- 1State Key Laboratory of Freshwater Ecology and Biotechnology, Institute of Hydrobiology, Chinese Academy of Sciences, Wuhan, China
- 2University of Chinese Academy of Sciences, Beijing, China
- 3Hainan Academy of Ocean and Fisheries Sciences, Haikou, China
- 4Colorado Parks and Wildlife, Aquatic Research Section, Fort Collins, CO, United States
With the intensification of eutrophication, many artificial water supply lakes that act as a biological filter for water diverted from rivers have been built to alleviate water scarcity in Eastern China. In this study, we selected Lake Yanlong, a representative artificial water supply lake in Yancheng City, as our experimental lake to explore how the community composition of fishes changed among different habitat types and assess potential consequences for effective water treatment. From October 2015 to October 2020, we conducted quarterly surveys of fish communities and environmental factors in the Mangshe River (MR; water for lake) compared to four different water treatment zones of Lake Yanlong (PZ, the pretreatment zone with inlet from the MR; EZ, the emergent macrophyte zone; SZ, the submerged macrophyte zone; DZ, the deep purification zone with outlet to urban waterworks). A total of 16,339 individual fish belonging to 11 families and 49 species were collected. Six of the eight dominant species observed across zones were small-bodied fishes. Despite reduced fish species richness, the relative abundance and biomass of fishes tended to be higher in Lake Yanlong relative to the MR. The Shannon-Wiener diversity index, Pielou evenness index, Simpson’s diversity index all decreased from the MR to the DZ in the following sequence: MR < PZ < EZ < SZ < DZ. Analysis of similarities and similarity percentage analysis confirmed that fish communities differed significantly among zones and Coilia ectenes, Carassius auratus, Pseudobrama simony, Hemiculter leucisculus, and Hemiculter bleekeri were the major differentiating species. Mantel’s test and redundancy analysis revealed that water depth, aquatic vegetation coverage, and phytoplankton concentrations were the major factors determining the spatial distribution of fishes when moving from the MR to the DZ of Lake Yanlong. Stocking piscivorous fish can be used as an effective measure to control the abundance of prolific small-bodied fishes in Lake Yanlong. The details backing these findings are important for understanding how the community composition of fishes among habitat types in Lake Yanlong influence water quality, and to develop suitable biomanipulation strategies for the management of fish resources and maintaining proper function of these artificial water supply lakes.
Introduction
Eutrophication, caused by both natural and anthropogenic disturbance, is a pervasive problem facing surface water bodies (Smith and Schindler, 2009; Bhagowati and Ahamad, 2018) and has been an important topic for academic and social debate over the past few decades (Bonsdorff, 2021). The middle and lower reaches of the Yangtze River Basin (MLYRB) contains the majority of shallow lakes in China, with 651 lakes larger than 1 km2 and 18 lakes larger than 100 km2, accounting for about 1/3 of the total surface area of lakes in China (Zhang et al., 2019; Tang, 2020). With rapid socioeconomic development and the acceleration of urbanization since the 1980s, industrial and agricultural pollution as well as discharge of domestic sewage has resulted in the eutrophication of most lakes in the MLYRB (Zhu et al., 2019; Tang, 2020; Zou et al., 2020). Increasing levels of eutrophication pose a great threat to drinking water quality and human health (Qin et al., 2010; Moal et al., 2019), which has become an important ecological and socioeconomic problem in the MLYRB, particularly in the Eastern Plain of China (EPC) (Zou et al., 2020).
To alleviate this risk, the central and local governments of China have launched a series of water pollution control projects over the last decade (Guo et al., 2019), including the construction of artificial water supply lakes that act as a biological filter for water diverted from rivers within the EPC (Cai et al., 2019). Compared to natural lakes in the region, these artificial water supply lakes are often characterized by short construction time, small area, simple and unstable ecosystem structure, and are prone to degradation by human activities (e.g., improper fisheries management) (Cai et al., 2019; Li S. et al., 2021). Moreover, water quality and other ecosystem components (e.g., fish communities and extent of submerged aquatic vegetation) within these artificial lakes are affected by shifts in the environmental conditions of the rivers that feed them (Cai et al., 2019). There has been extensive research aimed toward developing restoration strategies for maintaining ecosystem function in natural lakes within the MLYRB [e.g., Taihu Lake (Wang et al., 2019) and Dongting Lake (Li B. et al., 2021)]. However, maintaining proper ecosystem function and identifying effective restoration strategies for artificial water supply lakes is much less common, despite their importance for protecting human health in the EPC. Therefore, it is necessary to understand the physical and biological characteristics of these artificial lakes, how different components work together to maintain core ecosystem functions and delivery of goods and services (e.g., purification of drinking water), and to establish effective restoration strategies that are suitable for these lakes given their unique properties when compared to natural lakes.
As the largest group of vertebrates and the primary mid- to upper-trophic level consumers in freshwater ecosystems, fish play important roles in the functioning of lakes by affecting the physical environment, trophic interactions, and community dynamics (Mello et al., 2009; Jeppesen et al., 2010). Under some circumstances, by consuming small zooplanktivorous fish, piscivorous fish can reduce grazing pressure on zooplankton communities, which in turn can increase grazing pressure on phytoplankton to an extent that improves water clarity—a more desirable state for many lakes that can also help support submerged macrophyte production (Mehner et al., 2004). Filter-feeding fish can also control undesirable cyanobacterial blooms by grazing phytoplankton directly (Xie and Liu, 2001). Alternatively, an unbalanced fish community can have a negative impact on water quality and lake ecosystem function (Cai, 2017; Guo et al., 2022). For example, the overabundance of herbivorous fish can precipitate submerged macrophyte loss in shallow lakes through over-grazing (Zhen et al., 2018). Often affected by overfishing and habitat loss, piscivorous fish in most MLYRB lakes and rivers have dramatically declined over the past decades, which has led to the proliferation of small-bodied fishes (Mao et al., 2011; Cai et al., 2019). Most of these small-bodied fish in the MLYRB feed on zooplankton (especially cladocerans and copepods), leading to increased phytoplankton biomass and reduced water clarity (Zhang, 2005). Furthermore, some studies demonstrated that declines in fish species diversity and the proportion of piscivorous fish present in aquatic ecosystems were accompanied by the deterioration of water quality (Karr, 1981, 1991; Karr et al., 1986). Given the strong roles fish play in aquatic ecosystem function, fish community structure has been regarded as an important ecological indicator and suitable for assessing the status of aquatic ecosystems (Oberdorff et al., 2001).
The physical environment can also have reciprocal effects on the composition and spatial distribution of fish communities through a variety of mechanisms (Geheber and Piller, 2012; Cote et al., 2013). Previous studies demonstrated that water velocity, macrophytes, water depth and trophic state were the primary factors mediating fish community dynamics (Brinsmead and Fox, 2002; Mehner et al., 2005; Li et al., 2010). For example, river fishes are generally more slender-bodied, and have smaller fins than fishes occupying lakes to reduce lotic drag (Brinsmead and Fox, 2002). In a survey of Xiaosihai Lake, China, areas with greater submerged macrophyte coverage supported a higher biomass and abundance of small fish (Li et al., 2010). Moreover, the coverage of submerged macrophytes is strongly correlated to water depth and trophic state, whereby deep or eutrophic lakes are often devoid of submerged macrophytes needed to support certain species of fish (Jeppesen et al., 1990). Thus, the composition and spatial distribution of fish communities are shaped by both environmental factors and biotic interactions, which could have consequences for ecosystem function (Mehner et al., 2005; Mehner and Brucet, 2021). Understanding these complexities may help inform proper restoration strategies in degraded lakes. However, the interdependencies among fish and the physical environment within artificial water supply lakes of the MLYRB are poorly studied.
The design and construction of artificial water supply lakes are tailored toward water treatment for human consumption. As a result, water quality and the physical habitat available for fish can vary from the inlet (where water is pumped from adjacent rivers) to the outlet as water travels through different purification zones of the lakes. Therefore, the community composition of fishes could vary along this gradient. Lake Yanlong (33°20′N, 120°1′E), built in 2012 and located at the intersection of the lower Yangtze River Basin and the lower Huaihe River Basin, is the first artificial water supply lake in the ECP and represented an ideal system for studying fish distribution and community composition (Zuo et al., 2015). The lake collects raw water from its waterhead river—the Mangshe River (MR)—through a pump station, and finally supplies water to the urban waterworks once traveling through four distinct purification zones: the pretreatment zone (PZ), the emergent macrophyte zone (EZ), the submerged macrophyte zone (SZ), and finally the deep purification zone (DZ). In this study, we conducted a quarterly survey of the fish community and environmental factors within each distinct purification zone of Lake Yanlong (PZ, EZ, SZ, DZ), as well as the adjacent Mangshe River (MR) from October 2015 to October 2020. We aimed to explore: (1) how fish species composition and diversity differed between Lake Yanlong and the Mangshe River; (2) the key environmental factors that could differentiate the fish community of the Mangshe River from Lake Yanlong as well as the distribution of fishes within the lake; and (3) suitable biomanipulation strategies for the management of fish resources and water quality in these artificial water supply lakes.
