- 1Bird and Mammal Evolution, Systematics and Ecology Lab, Department of Zoology, Institute of Biosciences, Universidade Federal do Rio Grande do Sul, Porto Alegre, Brazil
- 2PPGECO – Graduate Program in Ecology, Universidade Federal do Rio Grande do Sul, Porto Alegre, Brazil
- 3Centre for Environmental and Marine Studies, Universidade de Aveiro, Aveiro, Portugal
The Pampa is the least protected and one of the least sampled for bats among the Brazilian domains. This leads to significant Linnean and Wallacean shortfalls for bats in the Brazilian-Uruguayan savanna ecoregion. Here, we aimed to model the occupancy of aerial insectivorous bats in response to landscape structure at different scales, considering the influence of microclimate on bat detection. We acoustically monitored 68 locations during the spring and summer of 2019/2020, gathering data on temperature and humidity associated with each acoustic record using data loggers. We detected at least 11 species of the Molossidae and the Vespertilionidae families, of which 9 were used in the model. The response to landscape structure was species-specific: the occupancy probability of Eptesicus brasiliensis and Molossus cf. currentium increased with landscape connectivity at the 500 m scale while Eptesicus furinalis and Histiotus cf. velatus were negatively affected by landscape connectivity at the 5.0 km scale. Molossus occupancy probability responded negatively to landscape heterogeneity at the 3.0 km scale, while Promops centralis responded positively to landscape heterogeneity at the 5.0 km scale. Molossus rufus responded negatively to native vegetation cover and positively to landscape heterogeneity at the 5.0 km scale. Myotis albescens and Molossops temminckii did not respond significantly to any of the evaluated landscape metrics. Our results show that different bat species perceive the landscape differently, regardless of the guild of use of space – edge- or open-space forager. Our estimate of projected occupancy for the areas contiguous to those sampled ranged from 0.45 to 0.70 for the whole of the bat taxa, suggesting that the landscape, particularly where it still maintains its native elements, is reasonably favourable to aerial insectivores.
Introduction
Habitat loss, fragmentation, and degradation are the most cited causes of species extinction and biodiversity decline globally (Taubert et al., 2018). Intensive land use changes alter the structure of the landscape and limit the potential occurrence, dispersal, and colonisation by different species (Fahrig, 2003; Jetz et al., 2007; Crooks and Sanjayan, 2010). The conversion of open habitats into monoculture plantations of fast-growing trees or their degradation through intensive use for agriculture and livestock production has changed the dynamics and structure of the landscape (Ellis et al., 2010; de Oliveira et al., 2017; Souza et al., 2020). Such landscape changes are of particular concern in tropical countries (Phalan et al., 2013), like Brazil, which despite being a megadiverse, is also one of the world’s largest producers of agricultural commodities (Myers et al., 2000; de Sousa-Neto et al., 2018).
The main consequence of intense land use change is habitat homogenisation. This is of particular concern as heterogeneous and complex environments are drivers of diversification, governing species turnover (Tews et al., 2004). Indeed, patches and networks of distinct natural elements provide increased resource availability, including food, roosts and shelters, and niche space for other activities throughout the animals’ life histories, favouring increased coexistence, persistence, and species diversification (Stein et al., 2014). The diversity of natural elements between different habitats is also paramount for landscape connectivity (Bennett et al., 2006). Indeed, connectivity is a key element in landscape structure and can be defined as the degree to which the landscape facilitates or restricts the movement of organisms between fragments (Calabrese and Fagan, 2004; Taylor et al., 2010). The smaller the distance between patches of similar habitat, the more connected they are. Patches that are more connected to each other facilitate exchanges between subpopulations, reducing the risk of population decline and local extinction (Bennett et al., 2006).
In addition to connectivity and heterogeneity, the amount of available habitat is another factor explaining species richness and diversity across landscapes and is usually a better predictor of these biodiversity dimensions than the size or isolation of a given fragment, as proposed in the habitat amount hypothesis (Fahrig, 2013). Furthermore, different animal species respond differently to landscape structure and this response may be affected both by spatial and temporal scales (Wiens, 1989; Chave, 2013). However, our understanding is still poor of how changes in the landscape, especially those resulting from anthropogenic action, affect the components of biodiversity, including different taxa, different diversity facets, and different environmental and landscape scenarios.
Brazil harbours a rich bat fauna, with 182 species recognised to date (Garbino et al., 2020, 2022). Insectivorous species constitute half of this diversity and are, in general, poorly sampled throughout Brazil, since most studies on neotropical bats have been carried out on species more easily captured by mist nets (but see Arias-Aguilar et al., 2018; Hintze et al., 2020; Falcão et al., 2021). Species of this guild have great potential as bioindicators of environmental quality (Jones et al., 2009), playing an important role as biological control agents in altered landscapes, acting in the suppression of insect populations, including pests of agricultural crops (Cleveland et al., 2006; Rodríguez-San Pedro et al., 2018; Cohen et al., 2020; Kolkert et al., 2020; Aguiar et al., 2021). Despite this, bats may have different sensitivities to changes in landscape composition and structure. Such sensitivity depends on intrinsic factors that include body size, diet, flight, and dispersal abilities (Schnitzler et al., 2003; Lino et al., 2019). Undeniably, species with greater ability to fly and disperse are more likely to persist in altered landscapes, because they can search for favourable habitats within a less favorable matrix, while less vagile species may not be able to do the same, becoming restricted to suboptimal habitat conditions, with potentially severe consequences for their genetic diversity (Lino et al., 2019) and, ultimately, for their persistence.
Climate, habitat, and biotic interactions seem to be the factors most contributing to differences in bat composition, activity, and diversity (Estrada-Villegas et al., 2012; Appel et al., 2021; da Costa et al., 2021; Smith et al., 2021; Ramos Pereira et al., 2022). Climatic conditions are responsible for determining the temporal and spatial availability of resources at large scales and, at small scales may impose activity restrictions associated with metabolic costs, for instance, those associated with maintaining high and stable body temperatures when the ambient temperature is low. Moreover, the effects of temperature and relative humidity influence, in a complex and non-linear way, the propagation and attenuation of sounds emitted by echolocating bat species (Snell-Rood, 2012; Mutumi et al., 2016; Chaverri and Quirós, 2017). In terms of spatial resolution, different bat guilds perceive their habitat in different ways, both in terms of scale and landscape pattern (Bellamy et al., 2013; Ducci et al., 2015; Mendes et al., 2017; Falcão et al., 2021). So, due to the specificity of bat responses to the landscape, it is important to understand how different species and guilds respond to different habitat changes and configurations, considering the matrix and its quality.
The Uruguayan savanna ecoregion extends from the extreme south of Brazil, throughout Uruguay and a small section of the Argentinean province of Entre Ríos. In Brazil, this ecoregion is represented by the Pampa, characterised by extensive natural grasslands with shrubs and rocky outcrops (Pillar et al., 2009). In recent decades, the Pampean landscape has suffered with the conversion of natural areas into monocultures and the introduction of exotic species in silviculture (Roesch et al., 2009). Recent estimates indicate that the Pampa lost 21.4% of the remaining native vegetation cover between 1985 and 2020, remaining only 46% of the original landscape (Souza et al., 2020). Although the Pampa is widely studied from the floristic point of view and in what concerns past and present human occupation (Guido et al., 2016; Leidinger et al., 2017), it remains largely understudied about the geographic distribution and patterns of occupancy of many animals (Konze, 2015; Queirolo, 2016; Tirelli et al., 2018). Bats are no exception to this pattern (Bernard et al., 2011), while it is known that most species occurring here are aerial insectivores, either open-space or edge-space foragers (Noronha, 2016).
Here, we use the history of acoustic detection and non-detection of bats to investigate the influence of landscape structure and microclimate on the occupancy and detection of aerial insectivores in the Brazilian Pampa. We hypothesise that the detection of all species should be influenced by microclimatic variables, and we predict that bat activity and, consequently bat detection, will increase positively with temperature, due to increased activity of their ectothermic prey, flying arthropods, and humidity, due to increased airborne sound propagation. Extremely low or high temperatures or humidity levels should negatively influence bat detection due to increased costs with thermal balance and reduced sound propagation or unpredictable echo behaviour in the lower and upper extremes of the humidity, respectively. We also hypothesise that species occupancy is influenced by the structure of the landscape, and we predict that (i) connectivity between patches of the same habitat positively affects the occupancy of edge-space foraging bats, as this guild should be favoured by forest patches offering extra roosts, perches, and food resources; (ii) heterogeneity should affect positively the occupancy of open-space bats, by favouring a more diverse and abundant prey community at different times of the night; and (iii) a greater amount of native vegetation cover should positively affect all species occurrences, as it offers greater carrying capacity, but potentially influencing, even more, the occupation of edge foragers.
Materials and methods
Study area
We sampled five areas in the Brazilian Pampa (within the Brazilian-Uruguayan savanna ecoregion; Figure 1) in the municipalities of Alegrete, Cacequi, and Quaraí, spanning a wide range of pristine habitats, including native grasslands, meadows, gallery forests, shrub formations, and rock outcrops, as well as areas that harbour different levels of cattle raising on natural grasslands and agriculture performed in converted areas. Sampling sites were classified into one of the two main physiognomies occurring in the region: (i) shallow soil fields, mostly composed of undergrowth vegetation, with a predominance of grasses, legumes, and composite plants that grow from basalt formations with low moisture retention and (ii) mixed stands of andropogon grass, where most of the area has already been transformed into rice and soybean crops; in pastures with overgrazing, the proportion of bare soil increases, benefiting the development of composite vegetation, such as plants of the genera Soliva, Vernonia, and Senecio (Boldrini, 2009).
