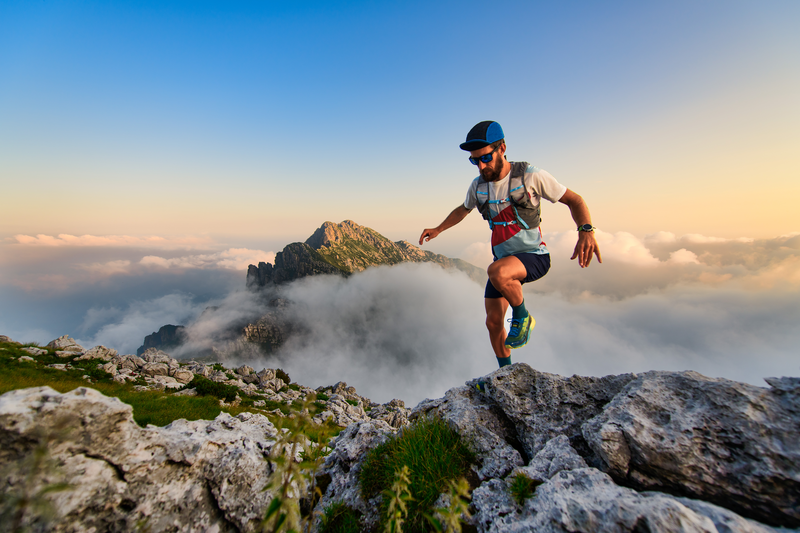
94% of researchers rate our articles as excellent or good
Learn more about the work of our research integrity team to safeguard the quality of each article we publish.
Find out more
ORIGINAL RESEARCH article
Front. Ecol. Evol. , 14 July 2022
Sec. Conservation and Restoration Ecology
Volume 10 - 2022 | https://doi.org/10.3389/fevo.2022.953349
This article is part of the Research Topic Disturbance, Resilience and Restoration of Wetlands View all 20 articles
Wetlands store >30% of the global soil carbon pool, which is important for global carbon cycling. However, with global warming and the increase in regional human activities, an increasing number of wetlands are being threatened by fires, which have serious effects on carbon cycling in wetlands. Although plant litter decomposition is one of the key stages of carbon cycling in wetlands, it is still unclear whether fires affect residual plant litter decomposition in burnt wetlands and whether the fire season also causes different effects. To address these knowledge gaps, a plant litter decomposition experiment was conducted during the growing season in autumn burnt, spring burnt, and unburnt sites in a Calamagrostis angustifolia wetland in the Sanjiang Plain (Northeast China). The results show that autumn burning promotes more mass loss (i.e., 15.9 ± 1.6% in autumn burnt sites and 14.8 ± 1.7% in autumn unburnt sites) and accelerates the decomposition of plant litter, whereas spring burning decreases the decomposition rates of plant litter (i.e., 15.7 ± 1.7% in spring burnt sites and 22.0 ± 2.5% in spring unburnt sites). As the decomposition time increased, the accumulation index indicated that carbon was released from plant litter to the surrounding environment accompanied by mass loss and nutrient elements accumulated in the residual plant litter. The N/P ratio of plant litter decreased from ca. 20 on day 26th to ca. 9 on day 121st, indicating that N acts as the limiting element for plant litter decomposition in C. angustifolia wetlands, and the limitation increased with increasing decomposition time. Our results also suggest that the autumn burning may promote more carbon loss and nutrient elements accumulated in plant litter in C. angustifolia wetlands than the spring burning.
Wetlands, which include both peatlands and other biomass accumulation-flooded environments, store more than 30% of the global soil carbon while covering only 8–10% of the world’s land surface (Yu et al., 2010). Due to surface plant growth and an anaerobic soil environment, wetlands with high carbon accumulation rates acted as one of the most important carbon sink ecosystems during the Holocene (Nilsson et al., 2008). With the state of global warming in recent years, carbon storage in wetlands has been threatened by environmental disturbances. Wetlands have switched from carbon sinks to carbon source ecosystems, which have serious effects on global carbon cycling (Gallego-Sala et al., 2018; Loisel et al., 2020). Additionally, most of the carbon (C) in wetlands is stored in northern regions, which are located in mid-high latitude regions and are more sensitive to climate change than other regions (Yu et al., 2010). Global warming has also markedly increased the intensity and frequency of fires over the last century and which will continue to increase in the current century (Flannigan et al., 2013; Gao et al., 2018). Recently, nearly 4% of the earth’s land surface has been burnt and this has serious consequences on wetlands and other ecosystems (Battisti et al., 2016; Just et al., 2017; van der Werf et al., 2017). Fires not only consume surface plants and emit ca. 2,200 Tg carbon to the atmosphere, but also cause serious effects on residual carbon cycling (i.e., soil carbon mineralization and residual litter decomposition) in natural ecosystems because they increase the accumulation of pyrogenic carbon and ash (Butler et al., 2017; Jones et al., 2019; Cong et al., 2020). Thus, fires have become an important disturbance factor for carbon cycling in wetland ecosystems (Flannigan et al., 2009; Turetsky et al., 2015).
For wetlands, fires increase the species diversity and abundance of plants in the next growing season and this has great potential to cause more carbon to accumulate in the soil carbon pool (Marrs et al., 2019; Gao et al., 2021). Apart from the amount of aboveground biomass, the decomposition of plant litter also acts as an important factor affecting the amount of carbon accumulated in wetlands. Most plant litter decomposes in the surface aerobic layers, and some residual plant litter acts as long-term carbon stored in the anaerobic layers (Reddy and DeLaune, 2008). Due to changes in available nutrients, microbial activities, and hydrological processes, fires also have great potential to influence the decomposition process of plant litter, which is closely related to these changing factors (Straková et al., 2012; Clarkson et al., 2013; Song et al., 2021). For example, in the Eucalyptus pilularis forest, fire frequency and intensity have serious effects on the decomposition process of plant litter and decrease the decomposition rates in long-term high-frequency burning sites (Brennan et al., 2009). Except for fire frequency and intensity, the fire season also causes serious effects on the microbial activities and soil moisture in burnt wetlands and had been proofed that leads to markedly different effects on plant growth and organic matter mineralization rates in autumn and spring burnt sites (Zhao et al., 2012; Gao et al., 2021). Although these effects of the fire season on plant litter decomposition may also differ, however, these effects remain uncertain.