Materials and Methods
Study Area
The Mangshe River is 43 km long and drains a basin area of 64,000 ha. It flows through the northern Lixiahe Plain of Jiangsu Province and used to be the primary drinking water source for residents of Yancheng City. The water quality of MR fluctuates throughout the year, and is typically classified between levels III to IV according to the environmental quality standards for surface water in China (GB3838-2002, Ministry of Environmental Protection of China, 2002). Additionally, the MR usually shows characteristics of organic pollution during summer (Zuo et al., 2015). In order to ensure the safety of drinking water for residents of Yancheng city, Lake Yanlong, an artificial water supply lake collecting raw water from the MR, was built in June 2012. After replacing the MR as the main source of drinking water in Yancheng City with Lake Yanlong, drinking water quality has improved and has become more stable (Zuo et al., 2015).
Lake Yanlong (210.9 ha) is a complex ecosystem exhibiting both wetland- and reservoir-type features and includes four distinct purification zones (Figure 1). Water enters the PZ first, which pretreats water from the MR mainly by means of precipitation, oxygenation and exposure to an established biofilm, and is characterized by relatively deep depths (mean ± SE; 3.25 ± 0.30 m) and relatively short water residence times as water moves through at measurable velocities (0.07 ± 0.04 m/s). Water enters the EZ second, which purifies water through assimilation, absorption and transformation of pollutants by emergent macrophytes (mainly Phragmites australis and Typha orientalis), and is characterized by relatively shallow depths (0.78 ± 0.19 m) and a high spatial coverage of emergent plants (86.3% ± 46.3%). Water enters the SZ third, which further purifies water through absorption and transformation of pollutants by submerged macrophytes (mainly Myriophyllum verticillatum, Ceratophyllum demersum, and Vallisneria natans). The DZ is the last purification zone before water exits the lake via a pumping station, which like the PZ, purifies water mainly through the settling of particulates, but is deeper (4.75 ± 0.22 m) and encompasses the largest surface area and storage capacity (4.2 × 106 m3) of all zones. These four distinct purification zones are separated by sluices, which act as obstacles to fish movement among zones. The main characteristics of the five different zones (MR, PZ, EZ, SZ, and DZ) are shown in Table 1 (Ren, 2021; Guo et al., 2022).
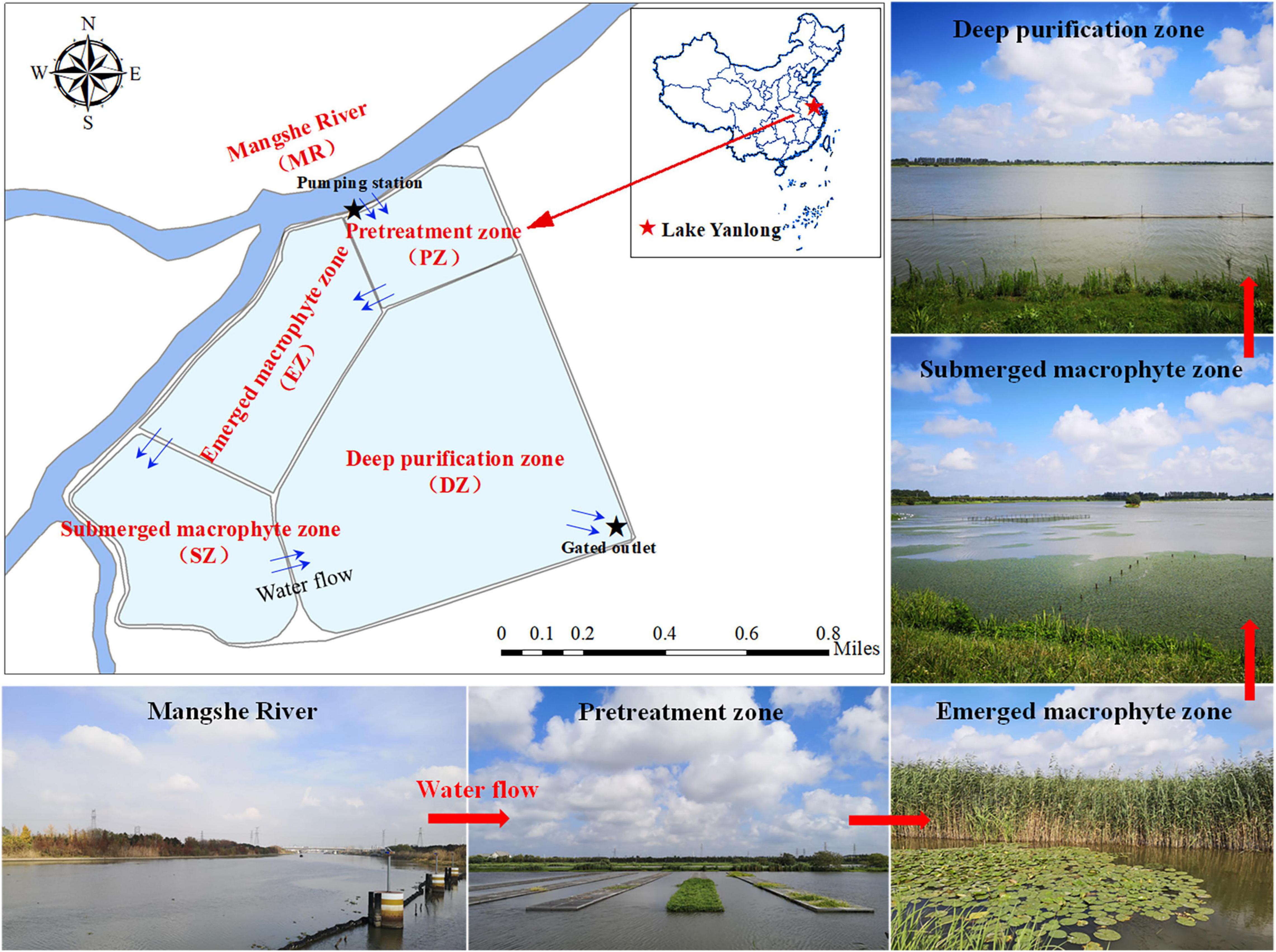
Figure 1. Pictures and map of the Mangshe River (MR) and Lake Yanlong, showing the distribution of different purification zones (PZ, the pretreatment zone; EZ, the emergent macrophyte zone; SZ, the submerged macrophyte zone; DZ, the deep purification zone).
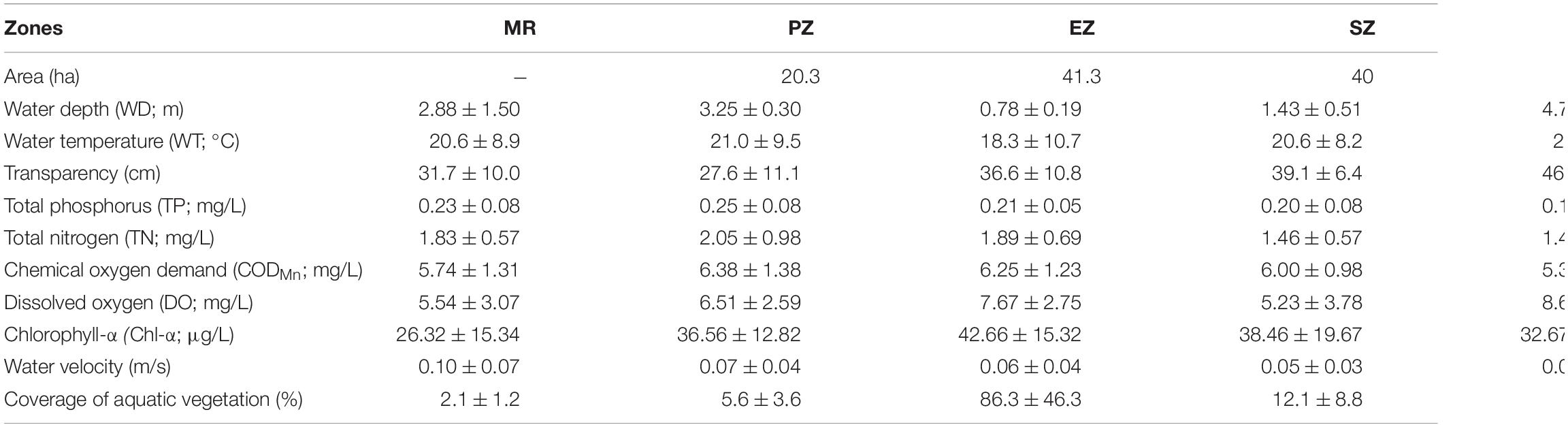
Table 1. Annual means (±SE) of physicochemical parameters measured in the Mangshe River (MR) and different purification zones of Lake Yanlong (PZ, the pretreatment zone; EZ, the emergent macrophyte zone; SZ, the submerged macrophyte zone; DZ, the deep purification zone).
