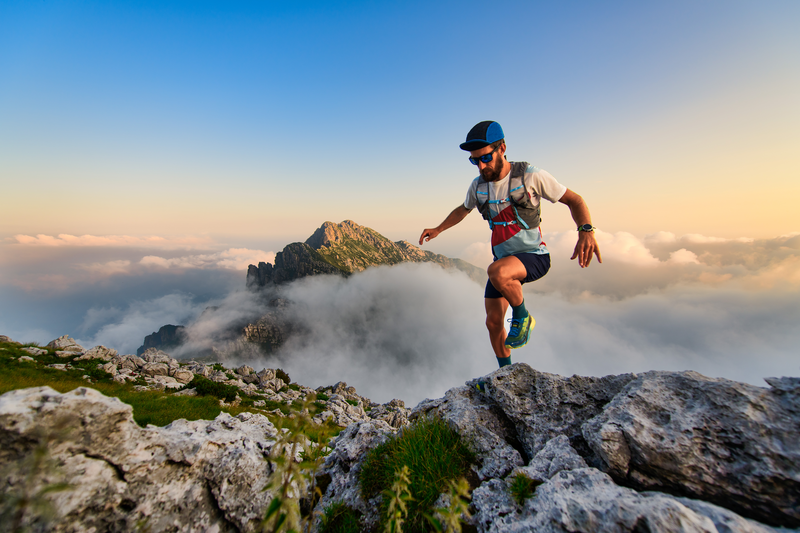
94% of researchers rate our articles as excellent or good
Learn more about the work of our research integrity team to safeguard the quality of each article we publish.
Find out more
ORIGINAL RESEARCH article
Front. Ecol. Evol. , 12 October 2022
Sec. Behavioral and Evolutionary Ecology
Volume 10 - 2022 | https://doi.org/10.3389/fevo.2022.951967
Many prey species possess eyespots: paired markings that often consist of two or more concentric circles. Predators are wary of such prey because eyespots are conspicuous and/or mistaken for vertebrate eyes. Here we used naïve domestic chicks as predators of artificial moth-like prey to test the hypothesis that both eyespots configuration and predator approach direction affect the antipredator efficacy of eyespots. We found that when chicks approached prey straight on, eyespots configuration did not influence attack latency. Chicks that approached from either the left or the right, were slower to attack prey in which the central circle of the eyespot was centrally placed or shifted in the direction of the chick’s approach, compared to prey in which the central circle had been shifted away from the direction of approach. These findings suggest that eyespots composed of concentric circles may protect prey against predators approaching from a wider range of directions than eyespots composed of eccentric circles. They are also consistent with the idea that eyespots are mistaken for eyes, and are perceived to pose a lesser risk when their “gaze” is averted from the approaching predator.
Many prey species possess markings that subjectively appear to resemble vertebrate eyes (Janzen et al., 2010). These are known as eyespots and are conspicuous circular or quasi-circular markings (Mukherjee and Kodandaramaiah, 2015). Whilst in some species eyespots appear to play a role in mate choice (Robertson and Monteiro, 2005; Huq et al., 2019), one of their key functions is to protect prey from predators (Blest, 1957; Stevens, 2005; Kjernsmo and Merilaita, 2013; Prudic et al., 2015). They are among the most studied wing color pattern elements in lepidoptera (Stevens, 2005; Kodandaramaiah, 2011), and their antipredator benefits have been predicted or established in various taxa (Stevens, 2005) including Lepidoptera (Lyytinen et al., 2004; Vallin et al., 2005, 2011; Wiklund, 2005; Olofsson et al., 2010, 2012; Merilaita et al., 2011; Ho et al., 2016; Halali et al., 2019; Chan et al., 2021), Orthoptera (Steiner, 1981; Castner, 1995), Coleoptera (Buschman, 1988), and fishes (Altbäcker and Csányi, 1990; Meadows, 1993; Gagliano, 2008; Kjernsmo and Merilaita, 2013; Kjernsmo et al., 2016; Hemingson et al., 2021).
Eyespots reduce the chance of being eaten in a number of ways. Peripherally positioned eyespots, such as those of the squinting bush brown (Bicyclus anynana) and the woodland brown (Lopinga achine) butterflies, can deflect attacks to non-vital body parts (Olofsson et al., 2010; Skelhorn et al., 2014). The eyespots are thought to draw predators’ attention, causing them to direct their attacks toward dispensable areas (e.g., wing margins), enhancing the chances that prey will survive the attack (Blest, 1957; Kjernsmo and Merilaita, 2013; Prudic et al., 2015). Larger eyespots such as those of the peacock butterfly Aglais io elicit an aversive response in predators (Vallin et al., 2005, 2010, 2011; Kodandaramaiah et al., 2009). The question of why predators should find eyespots aversive has caused considerable debate (Stevens, 2005; Stevens and Ruxton, 2014; Mukherjee and Kodandaramaiah, 2015). However, there is now evidence for two non-exclusive explanations. Eyespots appear to exploit predators’ innate or learned aversions to conspicuously colored prey (Stevens et al., 2008, 2009), and mimic the eyes of the predators’ own predators (Blut et al., 2012; De Bona et al., 2015; Kjernsmo and Merilaita, 2017).
