- 1Laboratorio de Insectos de Importancia Agronómica, Instituto de Genética “Ewald A. Favret”, CICVyA, Instituto Nacional de Tecnología Agropecuaria-GV- Instituto de Agrobiotecnología y Biología Molecular, Consejo Nacional de Investigaciones Científicas y Técnicas, Buenos Aires, Argentina
- 2Laboratorio de Biomoléculas, Centro de Investigación en Hidratos de Carbono, Consejo Nacional de Investigaciones Científicas y Técnicas, Buenos Aires, Argentina
- 3Cátedra de Biomoléculas, Facultad de Agronomía, Universidad de Buenos Aires, Buenos Aires, Argentina
- 4Departamento de Ecología, Genética y Evolución, Facultad de Ciencias Exactas y Naturales, Universidad de Buenos Aires, Buenos Aires, Argentina
- 5Instituto de Ecología, Genética y Evolución de Buenos Aires, Consejo Nacional de Investigaciones Científicas y Técnicas, Buenos Aires, Argentina
- 6Cátedra de Terapéutica Vegetal, Facultad de Agronomía y Zootecnia, Universidad Nacional de Tucumán, San Miguel de Tucumán, Argentina
- 7Consejo Nacional de Investigaciones Científicas y Técnicas, Buenos Aires, Argentina
In some tephritid fruit flies, exposure to volatile compounds from host plants increases male sexual success. This phenomenon has been used to boost sterile males’ sexual competitiveness in the framework of the sterile insect technique (SIT). Previous studies revealed that males of Anastrepha fraterculus (Diptera: Tephritidae) exposed to volatiles from guava (Psidium guajava) fruit (GF) and guava essential oil (GEO) exhibit intensified courtship behavior and have greater copulatory success relative to unexposed males. Similar results were achieved in these flies through exposure to moradillo (Schinus polygama) essential oil or lemon (Citrus limon) essential oil. To identify the responsible compounds involved in these effects, we compared the volatile chemical profiles of GF, GEO, moradillo essential oil, and lemon essential oil. We selected five candidate compounds: (E)-β-ocimene, (Z)-β-ocimene, limonene, β-caryophyllene, and α-humulene. Using the electroantennographic detection (EAD) technique, we verified that males are able to detect all the candidate compounds and built dose-response curves between 0.01 and 100 μg/μl for each compound. We confirmed a stimulating effect on the courtship behavior of males for (E/Z)-β-ocimene and (R)-limonene, whereas β-caryophyllene and α-Humulene did not affect male courtship behavior. For those compounds that sexually stimulated males, we found a dose-dependent effect. Males’ behavioral response to the semiochemicals was maximum when (R)-limonene was combined with (E/Z)-β-ocimene, but the response was reduced when β-caryophyllene and α-humulene were included, which suggests some sort of negative interaction between them. Our results may contribute to the ongoing development of the SIT in this species.
Introduction
Many tephritid species exhibit a lek mating system in which males aggregate in specific sites to attract or court females (Arita and Kaneshiro, 1989; Díaz-Fleischer and Aluja, 1999). This calling behavior consists of emitting acoustical, visual, and chemical signals, and involves the display of energetically expensive behaviors to release pheromones (e.g., wing fanning and expansion of salivary glands) (Yuval et al., 1998; Sivinski et al., 1999). Males’ attractiveness and mating success positively correlate with the calling effort (Whittier et al., 1994; Segura et al., 2007; Niyazi et al., 2008). Therefore, the male capability to synchronize calling activity with the availability of food, among other suitable environmental conditions, is expected to be critical to improving male fitness. In this sense, volatile organic compounds (VOCs) from hosts could act not only as conductive feeding, oviposition, and rendezvous sites (Landolt and Phillips, 1997; Tumlinson, 2014; Moreau et al., 2017; Xu and Turlings, 2018) but also as environmental signals that trigger the synthesis/release of pheromones or switch on sexual behaviors (Reddy and Guerrero, 2004; Borrero-Echeverry et al., 2018). In fact, host plant VOCs stimulate calling behavior and sexual success in a number of tephritid fruit fly species (reviewed by Segura et al., 2018). For example, Ceratitis capitata males increase their mating success when they are exposed to α-copaene, a phytochemical widely distributed among C. capitata host plants (Shelly, 2001). This compound also acts as a long-distance cue for male aggregation in lekking sites (Nishida et al., 2000). Similarly, the male mating success of Bactrocera oleae increases when males are exposed to α-pinene, a compound emitted by olive fruit, the only host of this species (Gerofotis et al., 2013; Kokkari et al., 2021). The stimulating effects of these plant VOCs may have probably evolved during the adaptation of these flies to the exploitation of their hosts. In addition, in some cases, the same compounds released by host plants are also present in males’ pheromonal blends (Segura et al., 2018). For example, α-pinene and α-copaene are found in the sex pheromone blend of B. oleae and C. capitata, respectively (Mazomenos and Haniotakis, 1981; Vaníèková et al., 2012). This is in line with the sensory exploitation hypothesis, according to which host-like pheromone odors have evolved to exploit females’ pre-existing sensory bias toward host fruit odors (Ryan, 1990).
Anastrepha, like Ceratitis and Bactrocera, is a tephritid genus of fruit flies. However, only a few studies have evaluated male sexual responses to VOCs exposure in Anastrepha (Segura et al., 2018). Volatiles from ginger root oil (GRO), which majorly contain α-copaene, were tested on several Anastrepha species, namely Anastrepha fraterculus, Anastrepha ludens, Anastrepha obliqua, and Anastrepha serpentina (Flores et al., 2011; Ruiz et al., 2021). However, except for A. serpentina, male mating success was not enhanced by the exposure to GRO volatiles in these species. On the other hand, fruits of preferred host plants have provided better sources of sexually stimulating VOCs in these flies (Vera et al., 2013; Bachmann et al., 2015; Morató et al., 2015). For instance, A. ludens mating success is increased in males exposed to volatiles from grapefruit essential oil (Morató et al., 2015).
Anastrepha fraterculus sp. 1 morphotype constitutes another example of tephritid flies that are sexually stimulated by host plant VOCs. This species belongs to the Anastrepha fraterculus cryptic species complex, which has a wide distribution range in the American continent (Hernández-Ortiz et al., 2019) and generates a high economic impact on the agribusiness of this region (Cladera et al., 2014; De León et al., 2021). A recent review of their natural hosts showed that, despite the differences in the host range used by different populations at the regional level, the native Psidium guajava is the only host shared by all populations (Hernández-Ortiz et al., 2019). Therefore, the chemosensory system of A. fraterculus is likely adapted to perceive VOCs from guava fruit as an environmental cue for host availability or the localization of sexual mates. In fact, previous studies revealed that males exposed to volatiles from guava (P. guajava) fruit (GF) perform calling behavior more frequently, release a higher amount of sex pheromone, and achieve a higher copulatory success relative to unexposed males (Vera et al., 2013; Bachmann et al., 2015). Similar results were obtained when males were exposed to a commercial guava essential oil (GEO) (Belliard et al., 2022) or a blend of seven representative compounds of GF [(R)-limonene, β-myrcene, (E)-β-ocimene, α-humulene, E-2-hexenal, ethyl butanoate, and ethyl hexanoate], although the effect of the blend was lower compared to the GF effect (Bachmann et al., 2015). Knowing which of these compounds are responsible for stimulating A. fraterculus male sexual behavior could help to understand this phenomenon and further evaluate its potential for pest management.
Recently, Ruiz et al. (2021) assessed the effect of odors from seven essential oils, from exotic hosts and non-host plants, as well as two standard compounds [(R)-limonene 98% and citral (mixture of neral and geranial) 95%] on A. fraterculus male mating success. They found an increased male mating success when males were exposed to lemon (Citrus limon) essential oil or moradillo (Schinus polygama) essential oil. In addition, when males were exposed to R-limonene, the major compound of lemon essential oil (LO), they achieved a higher number of mates. However, when males were exposed to grapefruit (Citrus paradisi) essential oil (GFO) and orange (Citrus sinensis) essential oil (OO), which also contain R-limonene as the major compound, their sexual success was not affected (Ruiz et al., 2021). The limonene relative abundance varies between citrus species (González-Mas et al., 2019), and detrimental effects have been shown for tephritid flies exposed to sources with high concentrations of this compound (Salvatore et al., 2004; Papachristos et al., 2009; Oviedo et al., 2020; Zeni et al., 2021). Thus, limonene’s effect on male sexual behavior appears to be dose-dependent, but it may also depend on the presence of other compounds. Guava and moradillo are native plants with marked differences in their chemical composition (Soares et al., 2007; Jofré Barud, 2018). The fact that volatiles from these fruits, for which limonene is not a major compound, act as male sexual enhancers in A. fraterculus (Vera et al., 2013; Bachmann et al., 2015; Ruiz et al., 2021; Belliard et al., 2022) suggests that other compounds should be involved.
Due to the economic impact of A. fraterculus, diverse control methods are under development, including the sterile insect technique (SIT) (Cladera et al., 2014; Mastrangelo et al., 2018, 2019, 2021; De León et al., 2021). This technique involves the field release of mass-reared sterile males, which must achieve mating with wild females to compromise the reproductive output of the wild population (Knipling, 1955). Therefore, the success of the SIT strongly depends on the sterile males’ sexual competitiveness. In this context, phytochemicals have been used in the framework of the SIT to boost male sexual competitiveness in other fruit fly pests (Hendrichs, 2000; Enkerlin, 2005; Pereira et al., 2013; Enkerlin et al., 2017). For example, the exposure of C. capitata sterile males to volatile compounds of ginger root oil (GRO) is currently being used to increase their sexual competitiveness (Pereira et al., 2021). The identification of α-copaene as a stimulator of male sexual behavior in this species (Nishida et al., 2000; Shelly, 2001) was key to find GRO (which is rich in α-copaene) as an efficient and easy-to-use source of stimulating phytochemicals (Shelly T. E. et al., 2004). Transferring this methodology to A. fraterculus or other pests implies finding compounds capable of stimulating male sexual behavior and mating success. Therefore, studying the volatile compounds released from host plants and present in the sex pheromone blend can be a good approximation to identify candidate stimulating compounds.
The goal of this study was to identify candidate compounds involved in the exacerbated male sexual behavior that follows exposure to guava volatiles in A. fraterculus, providing insights from applied and evolutionary perspectives. We characterized the volatiles released by GF and GEO through chromatography coupled to mass spectrometry (GC-MS) and searched for common compounds. We selected candidate compounds after comparing our findings with the previously published chemical profiles of the essential oils tested by Ruiz et al. (2021). In addition, we used electroantennographic detection (EAD) to confirm that males are able to detect these candidate compounds and GC-MS to examine if these compounds are present among the volatiles released by A. fraterculus males. Furthermore, we performed bioassays to test if exposure to each candidate compound stimulates male sexual behavior, taking into account the possibility of dose-dependent and between-compounds interaction effects.
Materials and methods
Insects
All insects employed in this study correspond to the Brazilian 1 morphotype of A. fraterculus sp. 1 and belong to a laboratory colony that was kept for ca. 200 generations (approximately 10 generations per year) at Instituto Nacional de Tecnología Agropecuaria (INTA, Buenos Aires, Argentina). The colony derived from pupae obtained from guavas (P. guajava) collected in Horco Molle (Tucumán, Argentina) in 1997. Assayed flies emerged from pupae kept in glass containers inside an incubator (Sanyo MLR350, Japan) at 25 ± 1°C and under a photoperiod of 12L:12D. Emerged females were discarded since we only address male calling behavior in this study.
