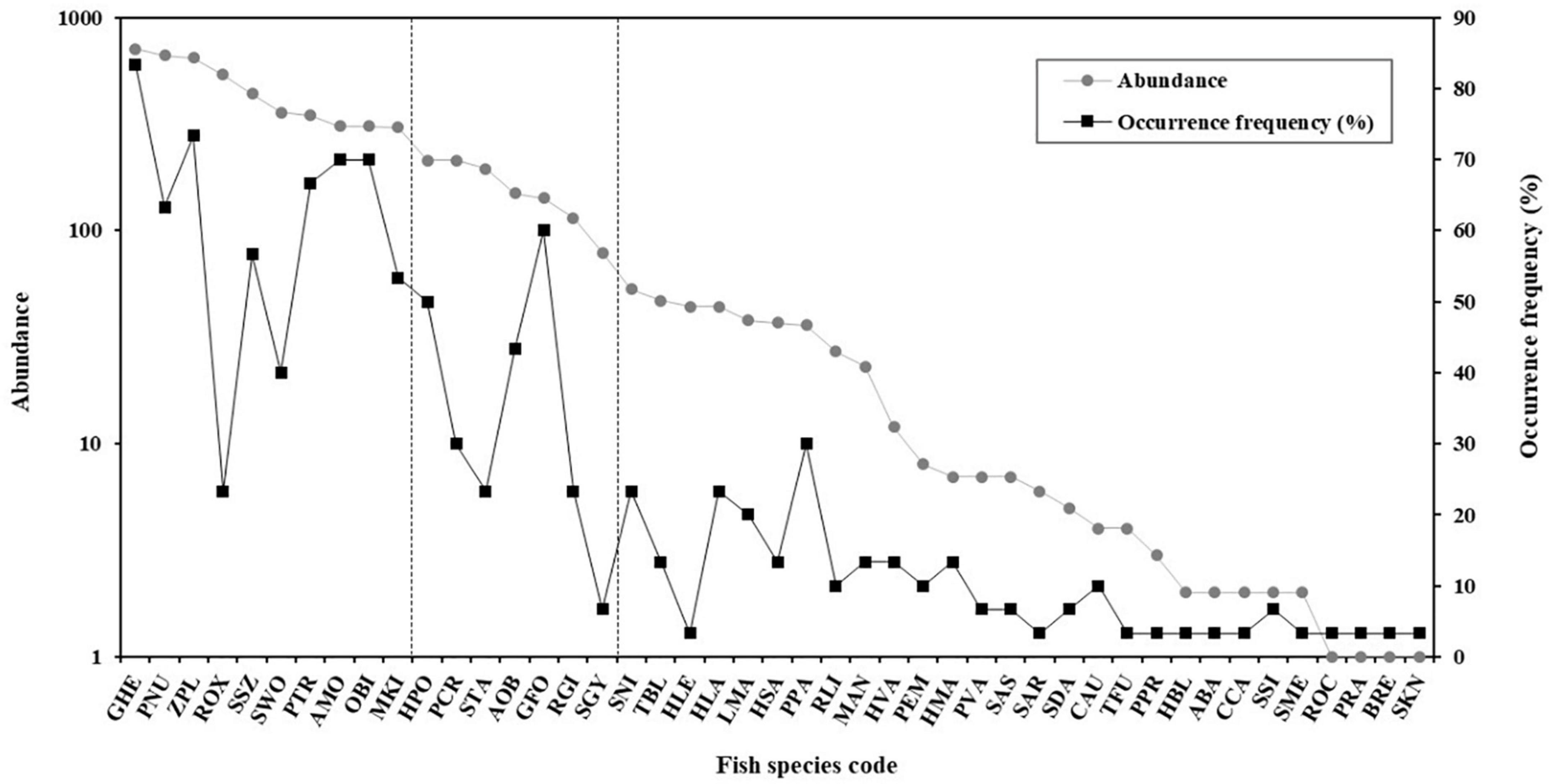
Figure 2. Rank-abundance (total number of individuals captured) and occurrence frequency (percentage of sampling locations in which the species occurred) of the 45 species captured from the Datong and Xiaotong rivers. Fish species codes are shown in Table 1.
Of the 45 species, 39 species were classified as small-sized species because their first maturity ages were less than 2 years (Ye et al., 2006) and maximum standard lengths were small (Table 1). Only six large and medium-sized species (maturity over two years) were recorded and they were Procypris rabaudi, Silurus meridionalis, Spinibarbus sinensis, Siniperca knerii, Bangana rendahli and Cyprinus carpio. The standard lengths of the 45 fish species ranged from 12 to 419 mm (Table 1). Twenty-nine species (64.44% of the total fish species) were below 100 mm (mean SL), 12 species (26.67%) between 100 and 200 mm, and the other four species (8.89%) had mean SL above 200 mm (Table 1 and Figure 3).
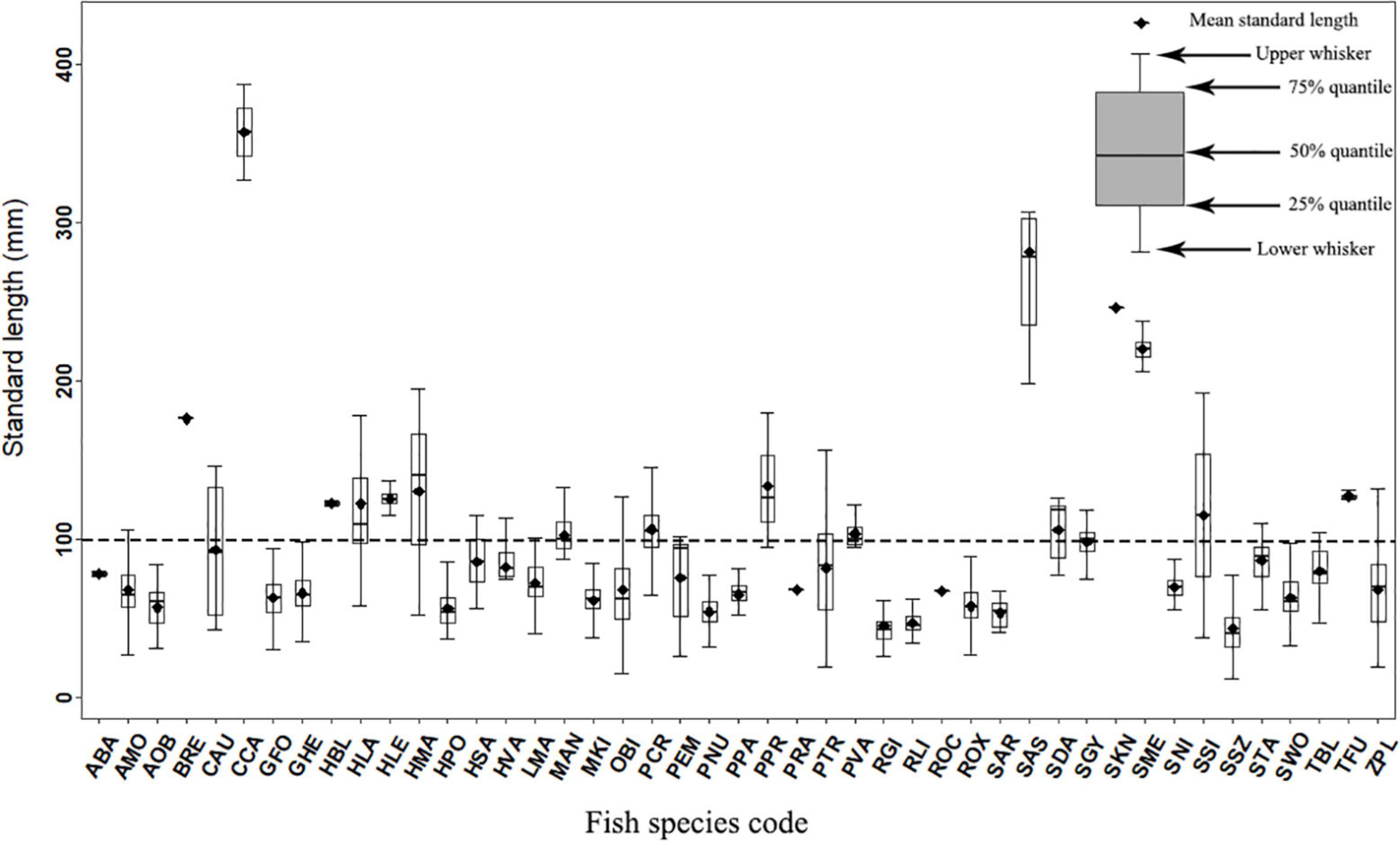
Figure 3. Box-Whisker plots of standard length for 45 species captured from the Datong and Xiaotong rivers.
