- 1Animal Behavior Lab, Free University of Berlin, Berlin, Germany
- 2Museum für Naturkunde–Leibniz Institute for Evolution and Biodiversity Science, Berlin, Germany
- 3Deutsche Fledermauswarte e.V., Berlin, Germany
- 4Naturschutz, Landwirtschaft, Gartenbau, Schifffahrt und Wasserwirtschaft, Landratsamt Garmisch-Partenkirchen, Garmisch-Partenkirchen, Germany
- 5ChiroTEC–Verhaltenssensorik und Umweltgutachten, Lohra, Germany
Bats employ a variety of social calls for communication purposes. However, for most species, social calls are far less studied than echolocation calls and their specific function often remains unclear. We investigated the function of in-flight social calls during autumn swarming in front of a large hibernaculum in Northern Germany, whose main inhabitants are two species of Myotis bats, Natterer’s bats (Myotis nattereri) and Daubenton’s bats (Myotis daubentonii). We recorded social calls in nights of high swarming activity and grouped the calls based on their spectro-temporal structure into ten types and verified our visual classification by a discriminant function analysis. Whenever possible, we subsequently assigned social calls to either M. daubentonii or M. nattereri by analyzing the echolocation calls surrounding them. As many bats echolocate at the same time during swarming, we did not analyze single echolocation calls but the “soundscape” surrounding each social call instead, encompassing not only spectral parameters but also the timbre (vocal “color”) of echolocation calls. Both species employ comparatively similar social call types in a swarming context, even though there are subtle differences in call parameters between species. To additionally gain information about the general function of social calls produced in a swarming context, we performed playback experiments with free-flying bats in the vicinity of the roost, using three different call types from both species, respectively. In three out of six treatments, bat activity (approximated as echolocation call rate) increased during and after stimulus presentation, indicating that bats inspected or approached the playback site. Using a camera trap, we were sometimes able to identify the species of approaching bats. Based on the photos taken during playbacks, we assume one call type to support interspecific communication while another call type works for intraspecific group cohesion.
Introduction
Information is transmitted from a signaler to a receiver not only between individuals of one species (conspecifics), but often also between individuals belonging to different species (heterospecifics). This information transfer, referred to as communication, incorporates different sensory modalities, allowing animals to communicate via olfactory, visual, tactile, and acoustic signals (Bradbury and Vehrencamp, 2011). Acoustic stimuli provide a variety of information about an individual at a distance, such as individual identity (Carlson et al., 2020), emotional state (Briefer, 2012) and population affiliation (Podos and Warren, 2007). Correspondingly, the functions of intraspecific communication are diverse and can be essential for fitness, survival or reproductive success (Maynard Smith and Harper, 2003; Wilkins et al., 2013). For obvious reasons, animals should respond stronger to conspecific than to heterospecific communication signals (Ord and Stamps, 2009) but interspecific communication occurs as well. In the majority of cases, this form of communication is most accurately described as eavesdropping, where individuals gain information by listening in on the communication signals between heterospecifics. Across many vertebrates, eavesdropping is commonly used and the increased information uptake can provide benefits to the listener such as increased foraging opportunities or earlier detection of predators (Oda and Masataka, 1996; Mönkkönen and Forsman, 2002; Lea et al., 2008; Magrath et al., 2015).
For bats, acoustic signals are highly developed and not only important for communication but also for orientation in a predominantly dark environment. As nocturnal, fast-moving animals, bats rely mainly on echolocation calls as acoustic cues to perceive their surroundings (Fenton, 1984). Such calls are often species-specific, adapted to prey preferences or foraging technique (Schnitzler and Kalko, 2001; Neuweiler, 2003; Siemers and Schnitzler, 2004) and enable the bats to extraordinary spatial discrimination (Simmons et al., 1983). Although in echolocation the sender is also the receiver of the signal, the calls may encode information on individual identity (Kazial et al., 2008; Yovel et al., 2009; Voigt-Heucke et al., 2010), colony membership (Masters et al., 1995; Jameson and Hare, 2009), sex (Jones et al., 1992; Siemers et al., 2005; Knörnschild et al., 2012), or age (Jones et al., 1992; Masters et al., 1995) which can be processed by conspecifics. Echolocation calls may also facilitate species recognition interspecifically and have a communicative potential allowing interspecific eavesdropping in the wild (Schuchmann and Siemers, 2010; Dorado-Correa et al., 2013).
In contrast to echolocation, social vocalizations have purely communicative purposes and thus the goal to elicit a behavioral response from other individuals (Rendall et al., 2009; Bradbury and Vehrencamp, 2011). With their lower frequency, longer duration and more variable structure than echolocation calls, social vocalizations are better suited for information transfer and detection over longer distances and many bat species exhibit a diverse repertoire of social vocalizations serving a variety of behavioral functions (Pfalzer and Kusch, 2003; Middleton et al., 2014; Chaverri et al., 2018). Agonistic calls are emitted to defend foraging sites (Barlow and Jones, 1997), contact calls facilitate group cohesion (Chaverri et al., 2010; Arnold and Wilkinson, 2011), isolation calls are essential for females to identify their pups (Bohn et al., 2007; Knörnschild et al., 2013) and some bat species are known to employ multi-syllabic songs for male advertisement (Behr and von Helversen, 2004; Sachteleben and von Helversen, 2006) while others rely on shorter courtship calls (Barclay and Thomas, 1979; Knörnschild et al., 2014).
In bat social calls, more personal information is transmitted than in echolocation calls. Therefore, social vocalizations should be of higher diversity and more species-specific to reach the intended receiver than echolocation calls (Fenton, 1994; Chaverri et al., 2018). Nevertheless, social vocalizations can be used in interspecific eavesdropping, e.g., distress calls may attract heterospecifics who approach the calls to investigate the situation by themselves (Carter et al., 2015) and thus increase the chance of repelling predators (Russ et al., 2004) or even deter predators directly (Ancillotto et al., 2022). This interspecific communication might be further facilitated, especially in the case of distress calls, by shared acoustic call features (Hechavarría et al., 2020). Several studies have directly compared the effect of conspecific and heterospecific social calls on bats, with the overall effect that heterospecific social calls elicit a weaker response, if any, than conspecific social calls (Fenton et al., 1976; Schöner et al., 2010; Furmankiewicz et al., 2011; Hörmann et al., 2021). Despite this fact, bat vocalizations produced in the same general social context often have a noticeable similar spectro-temporal structure, especially in closely related species (Knörnschild et al., 2010; Bosia et al., 2022), which should facilitate interspecific eavesdropping at the very least.
Social vocalizations with known functions can provide valuable information about a species’ biology (Bohn and Gillam, 2018; Chaverri et al., 2018). However, for most bats, social vocalizations are far less studied than echolocation calls and their specific function often remains unclear. This is particularly the case for social calls that are not produced in the bats’ roost but on the wing. A behavior highly associated with a large amount of in-flight social calls and social group interactions is autumn swarming. Prior to hibernation many temperate zone bat species that hibernate in underground sites are engaged in such interaction, characterized by intense flight activity, chase flights and circling in and around the entrances of the roost without entering, accompanied by a large amount of both echolocation calls and social vocalizations (Fenton, 1969; Parsons et al., 2003). Various, not mutually exclusive functions of swarming are suggested, such as the finding and assessment of suitable hibernacula (van Schaik et al., 2015; Stumpf et al., 2017) or the facilitation of gene flow between otherwise isolated colonies and promiscuous mating behavior (Kerth et al., 2003; Veith et al., 2004; Rivers et al., 2005; Burns and Broders, 2015). Although bats produce high numbers of social calls during autumn swarming, comprehensive studies on their function are scarce (Furmankiewicz et al., 2013; Schmidbauer and Denzinger, 2019).
To expand our knowledge on social calls produced on the wing, we described the social call repertoire during autumn swarming at a large German hibernaculum. During winter, the hibernaculum is mainly inhabited by two Vespertilionid bats, Natterer’s bats (Myotis nattereri) and Daubenton’s bats (Myotis daubentonii), both of which also predominate the swarming population. We expected to record a variety of social calls due to the various functions of swarming. In addition, we conducted playback experiments with three social calls of both species, respectively, to get insights into the function and species-specificity of those social calls. If calls were used for group cohesion, we would expect a higher bat activity (indicated by a higher echolocation call rate) or even phonotaxis in response to our playbacks. If calls were used to keep other individuals at bay, we would expect the opposite effect. Photos taken of bats entering the playback area helped us to identify some reacting bats to species level and provided evidence whether calls served an intraspecific or interspecific communicative function.
Materials and methods
Study site and sound recordings
We observed swarming bats during 45 nights in two consecutive swarming seasons (August to November 2018 and August to October 2019) at both entrances of the Kalkberg cave (Bad Segeberg, Northern Germany, 10°18′57′′’E, 53°56′09′′’N) and conducted sound recordings on nights with very high swarming activity at various times between sunset and sunrise. Among the 30,000 hibernating bats in the natural cave are various Myotis species, with Natterer’s bat (M. nattereri) and Daubenton’s bats (M. daubentonii) making up for about 90% of the winter population at the hibernaculum (winter roost). Further inhabitants are pond bat (Myotis dasycneme), Bechstein’s bat (Myotis bechsteinii), greater mouse-eared bat (Myotis myotis), Brandt’s bat (Myotis brandtii) and brown long-eared bat (Plecotus auritus).
Prior to hibernation, between August and November, the vicinity of the cave is extensively used for autumn swarming. We recorded the social calls of swarming bats using a high-quality ultrasonic microphone (Avisoft USG 116 Hm with condenser microphone CM16; frequency range 1–200 kHz, sampling rate 500 kHz, 16-bit depth resolution) connected to a small computer (Dell Venue 8) running the software Avisoft Recorder (v4.2.05, R. Specht, Avisoft Bioacoustics, Glienicke, Germany). Both entrances of the cave have been monitored with light barriers since 1991 (ChiroTEC, Lohra, Germany). During the recording nights in 2018, the maximum activity (sum of individual arrivals and departures counted via light beam interruptions) was 10,415 and the minimum activity was 1,182. During the recording nights of 2019, the maximum activity was 11,678 and the minimum activity was 2,162. This means that we were recording during periods of high swarming activity, with dozens to hundreds individuals in the air at the same time (see Supplementary Video 1 for a video of swarming bats).
To complement our in-flight recordings, we recorded the social calls of several M. daubentonii and M. nattereri individuals roosting together in small crevices at another large German hibernaculum (Spandau Citadel; 13°12′46′′E 52°32′28′′N). Species identity of bats was achieved visually because the crevices were accessible and allowed us to see the bats’ faces clearly.
Moreover, we searched an already existing data set (Wimmer and Kugelschafter, 2015) for social calls emitted by single bats while they were flying in ten different underground hibernacula across Bavaria and Baden-Wurttemberg (Germany). In this data set, species identity was confirmed via photos taken from a camera connected to a light barrier. A bat passing through the light beam triggered both a photo and a sound recording, thus assigning species identity to each recording (see Wimmer and Kugelschafter (2015) for details on recording equipment). We used this data set to check whether the social call types found in single bats with clear species ID correspond to the call types we recorded from swarming bats.