Fruits, essential oil, and synthetic compounds
Guava fruits were collected in Horco Molle (Tucuman, Argentina) and shipped within 48 h to INTA (Buenos Aires, Argentina), while undiluted guava essential oil obtained from fruits by cold-pressed was purchased from Salvia Cosmeceuticals (Delhi, India). The synthetic compounds R-limonene (CAS 5989-27-5), β-caryophyllene (CAS 87-44-5), and β-ocimene E-Z isomers mix (CAS 13877-91-3) were purchased from Sigma-Aldrich (Saint Louis, MO, United States), while α-humulene (CAS 6753-98-6) was obtained from PhytoLab (Vestenbergsgreuth, Germany). All of them presented purities between 80 and 97%.
Volatile’s collection
In order to obtain volatile compounds from GF and GEO, a piece of 3 g of GF and 200 μl of GEO (dispensed on a filter paper) were placed separately in glass aeration chambers (46 cm long × 4 cm i.d.). An airstream (500 ml/min), filtered using activated charcoal, was pushed through the chambers for 4 h (9:30–13:30) at 25°C. In addition, empty glass aeration chambers and glass aeration chambers containing clean filter paper were used as negative controls for GF and GEO collections, respectively. Volatiles from each chamber were collected onto traps with 20 mg of Hayesep Q adsorbent (Grace, Deerfield, IL, United States). The same procedure was applied to collect volatile compounds released by A. fraterculus calling-males. In this case, 15 mature males were placed in each glass aeration chamber during the calling activity peak (8:30–12:30). Empty glass aeration chambers were used as blanks. Ten replicates were performed for each guava volatile source and seven replicates for calling males. Collected compounds were eluted from the traps using 200 μl of dichloromethane (HPCL grade, Sintorgan, Argentina) and stored at -20°C until chemical analysis.
Chemical characterization of volatile samples and selection of candidate compounds
Chemical characterization of guava and flies’ volatiles was performed by gas chromatography coupled to mass spectrometry detector (GC-MS, Agilent Technologies, CA, United States). The GC was equipped with an HP-5 capillary column (30 m length, 0.32 mm inner diameter and 0.25 μm film thickness; Agilent Technologies, CA, United States) and the MS was set in electron impact (EI) ionization at 70 eV mode. One microliter of each sample was injected at 240°C in splitless mode and Helium was used as the carrier gas at 0.7 ml/min (inlet pressure: 20.48 kPa). The oven temperature was programmed to increase at 10°C/min from 35°C (isothermal for 1 min) to 230°C and hold at 230°C for 15 min. GF and GEO compounds were identified by comparing their relative retention index (RI) with those reported in bibliography (Adams, 2007) and their mass spectra with those provided by NIST/EPA/NIH mass spectral library (NIST 08, National Institute of Standards and Technology, MD, United States). For each identified compound, its peak area was calculated and relativized to the total area of all identified compounds in the sample.
We considered as candidate compounds those present in both guava volatile profiles (GF and GEO) and also in volatile profiles of other odor sources that were shown to improve the mating success of A. fraterculus males through volatiles exposure (Ruiz et al., 2021). We confirmed the identity of candidate compounds by chromatographic analysis of synthetic standards. The chromatographic column that we used to identify guava’s volatiles does not separate limonene optical isomers (R and S). However, the detection of limonene was considered as indicative of the presence of R-limonene since this isomer was previously reported in EOs from guava and other Myrtaceae species (Siddique et al., 2015; Hassan et al., 2020).
Electroantennography
The olfactory response of A. fraterculus males was tested by electroantennography (EAG) for five dilutions (0.01, 0.1, 1, 10, 100 μg/μl) of each candidate compound using n-hexane (HPLC grade, Sintorgan, Argentina) as solvent.
For each replicate, a fresh male head was carefully excised and mounted through its base on the tip of a glass capillary with phosphate buffer saline solution (PBS) (137 mM NaCl, 2.7 mM KCl, 8 mM Na2HPO4 and 2 mM KH2PO4) containing the reference electrode. Using another PBS-filled capillary, the distal end of an antenna was contacted with the recording electrode. Electrodes were connected from their base to a signal amplifier (iDAC2; Syntech, Hilversum, Netherlands). The mounted antenna was placed under a stream of charcoal-filtered and humidified air (5 ml/min) which was delivered through a glass tube (1 cm i.d.). Five microliters of each sample were adsorbed onto a filter paper (2 cm × 1 cm; Whatman N1, Whatman, United Kingdom). After solvent evaporation, the filter paper was placed inside a Pasteur pipette. The tip of the Pasteur pipette was supported on a glass tube hole and the sample was injected into the airstream by an air puff from the base of the pipette. Using 1-hexanol (1μg/μl) as a positive standard and n-Hexane as the solvent control, samples were delivered to each antenna in the following order: positive standard, solvent control, different doses of the tested compound in increasing order, solvent control, and positive standard. Each stimulus was applied for 1 s and consecutive stimulus were separated in time by 60 s to prevent or minimize antennal adaptation. Eight to ten replicates were performed for each tested compound so each mounted antenna was assayed for only one candidate compound.
All males used in this assay were 8 to 12-day-old adults maintained in containers with water and food [3:1, sugar: brewer’s yeast (CALSA, Tucumaìn, Argentina)] ad libitum since their emergence.
Bioassay I: Male calling behavior after exposure to candidate compounds
In A. fraterculus, the time males spend calling within a lek positively correlates with mating success (Segura et al., 2007; Bachmann et al., 2015). Therefore, to survey the potential effect of exposure on male mating success, we observed calling behavior on males exposed to four different concentrations of each candidate compound. Based on the calling behavior described for A. fraterculus males (Gomez Cendra et al., 2011), we considered that males were calling when they were observed performing wing fanning and/or exposing its salivary glands and anal tissue. These behaviors have been used in our previous studied (Bachmann et al., 2015, 2019; Belliard et al., 2022) as indicative of male calling. We tested four doses as follows: 0 (solvent control), 1, 10, and 100 μg/μl. n-Hexane (HPLC grade, Sintorgan, Argentina) was used as solvent for the dilutions. The selection of these concentrations followed a pilot test in which we found (for limonene) that higher concentrations were detrimental for male calling activity.
Newly emerged males were transferred randomly in groups of five individuals to 3 L glass containers with water, where they were fed ad libitum [3:1, sugar: brewer’s yeast (CALSA, Tucumaìn, Argentina)]. Each group (i.e., replicate) was randomly assigned to one of the four possible doses. Exposure of males to different compound concentrations or solvent (control) was carried out for 4 h (12:00–16:00) for two days starting 10 days after males’ emergence. For this procedure, 20 μl of the assigned dilution were dispensed on a piece of filter paper (2 cm2), which was placed inside a plastic container covered with a mesh to prevent contact between the filter paper and the insects. The plastic container with the filter paper was then placed inside the glass container that housed the experimental males. When males were 12 days old, the number of calling males per group were recorded four times for 1 hour (8:30, 8:50, 9:10, and 9:30). We used three batches of flies (groups of flies obtained from eggs collected the same day from the laboratory colony), totalizing 24–25 replicates (7–9 per batch) per dose, for each candidate compound. The containers with males were kept inside an environmental chamber (Sanyo MLR350, Japan) at 25 ± 1°C and a photoperiod of 12L:12D. Four chambers were employed, one for each dose treatment. From batch to batch, treatment levels were rotated among chambers.
Bioassay II: Male calling behavior after exposure to selected volatile blends
In order to study possible interactions among compounds, we assayed exposure to two mixtures of candidate compounds. One of them contained all candidate compounds and the other contained only those that proved to increase male calling activity in bioassay I, which were limonene and β-ocimene. In both mixtures, each compound was used at the concentration at which it elicited the highest response in bioassay I. n-hexane and limonene (10 g/μl) were used as solvent control and a positive control, respectively. Exposure was performed as in bioassay I. Three batches of flies were used to assess 24 replicates (7–9 per batch) per treatment.
Data analysis
All statistical analyses were carried out in R (v 4.1.1; R Core Team, 2021). We used Agilent MassHunter Qualitative Analysis Software (B.06.00) to calculate relative areas of identified compounds from GC-MS assays. For the chemical characterization of volatile samples, we calculated descriptive statistics with stat.desc function from pastecs package (Grosjean and Ibanez, 2018).
For electroantennography analysis, the amplified signals were recorded, calculated, and normalized to the positive standard by using the Syntech software (v1.2.5, Syntech, Hilversum, Netherlands). For each candidate compound separately, the normalized EAG response was compared between n-hexane (solvent control) and each dilution. We applied a Restricted Maximum Likelihood (REML) approach for fitting a linear mixed-effects model with a Gaussian error structure using the lmer function from the lme4 and lmerTest packages (Bates et al., 2015; Kuznetsova et al., 2017). The model included the antenna as a random factor and the concentration as a within-antenna, categorical fixed factor. Model assumptions of normal distribution and homoscedasticity of residuals were evaluated with the shapiro.test function from the stats package (R Core Team, 2021) and leveneTest function from car package (Fox and Weisberg, 2019), respectively, and also graphically. In order to compare the male’s olfactory response between candidate compounds, the net normalized EAG response (i.e., absolute response minus solvent control response) was modeled with the nls function from the stats package (R Core Team, 2021). The response of each antenna was described as a function of concentration using the following two-parameter, first-order kinetic function: a × (1 - exp (-b × concentration)), where a represents the expected maximum response (at saturating dosages) and b represents the sensitivity of the response to pre-saturating concentration (Byers, 2013). As this function is strictly increasing, for those antennae that exhibited a response drop at the highest concentrations, the last response values needed to be adjusted prior to fitting the model; that is, each of the dropping records was replace with the drop values average so the drop was removed without affecting the antenna average response. Following parameter estimations, we compared both parameters separately across candidate compounds by performing Kruskal—Wallis tests using the kruskal.test function from the stats package (R Core Team, 2021).
To estimate the effects of each candidate compound at different doses (bioassay I) or mixture treatments (bioassay II) on calling activity, we first calculated a calling index defined as the number of calling males divided by the total number of males of the group (Belliard et al., 2022). Then, we performed a generalized linear model with binomial distribution [logit link function, glmer function from lme4 package (Bates et al., 2015)]. Repeated measurements of the calling index were modeled as a function of dose or mixture treatment, for bioassay I or II, respectively. In each case, dose or treatment was included as a fixed factor, while the batch was treated as a random factor. For both bioassays, all pairwise comparisons were performed using emmeans function from emmeans package (Lenth et al., 2018).
Results
Chemical characterization of volatile samples and selection of candidate compounds
A total of 24 and 22 putative compounds were identified from GF and GEO samples, respectively. The identity of these compounds and their peak areas relative to the total area are shown in Table 1. Seven compounds were shared by both volatile profiles. However, only five of them—limonene, E-β-ocimene, Z-β-ocimene β-caryophyllene, and α-humulene—have also been reported during chemical characterization of other sources of volatile phytochemicals that increased A. fraterculus mating success following exposure (Table 1 and Figure 1).