The 45 fish species were classified into three trophic guilds: omnivore, herbivore, and carnivore (Table 1). The trophic guild with the highest proportion in the total catch was omnivore (53.33% of the total fish species; e.g., G. herzensteini, P. nudiventris, R. oxycephalus), followed by carnivores (37.78%), herbivores (8.89%) and detritivores (6.67%).
Spatial variations
Overall, both the Datong River and Xiaotong River had high species diversity and richness (Figure 4). Spatially, in the Datong River, species diversity was lower both in the upstream (LHK) and tributary (TX) than in the midstream (CP) and downstream (WS). In the Xiaotong River, there were slight differences in diversity between the upstream (NT), midstream (QYK) and downstream (FY), but they also showed a trend of increasing gradually from upstream to downstream. Species richness was the lowest in the upstream (LHK) of the Datong River, followed by the midstream (CP) and tributaries (TX), and the highest in the downstream (WS). Similar to the Datong River, species richness gradually increased from upstream to downstream in the Xiaotong River.
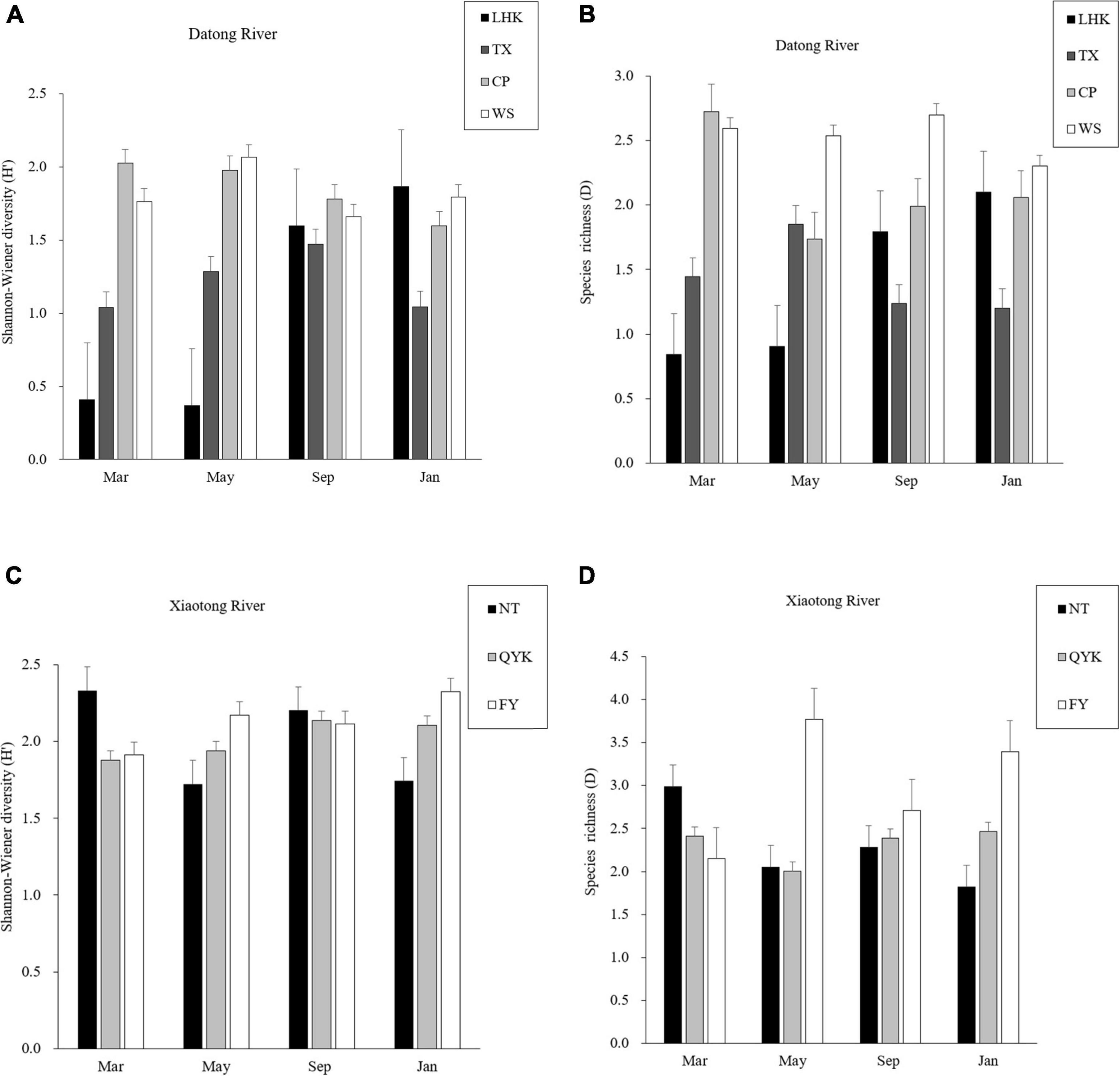
Figure 4. Mean value and standard deviation (SD) of Shannon-Winener diversity (H’), species richness (D) at seven reaches (NT, QYK, FY, LHK, TX, CP, WS) in 4 months. (A) Shannon-Wiener diversity (H’) of Datong river, (B) species richness (D) of Datong river, (C) Shannon-Wiener diversity (H’) of Xiaotong river, (D) species richness (D) of Xiaotong river.
One-way repeated-measured ANOVAs revealed that Shannon-Wiener diversity, species richness and Cypriniformes diversity significantly differed between the seven reaches (P < 0.05), but species evenness was not significantly different between reaches (P > 0.05; Table 2).