Decomposition is not only important for carbon capture in wetlands but is also an important factor for nutrient element cycling in wetlands. Dynamic nutrient elements are influenced by the chemical properties of plant litter and available nutrients in the surrounding soil and water environment (Wang and Roulet, 2017; Song et al., 2018). Due to high temperatures during burning, fires promote nutrient element forms (e.g., P) in soils to change from organic to inorganic forms, which are more beneficial for plants and microbes (Wang et al., 2015). Residual fire products, such as ash and PyC, contain high amounts of available nutrients and accumulate on the surface of the burnt sites, which also increase the amount of available nutrients (Giardina et al., 2000; Pingree and DeLuca, 2018). Thus, the changes in available nutrients in burnt sites also act as major factors that influence the dynamics of nutrient elements in plant litter during decomposition. Compare to spring burning, autumn burning promotes the availability of more nutrient elements for plant growth in seasonally frozen regions, and the effects of autumn burning on plant growth are more direct than those of spring burning (Gao et al., 2021). However, it is unclear whether the burning season also has different effects on nutrient element dynamics in the decomposition process of plant litter in burnt and unburnt sites.
To address these knowledge gaps, we examined the effects of the burning season (i.e., spring burning and autumn burning) on plant litter decomposition in a typical Calamagrostis angustifolia wetland in the Sanjiang Plain (China) (Zhao et al., 2012). Based on a 121-day field decomposition experiment, the mass loss, carbon, and selected nutrient elements (i.e., N, P, and S) in residual plant litter in autumn, spring, and unburnt sites were analyzed in detail. The objective of this study was to provide insights into the impact of the fire season on plant litter mass loss during the decomposition process at burnt and unburnt sites. Second, we aimed to identify the differences in carbon loss and nutrient dynamics of plant litter during decomposition in burnt and unburnt sites. Third, the potential effects of nutrient elements on the mass loss of plant litter in the burnt and unburnt sites were also evaluated.
The climate characteristics in the Sanjiang Plain are of a cold-temperate continental monsoon, with an annual mean temperature of 1.6°C and annual mean precipitation of 600 mm (Gao et al., 2014). The burnt wetland in the Sanjiang Plain is adjacent to farmland, and nine sites within a homogenous area of C. angustifolia wetland were selected for the plant litter decomposition experiment (Figure 1). Details of the burnt experimental design and study sites are presented in our previous studies (Zhao et al., 2012; Gao et al., 2021). The plant litter for the decomposition experiment was collected in October 2007 in autumn-burnt sites and in May 2008 in spring-burnt sites before prescribed burning. Part of the plant litter was dried at 85°C for 48 h and used to analyze the original contents of water and elements. The remaining plant litter selected for plant litter decomposition experiments were cut into small pieces (ca. 10 cm), and every 10 g dry weight plant litter (calculated by water content and wet weight of fresh plant litters) was placed in a decomposition bag (Nylon, 20 cm × 20 cm, 100 mesh). For the autumn burnt and unburnt sites, the decomposition bags were placed on the surface of the soil on 20 October 2007. For the spring burnt and unburnt sites, the decomposition bags were placed on the surface of the soil on 11 May 2008. The plant litter of spring burnt and unburnt sites was collected before spring burning, and the litter was decomposed in the natural environment from autumn 2007 to spring 2008. Therefore, the decomposition times were the same as those for the decomposition bags at the autumn burnt and unburnt sites. Because all plant litter was collected from the same vegetation community, the original contents of carbon and nutrients in plant litter was similar for all sites. Considering the monthly average temperature in the Sanjiang Plain (Figure 1), the temperature from May to September is beneficial for microbial activity, and most of the mass loss occurred during this period (Fick and Hijmans, 2017). Thus, the start decomposition time in the present experiment was set on 11 May, when the plant decomposition bags were placed in the spring burnt and unburnt sites. The sampling times were June 6th (26 days), July 6th (56 days), August 10th (91 days), and September 9th (121 days). Three plant decomposition bags were collected from each site for further analysis.
Figure 1. Location of the studied sites (i.e. spring burnt sites, SB; autumn burnt sites, AB; unburnt sites, UB) in Sanjiang Plain, Northeast China (Gao et al., 2021), and the average monthly temperature and precipitation in Sanjiang Plain (Fick and Hijmans, 2017).
The total mass loss of plant litter in decomposition bags was calculated as the difference between the original dry weight and dry weight (85°C, 48 h) of residual plant litter. The carbon (C) content of the plant litter was determined using the external heating potassium dichromate oxidation method (Wu and Tao, 1993). The total nitrogen (N) content of the plant litter was measured after digestion (concentrated H2SO4 and catalytic) using a flow continuous chemistry analyzer (Cong et al., 2019). The total phosphate (P) and sulfur (S) contents of the plant litter after digestion (HNO3/HClO4/HF) were determined by atomic emission spectrometry with inductively coupled plasma (ICPS-7500) (Gao et al., 2014). The C contents in the origin plant litter was 441.5 ± 8.8 g kg–1 and the N, P, and S contents of the original plant litter were 2,818.1 ± 331.2 mg kg–1, 199.5 ± 8.5 mg kg–1, and 538.9 ± 9.0 mg kg–1, respectively.
Accumulated or released carbon and selected elements were indicated by the accumulation index (AI), which was calculated as follows:
where Mt is the dry mass weight (g) of the plant litter at sampling time t, Xt is the element content (mg kg–1) of the plant litter at sampling time t, M0 is the dry mass weight of the original plant litter, and X0 is the element content (mg kg–1) of the original plant litter. Because the decomposition starting time of plant litter in spring burnt and unburnt sites was similar to that in autumn burnt and unburnt sites, the X0 used for litter bags in spring unburnt and burnt sites was the same as the elemental contents of the original plant litter collected in autumn. The AI lower than 100% indicates the selected elements released from the plant litter to the surrounding environment, and the AI higher than 100% indicates the selected elements accumulated in the plant litter.
A two-way analysis of variance (two-way ANOVA) via SPSS 22 (SPSS, Inc.) was used to evaluate whether the different burnt sites and sampling time were associated with significant differences in mass loss, AI of carbon and nutrients, and the mass ratio of selected elements in plant litters. Sampling time (i.e., day 26th, day 56th, day 91st, and day 121st) and site types (i.e., autumn burnt, autumn unburnt, spring burnt, and spring unburnt) were the factors tested. The ANOVA results were then applied with the different treatments being grouped by Tukey’s honestly significant differences (Tukey-HSD) test, respectively. Significant differences are reported at the 0.05 probability level (i.e., P < 0.05).