The specific design of eyespots has received much less attention (though see: Stevens et al., 2008; Blut et al., 2012; Hossie et al., 2015; Kjernsmo and Merilaita, 2017 and related papers on responses of birds and reptiles to eye-like stimuli, e.g., Gagliardi et al., 1976; Hennig, 1977). There are lots of ways to be conspicuous and several ways to resemble eyes. However, many (although by no means all) eyespots consist of multiple concentric rings of contrasting colors (Skelhorn et al., 2016). This may well be due to the radial development of pigmented cells from each eyespot’s center (Monteiro, 2015). Alternatively, concentric circles with either a bright or dark central region, and the opposite value in its surround may be particularly effective at stimulating vertebrate visual systems and associated brain regions (e.g., the optic tectum; Mey and Thanos, 2000). This common eyespot configuration may be highly conspicuous to the circular receptive fields of vertebrates (as suggested by Stevens, 2005; Stevens et al., 2007, 2008), and conspicuous signals can be especially aversive to birds (Stevens et al., 2008; Halpin et al., 2020). Additionally, concentric circles subjectively resemble forward-facing eyes. Several species of birds and reptiles are sensitive to subtle eye gaze cues, and respond more aversively when gaze is directed toward them compared to when it is averted (Hennig, 1977; Carter et al., 2008; von Bayern and Emery, 2009; Clucas et al., 2013; Garland et al., 2014; Goumas et al., 2019, 2020). Consequently, if eyespots are perceived as predatory eyes, then we might expect forward-facing eyespots to be particularly effective because they appear to gaze at predators approaching from a wide range of different directions. Conversely, the efficacy of eyespots made up of eccentric circles should be heavily influenced by predator approach direction, being most effective when eyespots appear to “gaze” toward the approaching predator and least effective when they “gaze” away from it. Here we test these ideas using naïve domestic chicks Gallus gallus domesticus as predators of artificial moth-like prey.
A total of 126 domestic chicks (Gallus gallus domesticus) of the “Hubbard Brown” strain served as predators. Chicks were acquired in two batches (n = 63 per batch) from a commercial hatchery on the day they hatched. They were housed in cages measuring 120 cm × 50 cm × 50 cm, and were subject to a 14L: 10D cycle using uncovered fluorescent lights (OSRAM L360Q/965 Biolux). Cage temperatures were maintained at 25–28°C using a room heater. Water was provided ad lib., as were chick starter crumbs except during training and experimenting when food restriction was necessary. When access to food was restricted, chicks had access to water. Chicks received mealworms (Tenebrio molitor) twice a day in their home cages. All subjects were marked with non-toxic Sharpie marker pens which did not have any adverse effects on their behavior. Weights were monitored for welfare purposes throughout the experiment, and all chicks gained weight as the experiment progressed. No specific measures were taken to obscure the eyes of the staff performing routine husbandry. However, during all training and experimental trials, chicks were viewed from above and behind the arena in an attempt to ensure that chicks could not see the experimenter’s head.
Artificial moths were created by pinning paper triangles (base 44 mm, height 37 mm) over mealworms (Tenebrio molitor) to completely obscure them from view. We used Adobe Illustrator 14 (13) to create three types of prey. All three types possessed a pair of eyespots that consisted of a larger white circle containing a smaller gray circle. In left-shifted prey, the smaller circle was shifted to the moth’s left; in central prey, the smaller circle was in the center of the larger circle; and in right-shifted prey, the smaller circle was shifted to the moth’s right. We also created three training stimuli with the same conspicuousness as the test stimuli (measured as luminance), but without eyespots (see Figure 1).
Figure 1. Images of the artificial moth stimuli. From left to right: training prey, left-shifted prey (the central circle is shifted to the moth’s left), central-circle prey, and right-shifted prey (the central circle is shifted to the moth’s right).