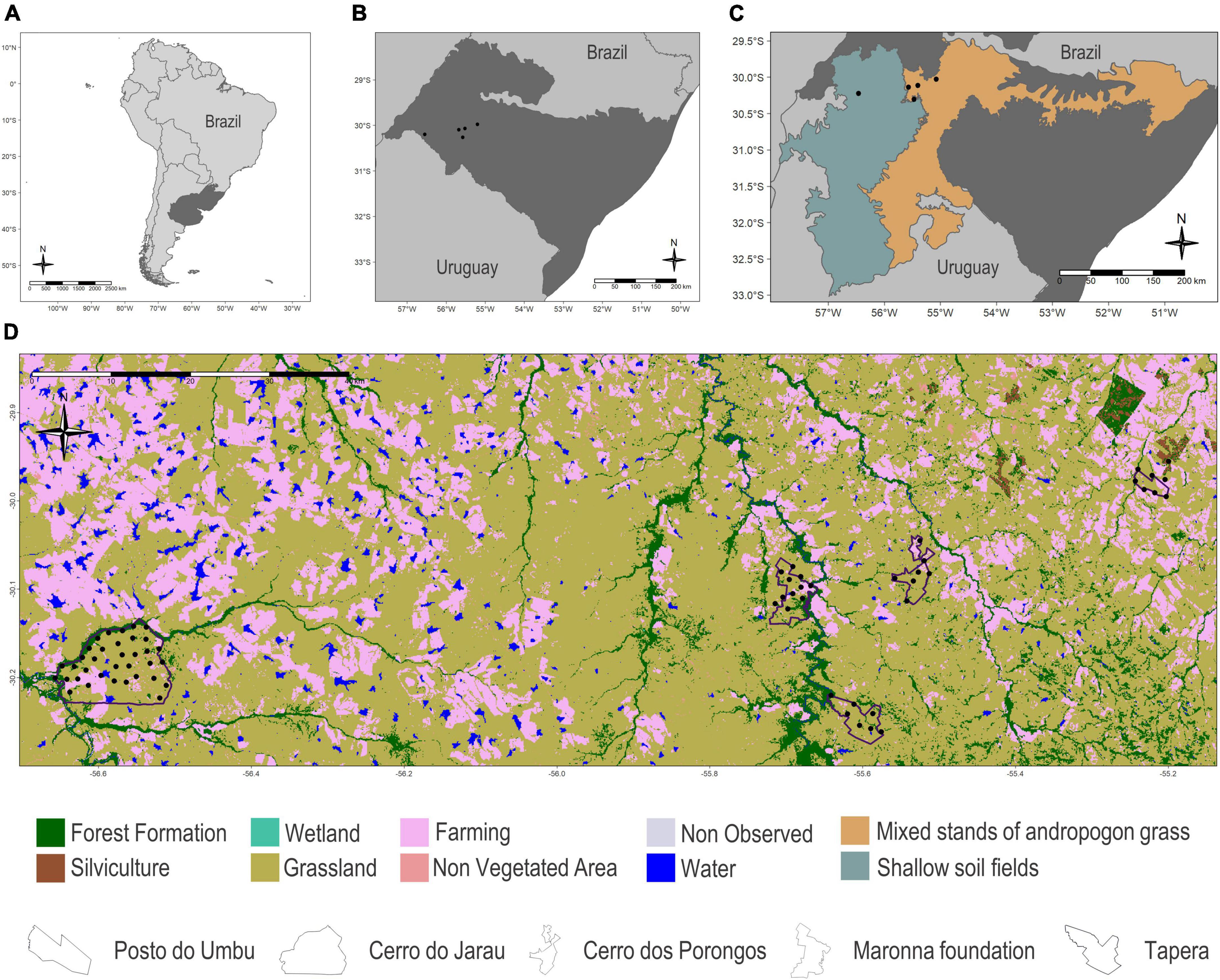
Figure 1. (A) Location of the Brazilian-Uruguayan savanna ecoregion within South America in dark grey. (B) Location of the five areas in the municipalities of Alegrete, Cacequi, and Quaraí, Brazilian Pampa, for bat acoustic sampling during the spring and summer of 2019 and 2020. (C) The two main physiognomies occurring in the Brazilian and Uruguayan grasslands: in turquoise the shallow soil fields and in orange the mixed stands of andropogon grass. (D) Land use and land cover map (MapBiomas Pampa Sudamericano Project, 2021) for the five sampled areas (Posto do Umbu, Tapera, Cerro do Jarau, Maronna foundation, and Cerro dos Porongos).
The climate is sub-tropical, with average annual temperatures ranging from 16 to 22°C and annual rainfall varying from 1,200 to 1,600 mm (Pillar and Lange, 2015). The geological formation is complex, including recent marine sedimentary deposits to ancient pre-Cambrian formations, in some areas of the South-Rio-Grandense Shield (Chernicoff and Zappettini, 2004). The relief is relatively homogeneous and flat, varying from sea level up to about 400 m elevation (Pillar and Lange, 2015). Currently, only 46% of Brazilian Pampa remains covered by native vegetation and only 2% is legally protected by conservation units; this makes the Pampa the least protected domain in Brazil (Souza et al., 2020). Still, many landowners subscribed the Alianza del Pastizal’s initiative, aiming for the conservation of natural grasslands through the production of certified environmentally sustainable meat, an initiative led by BirdLife International and developed through local partners in Brazil, Uruguay, Argentina, and Paraguay (Save Brasil, Aves Uruguay, Aves Argentina, and Guyra Paraguay).
Acoustic monitoring and analysis
We monitored 68 sites located at least 1.5 km apart, during the spring and summer of 2019 and 2020 (Figure 1). Autumn and winter are rainy, windy, and cold, which deemed acoustic bat monitoring inefficient. We sampled bats using automated sound detectors – AudioMoths (Silicon Labs) – set at a sampling rate of 256 kHz, 16 bits, programmed to record cuts of 15 s every 2 min from sunset to sunrise, for at least five nights in each site. We also coupled automated temperature and humidity sensors to each active AudioMoth to obtain detailed information on the microclimatic conditions associated with each bat record.
Species identification was done through manual analysis of recordings in Raven Pro 1.6 Software (K. Lisa Yang Center for Conservation Bioacoustics, 2022) selecting a Hamming window with 1,024 Fast Fourier Transformation (FFT; overlap 93%) to minimise the effect of spectral dispersion. We evaluated the following parameters: the overall shape of the pulse (constant frequency, CF; modulated frequency, FM; quasi-constant frequency, qCF; and variations therein), frequency modulation (FM), number of harmonics (nH), peak frequency (PF), duration (D), inter-pulse interval (IPI), frequency of maximum energy (FME), maximum frequency (Fmax), minimum frequency (Fmin), and bandwidth (BW = Fmax – Fmin). Whenever possible, we identified bat recordings to the species level, following acoustic identification keys for Neotropical and Brazilian bats (Barataud et al., 2013; López-Baucells et al., 2016; Arias-Aguilar et al., 2018). We quantified bat activity using sequences with at least three consecutive good quality (signal to noise ratio ≥ 12 dB; Jung et al., 2014) echolocation calls in a recording; each sequence that met this criterion was considered a “bat pass.” Species were classified as edge-space or open-space foragers according to Denzinger and Schnitzler (2013).
Landscape metrics
To test for spatial autocorrelation in our dataset, we calculated Moran’s I index (Supplementary Table 1) for each sampled area, using the “Moran.I” function from the R package ape (Paradis et al., 2019). We obtained the landscape metrics from a categorical land use raster of the Brazilian-Uruguayan savanna ecoregion for 2019 (MapBiomas Pampa Sudamericano Project, 2021). We created circular buffers of seven sizes (500 m, 1.0 km, 1.5 km, 2.0 km, 3.0 km, 4.0 km, and 5.0 km in radius) using the location of the AudioMoth as a centroid in each sampling site to extract the landscape metrics. Smaller buffers do not reflect the nightly dispersal ability of most of the species that potentially occur in the area. We calculated six structural landscape metrics for all buffers sizes at Landscape and Class levels (Table 1), using a classification raster of eight categories: forest formation, silviculture, wetland, grassland, farming, non-vegetated area, non-observed, and water. For class-level metrics, we used eight directions (queen’s case) that correspond to the number of directions in which patches may be connected, as recommended in McGarigal et al. (2012). All metrics were extracted using the landscapemetrics package (Hesselbarth et al., 2019) in R Program version 4.0.4 (R Core Team, 2021).

Table 1. Selected landscape metrics and respective level, code and raster reclassification, for bat acoustic sampled during the spring and summer of 2019 and 2020 in the Brazilian Pampa.
Single-season occupancy models and extrapolations
We used detection/non-detection records to build single-season occupancy models (MacKenzie et al., 2002, 2017). We treated each survey location as a sample unit and each night was halved to represent a survey occasion, resulting in two occasions per night per site. We used mean temperature and mean air relative humidity as detection covariates (p). We used the abovementioned landscape metrics as occupancy covariates (Ψ). All covariates were standardised by subtracting the mean and dividing by the standard deviation, resulting in mean μ = 0 and standard deviation σ = 1. Multicollinearity was evaluated using the variance inflation factor (VIF), using the “vifstep” function from R package usdm (Naimi, 2017), and only variables with VIF < 3 were used for the modelling (Zuur et al., 2010). After the VIF analysis, our model included three landscape metrics at all selected scales (Enn_mn, Shei, and Pland_nvc), all showing a VIF value below 3 (Supplementary Table 2).
We fitted all models using the “occu” function in the unmarked R package (Fiske and Chandler, 2011). Detectability was modelled using the covariates mean relative humidity (humi) and mean temperature (temp), including the null model. Occupancy probability was modelled using the best detection model combined with landscape variables at each of the seven scales analysed, totalling 4 models for detection (Supplementary Table 3) and 21 models for occupancy by species (Supplementary Table 4). We ranked the models using the second-order Akaike Information Criterion (AICc) and the determination coefficient (r2), and considered as the best-supported models for those with ΔAICc < 2 (Burnham and Anderson, 2002). To evaluate models’ goodness-of-fit (GoF), we used three discrepancy measures: sum of squared errors, Pearson’s Chi-squared, and Freeman-Tukey Chi-squared, with at least 1,000 bootstraps. Additionally, to evaluate the model’s fit based on the frequencies of the detection histories, we used the goodness-of-fit test recommended by MacKenzie and Bailey (2004), where well-fitted models should return a p-value > > 0.05. As a measure of dispersion, we used the c-hat value as follows: c-hat < 1 indicates underdispersion, we keep the value of c-hat at 1; 1 < c-hat < 3 indicates moderate overdispersion, so we multiply the variance-covariance matrix of the estimates by c-hat, and as result, the SEs of the estimates are inflated, according to Burnham and Anderson (2002). This was done using the R package AICcmodavg (Mazerolle, 2020). To predict the probability of occupancy at each location, we used the “modavgPred” function in the AICcmodavg R package (Mazerolle, 2020), while considering the uncertainty of defining the best model using the averaged parameters across the best-ranked models (AICc < 2; Burnham and Anderson, 2002). In addition, we also used the average of the best models to see how occupancy changes with the covariates.
To spatially extrapolate our results, we used a raster of the MapBiomas at a scale of 1:250,000 (MapBiomas Pampa Sudamericano Project, 2021) and cropped it with the adapted shapefile of ecological systems from the Uruguayan Savanna Ecoregion at a scale of 1:500,000 (Hasenack et al., 2010). With the final raster on the scale of 1:250,000, we extracted the polygons representing the two mains sampled phytophysiognomies (shallow soil fields and mixed stands of andropogon grass), covering areas of the Brazilian and Uruguayan Pampa for each modelled scale. We created a square grid for each scale modelled on the entire polygon, extracted the coordinates of each cell, and extracted the same landscape metrics used as occupancy covariates, creating a new data frame to predict and extrapolate species occurrence across the region, using the model-averaged parameters. We standardised this data, by the mean and standard deviation, and used this information to extrapolate our predictions and to create occurrence maps for each species for the region using raster (Hijmans, 2012), rgdal (Bivand et al., 2015), sp (Pebesma and Bivand, 2005), and tmap R package (Tennekes, 2018).
Results
We detected bat-passes in 8,111 (8.15%) out of 99,526 recordings. We recognised the occurrence of at least 23 taxa in the studied area, and we were able to identify 11 species of the Molossidae and the Vespertilionidae families. Four species were categorised as open-space foragers – Molossus cf. currentium, Molossus, Molossus rufus, and Promops centralis. Seven species were categorised as edge-space foragers, including one molossid – Molossops temminckii – and six vespertilionids – Eptesicus brasiliensis, Eptesicus furinalis, Lasiurus blosevillii, Myotis albescens, Myotis ruber, and Histiotus cf. velatus (Table 2).