The variations in litter mass loss rates and AI-C at the four sampling times are shown in Figure 2. There were significant differences in mass loss rates and AI-C at different sampling times and sites (Table 1). As the sampling time increased, the mass loss rates increased from ca. 5% on day 26th to ca. 18% on day 121st. The mass loss rates in spring unburnt sites in the four sampling times were higher than those in the other three site types. The mass loss rate in spring unburnt sites was 21.95 ± 2.54% on day 121st and markedly higher than those in the other three site types, which were around 15%. The mass loss rates in the spring and autumn burnt sites were similar and slightly higher than those in the autumn unburnt sites. The AI-C of plant litter at the four sampling sites gradually decreased from ca. 97% on day 26th to ca. 85% on day 121st. The AI-C of plant litter in autumn-burnt sites was slightly higher than that in autumn-unburnt sites. However, except on day 26th, the AI-C of plant litter in the spring burnt sites was markedly higher than that in the spring unburnt sites. After 121 days of decomposition, the AI-C in spring unburnt sites was the lowest at 81.08 ± 2.86% and the AI-C in spring burnt sites were the highest (86.35 ± 1.96%). The AI-C in the autumn burnt and unburnt sites were in the middle and slightly close to those in the spring burnt sites.
Figure 2. Mass loss (A) and the AI-C (B) in residual plant litters in autumn burnt (AB), autumn unburnt (AUB), spring burnt (SB), and spring unburnt (SUB) sites. Different lowercase letters (a-d) indicate significant differences (Tukey-HSD test) among sampling times and different uppercase letters (A-B) indicate significant differences (Tukey-HSD test) between site types.
Table 1. Two-way analysis of variance (ANOVA) of mass loss, AI of selected elements, and elements mass ratio.
There were significant differences in AI-N, AI-P, and AI-S in the different types of burnt sites, and the sampling time only caused significant differences in AI-P and AI-S. The interaction between burn site type and sampling time also had significant effects on AI-P and AI-S (Figure 3). On day 26th, the AI-N in residual litter was slightly higher than 100% in burnt sites and markedly lower than those in unburnt sites, which were approximately 120%. In the spring unburnt sites, the AI-N decreased gradually from 122.93 ± 15.82% on day 26th to 96.15 ± 14.17% on day 121st. In contrast, in other site types, the AI-N increased, and the highest value appeared on day 56th or 91st, and then decreased gradually. The AI-P in residual plant litter decreased before day 56th, and the lowest AI-P was 63.52 ± 3.95% in spring unburnt sites on day 56th. On day 91st, the AI-P in autumn unburnt sites and spring burnt sites was higher than 200% and markedly higher than those in the other two site types. On day 121st, the AI-P gradually decreased and was similar in all four site types, ranging from 137.18 ± 8.39 to 166.76 ± 29.35%. Similar to the variation of AI-P, the AI-S in residual plant litter also decreased before day 56th, and then increased on days 91st and 121st. The AI-S in the spring burnt and unburnt sites was approximately 120% on day 56th and significantly higher than those in the autumn burnt and unburnt sites, which were around 73%.
Figure 3. The AI-N (A), AI-P (B), and AI-S (C) in residual plant litters in autumn burnt (AB), autumn unburnt (AUB), spring burnt (SB), and spring unburnt (SUB) sites. Different lowercase letters (a-c) indicate significant differences (Tukey-HSD test) among sampling times and different uppercase letters (A-B) indicate significant differences (Tukey-HSD test) between site types.
The mass ratios of C/N, C/P, C/S, and N/P were selected as typical element ratios in this study (Figure 4), and the effects of sampling time and site type on these ratios were significant, with the exception of the C/N ratio. Only site type had a significant effect on the C/N ratio, and there was no significant effect of sampling time on C/N ratios. The C/N ratio ranged from 103.6 ± 14.8 to 156.1 ± 7.0%. Both the highest and lowest values occurred on day 91st at the autumn burnt site and autumn unburnt site, respectively. There was no obvious change in the C/N ratio during the 121-day decomposition, and the C/N ratio fluctuated overall. Unlike the C/N ratio, the C/P ratio in all four site types decreased significantly from day 56th to day 91st. The C/P ratios on day 91st and 121st were approximately 1,000 and significantly lower than those on day 26th and 56th, which were approximately 3,000. Similar to the C/P ratios, the N/P ratio decreased from approximately 20 on day 56th to ca. 9 on day 121st. After 121 days of decomposition, the N/P ratios in all four site types were similar and ranged between 9.56 ± 1.33 and 10.00 ± 1.95. The range of the C/S ratio during the 121-day decomposition was between 472.3 ± 92.1 and 1,215.9 ± 50.6, which was slightly weaker than the changes in the C/P and N/P ratios. The C/S ratio decreased in autumn burnt and unburnt sites before day 91st, and then increased on day 121st. In the spring burnt and unburnt sites, the C/S ratio increased from day 26th to day 56th, and then decreased on day 91st. The lowest C/S ratio in the spring unburnt and burnt sites appeared on day 91st, and increased by a little on day 121st.
Figure 4. The mass ratio of C/N (A), C/P (B), N/P (C), and C/S (D) in residual plant litters in autumn burnt (AB), autumn unburnt (AUB), spring burnt (SB), and spring unburnt (SUB) sites. Different lowercase letters (a-d) indicate significant differences (Tukey-HSD test) among sampling times and different uppercase letters (A-C) indicate significant differences (Tukey-HSD test) between site types.
Comparing the mass loss rates in autumn burnt and unburnt sites, the mass loss rates in autumn burnt sites were higher than those in unburnt sites. However, the mass loss rates in the spring burnt sites were lower than those in the unburnt sites (Figure 2). These results showed that autumn burning increased plant litter decomposition rates and that spring burning decreased plant litter decomposition rates. Plant litter decomposition rates are mainly influenced by microbial activities, which are controlled by surrounding environmental factors (e.g., temperature, water table, available nutrients, aboveground plant community, and pH) (Ward et al., 2015; Wang et al., 2019; Yu et al., 2020). Burning also acts as an important factor that not only promotes the release of nutrients from the residual plant to the environment and increases nutrient availability, but can also promote microbial activities (Medvedeff et al., 2015; Wang et al., 2015; Singh et al., 2017). In our experimental sites, there was more DOC and MBC in burnt sites than in unburnt sites during the entire growing season from May to September (Zhao et al., 2012). The greater nutrient availability and greater activity of microbial metabolism in the surface soils not only promoted more soil carbon decomposition and aboveground plant growth, but also caused the decomposition rates of plant litter in autumn burnt sites to be higher than those in autumn unburnt sites. However, because the microbial activities in burnt sites decreased after burning and required several months for recovery (Medvedeff et al., 2013), the short time interval between burning and plant litter decomposition in spring burnt sites may lead to microbial activities not recovering. The weak activity of microbial metabolism in spring burnt sites was speculated to be the major reason why the plant litter decomposition rates in spring burnt sites were lower than those in the spring unburnt sites.