All prey consisted of a triangle 100 pt in width and 86.6 pt in height and filled with mid gray (RGB: 164, 164, 164; C: 38 M: 31 Y: 31 K:0). To produce test stimuli that possessed eyespots, we added two white circles 31.4 pt in diameter and two gray circles 16.0 pt in diameter. The centers of the white circles were always positioned 30.5 and 69.5 pt to the right of, and 18.4 pt above, the left apex of the triangle (thus ensuring that each eyespot was equidistant from the triangle’s midline). However, the position of the smaller circles differed among the three test stimuli: to produce central prey, we positioned the centers of the gray circles in the same positions as the centers of the white circles; to produce left- and right-shifted eyespots, we shifted the smaller circles 6.6 pt to the prey’s left or right, respectively. We then created three training stimuli with similar luminance to the test stimuli, but without eyespots. These had “mottled” patterns, and were created by drawing 10 irregular white shapes of similar area and positioning them so that they were spread over the entire triangle. The combined area of the white shapes matched the area of two white circles minus that of the two gray circles (that is, the white area on a mottled stimulus was the same as that on an experimental stimulus). This was achieved by adjusting the shapes until the luminance of the mottled stimulus matched the luminance of the experimental stimuli. Three training stimuli were produced by rotating and repositioning the shapes (an example of one of the training stimuli and all of the test stimuli can be seen in Figure 1).
On day one post-hatch, chicks were allowed to acclimate to the laboratory for 1 h, before being randomly assigned to one of three experiments (N = 42 per experiment). Chicks in each of the three experiments were then randomly assigned to one of three experimental groups (N = 14 per experimental group). All birds were trained to attack training stimuli pinned to the back wall of a runway. The protocol was identical in all three experiments, but each experiment used a different runway (Figure 2). All runways were made of reinforced cardboard covered in black paper. The distance between the start point (at which chicks were introduced to the runway) and the center of the rear wall was 50 cm, and the distance between the right and the left wall was 15 cm. However, the angle between the side walls and rear wall differed among experiments. From the inside of the runway, the angle between the left-hand wall (as viewed when facing the rear wall) and the rear wall was 135° in Experiment 1, 90° in Experiment 2, and 45° in Experiment 3. This arrangement meant that chicks were only able to approach prey items pinned to the rear wall from the prey’s left, straight on, and from the prey’s right, respectively.
Figure 2. Diagrams of the experimental runways used in Experiment 1 (left), Experiment 2 (center), and Experiment 3 (right). A single prey item was pinned to the center of the rear wall 10 cm above the floor. The distance between the start point (at which chicks were introduced to the runway) and the center of the rear wall was 50 cm, and the distance between the right and the left wall was 15 cm. The angle between the side walls and rear wall differed among experiments. From the inside of the runway, the angle between the left-hand wall (as viewed when facing the rear wall) and the rear wall was 135° in Experiment 1, 90° in Experiment 2 and 45° in Experiment 3. This arrangement meant that chicks were only able to approach prey items pinned to the rear wall from the prey’s left, straight on, and from the prey’s right, respectively.
On day one post-hatch, chicks were trained to forage alone in the runways in six training trials at regular intervals throughout the day. In all trials, chick crumbs were scattered over the white laminated floor of the experimental runway, and chicks were then placed in the runway for 2 min. In the first two trials, chicks were placed in runways in groups of three; in the following two trials, in groups of two; and in the final two trials chicks were placed in runways individually. Chicks had restricted access to food for 30 min prior to each of the final three trials. By the end of trial six, all chicks were eating chick crumbs in the runways.
On day 2 post-hatch chicks were trained to eat mealworms pinned to the rear wall of the experimental runways. Each chick received four trials at regular intervals throughout the day. In each trial, a single mealworm was pinned to the center of the rear wall 10 cm from the runway floor. Chicks had restricted access to food for 30 min and were then placed in the runway and left there until they attacked the mealworm. This process was repeated on day 3 post-hatch, but in these trials a training stimulus (mottled paper triangle, Figure 1) was pinned over the mealworm partly obscuring it from view. Finally, on day 4 post-hatch, each chick received a further four trials in which the training stimulus completely obscured the mealworm from view: the base of the stimulus was always positioned in the center of the back wall, 10 cm from the runway floor. We used three training stimuli (as described above) during trials on days 3 and 4 to ensure that our findings were not restricted to a specific training regimen. Each regimen was received by two birds from each of the three experimental groups in each of the three experiments. Birds received either stimulus one, stimulus two, stimulus three, stimuli one and two, stimuli one and three, stimuli two and three, or all three stimuli. All chicks attacked the mealworm within 10 min in every training trial, and by the end of the final trial, all chicks attacked the artificial prey item within 2 s of entering the arena.
On day 5 post-hatch, chicks were randomly assigned to one of three experimental groups (n = 14 per group). All chicks received a single test trial. Each chick had restricted access to food for 30 min before being placed into the same runway used in training. A single prey item was pinned to the center of the rear wall 10 cm above the floor. The position of the central circles of the eyespot (analogous to the iris of a real eye) differed among experimental groups. In each experiment, one group of chicks encountered prey with circles shifted to the prey’s left, one encountered prey with centered circles, and one encountered prey with circles shifted to the prey’s right (see Figure 1). To human observers positioned directly in front of the prey, these eyespots appeared to “gaze” to the prey’s left, straight on, and right, respectively. Chicks remained in the runway until they attacked the prey or until 10 min had elapsed (whichever came first). We recorded the latency to attack the prey, and the presence/absence of approach-retreat behavior: repeatedly approaching and retreating from the prey (Skelhorn et al., 2014, 2015). Only five chicks failed to attack the prey in 10 min (two in experiment 1, two in experiment 2, and one in experiment 3), and these were included in the analyses by awarding them an attack latency of 601 s. There were no qualitative differences between these analyses and analyses in which these chicks were excluded (see Supplementary material). Chicks were trained and tested in the same random order throughout the experiments.
