- 1Department of Biology, Tufts University, Medford, MA, United States
- 2Evolutionary Ecology Group, Department of Biology, University of Antwerp, Antwerp, Belgium
Bioluminescent insects have been the subject of scientific interest and popular wonder for millennia. But in the 21st century, the fireflies, click beetles, and cave glow-worms that brighten our nights are threatened by an unprecedented competitor: anthropogenic light pollution. Artificial lights can obscure the light-based signals on which these and other bioluminescent organisms rely to court mates, deter predators, and attract prey. In the following review we summarize a recent influx of research into the behavioral consequences of artificial light at night for firefly beetles (Coleoptera: Lampyridae), which we organize into four distinct courtship signaling systems. We conclude by highlighting several opportunities for further research to advance this emerging field and by offering a set of up-to-date lighting recommendations that can help land managers and other stakeholders balance public safety and ecological sustainability.
Introduction
Advances in lighting technology have rapidly increased the scale and intensity of anthropogenic light pollution over the past century. Recent estimates suggest that at least 22.5% of the habitable land surface of the earth currently experiences artificially bright night skies (Falchi et al., 2016), and that night skies have become 49% brighter on average within the last 25 years (Sánchez de Miguel et al., 2021). Artificial light sources responsible include streetlights, car headlights, security lights, facade lighting, stadium lighting, illuminated advertisements, and sources associated with agriculture and industry, e.g., greenhouse lighting or uncurtained office windows (Luginbuhl et al., 2009). Atmospheric scattering of upwelling light produces skyglow, a bright haze visible many kilometers beyond its source that further contributes to light pollution (Kyba et al., 2015; Figures 1A–E).
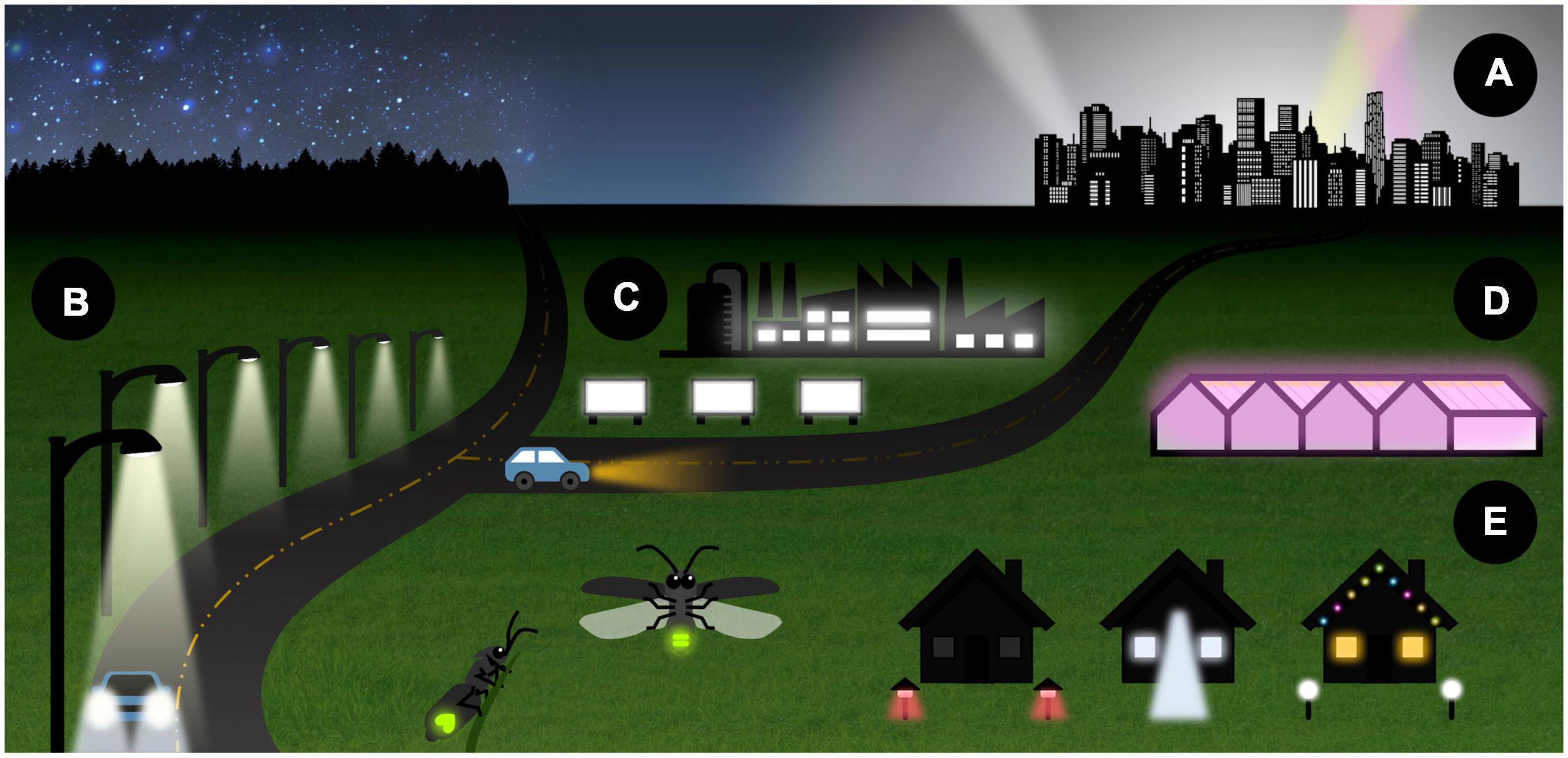
Figure 1. Artificial light at night (ALAN) emanates from diverse sources that vary widely in intensity as well as temporal, spatial, and spectral distribution. Major sources of ALAN include (A) diffuse skyglow, sometimes visible at a great distance from urban centers, (B) artificial lights used in transportation such as streetlights and car headlights, (C) commercial lighting, including billboards and the lit windows of commercial buildings, (D) agricultural lighting, including grow lights used in greenhouses, and (E) private lighting such as security lights, decorative lights, and lit windows.
Unprecedented increases in night sky brightness threaten all taxa that have evolved under predictable cycles of light and dark (Gaston et al., 2017). However, nocturnal insects may be especially vulnerable to the encroachment of artificial light at night (ALAN) into their habitats due to their high visual sensitivity (Warrant, 2017), small body size relative to artificial light sources, and tendency toward positive phototaxis or “flight-to-light” behavior (Owens and Lewis, 2018; Owens et al., 2020). Certain charismatic nocturnal insect taxa capable of bioluminescent communication, fireflies the most successful and species among them (Oba and Schultz, 2014), are likely to be both particularly at risk and particularly able to inspire public interest in dark sky conservation.
Worldwide, over 2200 species of firefly and glow-worm beetles (family Lampyridae, hereafter referred to collectively as fireflies), are currently described (Martin et al., 2019). All firefly species bioluminesce in their larval stage, an adaptation which presumably originated to warn predators of their unpalatable chemical defenses (Branham and Wenzel, 2003; Powell et al., 2022; but note Kok et al., 2019). Most species subsequently co-opted this ability to produce both aposematic signals and sexual advertisements during their brief adult stage (Leavell et al., 2018). Firefly adults typically restrict their courtship activity to a characteristic crepuscular or nocturnal temporal niche, with flight periods lasting from only 20 min up to several hours. Certain species in the genus Photuris have further adapted their signals into foraging lures (Lloyd, 2017).
All types of bioluminescent signal are susceptible to environmental masking (Johnsen et al., 2004). Natural light from the sun or moon can be a predictable obstacle to visibility (Gunn and Gunn, 2012; see also Branham and Faust, 2019), but artificial light from local sources is not only significantly brighter but also spatially, temporally, and spectrally novel. Consequently, ALAN likely acts as a strong selective pressure (Hopkins et al., 2018) that has rapidly intensified in recent years following the development of increasingly efficient and broad-spectrum lighting technologies such as LEDs (Elvidge et al., 2010). If artificial light masks bioluminescent signals, increases in predation rates and decreases in mate success could cause firefly populations to rapidly decline.
In this review, we update an earlier synthesis (Owens and Lewis, 2018) to summarize a flurry of recent studies examining the influence of ALAN on the bioluminescent behaviors of fireflies. Throughout we discuss relevant risk factors that are likely to make certain species especially vulnerable to artificial light. We conclude by pointing out persistent gaps in our understanding of firefly conservation behavior, highlighting several future research directions that we believe will advance this emerging field, and offering a set of informed guidelines to help conservationists develop and maintain “firefly friendly” light environments for at-risk species. The studies reviewed below (Table 1) employed artificial lights with widely varying intensities as well as different temporal, spatial, and spectral distributions; to simplify comparisons, where possible we report intensities in lux, an incomplete but relatively accessible measure of the brightness of broad-spectrum light as viewed by humans.
Firefly visual systems
To understand the effects of ALAN on fireflies, it is crucial to first understand how they perceive light. Fireflies are red-green colorblind (Figure 2; Buck, 1937; Booth et al., 2004; Owens et al., 2018). The common ancestor of all beetles lacked a blue photoreceptor, presumably sacrificing spectral sensitivity to increase total sensitivity (Sharkey et al., 2017). Fireflies appear to have maintained this inherited configuration, with only one unique copy each of UV-sensitive (UVS) and long-wavelength-sensitive (LWS) photoreceptors detected in all species investigated to date (Oba and Kainuma, 2009; Sander and Hall, 2015). Firefly LWS photoreceptors can be tuned to absorb conspecific bioluminescence through the addition of overlying filter pigments (Cronin et al., 2000). Neural comparisons of input from LWS and UVS photoreceptors may permit limited color discrimination in the UV-blue-green range (e.g., Booth et al., 2004).