In addition to the direct effects of burning on microbial activity, the effects of burning on plant growth and soil moisture may also influence the decomposition rates of plant litter. Our previous study found that stem density in spring burnt sites was markedly higher than that in autumn burnt sites and unburnt sites, and the aboveground biomass and biomass per plant in autumn burnt sites were higher than those in spring burnt and unburnt sites (Gao et al., 2021). The temperature of surface soils is mainly influenced by micro-geomorphology and surface plants (Song et al., 2013; Goncharova et al., 2019). Because the decomposition process of plant litter mainly occurs during the growing season in the Sanjiang Plain, surface plants also directly influence the temperature of surface soils. The high density of aboveground vegetation communities in spring burnt sites decreases the solar radiation and surface soil temperature during the daytime, which is speculated to be another factor that decreases the decomposition rates of plant litter. The increase in aboveground plants also increases the underground biomass, which is the major oxygen source in rhizospheric soil under the water table and may increase the oxygen concentrations in the water and surface soil layers (Reddy and DeLaune, 2008). The higher aboveground biomass in autumn-burnt sites results in increased oxygen availability under the water table for microbial metabolism and accelerates the decomposition rates of plant litter. Except for the increase in aboveground biomass, burning also decreases the water-retention capacity of surface soils (Thompson and Waddington, 2013). This leads to lower soil moisture in the burnt sites, and higher available oxygen than that in the unburnt sites. Thus, burning not only alters the microbial activities directly, but also changes the aboveground plant growth and water retention capacity, which causes serious effects on the decomposition rates of plant litter indirectly. The enhanced microbial activities and high aboveground biomass resulted in greater oxygen availability in rhizospheric soil, which were speculated to be two major factors that increased the decomposition rates of plant litter in autumn-burnt sites.
Carbon is the most important element in residual plant litter, which contains a carbon content higher than 40%. The mass loss of plant litter leads to carbon release to the surrounding environment. The AI-C in residual plant litter decreased gradually with an increase in mass loss rates during the 121-day decomposition experiment, which was similar to the results of previous studies (Brennan et al., 2009; Gorecki et al., 2021). Due to the high mass loss of plant litter in autumn burnt sites, the AI-C in autumn burnt sites was also lower than that in autumn unburnt sites. Conversely trends were observed in the spring burnt/unburnt sites. Our results showed that autumn burning promoted the release of more carbon from residual plant litter to the surrounding environment. Additionally, autumn burning also promotes the aboveground biomass and the mineralization rates of surface soils (Zhao et al., 2012; Gao et al., 2021). Thus, autumn burning increased all links of carbon cycling in the wetlands and accelerated carbon turnover rates. However, the effects of spring burning on carbon turnover in seasonally frozen wetlands were more complex than those of autumn burning, and it not only decreased the carbon loss rates in residual plant litter, but also caused no significant increase in aboveground biomass compared to unburnt sites (Gao et al., 2021). The potential reasons for this were similar to the reasons for the limitation of mass loss in the spring burnt sites, as previously discussed, and unrecovered microbial activities in the spring unburnt sites were speculated to be the major reason. Thus, similar to the results and potential reasons of mass loss in different sites, the autumn burnt sites were more beneficial for microbial activities and accelerated the release of carbon from plant litter to the surrounding environment.
The dynamic changes in the selected nutrient elements in the residual plant litter are shown in Figure 3. Unlike the changes in AI-C, the AI of nutrient elements gradually increased with increasing decomposition time, and the highest AI of nutrient elements (i.e., AI-P) was higher than 300%, which means that the nutrient elements accumulated in the plant litter during the 121-day decomposition. Nutrients were also released to the surrounding environment during the mass loss of plant litter, which is similar to that seen for carbon loss in plant litter. In contrast, microbes can absorb nutrient elements from the surrounding environment (e.g., water and soils) for their metabolism and accumulate nutrient elements in the plant litter (Cui et al., 2021; Wang et al., 2022). More nutrients absorbed than nutrient loss in microbes accompany the decomposition of plant litter, which causes the AI of all selected nutrient elements (i.e., N, P, and S) to increase gradually during the 121-day decomposition period (Figure 3). Compared to that of unburnt sites, the AI of nutrient elements in residual plant litters in autumn burnt sites were lower than those in autumn unburnt sites. The AI of nutrient elements in residual plant litter in the spring burnt sites was higher than that in the spring unburnt sites. The AI of nutrients is not only controlled by microbial activity but is also influenced by the amount of available nutrients in the surrounding environment (Aerts and de Caluwe, 1997; Song et al., 2021). High mass loss rates of plant litter in autumn burnt sites and spring unburnt sites were also found, indicating that the activity of microbial metabolism in these two site types was higher than that in other sites (Figure 2). The nutrients in the decomposed part of plant litter are more easily released into the surrounding environment, which was speculated to be the reason for the slightly lower AI of nutrients in high mass loss rates of plant litter than that in low mass loss rates of plant litter.