All procedures were in accordance with UK Home Office regulations and the Association for the Study of Animal Behavior’s Guidelines for the Treatment of Animals in Research and Teaching. The University Psychology Ethics Committee approved the experiment (proposal 2013/319). Chicks were donated to a free-range smallholding at the end of the study.
We square root transformed the latency data for positive skew in the raw data, and analyzed the effect of eyespot design on the latency to approach with a general linear model with a Gaussian distribution and identity link function. We calculated standardized effect sizes (Cohen’s d) using Package ‘rstatix’ (Kassambara, 2021). We analyzed the approach retreat behavior with Fisher’s exact tests. We calculated standardized effect sizes (Cohen’s d) using the package rstatix. The GLM and associated post-hoc tests were conducted using R version 4.1.2. The Fisher’s tests were conducted using GraphPad1.
By the end of training, all chicks readily attacked the training prey within 2 s. In the test trial, however, all chicks took longer than 2 s to attack the test stimulus, indicating that the eyespots had a deterrent effect. In Experiment 1, when chicks approached from the prey’s left, there was a significant difference in the average latency to approach the prey (main effect: F2, 39 = 14.06, p < 0.0001; see Figure 3). The average attack latency did not differ between the left-shifted group and the central group (estimate = −0.09 ± 1.74, df = 39, t = −0.049, P = 0.9987). However, chicks in both these groups took longer to attack prey than chicks in the right-shifted group (left: estimate = 7.97 ± 1.74, df = 39, t = −4.567, P = 0.0001, Cohen’s d = 1.16; central: estimate = 8.06 ± 1.74, df = 39, t = −4.617, P = 0.0001, Cohen’s d = 1.52). In Experiment 2, when chicks approached prey straight on, there was no significant difference in the average attack latency among our experimental groups (main effect: F2, 39 = 0.009, P = 0.992; see Figure 3). Finally, in Experiment 3, when chicks approached from the prey’s right, there was a significant difference in the average latency to approach the three prey types (main effect: F2, 39 = 15.91, p < 0.0001; see Figure 3). The average attack latency did not differ between right-shifted group and the central group (estimate = −1.24 ± 1.79, t = −0.696, p = 0.7669). However, chicks in both these groups took longer to attack prey than chicks in the left-shifted group (right: estimate = −9.28 ± 1.79, df = 39, t = −5.196, P < 0.0001, Cohen’s d = −1.88; central: estimate = −8.03 ± 1.79, df = 39, t = 4.50, P = 0.0002, Cohen’s d = −1.44).
Figure 3. Time taken to attack the test stimuli for birds in each of the three experiments. light gray bars represent groups given left-shifted prey; mid-gray bars groups given prey with central circles; and dark gray bars groups given right-shifted prey (N = 14 in each group). Boxplots show the median, interquartile range, and the whiskers represent the largest and smallest value within 1.5 times the 25th and 75th percentile. Outliers are represented by small black dots.
Similar trends were seen in approach retreat behavior: an established measure of wariness, where chicks repeatedly approach and retreat from prey before making contact with it (Skelhorn et al., 2014, 2015). In Experiment 1, when chicks approached prey from the prey’s left, there was no significant difference between the left-shifted group and the central group in the number of chicks that demonstrated this behavior (P = 0.4815; see Figure 4). However, in both these groups more chicks demonstrated this behavior than in the right-shifted group (right vs. left: P = < 0.0001; right vs. straight: P = 0.0004). In Experiment 2, when chicks approached prey straight on, there was no significant difference in the number of chicks demonstrating this behavior among our experimental groups (P > 0.1 in all cases; see Figure 4). Finally, in Experiment 3, when chicks approached from the prey’s right, the right-shifted group and the central group did not differ in the number of chicks that demonstrated this behavior (Fisher’s exact test, P = 0.4815; see Figure 4). However, more chicks in the right-shifted group demonstrated this behavior than in the left-shifted group (left vs. right: P = 0.0006), and there was a similar trend for the central group that was not significant after correcting for multiple comparison (left vs. central: P = 0.0183).
Figure 4. Number of chicks showing approach retreat behavior (dark gray bar) and those that did not (light gray bar) in each experimental group. The x-axis shows the approach direction, and the prey image above the bars shows the type of prey encountered.