To help support a clear-water state in Lake Yanlong, which can bolster macrophyte production, biomanipulation was used. Different types of native piscivorous fish were stocked in each purification zone of Lake Yanlong from 2017 through 2020 to consume small-bodied fishes. By the end of June 2020, a total of 3.2 × 104 individual topmouth culter Culter alburnus, 3.3 × 104 individual mandarin fish Siniperca chuatsi, 2.0 × 104 individual catfish Clarias gariepinus and 1.87 × 104 individual snakehead fish Channa argus were stocked into the lake. Species and numbers stocked varied among zones to account for differences in surface area and habitat characteristics (Table 2).
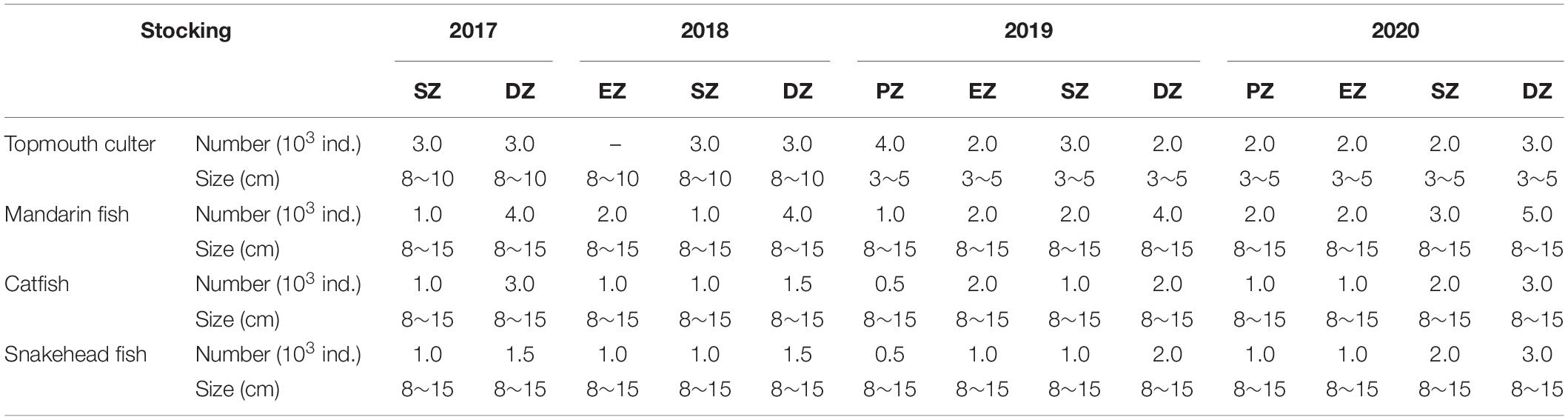
Table 2. The total number and individual size of piscivorous fish species stocked annually into the four purification zones of Lake Yanlong (PZ, the pretreatment zone; EZ, the emergent macrophyte zone; SZ, the submerged macrophyte zone; DZ, the deep purification zone) from 2017 to 2020.
Fish Sampling
A total of nine standard fish sampling sites (one site both for MR and PZ, two sites both for EZ and SZ, and three sites for DZ) were established across the MR and all purification zones of Lake Yanlong. The number of sites within each zone reflected the relative size of each region. Fishes were sampled quarterly from October 2015 to October 2020 using multi-mesh monofilament gill nets. The design of the gill nets was modified according to the standard method (Appelberg, 2000). Each net consisted of twelve 2.5 m long × 1.5 m tall mesh panels placed in random order (8.5, 4.0, 12.5, 2.0, 11.0, 1.6, 2.5, 4.8, 3.1, 1.0, 7.5, and 6.0 cm stretch measure). Three nets (two pelagic surface nets and one benthic net) were set in series at each sampling site overnight (12–15 h) for three consecutive nights each season (January, April, July, October). Fishes were separated and identified to the species level, and individually measured for total length to the nearest 1 mm and body weight to the nearest 0.01 g. We calculated the species richness, mean quarterly catch per unit effort in both numerical (CPUEN; ind./m2/12 h) and biomass (CPUEB; g/m2/12 h) terms. Small-bodied fishes were defined by having an age-at-first-maturity of less than 2 years (Cai et al., 2019). Each fish species was classified for feeding type (omnivorous, piscivorous, planktivorous, or herbivorous) and propensity for movement (migratory and resident) according to Ni and Wu (2006).
Environmental Variables
On each sampling occasion, eleven environmental variables were measured in conjunction with fish sampling at each sampling site (Table 1). Dissolved oxygen (DO; mg/L) and surface water temperature (WT;°C) were monitored using a YSI ProPlus meter (Thermo Fisher Scientific Company, Waltham, United States). Water depth (WD; m) was measured using a Speedtech SM-5 Portable Depth Sounder. Turbidity (NTU) was measured using a Hach 2100P portable turbidity meter. Chemical oxygen demand (CODMn; mg/L), total nitrogen (TN; mg/L) and total phosphorus (TP; mg/L) were determined according to the standard methods described in American Public Health Association [APHA] (1992). Chlorophyll-α concentration (Chl-α; μg/L) was determined using a fluorimeter with methanol extraction of the filtrate (Holm-Hansen and Riemann, 1978). The biomass of aquatic vegetation (BAV; g/m2) was recorded using a grass collection sampler (Guo et al., 2022). Family-specific zooplankton (NZ; ind./L) and phytoplankton density (NP; ind./L) were estimated following the standard methods described in Dumont (2002) and Paredes and Montecino (2011), respectively.
Species Diversity Indices
The following indices were calculated for each sampling occasion and used to quantify fish species diversity and rank the relative importance of each species among the MR and different purification zones of Lake Yanlong:
(i) Shannon–Wiener diversity index (HN) (Shannon and Weaver, 1949)
(ii) Pielou evenness index (J) (Pielou, 1975)
(iii) Simpson diversity index (D) (Whittaker, 1972)
(iv) Simpson dominance index (λ) (Simpson, 1949)
(v) The index of relative importance (IRI) (Pianka, 1971)
where Pi is the proportional abundance of species i referenced to the total number of fish individuals sampled; S is the total number of species; Ni is the numerical percentage of species i; Wi is the biomass percentage of species i; and Fi is the percent frequency of occurrence of species i. When IRIi is larger than 1000, this species is defined as the dominant species (Yang et al., 2018).
Data Analysis
Differences in Fish Community Composition and Diversity
Potential differences in mean diversity indices (HN, J, D, λ) and the relative abundance and biomass (CPUEN and CPUEB) of different fishes observed from October 2015 to October 2020 among the four purification zones of Lake Yanlong and of the MR were tested using multiple non-parametric, one-way ANOVA’s (Kruskal–Wallis tests) implemented in the R package “coin” (Hothorn et al., 2008). The different zones (averaging across sampling quarters) represented the fixed factor. Where applicable, Wilcoxon tests were used for post hoc comparisons and to determine which zones differed using the “rstatix” package (Kassambara, 2021). Moreover, we used one-way analysis of similarity (ANOSIM) to identify the significance of observed differences in fish community composition and then used similarity percentage analysis (SIMPER) to explore the discriminating species of fish within communities among zones using the “vegan” package (Oksanen et al., 2022).
Environmental Effects on Fish Community Composition
Spearman correlation analyses and Mantel’s tests were used to explore the relationship between different environmental variables and the dominant fish species in each purification zone and the MR by using quarterly measurements, also implemented in R using package “linkET” (Huang, 2022). Redundancy analysis (RDA; provided within the “vegan” package) was then used to identify the key environmental factors that could explain differences in the abundance of dominant species among zones. Prior to these analyses, variance inflation factors (VIFs) were adopted to examine multicollinearity among environmental factors. Collinear environmental variables detected by forward-backward selection were excluded until all remaining variables had VIFs less than 5 (Gou et al., 2021). In addition, hierarchical partitioning (HP) and permutation analyses were performed to acquire the independent explanation power and variance of each environmental factor using the “rdacca.hp” package (Lai et al., 2022). Environmental variables were standardized to a mean of zero and unit variance by the “decostand” function in the “vegan” package. Lastly, CPUEN of dominant species were log10(x + 1) transformed prior to analysis (James and McCulloch, 1990).
Effect of Stocking Piscivorous Fish on Small-Bodied Fishes and Species Diversity
To assess the effect of stocking of piscivorous fishes on small-bodied fishes and fish community diversity, we compared mean relative abundance and biomass values (CPUEN and CPUEB) of small-bodied fishes and fish diversity indices (HN, J, D) among the four purification zones of Lake Yanlong before (data from October of 2015 and 2016) and after (data from October of 2017, 2018, 2019, and 2020) stocking of piscivorous fishes occurred. For this analysis, we used Mann-Whitney tests implemented in the R package “stats” (R Core Team, 2021).
All statistical analyses and figures were made using R software (Version 4.1.2). Differences were considered significant at an α level of 0.05 (i.e., p-value < 0.05) for all tests.