Acoustic analysis of social vocalization and grouping into call types
Social calls were detected visually from the recordings and analyzed in Avisoft SASLabPro (v5.2.13, R. Specht, Glienicke, Germany). Based on their spectro-temporal structure we grouped the calls into ten distinct types. Eight call types were monosyllabic and two call types were multisyllabic: one consisted of the same syllable repeated several times, the other consisted of two different syllable types. We selected high-quality social calls without interfering echolocation calls to measure their parameters. Start and end of calls were determined manually based on the oscillograms. Even though some calls were multiharmonic, we measured only the fundamental frequency (first harmonic) because it contained most of the sound energy. Measurements were taken from oscillograms and spectrograms generated using a 1,024-point fast Fourier transformation, a frame size of 100% and a Hamming window with 93.75% overlap. We measured one waveform parameter (energy), two temporal parameters (duration, time to maximum amplitude) and five spectral parameters (peak frequency, minimum frequency, maximum frequency, bandwidth and entropy) in Avisoft SASLabPro. Entropy is a measure of the width and uniformity of the power spectrum (on a scale of 0–1, white noise has an entropy value of 1 and a pure tone has an entropy value of 0). Spectral parameters were measured at start, center and end of the call and also averaged over the entire call. Additionally, we measured the above-mentioned five spectral parameters at ten locations evenly distributed over the entire call to estimate the frequency and entropy curvature of the call. Derived curvature parameters combined various frequency (or entropy) measurements, thus reducing multicollinearity between original acoustic parameters. We performed principal component analyses (PCAs) with varimax rotation separately for frequency parameters and entropy parameters. For the frequency curvature, we extracted five principal components (with eigenvalues >1) which explained 92.16% of the total variance. For the entropy curvature, we extracted three principal components (with eigenvalues >1) which explained 72.44% of the total variance. Both PCAs fulfilled Kaiser–Meyer–Olkin (KMO) and Bartlett’s test criteria. In total, we measured 266 social calls.
To confirm our preliminary visual classification of social calls based on their spectro-temporal appearance, we performed a discriminant function analysis (DFA). Even though we had only ten different social call types, our DFA had twelve groups (one call type, the squawk, was recorded separately from both roosting M. daubentonii and M. nattereri, and another call type, the combined UI-shape call, consisted of two different syllables). In total, we included measurements for 266 social calls (6–49 calls per type; mean: 22.17 calls). We selected 18 acoustic parameters, checked them for multicollinearity and included them simultaneously into the DFA: energy, duration, time to maximum amplitude, peak frequency (start), peak frequency (end), peak frequency (center), peak frequency (mean), minimum frequency (mean), maximum frequency (mean), entropy (mean), frequency curvature 1–5 and entropy curvature 1–3. We used a cross-validation procedure to estimate the correct classification success, which classified each call based on discriminant functions established with all calls except the call being classified (n-1 cross-validation procedure). The DFA was adjusted to the unequal number of analyzed calls per type by computing group sizes based on prior probabilities. We subsequently checked for each group in our DFA whether the obtained classification success was better than a random classification (8.33%).
Species identification via feature analysis
Although some social calls have a similar spectro-temporal structure, they might be emitted by different species. To assign the social calls to species level we performed an analysis of the surrounding echolocation calls, focusing on the total soundscape rather than single calls. To do so we analyzed 1s-echolocation-snippets surrounding the social call (test data, Figure 1) to identify the predominantly swarming species (M. daubentonii or M. nattereri) directly before and after the social call was produced. Naturally, it is not a guarantee that the social call in question was produced by the species who was predominantly swarming at the time of social call production but it is an approximation at the very least (and currently the only method available to assign social calls of multiple swarming bat species to species level). Echolocation calls were assigned to species level (M. daubentonii or M. nattereri) based on a set of reference data [recorded by Wimmer and Kugelschafter (2015)] consisting of identified call sequences of single individuals from both species. This reference data set was used as a training set in a DFA and the echolocation snippets surrounding the social calls were used as a test data set. We only considered a species identification to be reliable if both echolocation snippets surrounding a social call were assigned to the same species by the DFA with a probability higher than 90%.
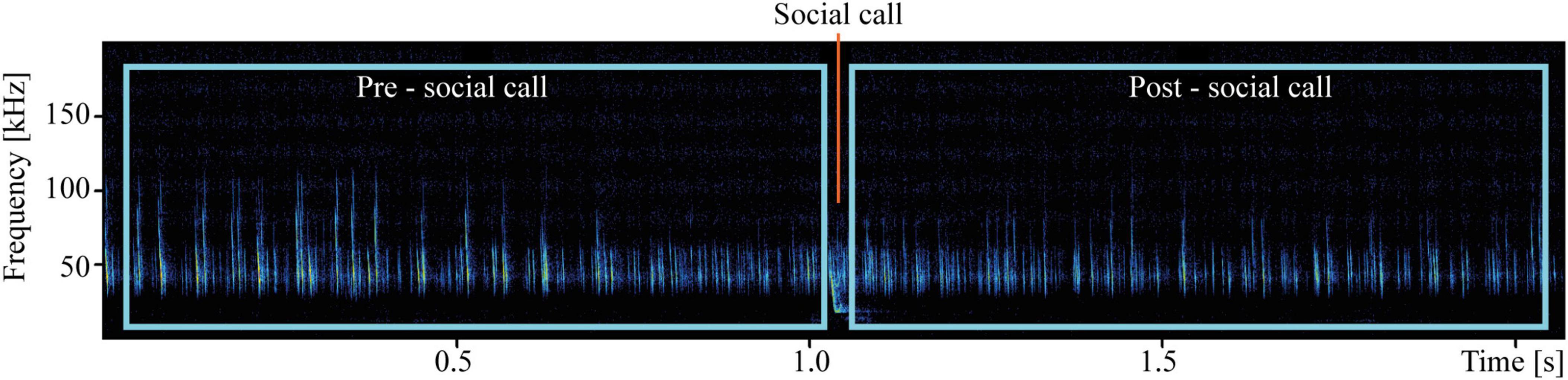
Figure 1. One second of overlapping echolocation calls from multiple swarming bats (“swarming soundscape”) before and after a social call was produced. The swarming soundscape was analyzed to extract acoustic parameters and assign the social call to a predominantly calling species based on a set of identified reference data.
Echolocation snippets consisted of many overlapping echolocation calls (the “swarming soundscape”) which we analyzed as a whole instead of focusing on single echolocation calls. For the test data set, start, end and peak frequency of the echolocation snippets were calculated with a custom-made MATLAB routine over the entire file in 10 ms frames using the meanfreq function from the Signal Processing toolbox. For the reference data set, start, end, and peak frequency of single echolocation calls were measured in Avisoft SASLab Pro (threshold of −24 dB relative to the peak amplitude; values averaged over the entire call). For both the test and the reference data set, the spectral centroid was calculated in Avisoft SASLab Pro (threshold: −28 dB relative to peak amplitude) and an acoustic feature extraction technique was used to extract five linear frequency cepstral coefficients (Hamming window; test data: 100 ms frame; reference data: 3 ms frame) with a custom-made routine in the speech processing toolbox “voicebox” in MATLAB (v. R2018b). Linear frequency cepstral coefficients (LFCCs) are spectral-based representations of entire signals and incorporate timbre (vocal “color”) as well as classical spectral parameters (Zhou et al., 2011). For details on feature extraction, please see Bergmann et al. (2022). Due to the different requirements for recording quality, another subset of social calls was used for the species identification described above than for the parameter measurements of social calls described in the previous section.
To assess the species identity of echolocation snippets surrounding social calls (i.e., the identity of the predominantly echolocating species in each recording), we performed a DFA in which the reference data set (with known species ID, 120 echolocation call sequences) functioned as training set and the echolocation snippets surrounding the social calls functioned as a test data set (854 echolocation snippets with unknown species ID) using the parameters spectral centroid, start frequency, peak frequency and mean and standard deviation of the LFCCs 1 and 3. For details on parameter selection, please see Bergmann et al. (2022).
Subsequently, we tested for species-specific differences in acoustic parameters of selected social calls by calculating a MANOVA with selected acoustic parameters as dependent variables and species ID, call type and their interaction as independent variables. The data set consisted of 57 social calls with sufficient quality for acoustic measurements (26 from M. daubentonii and 31 from M. nattereri) which had been previously classified to species level based on their surrounding echolocation calls. The social calls belonged to four different call types (FM pulses, U-shape, L-shape, inverted N-shape). We included eight acoustic parameters, namely duration, peak frequency at start, center and end of a call, and peak, minimum and maximum frequency as well as entropy averaged over the entire call.
Playback stimuli
For the playbacks, we selected three commonly used social calls of M. daubentonii and M. nattereri, respectively. The calls were recorded from swarming bats at the Kalkberg cave in 2018 and 2019 (inverted N-shape and U-shape call) or at the Spandau Citadel (squawks) from visually identified bats roosting in crevices. U-shape and inverted N-shape calls were classified to species level as described above. We trimmed the recordings close to the social calls and eliminated background noise or contemporaneously emitted echolocation calls. For the inverted N-shape call, the noise was reduced in Cool Edit 2000 (Syntrillium Software Corporation, Phoenix, AZ, USA) and silence was inserted around syllables until a total file length of 100 ms. After that, another noise reduction was conducted in Avisoft SASLabPro (FFT 1024; precision 4; removed noise below −70 dB; reduced noise by 80 dB) and remaining artifacts were erased manually, whenever necessary. For the U-shape calls the noise was reduced in Avisoft SASLabPro (FFT 1024; precision 4; removed noise below −60 dB; reduced noise by 30 dB), residual noise was erased manually and a second noise reduction was applied whenever necessary. The squawks did not require noise reduction, as they were recorded from bats in crevices and not in a swarming context. Thus, neither noise nor echolocation calls were present in those recordings, and the files were trimmed close to the social calls. For each final playback file of 30 s length, 15 calls, randomly drawn from the library of playback stimuli, were compiled in random order intermitted by silence in Cool Edit 2000. For the inverted N-shape calls, the library of high-quality playback stimuli consisted of 14 M. daubentonii and 11 M. nattereri calls, the U-shape of 9 M. daubentonii and 11 M. nattereri calls and the squawk files assembled 55 M. daubentonii and 42 M. nattereri calls.