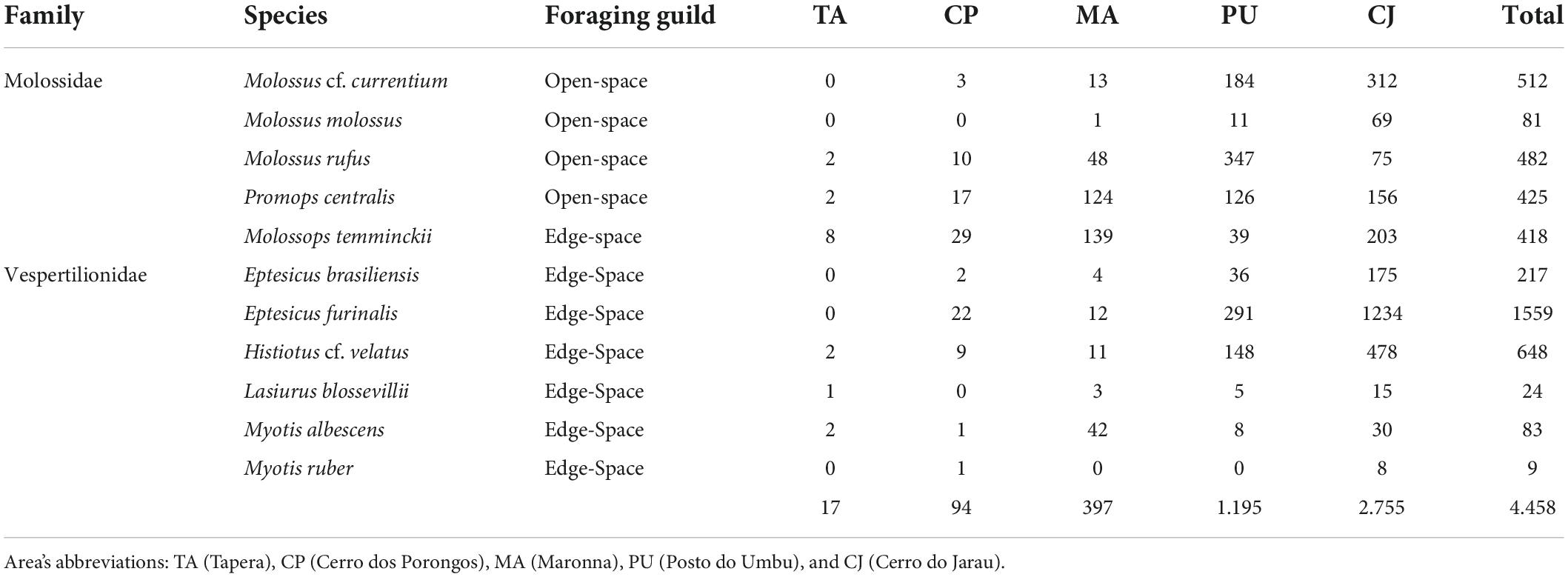
Table 2. Total number of bat passes in each of the five sampled areas, and information on species, family, and foraging guild, regarding bat acoustic sampling during spring and summer 2019 and 2020 in the Brazilian Pampa.
Site occupancy models
Lasiurus blosevillii and Myotis ruber were excluded from occupancy modelling because they accounted for less than 1% of the total bat passes. Of the nine bat species included in the occupancy modelling, E. furinalis presented the highest finite-sample occupancy (ψFS = 55.97), followed by M. cf. currentium (ψFS = 55.81), H. cf. velatus (ψFS = 54.47), P. centralis (ψFS = 51.83), M. temminckii (ψFS = 47.98), E. brasiliensis (ψFS = 44.78), M. rufus (ψFS = 44.69), M. albescens (ψFS = 36.33) and, finally, M. molossus (ψFS = 34.33). Most species were registered in over half the sites; however, the highest number of bat passes does not necessarily reflect occurrence in more sites (Table 2 and Figure 2).
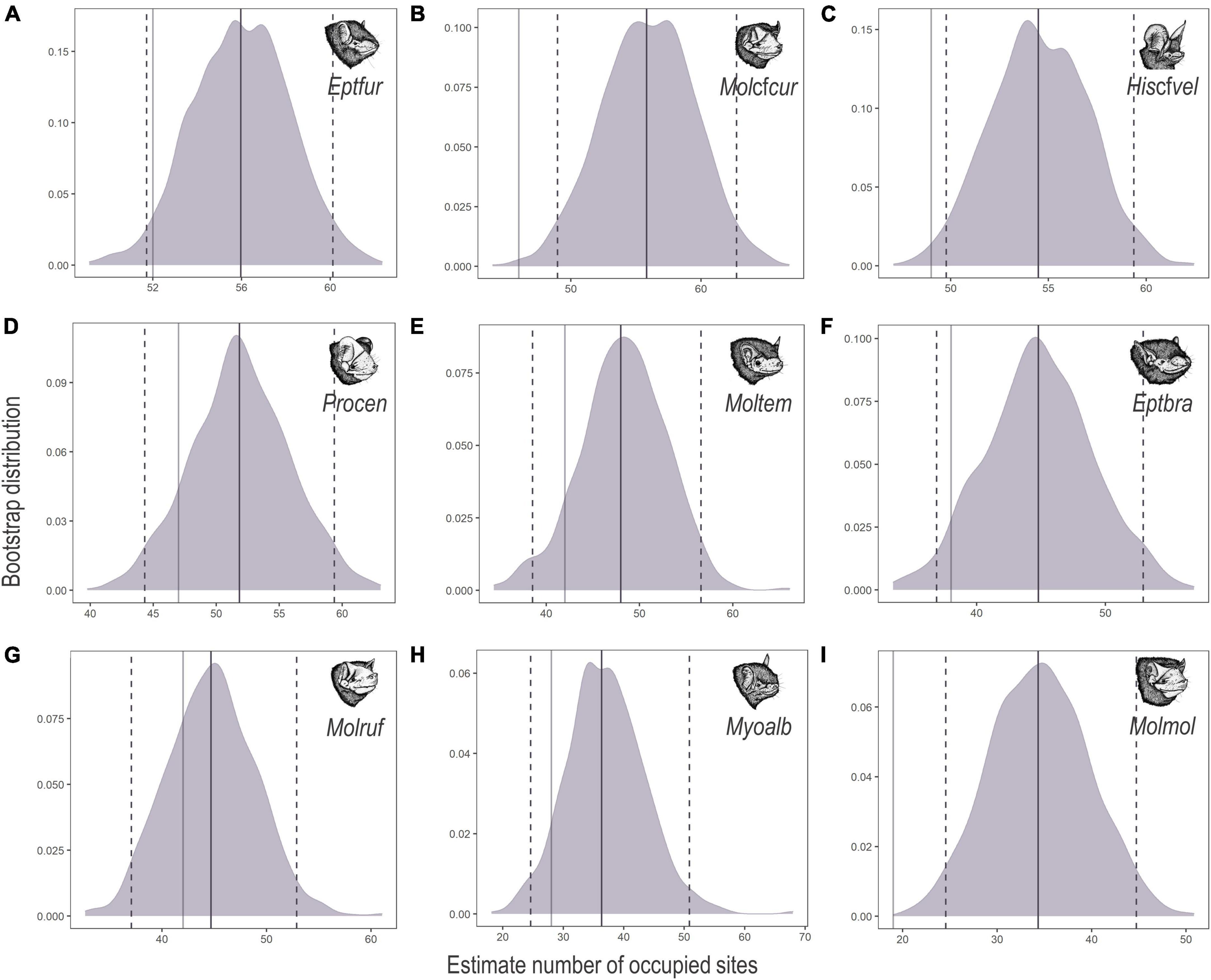
Figure 2. Bootstrap distributions of the estimated number of sites occupied (ψFS) by (A) Eptesicus furinalis (Eptfur), (B) Molossus cf. currentium (Molcfcur), (C) Histiotus cf. velatus (Hiscfvel), (D) Promops centralis (Procen), (E) Molossops temminckii (Moltem), (F) Eptesicus brasiliensis (Eptbra), (G) Molossus rufus (Molruf), (H) Myotis albescens (Myoalb), and (I) Molossus molossus (Molmol) across 68 acoustic survey sites in the Brazilian Pampa during spring and summer 2019 and 2020. Mean occupancy estimates (solid purple lines) and 95% confidence intervals (dashed purple lines) are shown, along with the total number of survey sites at which each species was detected (solid grey lines). Panels are in order of decreasing probability of occupancy.
The models of occupancy converged and showed good fits for the nine species, with discrepancy values ranging from 0.01 to 0.93 and c-hat ranging from 0.55 to 2.88. Detection estimates ranged from 0.10 to 0.55, while occupancy estimates ranged from 0.51 to 0.99 (Figure 3).
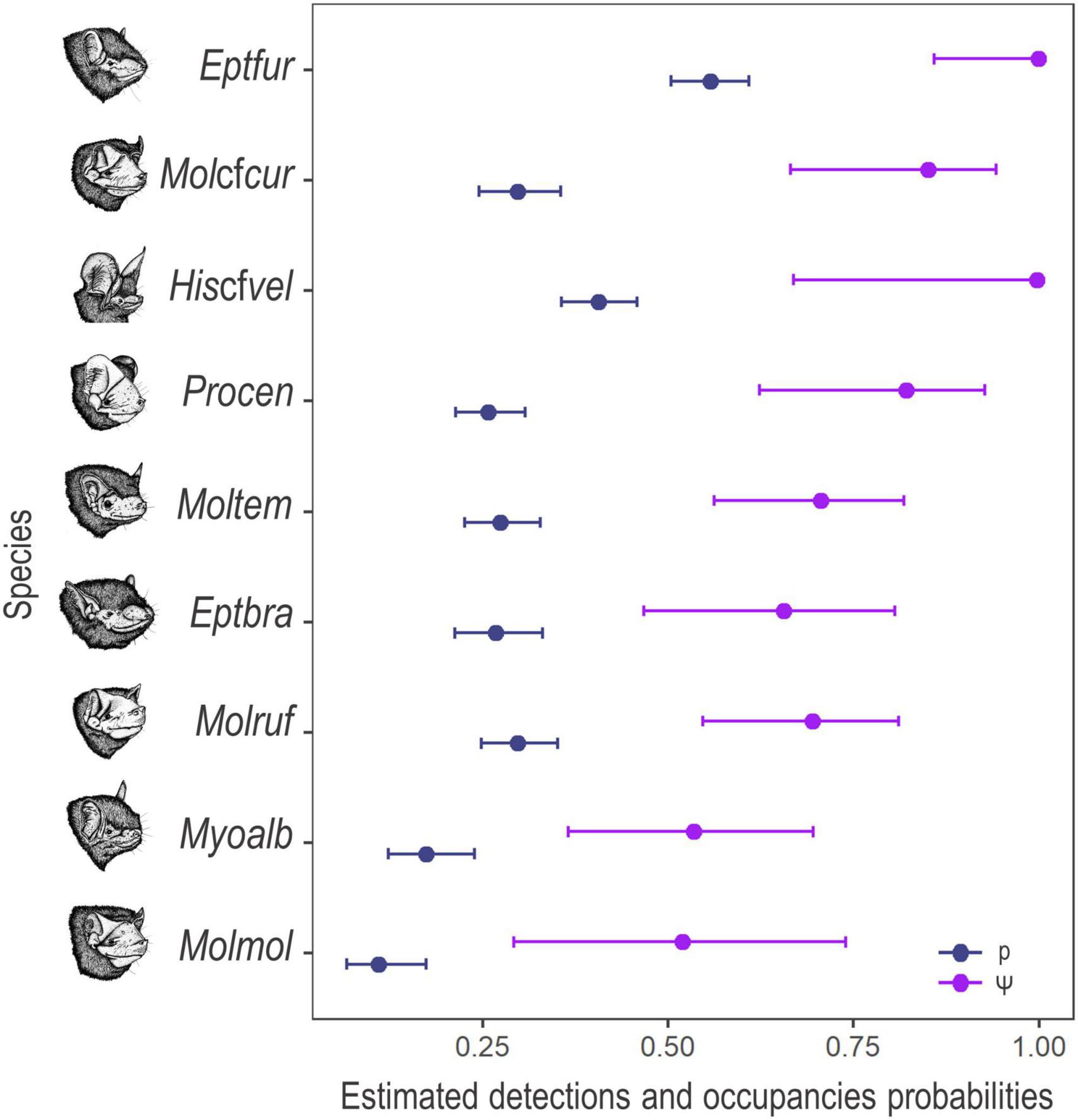
Figure 3. Estimated detection (blue colour) and occupancy (purple colour) mean probabilities and 95% confidence interval for the nine species of bats, recorded in the Brazilian Pampa during the spring and summer of 2019 and 2020. Eptesicus furinalis (Eptfur), Molossus cf. currentium (Molcfcur), Histiotus cf. velatus (Hiscfvel), Promops centralis (Procen), Molossops temminckii (Moltem), Eptesicus brasiliensis (Eptbra), Molossus rufus (Molruf), Myotis albescens (Myoalb), and Molossus molossus (Molmol).