Results
Species Composition and Richness
In total, 16,339 individual fish belonging to 11 families and 49 species were collected from all purification zones of Lake Yanlong and the MR between October 2015 and October 2020 (Supplementary Table 1). Fish species richness was highest in the MR (39 species), followed by the SZ (31 species), the EZ (30 species), and richness was lowest in the DZ (22 species). The 49 fish species were divided into resident fish (39 species) and migratory fish (10 species) based on their propensity for movement. The species richness of migratory fish (e.g., Elopichthys bambusa and Squaliobarbus curriculus) in the MR was the highest (10 species), but decreased greatly after entering Lake Yanlong (five species in PZ; four species in EZ; six species in SZ; four species in DZ). Compared to migratory fish, resident fish (e.g., Coilia ectenes and Pseudobrama simony) were more prolific in the four purification zones of Lake Yanlong, especially in the EZ (26 species) and SZ (25 species), which reflected the transition from riverine to wetland- and reservoir-type habitats (Figure 2). Based on typical food items ingested, these 49 fish species were also classified into four trophic guilds: omnivores (23 species), piscivores (14 species), planktivores (7 species) and herbivores (5 species). For omnivorous fish, species richness differed between MR and the other four zones, being highest in MR (22 species), and lowest in DZ (8 species); For piscivorous fish, species richness was highest in EZ (12 species), followed by MR (7 species), and only 6 species each for PZ, SZ and DZ; For planktivorous fish, species richness was highest in SZ (6 species), followed by MR (5 species) and DZ (5 species), and only 1 species each for EZ; For herbivorous fish, species richness was highest in EZ, SZ and MR (each with 5 species), followed by PZ (4 species), and DZ (3 species) (Figure 2).
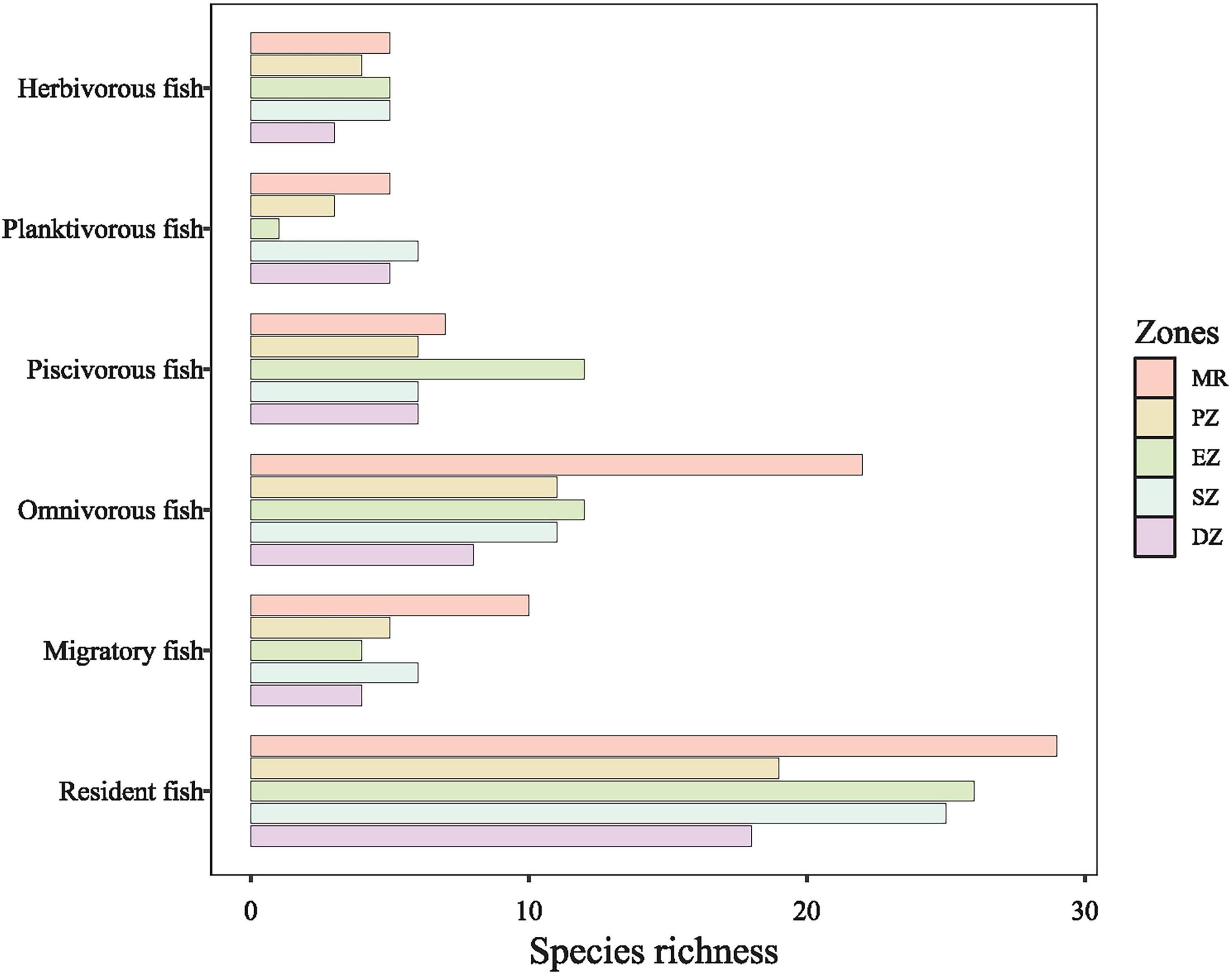
Figure 2. The total number of fish species sampled (i.e., species richness) parsed into different different feeding types (omnivores, piscivores, planktivores, and herbivores) and propensity for movement (migratory or resident) among the Mangshe River (MR) and different purification zones of Lake Yanlong (PZ, the pretreatment zone; EZ, the emergent macrophyte zone; SZ, the submerged macrophyte zone; DZ, the deep purification zone).
Distribution of Dominant Species
A total of eight dominant species (IRI > 1,000) were identified among the MR and four purification zones. Six of the eight dominant species (P. simony, Carassius auratus, Hemiculter leucisculus, C. ectenes, Hemiculter bleekeri and Toxabramis swinhonis) were small-bodied fishes that feed primarily on zooplankton, algae and plants debris. In addition, the total number of dominant species identified varied among zones, being highest in the PZ (five dominant species), followed by EZ (four dominant species), DZ (three dominant species), SZ (two dominant species), and was lowest in the MR (one dominant species). In the MR, only H. bleekeri met conditions for dominance (IRI = 1323.0), and represented 10.0% of the total numerical catch across study years. In the PZ, P. simony (IRI = 3168.3) and C. ectenes (IRI = 2217.5) were the most dominant species, accounting for 16.5% and 12.5% of the total catch biomass across years, respectively. C. auratus (IRI = 5243.3), P. simony (IRI = 6232.0) and C. ectenes (IRI = 5092.7) were the most dominant species in the EZ, SZ and DZ, respectively. Lastly, H. leucisculus was relatively common in the PZ, EZ, SZ and DZ, while T. swinhonis and Cyprinus carpio only met conditions for dominance in the PZ and EZ, respectively (Table 3).
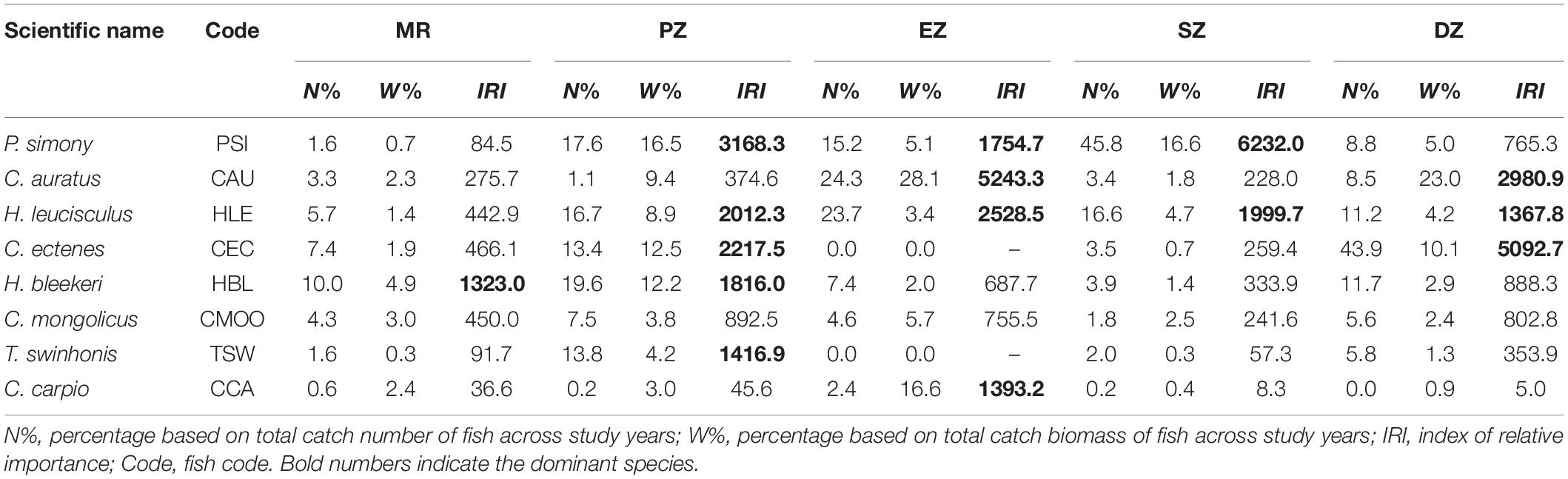
Table 3. The percent relative abundance (N%) and biomass (W%) and the index of relative importance (IRI) of dominant fish species sampling from the Mangshe River (MR) and from different purification zones of Lake Yanlong (PZ, the pretreatment zone; EZ, the emergent macrophyte zone; SZ, the submerged macrophyte zone; DZ, the deep purification zone).