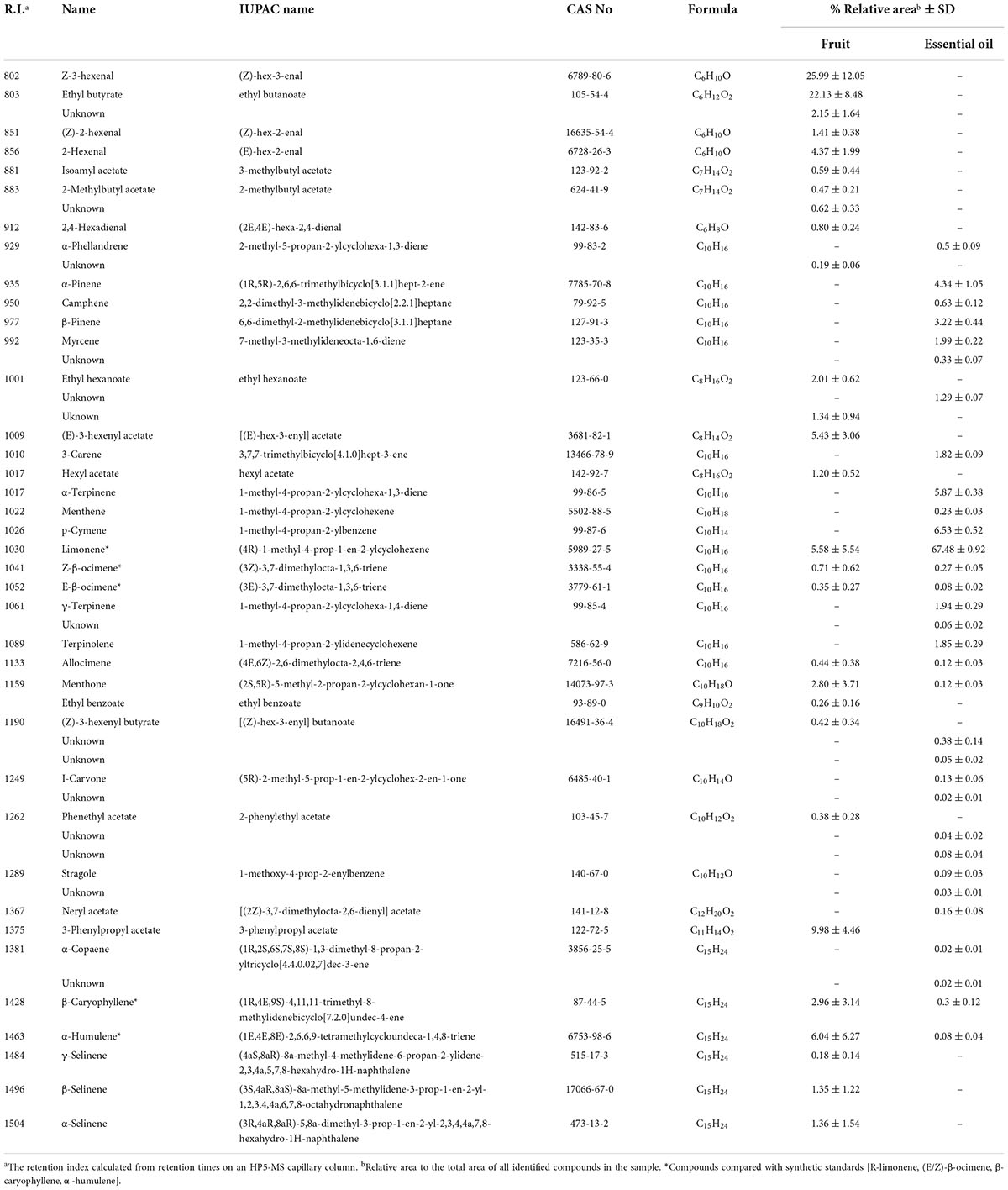
Table 1. Volatile compounds from guava (Psidium guava) fruit and guava essential oil identified by GC-MS.
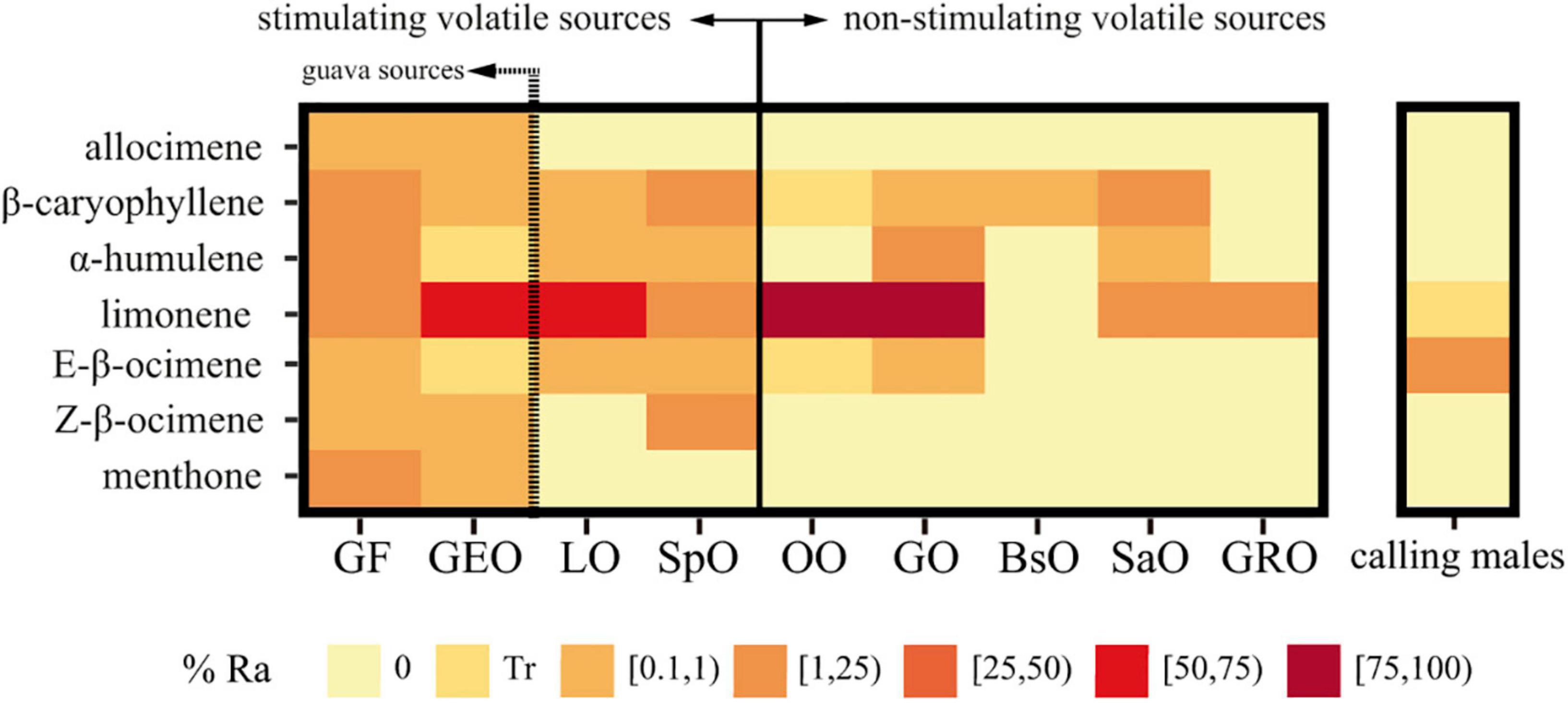
Figure 1. Heatmap of the relative abundance of the seven volatile compounds shared by guava fruit and guava essential oil for different volatile plant sources and calling males. Some of these volatile sources [Psidium guajava fruit (GF), Psidium guajava essential oil (GEO), Citrus limon essential oil (LO), and Schinus polygama essential oil (SpO)] are known to stimulate A. fraterculus male sexual behavior (Vera et al., 2013; Bachmann et al., 2015; Ruiz et al., 2021; Belliard et al., 2022). The rest of them [Citrus sinensis essential oil (OO), Citrus paradisi essential oil (GO), Baccharis spartioides essential oil (BsO), Schinus areira essential oil (SaO), and Zingiber officinale root oil (GRO)] were found as non-stimulating sources (Ruiz et al., 2021). Calling males volatiles were collected from 11-day-old mature males. Data correspond to peak areas relative to the total area from GC-MS analysis of each volatiles source. Data for GF and GEO were generated in the present study while data for non-guava resources were obtained from Ruiz et al. (2015) and Jofré Barud (2018). Traces (Tr) stands for relative area < 0.1%.
E-β-ocimene was found among the five major volatile compounds released by calling-males (% Relative area ± SD = 10.11 ± 0.45). The other candidate compounds were absent (β-caryophyllene and α-humulene) or only in trace (Relative area <0.1) amounts (limonene) (Table 2 and Figure 1).
Electroantennography
All the candidate compounds evaluated (R-limonene, (E/Z)-β-ocimene, β-caryophyllene, and α-humulene) were detected by the insect antenna (Figure 2) The male’s olfactory response to these compounds was stronger than for the solvent controls for at least 3 out of the 5 tested doses (all p-values < 0.01). However, neither the response triggered at saturating dosages (parameter a) nor the sensitivity of the response to pre-saturating concentration (parameter b) differed between compounds (p-values = 0.713 and 0.142, respectively).
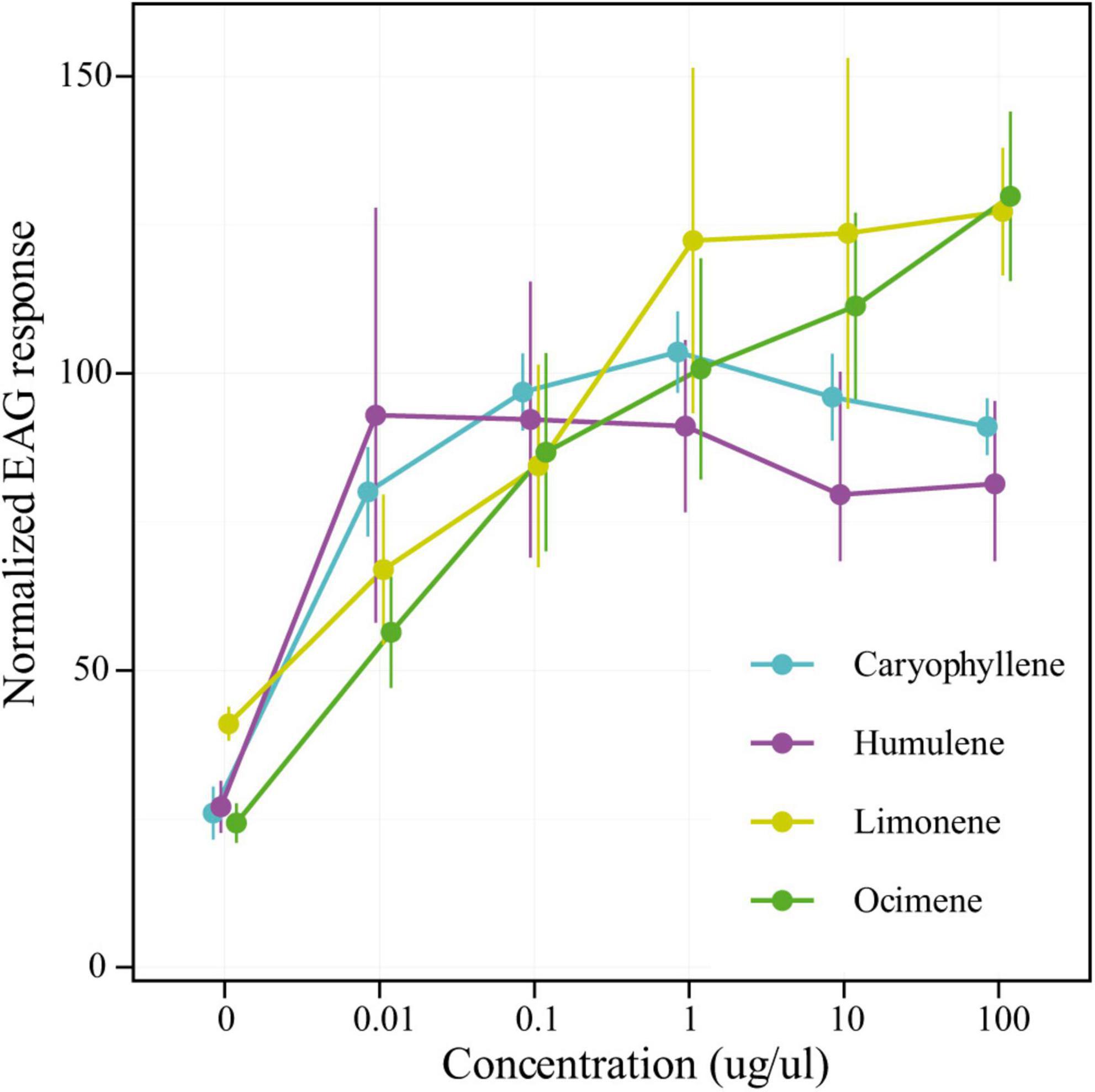
Figure 2. Antennal responses of male A. fraterculus to candidate compounds. Normalized EAG responses (mean ± SE) to five concentrations are shown for each synthetic candidate compound: R-limonene, (E/Z)-β-ocimene (green), β-caryophyllene (cyan), and α-humulene (magenta). The responses for 0-μg/μl doses correspond to n-hexane, the solvent used for dilutions. All responses were normalized to the male antennal response to 1 μg/μl 1-hexanol.