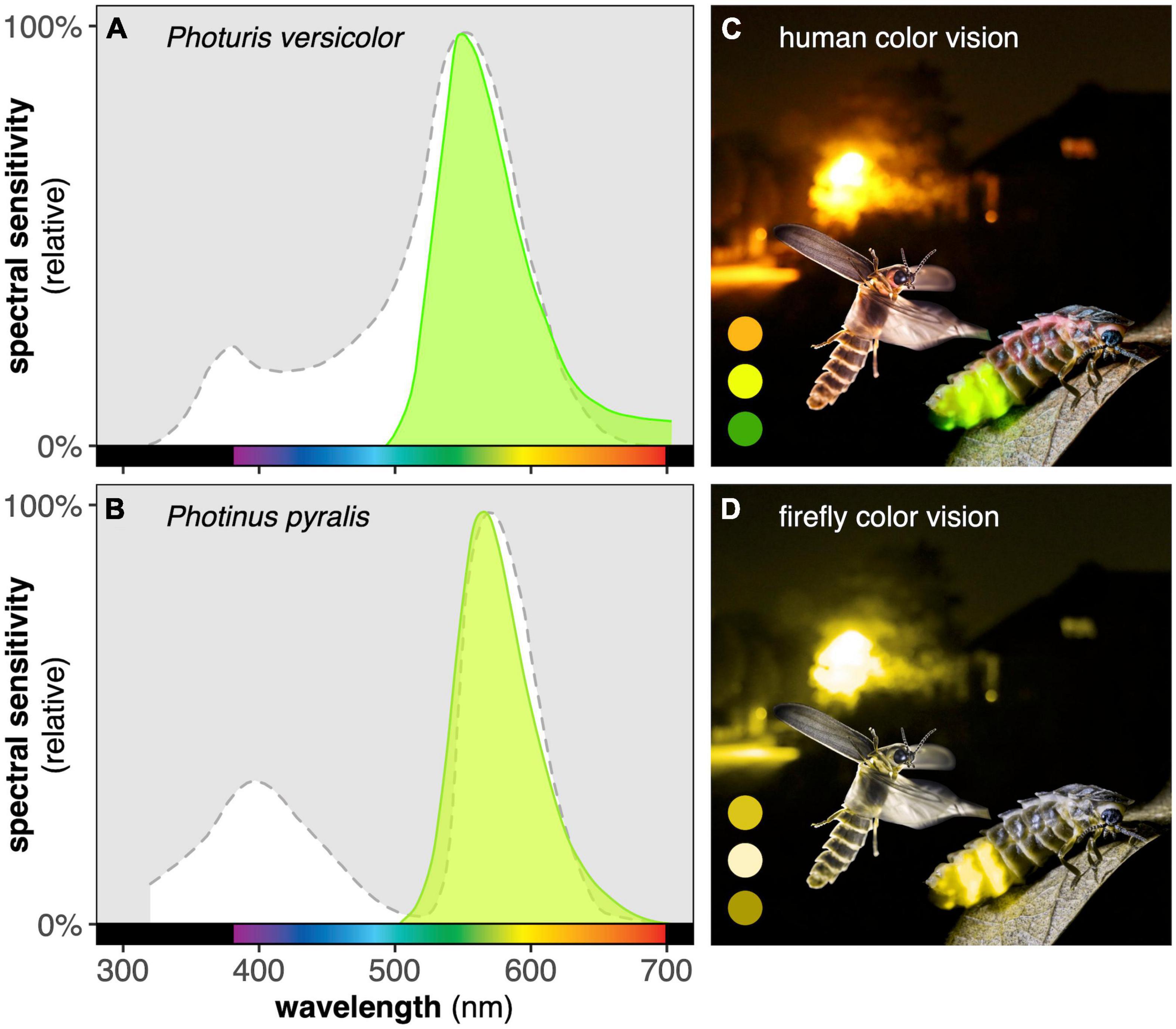
Figure 2. Firefly eyes have been optimized to absorb conspecific signals. The spectral sensitivities of nocturnal Photuris versicolor (A) and crepuscular Photinus pyralis (B) fireflies both peak near the peak wavelength of conspecific bioluminescence (emission spectra shown in green). Fireflies only possess one LWS photoreceptor and are therefore fully red-green colorblind. This means that an image containing a range of green, yellow, and orange regions, as viewed by most humans (C), will appear nearly monochromatic to fireflies (D), with orange and green regions indistinguishable from dim yellow regions. Spectral sensitivities of both species and spectral distribution of P. versicolor bioluminescence modified from Lall et al. (1980a) and Lall (1981); spectral distribution of P. pyralis bioluminescence modified from Hall et al. (2016). Image credits: background by MVDB, stationary female glow-worm by Robert Canis (robertcanis.com), flying male glow-worm by Hubert Polacek; protanopia color vision simulation from pilestone.com.
The bioluminescence emission spectrum and corresponding long-wavelength spectral sensitivity of a given species are usually characteristic of its particular temporal or photic niche. Nocturnal fireflies tend to produce green bioluminescence and to be broadly sensitive to long wavelengths (Figure 2A). In contrast, the compound eyes of crepuscular fireflies often contain red or pink filter pigments that screen out the blue-green ambient light of twilight, increasing the visibility of their signals against surrounding foliage (Cronin et al., 2000; Lall et al., 2009); many of these species produce yellow bioluminescence to maximize visibility within this visual system (Figure 2B; Lall et al., 1980b). Notably some nocturnal fireflies with green bioluminescence, including Photuris versicolor (Cronin et al., 2000) and Lampyris noctiluca (Booth et al., 2004) have yellow filter pigments in the frontal-dorsal regions of their compound eyes, which have been posited to screen out downwelling skylight.
In contrast to the large and complex compound eyes of adults, most firefly larvae possess only a single pair of bilateral stemmata (Murphy and Moiseff, 2019). Despite structural differences, however, the simple eyes of Photuris larvae are functionally similar to the compound eyes of Photuris adults: they are most sensitive to light in the blue-to-green region of the visible light spectrum and appear capable of discriminating colors in this region as well (Murphy and Moiseff, 2019). Photuris larvae move away from artificial light even after their optic nerve has been severed, suggesting that an alternative sensory pathway transmits information on ambient light intensity to the brain (Murphy and Moiseff, 2020). Intrinsically photosensitive areas of the brain, previously documented in Luciola lateralis and Luciola cruciata adults (Hariyama, 2000), may be responsible.
Firefly courtship signaling systems
Fireflies employ diverse visual and chemical signals as sexual advertisements (Ohba, 2004; Lloyd, 2008; da Silveira and Mermudes, 2014), but most species can be categorized into one of four primary signaling systems [Figure 3; adapted from Lewis (2009)]. The impact of artificial light is likely to be contingent upon these different courtship behaviors, briefly described below.
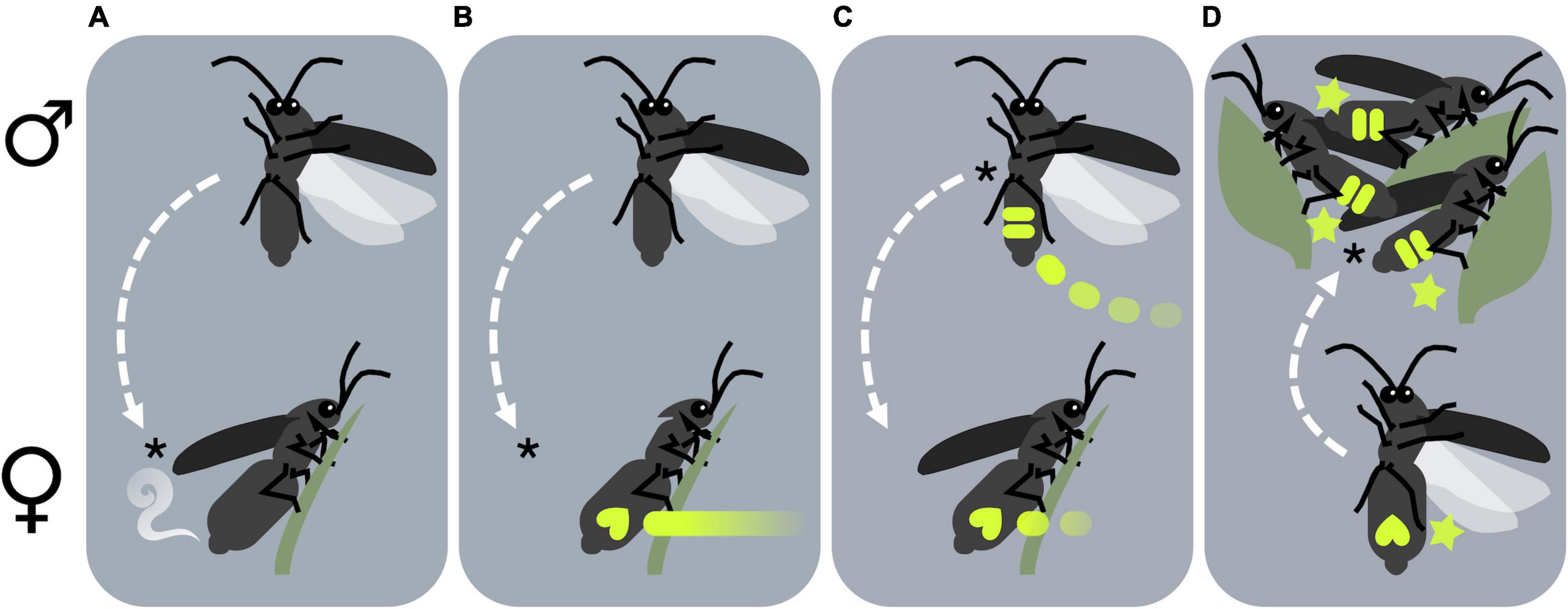
Figure 3. Fireflies may be grouped according to their courtship behavior into one of four signaling systems: either (A) dark, (B) glow-worm, (C) roving flashing, or (D) stationary synchronous fireflies (see main text for explanations of each signaling system). Each panel depicts males (above) and females (below), with asterisks (*) denoting the primary signaler (the sex which initiates courtship), and dashed arrows indicating the direction of movement toward the mating location.
Dark fireflies (Figure 3A) are species with diurnal adults that do not employ bioluminescent courtship signals. Instead, females are known or presumed to use pheromones to attract flying males, which tend to have elongated and elaborated antennae but limited vision (da Silveira and Mermudes, 2014; Stanger-Hall et al., 2018). Male attraction to female pheromones has been documented in Lucidota atra (Lloyd, 1972), Lucidina biplagiata (Ohba, 2004), and Phosphaenus hemipterus (De Cock and Matthysen, 2005).
Glow-worm fireflies or glow-worms (Figure 3B) are sexually dimorphic species with brachypterous or apterous females that are incapable of flight, and which initiate courtship by emitting continuous glows while perched near the ground. In some species such as Pyrocoelia rufa (Ohba, 2004) females attract males with pheromones in addition to continuous glows. The European glow-worm L. noctiluca is widespread throughout Europe and the UK, where glow-worm fireflies predominate (De Cock, 2009). Glow-worm males are fully winged and search for female glow signals, and possibly pheromones, from the air. In some species such as the blue ghost Phausis reticulata (De Cock et al., 2014) and the tracker ghost Amydetes fastigiata (Vaz et al., 2021) males also emit prolonged glows. Due to the presumably limited dispersal ability of flightless females, glow-worm fireflies may be highly vulnerable to habitat degradation and fragmentation resulting from ALAN.