Playback set up and analysis
We conducted the playbacks in 14 nights between 30.08.2020 and 15.09.2020 at three locations close to the entrances of the Kalkberg cave (Supplementary Figure 1). At each location, we observed swarming bats in former years. Each playback trial had a total duration of 90 s and consisted of a silent pre-observation, stimulus presentation and silent post-observation phase. Each phase was 30 s long, as social calls are often emitted in sequence and during this time passing bats have the chance to change their course and show phonotaxis behavior (Figure 2). To broadcast the stimuli we used a BatLure Ultrasound Speaker (Pettersson Elektronik AB, Uppsala, Sweden) mounted on a tripod and directed upward (Supplementary Figure 1 location B and C) or hanging at a wall and directed forward (Supplementary Figure 1 location A). Sound pressure levels of stimuli were kept constant (100 dB SPL at 1 m) during the playbacks and were lower than what is reported for echolocation calls of our focal species (Melcón et al., 2007; Jakobsen et al., 2013). In each night, playbacks of all call types were conducted at all locations in a randomized order between 10 p.m. and 2 a.m. In total, we conducted 40 playbacks consisting of six trials each (i.e., broadcasting three different call types from both species). All playbacks were started manually after three bats passed the video-recorded sector and ended automatically after 90 s.
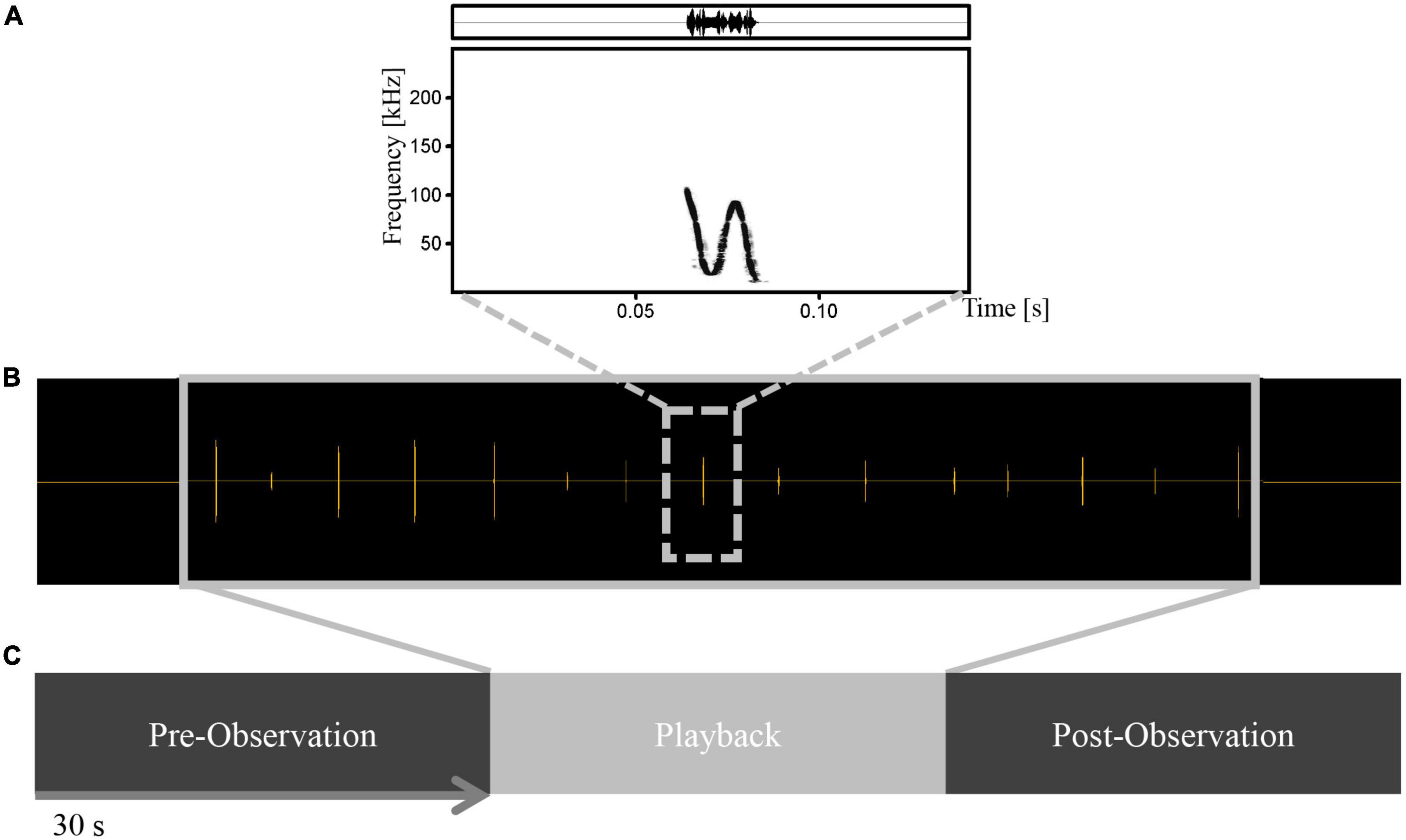
Figure 2. For each playback sequence, 15 social calls (A) were combined into 30-s-long playback files (B). One trial for each call type consisted of three experimental phases: Pre-observation, playback, and post-observation (C). Pre- and post-observation phase consisted of silence.
We recorded the vocal response of bats during playbacks using an ultrasonic microphone (similar set up as for sound recordings) pointed in the same direction as the speaker such that bat calls directed to the speaker would be highest in amplitude, facilitating discrimination between playback and corresponding bat activity at the playback site. The audio files were analyzed semi-automatically using a pulse train analysis in Avisoft SASLabPro (all echolocation calls that exceeded a threshold of −24 dB relative to the oscillogram’s maximum amplitude were counted). To interpret the bats’ behavior in response to the playbacks, we compared the number of emitted echolocation calls between the pre-observation phase and the mean number of calls emitted in the playback and post-observation phase. The number of emitted echolocation calls depend on both the number of bats and their calling rate. We used a binomial test, separately for each of the six combinations of stimulus type and species ID. All statistical tests were conducted using SPSS (version 28, SPSS Inc., Chicago, IL, U.S.A.).
In addition, we observed bat flight behavior using a thermal camera (FLIR E95, FLIR Systems, Wilsonville, OR, US) directed at the speaker at a distance of approximately 6 m. Whenever an approaching bat was visible on the thermal camera’s screen, we took a photo by triggering a remote-control release, which operated a camera (Nikon D3S, Nikon Corporation, Tokyo, Japan). A set of seven flashlights (Yongnuo YN560, Shenzhen Yong Nuo Photographic Equipment, Shenzen, China) was assembled around the speaker and triggered via slave function from another flash light on top of the camera to illuminate approaching bats. We identified the photographed bats to species level based on the characteristics of their wings, ears and/or tails. However, not all approaching and passing bats could be photographed and not all of the photographed bats could be identified to species level. Nevertheless, the photo set-up is a good non-invasive approach to complement other playback results as it allowed us to get a rough estimate of the species-specificity of social calls without having to catch approaching individuals.
Results
Ten call types were produced by swarming bats
We analyzed 2,135 recordings containing one or more social calls and identified ten call types emitted during swarming (Figure 3, for original recordings see Supplementary Audio 1). We grouped the calls into types based on their spectro-temporal structure (Table 1) and confirmed our grouping by a DFA, which classified 77.4% of all calls to the correct type (Figure 4, for detailed DFA results see Table 2).
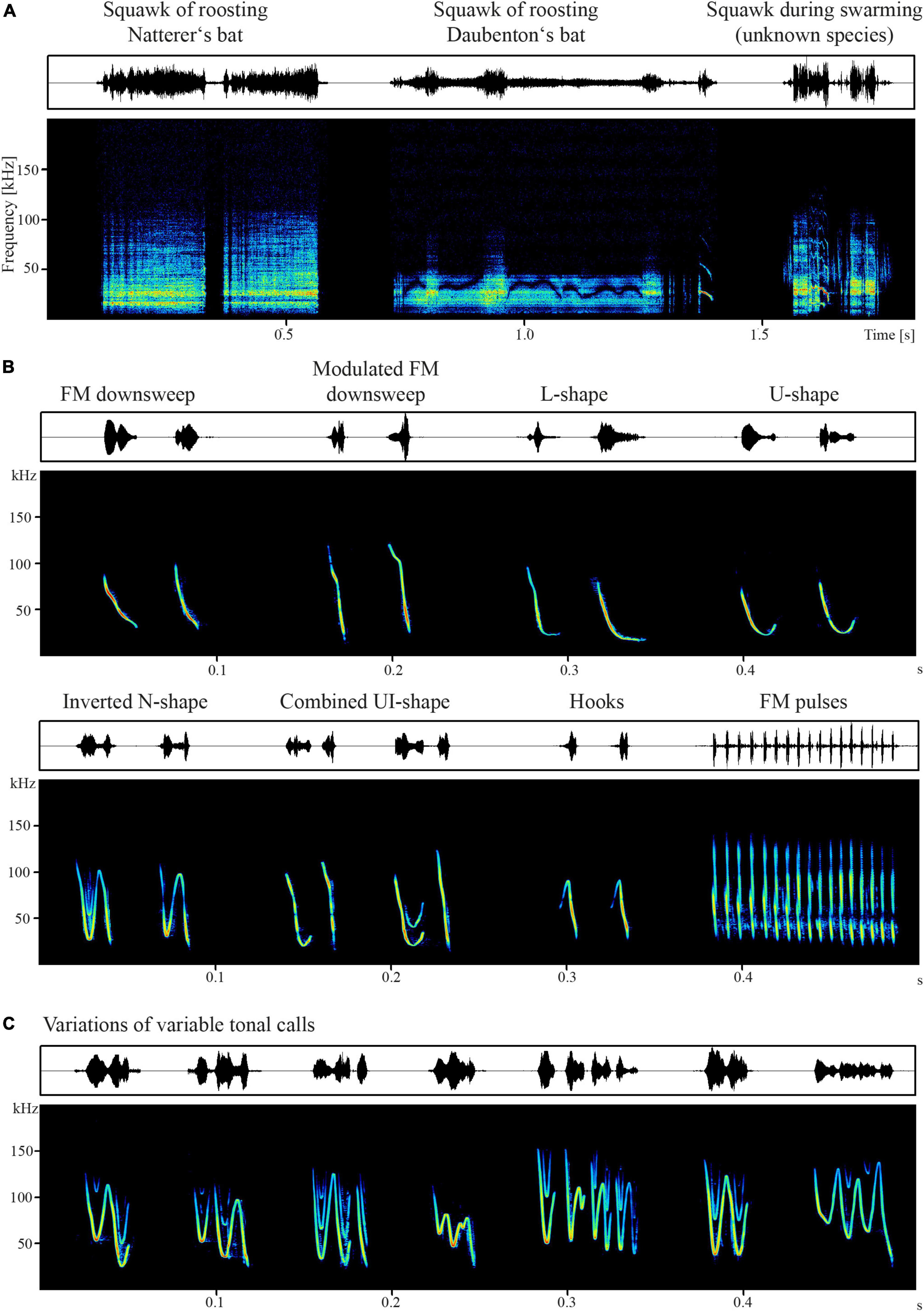
Figure 3. Spectrograms of all call types we found during autumn swarming. The calls were grouped based on their spectro-temporal structure and confirmed through a discriminant function analysis with temporal and spectral parameters. (A) Squawks recorded at the Spandau Citadel from identified bats in crevices (left and middle) and from an unidentified bat during autumn swarming at the Kalkberg cave (right). (B) Short tonal calls emitted singly or in sequence. (C) Variable tonal calls grouped into one group, usually longer than other tonal calls and often emitted in sequence. Spectrograms were created using Avisoft SASLabPro with a Hamming window, 100% frame size and an overlap of 87.5% (B,C) or 50% (A).