Bioassay I: Male calling behavior after candidate compounds exposure
Males exposed to β-ocimene and R-limonene showed a higher calling index than control males when they were exposed to 1 and 10 μg/μl, respectively. For limonene, the male calling index odds (which stands for the probability that a male is calling divided by its complement) in males exposed to 10 μg/μl were on average 2.65 times (Zratio = 5.16, p < 0.0001) higher than in control males and 4.06 times (Zratio = 6.40, p < 0.0001) higher than in males exposed to 100 μg/μl (Figure 3A). In addition, limonene showed a detrimental effect on the calling index when males were exposed to pure limonene (∼800 μg/μl). In this case, the calling index odds were on average 2.22 times (Zratio = 3.36, p = 0.001) lower in exposed males than in control males (Figure 3A). For β-ocimene treatment, the male calling index odds were on average 2 times (Zratio = 4.63, p < 0.0001) higher in males exposed to 1 μg/μl than in control males and 2.60 times (Zratio = 5.39, p < 0.0001) higher than in males exposed to 100 μg/μl (Figure 3B). No effects on male calling index for any of the tested doses were found after exposure to β-caryophyllene (Chisq = 5.42; p = 0.143) (Figure 3C) or α-humulene (Chisq = 1.07, p = 0.785) (Figure 3D).
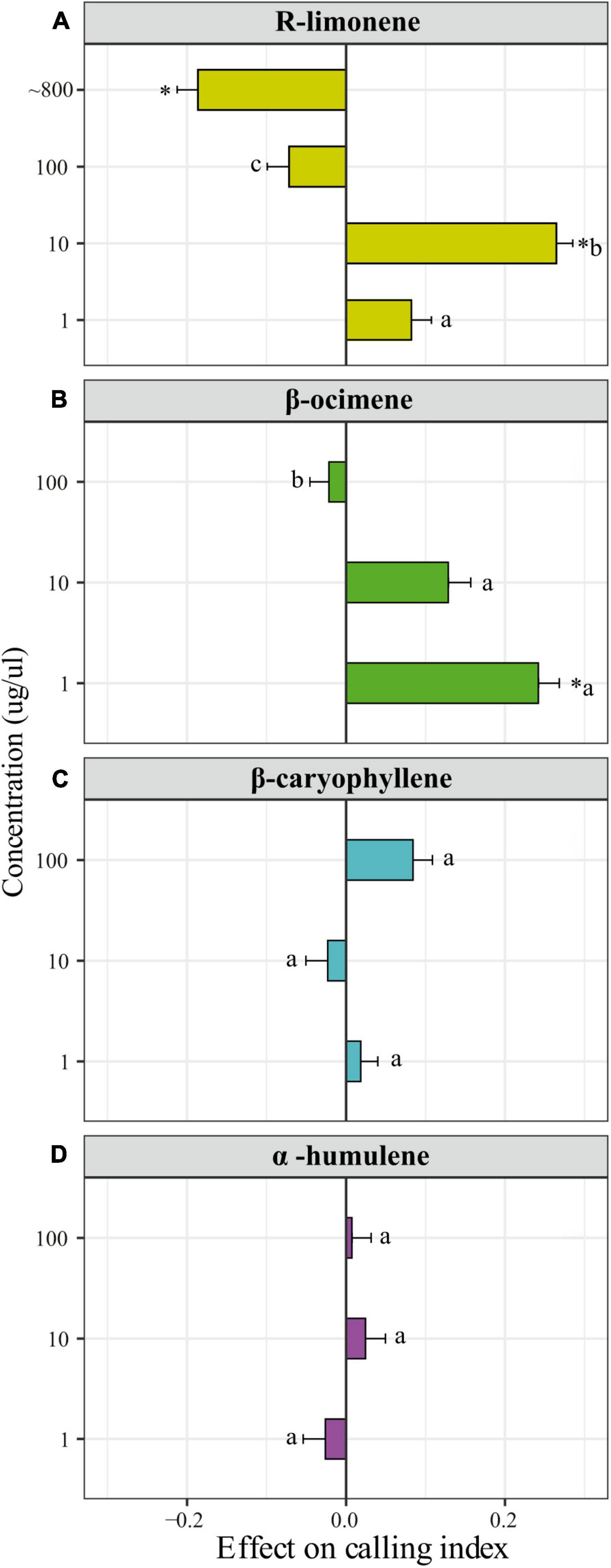
Figure 3. Dose-dependent effect of candidate compounds on A. fraterculus male calling behavior. Mean (±SE) effect on the male calling index (the calling index of exposed males minus the calling index of control males) is shown for males exposed to different concentrations of (A) R-limonene, (B) (E/Z)-β-ocimene, (C) β-caryophyllene, and (D) α-humulene. The calling index stands for the proportion of calling males (number of calling-males/number of assayed males). Control males were exposed to n-hexane. *Indicates significant differences between exposed and control males (P < 0.05, Tukey test) while letters indicate significant differences across treatments (P < 0.05, Tukey test) except for R-limonene at ∼800 μg/μl for which a separated assay was performed.
Bioassay II: Male calling behavior after candidate compounds blends exposure
Males exposed to the mixture of all the four candidate compounds showed a calling index odds 1.6 times higher than non-exposed males (Zratio = 4.49, p < 0.0001). However, the effect of this mixture on the calling index did not differ from the effect of 10 μg/μl of limonene (Zratio = 1.33, p = 0.55), which was on average 1.06 times higher than control (Zratio = 4.49, p < 0.0001). The maximum calling index was found for males exposed to the mixture of limonene and β-ocimene. Males exposed to this mixture showed calling index odds on average 4.24 times (Zratio = 8.35, p < 0.0001) higher than that for non-exposed males and 1.5 times (Zratio = 4.51, p < 0.0001) higher than males exposed to 10 μg/μl of limonene (Figure 4).
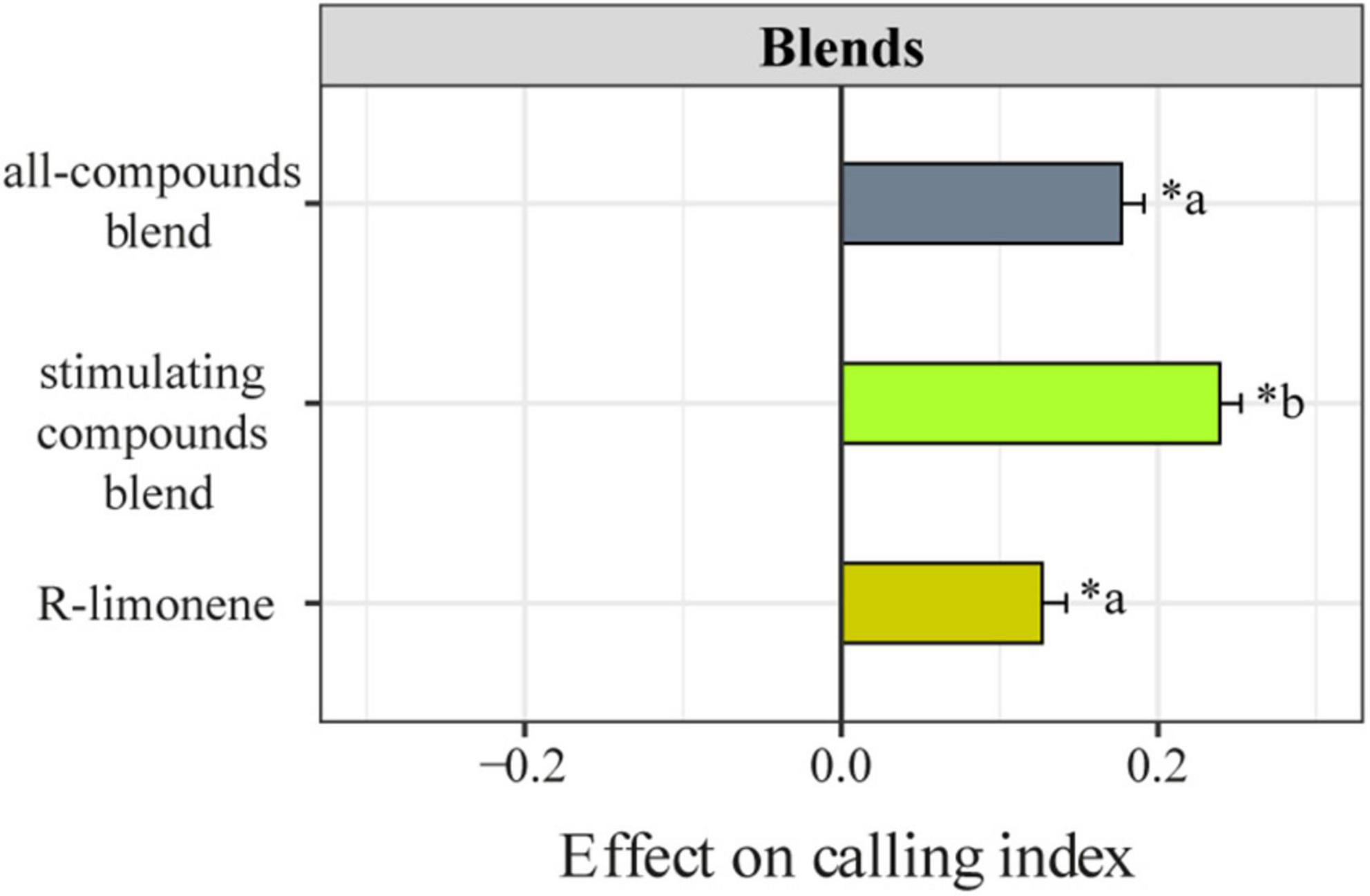
Figure 4. Effect of candidate compounds blends on A. fraterculus male calling behavior. Mean (± SE) effect on male calling index (calling index of exposed males minus calling index of control males) is shown for males exposed to R-limonene (yellow), the stimulating compounds blend (i.e., a blend of R-limonene and (E/Z)-β-ocimene) (green), the all-compounds blend (i.e., a blend of R-limonene (E/Z)-β-ocimene, β-caryophyllene, and α-humulene) (gray). Calling index stands for the proportion of calling males (number of calling-males/number of males assayed). All candidate compound dilutions were made using n-hexane as solvent. * Indicates significant differences between exposed and control males (P < 0.05, Tukey test) while letters indicate significant differences across treatments (P < 0.05, Tukey test).