Our study shows that the effectiveness of artificial eyespots is dependent on their configuration and the approach direction of the predator. When chicks approached prey straight on, eyespot configuration had no significant effect on our measures of chick behavior. However, when chicks approached prey from either the left or right, chicks were slower to attack prey with eyespots shifted in the direction of their approach compared to when they encountered prey with the central circle of the eyespot shifted away from the direction of their approach. Chicks were also more likely to demonstrate aversive responses (approach-retreat behavior), when they encountered prey with the central circle of the eyespot centrally placed or shifted in the direction of the chick’s approach. This suggests that eyespots consisting of concentric circles could offer greater protection than eyespots with left- or right-shifted central circles in situations where individual predators approach from a range of different directions, as is likely the case for moths and butterflies (Stevens, 2005), but not for caterpillars (Hossie et al., 2015).
Although we did not directly test whether chicks mistook our artificial eyespots for eyes, our findings are consistent with this idea. Chicks may have found prey with the central circles shifted away from the direction of approach less aversive than other prey because they were perceived to have eyes that gazed away from, rather than toward, the approaching chick. This would reduce the risk of the chick being detected by the predator, competitor, or prey that the eyes were perceived to belong to. This explanation is in line with previous work demonstrating that birds respond more aversively to eyes that gaze toward rather than away from them (Carter et al., 2008; von Bayern and Emery, 2009; Clucas et al., 2013; Garland et al., 2014; Goumas et al., 2019, 2020). We didn’t, however, find a difference in protection between central eyespots and those shifted in the predator approach direction. This could be because eyespots with concentric circles appear to gaze directly at observers approaching from the side. In humans this perceptual phenomenon is known as the “Mona Lisa” effect (Bruce and Young, 1998), because the forward-facing eyes of that portrait appear to follow viewers around the room. Alternatively, the central circle of our eyespots may have been shifted too far toward the direction of approach, causing the prey to “look” slightly past the birds rather than directly at them.
It is more difficult to explain our findings in terms of prey conspicuousness as our prey were designed to be equally conspicuous by all commonly used measures. The number of gray and white pixels, the size and shape of boundaries between light and dark areas, and minimum, maximum and mean luminance were consistent across prey types. It is possible that the central gray circle appeared to merge with the outer gray area of the prey when it was shifted away from the direction of approach, thus reducing perceived internal contrast. However, human observers perceived a distinct inner circle when viewing the prey from the start of the experimental runway. In addition, our findings provide no support for the idea that concentric circles are particularly aversive because they are more effective at stimulating vertebrate visual systems (Stevens et al., 2007, 2008). Predators approaching prey straight on were equally reluctant to attack prey with concentric and eccentric circles.
It could be argued that we should have included control groups that were given prey without eyespots. We decided against this because our aim was to establish how the relative aversiveness of the stimuli changed with viewing angle (i.e., across experiments), not how aversive eyespots were compared to a specific control stimulus. We also chose to use artificial stimuli in a laboratory environment as this allowed us to manipulate prey appearance and predator approach direction in a controlled manner. The use of paper prey with achromatic eyespots has been fundamental in establishing the antipredator benefits of eyespots (Blest, 1957; Scaife, 1976; Coss, 1979), yet such prey may not capture the nuances of real prey (De Bona et al., 2015). Whilst future work comparing how predators’ responses to artificial and live prey would be beneficial, we predict that predators are likely to have a broad view of what is considered a conspicuous/eye-like stimulus because the cost of failing to respond to such stimuli is potentially very high. It could also be argued that the question we address is irrelevant because eyespots design is not the result of predator-driven selection, but rather developmental constraints (Monteiro, 2015). This, however, misses two key points. Conspicuous eyespots are only likely to evolve if they are beneficial to prey, and we cannot fully understand the benefit of eyespots without establishing how, and under what circumstances, they work. Moreover, not all eyespots consist of concentric circles, meaning that in some species developmental constraints are either absent or have been overcome.
Irrespective of these limitations, our results clearly demonstrate that eyespot design and predator approach direction can influence the antipredator benefits of eyespots. Consequently, the microhabitat in which prey position themselves and the feeding ecology of predators could affect eyespot efficacy (and perhaps evolution), and these factors should be carefully considered when designing future experiments.
The original contributions presented in this study are included in the article/Supplementary material, further inquiries can be directed to the corresponding author.
This animal study was reviewed and approved by the University of Exeter Psychology Ethics Committee (proposal 2013/319).
HR: conceptualization, methodology, formal analysis, data curation, writing—original draft, and visualization. JS: conceptualization, methodology, investigation, resources, data curation, writing—original draft, and funding acquisition. Both authors contributed to the article and approved the submitted version.