There are also several differences in the changing trends of the AI for different nutrient elements. For example, AI-N increased gradually before day 91st, and the average values of AI-N during this period were higher than 100%, which means that the N accumulation rates were higher than the loss rates at the beginning of decomposition. The AI-P decreased gradually, and the average values were lower than 100% before day 56th, which means that P was released from the plant litter to the surrounding environment before this day. Although N and P are both important nutrients for microbial metabolism, there are marked differences in biogeochemical cycling in natural ecosystems, especially in anaerobic environments. For example, microbes can fix N from the atmosphere and convert organic N to inorganic N in both aerobic and anaerobic environments (Reddy and DeLaune, 2008). The natural sources of inorganic P are mainly dust and rock weathering, and organic P from the decomposition of plant litter and organic matter. The transfer of organic to inorganic P through microbial metabolism occurs only in aerobic layers (Reddy and DeLaune, 2008). Differences in the biogeochemical processes of N and P lead to differences in the availability of microbial activities (Zhao et al., 2022). In particular, for plant litter decomposition experiment in the present study, which are located under the water table, the flooding environment causes difficulty in the conversion of organic P in surface soils to inorganic P; microbes cannot absorb organic P directly. Thus, the AI-P in the residual plant litter before day 56th was lower than 100%, and the P in the plant litter released to the surrounding environment was accompanied by mass loss. However, because microbes absorb N in an anaerobic environment more easily than P, the AI-N in the residual litter at the beginning of decomposition was higher than 100%. With increasing decomposition time, the mass loss rate gradually decreased, and the microbes adapted to the environment and gradually absorbed P from the surrounding environment. The AI-P markedly increased on days 91st and 121st, and the increasing trend was more obvious than that for AI-N. Previous studies also found that plant litter could accumulate P, and the AI-P was higher than 800% (Qualls and Richardson, 2000). The potential reason for this result was speculated to be that the N in plant litter may be released into the atmosphere as N2 or N2O through the denitrification process, and the mobility of N is markedly higher than that of P (e Silva et al., 2007; Rubol et al., 2012). The loss of N in residual plant litter was easier than the loss of P, and there was no marked increase in N with increasing decomposition time. The biogeochemical cycling of S in an anaerobic environment was similar to that of P, and the transfer of organic S to inorganic S only occurred in the aerobic environment (Reddy and DeLaune, 2008). The AI-S in the residual plant litter before day 56th was lower than 100% in total, which means that the S released from plant litter to the surrounding environment was accompanied by mass loss. S may also be emitted to the atmosphere through H2S under the anaerobic environment, which is similar to the loss process of N. Thus, the biogeochemical character of S led to a marked increase in the AI-S in residual plant litter after day 56th, and the increasing trend was weaker than that of AI-P.
Shortly, the P and S were mainly released from plant litter to the surrounding environment before day 56th, and then accumulated in plant litter through the microbes absorbed from the surrounding environment. Because S could be released into the atmosphere through H2S under anaerobic conditions, the increasing trend of AI-P in the residual plant litter was more marked than that of AI-S. Compared to P and S, N was more easily utilized by microbes in anaerobic environments, and more N accumulated in the residual plant litter during the 121-day decomposition time in the present study.
The element ratios are widely used to evaluate the decomposition and nutrient changes of residual plant litter, and we selected C/N, C/P, N/P, and C/S to reflect the decomposition degree, nutrient limit, and acid stress, respectively (Güsewell and Verhoeven, 2006; Wang et al., 2019). The C/N ratio is a good indicator of the degree of decomposition because it reflects the ratio of carbohydrate to protein, and N is more stable than C during litter decomposition (Broder et al., 2012; Sun et al., 2012). As the C/N ratio decreased, the residual carbon decreased and the decomposition degree of plant litter increased. In the present study, the C/N ratio in the burnt sites was higher than that in the unburnt sites. The C/N ratio in the autumn burnt sites was higher than that in the spring burnt sites before day 91st, and then lower than that in the spring burnt sites on day 121st (Figure 4). Considering the variation trend of AI-N and AI-C, changes in the C/N ratio were mainly influenced by the accumulation of N, especially for the autumn burnt sites. The low N accumulation rates of residual plant litter in autumn-burnt sites was the major reason why the C/N ratio was higher than that in unburnt sites. Because N also accumulates through microbial activity at the initial stage of plant litter decomposition, the C/N ratio may not be a good indicator of carbon loss in plant litter.
In addition to the C/N ratio, the C/P ratio can be used to reflect the P available during the plant litter decomposition process. The C/P ratio of plant litter before day 56th was markedly higher than that after day 91st, and the C/P ratio of plant litter in the autumn burnt sites was markedly higher than that in the autumn unburnt sites—especially on days 56th and 91st. Due to the small amount of P absorbed by microbes in the initial stage, the P in the plant litter was released to the surrounding environment, accompanied by mass loss. The low P content in the residual plant litter caused the C/P ratios to be higher than 3,000. Similar results were also found in a previous study in which litter decomposition was strongly limited by a high C/P ratio in the initial stage (Aerts and de Caluwe, 1997). After day 56th, the increase in AI-P in residual plant litter indicated the accumulation of P from the surrounding environment and a significant decrease in the C/P ratio. Compared to unburnt sites, autumn burning significantly increased the C/P ratio, which also indicated that the degree of P loss in the initial stage of litter decomposition was higher than that in unburnt sites. The potential reason for this is that microbial metabolism in autumn-burnt sites was more active than that in unburnt sites, and more C and P loss occur during decomposition. Compared to the loss of C, the loss of P in autumn burnt sites was more serious than that in unburnt sites, and thus increased the C/P ratios in total.
In addition to the amount of N and P, the N/P ratio is also important for the decomposition of plant litter. The critical N/P ratio (threshold between N and P limitation) was 25 for graminoid leaf litter (Güsewell and Verhoeven, 2006). In the present study, the N/P ratio was lower than 20, which indicates that the decomposition environment is the N limitation of litter decomposition, and bacteria were most abundant on cellulose (Güsewell and Gessner, 2009). Before day 56th, the N/P ratios ranged between 16.21 ± 2.33 and 23.32 ± 5.06, and the N/P ratios in unburnt sites were higher than those in autumn burnt sites, while they were lower than those in spring burnt sites. As the decomposition time increased, more P accumulated in the plant litter, and the N/P ratio decreased significantly and was lower than 10 (Figure 4D). The present results show that the plant litter decomposition process in the studied C. angustifolia litter was N limitation, and spring burning increased the N/P ratio and slightly decreased the N limitation effects on litter decomposition. Although P was lost at the initial stage of plant litter decomposition, the N/P ratio was still lower than 25, indicating that the residual P in plant litter was sufficient for microbial metabolism. As the AI-P increased significantly on day 91st, the N/P ratio significantly decreased, which means that the N limitation environment for litter decomposition was clearer and the changes in N content in residual plant litter were the major factors that influenced the decomposition rates of plant litter.