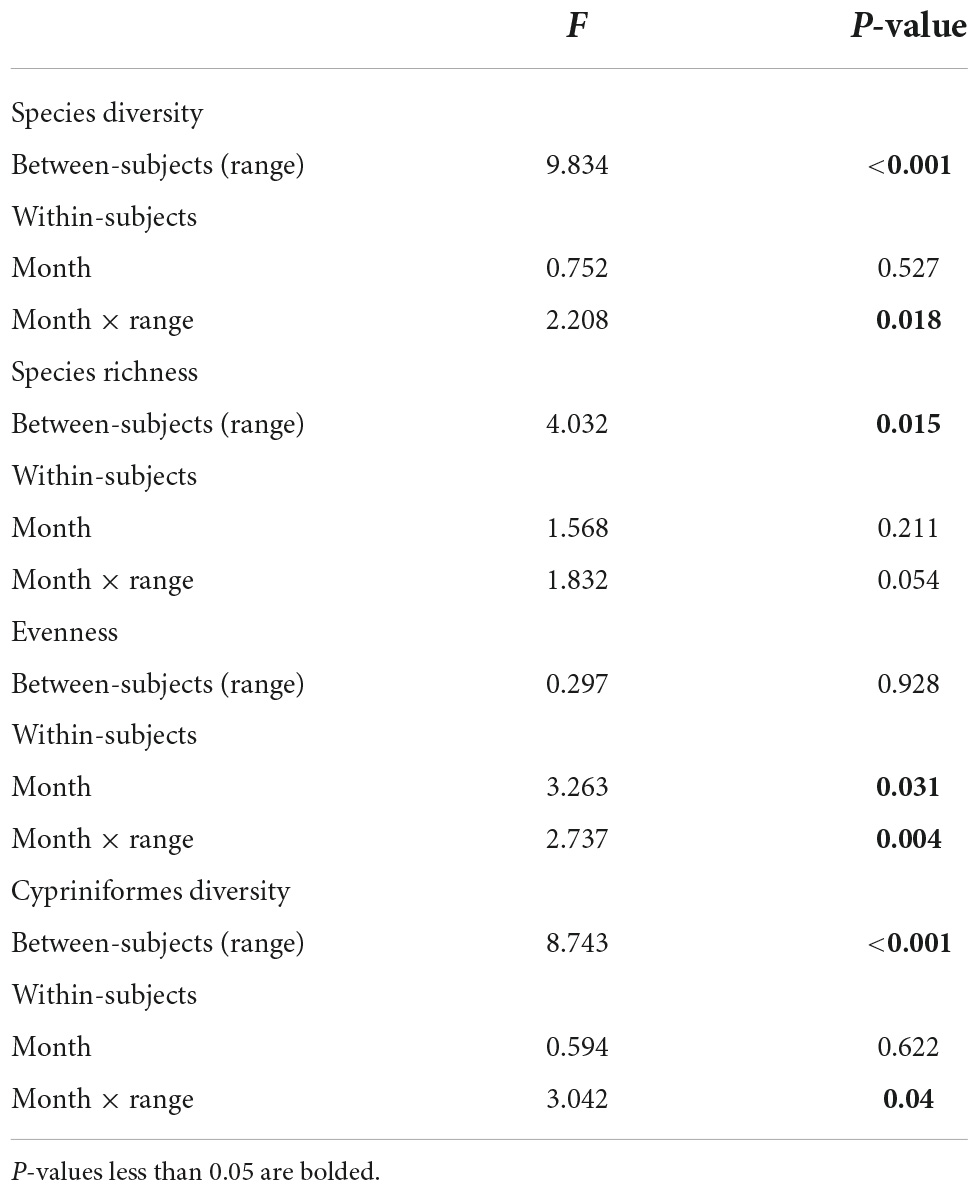
Table 2. One-way repeated measured ANOVA of effects of seven reaches (NT, QYK, FY, LHK, TX, CP, WS) in 4 months (March, May, September, January) on species diversity, richness, evenness and Cypriniformes diversity.
NMDS revealed clearly spatial groups of the sampling sites in the Datong and Xiaotong rivers. The sites in the downstream of the Datong River (WS) were clustered on the right, the ones in the upstream (LHK) were clustered to the left, while those in the midstream (CP) and the tributary (TX) were clustered at the bottom and middle, respectively (Figure 5). The sites in the upstream (NT), midstream (QYK) and downstream (FY) of the Xiaotong River were clustered at the top, middle and bottom, respectively (Figure 5).
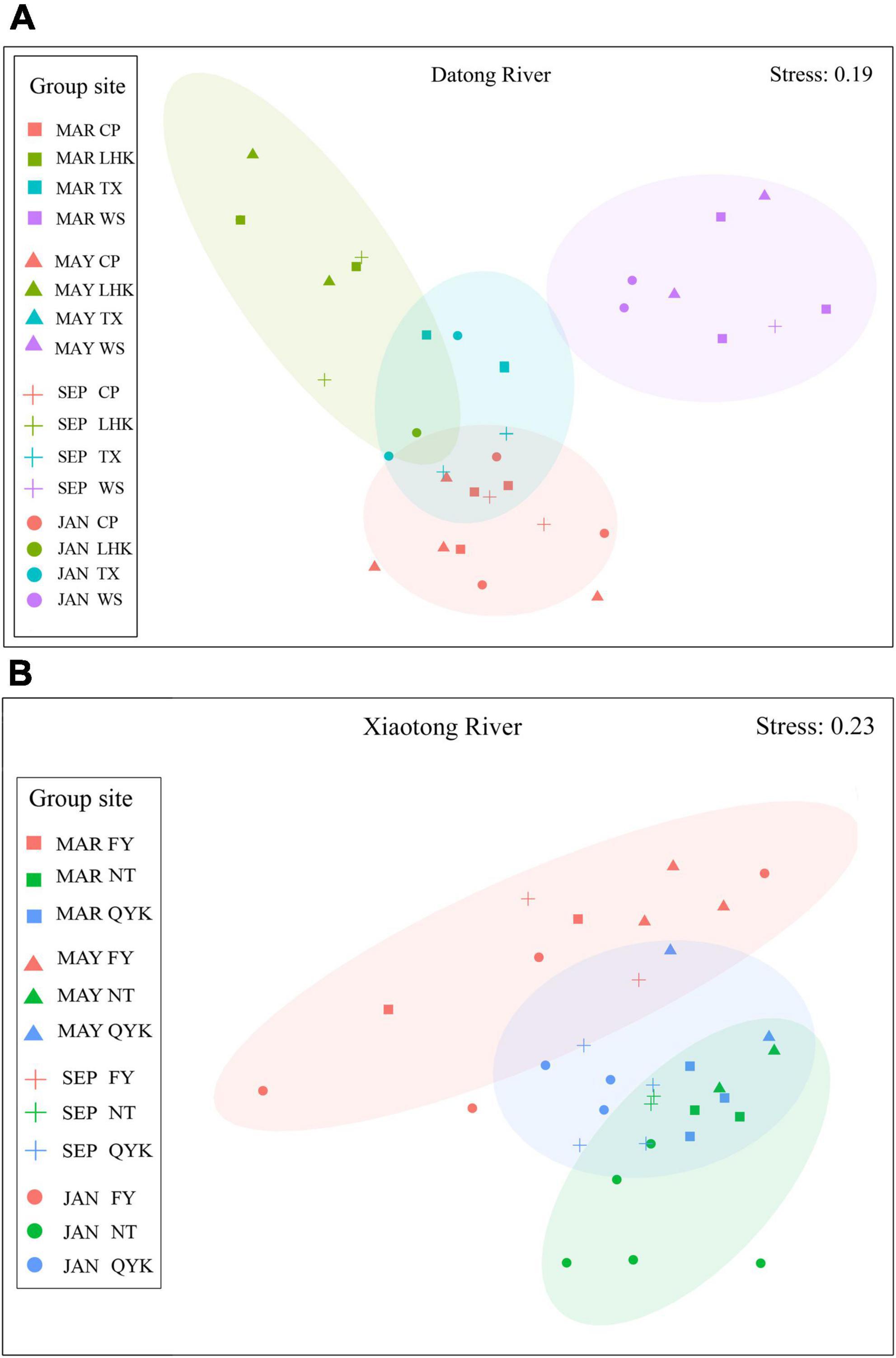
Figure 5. Non-metric multi-dimensional scaling (NMDS) by reaches (Datong River: LHK, TX, CP, WS; Xiaotong River: NT, QYK, FY) and months (March, May, September, January) for the abundance data of the Datong (A) and Xiaotong (B) rivers. Each symbol represents a month (dot, January; square, March; triangle, May; cross, September). Each color represents a range (orange, CP and FY; green, LHK and NT; blue, TX and QYK; purple, WS).
Similar to the NMDS results, two-way ANOSIM results indicated that there were significant spatial differences in fish community structure between the four reaches in the Datong River (Global R = 0.78, p = 0.0001) and between the three reaches in the Xiaotong River, although there was a clear spatial overlap in community structure (Global R = 0.39, p = 0.001; Table 3).