Effect of microclimate on bat detection probability
Among the sampled sites, the mean temperature ranged from 8.03 to 31.99°C while the mean relative humidity varied from 37.1 to 99.88%. Best supported models (ΔAICc < 2 and β p-value < 0.05) for the probability of detection varied by species, guild, and scale. Considering the highest weighted models, mean temperature and mean relative humidity positively affected the detection probability of the edge-space forager E. brasiliensis and the open-space forager M. cf. currentium at the 500 m scale and of the edge-space forager H. cf. velatus at the 5.0 km scale (Table 3). The detection probability of the edge-space forager M. temminckii increased with mean temperature at the 500 m scale, the open-space forager M. molossus responded positively to the temperature at the intermediate scale of 3.0 km, and the edge-space forager E. furinalis and the open-space forager M. rufus had a similar response, but at the larger scale of 5.0 km. The detection of M. albescens and P. centralis did not respond to any of the microclimatic variables.
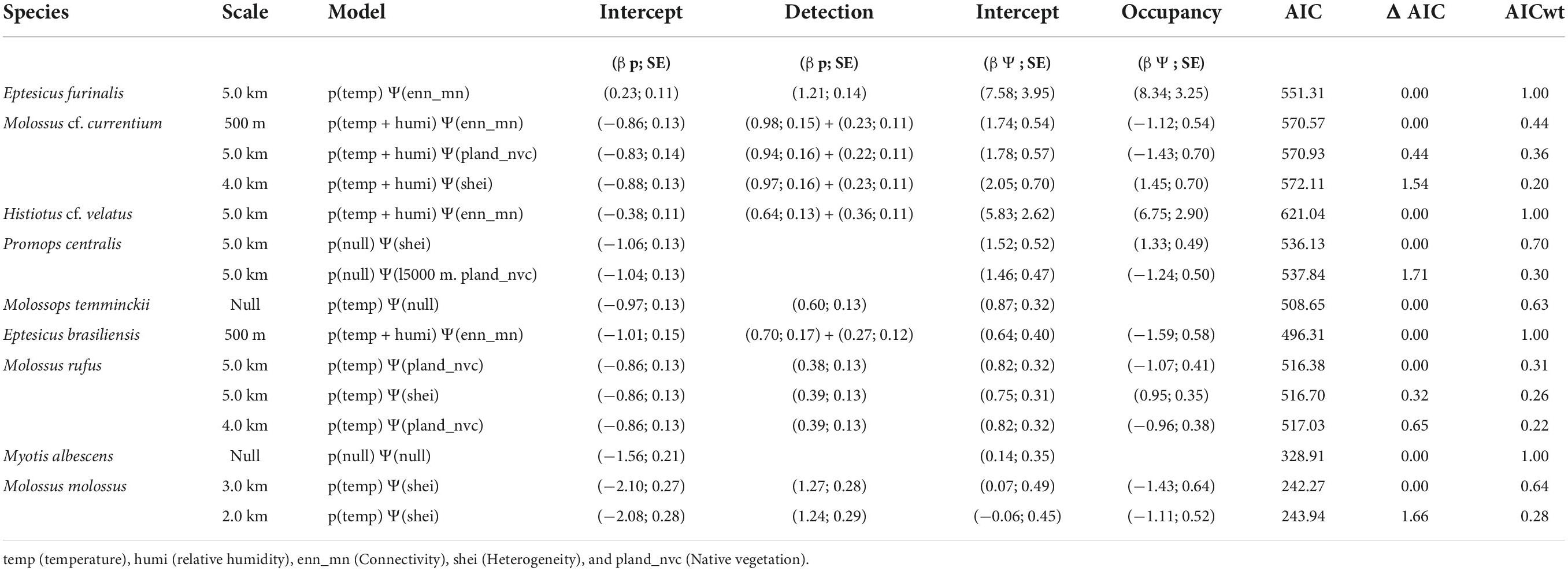
Table 3. Summary of the best-fit models and the significant variables for detection (p) and occupancy (Ψ) for nine species of bats recorded in the Brazilian Pampa during spring and summer 2019 and 2020.
Effect of landscape structure on bat site-occupancy probability
Considering the highest weighted models, connectivity is increased, that is, the closer the fragments of favourable habitat are, the greater the chances of occupancy by E. brasiliensis and M. cf. currentium at the 500 m scale. The open-space forager M. molossus was the only species whose probability of occupancy responded to landscape heterogeneity, and negatively, at the 3.0 km scale. The probability of occupancy of the edge-space foragers E. furinalis and H. cf. velatus was negatively affected by connectivity at the 5.0 km scale, while the open-space forager P. centralis responded positively to landscape heterogeneity at the same scale. M. rufus responded negatively to native vegetation cover and positively to landscape heterogeneity at the 5.0 km scale. Meanwhile, the occupancy of the edge-space foragers M. albescens and M. temminckii did not significantly respond to any of the evaluated landscape metrics (Table 3).
Spatial extrapolation of the species’ occupancy
Species showing the highest estimated occupancy probabilities (values ranging from βψ 0.55 to 1.00) in the two main phytophysiognomies – shallow soil fields and mixed stands of andropogon grass – present in the Brazilian and Uruguayan portion of the Uruguayan Savanna were E. furinalis and H. cf. velatus. Those were followed by M. rufus and P. centralis (values ranging from βψ 0.34 to 0.99), and finally, E. brasiliensis, M. cf. currentium, and M. molossus with moderate occupancy probabilities (values ranging from βψ 0.04 to 0.89; Figure 4).
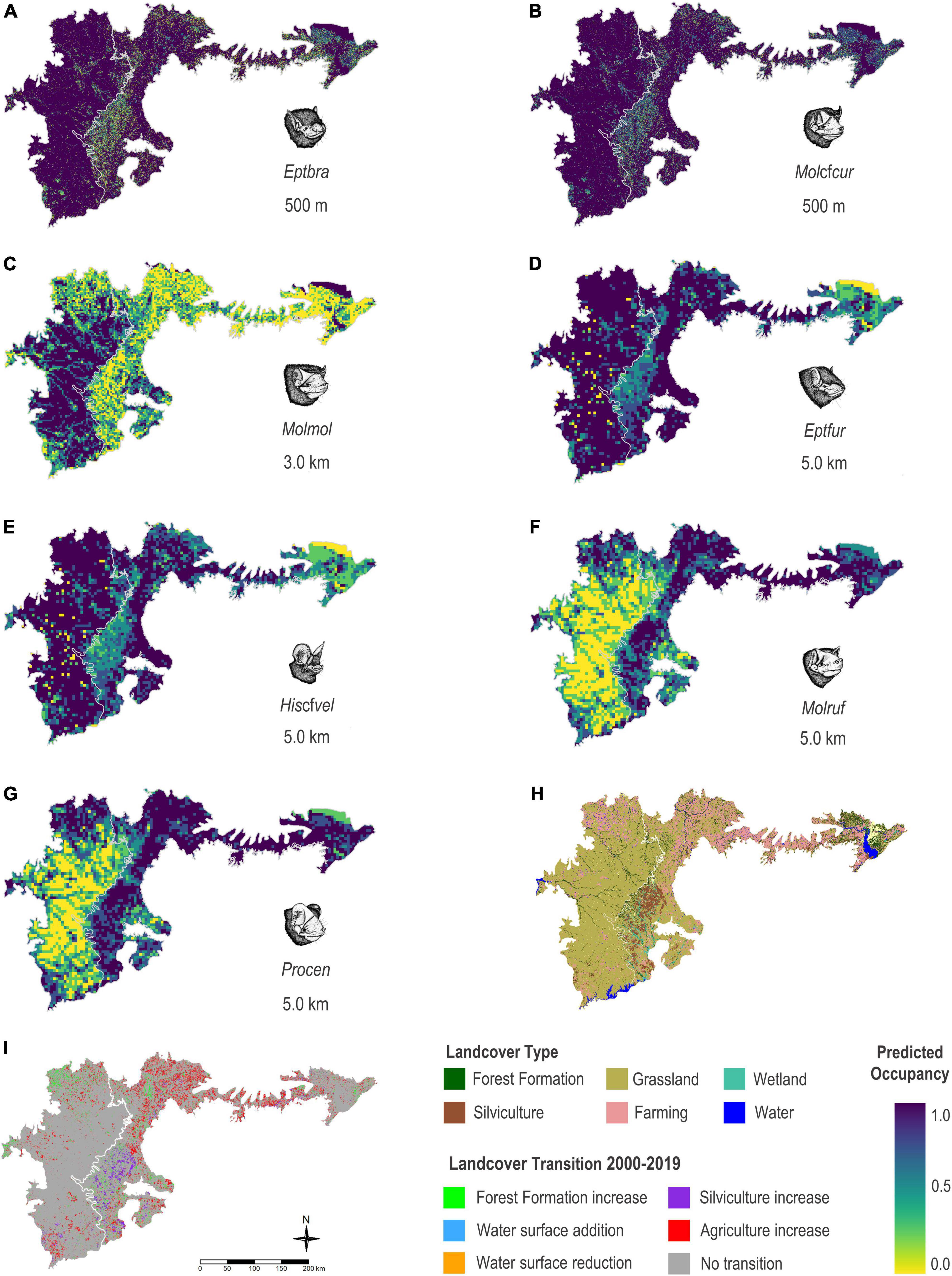
Figure 4. Estimated occupancy for nine species of bats for the area occupied by two main phytophysiognomies present in the Brazilian and Uruguayan portion of the Uruguayan Savanna – shallow soil fields and mixed stands of andropogon grass, based on the modelling results using the bat acoustic data gathered in the Brazilian Pampa during spring and summer 2019 and 2020. (A) Eptesicus brasiliensis (Eptbra), (B) Molossus cf. currentium (Molcfcur), (C) Molossus molossus (Molmol), (D) Eptesicus furinalis (Eptfur), (E) Histiotus cf. velatus (Hiscfvel), (F) Molossus rufus (Molruf), (G) Promops centralis (Procen), (H) map showing landcover types, and (I) map showing the land use and land cover transition between the years 2000 and 2019.