The release of S from plant litter to the surrounding environment may decrease the pH of the water environment and cause S stress on microbial activities for plant litter decomposition (Wang et al., 2019). As the decomposition time increased, the C/S ratio decreased, indicating that the loss rates of S were lower than the C loss rates. More S accumulated in plant litter and the marked decrease in the C/S ratio indicated that S stress on microbial metabolism increased and may become a stress factor that decreases the decomposition rates of plant litter. After 121 days of decomposition, the C/S ratios in plant litter in autumn burnt sites were higher than those in autumn unburnt sites; opposite trends were found in spring burnt sites. Autumn burning increased the C/S ratio in residual plant litter, which decreased the S stress for plant litter decomposition, and spring burning increased the S stress for plant litter decomposition. The difference of S stress may also explain why more plant litter was decomposed in autumn burnt sites than those in autumn unburnt sites.
The overall trends of selected element ratios in residual plant litter in burnt and unburnt sites were similar, and the ratio of C/P and C/S decreased significantly on day 91st. The N/P ratio during the entire decomposition process was lower than 25, indicating that the decomposition environment was limited by N. The accumulation of P after day 91st increased the importance of the N content in plant litter decomposition. Similar to the previous discussion, the nutrient element ratios also showed that autumn burning promoted a nutrient environment more suitable for plant litter decomposition, while spring burning slightly decreased the nutrient availability for plant litter decomposition.
Based on a 121-day plant litter decomposition experiment in burnt and unburnt sites during the growing season, our results showed that the decomposition rates of plant litter in burnt and unburnt sites were markedly different, and that the burning season acts as a major factor that influences the decomposition process of residual plant litter. As the decomposition time increased, the carbon released to the surrounding environment was accompanied by mass loss, and nutrient elements accumulated in the residual plant litter. Autumn burning promoted carbon and mass loss from plant litter, whereas the mass loss rates of plant litter in spring burnt sites were lower than those in spring unburnt sites. P and S were released from plant litter to the surrounding environment before day 56th, and then accumulated more markedly in the plant litter. Unlike P and S, N accumulated in the residual plant litter during the 121-day decomposition period. The N/P ratio of plant litter in the spring burnt sites was significantly higher than that in other site types, and significantly decreased from ca. 20 on day 56th to ca. 9 on day 121st. A low N/P ratio indicates that N is the limiting nutrient element for plant litter decomposition in C. angustifolia wetlands, and the limitation of N became more serious as the decomposition time increased. Our results also suggest that the autumn burning accelerate the plant litter decomposed and nutrient elements accumulated in the residual plant litter, which may more benefit of elements cycling in C. angustifolia wetlands than the spring burning.
The raw data supporting the conclusions of this article will be made available by the authors, without undue reservation.
CG, GW, and HZ designed the research and provided the funding. CG, JC, and HZ ran the experiment and data analysis. CG and HZ wrote the manuscript with input from GW, JC, and DH. All authors contributed to the article and approved the submitted version.
Financial support was provided by the National Natural Science Foundation of China (Nos. 42171103 and 42101108), the Jilin Association for Science and Technology (QT202126), the Young Scientist Group Project of Northeast Institute of Geography and Agroecology, Chinese Academy of Sciences (2022QNXZ01), and the Youth Innovation Promotion Association CAS (No. 2020235).
The authors declare that the research was conducted in the absence of any commercial or financial relationships that could be construed as a potential conflict of interest.
All claims expressed in this article are solely those of the authors and do not necessarily represent those of their affiliated organizations, or those of the publisher, the editors and the reviewers. Any product that may be evaluated in this article, or claim that may be made by its manufacturer, is not guaranteed or endorsed by the publisher.
We thank Yuxia Zhang, Haiyang Zhao, and Shaoqing Zhang for sample analyses, and Yang Wang for help with the experimental burning. Assistance of the Sanjiang Mire Wetland Experimental Station, Chinese Academy of Sciences is also gratefully acknowledged.
Aerts, R., and de Caluwe, H. (1997). Nutritional and plant-mediated controls on leaf litter decomposition of Carex species. Ecology 78, 244–260
Battisti, C., Poeta, G., and Fanelli, G. (2016). An Introduction to Disturbance Ecology. Berlin: Springer International Publishing.
Brennan, K. E., Christie, F. J., and York, A. (2009). Global climate change and litter decomposition: more frequent fire slows decomposition and increases the functional importance of invertebrates. Glob. Change Biol. 15, 2958–2971. doi: 10.1111/j.1365-2486.2009.02011.x
Broder, T., Blodau, C., Biester, H., and Knorr, K. H. (2012). Peat decomposition records in three pristine ombrotrophic bogs in southern Patagonia. Biogeosciences 9, 1479–1491. doi: 10.5194/bg-9-1479-2012
Butler, O. M., Lewis, T., and Chen, C. (2017). Fire alters soil labile stoichiometry and litter nutrients in Australian eucalypt forests. Int. J. Wildland Fire 26, 783–788. doi: 10.1071/WF17072
Clarkson, B. R., Moore, T. R., Fitzgerald, N. B., Thornburrow, D., Watts, C. H., and Miller, S. (2013). Water Table Regime Regulates Litter Decomposition in Restiad Peatlands. N. Zealand. Ecosyst. 17, 317–326. doi: 10.1007/s10021-013-9726-4