Discussion
In this study, we explored the chemical profiles of guava volatiles sources (GF and GEO) that can accentuate A. fraterculus male courtship behavior so as to find specific compounds that trigger this phenomenon. We first selected five candidate compounds (α-humulene, β-caryophyllene, E-β-ocimene, Z-β-ocimene, and R-limonene) which were not only present in GF and GEO but also in other volatile sources that stimulate male sexual behavior (LO and SpO). Our analyses revealed that all candidate compounds elicit a response on male antennae, but only R-limonene and (E/Z)-β-ocimene enhanced calling behavior of exposed males. The male response to these compounds may have evolved as an adaptative response to synchronize sexual behavior with the availability of guava fruit, an important native host of A. fraterculus. Our results support the future evaluation of R-limonene, (E/Z)-β-ocimene, and combinations of these compounds in a SIT context (i.e., mass-reared and sterilized males) with the goal of improving the sexual competitiveness of males to be released as part of this pest control strategy.
The repertoires of volatile compounds we identified from GF and GEO were remarkably different: only seven out of 39 compounds were shared between these sources. Also, these common compounds showed very different relative abundances between GF and GEO. These results are not surprising if we take into account that essential oils are the outcome of an extraction process that gives a partial perspective of the volatiles mixture released by the plant (Figueiredo, 2017). In addition, guava fruit used to obtain the commercial essential oil (Nueva Delhi, India) and the fresh guava fruit (collected in Tucuman, Argentina) used in this study likely belong to different P. guajava chemotypes with different types and proportions of the chemical constituents (Figueiredo, 2017; de Souza et al., 2018). In this study, however, we ensured to use the same GF chemotype and GEO batch that we had previously showed to enhance A. fraterculus male sexual behavior (Vera et al., 2013; Bachmann et al., 2015; Belliard et al., 2022). The fact that different headspace sampling techniques provide non-overlapping lists of compounds (Rizzolo et al., 1992) may indicate that other compounds, not detected here, could be shared between GF and GEO. This is an experimental limitation of our approach.
The effect of R-limonene exposure on male courtship behavior is in agreement with Ruiz et al. (2021), who showed that A. fraterculus males previously exposed to this compound exhibit higher mating success. Also, R-limonene is the major volatile compound of citric essential oils that were found to increase the sexual performance of A. fraterculus, A. ludens, and C. capitata (Shelly T. et al., 2004; Shelly, 2009; Morató et al., 2015; Kouloussis et al., 2017; Ruiz et al., 2021). However, male exposure to R-limonene as a pure compound did not affect male mating success in C. capitata (Juan-Blasco et al., 2013). E-β-ocimene, which is a major component of A. fraterculus sex pheromone blend (Bachmann, 2016), had not been directly implied in the stimulation of male calling behavior. Nonetheless, it was used in the past as part of a synthetic blend that proved to increase male mating success in this species (Bachmann et al., 2015). For some compounds, insects may respond differentially to enantiomers (Flath et al., 1994; Giatropoulos et al., 2012; Borrego et al., 2021). Given that Z-β-ocimene isomer was also reported in the A. fraterculus sex pheromone blend (Bøízová et al., 2013) and that we tested the effect of β-ocimene using a mix of isomers (E and Z), further studies are necessary to determine the effect of each isomer alone. The other two compounds we evaluated, β-caryophyllene and α-humulene, did not affect A. fraterculus male calling behavior when exposed to only one of these compounds. The potential of β-caryophyllene to stimulate tephritid fruit flies’ sexual behavior has had not been assayed before, although this compound was found to attract flies of several tephritid species (Kamiji et al., 2018; Wee et al., 2018; Jaleel et al., 2019). Exposure to α-humulene was tested on C. capitata males by Shelly and Nishimoto (2015), who found a negative effect on male mating performance.
The effects of limonene and (E/Z)-β-ocimene were dependent on the exposure dose. R-limonene enhanced male calling behavior at 10 μg/μl while no effects were observed for the lower dose of 1 μg/μl. Moreover, a detrimental effect was observed for pure R-limonene (∼800 μg/μl). Toxic effects of limonene and EOs containing high proportions of limonene were previously reported for tephritid species, including A. fraterculus (Salvatore et al., 2004; Papachristos et al., 2009; Ruiz et al., 2014; Papanastasiou et al., 2017; Oviedo et al., 2018, 2020), where survival, emergence, and fecundity were affected by exposure to these phytochemicals. In agreement, Ruiz et al. (2021) have found that only the EO of lemon, as opposed to the EOs of orange and grapefruit, was able to stimulate A. fraterculus males. Among these three EOs, lemon EO had the lowest R-limonene concentration (Ruiz et al., 2015; González-Mas et al., 2019). Ruiz et al. (2021) also found that males exposed to pure R-limonene achieved more matings during the assay than non-exposed males. While Ruiz et al. (2021) exposed flies for one hour, in the present work the exposure lasted four hours. Therefore, the time of exposure (together with exposure dose) may likely modulate the treatment response. On the other hand, (E/Z)-β-ocimene did not reduce male calling index at any evaluated dose, although we did not evaluate concentrations higher than 100 μg/μl for this compound. Nonetheless, (E/Z)-β-ocimene only stimulated calling males at the lowest tested dose of 1 μg/μl, again showing a dose-dependent effect. Similarly, in C. capitata, the stimulating effect of GRO exposure on male mating success is maximized at low doses (Paranhos et al., 2013).
Individual volatile compounds response often depends on the volatile context in which they are perceived (Schröder and Hilker, 2008; Beyaert et al., 2010; Bruce and Pickett, 2011; Conchou et al., 2019). Thus, the combination of some compounds could activate synergistic or antagonistic behavioral responses on the flies (Bruce and Pickett, 2011; Conchou et al., 2019). For instance, compounds that alone do not trigger any behavioral response may play a key role when perceived in a blend (Beyaert et al., 2010). For this reason, we tested two blends of our candidate compounds: one of them made only with the two stimulating compounds (i.e., R-limonene and β-ocimene) and the other made of all our candidate compounds (i.e., R-limonene, E/Z β-ocimene, β-caryophyllene, and α-humulene). Males exposed to the blend of the stimulating compounds exhibited a higher calling index than those exposed to R-limonene alone and those exposed to the complete blend (all five compounds). Therefore, limonene and β-ocimene seem to have partially additive effects on male calling behavior while β-caryophyllene and α-humulene might be interacting antagonistically with stimulating compounds. In accordance to our results, A. fraterculus males increased their mating success when exposed to a synthetic blend containing limonene, β-ocimene, and α-humulene among other synthetic compounds (Bachmann et al., 2015). While Bachmann et al. (2015) used the same concentration for all compounds, in our blends, the concentration of each compound was the one for which the compound showed the highest stimulating effect when tested alone. However, the relative abundance of the compounds we evaluated in both blends do not represent those of the guava fruit, to which males could be exposed in nature [although in nature males could be stimulated by other structures of guava trees, as reported for C. capitata males by Shelly and Villalobos (2004)]. Aromas with different proportions of the same compounds could be interpreted by insects as different messages (Conchou et al., 2019). Thus, exploring combinations with proportions found in natural resources may help understanding the ecological relevance of these compounds and find a blend that maximizes the male calling activity.
Anastrepha fraterculus is a cryptic species complex, in which distinct populations differ in the composition of the volatiles released by calling-males (Lima et al., 2001; Cáceres et al., 2009; Bøízová et al., 2013; Vaníèková et al., 2015). In agreement with previous studies on flies that derived from the same population as the ones we used (Cáceres et al., 2009; Bachmann, 2016), we confirmed the presence of E-β-ocimene as one of the major compounds and limonene as a minor compound of the volatile blend released by A. fraterculus sp. 1 calling-males. In fact, we only found traces of limonene among the volatile compounds recovered from calling males. However, Bøízová et al. (2013) found higher proportions of limonene in the volatile compounds released by calling-males of the same A. fraterculus morphotype fed on a different diet and bred under different conditions. As it was found in C. capitata, diet and breeding conditions may affect the composition of the sex pheromones blend (Merli et al., 2018). In addition, we found that both compounds, limonene and β-ocimene, were able to elicit an electrophysiological response on virgin mature male antennae. Curiously, E-β-ocimene but not limonene triggered an electrophysiological response in virgin mature females’ antennae, when they were exposed to the volatile blend collected from A. fraterculus sp. 1 calling-males (Bachmann, 2016), which suggests that E-β-ocimene, but not limonene, is a sex pheromone. This is particularly relevant in the context of the mating system of this species, in which males aggregate near/on the host to release the pheromone and increase the signal intensity. Albeit not a sex pheromone compound, limonene from the hosts may be an aggregation cue in a sexual context. Still, the role of these compounds must be tested through behavioral studies involving males and females.
The differences in limonene and β-ocimene concentrations among volatile compounds released by calling males were proposed as a mechanism of pre-mating reproductive isolation between A. ludens and A. suspensa that may has evolved during the initial stages of speciation (Rocca et al., 1992). Unlike A. fraterculus morphotype sp. 1, calling males of A. fraterculus sp. 3 release limonene as a major compound and β-ocimene as one of the less abundant ones (Bøízová et al., 2013; Milet-Pinheiro et al., 2015). Also, A. fraterculus sp. 3 virgin females are able to detect limonene from calling-males’ volatile blends (Milet-Pinheiro et al., 2015). Therefore, the relative amount of limonene and β-ocimene in the sex pheromone blend may also play a role as a reproductive barrier between A. fraterculus morphotypes. Additional studies are nonetheless needed in order to further test this hypothesis and separate the genetic and environmental components that determine the sex pheromone composition.