The projected occupancy of the edge-space foragers, H. cf. velatus, E. brasiliensis, E. furinalis, and the open-space forager, M. cf. currentium, was higher in areas characterised by native forests including the espinilho tree, riparian forests, and water bodies, but was still moderate in areas characterised by monoculture plantations of fast-growing exotic trees and agriculture. On the other hand, the projected occupancy of the open-space forager M. molossus was higher in large open areas of native grasslands, with patches of native forest formations and small and medium water bodies, with considerable restriction of potential occurrence in environments with an excess of silviculture and agriculture. On the other hand, the projected occupancy of M. rufus and P. centralis was more related to heterogeneous elements of the landscape, floodplains, and large bodies of water, but still moderate in matrices of silviculture and agriculture.
Discussion
Different bats perceive the landscape differently
We predicted that species occupancy should be influenced by landscape structure, with structural connectivity as the most important factor affecting the occupancy of edge-space foraging bats. This prediction assumed that, in altered landscapes, well-connected woodland patches should provide increased availability of roosts, temporary perches, and foraging resources, determining the occupancy of edge-space aerial insectivorous bats. Indeed, the occupancy of three of the five edge-space foragers detected, E. brasiliensis, E. furinalis, and H. cf. velatus responded to the structural landscape connectivity, although the first positively and the two latter negatively. Also, the occupancy of the open-space forager M. cf. currentium was positively affected by this landscape trait.
Molossus cf. currentium and Eptesicus brasiliensis are seldom captured by mist nets and, therefore, we do not have basic information about these species in the Brazilian-Uruguayan savanna ecoregion (Reis et al., 2017; Barquez and Díaz, 2020). Our results indicate that these bats prefer well-connected natural landscape elements rather than extensive open areas or anthropogenic elements at local scales. Similar results were found for aerial insectivorous bat assemblages in modified landscapes of the Brazilian Atlantic Forest, where bat activity and composition responded to landscape structure at small scales (Falcão et al., 2021). Landscape elements, particularly trees, especially in open areas, may provide roosting and foraging sites with potentially greater insect availability and protection from wind (Russ et al., 2003). Open-space foragers, despite their general increased dispersal ability, may also avoid flying in completely open areas to minimise predation risks (Jones and Rydell, 1994) or because they use linear elements of the landscape, such as forest patches, perhaps for increased spatial orientation (Verboom and Huitema, 1997).
The occupancy of E. furinalis and H. cf. velatus was negatively affected by structural landscape connectivity at a larger scale. Previous studies have shown that the connectivity between forest patches is directly related to the quality and permeability of the surrounding matrix (Kupfer et al., 2006; Watling et al., 2011). In modified landscapes, the matrix can positively influence the occurrence and persistence of many species, for example, by providing greater diversity of prey, corridors, and stepping stones, or negatively, for instance, acting as a dispersal barrier (Ricketts, 2001; Bernard and Fenton, 2007; Watling et al., 2011; Brändel et al., 2020; Farneda et al., 2020; Mendes and Srbek-Araujo, 2021). E. furinalis and H. cf. velatus seem to avoid areas composed of large extensions of exotic trees and monocultures. Possibly, such a matrix reduces the possibility of acquiring resources, such as food, protection, and roosts even for bats able to fly long distances, making it difficult for them to move between the favourable neighbouring fragments of native vegetation. Occupancy should thus be higher in areas showing small patches of native or riparian vegetation, or even other isolated elements in the landscape, such as scattered trees. Our results are consistent with those found for aerial insectivorous bats in rural landscapes in southeastern Australia (Lumsden and Bennett, 2005) and for fruit bats in the northernmost portion of Mexico (Galindo-González and Sosa, 2008), where in both cases, trees dispersed in rural land mosaics showed high value as foraging or roosting habitat for bats.
We also predicted that landscape heterogeneity would positively affect the occupancy of open-space bats. Still, only the occupancy probabilities of P. centralis increased with landscape heterogeneity at the largest scale. The occupancy by P. centralis seems to be favoured by the combination of suitable habitat patches immersed in a complex mosaic of other land use cover types in the surrounding matrix. Positive relationships between heterogeneous habitats and bat species diversity are well-documented at local and regional scales (Ramos Pereira et al., 2009; Frey-Ehrenbold et al., 2013; Mendes et al., 2017; Monck-Whipp et al., 2018). Artificial elements dispersed in the landscape, such as artificial ponds, created for irrigation in agricultural landscapes, perhaps provide greater variability and availability of foraging resources and drinking opportunities for bats (Korine et al., 2015; Ancillotto et al., 2019). The presence of ponds and even artificial lighting can change the dynamics of competitive exclusion by food disputes between bats with different sensitivities (Arlettaz et al., 2000; Russo and Ancillotto, 2015), promoting more diverse and abundant prey assemblages at different times of the night. Like most molossids, P. centralis presents great plasticity in its echolocation calls, probably allowing individuals of this species to explore a wide range of habitats, including urban areas (Hintze et al., 2020).
Contrary to our expectations, the occurrence of M. molossus seems to follow an opposite trend – this species preferred less heterogeneous habitats at medium scales (2.0 – 3.0 km). M. molossus is considered relatively common in urban areas and usually uses artificial roosts (Pacheco et al., 2010). Despite this, our models suggest that this species avoids areas with excess silviculture, preferring homogeneous areas in large open landscapes characterised by native grasslands and scattered native trees. M. molossus seems to take advantage of linear landscape elements, which is in line with previous findings, where linear elements in agricultural landscapes appear to be more attractive for some aerial insectivorous bats (Verboom and Huitema, 1997; Boughey et al., 2011; Toffoli, 2016; Rodríguez-San Pedro et al., 2018; Finch et al., 2020), probably because they are used as orientation landmarks and reference points during flight (Schnitzler et al., 2003; Schaub and Schnitzler, 2007), creating corridors for daily commute routes or occasional regional dispersal (Gelling et al., 2007).
Increased native vegetation cover does not favour the probability of occupancy of the black mastiff bat, M. rufus, contradicting our hypothesis that this landscape feature would favour the occupation of all species, especially the edge-space foragers. M. rufus is the largest species of this genus in the Brazilian territory and presents gregarious and synanthropic habits (Barquez et al., 1999; Esbérard et al., 1999). Our results suggest that, as for P. centralis, M. rufus presents higher occupancy in areas with greater heterogeneity and environmental complexity. This is especially concerning as areas, where the occupancy of the two species is maximum, are being rapidly converted by humans, through intensive livestock production in natural grasslands, and silviculture and agriculture in otherwise converted fields.
Finally, edge-space foragers M. temminckii and M. albescens did not respond to any landscape metrics at the different scales evaluated. M. temminckii is the only species of the Molossidae capable of exploring background-cluttered environments while remaining able to exploit clearings and overall open environments, by changing from short downward frequency-modulated calls with short pulse intervals in cluttered environments to long upward frequency-modulated calls with longer intervals and shorter bandwidth in uncluttered sites (Oliveira et al., 2018). M. albescens is one of the most widely distributed species of its genus, occurring from southern Mexico to Brazil (Braun et al., 2009), this may be mirrored by a wide dietary plasticity, favoured by the changes in the activity of different species of prey at different thermal niches during the night. M. albescens is known for its trawler-style foraging and is therefore often captured in wetlands, using its high-frequency echolocation calls to detect its prey and capture it with its feet (Fenton and Bogdanowicz, 2002). Perhaps the phenotypic plasticity of M. temminckii and the foraging style of M. albescens make them less sensitive to connectivity, heterogeneity, or the amount of native vegetation cover in open grasslands at the analysed scales or they perceive the landscape at even larger scales. Due to the high specificity of bat responses to landscape configuration, the multiple-scale approach proved to be crucial to understand how bats respond to different changes in the composition and configuration of the landscape, considering the matrix and its quality (Meyer and Kalko, 2008; Martins, 2016).
Microclimate and bat detection: Finding species optimum detection interval
Temperature and relative humidity had, overall, positive effects on the detection of most bat species. However, nightly temperatures probably did not get high enough (maximum temperature recorded 33°C, but in 95% of the nights below 20°C) to test the prediction that, above a given limit, bats would decrease their activity in response to the increased cost of overheating. However, our data suggest that the optimum temperature range is between 19°C and 24°C, the interval within which we recorded most bat passes (Supplementary Figure 1). Generally, the feeding activity of aerial insectivores tends to increase on warmer nights due to the greater activity of their prey, flying arthropods (Racey and Swift, 1985; Aldridge and Rautenbach, 1987; Schowalter, 2006). Thus, we can assume that if bats are more active, they will soon be more detected, as has been effectively observed in temperate environments (Kaiser and O’Keefe, 2015; Blakey et al., 2019). Still, above certain temperatures, the cost of endothermy, specifically cooling may become too high for animals with such increased metabolism. Indeed, despite the advantages and efficiency of air transport, the flight is a metabolically expensive mode of locomotion: the metabolic rates of flying bats can be as high as 3 to 5 times those of terrestrial mammals of the same size, with heart rates going over 1,000 beats per minute (Thomas and Suthers, 1972; Maina, 2000). Bats have several metabolic adaptations and perhaps they also show behavioural adaptations, avoiding too high temperatures even if their preys are still available, particularly if the gain by ingestion does not compensate for the physiological risk of overheating. However, nightly temperatures above 25°C seem to be rare even in the Pampean summer, so a scenario where bats trade-off hunting for inactivity because of potential overheating seems unlikely.
Reducing the Wallacean shortfall through acoustic monitoring
Here, we present the first comprehensive study of aerial insectivorous bats in the Brazilian Pampa using acoustic monitoring. Previous records resulted almost exclusively from mist-net sampling and roost searches (but see Barros, 2012), and represented less than 20% of the species occurring in Brazil (Garbino et al., 2020, 2022). With acoustic monitoring we added to the Pampa biome, records for two new species – P. centralis and M. cf. currentium – and records for at least six species from the genera Cynomops, Eumops, Nyctinomops, Eptesicus, Histiotus, and Molossus were to be identified in the future, when we can unambiguously identify the species of those complexes through their echolocation calls. Therefore, we estimate that the bat fauna of the Brazilian Pampa may easily exceed 40 species. Through acoustic monitoring, it is evident that the bat fauna of the Pampa is far more diverse than previously thought, deserving more attention, both from researchers and government agencies.
Most detected species are categorised as least concern, although E. brasiliensis and P. centralis are also still lacking basic ecological information, including distribution ranges, population sizes, and regional threats to their conservation, which is certainly preventing an adequate assessment of their conservation status; M. cf. currentium is classified as data deficient in Brazil (ICMBio, 2018). Indeed, it was recently shown that the distribution of P. centralis is much broader in South America than previously predicted, adding over 3.8 million km2 to its former known area (Hintze et al., 2020). Our work has shown that the distribution of this species goes even further south in the Neotropic and that its occupancy is far from low in the Pampean landscapes. Also, if confirmed, the detection of M. cf. currentium would represent the southernmost record of the species in Brazil, and the first for the Brazilian portion of the Uruguayan Savanna; this species also shows a high probability of occupancy in the region, potentially suggesting that this is not its distribution limit and that, perhaps, it has a much wider distribution range than previously thought.