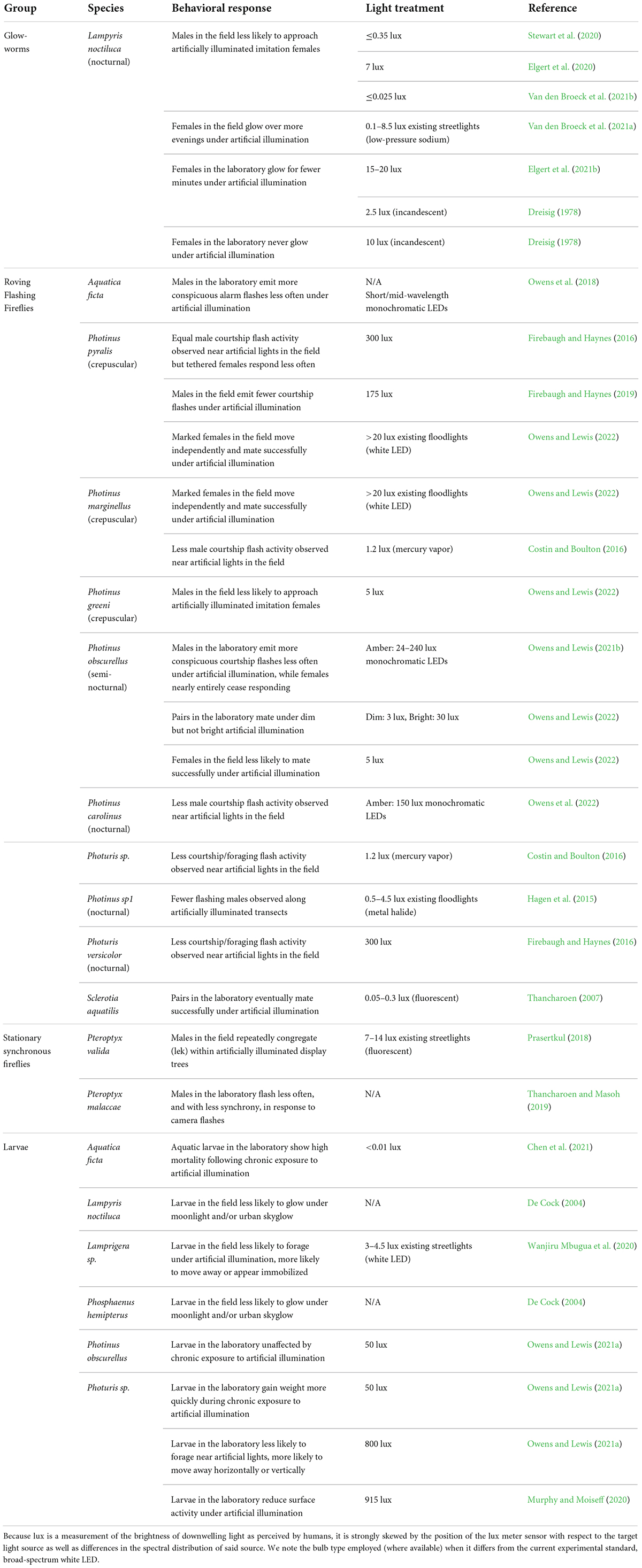
Table 1. Conclusively documented behavioral and developmental effects of artificial light on fireflies. Where appropriate, the intensity of each artificial light treatment has been provided in lux.
Roving flashing fireflies (Figure 3C) are species where both sexes signal using discrete bursts of light. Females of these species are often sedentary, though usually fully winged and capable of flight. Flying males advertise by repeating a species-specific flash pattern at regular intervals, and receptive females answer with response flashes (Ohba, 2004; Lewis and Cratsley, 2008). This flash exchange – known as a courtship dialog – continues until the male locates and mounts the female, and is characteristic of many firefly genera including Luciola, Aquatica, Pyractomena, and Photinus (Stanger-Hall and Lloyd, 2015). Females in the genus Photuris (Souto et al., 2019) are specialist predators of other flashing fireflies, luring patrolling males by mimicking the response flashes of conspecific females (Lloyd, 1980), hawking them from the air (Lloyd and Wing, 1983), and stealing them from spider webs (Faust, 2012). In a few species, such as Photinus carolinus, Photinus knulli, and Photuris frontalis in the United States (Sarfati et al., 2021, 2022), Photinus palaciosi in Mexico, and Luciola sp. in eastern Europe (Baldaccini et al., 1969), flying males synchronize their courtship advertisements when they reach sufficient densities.
Stationary synchronous fireflies (Figure 3D) have in recent decades become popular tourist attractions due to the visual spectacle of their courtship displays (Cheng et al., 2021; Lewis et al., 2021). Males of these species form conspicuous leks, congregating en masse each evening within particular visually prominent display trees (Cratsley et al., 2012; Prasertkul, 2018; Jaikla et al., 2020b). Stationary males perch on leaves and collectively signal by flashing together in tight synchrony (Cratsley et al., 2012; Prasertkul, 2018; Jaikla et al., 2020b). Flying and flashing females orient toward display trees from surrounding areas. Once females reach the lek, complex courtship interactions precede mating (Case, 1980). Example include southeast Asian mangrove species Pteroptyx tener, Pteroptyx malaccae (Jusoh et al., 2018), and Pygoluciola qingyu (Fu and Ballantyne, 2008).
In the following section we review what is currently known about how ALAN impacts adult courtship in each of these distinct signaling systems, then consider how ALAN impacts adult survivorship and larval development.
Effects of artificial light at night on firefly behavior
Courtship
Dark fireflies
Dark firefly adults do not rely on light signals for courtship (Figure 3A). The impact of artificial light on courtship and mating success in these species is therefore likely to depend upon how novel external light cues interact with the internal timekeeping mechanisms (“clocks”) that regulate daily and seasonal rhythms of activity in most terrestrial taxa (Gaston et al., 2017), and whether this results in temporal disorientation (sensu Owens and Lewis, 2018). All fireflies, including species with non-bioluminescent adults, pupate and eclose at certain times of year, and partake in behaviors such as courtship, mating, and oviposition at certain times of day. Artificial light exposure late in the day or summer may prolong daily and seasonal activity, delaying dormancy, while artificial light exposure early in the day or spring may advance activity. Artificial light rich in blue wavelengths may be especially disorienting, as these wavelengths have been shown to most strongly suppress production of melatonin in Drosophila and other taxa (Cashmore et al., 1999).
Glow-worm fireflies
Because patrolling male glow-worms are attracted to continuous glows (Figure 3B), they may confuse continuously operating artificial lights for large receptive females and thereby fall into a potent evolutionary trap (Haynes and Robertson, 2021). Glow-worm flight-to-light behavior has not been documented on a large scale (but see Bek, 2015; Kivelä, 2022). However, small points of artificial light (e.g., from LEDs) have been effectively used to trap males both as a part of surveys (Pacheco et al., 2016) and studies of glow-worm courtship (De Cock et al., 2014). Bespoke “imitation females” have been used to elucidate several aspects of glow-worm physiology and behavior (e.g., Booth et al., 2004).
The European glow-worm L. noctiluca enjoys a wide distribution (De Cock, 2009), and has served as a model organism for the majority of studies investigating the impact of ALAN on the courtship and mate success of bioluminescent fireflies (Table 1). Stewart et al. (2020), Elgert et al. (2021a), and Van den Broeck et al. (2021b) all found that when imitation female glow signals were illuminated by broad-spectrum white LEDs, they attracted fewer L. noctiluca males compared to unilluminated controls (see also Ineichen and Rüttimann, 2012; Bird and Parker, 2014; Kivelä, 2022), and that this difference was magnified under brighter illumination. ALAN spectra, intensity, and directionality have become a focus of recent research as they might be adjusted to minimize detrimental effects. Elgert et al. (2020) found that 7 lux of downwelling ALAN, which is dimmer than the light cast by most streetlights, significantly decreased the attractiveness of imitation females to males. Stewart et al. (2020) found effects of horizontally-directed ALAN up to 40 m away from the source, where it measured approximately 0.35 lux. Using a similar experimental setup with upwelling ALAN, Van den Broeck et al. (2021b) saw significantly decreased attractiveness of imitation females under only 0.025 lux of ALAN, similar in intensity to moonlight (Kyba et al., 2017). The authors also compared the impact of illumination by cool vs. warm white LEDs, which emit different ratios of blue to longer wavelengths, and found that both treatments interfered equally with the ability of males to locate imitation females.
Limiting the duration of artificial light could be another way to minimize detrimental effects. Van den Broeck et al. (2021a) observed the effects of long-term exposure to ALAN on the mate success of live females in the field by repeatedly checking whether or not they were glowing (L. noctiluca females cease glowing once they have mated; Tyler, 2002). While females in dark sites (< 0.1 lux) glowed for only a single evening, females in areas lit by low-pressure sodium street lights (0.1–8.5 lux) glowed for a median of six and as many as 24 evenings, indicating that these artificially illuminated females experienced significantly decreased mate success. However, in a recent laboratory experiment Elgert et al. (2021b) observed that females were significantly more likely to cease glowing when artificially illuminated: on average, females went dark after only 26 min of continuous ALAN exposure. This behavioral difference could be explained by the brighter (15–20 lux) and more direct artificial light used in the lab study, or by the relatively short timescale. In an early laboratory experiment, Dreisig (1978) found that 2.5 lux of artificial light reduced the duration of glow activity from an average of 139 min to only 51 min and that females never began to glow under 10 lux of artificial light. Between 0.001 and 1.0 lux the duration of glow activity was similar to that under natural conditions. The suppressive effect of ALAN on the production of glow signals by female glow-worms may therefore be weaker in the field, where artificial illumination is comparatively dim and indirect, and may also abate over multiple evenings.
Both abbreviated and prolonged glowing periods due to ALAN could be associated with fitness costs. L. noctiluca females that never glow are significantly less likely to attract nearby males (De Cock et al., 2014), while those that glow over multiple evenings likely do so only because they remain unmated (Tyler, 2002). Even if these females do eventually mate, delays are energetically costly: glow-worms are capital breeders that rarely feed as adults (Wing, 1989; Tyler, 2002), and the energy they require for self-maintenance and bioluminescence depletes over time (Baudry et al., 2021). Some of this energy comes from metabolized eggs (Wing, 1989), steeply reducing fecundity (Horne et al., 2017; Hopkins et al., 2021). Prolonged glowing periods could also increase predation risk, although few predators are able or willing to overcome glow-worm chemical defenses (see section “Predator-prey interactions” below; De Cock and Matthysen, 2001; Lewis et al., 2011). Small females may be especially vulnerable to artificial light, both because their glows tend to be dimmer (Borshagovski et al., 2020), and therefore less visible under artificial illumination, and because they have fewer energy reserves with which to produce them. This may explain why smaller females cease glowing earlier under ALAN than do their larger counterparts (Elgert et al., 2021b; Hopkins et al., 2021).
Roving flashing fireflies
Fireflies that exchange precisely timed, discrete flashes as part of courtship (Figure 3C) seem unlikely to mistake continuously glowing artificial light sources for conspecifics. However, like other bioluminescent taxa, these species are nevertheless vulnerable to masking effects of artificial light.
Several recent studies have examined the impact of ALAN on courtship signaling by North American flashing fireflies, primarily in the genera Photinus and Photuris (Table 1). Under moderately dim artificial light, a mixed species assemblage reduced their courtship flash activity (number of flash patterns per minute) to 50% of the baseline rate (1.2 lux; Costin and Boulton, 2016). Males of the common crepuscular species Photinus pyralis flashed at 75% of their baseline rate when placed directly beneath a bright artificial light source (175 lux; Firebaugh and Haynes, 2019), while nocturnal Photuris versicolor fireflies flashed at 30% of baseline within 10 m of a similar source (300 lux; Firebaugh and Haynes, 2016). In the laboratory, males of the semi-nocturnal species Photinus obscurellus flashed at approximately 50% of baseline under dim and bright artificial light (24 or 240 lux; Owens and Lewis, 2021b; see also Owens and Lewis, 2022). The number of semi-nocturnal Photinus sp1 males observed flashing in a transect 60 m from a bright floodlight was 13% of baseline (4 lux; Hagen et al., 2015). Similarly, at a popular firefly ecotourism site, males of the fully nocturnal, synchronous species P. carolinus flashed at only 4% of baseline within 5 m of an artificial light source (150 lux, broad-spectrum amber; Owens et al., 2022). Reductions in male courtship flash activity are frequently assumed to correspond to reductions in mate success, but a recent report suggests the strength of this relationship also varies by species (Owens and Lewis, 2022).