Cong, J., Gao, C., Han, D., Li, Y., and Wang, G. (2020). Stability of the permafrost peatlands carbon pool under climate change and wildfires during the last 150 years in the northern Great Khingan Mountains, China. Sci. Total Environ. 712:136476. doi: 10.1016/j.scitotenv.2019.136476
Cong, J., Gao, C., Han, D., Liu, H., and Wang, G. (2019). History metal (Pb, Zn, and Cu) deposition and Pb isotope variability in multiple peatland sites in the northern Great Hinggan Mountains, Northeast China. Environ. Sci. Pollut. R. 26(21), 21784-21796. doi: 10.1007/s11356-019-04432-7
Cui, W., Mao, Y., Tian, K., and Wang, H. (2021). A Comparative Study of Manipulative and Natural Temperature Increases in Controlling Wetland Plant Litter Decomposition. Wetlands 41:48. doi: 10.1007/s13157-021-01445-2
e Silva, C. A. R., Oliveira, S. R., Rêgo, R. D., and Mozeto, A. A. (2007). Dynamics of phosphorus and nitrogen through litter fall and decomposition in a tropical mangrove forest. Mar. Environ. Res. 64, 524–534. doi: 10.1016/j.marenvres.2007.04.007
Fick, S. E., and Hijmans, R. J. (2017). WorldClim 2: new 1-km spatial resolution climate surfaces for global land areas. Int. J. Climatol. 37, 4302–4315. doi: 10.1002/joc.5086
Flannigan, M., Cantin, A. S., De Groot, W. J., Wotton, M., Newbery, A., and Gowman, L. M. (2013). Global wildland fire season severity in the 21st century. Forest Ecol. Manag. 294, 54–61. doi: 10.1016/j.foreco.2012.10.022
Flannigan, M. D., Krawchuk, M. A., de Groot, W. J., Wotton, B. M., and Gowman, L. M. (2009). Implications of changing climate for global wildland fire. Int. J. Wildland Fire 18, 483–507. doi: 10.1071/WF08187
Gallego-Sala, A. V., Charman, D. J., Brewer, S., Page, S. E., Prentice, I. C., Friedlingstein, P., et al. (2018). Latitudinal limits to the predicted increase of the peatland carbon sink with warming. Nat. Clim. Change 8, 907–913. doi: 10.1038/s41558-018-0271-1
Gao, C., Bao, K., Lin, Q., Zhao, H., Zhang, Z., Xing, W., et al. (2014). Characterizing trace and major elemental distribution in late Holocene in Sanjiang Plain, Northeast China: Paleoenvironmental implications. Quatern. Int. 349, 376–383. doi: 10.1016/j.quaint.2014.01.022
Gao, C., He, J., Cong, J., Zhang, S., and Wang, G. (2018). Impact of forest fires generated black carbon deposition fluxes in Great Hinggan Mountains (China). Land Degrad. Dev. 29, 2073–2081. doi: 10.1002/ldr.2837
Gao, C., Wang, G., Santin, C., Doerr, S. H., Cong, J., and Zhao, H. (2021). Response of Calamagrostis angustifolia to burn frequency and seasonality in the Sanjiang Plain wetlands (Northeast China). J. Environ. Manage. 300:113759. doi: 10.1016/j.jenvman.2021.113759
Giardina, C. P., Sanford, R. L., Døckersmith, I. C., and Jaramillo, V. J. (2000). The effects of slash burning on ecosystem nutrients during the land preparation phase of shifting cultivation. Plant Soil 220, 247–260. doi: 10.1023/A:1004741125636
Goncharova, O. Y., Matyshak, G. V., Epstein, H. E., Sefilian, A. R., and Bobrik, A. A. (2019). Influence of snow cover on soil temperatures: Meso- and micro-scale topographic effects (a case study from the northern West Siberia discontinuous permafrost zone). Catena 183:104224. doi: 10.1016/j.catena.2019.104224
Gorecki, K., Rastogi, A., Strozecki, M., Gabka, M., Lamentowicz, M., Lucow, D., et al. (2021). Water table depth, experimental warming, and reduced precipitation impact on litter decomposition in a temperate Sphagnum-peatland. Sci. Total Environ. 771:145452. doi: 10.1016/j.scitotenv.2021.145452
Güsewell, S., and Gessner, M. O. (2009). N : P ratios influence litter decomposition and colonization by fungi and bacteria in microcosms. Funct. Ecol. 23, 211–219. doi: 10.1111/j.1365-2435.2008.01478.x
Güsewell, S., and Verhoeven, J. T. A. (2006). Litter N:P ratios indicate whether N or P limits the decomposability of graminoid leaf litter. Plant Soil 287, 131–143. doi: 10.1007/s11104-006-9050-2
Jones, M. W., Santín, C., van der Werf, G. R., and Doerr, S. H. (2019). Global fire emissions buffered by the production of pyrogenic carbon. Nat. Geosci. 12, 742–747. doi: 10.1038/s41561-019-0403-x
Just, M. G., Hohmann, M. G., and Hoffmann, W. A. (2017). Invasibility of a fire-maintained savanna–wetland gradient by non-native, woody plant species. Forest Ecol. Manag. 405, 229–237. doi: 10.1016/j.foreco.2017.09.052
Loisel, J., Gallego-Sala, A. V., Amesbury, M. J., Magnan, G., Anshari, G., Beilman, D. W., et al. (2020). Expert assessment of future vulnerability of the global peatland carbon sink. Nat. Clim. Change 70–77. doi: 10.1038/s41558-020-00944-0
Marrs, R., Marsland, E.-L., Lingard, R., Appleby, P., Piliposyan, G., Rose, R., et al. (2019). Experimental evidence for sustained carbon sequestration in fire-managed, peat moorlands. Nat. Geosci. 12, 108–112. doi: 10.1038/s41561-018-0266-6
Medvedeff, C. A., Inglett, K. S., and Inglett, P. W. (2015). Can Fire Residues (Ash and Char) Affect Microbial Decomposition in Wetland Soils? Wetlands 35, 1165–1173. doi: 10.1007/s13157-015-0703-x
Medvedeff, C. A., Inglett, K. S., Kobziar, L. N., and Inglett, P. W. (2013). Impacts of Fire on Microbial Carbon Cycling in Subtropical Wetlands. Fire Ecol. 9, 21–37. doi: 10.4996/fireecology.0901021
Nilsson, M., Sagerfors, J., Buffam, I., Laudon, H., Eriksson, T., Grelle, A., et al. (2008). Contemporary carbon accumulation in a boreal oligotrophic minerogenic mire - a significant sink after accounting for all C-fluxes. Global Change Biol. 14, 2317–2332. doi: 10.1111/j.1365-2486.2008.01654.x
Pingree, M. R. A., and DeLuca, T. H. (2018). The influence of fire history on soil nutrients and vegetation cover in mixed-severity fire regime forests of the eastern Olympic Peninsula. Washington, USA. Forest Ecol. Manag. 422, 95–107. doi: 10.1016/j.foreco.2018.03.037
Qualls, R. G., and Richardson, C. J. (2000). Phosphorus enrichment affects litter decomposition, immobilization, and soil microbial phosphorus in wetland mesocosms. Soil Sci. Soc. Am. J. 64, 799–808. doi: 10.2136/sssaj2000.642799x
Reddy, K. R., and DeLaune, R. D. (2008). Biogeochemistry of Wetlands: Science and Applications. Boca Raton: CRC press.