Analyses of similarity percentage (SIMPER) indicated that R. oxycephalus was a typical species of the upstream (LHK) and tributary (TX) reaches, contributing, respectively, to 29.76 and 12.48% of the similarity of the upstream (LHK) and tributary (TX) in the Datong River (Table 4). T. bleekeri was plentiful in the upstream (LHK) and contributed 11.91% to the similarity of the upstream (LHK). Moreover, Z. platypus was widely distributed in the tributary (TX) and midstream (CP) and contributed to 14.09 and 7.02% of the similarity. S. szechuanensis and P. nudiventris were widely distributed in the midstream (CP) and contributed to 10.69 and 7.19% of the similarity. S. taeniatus, S. gymnocheilus and P. parva were mainly distributed in the downstream (WS), contributing, respectively, to 15.08, 13.34, and 11.41% of the similarity of the downstream (WS).
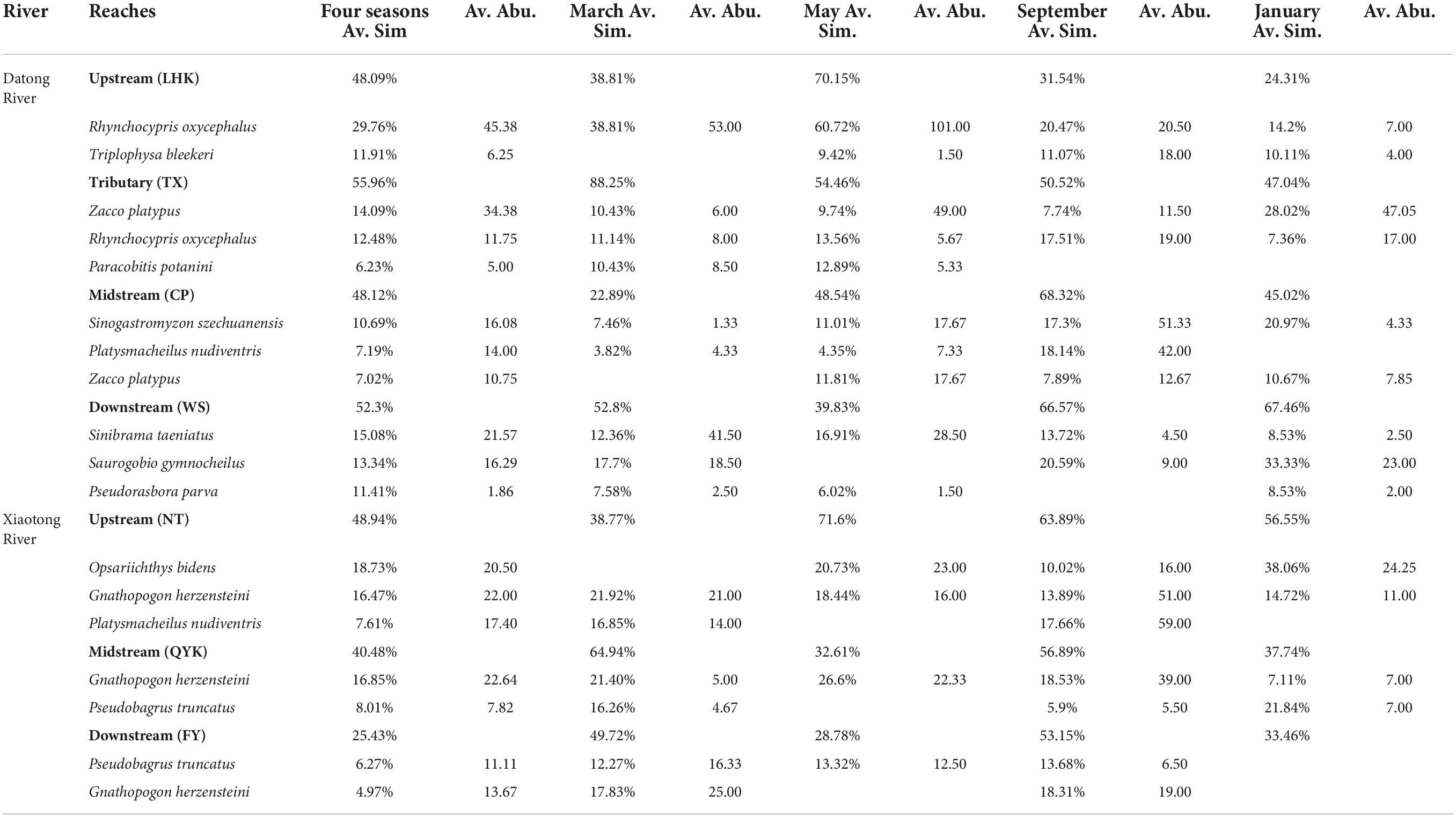
Table 4. Results of SIMPER analysis identifying the contribution of fish species to differences among the seven reaches (LHK, TX, CP, WS, NT, QYK, FY) in four months (March, May, Sep, Jan) in Datong and Xiaotong rivers.
In the Xiaotong River, G. herzensteini was the most common species, being widely distributed throughout all the river reaches. However, P. truncatus was more abundant in the midstream (QYK) and downstream (FY), contributing, respectively, to 8.01 and 6.27%. O. bidens and P. nudiventris were more plentiful in the upstream (NT) of the Xiaotong River, contributing to 18.73 and 7.61% of the similarity of the upstream (NT).
Temporal variations
Generally, the species diversity and richness of the Datong River and Xiaotong River did not change significantly between seasons (Figure 4). Seasonally, species diversity and richness did not differ significantly between the 4 months in the midstream (CP), tributary (TX) and downstream (WS) of the Datong River, whereas they were lower in March and May than in September and January in the upstream (LHK). In the Xiaotong River, the species diversity in the midstream (QYK) and downstream (FY) also did not vary significantly across the 4 months. The upstream (NT) had higher diversity in March and September, and lower diversity in May and January. The variation of richness with season was not obvious in the upstream (NT) and midstream (QYK) of the Xiaotong River, whereas the downstream (FY) reach had lower richness in March and higher richness in May, September and January.
One-way repeated-measured ANOVAs showed that there were no significant differences in Shannon-Wiener diversity, species richness and Cypriniforms diversity between the 4 months (P > 0.05), while species evenness significantly differed between months (P < 0.05; Table 2). The interactions of reach × month were also significant in fish diversities except species richness (P < 0.05; Table 2). Whereas, there was no clear clustering of sampling sites according to the different months in NMDS (Figure 5). Two-way ANOSIM results also indicated that there were no seasonal variations of the fish assemblages in each reach and the community structure overlapped significantly in the Datong River (Global R = 0.17, p = 0.0875) and Xiaotong River (Global R = 0.15, p = 0.0826; Table 3).
SIMPER analysis revealed that R. oxycephalus dominated the upstream (LHK) across the 4 months in the Datong River, and T. bleekeri was common in May, September and January (excluding March; Table 4). In the tributary (TX), Z. platypus and R. oxycephalus were abundant across the 4 months, P. potanini was abundant in March and May. S. szechuanensis dominated the midstream (CP), P. nudiventris was abundant in March, May and September (excluding January) and Z. platypus was common in May, September, January (excluding March). In the downstream (WS), S. taeniatus was abundant across the 4 months, S. gymnocheilus was abundant in March, September and January (excluding May) and P. parva was common in March, May and January (excluding September).
In the Xiaotong River, G. herzensteini dominated the upstream (NT), midstream (QYK) and downstream (FY) across the four seasons. O. bidens was plentiful in May, September and January (excluding March) and P. nudiventris was common only in March and September in the upstream (NT). In the midstream (QYK), P. truncatus was abundant in March, September and January (excluding May). P. truncatus was common in March, May and September (excluding January).
Discussion
Size and trophic guild changes due to the anthropogenic disturbances
The frequency distribution of species length showed that the Datong and Xiaotong rivers were dominated by small-sized individuals, which accounted for 99.81% of the total individuals and 91.11% of the total species. Instead, the large and medium-sized fishes such as S. asotus, S. meridionalis, C. carpio and S. knerii had a narrow geographic distribution and low quantities. It is likely to have been caused by frequent fishing that targeted the more commercially valuable large and medium-sized species. Some studies have also shown that smaller fish dominating the catch are often the signs of overfishing (Beverton and Holt, 1993; Marcos and Christopher, 2004).