How ALAN impacts female courtship flash activity has been less well studied, perhaps because in flashing fireflies, females are typically more cryptic. In most species, however, female behavior determines mate success: the likelihood of any male successfully locating and mating with a female depends on how often she answers his advertisement flashes (Demary et al., 2005; Owens and Lewis, 2022). Recent studies on two Photinus species suggest that females are even more sensitive than males to downwelling ALAN (Firebaugh and Haynes, 2016; Owens and Lewis, 2021b). In P. pyralis, white light (175 lux) suppressed female response rates to around 50% of baseline (Firebaugh and Haynes, 2016, 2019). In P. obscurellus, female response rates response rates declined to only 10% of baseline under white light (24 or 240 lux), and 50% of baseline under dim red light (Owens and Lewis, 2021b). Females of these species perch near the ground and search the sky for male advertisements, and may therefore be more likely to look directly into an artificial light source than are patrolling males searching the ground for females. Whether subsequent light-adaptation of their compound eyes prevents females from perceiving male flashes entirely or reduces male attractiveness by making their courtship flashes appear dimmer remains unknown. Regardless, reductions in female response flash activity are once again assumed to correspond to reductions in mate success.
Direct investigations into the impact of ALAN on mate success in flashing fireflies have had surprisingly mixed results. In the field, males of the crepuscular species Photinus greeni almost never approached imitation females that were directly illuminated when there were unilluminated competitors nearby (5 lux; Owens and Lewis, 2022). However, an early study by Thancharoen (2007) found that pairs of crepuscular Sclerotia aquatilis fireflies enclosed together in the laboratory (theoretically obviating the effects of sexual competition and/or impaired mate location) mated successfully under very dim light (0.05–0.3 lux), although courtship and mounting behaviors were prolonged. P. obscurellus pairs enclosed together in the laboratory mated successfully under dim light (3 lux) but not bright light (30 lux; Owens and Lewis, 2022). Dim light (5 lux) was relatively more disruptive to the mate success of P. obscurellus females permitted to move freely in the field, yet even bright light (>20 lux) had no detectable impact on the mate success of females of the crepuscular species P. pyralis and Photinus marginellus (Owens and Lewis, 2022).
Some roving flashing fireflies appear therefore capable of behaviorally adapting to ALAN. P. obscurellus males in the laboratory plastically increased the intensity, duration, and density (number of flashes per flash pattern) of their advertisement flashes in response to increases in ambient light levels, although these behavioral adaptations were unable to rescue baseline female response rates (Owens and Lewis, 2021b; see also Owens and Lewis, 2022). Other species, especially those active near sunset, may be even more capable of competing with visual noise (but note Borshagovski et al., 2020) or may be able to rely on redundant, as yet undescribed pheromone cues when engaging in courtship and mating activity in a less informative visual environment.
Stationary synchronous fireflies
Among the most visually impressive of all animal courtships are the communal displays (Figure 3D) created by various lekking fireflies that range from East India through Thailand, Malaysia and Indonesia across to the Philippines and Papua New Guinea (Ballantyne and Lambkin, 2013; Jusoh et al., 2018; Jaikla et al., 2020a). Such fireflies include several Pteroptyx species, in which thousands of males gather in visually prominent display trees along tidal rivers and flash synchronously to create a collective courtship signal that attracts flying females from surrounding areas (Wong and Yeap, 2012; Jusoh et al., 2018; Jaikla et al., 2020a). These congregating fireflies are star attractions in a booming ecotourism sector throughout this region (Lewis et al., 2021), yet surprisingly little is known about how ALAN impacts their behavior or population persistence.
In addition to the disappearance of their mangrove forest habitat, Wong and Yeap (2012) mention bright artificial lights associated with shrimp farms and tourism infrastructure as possible threats to Pteroptyx fireflies in Malaysia. However, Prasertkul (2018) recorded large congregations of Pteroptyx valida and P. malaccae that remained year-round in close proximity to artificial illumination from fluorescent streetlights (3–14 lux) and house lights (0.5–5 lux) in an urban park near Bangkok, Thailand. It thus appears, at least in this urban setting, that light pollution does not prevent Pteroptyx males from congregating. It remains unknown whether artificial light might impact mate attraction in congregating fireflies, for example by disrupting male flash synchrony or decreasing female recruitment into congregations. Even after females arrive within Pteroptyx display trees, the sexes continue to communicate using flash signals (Case, 1980). In apparent competitive interactions, males flash while aiming their lanterns directly toward nearby males. Before attempting copulation, males also flash while curling their lanterns directly in front of the eyes of the focal female. Additional work is needed to determine whether ALAN affects these close-range sexual interactions.
When poorly managed, tourism exposes local firefly populations to artificial light in numerous ways: distant semi-permanent sources associated with infrastructure (e.g., signage, buildings, and roads) and close-range, transient flash photography. During field observations of Pteroptyx spp., Lloyd (1973) reported that males were readily attracted to flashes from a small incandescent penlight, including some males that approached from up to 75 feet away. Thancharoen and Masoh (2019) studied small aggregations of P. malaccae brought into the laboratory to examine how tourist photography might impact courtship and oviposition. Flash illumination from smartphones and digital cameras, as well as red autofocus lights, temporarily reduced male flash activity, although most males eventually resumed flashing and successfully mounted females. Males also exhibited decreased synchrony following all types of camera illumination, suggesting that unregulated tourist photography could impede their ability to synchronize in the field. Based on these findings, recently published guidelines for sustainable firefly tourism recommend limiting flashlight and phone use and prohibiting flash photography (Lewis et al., 2021).
Predator-prey interactions
Many, but not all, fireflies have protective chemical defenses (Eisner et al., 1997; Tyler et al., 2008; Fu et al., 2009; Berger et al., 2021) that prevent attack by most predators, especially vertebrates (Day, 2011). For firefly adults, bioluminescence is a main component of a multimodal aposematic signal that is also thought to include reflectance-based red, yellow, and black warning coloration (Stevens and Ruxton, 2012), wing beat frequencies (Leavell et al., 2018), and ultrasonic clicks (Krivoruchko et al., 2021). Firefly larvae employ bioluminescence exclusively as an aposematic signal, in combination with warning coloration (De Cock and Matthysen, 2001), and likely have done so for close to 150 million years (Martin et al., 2017; Powell et al., 2022). By interfering with the detection of these reflectance- and emission-based visual signals of unpalatability, ALAN has the potential to heighten predation on firefly adults and larvae (Briolat et al., 2021).
Owens et al. (2018) exposed male Aquatica ficta fireflies to artificial illumination of varying spectra and intensity while recording the aposematic alarm flashes they produced in response to the constraints of the experimental apparatus. Alarmed A. ficta males responded to ALAN much as did advertising P. obscurellus males in a later study (Owens and Lewis, 2021b): their flashes became more conspicuous (brighter and longer) under short- to mid-wavelength artificial light, but were produced at only 45% of the baseline rate. Similarly, an early field study found that L. noctiluca and P. hemipterus glow-worm larvae glowed less often under bright night skies, especially when passing clouds diffused moonlight and artificial light from nearby developments into their usually dark habitats (De Cock, 2004).
For roving flashing fireflies in the genus Photuris, bioluminescence functions in their roles as both predator and prey (Souto et al., 2019). Firebaugh and Haynes (2019) attempted to document interactions between female P. versicolor predators and male P. pyralis prey within artificially illuminated field enclosures (175 lux). ALAN reduced the flash activity of both species, but no predation was observed in either lit or unlit field enclosures; hence the influence of ALAN on these particular predator-prey interactions remains unclear (Lewis and Owens, 2019).
Development
The effects of ALAN on earlier firefly life stages are relatively unexplored (Table 1). Owens and Lewis (2021a) conducted laboratory experiments to investigate how ALAN affects the development of immature Photuris sp. and P. obscurellus fireflies. Long-term exposure to artificial light at night (50 lux) did not impact overall survivorship or the duration of egg, larval, and pupal stages in either species, both of which spend the majority of their larval lifespan underground. It did however, accelerate weight gain of Photuris larvae, perhaps by prolonging perceived daylength – assessed before or during nightly foraging bouts on the soil surface – and thus delaying diapause (Gaston et al., 2017). Rearing aquatic A. ficta larvae under very dim ALAN for 2 weeks resulted in high mortality both immediately following and several months after exposure (Chen et al., 2021). Subsequent gene expression profiling of these larvae suggested that ALAN may perturb hormone regulation and suppress reproductive development.
Three recent studies report on larval movements in response to ALAN. Wanjiru Mbugua et al. (2020) found that Lamprigera sp. larvae foraging in an urban park could often be found near paved trails, but avoided areas illuminated by streetlights (≥ 3 lux); the few larvae found directly beneath lit streetlights tended to be immobile. Similarly, Photuris larvae exposed in the laboratory to point sources of ALAN (800 lux) at the start of their nightly surface foraging period moved toward darker areas, but a significant number burrowed beneath the surface instead of dispersing across it (Owens and Lewis, 2021a). Murphy and Moiseff (2020) also found that uniform ALAN (∼915 lux) reduced surface movements of Photuris larvae. Such behavioral responses may arise because sufficiently bright ALAN appears to prolong daylength, and nocturnal larvae are inactive during the “day.” By immobilizing larvae, ALAN could increase their chances of being trampled in heavily trafficked areas (an already frequent occurrence: Lehtonen et al., 2021) and impede their ability to disperse into darker habitats where they might enjoy greater reproductive success as adults.
In contrast to most species, the later larval instars of L. noctiluca glow-worms (Tyler, 2002; Tyler, 2013) and related species (De Cock and Matthysen, 2001) regularly exhibit diurnal activity. While preparing to pupate, these “walkabout” larvae can often be found crawling over bare soil, rocks, or pavement in broad daylight (e.g., Lehtonen et al., 2021), presumably searching for pupation sites and, among females, associated display sites (Tyler, 2013). If so, individuals may not detect ALAN until they reach their relatively philopatric adult stage, which would explain why adult females can so often be found signaling unsuccessfully from brightly lit display sites (Ineichen and Rüttimann, 2012; Van den Broeck et al., 2021a). In fact, late-instar females that actively search for open habitat (Lehtonen et al., 2021) may use environmental light as a cue for habitat quality, in which case ALAN sources operating before dusk or after dawn could act as ecological traps (sensu Robertson et al., 2017).
Further research should reveal which, if any, particular aspects of artificial light environments (spatial, temporal, spectral, etc.) most affect fireflies of all life stages.
Future directions
Visual ecology
Because the firefly visual system is highly optimized, what a species perceives can reveal a great deal about how they might respond to artificial light. Unfortunately, little is known about the vision of most species. Electroretinography and photoreceptor gene sequencing efforts have both thus far primarily revealed the spectral sensitivities of North American (Lall et al., 2009; Sander and Hall, 2015) and Asian (Eguchi et al., 1984) roving flashing fireflies (but see Booth et al., 2004). Data on changes in overall sensitivity due to light-adaptation of the compound eye are also urgently needed to understand the mechanisms underlying the impact of artificial light on courtship and mate success (but see Lall, 1993; Oba and Kainuma, 2009; Smith, 2011). For example, it remains unknown whether glow-worm males and roving flashing firefly females fail to respond to artificially illuminated conspecifics because they are blind to their courtship signals, because their courtship signals appear less bright against the background, or because ALAN transitions fireflies from a mode of nocturnal courtship behavior to one of diurnal resting behavior through temporal disorientation.