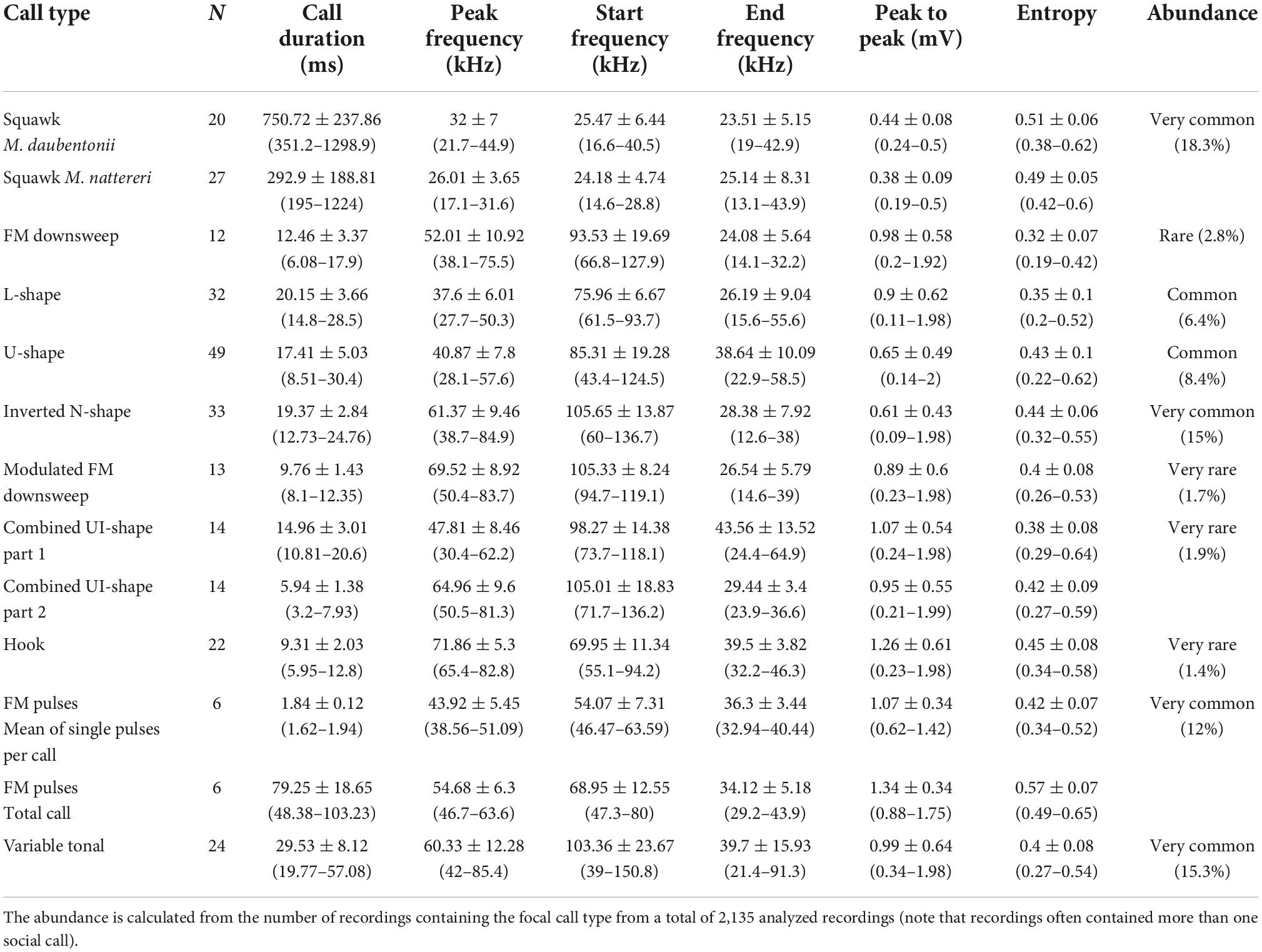
Table 1. Mean and standard deviation of social call parameters per call type (range is given in parentheses).
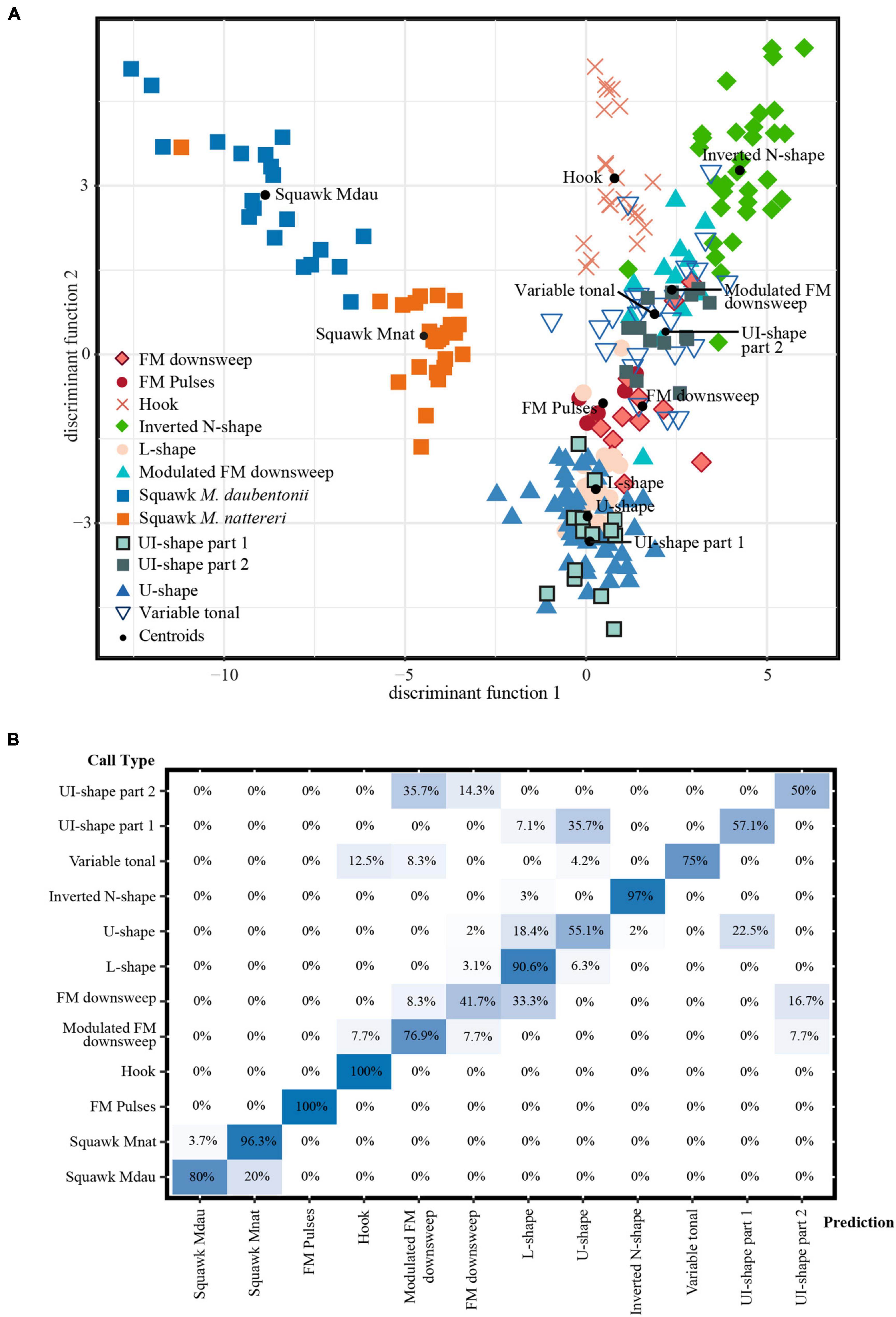
Figure 4. (A) Relative position of social calls produced by swarming Myotis bats based on their spectral and temporal parameters. The two-dimensional signal space is defined by the first two discriminant functions, which were most important for call type discrimination. The ten call types are represented by different symbols; black circles depict centroids and are labeled with the respective call type. One call type, the squawk, was further discriminated by species because squawks were recorded from identified bats in crevices. All other calls were recorded from bats on the wing. One of those in-flight social calls, the combined UI-shape consisted of two different parts which were entered separately into the DFA. (B) Confusion Matrix indicating the call types to which analyzed calls were assigned. 77.4% of cross-validated cases could be classified correctly. Mdau, Myotis daubentonii; Mnat, Myotis nattereri.
The longest social calls produced at the swarming site were squawks, atonal harsh screeches of constant low frequency, which were emitted frequently not only in-flight but also from individuals roosting in crevices in close vicinity of the swarming bats (Figure 3). Squawks recorded from roosting M. daubentonii and M. nattereri had clear species-specific acoustic differences (Figure 4) but these differences could not be analyzed for squawks produced by bats in a swarming context; squawks were often emitted from crevices near the swarming bats and as roosting bats do not echolocate the classification of echolocation calls would be misleading. Furthermore, we recorded a variety of frequency-modulated tonal calls which we assigned to nine distinct call types based on their spectro-temporal structure. Four call types (FM downsweep, modulated FM downsweep, L-shape and U-shape) were comparatively similar but could nevertheless be grouped into distinct call types by the DFA. Four other call types (inverted N-shape, combined UI-shape, hook, FM pulses) differed in their spectro-temporal structure to a greater degree (Figure 3) and were thus classified better by the DFA (Figure 4). Combined UI-shape and FM pulses were the only two multisyllabic calls produced by swarming bats. Inverted N-shape and hook were easy to recognize call types because they showed very little variation. The remaining call type (variable tonal call) was a broad call type category that encompassed all tonal calls with a high variability in frequency modulation and a wider range of start and end frequencies. Even though this was a very common call type, the different calls were too variable to group into meaningful subtypes. Variable tonal calls and inverted N-shape calls were easy to differentiate despite their similarity at first sight because inverted N-shape calls had very regular frequency modulations. Due to the high activity at the swarming site, we were rarely able to make a connection between a call and the associated behavior of the caller. Nevertheless, on some occasions, we could observe the FM pulses being emitted when swarming bats collided or got very close to each other. It is therefore possible that FM pulses are used to maintain or negotiate the distance between swarming individuals.