According to the River Continuum Concept, the upstream fish assemblage was expected to be dominated by herbivore, insectivore and frugivore fish since food sources are mainly from riparian vegetation and its associated fauna (Vannote et al., 1980; Araújo et al., 2009; Wang et al., 2019). In comparison, fishes from downstream were predicted to be dominated by carnivorous, omnivorous and detritivorous species. In the Datong and Xiaotong rivers, most of the fish were omnivorous and carnivorous species, which accounted for 91.11% of the total species. However, the resources of herbivorous species such as Onychostoma simum and Sinilabeo redahli have declined. In addition, the large- or medium-sized carnivorous species such as Silurus asotus, S. meridionalis and S. knerii, have declined but the small-sized carnivorous species such as P. crassilabris, Pseudobagrus truncatus and Liobagrus marginatoides still have some resources. One reason for this may stem from anthropogenic disturbances such as overfishing.
Spatial variations in fish assemblages along the upstream to downstream
We found significant longitudinal variation in fish assemblages in the Datong and Xiaotong rivers. Overall, our findings match general patterns for fish communities in riverine systems (Welcomme, 1985; Cowx and Welcomme, 1998; Vila-Gispert et al., 2002), where species are replaced gradually along the longitudinal gradient rather than abruptly. One-way repeated-measured ANOVA and related diversity indices in the Datong and Xiaotong rivers indicated that species richness, diversity and abundance gradually increased from upstream to downstream. Longitudinal position has been extensively studied as a major predictor of fish assemblage structure in riverine ecosystems. In accordance with a previous study in an undisturbed river ecosystem, aquatic animals tend to vary along an upstream to downstream gradient that implies the current velocity, deepness and the substrate control the spatial distribution of fish assemblages (Vila-Gispert et al., 2002). In our study, the variation of altitude, water depth, current velocity and substrate heterogeneity may be responsible for generating longitudinal variation in fish community structure in the Xiaotong and Datong rivers.
The two rivers are karst rivers which have karst landform characteristics. Although those two karst rivers were separated by the dams, they had diverse habitats such as riffle and pool mesohabitat and therefore the impact of Jiuyuxi and Shiniuzui dams was limited for the fish community structure. In addition, the similar results were found in some other karstic landsform rivers (White et al., 2009; Pedro et al., 2018). Even in karst landscapes, where stream continuity is naturally interrupted by obstacles such as subterranean stretches, the river continuum is still maintained (Vannote et al., 1980; White et al., 2009; Pedro et al., 2018). This suggested that karst geomorphic rivers may be somewhat resilient to disturbance (Kollaus et al., 2015). Nevertheless, despite fish community dynamic systems being resilient, karst river systems are not immune to anthropogenic disturbances such as overfishing (White et al., 2009). Therefore, in future studies, we need to be more focused on the influence of anthropogenic disturbances on the longitudinal structure of fish community in karst rivers.
No temporal variations in fish assemblages attribute to diverse mesohabitat
We found there was no significant seasonal variation in fish assemblages in the Datong and Xiaotong rivers. Species richness and Shannon-Wiener diversity of fish also did not change over the four seasons. Fluvial systems are dynamic and fish species diversity and assemblage are known to seasonally change in temperate rivers (Deudero et al., 1999; Carpentier et al., 2004) and tropical rivers (Dansoko et al., 1976; Laë, 1992; Garcia et al., 2003; Rueda and Defeo, 2003) in association with the flood periodicity and the availability of breeding habitat and food items (Winemiller and Jepsen, 1998; Junk and Wantzen, 2006). In the Datong and Xiaotong riverine system, a large number of pool and riffle mesohabitats alternated, that facilitated fish short-distance reproduction, feeding and overwintering migration, which accounted for the lack of temporal variance. The channel morphologies were predominantly categorized into cascade, step-pool, plane-bed, pool-riffle, and dune-ripple by Montgomery and Buffington’s (1997). The Datong and Xiaotong rivers are located in the karst landscape area of southwest China, and they not only have 128 karst caves and numerous underground rivers (Qi, 2018), but also have a variety of channel morphologies such as riffle and pool. Although we found no long-distance migratory fish in the Datong and Xiaotong rivers, most species had short-distance migration habits such as spawning, feeding and overwintering. Fishes migrate relatively short distances because adequate habitats exist in close proximity (Makrakis et al., 2012). The benefits would be that fish may not need to migrate long distances to spawning, feeding or overwintering grounds. We speculated that it may be a consequence of the diverse habitats caused by large distribution of pool-riffle mesohabitats, resulting in no significance of seasonal variation in fish community.
Similar findings have been found in freshwater rivers in China, such as the Zhougong River (Wang et al., 2019). The findings indicated that no seasonal change in fish assemblage were partially attributed to the physicochemical changes caused by cascade hydroelectric dams (e.g., channel blocking, hydrological alterations), pollution (e.g., phosphorus) and it is also likely that overfishing has exacerbated impacts (Kadye and Marshall, 2010; Wang et al., 2019). While Araújo et al. (2009) found seasonal differences in fish community structure in a large tropical river with no habitat diversity in southeastern Brazil. Therefore, when evaluating the seasonal variation of river, it is important to consider habitats, such as pool and riffle mesohabitats, as fish assemblages may be influenced by habitat diversity (Gorman and Karr, 1978; Schlosser, 1982; Martin-Smith, 1998; Goldstein and Meador, 2004; Erõs and Grossman, 2005; Erõs et al., 2010; Troia and Gido, 2015; Wolff and Hahn, 2017).
Conservation implications
Our findings indicated that large and medium-sized individuals, carnivorous and herbivorous species in the Datong and Xiaotong rivers had decreased due to overfishing. Due to water pollution, overfishing and dam construction, the Yangtze River faces a depletion of fishery resources (Liu et al., 2019). However, fishing has been prohibited in the Yangtze River basin since 2020, which includes the Datong and Xiaotong rivers, and fish population stocks have gradually recovered. We consequently suggest the government conduct an efficient, long-term evaluation of the river fishing ban’s effectiveness. In several countries, over-exploitation of fish caught for bait has been controlled through three types of action: (1) bans on fishing, (2) limits on the number of fish captured, (3) regulation of the type of equipment used (Pipitone et al., 2000; Bartholomew and Bohnsack, 2005; Cooke et al., 2012). The ban on the number of individuals caught is effective for population recovery in several areas ensuring the sustainability of fishing (Wynberg and Branch, 1991; Anderson et al., 2011). Nevertheless, population restoration was quite slow, thus we suggest that releasing mature and large-sized native fishes in the Datong and Xiaotong rivers would accelerate wild population recovery.
The spatial results indicated that fish communities had a continuous variation from upstream to downstream in the two rivers and are easily disrupted by anthropogenic perturbations. Therefore, protecting single sections of the two rivers is insufficient for conserving the entire rivers’ fish assemblage, and the managers should protect the whole river and maintain connectivity. Moreover, it is important in fish conservation to identify areas of high fish biodiversity and select nature reserves to mitigate the loss of fish biodiversity (Fu et al., 2003). Fortunately, most of the Datong and Xiaotong rivers have been incorporated into the “Sichuan Nuoshuihe Precious Aquatic Animal National Nature Reserve” in 2012 by the government (Liang et al., 2021). The Chishui River in China is a free-flowing river which varies gradually in geomorphologic structure along its longitudinal gradient, meanwhile, it is located in “National Nature Reserve for the Rare and Endemic Fishes in Upper Reaches of the Yangtze River” (Wu et al., 2011; Liu et al., 2021). As a result, the fish resource in the Chishui River has now been restored to a certain extent (Liu et al., 2021). Therefore, we consider that designating the Datong and Xiaotong rivers as a nature reserve currently is effective for protecting the longitudinal structure of the fish community.