We thank RC and KK for their reviews; Uwe Mayer and Orsola Rosa Salva for their helpful discussions about the manuscript; and Robert Burriss for help with constructing the stimuli, and for helping with drafts of the manuscript. We also thank the Max Planck Society for funding to HR, Exeter University for the start-up package that funded JS for the duration of the experiment, and Newcastle University for funding JS during data analysis and manuscript preparation.
The authors declare that the research was conducted in the absence of any commercial or financial relationships that could be construed as a potential conflict of interest.
All claims expressed in this article are solely those of the authors and do not necessarily represent those of their affiliated organizations, or those of the publisher, the editors and the reviewers. Any product that may be evaluated in this article, or claim that may be made by its manufacturer, is not guaranteed or endorsed by the publisher.
The Supplementary Material for this article can be found online at: https://www.frontiersin.org/articles/10.3389/fevo.2022.951967/full#supplementary-material
Altbäcker, V., and Csányi, V. (1990). The role of eyespots in predator recognition and antipredatory behaviour of the paradise fish, Macropodus opercularis L. Ethology 85, 51–57.
Blest, A. D. (1957). The Function of Eyespot Patterns in the Lepidoptera. Behaviour 11, 209–258. doi: 10.1163/156853956X00048
Blut, C., Wilbrandt, J., Fels, D., Girgel, E. I., and Lunau, K. (2012). The ‘sparkle’ in fake eyes – the protective effect of mimic eyespots in lepidoptera. Entomol. Exp. Appl. 143, 231–244. doi: 10.1111/j.1570-7458.2012.01260.x
Buschman, L. L. (1988). Light Organs of Immature Fireflies (Coleoptera: Lampyridae) as Eye-Spot/False-Head Displays. Coleopt. Bull. 42, 94–97.
Carter, J., Lyons, N. J., Cole, H. L., and Goldsmith, A. R. (2008). Subtle cues of predation risk: starlings respond to a predator’s direction of eye-gaze. Proc. Roy. Soc. B 275, 1709–1715. doi: 10.1098/rspb.2008.0095
Castner, J. L. (1995). Defensive Behavior and Display of the Leaf-Mimicking Katydid Pterochroza ocellata (L.) (Orthoptera: Tettigoniidae: Pseudophyllinae: Pterochrozini). J. Orthopt. Res. 4, 89–92. doi: 10.2307/3503462
Chan, I. Z. W., Ngan, Z. C., Naing, L., Lee, Y., Gowri, V., and Monteiro, A. (2021). Predation favours Bicyclus anynana butterflies with fewer forewing eyespots. Proc. Roy. Soc. B 288:20202840. doi: 10.1098/rspb.2020.2840
Clucas, B., Marzluff, J. M., Mackovjak, D., and Palmquist, I. (2013). Do A merican Crows Pay Attention to Human Gaze and Facial Expressions? Ethology 119, 296–302.
Coss, R. G. (1979). Delayed plasticity of an instinct: recognition and avoidance of 2 facing eyes by the jewel fish. Develop. Psychobiol. 12, 335–345. doi: 10.1002/dev.420120408
De Bona, S., Valkonen, J. K., López-Sepulcre, A., and Mappes, J. (2015). Predator mimicry, not conspicuousness, explains the efficacy of butterfly eyespots. Proc. Roy. Soc. B 282:20150202. doi: 10.1098/rspb.2015.0202
Gagliano, M. (2008). On the spot: the absence of predators reveals eyespot plasticity in a marine fish. Behav. Ecol. 19, 733–739. doi: 10.1093/beheco/arn013
Gagliardi, G. J., Gallup, G. G., and Boren, J. L. (1976). Effect of different pupil to eye size ratios on tonic immobility in chickens. Bull. Psychon. Soc. 8, 58–60.
Garland, A., Low, J., Armstrong, N., and Burns, K. C. (2014). Wild robins (Petroica longipes) respond to human gaze. Anim. Cogn. 17, 1149–1156. doi: 10.1007/s10071-014-0747-y
Goumas, M., Burns, I., Kelley, L. A., and Boogert, N. J. (2019). Herring gulls respond to human gaze direction. Biol. Lett. 15:20190405. doi: 10.1098/rsbl.2019.0405
Goumas, M., Collins, T. R., Fordham, L., Kelley, L. A., and Boogert, N. J. (2020). Herring gull aversion to gaze in urban and rural human settlements. Anim. Behav. 168, 83–88.
Halali, D., Krishna, A., Kodandaramaiah, U., and Molleman, F. (2019). Lizards as Predators of Butterflies: Shape of Wing Damage and Effects of Eyespots. Society 73:79.