The occupancy estimates projected for the regions neighbouring those sampled suggest that the landscape is reasonably favourable for the overall assemblage of aerial insectivorous bats, particularly the areas with native elements including the espinilho tree, Vachellia caven, wetlands, water, and riparian forests. This remains to be validated by further field data and for the remaining regions of the Uruguayan Savanna, but sites with those landscape elements are potentially the ones with higher probabilities of detection of aerial insectivore bats. Our results significantly add to the knowledge of Pampean bats and are alarming: environments characterised by native vegetation, highly suitable for the occupancy of a wide range of diversity of aerial insectivorous bat species, are rapidly disappearing by intense anthropogenic activities. In the last two decades, silviculture and agriculture have increased their areas by 34 and 44%, respectively, replacing native grasslands, particularly in mixed stands of the native andropogon grass. In shallow soil fields, intensive agriculture is almost impracticable and is here replaced by intensive livestock production. Such economic activities, when carried out inappropriately and at such excessive levels, often cause direct contamination of water resources by the release of pesticides and herbicides, leading to soil depletion and unproductivity, while facilitating the establishment of invasive plant species that generate not only loss of biodiversity, but also the de-characterisation of the gaucho cultural identity.
For all these reasons, future acoustic monitoring is paramount in areas of the Brazilian Pampa undergoing more intense anthropogenic changes, where acoustic monitoring will be key to increasing the knowledge of the distribution and occupancy of aerial insectivores, and how the ecosystem services provided by these bats, particularly in the context of the traditional agricultural systems, are being affected.
Data availability statement
The original contributions presented in this study are included in the article/Supplementary material, further inquiries can be directed to the corresponding author/s.
Author contributions
CC and MR contributed equally to the article and approved the submitted version.
Funding
We would like to thank the Rufford Foundation for granting our project Bats of the neglected Brazilian-Uruguayan savanna: occupancy, diversity, and conservation with a Small Grant (28810-1 ID). We also thank the Brazilian National Council for Scientific and Technological Development – CNPq for financial support in the form of an MSc scholarship to CC and a productivity grant to MR. This work was supported by the Coordenação de Aperfeiçoamento de Pessoal de Nível Superior – Brasil (CAPES) – Funding Code 001.
Acknowledgments
We thank all the landowners and land managers for their hospitality and support during our field activities and our colleagues at the lab for support in the field and data processing. Special thanks to Ugo Diniz for the bat illustrations used in the figures.
Conflict of interest
The authors declare that the research was conducted in the absence of any commercial or financial relationships that could be construed as a potential conflict of interest.
Publisher’s note
All claims expressed in this article are solely those of the authors and do not necessarily represent those of their affiliated organizations, or those of the publisher, the editors and the reviewers. Any product that may be evaluated in this article, or claim that may be made by its manufacturer, is not guaranteed or endorsed by the publisher.
Supplementary material
The Supplementary Material for this article can be found online at: https://www.frontiersin.org/articles/10.3389/fevo.2022.937139/full#supplementary-material
References
Aguiar, L. M. S., Bueno-Rocha, I. D., Oliveira, G., Pires, E. S., Vasconcelos, S., Nunes, G. L., et al. (2021). Going out for dinner—The consumption of agriculture pests by bats in urban areas. PLoS One 16:e0258066. doi: 10.1371/journal.pone.0258066
Aldridge, H. D. J. N., and Rautenbach, I. L. (1987). Morphology, echolocation and resource partitioning in insectivorous bats. J. Anim. Ecol. 56:763. doi: 10.2307/4947
Ancillotto, L., Bosso, L., Salinas-Ramos, V. B., and Russo, D. (2019). The importance of ponds for the conservation of bats in urban landscapes. Landsc. Urban Plan. 190:103607. doi: 10.1016/j.landurbplan.2019.103607
Appel, G., López-Baucells, A., Rocha, R., Meyer, C. F. J., and Bobrowiec, P. E. D. (2021). Habitat disturbance trumps moonlight effects on the activity of tropical insectivorous bats. Anim. Conserv. 24, 1046–1058. doi: 10.1111/acv.12706
Arias-Aguilar, A., Hintze, F., Aguiar, L. M. S., Rufray, V., Bernard, E., and Ramos Pereira, M. J. (2018). Who’s calling? Acoustic identification of brazilian bats. Mamm. Res. 63, 231–253. doi: 10.1007/s13364-018-0367-z
Arlettaz, R., Godat, S., and Meyer, H. (2000). Competition for food by expanding pipistrelle bat populations (Pipistrellus pipistrellus) might contribute to the decline of lesser horseshoe bats (Rhinolophus hipposideros). Biol. Conserv. 93, 55–60. doi: 10.1016/S0006-3207(99)00112-3
Barataud, M., Giosa, S., Leblanc, F., Rufray, V., Disca, T., and Tillon, L. (2013). Identification et écologie acoustique des chiroptères de Guyane française. Le Rhinolophe 19, 103–145.
Barquez, R. M., and Díaz, M. M. (2020). Nueva guía de los Murciélagos de Argentina, 1a ed. Chicago, IL: PCMA.
Barquez, R. M., Mares, M. A., and Braun, J. K. (1999). The Bats of Ar-gentina. Lubbock, TX: Special Publications Museum of Texas Tech Univer-sity. doi: 10.5962/bhl.title.142628
Barros, M. A. S. (2012). Atividade De Morcegos Insetívoros (Mammalia, Chiroptera) no pampa brasileiro: uso de hábtat e sazonalidade. Ph.D. thesis. Natal: Universidade Federal do Rio Grande do Norte.
Bellamy, C., Scott, C., and Altringham, J. (2013). Multiscale, presence-only habitat suitability models: Fine-resolution maps for eight bat species. J. Appl. Ecol. 50, 892–901. doi: 10.1111/1365-2664.12117
Bennett, A. F., Radford, J. Q., and Haslem, A. (2006). Properties of land mosaics: Implications for nature conservation in agricultural environments. Biol. Conserv. 133, 250–264. doi: 10.1016/j.biocon.2006.06.008
Bernard, E., Aguiar, L. M. S., and Machado, R. B. (2011). Discovering the Brazilian bat fauna: A task for two centuries? Mamm. Rev. 41, 23–39. doi: 10.1111/j.1365-2907.2010.00164.x
Bernard, E., and Fenton, M. B. (2007). Bats in a fragmented landscape: Species composition, diversity and habitat interactions in savannas of Santarém, Central Amazonia, Brazil. Biol. Conserv. 134, 332–343. doi: 10.1016/j.biocon.2006.07.021
Bivand, R., Keitt, T., and Rowlingson, B. (2015). rgdal: bindings for the geospatial data abstraction library. R package version 1.1-3. Available online at: https://cran.r-project.org/package=rgdal (accessed March 13, 2021).
Blakey, R. V., Webb, E. B., Kesler, D. C., Siegel, R. B., Corcoran, D., and Johnson, M. (2019). Bats in a changing landscape: Linking occupancy and traits of a diverse montane bat community to fire regime. Ecol. Evol. 9, 5324–5337. doi: 10.1002/ece3.5121
Boldrini, I. I. (2009). “A flora dos campos do Rio Grande do Sul,” in Campos sulinos: conservação e uso sustentável da biodiversidade, eds V. D. P. Pillar, S. C. Müller, Z. M. Castilhos, S. de, and A. V. A. Jacques (Brasília: MMA), 63–77.
Boughey, K. L., Lake, I. R., Haysom, K. A., and Dolman, P. M. (2011). Improving the biodiversity benefits of hedgerows: How physical characteristics and the proximity of foraging habitat affect the use of linear features by bats. Biol. Conserv. 144, 1790–1798. doi: 10.1016/j.biocon.2011.02.017
Brändel, S. D., Hiller, T., Halczok, T. K., Kerth, G., Page, R. A., and Tschapka, M. (2020). Consequences of fragmentation for neotropical bats: The importance of the matrix. Biol. Conserv. 252:108792. doi: 10.1016/j.biocon.2020.108792
Braun, J. K., Layman, Q. D., and Mares, M. A. (2009). Myotis albescens (Chiroptera: Vespertilionidae). Mamm. Species 846, 1–9. doi: 10.1644/846.1
Burnham, K. P., and Anderson, D. R. (2002). Model Selection And Multimodel Inference. New York, NY: Springer, doi: 10.1007/b97636
Calabrese, J. M., and Fagan, W. F. (2004). A comparison-shopper’s guide to connectivity metrics. Front. Ecol. Environ. 2, 529–536. doi: 10.1890/1540-9295(2004)002[0529:ACGTCM]2.0.CO;2
Chave, J. (2013). The problem of pattern and scale in ecology: What have we learned in 20 years? Ecol. Lett. 16, 4–16. doi: 10.1111/ele.12048
Chaverri, G., and Quirós, O. E. (2017). Variation in echolocation call frequencies in two species of free-tailed bats according to temperature and humidity. J. Acoust. Soc. Am. 142, 146–150. doi: 10.1121/1.4992029
Chernicoff, C. J., and Zappettini, E. O. (2004). Geophysical evidence for terrane boundaries in south-central argentina. Gondwana Res. 7, 1105–1116. doi: 10.1016/S1342-937X(05)71087-X
Cleveland, C. J., Betke, M., Federico, P., Frank, J. D., Hallam, T. G., Horn, J., et al. (2006). Economic value of the pest control service provided by Brazilian free-tailed bats in south-central Texas. Front. Ecol. Environ. 4, 238–243. doi: 10.1890/1540-9295(2006)004[0238:EVOTPC]2.0.CO;2
Cohen, Y., Bar-David, S., Nielsen, M., Bohmann, K., and Korine, C. (2020). An appetite for pests: Synanthropic insectivorous bats exploit cotton pest irruptions and consume various deleterious arthropods. Mol. Ecol. 29, 1185–1198. doi: 10.1111/mec.15393
Crooks, K. R., and Sanjayan, M. (2010). “Connectivity conservation: maintaining connections for nature,” in Connectivity Conservation, eds K. R. Crooks and M. Sanjayan (Cambridge, MA: Cambridge University Press), 1–20. doi: 10.1017/CBO9780511754821.001
da Costa, C. F., Arias-Aguilar, A., and Ramos Pereira, M. J. (2021). Aerial insectivorous bats in the Brazilian Pantanal: Diversity and activity patterns in response to habitat and microclimate. Mastozool. Neotrop. 28, 001–034. doi: 10.31687/saremMN.21.28.2.0.09.e0604
de Oliveira, T. E., de Freitas, D. S., Gianezini, M., Ruviaro, C. F., Zago, D., Mércio, T. Z., et al. (2017). Agricultural land use change in the Brazilian Pampa Biome: The reduction of natural grasslands. Land Use Policy 63, 394–400. doi: 10.1016/j.landusepol.2017.02.010
de Sousa-Neto, E. R., Gomes, L., Nascimento, N., Pacheco, F., and Ometto, J. P. (2018). “Land Use and Land Cover Transition in Brazil and Their Effects on Greenhouse Gas Emissions,” in Soil Management and Climate Change, eds M. Á Muñoz and R. Zornoza (Cambridge, MA: Elsevier), 309–321. doi: 10.1016/B978-0-12-812128-3.00020-3
Denzinger, A., and Schnitzler, H.-U. (2013). Bat guilds, a concept to classify the highly diverse foraging and echolocation behaviors of microchiropteran bats. Front. Physiol. 4:164. doi: 10.3389/fphys.2013.00164
Ducci, L., Agnelli, P., Di Febbraro, M., Frate, L., Russo, D., Loy, A., et al. (2015). Different bat guilds perceive their habitat in different ways: A multiscale landscape approach for variable selection in species distribution modelling. Landsc. Ecol. 30, 2147–2159. doi: 10.1007/s10980-015-0237-x
Ellis, E. C., Klein Goldewijk, K., Siebert, S., Lightman, D., and Ramankutty, N. (2010). Anthropogenic transformation of the biomes, 1700 to 2000. Glob. Ecol. Biogeogr. 19, 589–606. doi: 10.1111/j.1466-8238.2010.00540.x
Esbérard, C. E. L., Chagas, A. S., and Luz, E. M. (1999). Uso de residên-cias por morcegos no estado do rio de janeiro (Mammalia: Chiroptera). Rev. Bras. Med. Vet. 21, 17–20.