In terms of temporal dynamics in fish community structure of the two rivers, the following measures should be considered to reduce adverse impacts. (1) Protect the riffle and pool Mesohabitat diversity and reduce the damage to fish diversity caused by human disturbance. (2) Loss of connectivity between riffles and pools may lead to native fish extinctions, maintenance of migration routes of fish is essential for conservation of river fish in karst rivers (Chakona et al., 2022). Thus, it is necessary to take measures such as banning water resources exploitation when flows are below a certain level to keep connectivity between different habitats. (3) Sand excavation activities, particularly in those areas that provide for fish spawning, feeding or overwintering in the Datong and Xiaotong rivers, should be prohibited by the government. Currently, although these mesohabitats are relatively well-protected, there are also potential threats to fish mesohabitat from sand excavation. Moreover, a finding in an African karst river with seasonal variation in fish communities showed that damming and impoundment have negative impacts on fish and improvements in the design of dams to allow fish migration, regulation of the controlled use of the river water would help for fish diversity and abundance (Bem et al., 2021). Therefore, we also suggest that the water-related projects be limited, and the short and long-term biological impacts of dams be monitored to avoid the temporal structure of fish communities being disrupted by human factors.
Conclusion
In summary, we found that the Datong and Xiaotong rivers were dominated by small-sized individuals and few large-sized fish. Additionally, the population of herbivorous and large or medium-sized carnivorous species has declined. The results showed that fish assemblages in two rivers varied significantly along the longitudinal gradient, while there were no significant differences between the four seasons. The spatial differences and no seasonal change in fish assemblage were partially attributed to the diverse habitats of riffles and pools within a relatively short reach of the karst streams and the ecological habit of most fish species with short-distance migration for spawning, feeding or overwintering. Understanding the spatial and temporal dynamics of fish assemblages and what anthropogenic disturbances have contributed to these changes is of great significance to formulate efficient management policy. Nevertheless, the findings indicated that fish assemblages have been impacted by overfishing. Therefore, we suggest that the managers should take measures such as conducting long-term investigation, releasing mature and large-sized native fishes to restore populations and protecting the riffle and pool mesohabitats and channel connectivity.
Data availability statement
The original contributions presented in this study are included in the article/supplementary material, further inquiries can be directed to the corresponding author.
Ethics statement
Ethical review and approval was not required for the animal study because All individuals were released into the water where captured, except for a few specimens that were anesthetized by MS-222 solution and fixed with formaldehyde solution (5∼10%) after death.
Author contributions
PX: investigation, writing, analysis, software, and revision. XW: investigation and writing advice. KL and BW: investigation. CL: writing advice. ZS: revision and writing advice. All authors contributed to the article and approved the submitted version.
Funding
This work was supported by scientific research project of the Bureau of Aquatic Products of Sichuan Province.
Acknowledgments
We thank Qinchao Wen, Yi Lei, Shu Li, and Maohua Li for their assistance in the field sampling and Megan Price for her help in revising the manuscript.
Conflict of interest
BW was employed by Chengdu Jena Environmental Protection Technology Company.
The remaining authors declare that the research was conducted in the absence of any commercial or financial relationships that could be construed as a potential conflict of interest.
Publisher’s note
All claims expressed in this article are solely those of the authors and do not necessarily represent those of their affiliated organizations, or those of the publisher, the editors and the reviewers. Any product that may be evaluated in this article, or claim that may be made by its manufacturer, is not guaranteed or endorsed by the publisher.
References
Adams, S., Warren, M., and Haag, W. (2004). Spatial and temporal patterns in fish assemblages of upper coastal plain streams. Mississippi, USA. Hydrobiologia 528, 45–61. doi: 10.1007/s10750-004-1659-7
Allan, J. D. (2004). Landscapes and riverscapes: the influence of land use on stream ecosystems. Ann. Rev. Ecol. Evol. Syst. 35, 257–284. doi: 10.1146/annurev.ecolsys.35.120202.110122
Anderson, S. C., Flemming, J. M., Watson, R., and Lotze, H. K. (2011). Serial exploitation of global sea cucumber fisheries. Fish Fish. 12, 317–339. doi: 10.1111/j.1467-2979.2010.00397.x
Araújo, F., Pinto, B., and Teixeira, T. P. (2009). Longitudinal patterns of fish assemblages in a large tropical river in southeastern Brazil: evaluating environmental influences and some concepts in river ecology. Hydrobiologia 618, 89–107. doi: 10.1007/s10750-008-9551-5
Barletta, M., Barletta-Bergan, A., Saint-Paul, U., and Hubold, G. (2003). Seasonal changes in density, biomass, and diversity of estuarine fishes in tidal mangrove creeks of the lower caeté estuary (northern brazilian coast, east amazon). Mar. Ecol. Progr. 256, 217–228. doi: 10.3354/meps256217
Barletta, M., Barletta-Bergan, A., Saint-Paul, U., and Hubold, G. (2005). The role of salinity in structuring the fish assemblages in a tropical estuary. J. Fish Biol. 66, 45–72. doi: 10.1111/j.0022-1112.2005.00582.x
Barletta-Bergan, A., Barletta, M., and Saint-Paul, U. (2002). Community structure and temporal variability of ichthyoplankton in North Brazilian mangrove creeks. J. Fish Biol. 61, 33–51. doi: 10.1111/j.1095-8649.2002.tb01759.x
Bartholomew, A., and Bohnsack, J. A. (2005). A review of catch-and-release angling mortality with implications for no-take reserves. Rev. Fish Biol. Fish. 15, 129–154. doi: 10.1007/s11160-005-2175-1
Bem, J. D., Ribolli, J., RoPke, C., Winemiller, K. O., and Zanibonifilho, E. (2021). A cascade of dams affects fish spatial distributions and functional groups of local assemblages in a subtropical river. Neotr. Ichthyol. 19:e200133. doi: 10.1590/1982-0224-2020-0133
Beverton, R., and Holt, S. J. (1993). On the Dynamics of Exploited Fish Populations. Netherlands: Springer.
Carpentier, A., Paillisson, J. M., Feunteun, E., and Marion, L. (2004). Fish community structure in temporary lowland flooded grasslands. Knowledge Manag. Aquat. Ecosyst. 375, 1–14. doi: 10.1051/kmae:2005037
Chakona, A., Jordaan, M. S., Raimondo, D. C., Bills, R. I., Skelton, P. H., and van Der Colff, D. (2022). Diversity, distribution and extinction risk of native freshwater fishes of South Africa. J. Fish Biol. 100, 1044–1061. doi: 10.1111/jfb.15011
Clarke, K., and Warwick, R. (1994). An approach to statistical analysis and interpretation. Change Mar. Commun. 2, 117–143. doi: 10.7863/jum.2011.30.10.1415
Clarke, K. R., and Warwick, R. (2001). Change in marine communities. Appr. Stat. Anal. Interpr. 2, 1–68.
Cooke, S. J., Paukert, C., and Hogan, Z. (2012). Endangered river fish: factors hindering conservation and restoration. Endangered Species Res. 17, 179–191. doi: 10.3354/esr00426
Covich, A. P. (2006). Dispersal-limited biodiversity of tropical insular streams. Polish J. Ecol. 54, 523–547. doi: 10.1017/s0266467404002159
Cowx, I. G., and Welcomme, R. L. (1998). Rehabilitation of Rivers for Fish. Rome: European Inland Fisheries Advisory Commission.