Both species employed comparatively similar calls
Based on the surrounding echolocation calls we assigned a total of 305 social calls to either M. daubentonii or M. nattereri. Out of 854 analyzed echolocation snippets surrounding a social call, we could classify 760 to species level with a classification probability of more than 90% (DFA: Training N = 120, Test N = 854, Eigenvalue = 12.225, explained variation = 100%, Wilk’s λ = 0.076, χ2 = 295.648, p < 0.0001). A total of 150 of 760 snippets were discarded because the two snippets surrounding a social call were not assigned to the same species, thus making the classification ambiguous. The remaining 610 echolocation snippets allowed us to classify 305 social calls to species level (M. daubentonii or M. nattereri). Further, some social calls were discarded from analysis because they could not be assigned unequivocally to one of the ten formerly defined call types (51 calls). Only few FM downsweeps (6 calls), modulated FM downsweeps (5 calls) and hooks (1 call) were classified due to low number of recordings, unsuitable echolocation snippets or/and based on the classification constraints. Additionally, we discarded 34 variable tonal calls from further analysis as they were often emitted in long sequences and frequently interrupted the surrounding echolocation snippets. Also the 49 squawks were discarded because their species ID could not be reliably established based on the surrounding echolocation call soundscape (squawks were often emitted by roosting bats near the swarming area which did not echolocate). Of the analyzed inverted N-shape (29 calls) and L-shape (36 calls) calls, around half was classified as M. daubentonii, respectively (Figure 5). Two-thirds of the U-shape calls (65 calls in total) and around 80% of FM pulses (29 calls in total) were classified as M. daubentonii.
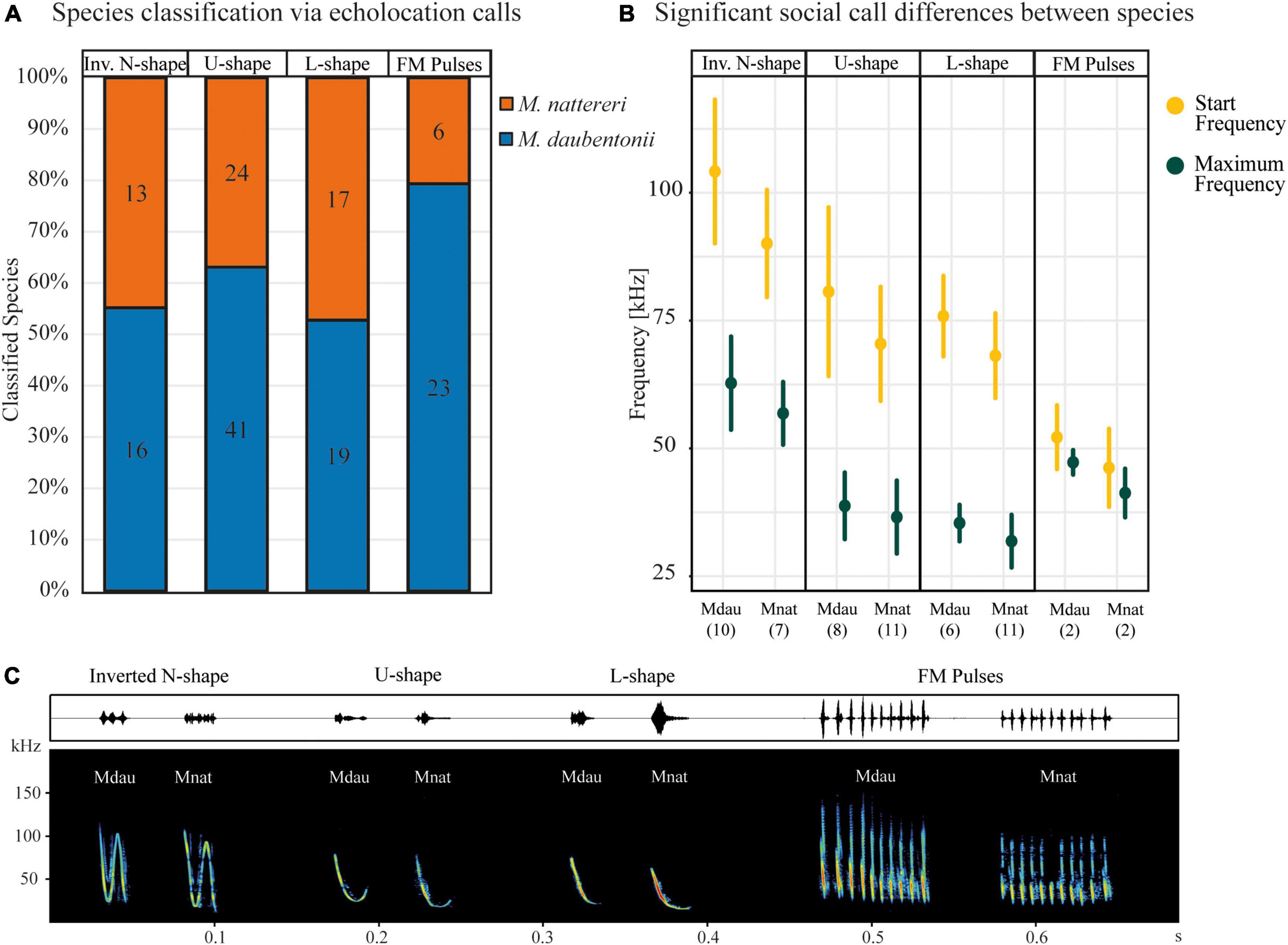
Figure 5. (A) Number and proportion of classified species based on the classification of echolocation snippets surrounding a social call. The total number is given within the columns. (B) For the classified calls, start frequency and maximum frequency (averaged over the entire call) differed significantly between species. Mean and standard deviation per species are depicted in the graph, number of analyzed calls per species is given in parentheses. (C) Examples of calls per species based on the classification results. Mdau, Myotis daubentonii; Mnat, Myotis nattereri.
In the data set of Wimmer and Kugelschafter (2015) we found FM Pulses, variable tonal calls and FM downsweeps emitted by both species in correspondence with the calls recorded during swarming (Supplementary Table 1). Furthermore, they recorded hooks of a lower frequency than ours from both species and squawks and L-shape calls of M. daubentonii and inverted N-shape calls of M. nattereri only.
Additionally, the acoustic properties of four social call types (inverted N-shape, U-shape, L-shape, FM pulses; only calls with sufficient quality for acoustic measurements were included) differed significantly between species and between call types (MANOVA; species ID: F8, 42 = 4.686, p < 0.001, partial η2 = 0.472; call type: F24, 122.4 = 21.694, p < 0.001, partial η2 = 0.799; species ID*call type: F24, 122.4 = 1.570, p = 0.059, partial η2 = 0.229). Two acoustic parameters differed significantly between species (between-subjects effects; peak frequency at the start of a call: p = 0.017; maximum frequency averaged over the entire call: p = 0.47) and seven acoustic parameters differed significantly between call types (between-subjects effects; all p < 0.001, except for entropy averaged over the entire call). When comparing species-specific properties within the same call type, calls classified as M. daubentonii had higher frequencies than calls classified as M. nattereri (Figure 5), even though it is the opposite for the species’ echolocation calls. These results indicate that both species employ social calls that are rather similar in their spectro-temporal structure.
The reaction to playbacks differed between call types
To investigate the reaction of free ranging bats to different social call types, we conducted playbacks (40 playbacks with six trials each) and broadcasted the inverted N-shape, the U-shape and the squawk call of M. daubentonii and M. nattereri, respectively. We tested whether bats reacted to the broadcasted calls with a higher rate of echolocation calls, which would suggest increased interest in the playback location, or even with phonotaxis. To do so, we analyzed all echolocation calls we recorded during the playbacks regardless of species specificity.
When U-shape calls were broadcasted, the echolocation call rate was significantly higher during playback and post-playback phase in comparison to the pre-playback for both species (Figure 6A, Binomial test; M. daubentonii: p = 0.04, M. nattereri: p = 0.019). For the inverted N-shape calls, calls of neither species led to an increased echolocation call rate (Binomial test; M. daubentonii: p = 0.215, M. nattereri: p = 0.563). The squawks from M. daubentonii triggered an increased echolocation call rate but the squawks of M. nattereri did not (Binomial test; M. daubentonii: p = 0.003, M. nattereri: p = 0.563).
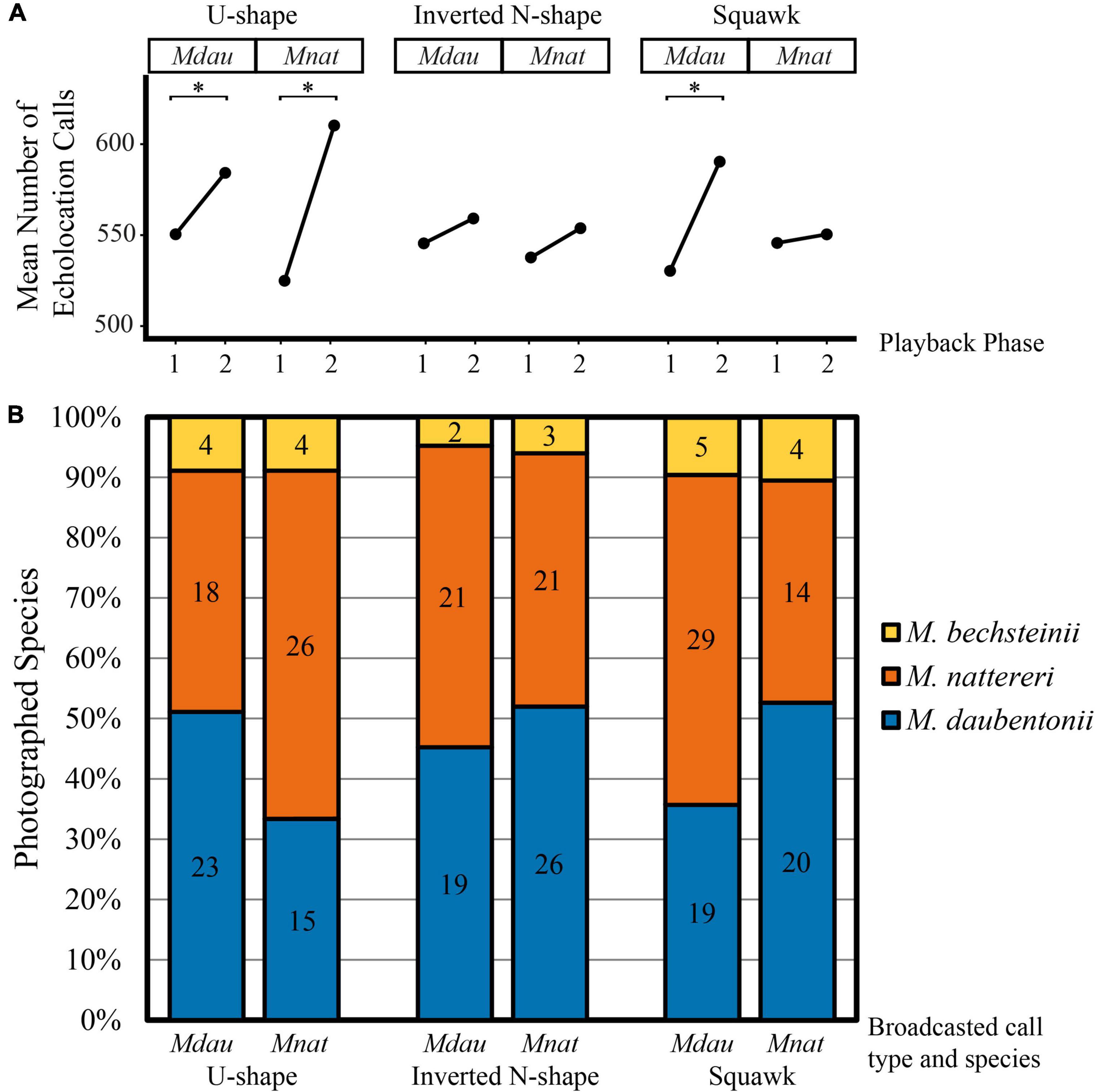
Figure 6. (A) Mean number of echolocation pulses recorded during the playbacks: Phase 1 is the pre-playback phase, phase 2 is the mean from playback and post-playback phase. When U-shaped calls of both species, respectively, and squawks of Myotis daubentonii were broadcasted, the mean number of echolocation pulses increased significantly (* marked by an asterisk, Binomial test). (B) Numbers and proportions of visually identified bat species approaching the speaker during playbacks of the different social call types. Species were identified from photos taken during playback and post-observation phase. Mdau, Myotis daubentonii; Mnat, Myotis nattereri.