Movement ecology
Fireflies that are negatively impacted by artificial light may be able to persist by sheltering in shaded refuges or dispersing to darker areas of the landscape. For example, species that typically occupy open habitats such as lawns, meadows, or grasslands may aggregate along forest edges or in forest clearings where their signals are more likely to be detected. Whether light-polluted populations are capable of fully transitioning to new habitat types remains unknown, as are the associated fitness costs: even if adults are relatively flexible in their habitat requirements their juvenile stages may not be. Field studies of firefly movement under artificial light are rare and almost exclusively involve single generations of largely subterranean larvae (Wanjiru Mbugua et al., 2020; Owens and Lewis, 2021a; see also Kakehashi et al., 2014) or species that are relatively resilient to ALAN (Firebaugh and Haynes, 2016; Owens and Lewis, 2022; but see Gardiner and Didham, 2021). The primary dispersing life stage (larvae, adult males or females) is also unknown for nearly every species (but see Kaufmann, 1965), despite how crucial this information is for conservation planning (Schultz et al., 2019).
In the absence of long-term survey data (see below), genetic differentiation can reflect the movement of firefly populations not just on evolutionary timescales (Suzuki et al., 2004; Lower et al., 2018; Kim et al., 2021) but also the approximately 20–200 years timescales most relevant to artificial light (e.g., Dayton and Szczys, 2021). Population genetic studies could reveal whether different species have moved toward or away from intensely light-polluted habitats over time, and whether particular sources have resulted in population sinks or barriers to gene flow, e.g., if lines of regularly spaced streetlights along roads intensify habitat fragmentation. Comparative field surveys and behavioral studies could help confirm and elaborate upon these results. For example, female L. noctiluca glow-worms are significantly less abundant near artificial lights (Gardiner and Didham, 2021) but marked individuals rarely leave artificially illuminated display sites either in the field or the laboratory (Elgert et al., 2020; Van den Broeck et al., 2021a), meaning that the current distribution may be symptomatic of a population sink.
Nocturnal insects such as moths, mayflies, and gnats can be maladaptively attracted to artificial lights (Haynes and Robertson, 2021). While male glow-worms have repeatedly been shown to approach artificial lights that are sufficiently dim and long-wavelength so as to resemble female conspecifics (Schwalb, 1961; Booth et al., 2004; Bek, 2015; Pacheco et al., 2016), fireflies are not commonly thought to be attracted to the broad-spectrum artificial lights used for public safety, unless they somehow simulate conspecific courtship signals (i.e., flash patterns). Surprisingly, however, one recent study captured significant numbers of P. pyralis and Photuris fireflies at non-flashing broad-spectrum light traps (Firebaugh and Haynes, 2019). Because species that are attracted to or simply not repelled by ALAN could be at significantly greater risk of courtship disruption and subsequent population decline, broader understanding of the phototactic tendencies of fireflies should be a research priority.
Evolutionary adaptation
Few studies have investigated whether fireflies may be evolving physiologically or behaviorally in response to artificial light, a novel selection pressure (Hopkins et al., 2018). Several possibilities merit further investigation.
The amplification of intensity-based sexual selection in light-polluted habitats may result in firefly populations that emit more intense bioluminescent courtship signals, possibly at negligible metabolic cost (Woods et al., 2007). At higher latitudes, L. noctiluca females possess larger light organs that emit brighter glows, presumably to attract males during the relatively short and bright summer nights (Borshagovski et al., 2020; compare to Owens et al., 2018). Simulated conspecific courtship advertisements of greater intensity also garner more frequent responses from P. pyralis females (Vencl and Carlson, 1998) and attract more L. noctiluca males, both in darkness (Hopkins et al., 2015) and under artificial light (Elgert et al., 2021a). Evolutionary adaptations that increase the conspicuousness of bioluminescent signals in order to preserve lines of visual communication may be augmented via the addition of more filter pigments in the compound eye that further narrow long-wavelength sensitivity to the peak wavelength of conspecific bioluminescence.
Alternatively, firefly populations may shift away from a primarily visual communication system toward greater reliance on chemical signals. Pheromones are broadly used as sexual signals by diurnal dark fireflies (Stanger-Hall et al., 2018), and also contribute to the multimodal courtship signals of some nocturnal glow-worms (Ohba, 2004); their use by other firefly species has yet to be fully investigated. Chemical signals should be relatively unaffected by ALAN and therefore may more reliably attract conspecifics within severely light-polluted habitats. Comparisons of multiple urban and rural populations of the same firefly species (e.g., Santangelo et al., 2022) can disentangle the conflicting possibilities described above to reveal whether artificial light promotes or inhibits bioluminescent signaling behavior. If consistent differences are observed, common garden experiments will be able to reveal whether these differences are the result of phenotypic plasticity or rapid evolution. Artificial selection on species that can be reared in the laboratory could then help elucidate the timescales on which such differences are likely to arise and quantify their benefits for reproductive fitness.
A third, related possibility is that some firefly species are already relatively resilient to artificial light due to favorable life history traits (e.g., flightedness, lack of habitat specificity, diurnal or crepuscular temporal niche, use of multimodal courtship signals, asynchronicity, etc.), and are gradually replacing less resilient species within severely light-polluted habitats (see Khattar et al., 2022). For example, P. pyralis, the most widespread roving flashing firefly in North America, has a crepuscular courtship period and appears to be relatively unaffected by artificial light (Firebaugh and Haynes, 2016; Owens and Lewis, 2022); P. pyralis and other crepuscular species may even be able to capitalize on resultant expansions in their temporal or photic niche, while fully nocturnal species are left at a disadvantage. Although it can be difficult to track the abundances of individual firefly species within a community due to the skill required for species identification (e.g., Lloyd, 2017), long-term expert surveys may be able to document changes in species composition over time and by doing so help predict the future for firefly populations on an increasingly light-polluted planet.
Conservation recommendations
Clearly much remains to be done. Although long-term survey data are sparse, both anecdotal reports and expert opinion suggest certain firefly populations have recently undergone population declines (Jusoh and Hashim, 2012; Khoo et al., 2016; Atkins et al., 2017; Faust, 2017; Lloyd, 2017; Gardiner and Didham, 2020; Lewis et al., 2020; Fallon et al., 2021; see also Chatragadda, 2020). A survey of international firefly experts ranked ALAN among the top three threats to fireflies in nearly every geographic region (Lewis et al., 2020). Even in the absence of evidence demonstrating that ALAN is a direct causal factor in firefly declines (but see Cornelisse et al., 2019), the myriad negative impacts of ALAN reviewed here suggest the urgent need for conservation action, especially as the extent and intensity of artificial light continues to grow (Vaz et al., 2021). Many straightforward measures have already been proposed to address the general catastrophe of insect declines (Harvey et al., 2020), and fireflies can act as flagship species to galvanize these efforts. We already know enough to recommend several high priority, no-regret conservation actions for keeping their magic alive, including:
• Prevent habitat degradation by removing or limiting ALAN within protected areas and other locations that support at-risk firefly species.
• Launch education and outreach programs to promote locally appropriate firefly-friendly lighting guidelines (see below).
• Establish standardized monitoring programs to track long-term population trends for fireflies occurring along an ALAN gradient.
Targeted community science monitoring programs (e.g., the UK Glow-worm Survey, Observatoire des vers luisants et lucioles, Night Lights of Kuala Selangor Nature Park, Mass Audubon Firefly Watch, etc.) can be of immense value in identifying firefly populations on the decline. These programs should be continued, expanded, and where possible unified into a single standardized platform that supports species identification (by trained observers or machine-learning algorithms, e.g., Goh et al., 2022). The responses of fireflies to ALAN are highly species-specific, and measures of general firefly activity without accompanying species information can be compromised by changes in species composition. Similarly, firefly surveys should aim to count individuals instead of flashes or glow signals, due to the known effects of ALAN on courtship signaling behavior.
The studies we have reviewed here also provide the basis for more specific lighting recommendations. The simplest and most effective conservation measure is to switch off any lights situated in and around known firefly and glow-worm habitats during mating seasons. On low-traffic roadways, motion sensors that switch off streetlights when vehicles are absent can offer an efficient conservation solution. Where removing artificial lights is not possible for safety reasons, proper shielding should be installed on streetlights to limit light spillage into firefly and glow-worm habitat. Given that extremely low levels of artificial light reduce mating success of L. noctiluca glow-worms (Van den Broeck et al., 2021b), simply dimming streetlights may not suffice to mitigate ALAN impact as it does for some other taxa. Gardiner and Didham (2021) recommend a minimum distance of 100 m between artificial light and glow-worm habitat. Vaz et al. (2021) point out the importance of establishing new protected areas within the darkest places where at-risk fireflies are found, as well as reducing ALAN within existing protected areas. Unfortunately, evidence suggests that no spectrum of artificial light is universally “firefly friendly” (Owens et al., 2018, 2022; Owens and Lewis, 2021b). However, red light is preferable to other colors as it is much less visible to insects generally (van der Kooi et al., 2020); green, yellow, and amber light should be avoided because they are likely to be especially disruptive due to their spectral overlap with firefly bioluminescence.
Author contributions
AO: conceptualization, writing – original draft, review and editing, and visualization. MV: writing – original draft, review and editing, and visualization. RD: writing – original draft, review and editing, and supervision. SL: conceptualization, writing – original draft, review and editing, and supervision. All authors contributed to the article and approved the submitted version.
Funding
This research was supported by a 2021–2022 PEO Scholar Award to AO and a FWO Flanders Ph.D. fellowship (Grant ID: 1143322N) to MV.
Conflict of interest
The authors declare that the research was conducted in the absence of any commercial or financial relationships that could be construed as a potential conflict of interest.
Publisher’s note
All claims expressed in this article are solely those of the authors and do not necessarily represent those of their affiliated organizations, or those of the publisher, the editors and the reviewers. Any product that may be evaluated in this article, or claim that may be made by its manufacturer, is not guaranteed or endorsed by the publisher.
References
Atkins, V., Bell, D., Bowker, A., Charig, M., Crew, J., Dale, M., et al. (2017). The status of the glow-worm Lampyris noctiluca L. in England. Lampyrid 4, 20–35.
Baldaccini, N. E., Fiaschi, V., and Papi, F. (1969). Rhythmic synchronous flashing in a Bosnian firefly. Ital. J. Zool. 3, 239–245.
Ballantyne, L. A., and Lambkin, C. L. (2013). Systematics and phylogenetics of Indo-Pacific Luciolinae fireflies (Coleoptera: Lampyridae) and the description of new genera. Zootaxa 3653, 1–162. doi: 10.11646/zootaxa.3653.1.1
Baudry, G., Hopkins, J., Watts, P. C., and Kaitala, A. (2021). Female sexual signaling in a capital breeder, the European glow-worm Lampyris noctiluca. J. Insect Behav. 34, 16–25. doi: 10.1007/s10905-020-09763-9
Bek, R. J. (2015). Investigating the Impact of Artificial Night Lighting on the Common European Glow-Worm. Leeds: University of Leeds.