Cyrus, D. P., and Blaber, S. (1987). The influence of turbidity on juvenile marine fishes in estuaries. Part 2. Laboratory studies, comparisons with field data and conclusions. J. Exp. Mar. Biol. Ecol. 109, 71–91. doi: 10.1016/0022-0981(87)90186-9
Dansoko, D., Breman, H., and Daget, J. (1976). Influence de la sécheresse sur les populations d’Hydrocynus dans le delta central du Niger. Cahier ORSTOM 10, 71–76. doi: 10.4000/books.pur.14075
Deudero, S., Merella, P., Morales-Nin, B., Massuti, E., and Alemany, F. (1999). Fish communities associated with FADs. Sci. Mar. 63, 199–207. doi: 10.3989/scimar.1999.63n3-4199
Dudgeon, D. (2000). Large-scale hydrological changes in tropical Asia: prospects for riverine biodiversity: the construction of large dams will have an impact on the biodiversity of tropical Asian rivers and their associated wetlands. BioScience 50, 793–806.
Erõs, T., and Grossman, G. D. (2005). Fish biodiversity in two Hungarian streams: a landscape-based approach. Arch. Hydrobiol. 162, 53–71. doi: 10.1127/0003-9136/2005/0162-0053
Erõs, T., Heino, J., Schmera, D., and Rask, M. (2010). Characterising functional trait diversity and trait-environment relationships in fish assemblages of boreal lakes. Freshw. Biol. 54, 1788–1803. doi: 10.1111/j.1365-2427.2009.02220.x
Fernandes, C. C. (1997). Lateral migration of fishes in Amazon floodplains. Ecol. Freshw. Fish. 6, 36–44. doi: 10.1111/j.1600-0633.1997.tb00140.x
Fráguas, P. S., and Pompeu, P. S. (2021). Hydropower affects fish trophic structure both downstream of the dam and upstream of the reservoir. Neotr. Ichthyol. 19:e200071. doi: 10.1590/1982-0224-2020-0071
Fraley, K. M., Falke, J. A., Yanusz, R., and Ivey, S. (2016). Seasonal movements and habitat use of potamodromous rainbow trout across a complex alaska riverscape. Trans. Am. Fish. Soc. 145, 1077–1092. doi: 10.1080/00028487.2016.1202320
Fu, C., Wu, J., Chen, J., Wu, Q., and Lei, G. (2003). Freshwater fish biodiversity in the Yangtze River basin of China: patterns, threats and conservation. Biodivers. Conserv. 12, 1649–1685. doi: 10.1023/A:1023697714517
Garcia, A. M., Raseira, M. B., Vieira, J. P., Winemiller, K. O., and Grimm, A. M. (2003). Spatiotemporal variation in shallow-water freshwater fish distribution and abundance in a large subtropical coastal lagoon. Environ. Biol. Fish. 68, 215–228. doi: 10.1023/a:1027366101945
Goldstein, R. M., and Meador, M. R. (2004). Comparisons of fish species traits from small streams to large rivers. Trans. Am. Fish. Soc. 133, 971–983. doi: 10.1577/t03-080.1
Gorman, O. T., and Karr, J. R. (1978). Habitat structure and stream fish communities. Ecology 59, 507–515. doi: 10.2307/1936581
Hadley, W. (2016). ggplot2: Elegant Graphics for Data Analysis, Vol. 35. New York, NY: Springer-Verlag, doi: 10.18637/jss.v035.b01
He, D., Kang, Z., Tao, J., Liu, C., Yang, J., and Chen, Y. (2017). Hydrologic connectivity driven natural stream fish assemblages in mountain streams in the Yangtze River basin: implications for stream fish conservation in monsoonal East Asia. Hydrobiologia 785, 185–206. doi: 10.1007/s10750-016-2920-6
Ibarra, A. A., Park, Y. S., Brosse, S., Reyjol, Y., Lim, P., and Lek, S. (2005). Nested patterns of spatial diversity revealed for fish assemblages in a west European river. Ecol. Freshw. Fish. 14, 233–242. doi: 10.1111/j.1600-0633.2005.00096.x
Ives, J. T., Mcmeans, B. C., Mccann, K. S., Fisk, A. T., Johnson, T. B., Bunnell, D. B., et al. (2019). Food-web structure and ecosystem function in the laurentian great lakes-toward a conceptual model. Freshw. Biol. 64, 1–23. doi: 10.1111/fwb.13203
Junk, W. J., and Wantzen, K. M. (2006). “Flood pulsing and the development and maintenance of biodiversity in floodplains,” in Ecology of Freshwater and Estuarine Wetlands, eds D. P. Batzer and R. R. Sharitz (Berkeley: University of California Press), 407–435. doi: 10.1525/9780520932890-013
Kadye, W. T., and Marshall, B. E. (2010). Habitat diversity and fish assemblages in an African river basin (Nyagui River, Zimbabwe). Afr. J. Ecol. 45, 374–381. doi: 10.1111/j.1365-2028.2006.00723.x
Kennard, M., Pusey, B., Arthington, A., Harch, B., and Mackay, S. (2006). Development and application of a predictive model of freshwater fish assemblage composition to evaluate river health in eastern Australia. Hydrobiologia 572, 33–57. doi: 10.1007/s10750-005-0993-8
Kollaus, K. A., Behen, K. P., Heard, T. C., Hardy, T. B., and Bonner, T. H. (2015). Influence of urbanization on a karst terrain stream and fish community. Urban Ecosyst. 18, 293–320. doi: 10.1007/s11252-014-0384-x
Kraft, N., Cornwell, W. K., Webb, C. O., and Ackerly, D. D. (2007). Trait evolution, community assembly, and the phylogenetic structure of ecological communities. Am. Nat. 170, 271–283. doi: 10.1086/519400
Laë, R. (1992). Influence de l’hydrologie sur l’évolution des pêcheries du delta central du Niger, de 1966 à 1989. Aquat. Living Resour. 5, 115–126. doi: 10.1051/alr:1992012
Liang, C., Liu, K., Wang, X. D., Li, S., Wu, B., Xiang, P., et al. (2021). The status of fish resources in Sichuan Nuoshui River Rare Aquatic Animal National Nature Reserve. Biodivers. Sci. 29, 938–949. doi: 10.17520/biods.2021010
Liu, F., Lin, P. C., Li, M. Z., Gao, X., Wang, C. L., and Liu, H. Z. (2019). Situations and conservation strategies of fish resources in the Yangtze River basin. Acta Hydrobiol. Sin. 43, 244–156. doi: 10.7541/2019.177
Liu, F., Wang, J., Zhang, F. B., Liu, H. Z., and Wang, J. W. (2021). Spatial organisation of fish assemblages in the Chishui River, the last free-flowing tributary of the upper Yangtze River, China. Ecol. Freshw. Fish. 30, 48–60. doi: 10.1111/eff.12562
Lowe-McConnell, R. H. (1999). Estudos Ecológicos de Comunidades Depeixes Tropicais. São Paulo: EDUSP, 534.
Magurran, A. E. (1988). “Diversity indices and species abundance models,” in Ecological diversity and its measurement, ed. A. Magurran (Dordrecht: Springer), 7–45. doi: 10.1007/978-94-015-7358-0_2
Magurran, A. E. (2004). Measuring biological diversity. Maldan, MA: Blackwell Science. doi: 10.2307/4126959
Makrakis, M., Miranda, L., Makrakis, S., Fontes Júnior, H., Morlis, W., Dias, J., et al. (2012). Diversity in migratory patterns among Neotropical fishes in a highly regulated river basin. J. Fish Biol. 81, 866–881. doi: 10.1111/j.1095-8649.2012.03346.x
Marcos, J. D., and Christopher, C. K. (2004). The Commercial Fishery of the Peruvian Amazon. Fisheries 29, 10–16. doi: 10.1007/s10695-008-9212-7
Martin-Smith, K. M. (1998). Relationships between fishes and habitat in rainforest streams in Sabah, Malaysia. J. Fish Biol. 52, 458–482. doi: 10.1006/jfbi.1997.0594
Melcher, A. H., Ouedraogo, R., and Schmutz, S. (2012). Spatial and seasonal fish community patterns in impacted and protected semi-arid rivers of Burkina Faso. Ecol. Eng. 48, 117–129. doi: 10.1016/j.ecoleng.2011.07.012
Montgomery, D. R., and Buffington, J. M. (1997). Channel-reach morphology in mountain drainage basins. Geol. Soc. Am. Bull. 109, 596–611.