Diversity Indices and Relative Abundance and Biomass
There were significant differences in the diversity indices (HN, J, D, λ) and relative abundance and biomass (CPUEN and CPUEB) among the MR and four purification zones when averaging across study quarters (Kruskal–Wallis test, p < 0.05 for all cases) (Table 4). In terms of the diversity indices, HN, J and D of the fish communities all showed a decreasing trend from the MR to DZ and followed the progression of: MR < PZ < EZ < SZ < DZ. Conversely, λ showed the opposite pattern (Figure 3). In terms of relative abundance and biomass, CPUEN and CPUEB both increased from the MR to Lake Yanlong. These values were lowest in the MR (9.6 ± 1.1 ind./m2/12 h and 387.1 ± 60.5 g/m2/12 h, respectively), and highest in the EZ (35.4 ± 5.1 ind./m2/12 h and 1895.7 ± 318.2 g/m2/12 h, respectively) (Figure 4 and Supplementary Table 2).
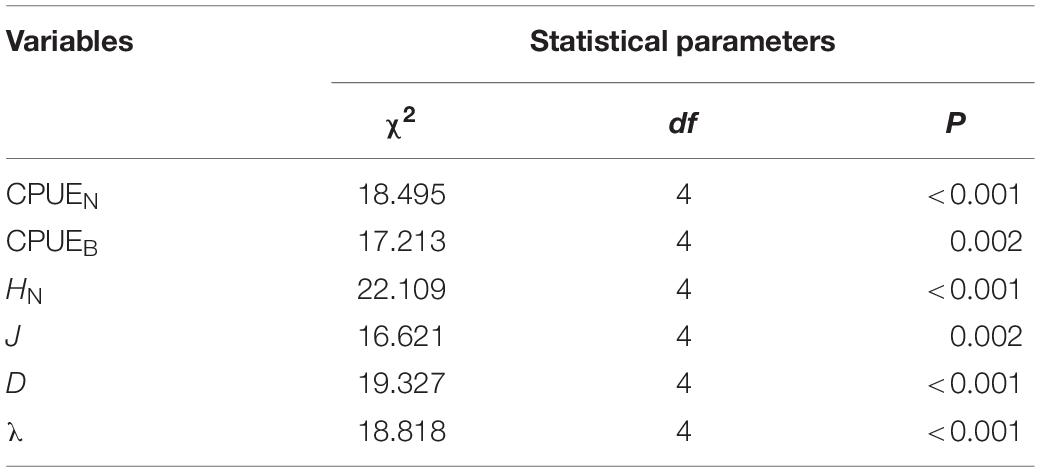
Table 4. Detailed information on results from the non-parametric, one-way ANOVA (i.e., Kruskal–Wallis test) models evaluating potential differences in the mean catch per unit effort of fishes [in both numerical (CPUEN) and biomass (CPUEB) terms] and the fish community diversity metrics (HN, J, D, λ) among habitat zones.
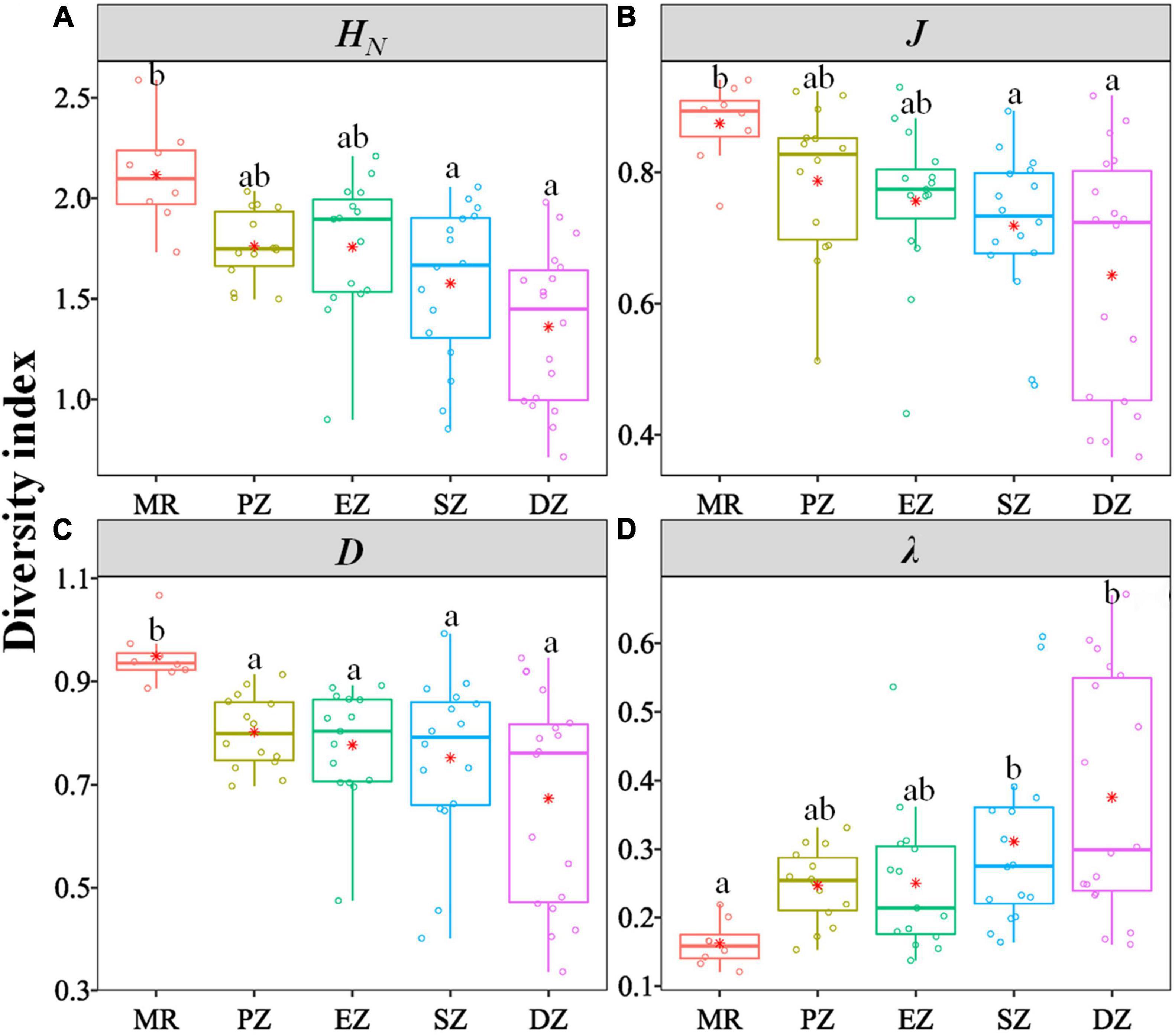
Figure 3. Box and whisker plots characterizing the distribution of different indices [(A) Shannon–Wiener diversity index HN, (B) Pielou evenness index J, (C) Simpson diversity index D, (D) Simpson dominance index λ] characterizing the biodiversity of fish communities inhabiting the Mangshe River (MR) and different purification zones of Lake Yanlong (PZ, the pretreatment zone; EZ, the emergent macrophyte zone; SZ, the submerged macrophyte zone; DZ, the deep purification zone). Different letters above bars denote significant differences among zones. Asterisks represent mean values.