During the playback and post-playback phase, we additionally photographed the passing or approaching bats and could identify individuals to species level in 273 photos (see Figure 7 for details of species identification). We were able to not only identify M. daubentonii and M. nattereri on the photos but also a much rarer bat species, M. bechsteinii (Figure 6B). While the bats’ reaction to broadcasted U-shape calls was slightly species-specific but far from exclusive, the bats’ reaction to broadcasted inverted N-shaped calls was not species-specific at all. Interestingly, when we broadcasted squawks, we took more photos of approaching heterospecific bats than of conspecifics. Based on these findings and with regard to former descriptions of the calls we suggest U-shape calls to assist in group cohesion, while low frequency squawks emitted in an aggressive context might relay roost location to passing bats and the N-shape calls (which are often emitted in combination with more complex variable tonal calls) could play a role in context of mating.
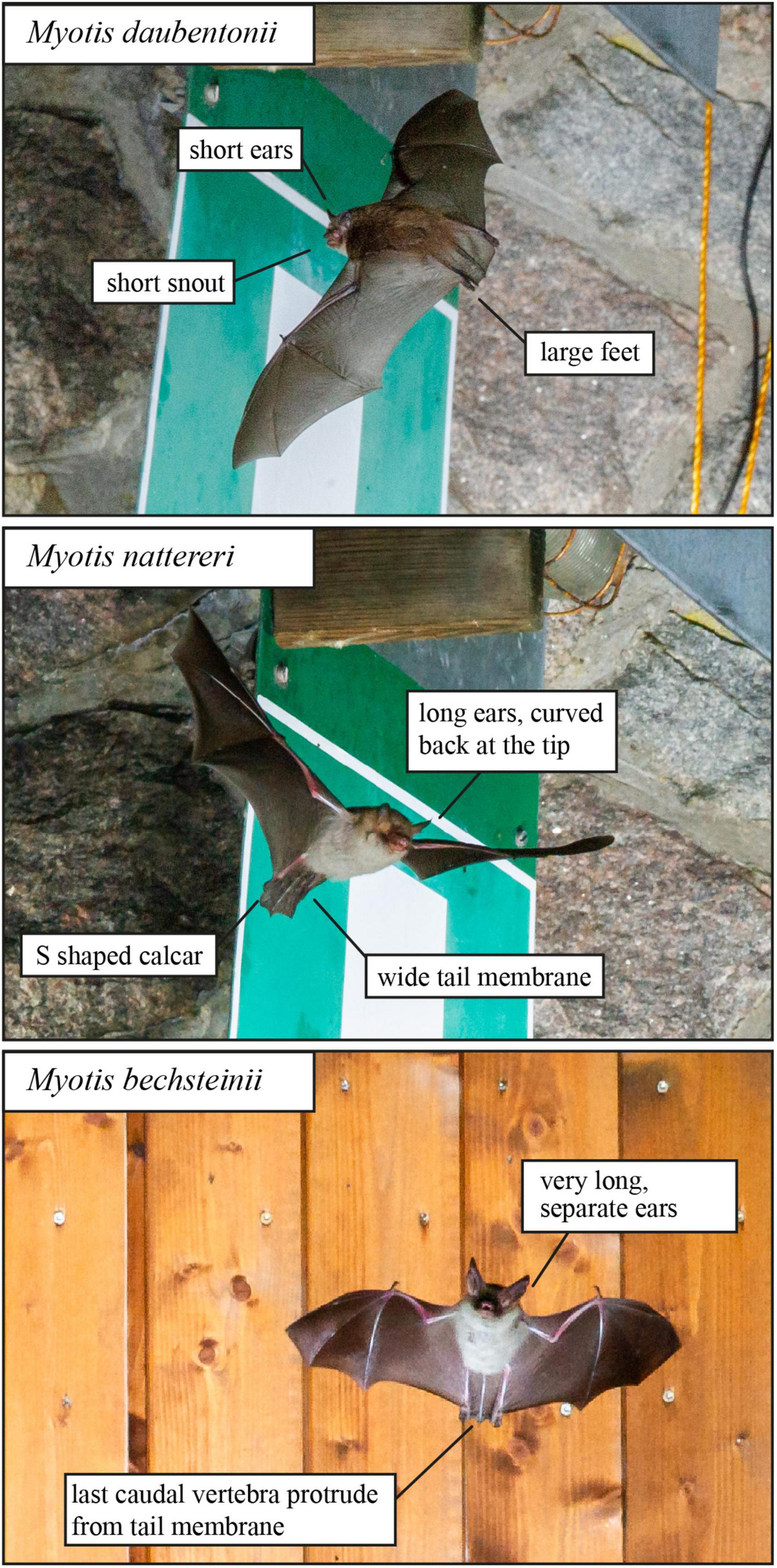
Figure 7. Examples of the three species which were photographed during the playbacks. Features used for species identification are labeled accordingly.
Discussion
By observing a shared swarming site over a period of many nights in two consecutive swarming seasons we could document a broader variety of social calls from two species of Myotis bats than described in a swarming context before. Based on their spectro-temporal structure we grouped the calls into ten distinct types and found evidence that some call types are produced by both observed species, M. daubentonii and M. nattereri. With regard to the playback results we assume that some of the calls facilitate interspecific communication while others are employed for intraspecific communication.
Pfalzer and Kusch (2003) described a variety of social calls from Vespertilionid bats in different contexts and organized them into four groups based on structure and function. Squawk-like, noisy calls were mostly observed in agonistic contexts (type A), repetitive trills were produced under distress (type B), cheep-like or curve-structured single pulses were used for mother-pup interaction or group cohesion (type C) and song-like, complex structure and multiple frequency modulated elements were produced for mate attraction or in a territorial context (type D). We observed all such call types during autumn swarming, indicating various functions of swarming (see Supplementary Table 1 for former classification of described call types).
We recorded a relatively high number of squawks, not only from crevices near the entrances to the hibernaculum, but also from swarming bats. Such calls are thought to be used aggressively or in a threatening context and were frequently recorded from captured bats or in association with roosting situations before (Middleton et al., 2014). Due to their long duration and low frequency, squawks have the potential to be audible over long distances. During the playback experiments, the squawks of Daubenton’s bats but not Natterer’s bats led to a significantly higher calling rate of passing bats, which might be caused by the longer duration of the Daubenton’s bats’ broadcasted squawks. However, the photos made during the experiment suggest that the reaction might not be species-specific as individuals of both species, M. daubentonii and M. nattereri, were approaching the speaker during and after the playback phase. Emitted during swarming, squawks should be well audible to passing bats and may serve to relay the location of the swarming site and thus the hibernaculum. Thus, we assume the calls to function as a cue for hibernacula and both heterospecifics and conspecifics tend to approach emitted squawk calls. As both species have comparatively similar hibernacula preferences and often hibernate in mixed-species groups, it is conceivable that squawks can facilitate interspecific eavesdropping to find suitable crevices.
Another commonly observed call type were FM pulses, which consist of a series of frequency-modulated, downward-sweeping elements of short duration and were also assigned to both species. Such calls are often observed in situations of distress (Middleton et al., 2014) and might work for intraspecific (Russ et al., 1998) but also interspecific communication during which heterospecifics can be attracted by distress calls to elicit a mobbing response to repel predators (Russ et al., 2004). During autumn swarming, we observed such calls being emitted in flight when bats were almost or actually colliding with other swarming bats. Such situations do not require species-specificity as both con- and heterospecifics might be the receiver of the call.
The high number of variable tonal calls we recorded corresponds well to the calls Pfalzer and Kusch (2003) described as Type D. Also Schmidbauer and Denzinger (2019) found such highly variable calls and assumed that those longer trills are closely linked to mating behavior as they were emitted in high numbers at an autumn swarming site but not at a maternity roost and both species are known to mate at autumn swarming sites (Encarnação et al., 2004; Pfeiffer and Mayer, 2013). Furthermore such comparatively long calls potentially enhance the signal efficacy and detectability in contrast to shorter calls (Morton, 1986). In consideration of their high variability and frequent repetition, we concur with Schmidbauer and Denzinger (2019) that the variable tonal calls may be produced in the context of courtship and mating.
Even though structural similarities can be seen between some variable tonal calls and the inverted N-shape calls, the second are characterized by very regular frequency modulations. Our inverted N-shape calls coincide with call type C Schmidbauer and Denzinger (2019) recorded from Natterer’s bats and probably also with the V-shaped call Pfalzer (2002) described, although he thought them to consist of two elements. We analyzed the surrounding echolocation calls and our results strongly suggest that the inverted N-shape calls, like various call types, are employed by both M. daubentonii and M. nattereri. To our knowledge, the inverted N-shape call was so far only ascribed to Natterer’s bats. However, to avoid errors, species identification solely based on the appearance of inverted N-shape calls will need some further investigation in the future. Although the calls did not lead to a change in echolocation calling rate during playback experiments, with regard to the structural similarities of the variable tonal calls we suggest them to be also mating related and maybe work in combination with variable tonal calls, as such calls were often emitted in rows.
Another common call type were U-shape calls, which are apparently also produced by both M. daubentonii and M. nattereri. Similar calls have been described in various situations so far and might be associated with tandem flights or group cohesion and coordination (Middleton et al., 2014). When broadcasted in playback experiments, these calls caused an increased echolocation call rate, suggesting phonotaxis or heightened interest in the playback area which corresponds well with the assumption of group cohesion as a function for U-shape calls. It is important to note that we recorded a high number of calls which could be placed on a continuum between the L- and U-shape calls. It was nevertheless possible to group them into two call types based on the differences at the calls’ end but there was large acoustic overlap. Large overlaps were furthermore present between the U-shape and the first part of the combined UI-shape calls, which is not surprising as the first part is also U-shaped. However, the combined UI-shape call is characterized by the combination of two parts and has an additional second part which is rather similar to the modulated FM downsweep. Schmidbauer and Denzinger (2019) were the first who described such calls frequently at an autumn swarming site and a maternity roost of Natterer’s bats and suggested them to function as contact calls.