Halpin, C. G., Penacchio, O., and Lovell, P. G. (2020). Pattern contrast influences wariness in naïve predators towards aposematic patterns. Sci. Rep. 10:9246. doi: 10.1038/s41598-020-65754-y
Hemingson, C. R., Siqueira, A. C., Cowman, P. F., and Bellwood, D. R. (2021). Drivers of eyespot evolution in coral reef fishes. Evolution 75, 903–914. doi: 10.1111/evo.14197
Hennig, C. W. (1977). Effects of simulated predation on tonic immobility in Anolis carolinensis: The role of eye contact. Bull. Psychon. Soc. 9, 239–242. doi: 10.3758/BF03336987
Ho, S., Schachat, S. R., Piel, W. H., and Monteiro, A. (2016). Attack risk for butterflies changes with eyespot number and size. Roy. Soc. Open Sci. 3:150614. doi: 10.1098/rsos.150614
Hossie, T. J., Skelhorn, J., Breinholt, J. W., Kawahara, A. Y., and Sherratt, T. N. (2015). Body size affects the evolution of eyespots in caterpillars. Proc. Natl. Acad. Sci. U.S.A. 112, 6664–6669. doi: 10.1073/pnas.1415121112
Huq, M., Bhardwaj, S., and Monteiro, A. (2019). Male Bicyclus anynana Butterflies Choose Females on the Basis of Their Ventral UV-Reflective Eyespot Centers. J. Insect Sci. 19:25. doi: 10.1093/jisesa/iez014
Janzen, D. H., Hallwachs, W., and Burns, J. M. (2010). A tropical horde of counterfeit predator eyes. Proc. Natl. Acad. Sci. U.S.A. 107, 11659–11665. doi: 10.1073/pnas.0912122107
Kassambara, A. (2021). Package ‘rstatix’. https://cran.r-project.org/web/packages/rstatix/index.html.
Kjernsmo, K., Grönholm, M., and Merilaita, S. (2016). Adaptive constellations of protective marks: eyespots, eye stripes and diversion of attacks by fish. Anim. Behav. 111, 189–195. doi: 10.1016/j.anbehav.2015.10.028
Kjernsmo, K., and Merilaita, S. (2013). Eyespots divert attacks by fish. Proc. Roy. Soc. B 280:20131458. doi: 10.1098/rspb.2013.1458
Kjernsmo, K., and Merilaita, S. (2017). Resemblance to the enemy’s eyes underlies the intimidating effect of eyespots. Am. Natural. 190, 594–600. doi: 10.1086/693473
Kodandaramaiah, U. (2011). The evolutionary significance of butterfly eyespots. Behav. Ecol. 22, 1264–1271. doi: 10.1093/beheco/arr123
Kodandaramaiah, U., Vallin, A., and Wiklund, C. (2009). Fixed eyespot display in a butterfly thwarts attacking birds. Anim. Behav. 77, 1415–1419. doi: 10.1016/j.anbehav.2009.02.018
Lyytinen, A., Brakefield, P. M., Lindstrom, L., and Mappes, J. (2004). Does predation maintain eyespot plasticity in Bicyclus anynana? Proc. Biol. Sci. 271, 279–283. doi: 10.1098/rspb.2003.2571
Meadows, D. W. (1993). Morphological Variation in Eyespots of the Foureye Butterflyfish (Chaetodon capistratus): Implications for Eyespot Function. Copeia 1993, 235–240. doi: 10.2307/1446319
Merilaita, S., Vallin, A., Kodandaramaiah, U., Dimitrova, M., Ruuskanen, S., and Laaksonen, T. (2011). Number of eyespots and their intimidating effect on naïve predators in the peacock butterfly. Behav. Ecol. 22, 1326–1331. doi: 10.1093/beheco/arr135
Mey, J., and Thanos, S. (2000). Development of the visual system of the chick: I. Cell differentiation and histogenesis. Brain Res. Rev. 32, 343–379.
Monteiro, A. (2015). Origin, development, and evolution of butterfly eyespots. Annu. Rev. Entomol. 60, 253–271.
Mukherjee, R., and Kodandaramaiah, U. (2015). What makes eyespots intimidating–the importance of pairedness. BMC Evolut. Biol. 15:34. doi: 10.1186/s12862-015-0307-3
Olofsson, M., Løvlie, H., Tibblin, J., Jakobsson, S., and Wiklund, C. (2012). Eyespot display in the peacock butterfly triggers antipredator behaviors in naïve adult fowl. Behav. Ecol. 24, 305–310. doi: 10.1093/beheco/ars167
Olofsson, M., Vallin, A., Jakobsson, S., and Wiklund, C. (2010). Marginal Eyespots on Butterfly Wings Deflect Bird Attacks Under Low Light Intensities with UV Wavelengths. PLoS One 5:e10798. doi: 10.1371/journal.pone.0010798
Prudic, K. L., Stoehr, A. M., Wasik, B. R., and Monteiro, A. (2015). Eyespots deflect predator attack increasing fitness and promoting the evolution of phenotypic plasticity. Proc. Roy. Soc. B 282:20141531. doi: 10.1098/rspb.2014.1531
Robertson, K. A., and Monteiro, A. (2005). Female Bicyclus anynana butterflies choose males on the basis of their dorsal UV-reflective eyespot pupils. Proc. Roy. Soc. B 272, 1541–1546. doi: 10.1098/rspb.2005.3142
Scaife, M. (1976). The response to eye-like shapes by birds. I. The effect of context: A predator and a strange bird. Anim. Behav. 24, 195–199. doi: 10.1016/S0003-3472(76)80115-7
Skelhorn, J., Dorrington, G., Hossie, T. J., and Sherratt, T. N. (2014). The position of eyespots and thickened segments influence their protective value to caterpillars. Behav. Ecol. 25, 1417–1422. doi: 10.1093/beheco/aru154
Skelhorn, J., Holmes, G. G., Hossie, T. J., and Sherratt, T. N. (2015). Multicomponent deceptive signals reduce the speed at which predators learn that prey are profitable. Behav. Ecol. 27, 141–147. doi: 10.1093/beheco/arv135
Skelhorn, J., Holmes, G. G., Hossie, T. J., and Sherratt, T. N. (2016). Eyespots. Curr. Biol. 26, R52–R54.