Berger, A., Petschenka, G., Degenkolb, T., Geisthardt, M., and Vilcinskas, A. (2021). Insect collections as an untapped source of bioactive compounds-Fireflies and cardiotonic steroids as a proof of concept. Insects 12:689. doi: 10.3390/insects12080689
Bird, S., and Parker, J. (2014). Low levels of light pollution may block the ability of male glow-worms to locate females. J. Insect Conserv. 18, 737–743. doi: 10.1007/s10841-014-9664-2
Booth, D., Stewart, A. J. A., and Osorio, D. (2004). Colour vision in the glow-worm Lampyris noctiluca: Evidence for a green-blue chromatic mechanism. J. Exp. Biol. 207, 2373–2378. doi: 10.1242/jeb.01044
Borshagovski, A.-M., Saari, P., Lehtonen, T. K., and Kaitala, A. (2020). When night never falls: Female sexual signalling in a nocturnal insect along a latitudinal gradient. Behav. Ecol. Sociobiol. 74:153. doi: 10.1007/s00265-020-02927-9
Branham, M. A., and Faust, L. F. (2019). Firefly flashing activity during the totality phase of a solar eclipse. Entomol. News 128:191. doi: 10.3157/021.128.0207
Branham, M. A., and Wenzel, J. W. (2003). The origin of photic behavior and the evolution of sexual communication in fireflies (Coleoptera: Lampyridae). Cladistics 19, 1–22. doi: 10.1111/j.1096-0031.2003.tb00404.x
Briolat, E. S., Gaston, K. J., Bennie, J., Rosenfeld, E. J., and Troscianko, J. (2021). Artificial nighttime lighting impacts visual ecology links between flowers, pollinators and predators. Nat. Commun. 12:4163. doi: 10.1038/s41467-021-24394-0
Buck, J. B. (1937). Studies on the firefly. II. The signal system and color vision in Photinus pyralis. Physiol. Zool. 10, 412–419. doi: 10.1086/physzool.10.4.30151427
Case, J. F. (1980). Courting behavior in a synchronously flashing, aggregative firefly, Pteroptyx tener. Biol. Bull. 159, 613–625. doi: 10.2307/1540827
Cashmore, A. R., Jarillo, J. A., Wu, Y. J., and Liu, D. (1999). Cryptochromes: Blue light receptors for plants and animals. Science 284, 760–765. doi: 10.1126/science.284.5415.760
Chatragadda, R. (2020). Decline of luminous firefly Abscondita chinensis population in Barrankula, Andhra Pradesh, India. Int. J. Trop. Insect Sci. 40, 461–465. doi: 10.1007/s42690-019-00078-7
Chen, Y.-R., Wei, W.-L., Tzeng, D. T. W., Owens, A. C. S., Tang, H.-C., Wu, C.-S., et al. (2021). Effects of artificial light at night (ALAN) on gene expression of Aquatica ficta firefly larvae. Environ. Pollut. 281:116944. doi: 10.1016/j.envpol.2021.116944
Cheng, S., Faidi, M. A., Tan, S.-A., Vijayanathan, J., Malek, M. A., Bahashim, B., et al. (2021). Fireflies in Southeast Asia: Knowledge gaps, entomotourism and conservation. Biodivers. Conserv. 30, 925–944. doi: 10.1007/s10531-021-02129-3
Cornelisse, T., Jepsen, S., Fallon, C., and Tyler, J. (2019). Petition for emergency listing of the Bethany Beach Firefly (Photuris bethaniensis) under the Endangered Species Act and to Concurrently Designate Critical Habitat. Portland, OR: Xerces Society.
Costin, K. J., and Boulton, A. M. (2016). A field experiment on the effect of introduced light pollution on fireflies in the Piedmont Region of Maryland. Coleopt. Bull. 70, 84–86. doi: 10.1649/072.070.0110
Cratsley, C., Prasertkul, T., and Thancharoen, A. (2012). Male aggregation behaviour in the fireflies Photinus ignitus and Pteroptyx malaccae. Lampyrid 2, 87–98.
Cronin, T. W., Järvilehto, M., Weckström, M., and Lall, A. B. (2000). Tuning of photoreceptor spectral sensitivity in fireflies. J. Comp. Physiol. A 186, 1–12. doi: 10.1007/s003590050001
da Silveira, L. F. L., and Mermudes, J. R. M. (2014). Systematic review of the firefly genus Amydetes Illiger, 1807 (Coleoptera: Lampyridae), with description of 13 new species. Zootaxa 3765, 201–248. doi: 10.11646/zootaxa.3765.3.1
Day, J. C. (2011). Parasites, predators and defence of fireflies and glow-worms. Lampyrid 1, 70–102.
Dayton, J., and Szczys, P. (2021). Metapopulation connectivity retains genetic diversity following a historical bottleneck in a federally endangered seabird. Ornithol. Appl. 123:duab037. doi: 10.1093/ornithapp/duab037
De Cock, R. (2004). The Adaptive Value of Luminescent Behaviour in Glow-Worms (Coleoptera: Lampyridae). Ann Arbor, MI: ProQuest Dissertations Publishing.
De Cock, R. (2009). “Biology and behaviour of European lampyrids,” in Bioluminescence in Focus—A Collection of Illuminating Essays, ed. V. B. Meyer-Rochow (Kerala: Research Signpost), 161–200.
De Cock, R., Faust, L., and Lewis, S. (2014). Courtship and mating in Phausis reticulata: Male flight behaviors, female glow displays, and male attraction to light traps. Fla. Entomol. 97, 1290–1307. doi: 10.1653/024.097.0404
De Cock, R., and Matthysen, E. (2001). Do glow-worm larvae use warning coloration? Ethology 107, 1019–1033. doi: 10.1046/j.1439-0310.2001.00746.x
De Cock, R., and Matthysen, E. (2005). Sexual communication by pheromones in a firefly. Phosphaenus hemipterus (Coleoptera: Lampyridae). Anim. Behav. 70, 807–818. doi: 10.1016/j.anbehav.2005.01.011
Demary, K., Michaelidis, C. I., and Lewis, S. M. (2005). Firefly courtship: Behavioral and morphological predictors of male mating success in Photinus greeni. Ethology 112, 485–492. doi: 10.1111/j.1439-0310.2005.01176.x
Dreisig, H. (1978). The circadian rhythm of bioluminescence in the glowworm, Lampyris noctiluca L. Behav. Ecol. Sociobiol. 3, 1–18. doi: 10.1007/BF00300044
Eguchi, E., Nemoto, A., Meyer-Rochow, V. B., and Ohba, N. (1984). A comparative study of spectral sensitivity curves in three diurnal and eight nocturnal species of Japanese fireflies. J. Insect Physiol. 30, 607–612. doi: 10.1016/0022-1910(84)90044-1
Eisner, T., Goetz, M. A., Hill, D. E., Smedley, S. R., and Meinwald, J. (1997). Firefly “femmes fatales” acquire defensive steroids (lucibufagins) from their firefly prey. Proc. Natl. Acad. Sci. U.S.A. 94, 9723–9728. doi: 10.1073/pnas.94.18.9723
Elgert, C., Hopkins, J., Kaitala, A., and Candolin, U. (2020). Reproduction under light pollution: Maladaptive response to spatial variation in artificial light in a glow-worm. Proc. R. Soc. B 287:20200806. doi: 10.1098/rspb.2020.0806
Elgert, C., Lehtonen, T. K., Kaitala, A., and Candolin, U. (2021a). Sexual selection for bright females prevails under light pollution. Curr. Zool. 67, 329–331. doi: 10.1093/cz/zoaa071
Elgert, C., Lehtonen, T. K., Kaitala, A., and Candolin, U. (2021b). The duration of artificial light defines sexual signalling in the common glow-worm. Behav. Ecol. Sociobiol. 75:154. doi: 10.1007/s00265-021-03093-2
Elvidge, C. D., Keith, D. M., Tuttle, B. T., and Baugh, K. E. (2010). Spectral identification of lighting type and character. Sensors 10, 3961–3988. doi: 10.3390/s100403961
Falchi, F., Cinzano, P., Duriscoe, D., Kyba, C. C. M., Elvidge, C. D., Baugh, K., et al. (2016). The new world atlas of artificial night sky brightness. Sci Adv 2:e1600377. doi: 10.1126/sciadv.1600377
Fallon, C. E., Walker, A. C., Lewis, S., Cicero, J., Faust, L., Heckscher, C. M., et al. (2021). Evaluating firefly extinction risk: Initial red list assessments for North America. PLoS One 16:e0259379. doi: 10.1371/journal.pone.0259379
Faust, L. (2012). Fireflies in the snow: Observations on two early-season arboreal fireflies Ellychnia corrusca and Pyractomena borealis. Lampyrid 2, 48–71.
Faust, L. F. (2017). Fireflies, Glow-worms, and Lightning Bugs. Athens: University of Georgia Press.
Firebaugh, A., and Haynes, K. J. (2016). Experimental tests of light-pollution impacts on nocturnal insect courtship and dispersal. Oecologia 182, 1203–1211. doi: 10.1007/s00442-016-3723-1
Firebaugh, A., and Haynes, K. J. (2019). Light pollution may create demographic traps for nocturnal insects. Basic Appl. Ecol. 34, 118–125. doi: 10.1016/j.baae.2018.07.005
Fu, X., Benno Meyer-Rochow, V., Tyler, J., Suzuki, H., and De Cock, R. (2009). Structure and function of the eversible organs of several genera of larval firefly. Chemoecology 19, 155–168. doi: 10.1007/s00049-009-0016-8
Fu, X. H., and Ballantyne, L. (2008). Taxonomy and behaviour of lucioline fireflies (Coleoptera: Lampyridae: Luciolinae) with redefinition and new species of Pygoluciola Wittmer from mainland China and review of Luciola LaPorte. Zootaxa 1733:1. doi: 10.11646/zootaxa.1733.1.1
Gardiner, T., and Didham, R. K. (2020). Glowing, glowing, gone? Monitoring long-term trends in glow-worm numbers in south-east England. Insect Conserv. Divers. 13, 162–174. doi: 10.1111/icad.12407
Gardiner, T., and Didham, R. K. (2021). Glow-worm abundance declines with increasing proximity to artificial lighting. Entomol. Gaz. 72, 1–11. doi: 10.31184/G00138894.721.1798
Gaston, K. J., Davies, T. W., Nedelec, S. L., and Holt, L. A. (2017). Impacts of artificial light at night on biological timings. Annu. Rev. Ecol. Evol. Syst. 48, 49–68. doi: 10.1146/annurev-ecolsys-110316-022745
Goh, K.-S., Lee, C.-M., and Wang, T.-Y. (2022). Species-specific flash patterns track the nocturnal behavior of sympatric Taiwanese fireflies. Biology 11:58. doi: 10.3390/biology11010058
Gunn, P., and Gunn, B. (2012). Lunar effects on the bioluminescent activity of the glow-worm Lampyris noctiluca and its larvae. Lampyrid 3, 1–16.