Females of many tephritid species are more attracted to ripening fruit than to immature fruit, and they also prefer ripening fruit for oviposition (Cunningham et al., 2016; Cortés-Martínez et al., 2021). During the ripening process of guava, an ancestral host of A. fraterculus complex (Hernández-Ortiz et al., 2019), the release of limonene and β-ocimene by the fruit increases (Soares et al., 2007). Therefore, it would not be surprising that the male sexual stimulation caused by the exposure to these compounds constitutes a mechanism useful to synchronize energetic investment in courtship with the availability of host fruit. Although the physiological mechanisms underlying tephritid male sexual stimulation after phytochemical exposure are poorly understood (Segura et al., 2018), they are likely associated with enhanced energy metabolism (Kumaran et al., 2014). In C. capitata, for instance, the male energy reserves (glycogen, lipids, and proteins) are reduced during lek participation (Yuval et al., 1998), when males display a set of energetically expensive courtship behaviors (Kaspi et al., 2000). These reserves drop is probably to maintain a high level of sugar available for muscle cells (Warburg and Yuval, 1997; Yuval et al., 1998). The mobilization of insect energy reserves is controlled by the adipokinetic hormone (AKH), which is synthesized in corpora cardiac cells under energy-demanding conditions (Van der Horst et al., 2001; Van der Horst, 2003; Arrese and Soulages, 2010; Nagata and Zhou, 2019). At least in D. melanogaster and B. dorsalis, the expression of AKH receptors (AKHR) is necessary for starved males to display courtship behavior at the same frequency as fed flies do (Lebreton et al., 2016; Hou et al., 2017). Also, in D. melanogaster starved flies, the AKH expression levels and circulating sugar concentration increased when the flies are exposed to food odor, likely to provide fuel to support food search activity (Lushchak et al., 2015). Considering that the stimulating effect of guava volatiles exposure on A. fraterculus male calling activity is only detected in flies fed on a low-quality diet (Belliard et al., 2022), it is possible that such an effect is modulated through the AKH pathway. Gene expression analysis based on RNA-seq and RNA interference studies will help identify the genes involved in the guava exposure effect and test this hypothesis.
In the present study, we identified two compounds, limonene and β-ocimene, which a part of the volatiles released by guava fruit and guava essential oil, that stimulated calling behavior in A. fraterculus males. In addition, we showed that (E/Z)-β-ocimene exposure requires lower doses than limonene treatment to generate the same effect on male sexual performance, although the use of a combination of both compounds could significantly improve the results. Furthermore, we found that other compounds released by guava fruit, which are also present in the guava essential oil, reduced the effect of limonene and β-ocimene, probably indicating some negative interaction among compounds. Our results also support the previously proposed use of GEO, LO, and limonene to improve the sexual performance of A. fraterculus sterile males in a SIT context (Ruiz et al., 2021; Belliard et al., 2022). However, since the male response is modulated by the exposure dose, the time of exposure, and the nutritional status of the flies (Belliard et al., 2022), the implementation of aromatherapy with limonene and β-ocimene may require careful tuning to avoid unwanted effects. In addition, searching for these compounds in non-tested EOs’ volatile profiles may help find cheaper and easy-to-obtain resources that can be employed in the context of pest management through the SIT, as in the case of C. capitata and the effective use of GRO to boost sterile males’ sexual competitiveness.
Data availability statement
The original contributions presented in this study are included in the article/supplementary material, further inquiries can be directed to the corresponding authors.
Author contributions
PCF and MTV contributed the material. SAB and GEB conducted the bioassays. SAB and JH conducted the statistical analyses. SAB and DFS wrote the manuscript. All authors conceived and designed the research and reviewed and approved the manuscript for publication.
Funding
This work was supported by Ministerio de Ciencia, Tecnología e Innovación (FONCYT-PICT 2017–0239; FONCYT-PICT 2019–04141; and IMPACT.AR DESAFÍO 43), CONICET (PIP 2021–2023-0329CO), and the Joint Division of FAO/IAEA (Contract 24779).
Acknowledgments
We want to especially thank Fabian Milla for technical assistance in insect colony maintenance and the members of the Laboratorio de Insectos de Importancia Agronómica (IGEAF-INTA) for critical remarks in different stages of this work.
Conflict of interest
The authors declare that the research was conducted in the absence of any commercial or financial relationships that could be construed as a potential conflict of interest.
Publisher’s note
All claims expressed in this article are solely those of the authors and do not necessarily represent those of their affiliated organizations, or those of the publisher, the editors and the reviewers. Any product that may be evaluated in this article, or claim that may be made by its manufacturer, is not guaranteed or endorsed by the publisher.
References
Adams, R. P. (2007). Identification of essential oil components by gas chromatography/mass spectrometry. J. Am. Soc. Mass Spectrom. 8:804.
Arita, L. H., and Kaneshiro, K. Y. (1989). Sexual selection and lek behavior in the Mediterranean fruit fly, Ceratitis capitata (Diptera: Tephritidae). Pacific Sci. 43, 135–143.
Arrese, E. L., and Soulages, J. L. (2010). Insect fat body: Energy, metabolism, and regulation. Annu. Rev. Entomol. 55, 207–225. doi: 10.1146/annurev-ento-112408-085356
Bachmann, G. E. (2016). Factores que Afectan el Éxito de Apareamiento de machos de la Mosca Sudamericana de la Fruta, Anastrepha fraterculus (Diptera: Tephritidae). PhD thesis dissertation, Universidad de Buenos Aires.
Bachmann, G. E., Devescovi, F., Nussenbaum, A. L., Milla, F. H., Shelly, T. E., Cladera, J. L., et al. (2019). Mate choice confers directbenefits to females of Anastrepha fraterculus (Diptera: Tephritidae). PLoS One 14: e0214698. doi: 10.1371/journal.pone.0214698
Bachmann, G. E., Segura, D. F., Devescovi, F., Juárez, M. L., Ruiz, M. J., Vera, M. T., et al. (2015). Male sexual behavior and pheromone emission is enhanced by exposure to guava fruit volatiles in Anastrepha fraterculus. PLoS One 10:e124250. doi: 10.1371/journal.pone.0124250
Bates, D., Maechler, M., and Ben Bolker, S. W. (2015). Fitting linear mixed-effects models using {lme4}. J. Stat. Softw. 67, 1–48. doi: 10.18637/jss.v067
Belliard, S. A., Fernández, P. C., Vera, M. T., and Segura, D. F. (2022). Timing of exposure and nutritional status affect male response to guava volatiles, a known courtship enhancer of Anastrepha fraterculus. J. Pest Sci. 95, 279–290. doi: 10.1007/s10340-021-01397-w
Beyaert, I., Wäschke, N., Scholz, A., Varama, M., Reinecke, A., and Hilker, M. (2010). Relevance of resource-indicating key volatiles and habitat odour for insect orientation. Anim. Behav. 79, 1077–1086. doi: 10.1016/j.anbehav.2010.02.001
Borrego, L. G., Ramarosandratana, N., Jeanneau, E., Métay, E., Ramanandraibe, V. V., Andrianjafy, M. T., et al. (2021). Effect of the Stereoselectivity of para-Menthane-3,8-diol Isomers on Repulsion toward Aedes albopictus. J. Agric. Food Chem. 69, 11095–11109. doi: 10.1021/acs.jafc.1c03897
Borrero-Echeverry, F., Bengtsson, M., Nakamuta, K., and Witzgall, P. (2018). Plant odor and sex pheromone are integral elements of specific mate recognition in an insect herbivore. Evolution 72, 2225–2233. doi: 10.1111/evo.13571
Bøízová, R., Mendonça, A. L., Vanícková, L., Mendonça, A. L., Eduardo Da Silva, C., Tomèala, A., et al. (2013). Pheromone analyses of the Anastrepha fraterculus (Diptera: Tephritidae) cryptic species complex. Florida Entomol. 96, 1107–1115. doi: 10.1653/024.096.0351
Bruce, T. J. A., and Pickett, J. A. (2011). Perception of plant volatile blends by herbivorous insects-finding the right mix. Phytochemistry 72, 1605–1611. doi: 10.1016/j.phytochem.2011.04.011
Byers, J. A. (2013). Modeling and regression analysis of semiochemical dose-response curves of insect antennal reception and behavior. J. Chem. Ecol. 39, 1081–1089. doi: 10.1007/s10886-013-0328-6
Cáceres, C., Segura, D. F., Vera, M. T., Wornoayporn, V., Cladera, J. L., Teal, P., et al. (2009). Incipient speciation revealed in Anastrepha fraterculus (Diptera; Tephritidae) by studies on mating compatibility, sex pheromones, hybridization, and cytology. Biol. J. Linn. Soc. 97, 152–165. doi: 10.1111/j.1095-8312.2008.01193.x
Cladera, J. L., Vilardi, J. C., Juri, M., Paulin, L. E., Giardini, M. C., Gómez Cendra, P. V., et al. (2014). Genetics and biology of Anastrepha fraterculus: Research supporting the use of the sterile insect technique (SIT) to control this pest in Argentina. BMC Genet. 15:S12. doi: 10.1186/1471-2156-15-S2-S12
Conchou, L., Lucas, P., Meslin, C., Proffit, M., Staudt, M., and Renou, M. (2019). Insect odorscapes: From plant volatiles to natural olfactory scenes. Front. Physiol. 10:972. doi: 10.3389/fphys.2019.00972
Cortés-Martínez, F., Cruz-López, L., Liedo, P., and Rojas, J. C. (2021). The ripeness stage but not the cultivar influences the attraction of Anastrepha obliqua to guava. Chemoecology 31, 115–123. doi: 10.1007/s00049-020-00332-2
Cunningham, J. P., Carlsson, M. A., Villa, T. F., Dekker, T., and Clarke, A. R. (2016). Do fruit ripening volatiles enable resource specialism in polyphagous fruit flies? J. Chem. Ecol. 42, 931–940. doi: 10.1007/s10886-016-0752-5
De León, A. A. P., Mitchell, R. D., Miller, R. J., and Lohmeyer, K. H. (2021). “Advances in integrated tick management research for area-wide mitigation of tick-borne disease burden,” in Area-Wide Integrated Pest Management, eds J. Hendrichs, R. Pereira, and M. J. B. Vreysen (Boca Raton, FL: CRC Press), doi: 10.1201/9781003169239-15
de Souza, T., da, S., Ferreira, M. F., da, S., Menini, L., Souza, J. R. C., et al. (2018). Chemotype diversity of Psidium guajava L. Phytochemistry 153, 129–137. doi: 10.1016/j.phytochem.2018.06.006
Díaz-Fleischer, F., and Aluja, M. (1999). “Behavior of tephritid flies: a historical perspective,” in Fruit Flies (Tephritidae): Phylogeny and Evolution of Behavior, eds M. Aluja and A. Norrbom (Boca Raton, FL: CRC Press), 57–88.
Enkerlin, W. R. (2005). “Impact of fruit fly control programmes using the sterile insect technique,” in Sterile Insect Technique. Principles and Practice in Area-Wide Integrated Pest Management, eds V. A. Dyck, J. Hendrichs and A. S. Robinson (Dordrecht: Springer) 651–676. doi: 10.1007/1-4020-4051-2_25
Enkerlin, W. R., Gutiérrez Ruelas, J. M., Pantaleon, R., Soto Litera, C., Villaseñor Cortés, A., Zavala López, J. L., et al. (2017). The Moscamed Regional Programme: review of a success story of area-wide sterile insect technique application. Entomol. Exp. Appl. 164, 188–203. doi: 10.1111/eea.12611
Figueiredo, A. C. (2017). Biological properties of essential oils and volatiles: Sources of variability. NVEO 4, 1–13.
Flath, R. A., Cunningham, R. T., Mon, T. R., and John, J. O. (1994). Male lures for mediterranean fruitfly (Ceratitis capitata Wied.): Structural analogs of α-copaene. J. Chem. Ecol. 20, 2595–2609. doi: 10.1007/BF02036194
Flores, S., Rivera, J. P., Hernández, E., and Montoya, P. (2011). The effect of ginger oil on the sexual performance of Anastrepha males (Dip- tera: Tephritidae). Florida Entomol. 94, 916–922.