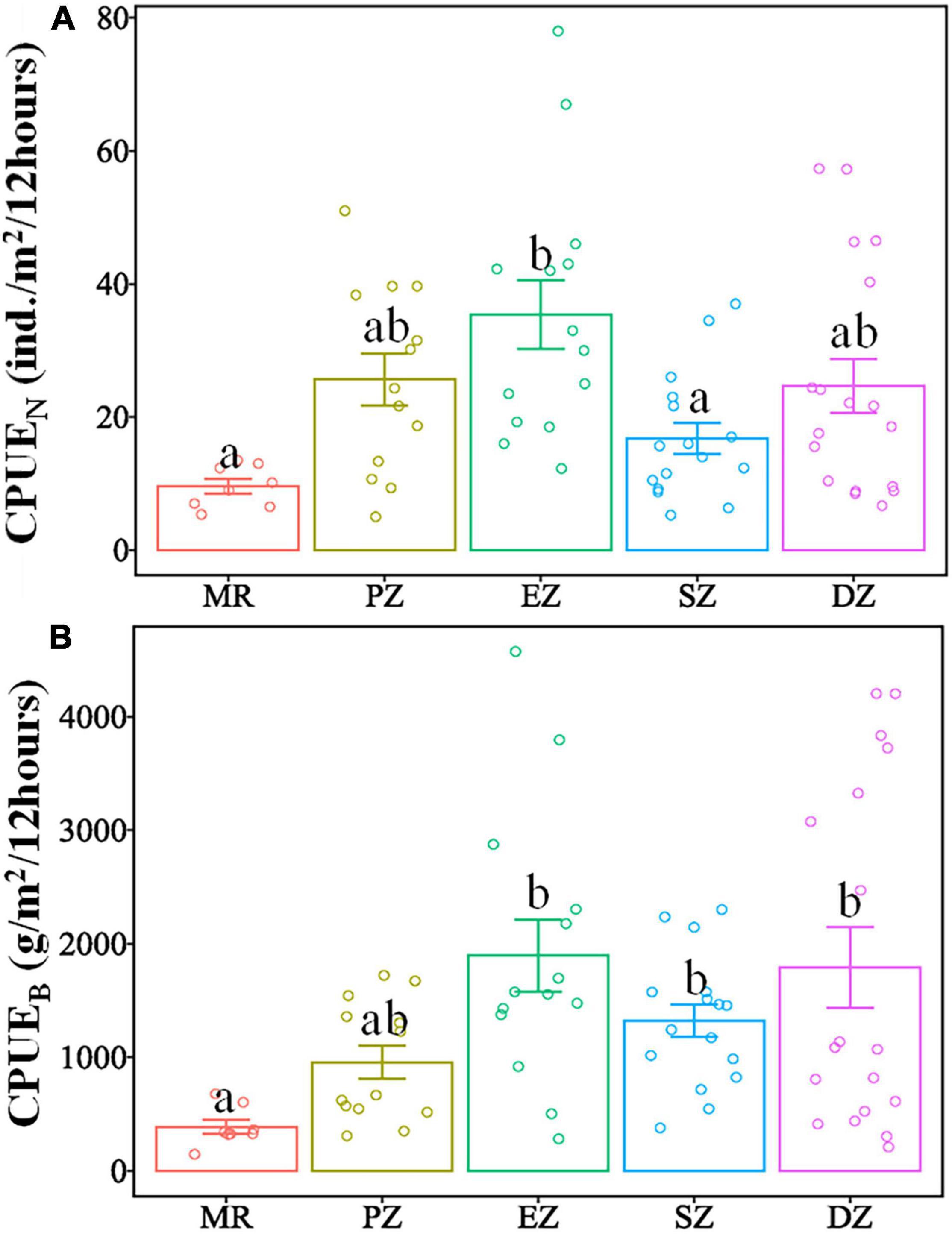
Figure 4. Mean catch per unit effort (CPUEN and CPUEB) of all fish species combined sampled from the Mangshe River (MR) and different purification zones of Lake Yanlong (PZ, the pretreatment zone; EZ, the emergent macrophyte zone; SZ, the submerged macrophyte zone; DZ, the deep purification zone) (A,B). Letters above bars denote significant differences among zones. Error bars represent 1 SE.
Dissimilarity and Differentiating Species Among Fish Communities
The ANOSIM analysis confirmed that fish community composition differed significantly among zones (R = 0.429, P < 0.001). Dissimilarity of fish was greatest between the MR and SZ (averaged 83.9%), followed by MR and EZ (83.8%), MR and DZ (83.0%), EZ and DZ (81.7%), and was lowest between PZ and SZ (71.6%) (Table 5). According to the SIMPER analysis, C. ectenes, C. auratus, P. simony, H. leucisculus, and H. bleekeri were the major differentiating species among zones. Specifically, C. ectenes had a higher contribution than the other differentiating species in the MR vs. DZ, PZ vs. DZ, EZ vs. DZ, and SZ vs. DZ. Contributions of C. ectenes for these comparisons were 30.4, 25.2, 20.7, and 28.1%, respectively. The species C. auratus had a higher contribution than the other differentiating species in the MR vs. EZ, PZ vs. EZ and the EZ vs. SZ, with contributions of 22.0, 18.3, and 22.2%, respectively. Next, H. bleekeri had a higher contribution in the MR vs. PZ and the PZ vs. SZ, with corresponding contributions of 19.8 and 18.2%, respectively. Lastly, P. simony had a higher contribution in the MR vs. SZ (30.9%) (Table 5).
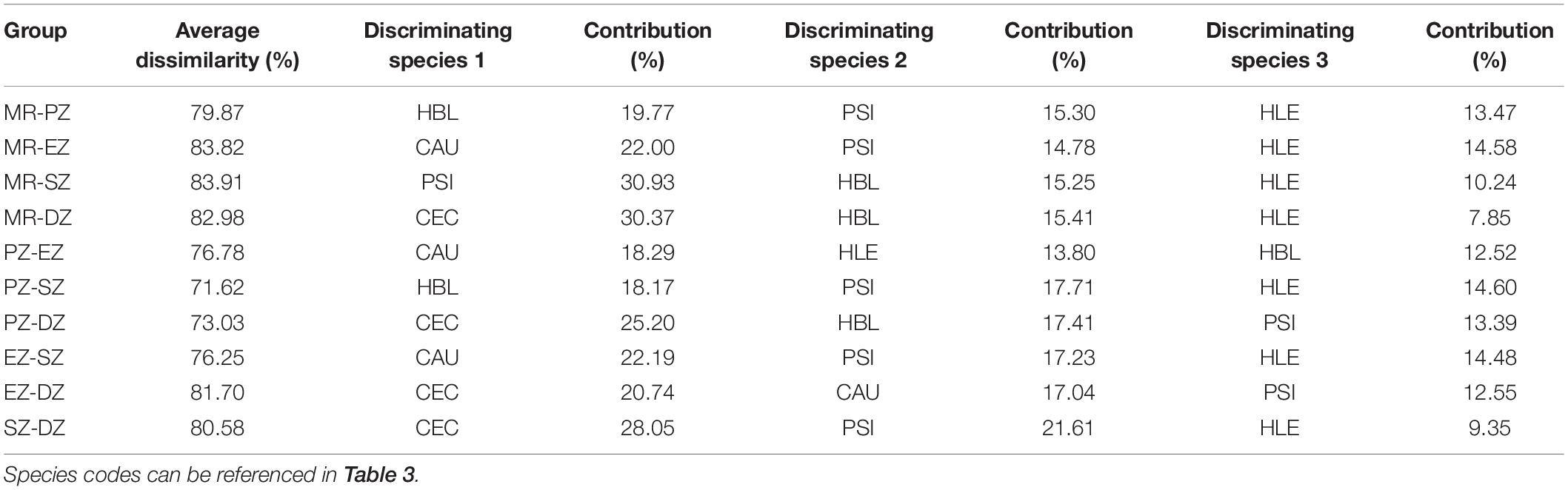
Table 5. Average dissimilarity and corresponding differentiating species of fish among the Mangshe River (MR) and different purification zones of Lake Yanlong (PZ, the pretreatment zone; EZ, the emergent macrophyte zone; SZ, the submerged macrophyte zone; DZ, the deep purification zone).
Environmental Effects on Fish Community Composition
The results from Mantel’s test comparing environmental variables to the relative abundance of dominant species indicated that WD was significantly correlated with the CPUEN of C. auratus (r = 0.08), C. carpio (r = 0.09), P. simony (r = 0.20) and C. ectenes (r = 0.25). Further, BAV was significantly correlated with the CPUEN of P. simony (r = 0.13) and CCA (r = 0.31), while NP was significantly correlated with the CPUEN of T. swinhonis (r = 0.20) and C. ectenes (r = 0.18) (p < 0.05 for all cases). Spearman correlation analyses using the 11 environmental variables measured showed that WD was significantly negatively correlated with CODMn (r = –0.24, p < 0.05) and significantly negatively correlated with BAV (r = –0.68, p < 0.001). The variable BAV was also significantly negatively correlated with NP (r = –0.23, p < 0.05). Alternatively, we observed significant positive correlations among most of the nutrient indices (i.e., TN, TP, CODMn) and Chl-α (Figure 5).