Hagen, O., Santos, R. M., Schlindwein, M. N., and Viviani, V. R. (2015). Artificial night lighting reduces firefly occurrence in Sorocaba, Brazil. Adv. Entomol. 03, 24–32. doi: 10.4236/ae.2015.31004
Hall, D. W., Sander, S. E., Pallansch, J. C., and Stanger-Hall, K. F. (2016). The evolution of adult light emission color in North American fireflies. Evolution 70, 2033–2048. doi: 10.1111/evo.13002
Hariyama, T. (2000). The brain as a photoreceptor: Intracerebral ocelli in the firefly. Naturwissenschaften 87, 327–330. doi: 10.1007/s001140050732
Harvey, J. A., Heinen, R., Armbrecht, I., Basset, Y., Baxter-Gilbert, J. H., Bezemer, T. M., et al. (2020). International scientists formulate a roadmap for insect conservation and recovery. Nat. Ecol. Evol. 4, 174–176. doi: 10.1038/s41559-019-1079-8
Haynes, K. J., and Robertson, B. A. (2021). A transdisciplinary research agenda for understanding insect responses to ecological light pollution informed by evolutionary trap theory. Curr. Opin. Insect. Sci. 45, 91–96. doi: 10.1016/j.cois.2021.02.004
Hopkins, G. R., Gaston, K. J., Visser, M. E., Elgar, M. A., and Jones, T. M. (2018). Artificial light at night as a driver of evolution across urban-rural landscapes. Front. Ecol. Environ. 114:8951. doi: 10.1002/fee.1828
Hopkins, J., Baudry, G., Candolin, U., and Kaitala, A. (2015). I’m sexy and I glow it: Female ornamentation in a nocturnal capital breeder. Biol. Lett. 11:20150599. doi: 10.1098/rsbl.2015.0599
Hopkins, J., Lehtonen, T. K., Baudry, G., and Kaitala, A. (2021). Costly mating delays drive female ornamentation in a capital breeder. Ecol. Evol. 11, 8863–8868. doi: 10.1002/ece3.7719
Horne, J., Horne, A., and Tyler, J. (2017). The influence of female body weight on egg production in the glow-worm Lampyris noctiluca (Coleoptera: Lampyridae). Lampyrid 4, 36–39.
Ineichen, S., and Rüttimann, B. (2012). Impact of artificial light on the distribution of the common European glow-worm, Lampyris noctiluca. Lampyrid 2, 31–36.
Jaikla, S., Lewis, S. M., Thancharoen, A., and Pinkaew, N. (2020a). Distribution, abundance, and habitat characteristics of the congregating firefly, Pteroptyx Olivier in Thailand. J. Asia-Pacific Biodivers. 13, 358–366. doi: 10.1016/j.japb.2020.06.002
Jaikla, S., Thancharoen, A., and Pinkaew, N. (2020b). Biology and rearing technique for the mangrove firefly, Pteroptyx valida, with discussion of additional instar in female. J. Asia-Pacific Biodivers. 13, 367–371. doi: 10.1016/j.japb.2020.05.002
Johnsen, S., Widder, E. A., and Mobley, C. D. (2004). Propagation and perception of bioluminescence: Factors affecting counterillumination as cryptic. Biol. Bull. 207, 1–16. doi: 10.2307/1543624
Jusoh, W. F. A., Ballantyne, L., Lambkin, C. L., Hashim, N. R., and Wahlberg, N. (2018). The firefly genus Pteroptyx Olivier revisited (Coleoptera: Lampyridae: Luciolinae). Zootaxa 4456, 1–71. doi: 10.11646/zootaxa.4456.1.1
Jusoh, W. F. A. W., and Hashim, N. R. (2012). The effect of habitat modification on firefly populations at the Rembau-Linggi estuary, Peninsular Malaysia. Lampyrid 2, 149–155.
Kakehashi, K., Kuranishi, R. B., and Kamata, N. (2014). Estimation of dispersal ability responding to environmental conditions: Larval dispersal of the flightless firefly, Luciola parvula. Ecol. Res. 29, 779–787. doi: 10.1007/s11284-014-1156-z
Kaufmann, T. (1965). Ecological and biological studies on the West African firefly Luciola discicollis (Coleoptera: Lampyridae). Ann. Entomol. Soc. Am. 58, 414–426. doi: 10.1093/aesa/58.4.414
Khattar, G., Vaz, S., Pereira Braga, P. H., Macedo, M., and da Silveira, L. F. L. (2022). Life history traits modulate the influence of environmental stressors on biodiversity: The case of fireflies, climate and artificial light at night. Divers. Distrib. 0, 1–12. doi: 10.1111/ddi.13584
Khoo, V., Kirton, L. G., and Nada, B. (2016). Firefly conservation: Monitoring the synchronous fireflies of the Selangor River in Malaysia. Antenna 40, 46–51.
Kim, T.-S., Kwon, K., and Jang, G.-S. (2021). Variations in the distribution and genetic relationships among Luciola unmunsana populations in South Korea. Land 10:730. doi: 10.3390/land10070730
Kivelä, L. (2022). The Effect of Artificial Light Spectrum on Mate Attraction in the Common Glow-Worm, Lampyris noctiluca. Helsinki: University of Helsinki.
Kok, P. J. R., van Doorn, L., and Dezfoulian, R. (2019). Predation by non-bioluminescent firefly larvae on a tepui-summit endemic toad. Curr. Biol. 29, R1170–R1171. doi: 10.1016/j.cub.2019.10.001
Krivoruchko, K., Goldshtein, A., Boonman, A., Eitan, O., Ben-Simon, J., Thong, V. D., et al. (2021). Fireflies produce ultrasonic clicks during flight as a potential aposematic anti-bat signal. iScience 24:102194. doi: 10.1016/j.isci.2021.102194
Kyba, C. C. M., Mohar, A., and Posch, T. (2017). How bright is moonlight? A&G 58, 31–31. doi: 10.1093/astrogeo/atx025
Kyba, C. C. M., Tong, K. P., Bennie, J., Birriel, I., Birriel, J. J., Cool, A., et al. (2015). Worldwide variations in artificial skyglow. Sci. Rep. 5:8409. doi: 10.1038/srep08409
Lall, A. B. (1981). Electroretinogram and the spectral sensitivity of the compound eyes in the firefly Photuris versicolor: A correspondence between green sensitivity and species bioluminescence emission. J. Insect Physiol. 27, 461–468. doi: 10.1016/0022-1910(81)90097-4
Lall, A. B. (1993). Nightly increase in visual sensitivity correlated with bioluminescent flashing activity in the firefly Photuris versicolor. J. Exp. Zool. 265, 609–612. doi: 10.1002/jez.1402650518
Lall, A. B., Chapman, R. M., Ovid Trouth, C., and Holloway, J. A. (1980a). Spectral mechanisms of the compound eye in the firefly Photinus pyralis. J. Comp. Physiol. 135, 21–27. doi: 10.1007/BF00660178
Lall, A. B., Seliger, H. H., Biggley, W. H., and Lloyd, J. E. (1980b). Ecology of colors of firefly bioluminescence. Science 210, 560–562. doi: 10.1126/science.210.4469.560
Lall, A. B., Cronin, T. W., Bechara, E. J. H., Costa, C., and Viviana, V. R. (2009). “Visual ecology of bioluminescent beetles: Visual spectral mechanisms and the colors of optical signaling in Coleoptera, Elateroidea: Lampyridae, Elateridae and Phengodidae,” in Bioluminescence in Focus - A Collection of Illuminating Essays, Ed. V. B. Meyer-Rochow (Kerala: Research Signpost).
Leavell, B. C., Rubin, J. J., McClure, C. J. W., Miner, K. A., Branham, M. A., and Barber, J. R. (2018). Fireflies thwart bat attack with multisensory warnings. Sci. Adv. 4:eaat6601. doi: 10.1126/sciadv.aat6601
Lehtonen, T. K., Babic, N. L., Piepponen, T., Valkeeniemi, O., Borshagovski, A.-M., and Kaitala, A. (2021). High road mortality during female-biased larval dispersal in an iconic beetle. Behav. Ecol. Sociobiol. 75:26. doi: 10.1007/s00265-020-02962-6
Lewis, S. M. (2009). “Bioluminescence and sexual signaling in fireflies,” in Bioluminescence in Focus: A Collection of Illuminating Essays, ed. V. B. Meyer-Rochow (Kerala: Research Signpost).
Lewis, S. M., and Cratsley, C. K. (2008). Flash signal evolution, mate choice, and predation in fireflies. Annu. Rev. Entomol. 53, 293–321. doi: 10.1146/annurev.ento.53.103106.093346
Lewis, S. M., Faust, L., and De Cock, R. (2011). The dark side of the light show: Predators of fireflies in the Great Smoky Mountains. Psyche 2012:634027. doi: 10.1155/2012/634027
Lewis, S. M., and Owens, A. C. S. (2019). A critique of Firebaugh & Haynes (2018) - Light pollution may create demographic traps for nocturnal insects. Basic Appl. Ecol. 35, 65–66. doi: 10.1016/j.baae.2019.01.002
Lewis, S. M., Thancharoen, A., Wong, C. H., López-Palafox, T., Santos, P. V., Wu, C., et al. (2021). Firefly tourism: Advancing a global phenomenon toward a brighter future. Conservat. Sci. Prac. 3:e391. doi: 10.1111/csp2.391
Lewis, S. M., Wong, C. H., Owens, A. C. S., Fallon, C., Jepsen, S., Thancharoen, A., et al. (2020). A global perspective on firefly extinction threats. BioScience 70, 157–167. doi: 10.1093/biosci/biz157
Lloyd, J. E. (1972). Chemical communication in fireflies. Environ. Entomol. 1, 265–266. doi: 10.1093/ee/1.2.265
Lloyd, J. E. (1973). Model for the mating protocol of synchronously flashing fireflies. Nature 245, 268–270. doi: 10.1038/245268a0
Lloyd, J. E. (1980). Male Photuris fireflies mimic sexual signals of their females’ prey. Science 210, 669–671. doi: 10.1126/science.210.4470.669
Lloyd, J. E. (2008). “Fireflies,” in Encyclopedia of Entomology, ed. J. L. Capinera (New York, NY: Springer), 1429–1452.