Naiman, R., and Latterell, J. (2005). Principles for linking fish habitat to fisheries management and conservation. J. Fish Biol. 67, 166–185. doi: 10.1111/j.0022-1112.2005.00921.x
Nazeer, S., Hashmi, M. Z., and Malik, R. N. (2016). Spatial and seasonal dynamics of fish assemblage along river Soan. Pakistan and its relationship with environmental conditions. Ecol. Indic. 69, 780–791. doi: 10.1016/j.ecolind.2016.05.034
Oksanen, J., Blanchet, F. G., Friendly, M., Kindt, R., Legendre, P., McGlinn, D., et al. (2022). vegan: Community ecology package. R package version 2.6-2. Available online at: http://mirrors.ustc.edu.cn/CRAN/web/packages/vegan/
Ostrand, K., and Wilde, G. (2002). Seasonal and spatial variation in a prairie stream-fish assemblage. Ecol. Freshw. Fish. 11, 137–149. doi: 10.1034/j.1600-0633.2002.00005.x
Pedro, R., Lopes, F. R., and Santos, P. P. (2018). Fish community of a small karstic Neotropical drainage and its relationship with the physical habitat. Mar. Freshw. Res. 69, 1312–1320. doi: 10.1071/mf17167
Pipitone, C., Badalamenti, F., D’Anna, G., and Patti, B. (2000). Fish biomass increase after a four-year trawl ban in the Gulf of Castellammare (NW Sicily, Mediterranean Sea). Fish. Res. 48, 23–30. doi: 10.1016/s0165-7836(00)00114-4
Poff, N. L., and Allan, J. D. (1995). Functional organization of stream fish assemblages in relation to hydrological variability. Ecology 76, 606–627. doi: 10.2307/1941217
Qi, C. L. (2018). Geological Heritage Landscape Evaluation of Guangwushan-Nuoshuihe National Geological Park and Studies on its Protective Development. Chengdu: Chengdu University of Technology.
Rahel, F. J., and Hubert, W. A. (1991). Fish assemblages and habitat gradients in a rocky mountain–great plains stream: Biotic zonation and additive patterns of community change. Tran. Am. Fish. Soc. 120, 319–332.
Rice, J. (2005). Understanding fish habitat ecology to achieve conservation. J. Fish Biol. 67, 1–22. doi: 10.1111/j.0022-1112.2005.00933.x
Rodriguez, M. A., and Lewis, W. M. (1997). Structure of fish assemblages along environmental gradients in floodplain lakes of the Orinoco River. Ecol. Monogr. 67, 109–128. doi: 10.2307/2963507
Rueda, M., and Defeo, O. (2003). Spatial structure of fish assemblages in a tropical estuarine lagoon: combining multivariate and geostatistical techniques. J. Exp. Mar. Biol. Ecol. 296, 93–112. doi: 10.1016/s0022-0981(03)00319-8
Schlosser, I. J. (1982). Fish community structure and function along two habitat gradients in a headwater stream. Ecol. Monogr. 52, 395–414.
Silvano, R. A., Do Amaral, B. D., and Oyakawa, O. T. (2000). Spatial and temporal patterns of diversity and distribution of the Upper Juruá River fish community (Brazilian Amazon). Environ. Biol. Fish. 57, 25–35. doi: 10.1023/a:1007594510110
Stanford, J. A., and Ward, J. V. (2001). Revisiting the serial discontinuity concept. River Res. Appl. 17, 303–310.
Troia, M. J., and Gido, K. B. (2015). Functional strategies drive community assembly of stream fishes along environmental gradients and across spatial scales. Oecologia 177, 545–559. doi: 10.1007/s00442-014-3178-1
Vannote, R. L., Minshall, G. W., Cummins, K. W., Sedell, J. R., and Cushing, C. E. (1980). The river continuum concept. Can. J. Fish. Aquat. Sci. 37, 130–137. doi: 10.1139/f85-129
Vila-Gispert, A., García-Berthou, E., and Moreno-Amich, R. (2002). Fish zonation in a Mediterranean stream: effects of human disturbances. Aquat. Sci. 64, 163–170. doi: 10.1007/s00027-002-8064-y
Wang, X. D., Li, S., Price, M., Lei, Y., Wu, B., Liu, K., et al. (2019). Longitudinal and seasonal patterns of fish assemblage structure in the Zhougong River. Sichuan Province, southwest China. Ecol. Indic. 107:105656. doi: 10.1016/j.ecolind.2019.105656
White, K., Davidson, G. R., and Paquin, P. (2009). Hydrologic evolution of the Edwards Aquifer recharge zone (Balcones fault zone) as recorded in the DNA of eyeless Cicurina cave spiders, south-central Texas. Geology 37, 339–342. doi: 10.1130/g25373a.1
Wickham, H., Chang, W., and RStudio. (2016). ggplot2: Create Elegant Data Visualisations Using the Grammar of Graphics. Available online at: https://cran.r-project.org/web/packages/ggplot2/ggplot2.pdf (accessed on May 03, 2022).
Winemiller, K. O., and Jepsen, D. B. (1998). Effects of seasonality and fish movement on tropical river food webs. J. Fish Biol. 53, 267–296. doi: 10.1111/j.1095-8649.1998.tb01032.x
Wolff, L. L., and Hahn, N. S. (2017). Fish habitat associations along a longitudinal gradient in a preserved coastal Atlantic stream, Brazil. Zoologia 34, 1–13. doi: 10.3897/zoologia.34.e12975
Wolter, C., Buijse, A. D., and Parasiewicz, P. (2015). Temporal and spatial patterns of fish response to hydromorphological processes. River Res. Appl. 32, 190–201. doi: 10.1002/rra.2980
Wu, J., Wang, J., He, Y., and Cao, W. (2011). Fish assemblage structure in the Chishui River, a protected tributary of the Yangtze River. Knowledge Manag. Aquat. Ecosyst. 400:11. doi: 10.1051/kmae/2011023
Wynberg, R., and Branch, G. (1991). An assessment of bait-collecting for Callianassa kraussi Stebbing in Langebaan Lagoon, Western Cape, and of associated avian predation. South Afr. J. Mar. Sci. 11, 141–152. doi: 10.2989/025776191784287592
Keywords: fish assemblage, spatial-seasonal dynamics, karst river, upper Yangtze River, habitat with riffles and pools
Citation: Xiang P, Wang X, Liu K, Wu B, Liang C and Song Z (2022) Spatio-temporal dynamics of fish assemblage in the Datong and Xiaotong rivers, karst tributaries in the upper Yangtze River drainage: Implications for ecological adaptation and conservation of fish in rivers. Front. Ecol. Evol. 10:956555. doi: 10.3389/fevo.2022.956555
Received: 30 May 2022; Accepted: 07 September 2022;
Published: 26 September 2022.
Edited by:
Min Zhang, China Institute of Water Resources and Hydropower Research, ChinaReviewed by:
Peng Ren, Dongying Research Institute for Oceanography Development, ChinaJianzhong Shen, Huazhong Agricultural University, China
Copyright © 2022 Xiang, Wang, Liu, Wu, Liang and Song. This is an open-access article distributed under the terms of the Creative Commons Attribution License (CC BY). The use, distribution or reproduction in other forums is permitted, provided the original author(s) and the copyright owner(s) are credited and that the original publication in this journal is cited, in accordance with accepted academic practice. No use, distribution or reproduction is permitted which does not comply with these terms.
*Correspondence: Zhaobin Song, zbsong@scu.edu.cn