Rubol, S., Silver, W. L., and Bellin, A. (2012). Hydrologic control on redox and nitrogen dynamics in a peatland soil. Sci. Total Environ. 432, 37–46. doi: 10.1016/j.scitotenv.2012.05.073
Singh, A. K., Kushwaha, M., Rai, A., and Singh, N. (2017). Changes in soil microbial response across year following a wildfire in tropical dry forest. Forest Ecol. Manag. 391, 458–468. doi: 10.1016/j.foreco.2017.02.042
Song, Y., Song, C., Ren, J., Tan, W., Jin, S., and Jiang, L. (2018). Influence of nitrogen additions on litter decomposition, nutrient dynamics, and enzymatic activity of two plant species in a peatland in Northeast China. Sci. Total Environ. 625, 640–646. doi: 10.1016/j.scitotenv.2017.12.311
Song, Y., Zhou, D., Zhang, H., Li, G., Jin, Y., and Li, Q. (2013). Effects of vegetation height and density on soil temperature variations. Chin. Sci. Bull. 58, 907–912. doi: 10.1007/s11434-012-5596-y
Song, Y.-B., Zhou, M.-Y., Qin, Y.-L., Cornelissen, J. H. C., and Dong, M. (2021). Nutrient effects on aquatic litter decomposition of free-floating plants are species dependent. Glob. Ecol. Conserv. 30:e01748. doi: 10.1016/j.gecco.2021.e01748
Straková, P., Penttilä, T., Laine, J., and Laiho, R. (2012). Disentangling direct and indirect effects of water table drawdown on above- and belowground plant litter decomposition: consequences for accumulation of organic matter in boreal peatlands. Glob. Change Biol. 18, 322–335. doi: 10.1111/j.1365-2486.2011.02503.x
Sun, Z., Mou, X., and Liu, J. S. (2012). Effects of flooding regimes on the decomposition and nutrient dynamics of Calamagrostis angustifolia litter in the Sanjiang Plain of China. Environ. Earth Sci. 66, 2235–2246. doi: 10.1007/s12665-011-1444-7
Thompson, D. K., and Waddington, J. M. (2013). Peat properties and water retention in boreal forested peatlands subject to wildfire. Water Resour. Res. 49, 3651–3658. doi: 10.1002/wrcr.20278
Turetsky, M. R., Benscoter, B., Page, S., Rein, G., van der Werf, G. R., and Watts, A. (2015). Global vulnerability of peatlands to fire and carbon loss. Nat. Geosci. 8, 11–14. doi: 10.1038/ngeo2325
van der Werf, G. R., Randerson, J. T., Giglio, L., van Leeuwen, T. T., Chen, Y., Rogers, B. M., et al. (2017). Global fire emissions estimates during 1997–2016. Earth Syst. Sci. Data 9, 697–720. doi: 10.5194/essd-9-697-2017
Wang, G., Yu, X., Bao, K., Xing, W., Gao, C., Lin, Q., et al. (2015). Effect of fire on phosphorus forms in Sphagnum moss and peat soils of ombrotrophic bogs. Chemosphere 119, 1329–1334. doi: 10.1016/j.chemosphere.2014.01.084
Wang, J., Ge, Y., Cornelissen, J. H. C., Wang, X. Y., Gao, S., Bai, Y., et al. (2022). Litter nitrogen concentration changes mediate effects of drought and plant species richness on litter decomposition. Oecologia 198, 507–518. doi: 10.1007/s00442-022-05105-y
Wang, Q., Kwak, J. H., Choi, W. J., and Chang, S. X. (2019). Long-term N and S addition and changed litter chemistry do not affect trembling aspen leaf litter decomposition, elemental composition and enzyme activity in a boreal forest. Environ. Pollut. 250, 143–154. doi: 10.1016/j.envpol.2019.04.007
Wang, Z., and Roulet, N. (2017). Comparison of plant litter and peat decomposition changes with permafrost thaw in a subarctic peatland. Plant Soil 417, 197–216. doi: 10.1007/s11104-017-3252-7
Ward, S. E., Orwin, K. H., Ostle, N. J., Briones, M. J., Thomson, B. C., Griffiths, R. I., et al. (2015). Vegetation exerts a greater control on litter decomposition than climate warming in peatlands. Ecology 96, 113–123. doi: 10.1890/14-0292.1
Wu, L., and Tao, Q. (1993). The improved method on quantify organic carbon in plant. Chin. J. Soil Sci. 24, 286–287. doi: 10.19336/j.cnki.trtb.1993.06.018
Yu, X., Ding, S., Lin, Q., Wang, G., Wang, C., Zheng, S., et al. (2020). Wetland plant litter decomposition occurring during the freeze season under disparate flooded conditions. Sci. Total Environ. 706:136091. doi: 10.1016/j.scitotenv.2019.136091
Yu, Z., Loisel, J., Brosseau, D. P., Beilman, D. W., and Hunt, S. J. (2010). Global peatland dynamics since the Last Glacial Maximum. Geophys. Res. Lett. 37, 1–5. doi: 10.1029/2010gl043584
Zhao, H., Tong, D. Q., Lin, Q., Lu, X., and Wang, G. (2012). Effect of fires on soil organic carbon pool and mineralization in a Northeastern China wetland. Geoderma 18, 532–539. doi: 10.1016/j.geoderma.2012.05.013
Keywords: wetland, plant litter, burning, litter decomposition, carbon
Citation: Gao C, Wang G, Cong J, Han D and Zhao H (2022) Burning alters the decomposition of residual plant litters in Calamagrostis angustifolia wetlands in the Sanjiang Plain (Northeast China). Front. Ecol. Evol. 10:953349. doi: 10.3389/fevo.2022.953349
Received: 26 May 2022; Accepted: 28 June 2022;
Published: 14 July 2022.
Edited by:
Arnaldo Marín, University of Murcia, SpainReviewed by:
Junhong Bai, Beijing Normal University, ChinaCopyright © 2022 Gao, Wang, Cong, Han and Zhao. This is an open-access article distributed under the terms of the Creative Commons Attribution License (CC BY). The use, distribution or reproduction in other forums is permitted, provided the original author(s) and the copyright owner(s) are credited and that the original publication in this journal is cited, in accordance with accepted academic practice. No use, distribution or reproduction is permitted which does not comply with these terms.
*Correspondence: Hongmei Zhao, emhhb2hvbmdtZWlAaWdhLmFjLmNu
Disclaimer: All claims expressed in this article are solely those of the authors and do not necessarily represent those of their affiliated organizations, or those of the publisher, the editors and the reviewers. Any product that may be evaluated in this article or claim that may be made by its manufacturer is not guaranteed or endorsed by the publisher.
Research integrity at Frontiers
Learn more about the work of our research integrity team to safeguard the quality of each article we publish.