Lloyd, J. E., and Wing, S. R. (1983). Nocturnal aerial predation of fireflies by light-seeking fireflies. Science 222, 634–635. doi: 10.1126/science.222.4624.634
Lower, S. E., Stanger-Hall, K. F., and Hall, D. W. (2018). Molecular variation across populations of a widespread North American firefly, Photinus pyralis, reveals that coding changes do not underlie flash color variation or associated visual sensitivity. BMC Evol. Biol. 18:129. doi: 10.1186/s12862-018-1251-9
Luginbuhl, C. B., Lockwood, G. W., Davis, D. R., Pick, K., and Selders, J. (2009). From the ground up I: Light pollution sources in flagstaff. Arizona. Publ. Astro. Soc. Pac. 121, 185–203. doi: 10.1086/597625
Martin, G. J., Branham, M. A., Whiting, M. F., and Bybee, S. M. (2017). Total evidence phylogeny and the evolution of adult bioluminescence in fireflies. Mol. Phylogenet. Evol. 107, 564–575. doi: 10.1016/j.ympev.2016.12.017
Martin, G. J., Stanger-Hall, K. F., Branham, M. A., Da Silveira, L. F. L., Lower, S. E., Hall, D. W., et al. (2019). Higher-Level phylogeny and reclassification of Lampyridae (Coleoptera: Elateroidea). Insect. Syst. Divers. 3:11. doi: 10.1093/isd/ixz024
Murphy, F., and Moiseff, A. (2019). Anatomy of the stemmata in the Photuris firefly larva. J. Comp. Physiol. A Neuroethol. Sens. Neural Behav. Physiol. 205, 151–161. doi: 10.1007/s00359-018-01312-2
Murphy, F., and Moiseff, A. (2020). Ambient illumination influence on Photuris firefly larval surface movements is not mediated by the stemmata. J. Insect Behav. 33, 30–37. doi: 10.1007/s10905-020-09743-z
Oba, Y., and Kainuma, T. (2009). Diel changes in the expression of long wavelength-sensitive and ultraviolet-sensitive opsin genes in the Japanese firefly, Luciola cruciata. Gene 436, 66–70. doi: 10.1016/j.gene.2009.02.001
Oba, Y., and Schultz, D. T. (2014). Eco-evo bioluminescence on land and in the sea. Adv. Biochem. Eng. Biotechnol. 144, 3–36. doi: 10.1007/978-3-662-43385-0_1
Ohba, N. (2004). Flash communication systems of Japanese fireflies. Integr. Comp. Biol. 44, 225–233. doi: 10.1093/icb/44.3.225
Owens, A. C. S., Cochard, P., Durrant, J., Farnworth, B., Perkin, E. K., and Seymoure, B. (2020). Light pollution is a driver of insect declines. Biol. Conserv. 241:108259. doi: 10.1016/j.biocon.2019.108259
Owens, A. C. S., Dressler, C., and Lewis, S. M. (2022). Costs and benefits of “insect friendly” artificial lights are taxon specific. Oecologia 199, 487–497. doi: 10.1007/s00442-022-05189-6
Owens, A. C. S., and Lewis, S. M. (2018). The impact of artificial light at night on nocturnal insects: A review and synthesis. Ecol. Evol. 8, 11337–11358. doi: 10.1002/ece3.4557
Owens, A. C. S., and Lewis, S. M. (2021a). Effects of artificial light on growth, development, and dispersal of two North American fireflies. J. Insect Physiol. 130:104200. doi: 10.1016/j.jinsphys.2021.104200
Owens, A. C. S., and Lewis, S. M. (2021b). Narrow-spectrum artificial light silences female fireflies. Insect Conserv. Divers. 14, 199–210. doi: 10.1111/icad.12487
Owens, A. C. S., and Lewis, S. M. (2022). Artificial light impacts the mate success of female fireflies. R. Soc. Open Sci. 9:220468. doi: 10.1098/rsos.220468
Owens, A. C. S., Meyer-Rochow, V. B., and Yang, E.-C. (2018). Short- and mid-wavelength artificial light influences the flash signals of Aquatica ficta fireflies. PLoS One 13:e0191576. doi: 10.1371/journal.pone.0191576
Pacheco, Y. M., Martin, G. J., and Bybee, S. M. (2016). On the phototactic response of Rwandan Diaphanes Motschulsky to a trap with a 630 nm red light. Coleopt. Bull. 70, 559–561. doi: 10.1649/0010-065X-70.3.559
Powell, G. S., Saxton, N. A., Pacheco, Y. M., Stanger-Hall, K. F., Martin, G. J., Kusy, D., et al. (2022). Beetle bioluminescence outshines extant aerial predators. Proc. R. Soc. B. 289:20220821. doi: 10.1098/rspb.2022.0821
Prasertkul, T. (2018). Characteristics of Pteroptyx firefly congregations in a human dominated habitat. J. Insect Behav. 31, 436–457. doi: 10.1007/s10905-018-9687-8
Robertson, B. A., Campbell, D.-R., Durovich, C., Hetterich, I., Les, J., and Horváth, G. (2017). The interface of ecological novelty and behavioral context in the formation of ecological traps. Behav. Ecol. 28, 1166–1175. doi: 10.1093/beheco/arx081
Sánchez de Miguel, A., Bennie, J., Rosenfeld, E., Dzurjak, S., and Gaston, K. J. (2021). First estimation of global trends in nocturnal power emissions reveals acceleration of light pollution. Remote Sens. 13:3311. doi: 10.3390/rs13163311
Sander, S. E., and Hall, D. W. (2015). Variation in opsin genes correlates with signalling ecology in North American fireflies. Mol. Ecol. 24, 4679–4696. doi: 10.1111/mec.13346
Santangelo, J. S., Ness, R. W., Cohan, B., Fitzpatrick, C. R., Innes, S. G., Koch, S., et al. (2022). Global urban environmental change drives adaptation in white clover. Science 375, 1275–1281. doi: 10.1126/science.abk0989
Sarfati, R., Gaudette, L., Cicero, J. M., and Peleg, O. (2022). Statistical analysis reveals the onset of synchrony in sparse swarms of Photinus knulli fireflies. J. R. Soc. Interface 19:20220007. doi: 10.1098/rsif.2022.0007
Sarfati, R., Hayes, J. C., and Peleg, O. (2021). Self-organization in natural swarms of Photinus carolinus synchronous fireflies. Sci. Adv. 7:eabg9259. doi: 10.1126/sciadv.abg9259
Schultz, C. B., Haddad, N. M., Henry, E. H., and Crone, E. E. (2019). Movement and demography of at-risk butterflies: Building blocks for conservation. Annu. Rev. Entomol. 64, 167–184. doi: 10.1146/annurev-ento-011118-112204
Schwalb, H. H. (1961). Contributions to the biology of the domestic lampyridae Lampyris noctiluca and Phausis splendidula and experimental analysis of their predatory and sexual behavior. Zoologiscbee Jahrbuch 88, 99–550.
Sharkey, C. R., Fujimoto, M. S., Lord, N. P., Shin, S., McKenna, D. D., Suvorov, A., et al. (2017). Overcoming the loss of blue sensitivity through opsin duplication in the largest animal group, beetles. Sci. Rep. 7:8. doi: 10.1038/s41598-017-00061-7
Smith, S. R. (2011). Changes in visual sensitivity as a function of bioluminescent flashing activity period in firefly species. Washington, DC: Howard University.
Souto, P. M., Campello, L., Khattar, G., Miras Mermudes, J. R., Monteiro, R. F., and Lima da Silveira, L. F. (2019). How to design a predatory firefly? Lessons from the Photurinae. Zool. Anz. 278, 1–13. doi: 10.1016/j.jcz.2018.10.006
Stanger-Hall, K. F., and Lloyd, J. E. (2015). Flash signal evolution in Photinus fireflies: Character displacement and signal exploitation in a visual communication system. Evolution 69, 666–682. doi: 10.1111/evo.12606
Stanger-Hall, K. F., Sander Lower, S. E., Lindberg, L., Hopkins, A., Pallansch, J., and Hall, D. W. (2018). The evolution of sexual signal modes and associated sensor morphology in fireflies. Proc. R. Soc. B 285:20172384. doi: 10.1098/rspb.2017.2384
Stevens, M., and Ruxton, G. D. (2012). Linking the evolution and form of warning coloration in nature. Proc. Biol. Sci. 279, 417–426. doi: 10.1098/rspb.2011.1932
Stewart, A. J. A., Perl, C. D., and Niven, J. E. (2020). Artificial lighting impairs mate attraction in a nocturnal capital breeder. J. Exp. Biol. 223:jeb229146. doi: 10.1242/jeb.229146
Suzuki, H., Sato, Y., Ohba, N., Bae, J.-S., Jin, B.-R., Sohn, H.-D., et al. (2004). Phylogeographic analysis of the firefly, Luciola lateralis, in Japan and Korea based on mitochondrial cytochrome oxidase II gene sequences (Coleoptera: Lampyridae). Biochem. Genet. 42, 287–300. doi: 10.1023/B:BIGI.0000039805.75118.8f
Thancharoen, A. (2007). The Biology and Mating Behaviour of An Aquatic Firefly Species, Luciola aquatilis sp. nov. Thancharoen (Coleoptera: Lampyridae). unpublished Ph.D thesis, Mahidol University, Bangkok.
Thancharoen, A., and Masoh, S. (2019). “Effect of camera illumination on flashing behavior of Pteroptyx malaccae,” in Bioluminescence, Ed. K. Sung-Bae (Berlin: Springer). doi: 10.5772/intechopen.85796
Tyler, J. (2013). What do glow-worms do on their day off? The diurnal behaviour of adult Lampyris noctiluca (L.). Lampyrid 3, 23–34.
Tyler, J., Mckinnon, W., Lord, G. A., and Hilton, P. J. (2008). A defensive steroidal pyrone in the glow-worm Lampyris noctiluca L. (Coleoptera: Lampyridae). Physiol. Entomol. 33, 167–170. doi: 10.1111/j.1365-3032.2007.00610.x
Van den Broeck, M., De Cock, R., Van Dongen, S., and Matthysen, E. (2021a). Blinded by the light: Artificial light lowers mate attraction success in female glow-worms. Insects 12:734. doi: 10.3390/insects12080734
Van den Broeck, M., De Cock, R., Van Dongen, S., and Matthysen, E. (2021b). White LED light intensity, but not colour temperature, interferes with mate-finding by glow-worm males. J. Insect Conserv. 25, 339–347. doi: 10.1007/s10841-021-00304-z
van der Kooi, C. J., Stavenga, D. G., Arikawa, K., Belušič, G., and Kelber, A. (2020). Evolution of insect color vision: From spectral sensitivity to visual ecology. Annu. Rev. Entomol. 66, 23.1–23.38. doi: 10.1146/annurev-ento-061720-071644
Vaz, S., Manes, S., Gama-Maia, D., Silveira, L., Mattos, G., Paiva, P. C., et al. (2021). Light pollution is the fastest growing potential threat to firefly conservation in the Atlantic Forest hotspot. Insect Conserv. Divers. 14, 211–224. doi: 10.1111/icad.12481
Vencl, F. V., and Carlson, A. D. (1998). Proximate mechanisms of sexual selection in the firefly Photinus pyralis. J. Insect Behav. 11, 191–207. doi: 10.1023/A:1021091806472
Wanjiru Mbugua, S., Hay Wong, C., and Ratnayeke, S. (2020). Effects of artificial light on the larvae of the firefly Lamprigera sp. in an urban city park, Peninsular Malaysia. J. Asia. Pac. Entomol. 23, 82–85. doi: 10.1016/j.aspen.2019.10.005
Warrant, E. J. (2017). The remarkable visual capacities of nocturnal insects: Vision at the limits with small eyes and tiny brains. Philos. Trans. R. Soc. Lond. B Biol. Sci. 372:20160063. doi: 10.1098/rstb.2016.0063
Wing, S. R. (1989). Energetic costs of mating in a flightless female firefly, Photinus collustrans. J. Insect Behav. 2, 841–847. doi: 10.1007/BF01049405
Wong, C. H., and Yeap, C. A. (2012). Conservation of congregating firefly zones (CFZs) in peninsular Malaysia. Lampyrid 2, 174–187.
Keywords: Lampyridae, bioluminescence, firefly, light pollution, artificial light at night (ALAN)
Citation: Owens ACS, Van den Broeck M, De Cock R and Lewis SM (2022) Behavioral responses of bioluminescent fireflies to artificial light at night. Front. Ecol. Evol. 10:946640. doi: 10.3389/fevo.2022.946640
Received: 17 May 2022; Accepted: 06 September 2022;
Published: 27 September 2022.
Edited by:
Rachael E. Bonoan, Providence College, United StatesReviewed by:
Maria Albo, Universidad de la República, UruguayArja Kaitala, University of Oulu, Finland
Copyright © 2022 Owens, Van den Broeck, De Cock and Lewis. This is an open-access article distributed under the terms of the Creative Commons Attribution License (CC BY). The use, distribution or reproduction in other forums is permitted, provided the original author(s) and the copyright owner(s) are credited and that the original publication in this journal is cited, in accordance with accepted academic practice. No use, distribution or reproduction is permitted which does not comply with these terms.
*Correspondence: Mira Van den Broeck, Mira.VandenBroeck@uantwerpen.be
†These authors have contributed equally to this work