Steiner, A. L. (1981). Anti-Predator Strategies. II. Grasshoppers (Orthoptera, Acrididae) Attacked by Prionyx Parkeriand Some Tachysphex Wasps (Hymenoptera, Sphecinae and Larrinae): A descriptive Study. Psyche 88:031458. doi: 10.1155/1981/31458
Stevens, M. (2005). The role of eyespots as anti-predator mechanisms, principally demonstrated in the Lepidoptera. Biol. Rev. 80, 573–588. doi: 10.1017/S1464793105006810
Stevens, M., Cantor, A., Graham, J., and Winney, I. S. (2009). The function of animal ‘eyespots’: Conspicuousness but not eye mimicry is key. Curr. Zool. 55, 319–326.
Stevens, M., Hardman, C. J., and Stubbins, C. L. (2008). Conspicuousness, not eye mimicry, makes “eyespots” effective antipredator signals. Behav. Ecol. 19, 525–531. doi: 10.1093/beheco/arm162
Stevens, M., Hopkins, E., Hinde, W., Adcock, A., Connolly, Y., Troscianko, T., et al. (2007). Field experiments on the effectiveness of ‘eyespots’ as predator deterrents. Anim. Behav. 74, 1215–1227. doi: 10.1016/j.anbehav.2007.01.031
Vallin, A., Dimitrova, M., Kodandaramaiah, U., and Merilaita, S. (2011). Deflective effect and the effect of prey detectability on anti-predator function of eyespots. Behav. Ecol. Sociobiol. 65, 1629–1636. doi: 10.1007/s00265-011-1173-7
Vallin, A., Jakobsson, S., Lind, J., and Wiklund, C. (2005). Prey survival by predator intimidation: an experimental study of peacock butterfly defence against blue tits. Proc. Roy. Soc. B 272, 1203–1207. doi: 10.1098/rspb.2004.3034
Vallin, A., Jakobsson, S., and Wiklund, C. (2010). Constant eyespot display as a primary defence-survival of male and female emperor moths attacked by blue tits. J. Res. Lepidopt. 43, 9–17.
von Bayern, A. M., and Emery, N. J. (2009). Jackdaws respond to human attentional states and communicative cues in different contexts. Curr. Biol. 19, 602–606. doi: 10.1016/j.cub.2009.02.062
Keywords: antipredator defense, eye gaze, mimicry, predator-prey, protective coloration, wariness
Citation: Skelhorn J and Rowland HM (2022) Eyespot configuration and predator approach direction affect the antipredator efficacy of eyespots. Front. Ecol. Evol. 10:951967. doi: 10.3389/fevo.2022.951967
Received: 24 May 2022; Accepted: 14 September 2022;
Published: 12 October 2022.
Edited by:
Martin J. Whiting, Macquarie University, AustraliaReviewed by:
Karin Kjernsmo, University of Bristol, United KingdomCopyright © 2022 Skelhorn and Rowland. This is an open-access article distributed under the terms of the Creative Commons Attribution License (CC BY). The use, distribution or reproduction in other forums is permitted, provided the original author(s) and the copyright owner(s) are credited and that the original publication in this journal is cited, in accordance with accepted academic practice. No use, distribution or reproduction is permitted which does not comply with these terms.
*Correspondence: Hannah M. Rowland, aHJvd2xhbmRAaWNlLm1wZy5kZQ==
Disclaimer: All claims expressed in this article are solely those of the authors and do not necessarily represent those of their affiliated organizations, or those of the publisher, the editors and the reviewers. Any product that may be evaluated in this article or claim that may be made by its manufacturer is not guaranteed or endorsed by the publisher.
Research integrity at Frontiers
Learn more about the work of our research integrity team to safeguard the quality of each article we publish.