Some further call types were recorded rather rarely, among them a very low number of modulated FM downsweeps, which coincides with the observation of Schmidbauer and Denzinger (2019) that those calls were much more abundant at a summer roost than at an autumn swarming site. Furthermore low numbers of FM downsweeps without modulation, and one call type (hook) that has not been described before. However, these call types were very rare and we have too little information to make assumptions about their function.
We are aware of the constraint of the species identification via surrounding echolocation calls. The social calls are often louder and audible over broader distances, while echolocation calls are stronger attenuated (Pfalzer and Kusch, 2003; Middleton et al., 2014; Chaverri et al., 2018), making the parallel recording of echolocation and social call difficult, especially in such a crowded swarming situation. Furthermore, an individual not belonging to the predominant species at time of recording might emit the social call and thus lead to ascribing the social call to the wrong species based on the classification result. Nevertheless, significant differences in call parameters between the assigned species were present indicating that both species employ calls of a very similar structure. Based on this, classification via social calls alone should be conducted with great care and other parameters for species identification should be taken into account. This is especially the case for Natterer’s bats which are often identified solely based on abundance of the inverted N-shape call even though our results indicate that M. daubentonii can also produce inverted N-shape calls.
Overall, the observed Myotis bats emitted a broad variety of social calls during autumn swarming. Noisy squawk calls seem to have an interspecifically attracting function to passing bats, while U-shape calls might facilitate group cohesion intraspecifically. For other calls we could not elucidate their function during swarming and given the great variety of social calls we could not cover the full repertoire in our playback experiments. Thus, recordings and more playbacks of various call types on and near swarming sites (ideally those used by only one bat species at a time) will be necessary to get further insights regarding species-specificity and call function. Nevertheless, with our work we provide a comprehensive description of the call repertoire at a shared autumn swarming site and thus make an important contribution to the knowledge about swarming and especially the use of social calls in free ranging Myotis bats.
Data availability statement
The raw data supporting the conclusions of this article will be made available by the authors, without undue reservation.
Ethics statement
This animal study was reviewed and approved by the Internal Ethics Committee of the Landesamt für Landwirtschaft, Umwelt und ländliche Räume Schleswig-Holstein under license LLUR_521_20180703.
Author contributions
AB, FG-R, and MK conceived and planned the study and analyzed the data. AB, BW, and KK collected the data. MK extracted acoustic parameters. AB and MK carried out the statistical analyses. AB wrote the first draft. All authors provided the feedback and guidance.
Funding
This work was supported by a stipend from the Elsa-Neumann Foundation to AB. Open access funding was provided by the Open Access Publication Initiative of the Free University of Berlin.
Acknowledgments
We thank the team of Noctalis GmbH and Matthias Göttsche for access to the Kalkberg cave and their logistic support and Juliane Lukas and Sebastian Bergmann for companionship during sound recordings and assistance during playback experiments at the Kalkerg. Moreover, we are grateful to Jörg Harder from BAT e.V. for giving us access to the bats at the Spandau Citadel. This study is part of the research cluster “Sound of the Anthropocene” based at the Museum für Naturkunde in Berlin, Germany.
Conflict of interest
Author KK was employed by ChiroTEC.
The remaining authors declare that the research was conducted in the absence of any commercial or financial relationships that could be construed as a potential conflict of interest.
Publisher’s note
All claims expressed in this article are solely those of the authors and do not necessarily represent those of their affiliated organizations, or those of the publisher, the editors and the reviewers. Any product that may be evaluated in this article, or claim that may be made by its manufacturer, is not guaranteed or endorsed by the publisher.
Supplementary material
The Supplementary Material for this article can be found online at: https://www.frontiersin.org/articles/10.3389/fevo.2022.950951/full#supplementary-material
References
Ancillotto, L., Pafundi, D., Cappa, F., Chaverri, G., Gamba, M., Cervo, R., et al. (2022). Bats mimic hymenopteran insect sounds to deter predators. Curr. Biol. 32, R408–R409. doi: 10.1016/j.cub.2022.03.052
Arnold, B. D., and Wilkinson, G. S. (2011). Individual specific contact calls of pallid bats (Antrozous pallidus) attract conspecifics at roosting sites. Behav. Ecol. Sociobiol. 65, 1581–1593. doi: 10.1007/s00265-011-1168-4
Barclay, R. M. R., and Thomas, D. W. (1979). Copulation call of Myotis lucifugus: A discrete situation-specific communication signal. J. Mammal. 60, 632–634. doi: 10.2307/1380109
Barlow, K. E., and Jones, G. (1997). Function of pipistrelle social calls: Field data and a playback experiment. Anim. Behav. 53, 991–999. doi: 10.1006/anbe.1996.0398
Behr, O., and von Helversen, O. (2004). Bat serenades–complex courtship songs of the sac-winged bat (Saccopteryx bilineata). Behav. Ecol. Sociobiol. 56, 106–115. doi: 10.1007/s00265-004-0768-7
Bergmann, A., Burchardt, L. S., Wimmer, B., Kugelschafter, K., Gloza-Rausch, F., and Knörnschild, M. (2022). The soundscape of swarming: Proof of concept for a noninvasive acoustic species identification of swarming Myotis bats. Ecol. Evol. 12:e9439. doi: 10.1002/ece3.9439
Bohn, K. M., and Gillam, E. H. (2018). In-flight social calls: A primer for biologists and managers studying echolocation. Can. J. Zool. 96, 787–800. doi: 10.1139/cjz-2017-0188
Bohn, K. M., Wilkinson, G. S., and Moss, C. F. (2007). Discrimination of infant isolation calls by female greater spear-nosed bats, Phyllostomus hastatus. Anim. Behav. 73, 423–432. doi: 10.1016/j.anbehav.2006.09.003
Bosia, T., Villalobos, F., and Schmidt, S. (2022). Evidence for vocal diversity during physical interference at the perch in sympatric Carollia species (Chiroptera: Phyllostomidae): A key to social organization and species coexistence? Zool. J. Linn. Soc. 194, 457–477. doi: 10.1093/zoolinnean/zlab040
Bradbury, J. W., and Vehrencamp, S. L. (2011). Principles of animal communication, Second Edn. Sunderland: Sinauer Associates.
Briefer, E. F. (2012). Vocal expression of emotions in mammals: Mechanisms of production and evidence. J. Zool. 288, 1–20. doi: 10.1111/j.1469-7998.2012.00920.x
Burns, L. E., and Broders, H. G. (2015). Maximizing mating opportunities: Higher autumn swarming activity in male versus female Myotis bats. J. Mammal. 96, 1326–1336. doi: 10.1093/jmammal/gyv141
Carlson, N. V., Kelly, E. M., and Couzin, I. (2020). Individual vocal recognition across taxa: A review of the literature and a look into the future. Philos. Trans. R. Soc. B 375:20190479. doi: 10.1098/rstb.2019.0479
Carter, G., Schoeppler, D., Manthey, M., Knörnschild, M., and Denzinger, A. (2015). Distress calls of a fast-flying bat (Molossus molossus) provoke inspection flights but not cooperative mobbing. PLoS One 10:e0136146. doi: 10.1371/journal.pone.0136146
Chaverri, G., Ancillotto, L., and Russo, D. (2018). Social communication in bats. Biol. Rev. 93, 1938–1954. doi: 10.1111/brv.12427
Chaverri, G., Gillam, E. H., and Vonhof, M. J. (2010). Social calls used by a leaf-roosting bat to signal location. Biol. Lett. 6, 441–444. doi: 10.1098/rsbl.2009.0964
Dorado-Correa, A. M., Goerlitz, H. R., and Siemers, B. M. (2013). Interspecific acoustic recognition in two European bat communities. Front. Physiol. 4:192. doi: 10.3389/fphys.2013.00192
Encarnação, J. A., Dietz, M., and Kierdorf, U. (2004). Reproductive condition and activity pattern of male Daubenton’s bats (Myotis daubentonii) in the summer habitat. Mamm. Biol. 69, 163–172. doi: 10.1078/1616-5047-00131
Fenton, M. (1994). Assessing signal variability and reliability: ‘To thine ownself be true’. Anim. Behav. 47, 757–764. doi: 10.1006/anbe.1994.1108
Fenton, M. B. (1969). Summer activity of Myotis lucifugus (Chiroptera: Vespertilionidae) at hibernacula in Ontario and Quebec. Can. J. Zool. 47, 597–602.
Fenton, M. B. (1984). Echolocation: Implications for ecology and evolution of bats. Q. Rev. Biol. 59, 33–53. doi: 10.1086/413674
Fenton, M. B., Belwood, J. J., Fullard, J. H., and Kunz, T. H. (1976). Responses of Myotis lucifugus (Chiroptera: Vespertilionidae) to calls of conspecifics and to other sounds. Can. J. Zool. 54, 1443–1448. doi: 10.1139/z76-167
Furmankiewicz, J., Duma, K., Manias, K., and Borowiec, M. (2013). Reproductive status and vocalisation in swarming bats indicate a mating function of swarming and an extended mating period in Plecotus auritus. Acta Chiropterol. 15, 371–385. doi: 10.3161/150811013X678991
Furmankiewicz, J., Ruczyński, I., Urban, R., and Jones, G. (2011). Social calls provide tree-dwelling bats with information about the location of conspecifics at roosts. Ethology 117, 480–489. doi: 10.1111/j.1439-0310.2011.01897.x
Hechavarría, J. C., Jerome Beetz, M., García-Rosales, F., and Kössl, M. (2020). Bats distress vocalizations carry fast amplitude modulations that could represent an acoustic correlate of roughness. Sci. Rep. 10:7332. doi: 10.1038/s41598-020-64323-7
Hörmann, D., Tschapka, M., Rose, A., and Knörnschild, M. (2021). Distress calls of nectarivorous bats (Glossophaga soricina) encode individual and species identity. Bioacoustics 30, 253–271. doi: 10.1080/09524622.2020.1720815
Jakobsen, L., Brinkløv, S., and Surlykke, A. (2013). Intensity and directionality of bat echolocation signals. Front. Physiol. 4:89. doi: 10.3389/fphys.2013.00089
Jameson, J. W., and Hare, J. F. (2009). Group-specific signatures in the echolocation calls of female little brown bats (Myotis lucifugus) are not an artefact of clutter at the roost entrance. Acta Chiropterol. 11, 163–172. doi: 10.3161/150811009X465785
Jones, G., Gordon, T., and Nightingale, J. (1992). Sex and age differences in the echolocation calls of the lesser horseshoe bat, Rhinolophus hipposideros. Mammalia 56, 189–194. doi: 10.1515/mamm-1992-0202
Kazial, K. A., Kenny, T. L., and Burnett, S. C. (2008). Little brown bats (Myotis lucifugus) recognize individual identity of conspecifics using sonar calls. Ethology 114, 469–478. doi: 10.1111/j.1439-0310.2008.01483.x
Kerth, G., Kiefer, A., Trappmann, C., and Weishaar, M. (2003). High gene diversity at swarming sites suggest hot spots for gene flow in the Endangered Bechstein’s bat. Conserv. Genet. 4, 491–499. doi: 10.1023/A:1024771713152
Knörnschild, M., Feifel, M., and Kalko, E. K. (2013). Mother–offspring recognition in the bat Carollia perspicillata. Anim. Behav. 86, 941–948. doi: 10.1016/j.anbehav.2013.08.011
Knörnschild, M., Feifel, M., and Kalko, E. K. (2014). Male courtship displays and vocal communication in the polygynous bat Carollia perspicillata. Behaviour 151, 781–798. doi: 10.1163/1568539X-00003171
Knörnschild, M., Glöckner, V., and von Helversen, O. (2010). The vocal repertoire of two sympatric species of nectar-feeding bats (Glossophaga soricina and G. commissarisi). Acta Chiropterol. 12, 205–215. doi: 10.3161/150811010X504707
Knörnschild, M., Jung, K., Nagy, M., Metz, M., and Kalko, E. (2012). Bat echolocation calls facilitate social communication. Proc. R. Soc. B 279, 4827–4835. doi: 10.1098/rspb.2012.1995
Lea, A. J., Barrera, J. P., Tom, L. M., and Blumstein, D. T. (2008). Heterospecific eavesdropping in a nonsocial species. Behav. Ecol. 19, 1041–1046. doi: 10.1093/beheco/arn064
Magrath, R. D., Haff, T. M., Fallow, P. M., and Radford, A. N. (2015). Eavesdropping on heterospecific alarm calls: From mechanisms to consequences. Biol. Rev. 90, 560–586. doi: 10.1111/brv.12122
Masters, W., Raver, K. A., and Kazial, K. A. (1995). Sonar signals of big brown bats, Eptesicus fuscus, contain information about individual identity, age and family affiliation. Anim. Behav. 50, 1243–1260. doi: 10.1016/0003-3472(95)80041-7
Melcón, M. L., Denzinger, A., and Schnitzler, H.-U. (2007). Aerial hawking and landing: Approach behaviour in Natterer’s bats, Myotis nattereri (Kuhl 1818). J. Exp. Biol. 210(Pt 24), 4457–4464. doi: 10.1242/jeb.007435
Middleton, N., Froud, A., and French, K. (2014). Social calls of the bats of Britain and Ireland. Exeter: Pelagic Publishing.