Fox, J., and Weisberg, S. (2019). An R companion to applied regression (Third). Thousand Oaks CA: Sage.
Gerofotis, C. D., Ioannou, C. S., and Papadopoulos, N. T. (2013). Aromatized to find mates: α-pinene aroma boosts the mating success of adult olive fruit flies. PLoS One 8:e81336. doi: 10.1371/journal.pone.0081336
Giatropoulos, A., Papachristos, D. P., Kimbaris, A., Koliopoulos, G., Polissiou, M. G., Emmanouel, N., et al. (2012). Evaluation of bioefficacy of three Citrus essential oils against the dengue vector Aedes albopictus (Diptera: Culicidae) in correlation to their components enantiomeric distribution. Parasitol. Res. 111, 2253–2263. doi: 10.1007/s00436-012-3074-8
Gomez Cendra, P., Calcagno, G., Belluscio, L., and Vilardi, J. C. (2011). Male courtship behavior of the South American fruit fly, Anastrepha fraterculus, from an Argentinean laboratory strain. J. Insect Sci. 11, 1–18. doi: 10.1673/031.011.17501
González-Mas, M. C., Rambla, J. L., López-Gresa, M. P., Amparo Blázquez, M., and Granell, A. (2019). Volatile compounds in citrus essential oils: A comprehensive review. Front. Plant Sci. 10:12. doi: 10.3389/fpls.2019.00012
Grosjean, P., and Ibanez, F. (2018). pastecs: Package for analysis of space-time ecological series. R package version 1.3.21. Available online at: https://CRAN.R-project.org/package=pastecs
Hassan, E. M., El Gendy, A. E. N. G., Abd-ElGawad, A. M., Elshamy, A. I., Farag, M. A., Alamery, S. F., et al. (2020). Comparative chemical profiles of the essential oils from different varieties of Psidium guajava L. Molecules 26:119. doi: 10.3390/molecules26010119
Hendrichs, J. (2000). Use of the sterile insect technique against key insect pests. Sustain. Dev. Int. 2, 75–80.
Hernández-Ortiz, V., Barradas-Juanz, N., and Díaz-Castelazo, C. (2019). “A review of the natural host plants of the Anastrepha fraterculus complex in the Americas,” in Area-Wide Management of Fruit Fly Pests, eds D. Perez-Staples, F. Diaz-Fleischer, P. Montoya, and M. T. Vera (Boca Raton, FL: CRC Press), 90–114. doi: 10.1201/9780429355738
Hou, Q. L., Chen, E. H., Jiang, H. B., Wei, D. D., Gui, S. H., Wang, J. J., et al. (2017). Adipokinetic hormone receptor gene identification and its role in triacylglycerol mobilization and sexual behavior in the oriental fruit fly (Bactrocera dorsalis). Insect Biochem. Mol. Biol. 90, 1–13. doi: 10.1016/j.ibmb.2017.09.006
Jaleel, W., He, Y., and Lü, L. (2019). The response of two Bactrocera species (Diptera: Tephritidae) to fruit volatiles. J. Asia. Pac. Entomol. 22, 758–765. doi: 10.1016/j.aspen.2019.05.011
Jofré Barud, F. (2018). Aceites esenciales como agentes semioquímicos de diferentes comportamientos plausibles de control de la misca de los frutos Ceratitis capitata (Wiedemann). PhD thesis dissertation, Córdoba: Universidad Nacional de Córdoba.
Juan-Blasco, M., San Andrés, V., Martínez-Utrillas, M. A., Argilés, R., Pla, I., Urbaneja, A., et al. (2013). Alternatives to ginger root oil aromatherapy for improved mating performance of sterile Ceratitis capitata (Diptera: Tephritidae) males. J. Appl. Entomol. 137, 244–251. doi: 10.1111/j.1439-0418.2011.01688.x
Kamiji, T., Kaneda, M., Sasaki, M., and Ohto, K. (2018). Sexual maturation of male Bactrocera correcta (Diptera: Tephritidae) and age-related responses to β - caryophyllene and methyl eugenol. Appl. Entomol. Zool. 53, 41–46. doi: 10.1007/s13355-017-0525-9
Kaspi, R., Taylor, P. W., and Yuval, B. (2000). Diet and size influence sexual advertisement and copulatory success of males in Mediterranean fruit fly leks. Ecol. Entomol. 25, 279–284. doi: 10.1046/j.1365-2311.2000.00266.x
Knipling, E. F. (1955). Possibilities of insect control or eradication through the use of sexually sterile males. J. Econ. Entomol. 48, 459–462.
Kokkari, A. I., Milonas, P. G., Anastasaki, E., Floros, G. D., Kouloussis, N. A., and Koveos, D. S. (2021). Determination of volatile substances in olives and their effect on reproduction of the olive fruit fly. J. Appl. Entomol. 145, 841–855. doi: 10.1111/jen.12929
Kouloussis, N. A., Gerofotis, C. D., Ioannou, C. S., Iliadis, I. V., Papadopoulos, N. T., and Koveos, D. S. (2017). Towards improving sterile insect technique: Exposure to orange oil compounds increases sexual signalling and longevity in Ceratitis capitata males of the Vienna 8 GSS. PLoS One 12:e188092. doi: 10.1371/journal.pone.0188092
Kumaran, N., Prentis, P. J., Mangalam, K. P., Schutze, M. K., and Clarke, A. R. (2014). Sexual selection in true fruit flies (Diptera: Tephritidae): Transcriptome and experimental evidences for phytochemicals increasing male competitive ability. Mol. Ecol. 23, 4645–4657. doi: 10.1111/mec.12880
Kuznetsova, A., Brockhoff, P. B., and Christensen, R. H. B. (2017). lmertest pack- age: tests in linear mixed effects models. J. Stat. Softw. 82, 1–26. doi: 10.18637/jss.v082.i13
Landolt, P. J., and Phillips, T. W. (1997). Host plant influences on sex pheromone behavior of phytophagous insects. Annu. Rev. Entomol. 42, 371–391. doi: 10.1146/annurev.ento.42.1.371
Lebreton, S., Mansourian, S., Bigarreau, J., and Dekker, T. (2016). The adipokinetic hormone receptor modulates sexual behavior, pheromone perception and pheromone production in a sex-specific and starvation-dependent manner in Drosophila melanogaster. Front. Ecol. Evol. 3:151. doi: 10.3389/fevo.2015.00151
Lenth, R., Singmann, H., Love, J., Buerkner, P., and Herve, M. (2018). emmeans: Estimated Marginal Means, aka Least-Squares Means. R package version 1.1.3. Available online at: https://CRAN.R-project.org/package=emmeans
Lima, I. S., House, P. E., and Nascimento, R. R. D. (2001). Volatile substances from male Anastrepha fraterculus Wied.(Diptera: Tephritidae): identification and behavioural activity. J. Braz. Chem. Soc. 12, 196–201.
Lushchak, O. V., Carlsson, M. A., and Nässel, D. R. (2015). Food odors trigger an endocrine response that affects food ingestion and metabolism. Cell. Mol. Life Sci. 72, 3143–3155. doi: 10.1007/s00018-015-1884-4
Mastrangelo, T., da Silva, F. F., Mascarin, G. M., and da Silva, C. B. (2019). Multispectral imaging for quality control of laboratory-reared Anastrepha fraterculus (Diptera: Tephritidae) pupae. J. Appl. Entomol. 143, 1072–1079. doi: 10.1111/jen.12716
Mastrangelo, T., Kovaleski, A., Botteon, V., Scopel, W., and de Lourdes Zamboni Costa, M. (2018). Optimization of the sterilizing doses and overflooding ratios for the South American fruit fly. PLoS One 13:e201026. doi: 10.1371/journal.pone.0201026
Mastrangelo, T., Kovaleski, A., Maset, B., Costa, M., de, L. Z., Barros, C., et al. (2021). Improvement of the mass-rearing protocols for the south american fruit fly for application of the sterile insect technique. Insects 12, 1–18. doi: 10.3390/insects12070622
Mazomenos, B. E., and Haniotakis, G. E. (1981). A multicomponent female sex pheromone of Dacus oleae Gmelin: Isolation and bioassay. J. Chem. Ecol. 7, 437–444. doi: 10.1007/BF00995766
Merli, D., Mannucci, B., Bassetti, F., Corana, F., Falchetto, M., Malacrida, A. R., et al. (2018). Larval diet affects male pheromone blend in a laboratory strain of the medfly. Ceratitis capitata (Diptera: Tephritidae). J. Chem. Ecol. 44, 339–353. doi: 10.1007/s10886-018-0939-z
Milet-Pinheiro, P., Navarro, D. M. A., De Aquino, N. C., Ferreira, L. L., Tavares, R. F., da Silva, R. C. C., et al. (2015). Identification of male-borne attractants in Anastrepha fraterculus (Diptera: Tephritidae). Chemoecology 25, 115–122. doi: 10.1007/s00049-014-0180-3
Morató, S., Shelly, T., Rull, J., and Aluja, M. (2015). Sexual competitiveness of Anastrepha ludens (Diptera: Tephritidae) males exposed to Citrus aurantium and Citrus paradisi essential oils. J. Econ. Entomol. 108, 621–628. doi: 10.1093/jee/tou054
Moreau, J., Desouhant, E., Louâpre, P., Goubault, M., Rajon, E., Jarrige, A., et al. (2017). How host plant and fluctuating environments affect insect reproductive strategies? Adv. Bot. Res. 81, 259–287. doi: 10.1016/bs.abr.2016.09.008
Nagata, S., and Zhou, Y. J. (2019). Feeding-Modulating Neuropeptides and Peptide Hormones in Insects, 1st Edn. Amsterdam: Elsevier Ltd, doi: 10.1016/bs.aiip.2019.10.002
Nishida, R., Shelly, T. E., Whittier, T. S., and Kaneshiro, K. Y. (2000). α-Copaene, a potential rendezvous cue for the Mediterranean fruit fly. Ceratitis capitata? J. Chem. Ecol. 26, 87–100. doi: 10.1023/A:1005489411397
Niyazi, N., Shuker, D. M., and Wood, R. J. (2008). Male position and calling effort together influence male attractiveness in leks of the medfly, Ceratitis capitata (Diptera: Tephritidae). Biol. J. Linnean Soc. 95, 479–487.
Oviedo, A., Van Nieuwenhove, G., Van Nieuwenhove, C., and Rull, J. (2018). Biopesticide effects on pupae and adult mortality of Anastrepha fraterculus and Ceratitis capitata (Diptera: Tephritidae). Aust. Entomol. 57, 457–464. doi: 10.1111/aen.12296
Oviedo, A., Van Nieuwenhove, G., Van Nieuwenhove, C., and Rull, J. (2020). Exposure to essential oils and ethanol vapors affect fecundity and survival of two frugivorous fruit fly (Diptera: Tephritidae) pest species. Bull. Entomol. Res. 110, 558–565. doi: 10.1017/S0007485320000085
Papachristos, D. P., Kimbaris, A. C., Papadopoulos, N. T., and Polissiou, M. G. (2009). Toxicity of citrus essential oils against Ceratitis capitata (Diptera: Tephritidae) larvae. Ann. Appl. Biol. 155, 381–389. doi: 10.1111/j.1744-7348.2009.00350.x
Papanastasiou, S. A., Bali, E. M. D., Ioannou, C. S., Papachristos, D. P., Zarpas, K. D., and Papadopoulos, N. T. (2017). Toxic and hormetic-like effects of three components of citrus essential oils on adult Mediterranean fruit flies (Ceratitis capitata). PLoS One 12:e177837. doi: 10.1371/journal.pone.0177837
Paranhos, B. J., McInnis, D., Morelli, R., Castro, R. M., Garziera, L., Paranhos, L. G., et al. (2013). Optimum dose of ginger root oil to treat sterile Mediterranean fruit fly males (Diptera: Tephritidae). J. Appl. Entomol. 137, 83–90. doi: 10.1111/j.1439-0418.2010.01595.x
Pereira, R., Yuval, B., Liedo, P., Teal, P. E. A., Shelly, T. E., McInnis, D. O., et al. (2013). Improving sterile male performance in support of programmes integrating the sterile insect technique against fruit flies. J. Appl. Entomol. 137, 178–190.