Estrada-Villegas, S., McGill, B. J., and Kalko, E. K. V. (2012). Climate, habitat, and species interactions at different scales determine the structure of a Neotropical bat community. Ecology 93, 1183–1193. doi: 10.1890/11-0275.1
Fahrig, L. (2003). Effects of Habitat Fragmentation on Biodiversity. Annu. Rev. Ecol. Evol. Syst. 34, 487–515. doi: 10.1146/annurev.ecolsys.34.011802.132419
Fahrig, L. (2013). Rethinking patch size and isolation effects: The habitat amount hypothesis. J. Biogeogr. 40, 1649–1663. doi: 10.1111/jbi.12130
Falcão, F., Dodonov, P., Caselli, C. B., dos Santos, J. S., and Faria, D. (2021). Landscape structure shapes activity levels and composition of aerial insectivorous bats at different spatial scales. Biodivers. Conserv. 30, 2545–2564. doi: 10.1007/s10531-021-02210-x
Farneda, F. Z., Meyer, C. F. J., and Grelle, C. E. V. (2020). Effects of land-use change on functional and taxonomic diversity of Neotropical bats. Biotropica 52, 120–128. doi: 10.1111/btp.12736
Fenton, M. B., and Bogdanowicz, W. (2002). Relationships between external morphology and foraging behaviour: Bats in the genus Myotis. Can. J. Zool. 80, 1004–1013. doi: 10.1139/z02-083
Finch, D., Schofield, H., and Mathews, F. (2020). Habitat associations of bats in an agricultural landscape: Linear features versus open habitats. Animals 10:1856. doi: 10.3390/ani10101856
Fiske, I. J., and Chandler, R. B. (2011). Unmarked: An R package for fitting hierarchical models of wildlife occurrence and abundance. J. Stat. Softw. 43:23. doi: 10.18637/jss.v043.i10
Frey-Ehrenbold, A., Bontadina, F., Arlettaz, R., and Obrist, M. K. (2013). Landscape connectivity, habitat structure and activity of bat guilds in farmland-dominated matrices. J. Appl. Ecol. 50, 252–261. doi: 10.1111/1365-2664.12034
Galindo-González, J., and Sosa, V. J. (2008). Frugivorous bats in isolated trees and riparian vegetation associated with human-made pastures in a fragmented tropical landscape. Southwest. Nat. 48, 579–589. doi: 10.1894/0038-4909(2003)048<0579:FBIITA>2.0.CO;2
Garbino, G. S. T., Brandão, M. V., and da Cunha Tavares, V. (2022). First confirmed records of Godman’s Long-tailed Bat, Choeroniscus godmani (Thomas, 1903) (Chiroptera, Phyllostomidae), from Brazil and Panama. Check List 18, 493–499. doi: 10.15560/18.3.493
Garbino, G. S. T., Gregorin, R., Lima, I. P., Loureiro, L., Moras, L., Moratelli, R., et al. (2020). Updated checklist of Brazilian bats. [Internet] Comitê da Lista de Morcegos do Brasil—CLMB. Sociedade Brasileira para o Estudo de Quirópteros (SBEQ). Versão 2020. Available online at: https://www.sbeq.net/lista-de-especies (accessed Apr 26, 2022).
Gelling, M., Macdonald, D. W., and Mathews, F. (2007). Are hedgerows the route to increased farmland small mammal density? Use of hedgerows in British pastoral habitats. Landsc. Ecol. 22, 1019–1032. doi: 10.1007/s10980-007-9088-4
Guido, A., Vélez-Martin, E., Overbeck, G. E., and Pillar, V. D. (2016). Landscape structure and climate affect plant invasion in subtropical grasslands. Appl. Veg. Sci. 19, 600–610. doi: 10.1111/avsc.12263
Hasenack, H., Weber, E., Boldrini, I. I., and Trevisan, R. (2010). Mapa de sistemas ecológicos da ecorregião das savanas uruguaias em escala 1:500.000 ou superior e relatório técnico descrevendo insumos utilizados e metodologia de elaboração do mapa de sistemas ecológicos. Porto Alegre: UFRGS.
Hesselbarth, M. H. K., Sciaini, M., With, K. A., Wiegand, K., and Nowosad, J. (2019). landscapemetrics: An open-source R tool to calculate landscape metrics. Ecography 42, 1648–1657. doi: 10.1111/ecog.04617
Hijmans, R. J. (2012). Introduction to the ‘raster’ Package. Technical Report, Raster Vignette. Available online at: http://cran.r-project.org/package=raster (accessed March 13, 2021).
Hintze, F., Arias-Aguilar, A., Dias-Silva, L., Delgado-Jaramillo, M., Silva, C. R., Jucá, T., et al. (2020). Molossid unlimited: Extraordinary extension of range and unusual vocalization patterns of the bat, Promops centralis. J. Mammal. 101, 417–432. doi: 10.1093/jmammal/gyz167
Jetz, W., Wilcove, D. S., and Dobson, A. P. (2007). Projected impacts of climate and land-use change on the global diversity of birds. PLoS Biol. 5:e157. pbio.0050157 doi: 10.1371/journal.pbio.0050157
Jones, G., and Rydell, J. (1994). Foraging strategy and predation risk as factors influencing emergence time in echolocating bats. Philos. Trans. R. Soc. London. Ser. B Biol. Sci. 346, 445–455. doi: 10.1098/rstb.1994.0161
Jones, G., Jacobs, D., Kunz, T., Willig, M., and Racey, P. (2009). Carpe noctem: The importance of bats as bioindicators. Endanger. Species Res. 8, 93–115. doi: 10.3354/esr00182
Jung, K., Molinari, J., and Kalko, E. K. V. (2014). Driving factors for the evolution of species-specific echolocation call design in new world free-tailed bats (Molossidae). PLoS One 9:e85279. doi: 10.1371/journal.pone.0085279
K. Lisa Yang Center for Conservation Bioacoustics (2022). Raven Pro: Interactive Sound Analysis Software (Version 1.6) [Computer software]. Ithaca, NY: The Cornell Lab of Ornithology.
Kaiser, Z. D. E., and O’Keefe, J. M. (2015). Factors affecting acoustic detection and site occupancy of Indiana bats near a known maternity colony. J. Mammal. 96, 344–360. doi: 10.1093/jmammal/gyv036
Kolkert, H., Andrew, R., Smith, R., Rader, R., and Reid, N. (2020). Insectivorous bats selectively source moths and eat mostly pest insects on dryland and irrigated cotton farms. Ecol. Evol. 10, 371–388. doi: 10.1002/ece3.5901
Konze, J. C. (2015). Assembleia De Aves Em Diferentes Coberturas Vegetais No Bioma Pampa, Rio Grande Do Sul, Brasil. Ph.D. thesis. Lajeado: Universidade do Vale do Taquari.
Korine, C., Adams, A. M., Shamir, U., and Gross, A. (2015). Effect of water quality on species richness and activity of desert-dwelling bats. Mamm. Biol. 80, 185–190. doi: 10.1016/j.mambio.2015.03.009
Kupfer, J. A., Malanson, G. P., and Franklin, S. B. (2006). Not seeing the ocean for the islands: The mediating influence of matrix-based processes on forest fragmentation effects. Glob. Ecol. Biogeogr. 15, 8–20. doi: 10.1111/j.1466-822X.2006.00204.x
Leidinger, J. L. G., Gossner, M. M., Weisser, W. W., Koch, C., Rosadio Cayllahua, Z. L., Podgaiski, L. R., et al. (2017). Historical and recent land use affects ecosystem functions in subtropical grasslands in Brazil. Ecosphere 8:e02032. doi: 10.1002/ecs2.2032
Lino, A., Fonseca, C., Rojas, D., Fischer, E., and Ramos Pereira, M. J. (2019). A meta-analysis of the effects of habitat loss and fragmentation on genetic diversity in mammals. Mamm. Biol. 94, 69–76. doi: 10.1016/j.mambio.2018.09.006
López-Baucells, A., Rocha, R., Bobrowiec, P., Bernard, E., Palmerim, J., and Meyer, C. (2016). Field Guide to Amazonian Bats. Brazil: National Institute of Amazonian Research, doi: 10.1093/jmammal/gyy071
Lumsden, L. F., and Bennett, A. F. (2005). Scattered trees in rural landscapes: Foraging habitat for insectivorous bats in south-eastern Australia. Biol. Conserv. 122, 205–222. doi: 10.1016/j.biocon.2004.07.006
MacKenzie, D. I., and Bailey, L. L. (2004). Assessing the fit of site-occupancy models. J. Agric. Biol. Environ. Stat. 9, 300–318. doi: 10.1198/108571104X3361
MacKenzie, D. I., Nichols, J. D., Lachman, G. B., Droege, S., Royle, J. A., and Langtimm, C. A. (2002). Estimating site occupancy rates when detection probabilities are less than one. Ecology 83, 2248–2255. doi: 10.1890/0012-9658(2002)083[2248:ESORWD]2.0.CO;2
MacKenzie, D. I., Nichols, J. D., Royle, J. A., Pollock, K. H., Bailey, L. L., and Hines, J. E. (2017). Occupancy Estimation and Modeling. Amsterdam: Elsevier, doi: 10.1016/C2012-0-01164-7
Maina, J. N. (2000). What it takes to fly: The structural and functional respiratory refinements in birds and bats. J. Exp. Biol. 203, 3045–3064. doi: 10.1242/jeb.203.20.3045
MapBiomas Pampa Sudamericano Project (2021). Collection [1] of the annual maps of land cover and land use. Available online at: https://pampa.mapbiomas.org/ (accessed Feb 21, 2021).
Martins, A. C. M. (2016). Morcegos em paisagens fragmentadas na Amazônia: uma abordagem em múltiplas escalas. 162 f., il. Ph.D. thesis. Brasilia: Universidade de Brasília.
Mazerolle, M. M. J. (2020). Package “AICcmodavg” - Model Selection and Multimodel Inference Based on (Q)AIC(c). R package version 2.2.3.
McGarigal, K., Cushman, S. A., and Ene, E. (2012). Spatial Pattern Analysis Program For Categorical And Continuous Maps. Amherst: University of Massachusetts.