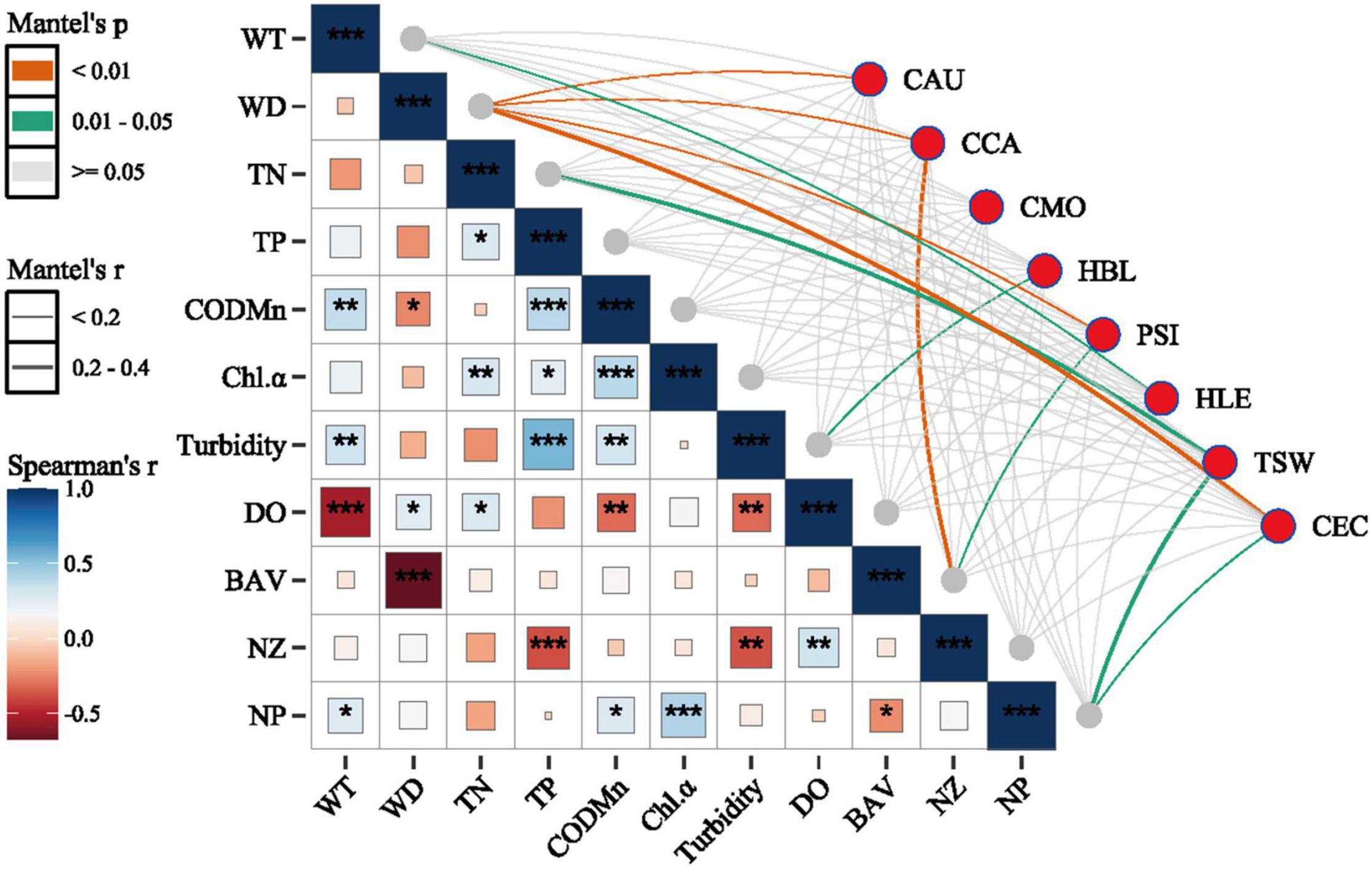
Figure 5. Results from Spearman correlation analyses and Mantel’s tests assessing associations among different environment variables and among environmental variables and the dominant species of fish sampled from the Mangshe River (MR) and different purification zones of Lake Yanlong (PZ, the pretreatment zone; EZ, the emergent macrophyte zone; SZ, the submerged macrophyte zone; DZ, the deep purification zone). WT, water temperature; WD, water depth; TN, total nitrogen; TP, total phosphorus; CODMn, chemical oxygen demand; Chl-α, Chlorophyll-α concentration; DO, dissolved oxygen; BAV, biomass of aquatic vegetation; NZ, density of zooplankton; NP, density of phytoplankton; CAU, Carassius auratus; CCA, Cyprinus carpio; CMO, Culter mongolicus; HBL, Hemiculter bleekeri; PSI, Pseudobrama simony; HLE, Hemiculter leucisculus; TSW, Toxabramis swinhonis; CEC, Coilia ectenes; * = 0.01 < p < 0.05, ** = 0.001 < p < 0.01, *** = p < 0.001.
The RDA model was significant (p < 0.001), indicating the existence of a relationship among environmental variables and the spatial distribution of dominant species. The model described 53.2 and 24.5% of variation in species distribution data along the first and second RDA axes (Figure 6A). All environmental variables (WT, WD, TN, TP, CODMn, Chl a, Turbidity, DO, BAV, NZ and NP) were selected for using in the RDA (VIFs were < 5 for all variables). According to the HP analysis and permutation tests, WD, NP and BAV explained 35.66, 19.08, and 15.13% of the total variation in fish community composition (all species) among zones, respectively. These were the same environmental variables that explained the abundance of dominant fish species among zones (PHP < 0.01 for WD, PHP < 0.05 for NP and BAV) (Figure 6B). In addition, C. ectenes preferred habitats (principally the PZ and DZ) with deeper water depths and lower aquatic vegetation cover, while H. bleekeri preferred deep habitats within the MR that lacked phytoplankton. Alternatively, P. simony, C. carpio, and C. auratus were found in shallow habitats (principally the EZ and SZ) with abundant aquatic vegetation (Figure 6A).
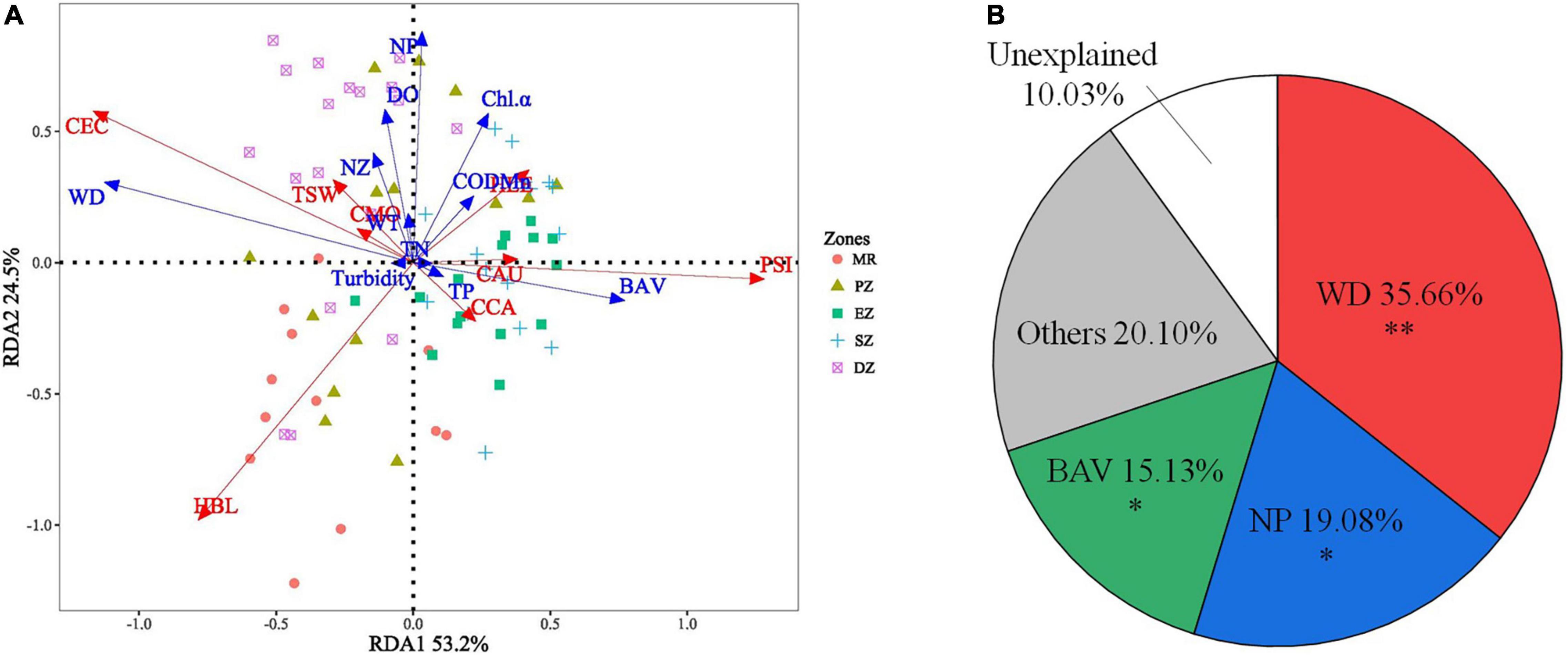
Figure 6. Results from the redundancy analysis (RDA) identifying the key environment variables explaining differences in the relative abundance of dominant fish species among the Mangshe River (MR) and purification zones of Lake Yanlong (A). The pie chart (B) describes results from the hierarchical portioning analysis (HP) characterizing the amount of independent explanatory power of each key environmental variable identified. WT, water temperature; WD, water depth; TN, total nitrogen; TP, total phosphorus; CODMn, chemical oxygen demand; Chl-α, Chlorophyll-α concentration; DO, dissolved oxygen; BAV, biomass of aquatic vegetation; NZ, density of zooplankton; NP, density of phytoplankton; CAU, Carassius auratus; CCA, Cyprinus carpio; CMO, Culter mongolicus; HBL, Hemiculter bleekeri; PSI, Pseudobrama simony; HLE, Hemiculter leucisculus; TSW, Toxabramis swinhonis; CEC, Coilia ectenes, * = 0.01 < p < 0.05, ** = 0.001 < p < 0.01.