Pereira, R., Yuval, B., Liedo, P., Teal, P. E. A., Shelly, T. E., McInnis, D. O., et al. (2021). “Improving sterile male performance in support of programmes integrating the sterile insect technique against fruit flies,” in Sterile Insect Technique: Principles and Practice in Area-Wide Integrated Pest Management, eds A. S. Dyck, V. A. Hendrichs, and J. Robinson (Boca Raton, FL: CRC Press), 631–656. doi: 10.1111/j.1439-0418.2011.01664.x
R Core Team (2021). R: A Language and Environment for Statistical Computing. Vienna: R Foundation for Statistical Computing.
Reddy, G. V. P., and Guerrero, A. (2004). Interactions of insect pheromones and plant semiochemicals. Trends Plant Sci. 9, 253–261. doi: 10.1016/j.tplants.2004.03.009
Rizzolo, A., Polesello, A., and Polesello, S. (1992). Use of headspace capillary GC to study the development of volatile compounds in fresh fruit. J. High Res. Chromato. 15, 472–477.
Rocca, J. R., Nation, J. L., Strekowski, L., and Battiste, M. A. (1992). Comparison of volatiles emitted by male caribbean and mexican fruit flies. J. Chem. Ecol. 18, 223–244. doi: 10.1007/BF00993755
Ruiz, M. J., Juárez, M. L., Alzogaray, R. A., Arrighi, F., Arroyo, L., Gastaminza, G., et al. (2015). Oviposition behaviour and larval development of Anastrepha fraterculus from Argentina in citrus. Entomol. Exp. Appl. 157, 198–213. doi: 10.1111/eea.12357
Ruiz, M. J., Juárez, M. L., Alzogaray, R. A., Arrigui, F., Arroyo, L., Gastaminza, G., et al. (2014). Toxic effect of citrus peel constituents on Anastrepha fraterculus Wiedemann and Ceratitis capitata Wiedemann immature stages. J. Agric. Food Chem. 62, 10084–10091. doi: 10.1021/jf503063b
Ruiz, M. J., Juárez, M. L., Jofré Barud, F., Goane, L., Valladares, G. A., Bachmann, G. E., et al. (2021). Lemon and Schinus polygama essential oils enhance male mating success of Anastrepha fraterculus. Entomol. Exp. Appl. 169, 172–182. doi: 10.1111/eea.13005
Ryan, M. J. (1990). Sexual selection, sensory systems and sensory exploitation. Oxford Surv. Evol. Biol. 7, 157–195.
Salvatore, A., Borkosky, S., Willink, E., and Bardón, A. (2004). Toxic effects of lemon peel constituents on Ceratitis capitata. J. Chem. Ecol. 30, 323–333. doi: 10.1023/B:JOEC.0000017980.66124.d1
Schröder, R., and Hilker, M. (2008). The relevance of background odor in resource location by insects: A behavioral approach. Bioscience 58, 308–316. doi: 10.1641/B580406
Segura, D. F., Belliard, S. A., Vera, M. T., Bachmann, G. E., Ruiz, M. J., Jofre-Barud, F., et al. (2018). Plant chemicals and the sexual behavior of male tephritid fruit flies. Ann. Entomol. Soc. Am. 111, 239–264. doi: 10.1093/aesa/say024
Segura, D., Petit-Marty, N., Sciurano, R., Vera, T., Calcagno, G., Allinghi, A., et al. (2007). Lekking behavior of Anastrepha fraterculus (Diptera: Tephritidae). Florida Entomol. 90, 154–162.
Shelly, T. E. (2001). Exposure to α-copaene and α-copaene-containing oils enhances mating success of male Mediterranean fruit flies (Diptera: Tephritidae). Ann. Entomol. Soc. Am. 94, 497–502.
Shelly, T. E. (2009). Exposure to grapefruits and grapefruit oil increases male mating success in the Mediterranean fruit fly (Diptera: Tephritidae). Proc. Hawaiian Entomol. Soc. 41, 31–36. doi: 10.1093/biomethods/bpx009
Shelly, T. E., and Nishimoto, J. (2015). Exposure to the plant compound α-Humulene reduces mating success in male Mediterranean fruit flies (Diptera: Tephritidae). Ann. Entomol. Soc. Am. 108, 215–221. doi: 10.1093/aesa/sav008
Shelly, T. E., and Villalobos, E. M. (2004). Host plant influence on the mating success of male Mediterranean fruit flies: variable effects within and between individual plants. Anim. Behav. 68, 417–426.
Shelly, T. E., McInnis, D. O., Pahio, E., and Edu, J. (2004). Aromatherapy in the Mediterranean fruit fly (Diptera: Tephritidae): Sterile males exposed to ginger root oil in prerelease storage boxes display increased mating competitiveness in field-cage trials. J. Econ. Entomol. 97, 846–853. doi: 10.1093/jee/97.3.846
Shelly, T., Dang, C., and Kennelly, S. (2004). Exposure to orange (Citrus sinensis L.) trees, fruit, and oil enhances mating success of male mediterranean fruit flies (Ceratitis capitata [Wiedemann]). J. Insect Behav. 17, 303–315. doi: 10.1023/B:JOIR.0000031532.29287.95
Siddique, S., Perveen, Z., Nawaz, S., Shahzad, K., and Ali, Z. (2015). Chemical composition and antimicrobial activities of essential oils of six species from family Myrtaceae. J. Essent. Oil Bear. Plants 18, 950–956.
Sivinski, J., Aluja, M., Dodson, G. N., Freidberg, A., Headrick, D. H., Kaneshiro, K. Y., et al. (1999). “Topics in the evolution of sexual behavior in the tephritidae,” in Fruit Flies (Tephritidae): Phylogeny and Evolution of Behavior, eds M. Aluja and A. Norrbom (Boca Raton, FL: CRC Press), 769–810.
Soares, F. D., Pereira, T., Maio Marques, M. O., and Monteiro, A. R. (2007). Volatile and non-volatile chemical composition of the white guava fruit (Psidium guajava) at different stages of maturity. Food Chem. 100, 15–21. doi: 10.1016/j.foodchem.2005.07.061
Tumlinson, J. H. (2014). The importance of volatile organic compounds in ecosystem functioning. J. Chem. Ecol. 40, 212–213. doi: 10.1007/s10886-014-0399-z
Van der Horst, D. J. (2003). Insect adipokinetic hormones: release and integration of flight energy metabolism. Comp. Biochem. Physiol. Part B Biochem. Mol. Biol. 136, 217–226. doi: 10.1016/S1096-4959(03)00151-9
Van der Horst, D. J., Van Marrewijk, W. J. A., and Diederen, J. H. B. (2001). Adipokinetic hormones of insect: Release, signal transduction, and responses. Int. Rev. Cytol. 211, 179–240. doi: 10.1016/S0074-7696(01)11019-3
Vaníèková, L., Bøízová, R., Pompeiano, A., Ferreira, L. L., de Aquino, N. C., de Farias Tavares, R., et al. (2015). Characterisation of the chemical profiles of Brazilian and Andean morphotypes belonging to the Anastrepha fraterculus complex (Diptera, Tephritidae). ZooKeys 193. doi: 10.3897/zookeys.540.9649
Vaníèková, L., Do Nascimento, R. R., Hoskovec, M., Ježková, Z., Bøízová, R., Tomèala, A., et al. (2012). Are the wild and laboratory insect populations different in semiochemical emission? the case of the medfly sex pheromone. J. Agric. Food Chem. 60, 7168–7176. doi: 10.1021/jf301474d
Vera, M. T., Ruiz, M. J., Oviedo, A., Abraham, S., Mendoza, M., Segura, D. F., et al. (2013). Fruit compounds affect male sexual success in the South American fruit fly, Anastrepha fraterculus (Diptera: Tephritidae). J. Appl. Entomol. 137, 2–10. doi: 10.1111/j.1439-0418.2010.01516.x
Warburg, M. S., and Yuval, B. (1997). Effects of energetic reserves on behavioral patterns of Mediterranean fruit flies (Diptera: Tephritidae). Oecologia 112, 314–319. doi: 10.1007/s004420050314
Wee, S. L., Chinvinijkul, S., Hong, K., and Ritsuo, T. (2018). A new and highly effective male lure for the guava fruit fly Bactrocera correcta. J. Pest Sci. 91, 691–698. doi: 10.1007/s10340-017-0936-y
Whittier, T. S., Nam, F. Y., Shelly, T. E., and Kaneshiro, K. Y. (1994). Male courtship success and female discrimination in the Mediterranean fruit fly (Diptera: Tephritidae). J. Insect Behav. 7, 159–170.
Xu, H., and Turlings, T. C. J. (2018). Plant volatiles as mate-finding cues for insects. Trends Plant Sci. 23, 100–111. doi: 10.1016/j.tplants.2017.11.004
Yuval, B., Kaspi, R., Shloush, S., and Warburg, M. S. (1998). Nutritional reserves regulate male participation in Mediterranean fruit fly leks. Ecol. Entomol. 23, 211–215. doi: 10.1046/j.1365-2311.1998.00118.x
Keywords: semiochemicals, β-ocimene, limonene, pheromone calling, phytochemicals, fruit flies, sterile insect technique
Citation: Belliard SA, Bachmann GE, Fernández PC, Hurtado J, Vera MT and Segura DF (2022) Identification of host plant volatile stimulants of Anastrepha fraterculus male courtship behavior. Front. Ecol. Evol. 10:943260. doi: 10.3389/fevo.2022.943260
Received: 13 May 2022; Accepted: 25 August 2022;
Published: 12 September 2022.
Edited by:
Ana Depetris Chauvin, Max Planck Institute for Chemical Ecology, GermanyReviewed by:
Alvin Kah-Wei Hee, Putra Malaysia University, MalaysiaMaria Albo, Universidad de la República, Uruguay
Dori Edson Nava, Embrapa Clima Temperado, Brazil
Copyright © 2022 Belliard, Bachmann, Fernández, Hurtado, Vera and Segura. This is an open-access article distributed under the terms of the Creative Commons Attribution License (CC BY). The use, distribution or reproduction in other forums is permitted, provided the original author(s) and the copyright owner(s) are credited and that the original publication in this journal is cited, in accordance with accepted academic practice. No use, distribution or reproduction is permitted which does not comply with these terms.
*Correspondence: Silvina Anahí Belliard, belliard.silvina@inta.gob.ar; Diego Fernando Segura, segura.diego@inta.gob.ar