Mönkkönen, M., and Forsman, J. T. (2002). Heterospecific attraction among forest birds: A review. Ornithol. Sci. 1, 41–51. doi: 10.2326/osj.1.41
Morton, E. S. (1986). Predictions from the ranging hypothesis for the evolution of long distance signals in birds. Behaviour 99, 65–86. doi: 10.1163/156853986X00414
Neuweiler, G. (2003). Evolutionary aspects of bat echolocation. J. Comp. Physiol. A 189, 245–256. doi: 10.1007/s00359-003-0406-2
Oda, R., and Masataka, N. (1996). Interspecific responses of ringtailed lemurs to playback of antipredator alarm calls given by verreaux’s sifakas. Ethology 102, 441–453. doi: 10.1111/j.1439-0310.1996.tb01138.x
Ord, T. J., and Stamps, J. A. (2009). Species identity cues in animal communication. Am. Nat. 174, 585–593. doi: 10.1086/605372
Parsons, K. N., Jones, G., and Greenaway, F. (2003). Swarming activity of temperate zone microchiropteran bats: Effects of season, time of night and weather conditions. J. Zool. 261, 257–264. doi: 10.1017/S0952836903004199
Pfalzer, G. (2002). Inter- und intraspezifische Variabilität der Soziallaute heimischer Fledermausarten (Chiroptera: Vespertilionidae). Berlin: Mensch & Buch Verlag.
Pfalzer, G., and Kusch, J. (2003). Structure and variability of bat social calls: Implications for specificity and individual recognition. J. Zool. 261, 21–33. doi: 10.1017/S0952836903003935
Pfeiffer, B., and Mayer, F. (2013). Spermatogenesis, sperm storage and reproductive timing in bats. J. Zool. 289, 77–85. doi: 10.1111/j.1469-7998.2012.00970.x
Podos, J., and Warren, P. S. (2007). The evolution of geographic variation in birdsong. Adv. Study Behav. 37, 403–458. doi: 10.1016/S0065-3454(07)37009-5
Rendall, D., Owren, M. J., and Ryan, M. J. (2009). What do animal signals mean? Anim. Behav. 78, 233–240. doi: 10.1016/j.anbehav.2009.06.007
Rivers, N. M., Butlin, R. K., and Altringham, J. D. (2005). Genetic population structure of Natterer’s bats explained by mating at swarming sites and philopatry. Mol. Ecol. 14, 4299–4312. doi: 10.1111/j.1365-294X.2005.02748.x
Russ, J. M., Racey, P. A., and Jones, G. (1998). Intraspecific responses to distress calls of the pipistrelle bat, Pipistrellus pipistrellus. Anim. Behav. 55, 705–713. doi: 10.1006/anbe.1997.0665
Russ, J., Jones, G., Mackie, I., and Racey, P. (2004). Interspecific responses to distress calls in bats (Chiroptera: Vespertilionidae): A function for convergence in call design? Anim. Behav. 67, 1005–1014. doi: 10.1016/j.anbehav.2003.09.003
Sachteleben, J., and von Helversen, O. (2006). Songflight behaviour and mating system of the pipistrelle bat (Pipistrellus pipistrellus) in an urban habitat. Acta Chiropterol. 8, 391–401. doi: 10.3161/150811006779398609
Schmidbauer, P., and Denzinger, A. (2019). Social calls of Myotis nattereri during swarming: Call structure mirrors the different behavioral context. PLoS One 14:e0221792. doi: 10.1371/journal.pone.0221792
Schnitzler, H.-U., and Kalko, E. K. V. (2001). Echolocation by insect-eating bats. BioScience 51, 557–569. doi: 10.1641/0006-35682001051[0557:EBIEB]2.0.CO;2
Schöner, C. R., Schöner, M. G., and Kerth, G. (2010). Similar is not the same: Social calls of conspecifics are more effective in attracting wild bats to day roosts than those of other bat species. BioScience 64, 2053–2063. doi: 10.1007/s00265-010-1019-8
Schuchmann, M., and Siemers, B. M. (2010). Behavioral evidence for community-wide species discrimination from echolocation calls in bats. Am. Nat. 176, 72–82. doi: 10.1086/652993
Siemers, B. M., and Schnitzler, H.-U. (2004). Echolocation signals reflect niche differentiation in five sympatric congeneric bat species. Nature 429, 657–661. doi: 10.1038/nature02547
Siemers, B. M., Beedholm, K., Dietz, C., Dietz, I., and Ivanova, T. (2005). Is species identity, sex, age or individual quality conveyed by echolocation call frequency in European horseshoe bats? Acta Chiropterol. 7, 259–264.
Simmons, J. A., Kick, S. A., Lawrence, B. D., Hale, C., Bard, C., and Escudi, B. (1983). Acuity of horizontal angle discrimination by the echolocating bat, Eptesicus fuscus. J. Comp. Physiolo. A 153, 321–330. doi: 10.1007/bf00612586
Stumpf, M., Meier, F., Grosche, L., Halczok, T. K., van Schaik, J., and Kerth, G. (2017). How do young bats find suitable swarming and hibernation sites? Assessing the plausibility of the maternal guidance hypothesis using genetic maternity assignment for two European bat species. Acta Chiropterol. 19, 319–327. doi: 10.3161/15081109ACC2017.19.2.008
van Schaik, J., Janssen, R., Bosch, T., Haarsma, A.-J., Dekker, J. J. A., and Kranstauber, B. (2015). Bats swarm where they hibernate: Compositional similarity between autumn swarming and winter hibernation assemblages at five underground sites. PLoS One 10:e0130850. doi: 10.1371/journal.pone.0130850
Veith, M., Beer, N., Kiefer, A., Johannesen, J., and Seitz, A. (2004). The role of swarming sites for maintaining gene flow in the brown long-eared bat (Plecotus auritus). Heredity 93, 342–349. doi: 10.1038/sj.hdy.6800509
Voigt-Heucke, S. L., Taborsky, M., and Dechmann, D. K. (2010). A dual function of echolocation: Bats use echolocation calls to identify familiar and unfamiliar individuals. Anim. Behav. 80, 59–67. doi: 10.1016/j.anbehav.2010.03.025
Wilkins, M. R., Seddon, N., and Safran, R. J. (2013). Evolutionary divergence in acoustic signals: Causes and consequences. Trends Ecol. Evol. 28, 156–166. doi: 10.1016/j.tree.2012.10.002
Wimmer, B., and Kugelschafter, K. (2015). Akustische Erfassung von Fledermäusen an unterirdischen Quartieren. München: GRIN Verlag.
Yovel, Y., Melcon, M. L., Franz, M. O., Denzinger, A., and Schnitzler, H.-U. (2009). The voice of bats: How greater mouse-eared bats recognize individuals based on their echolocation calls. PLoS Comput. Biol. 5:e1000400. doi: 10.1371/journal.pcbi.1000400
Zhou, X., Garcia-Romero, D., Duraiswami, R., Espy-Wilson, C., and Shamma, S. (2011). “Linear versus mel frequency cepstral coefficients for speaker recognition,” in Proceedings of the IEEE workshop on automatic speech recognition & understanding (Waikoloa, HI), 559–564. doi: 10.1109/ASRU.2011.6163888
Keywords: bats (Chiroptera), autumn swarming, Natterer’s bat, Daubenton’s bat, social calls, interspecific communication, vocalization, Myotis
Citation: Bergmann A, Gloza-Rausch F, Wimmer B, Kugelschafter K and Knörnschild M (2022) Similarities in social calls during autumn swarming may facilitate interspecific communication between Myotis bat species. Front. Ecol. Evol. 10:950951. doi: 10.3389/fevo.2022.950951
Received: 23 May 2022; Accepted: 02 November 2022;
Published: 29 November 2022.
Edited by:
Elise Huchard, UMR 5554 Institut des Sciences de l’Evolution de Montpellier (ISEM), FranceReviewed by:
Angeles Salles, University of Illinois at Chicago, United StatesAaron Corcoran, University of Colorado Colorado Springs, United States
Lilian Hoffmann, Federal University of Rio Grande do Sul, Brazil
Copyright © 2022 Bergmann, Gloza-Rausch, Wimmer, Kugelschafter and Knörnschild. This is an open-access article distributed under the terms of the Creative Commons Attribution License (CC BY). The use, distribution or reproduction in other forums is permitted, provided the original author(s) and the copyright owner(s) are credited and that the original publication in this journal is cited, in accordance with accepted academic practice. No use, distribution or reproduction is permitted which does not comply with these terms.
*Correspondence: Anja Bergmann, QW5qYS5CZXJnbWFubkBmdS1iZXJsaW4uZGU=; Mirjam Knörnschild, TWlyamFtLktub2VybnNjaGlsZEBtZm4uYmVybGlu