Mendes, E. S., Fonseca, C., Marques, S. F., Maia, D., and Ramos Pereira, M. J. (2017). Bat richness and activity in heterogeneous landscapes: Guild-specific and scale-dependent? Landsc. Ecol. 32, 295–311. doi: 10.1007/s10980-016-0444-0
Mendes, P., and Srbek-Araujo, A. C. (2021). Effects of land-use changes on Brazilian bats: A review of current knowledge. Mamm. Rev. 51, 127–142. doi: 10.1111/mam.12227
Meyer, C. F. J., and Kalko, E. K. V. (2008). Assemblage-level responses of phyllostomid bats to tropical forest fragmentation: Land-bridge islands as a model system. J. Biogeogr. 35, 1711–1726. doi: 10.1111/j.1365-2699.2008.01916.x
Monck-Whipp, L., Martin, A. E., Francis, C. M., and Fahrig, L. (2018). Farmland heterogeneity benefits bats in agricultural landscapes. Agric. Ecosyst. Environ. 253, 131–139. doi: 10.1016/j.agee.2017.11.001
Mutumi, G. L., Jacobs, D. S., and Winker, H. (2016). Sensory drive mediated by climatic gradients partially explains divergence in acoustic signals in two horseshoe bat species, Rhinolophus swinnyi and Rhinolophus simulator. PLoS One 11:e0148053. doi: 10.1371/journal.pone.0148053
Myers, N., Mittermeier, R. A., Mittermeier, C. G., da Fonseca, G. A. B., and Kent, J. (2000). Biodiversity hotspots for conservation priorities. Nature 403, 853–858. doi: 10.1038/35002501
Naimi, B. (2017). Package ‘usdm’- Uncertainty Analysis for Species Distribution Models. 18. Available online at: https://cran.r-project.org/web/packages/usdm/usdm.pdf (accessed March 13, 2021).
Noronha, B. T. (2016). Análise dos registros de Chiroptera no Rio Grande do Sul - métodos empregados e distribuição de espécies por municípios, com ênfase em áreas de parques eólicos. Ph.D. thesis. Porto Alegre: Universidade Federal do Rio Grande do Sul.
Oliveira, T. F., Ramalho, D. F., Mora, E. C., and Aguiar, L. M. S. (2018). The acoustic gymnastics of the dwarf dog-faced bat (Molossops temminckii) in environments with different degrees of clutter. J. Mammal. 99, 965–973. doi: 10.1093/jmammal/gyy070
Pacheco, S. M., Sodré, M., Gama, A. R., Bredt, A., Cavallini, E. M., Marques, S., et al. (2010). Morcegos urbanos: Status do conhecimento e plano de ação para a conservação no brasil. Chiropt. Neotrop. 16, 629–647.
Paradis, E., Blomberg, S., Bolker, B., Brown, J., Claramunt, S., Claude, J., et al. (2019). Package “ape” - Analyses of Phylogenetics and Evolution. R package version.
Pebesma, E. J., and Bivand, R. S. (2005). Classes and methods for spatial data in R. R News 5, 9–13.
Phalan, B., Bertzky, M., Butchart, S. H. M., Donald, P. F., Scharlemann, J. P. W., Stattersfield, A. J., et al. (2013). Crop expansion and conservation priorities in tropical countries. PLoS One 8:e51759. doi: 10.1371/journal.pone.0051759
Pillar, V. D. P., and Lange, O. (2015). Os Campos do Sul. 1st ed. Rede Campos Sulinos. Porto Alegre: UFRGS,
Pillar, V. D. P., Müller, S. C., de Castilhos, Z. M. S., and Jacques, A. V. Á (2009). Campos Sulinos - Conservação e uso Sustentável da Biodiversidade. Brasilia: Ministério do Meio Ambiente.
Queirolo, D. (2016). Diversidade e padrões de distribuição de mamíferos dos campos do Uruguai e sul do Brasil. Bol. Soc. Zoo. Uruguay 25, 92–246.
R Core Team (2021). R: A Language And Environment For Statisticalcomputing. Vienna: R Foundation for Statistical Computing.
Racey, P. A., and Swift, S. M. (1985). Feeding ecology of Pipistrellus pipistrellus (Chiroptera: Vespertilionidae) during pregnancy and lactation. I. foraging behaviour. J. Anim. Ecol. 54:205. doi: 10.2307/4631
Ramos Pereira, M. J., Marques, J. T., Santana, J., Santos, C. D., Valsecchi, J., de Queiroz, H. L., et al. (2009). Structuring of Amazonian bat assemblages: The roles of flooding patterns and floodwater nutrient load. J. Anim. Ecol. 78, 1163–1171. doi: 10.1111/j.1365-2656.2009.01591.x
Ramos Pereira, M. J., Stefanski Chaves, T., Bobrowiec, P. E., and Selbach Hofmann, G. (2022). How aerial insectivore bats of different sizes respond to nightly temperature shifts. Int. J. Biometeorol. 66, 601–612. doi: 10.1007/s00484-021-02222-w
Reis, N. R., Peracchi, A. L., Batista, C. B., Lima, I. P. (2017). História natural dos morcegos brasileiros: Chave de identifcação de espécies. Rio de Janeiro: Technical Books Livraria Ltda.
Ricketts, T. H. (2001). The matrix matters: Effective isolation in fragmented landscapes. Am. Nat. 158, 87–99. doi: 10.1086/320863
Rodríguez-San Pedro, A., Chaperon, P. N., Beltrán, C. A., Allendes, J. L., Ávila, F. I., and Grez, A. A. (2018). Influence of agricultural management on bat activity and species richness in vineyards of central Chile. J. Mammal. 99, 1495–1502. doi: 10.1093/jmammal/gyy121
Roesch, L. F., Vieira, F., Pereira, V., Schünemann, A. L., Teixeira, I., Senna, A. J., et al. (2009). The Brazilian Pampa: A fragile biome. Diversity 1, 182–198. doi: 10.3390/d1020182
Russ, J. M., Briffa, M., and Montgomery, W. I. (2003). Seasonal patterns in activity and habitat use by bats (Pipistrellus spp. and Nyctalus leisleri) in Northern Ireland, determined using a driven transect. J. Zool. 259, 289–299. doi: 10.1017/S0952836902003254
Russo, D., and Ancillotto, L. (2015). Sensitivity of bats to urbanization: A review. Mamm. Biol. 80, 205–212. doi: 10.1016/j.mambio.2014.10.003
Schaub, A., and Schnitzler, H. U. (2007). Echolocation behavior of the bat Vespertilio murinus reveals the border between the habitat types “edge” and “open space.”. Behav. Ecol. Sociobiol. 61, 513–523. doi: 10.1007/s00265-006-0279-9
Schnitzler, H.-U., Moss, C. F., and Denzinger, A. (2003). From spatial orientation to food acquisition in echolocating bats. Trends Ecol. Evol. 18, 386–394. doi: 10.1016/S0169-5347(03)00185-X
Schowalter, T. D. (2006). Insect Ecology: An Ecosystem Approach, 2nd Edn. San Diego, CA: Academic Press.
Smith, T. N., Furnas, B. J., Nelson, M. D., Barton, D. C., and Clucas, B. (2021). Insectivorous bat occupancy is mediated by drought and agricultural land use in a highly modified ecoregion. Divers. Distrib. 27, 1152–1165. doi: 10.1111/ddi.13264
Snell-Rood, E. C. (2012). The effect of climate on acoustic signals: Does atmospheric sound absorption matter for bird song and bat echolocation? J. Acoust. Soc. Am. 131, 1650–1658. doi: 10.1121/1.3672695
Souza, C. M., Shimbo, J. Z., Rosa, M. R., Parente, L. L., Alencar, A., Rudorff, B. F. T., et al. (2020). Reconstructing three decades of land use and land cover changes in brazilian biomes with landsat archive and earth engine. Remote Sens. 12:2735. doi: 10.3390/rs12172735
Stein, A., Gerstner, K., and Kreft, H. (2014). Environmental heterogeneity as a universal driver of species richness across taxa, biomes and spatial scales. Ecol. Lett. 17, 866–880. doi: 10.1111/ele.12277
Taubert, F., Fischer, R., Groeneveld, J., Lehmann, S., Müller, M. S., Rödig, E., et al. (2018). Global patterns of tropical forest fragmentation. Nature 554, 519–522. doi: 10.1038/nature25508
Taylor, P. D., Fahrig, L., and With, K. A. (2010). “Landscape connectivity: a return to the basics,” in Connectivity Conservation, eds K. R. Crooks and M. Sanjayan (Cambridge, MA: Cambridge University Press), 29–43. doi: 10.1017/CBO9780511754821.003
Tews, J., Brose, U., Grimm, V., Tielbörger, K., Wichmann, M. C., Schwager, M., et al. (2004). Animal species diversity driven by habitat heterogeneity/diversity: The importance of keystone structures. J. Biogeogr. 31, 79–92. doi: 10.1046/j.0305-0270.2003.00994.x
Thomas, S. P., and Suthers, R. A. (1972). The physiology and energetics of bat flight. J. Exp. Biol. 57, 317–335. doi: 10.1242/jeb.57.2.317
Tirelli, F. P., Trigo, T. C., Trinca, C. S., Albano, A. P. N., Mazim, F. D., Queirolo, D., et al. (2018). Spatial organization and social dynamics of Geoffroy’s cat in the Brazilian pampas. J. Mammal. 99, 859–873. doi: 10.1093/jmammal/gyy064
Toffoli, R. (2016). The importance of linear landscape elements for bats in a farmland area: The influence of height on activity. J. Landsc. Ecol. 9, 49–62. doi: 10.1515/jlecol-2016-0004
Verboom, B., and Huitema, H. (1997). The importance of linear landscape elements for the pipistrelle Pipistrellus pipistrellus and the serotine bat Eptesicus serotinus. Landsc. Ecol. 12, 117–125. doi: 10.1007/BF02698211
Watling, J. I., Nowakowski, A. J., Donnelly, M. A., and Orrock, J. L. (2011). Meta-analysis reveals the importance of matrix composition for animals in fragmented habitat. Glob. Ecol. Biogeogr. 20, 209–217. doi: 10.1111/j.1466-8238.2010.00586.x
Keywords: acoustic monitoring, Chiroptera, landscape structure, microclimate, site occupancy
Citation: da Costa CF and Ramos Pereira MJ (2022) Aerial insectivorous bats in the Brazilian-Uruguayan savanna: Modelling the occupancy through acoustic detection. Front. Ecol. Evol. 10:937139. doi: 10.3389/fevo.2022.937139
Received: 05 May 2022; Accepted: 12 September 2022;
Published: 29 September 2022.
Edited by:
Ludmilla M. S. Aguiar, University of Brasilia, BrazilReviewed by:
Jose Mora, Portland State University, United StatesDaniel Ramalho, University of Brasilia, Brazil
Copyright © 2022 da Costa and Ramos Pereira. This is an open-access article distributed under the terms of the Creative Commons Attribution License (CC BY). The use, distribution or reproduction in other forums is permitted, provided the original author(s) and the copyright owner(s) are credited and that the original publication in this journal is cited, in accordance with accepted academic practice. No use, distribution or reproduction is permitted which does not comply with these terms.
*Correspondence: Cíntia Fernanda da Costa, cintia_fc04@gmail.com