Effects of Stocking Piscivorous Fish on Small-Bodied Fishes and Fish Community Diversity
There were significant differences in mean relative abundance and biomass of all small-bodied fishes combined (25 species) among the four purification zones of Lake Yanlong before (CPUEN: 49.72 ± 1.01 ind./m2/12 h; CPUEB: 2342.40 ± 148.44 g/m2/12 h) and after (CPUEN: 17.65 ± 2.62 ind./m2/12 h; CPUEB: 381.74 ± 64.49 g/m2/12 h) stocking of piscivorous fishes (Mann–Whitney test, P < 0.001 for all case) (SupplementaryTable 3). In addition, the fish community diversity indices (HN, J, D) increased significantly among the four purification zones of Lake Yanlong before (HN: 1.32 ± 0.15, J: 0.64 ± 0.07, D: 0.61 ± 0.06) and after (HN: 1.66 ± 0.10, J: 0.72 ± 0.04, D: 0.76 ± 0.04) stocking of piscivorous fishes (Mann–Whitney test, P < 0.05 for all case) (Supplementary Table 3).
Discussion
Spatial Patterns in Fish Community Composition
The species composition, diversity, relative abundance and biomass of fishes among shifting habitat types from the Mangshe River to the outlet of Lake Yanlong were distinctly different. Compared to the Mangshe River, fish species richness in the four purification zones of Lake Yanlong was reduced, especially species of migratory fish (e.g., E. bambusa and S. curriculus). Migratory fish—those that spawn in rivers and migrate to lakes for feeding, growth and maturation—have been severely limited for key habitats for fulfilling their life history over the past several decades as a result of fragmentation imposed by the extensive construction of hydraulic engineering projects (Zhou et al., 2014; Liao et al., 2018). Similar to river-reservoir ecosystems created by dams, Lake Yanlong is connected to the Mangshe River by a large pumping station, which can block migratory channels and still negatively affect the reproduction and growth of migratory fish despite providing additional lake-type habitat that could otherwise be utilized by these fish if accessible (Bai et al., 2020).
Despite reduced species richness, the relative abundance and biomass of fishes tended to be higher in Lake Yanlong relative to the adjacent Mangshe River. Lake Yanlong is a newly constructed ecosystem formed through artificial excavation. It is still in the early stages of ecological development, and some potential niches remain vacant (Cai et al., 2019). Small-bodied fishes (e.g., P. simony, H. leucisculus, and C. auratus) introduced from the Mangshe River are considered r-strategists (or opportunistic strategists), which can quickly occupy vacant ecological niches. These fishes were able to increase in abundance quickly and became the dominant species after entering Lake Yanlong due to the lack of natural enemies (Cai et al., 2019; Jeschke et al., 2019). In this study, six of the eight dominant species observed across purification zones were small-bodied fishes, with C. auratus, P. simony, and C. ectenes accounting for 28.1, 45.8, and 43.9% of the CPUEN of fishes in the EZ, SZ, and DZ, respectively. High abundances of small-bodied fishes can reduce species diversity within fish communities and affect the complexity and stability of ecosystems (Lima et al., 2016; Liu et al., 2017). Some studies demonstrated that the overabundance of small-bodied fishes and declines in overall fish diversity were accompanied by the deterioration of water quality (Karr, 1981, 1991; Karr et al., 1986; Yu et al., 2021). Moreover, the present study revealed a significant reduction in the Shannon–Wiener diversity index, the Pielou evenness index and the Simpson’s diversity index of fishes inhabiting the Mangshe River versus Lake Yanlong. Therefore, it is essential to seek appropriate strategies that help enhance the biodiversity of artificial water supply lakes in order to maintain proper ecosystem function.
Environmental Effects on Fish Community Composition
A wide range of studies have shown that the spatial distribution and composition of fish communities are influenced by both biotic (e.g., competition and predation) and abiotic factors (e.g., nutrient levels and habitat heterogeneity) (Li et al., 2010; Liu et al., 2017; Cai et al., 2019; Whiterod et al., 2021). Mantel’s tests and the RDA model all revealed that water depth, aquatic vegetation coverage, and phytoplankton concentrations were the major factors determining the spatial distribution of fishes when moving from the Mangshe River to the DZ of Lake Yanlong. The present study also showed that water depth was the most important abiotic factor influencing fish distribution, as it influenced ecological conditions present within the water column (e.g., food availability and light penetration), which has been important in other related studies (Keast, 1978; Mehner et al., 2005; Fernandes et al., 2010; Li et al., 2010; Cai et al., 2019). Benthivorous fish (i.e., C. carpio and C. auratus) preferred to live in relatively shallow habitat, principally within the EZ and SZ, while pelagic fish (i.e., C. ectenes) generally inhabited deeper water, principally within the PZ and DZ. Phytoplankton is the primary food source for many planktivorous and omnivorous fish species, and can affect the spatial distribution of fishes by modifying the trophic state of and dissolved oxygen concentrations within the water column (McClatchie et al., 1997; Amarasinghe et al., 2012). In this study, the distribution of H. bleekeri, C. ectenes, and T. swinhonis was closely related to the abundance of phytoplankton. A previous study revealed that H. bleekeri preferred flowing water habitats that lacked phytoplankton (Li et al., 2020). Conversely, C. ectenes and T. swinhonis are very common zooplanktivorous fish within the MLYRB, and are better suited for living in habitats with abundant plankton (Liu et al., 2008), so their association to phytoplankton in this study was not surprising.
The biomass of submerged aquatic vegetation was also a key factor affecting the distribution of fishes within Lake Yanlong, which is in accordance with other studies (Li et al., 2010; Massicotte et al., 2015; Cai et al., 2019). Generally, the density of fish, especially small-bodied fish, is positively correlated with the biomass of submerged aquatic vegetation (Cai et al., 2019). Extensive studies have shown that aquatic vegetation can affect the distribution of small-bodied fishes in the following ways: (1) providing abundant food resources for small fish (such as periphytic algae, invertebrates, and plant detritus) (Schneck et al., 2011; Massicotte et al., 2015); (2) the branches and leaves of different aquatic plants often interweave to form complex habitat, which can provide refuge for small fish (Clark et al., 2003; Kovalenko et al., 2012); and (3) the stems and leaves of aquatic plants can provide suitable spawning substrate for fish that produce either adhesive or semi- adhesive eggs (Su and Yao, 2002).
Strategies for Manipulating Fish Community Composition in Artificial Water Supply Lakes
Aforementioned studies and results from the present study indicate that the fish community in Lake Yanlong has undergone the process of miniaturization (i.e., over-abundance of small-bodied fishes), which has implications for ecosystem function, and is a common problem facing other artificial water supply lakes. Planktivorous small-bodied fishes (especially T. swinhonis and C. ectenes) in the MLYRB can exert heavy grazing pressure on the zooplankton community, leading to the release of phytoplankton, declines in water clarity and submerged macrophyte productivity, and loss of primary ecosystem function (i.e., purification of drinking water for humans) (Post and McQueen, 1987). Traditional biomanipulation, which aims to reduce the biomass of small-bodied fishes by stocking piscivorous fish, thus protecting large-bodied zooplankton capable of controlling phytoplankton, has been carried out extensively in temperate and subtropical lakes (Beklioglu et al., 2003; Mehner et al., 2004; Li et al., 2018). Based on the principle of traditional biomanipulation, we stocked native piscivorous fish (including C. alburnus, S. chuatsi, C. gariepinus and C. argus) into each purification zone of Lake Yanlong from 2017 through 2020. Our results revealed that the abundance and biomass of overabundant small-bodied fishes have declined significantly since biomanipulation started in 2017, indicating that the long-term stocking of piscivorous fish can effectively control small-bodied fishess. This in turn has supported environmental conditions more suitable for stimulating submerged macrophyte production and more efficient water purification. Some previous studies arrived at the same conclusion (Jeppesen et al., 1997; Li et al., 2018).
Stocking of piscivorous fishes started 5 years after the construction of Lake Yanlong was completed (2012). During this period, small-bodied fishes occupied most of the ecological niches available in the lake. Because small-bodied fish were already well established before stocking was initiated, they could have limited the early growth and survival of piscivorous fish, necessitating the need for repeated stocking efforts. In addition, previous studies demonstrated that repeated biomanipulation (such as stocking piscivorous fish or removing benthivorous and zooplanktivorous fishes) was necessary for achieving and maintaining desired effects over the long-term in shallow eutrophic lakes (Søndergaard et al., 2008; Li et al., 2018; Setubal and Riccardi, 2020; Guo et al., 2022).
Although stocking of piscivorous fish positively influenced aquatic ecosystem function in the present study, stocking piscivores could have unintended consequences worth noting. For example, the unintentional introduction of non-native species and or genetic hybridization that could reduce overall biodiversity (Buoro et al., 2016; Cucherousset et al., 2020). Therefore, we propose three mitigation strategies for the management of fish resources and improving the water quality of artificial water supply lakes. First, the stocking of piscivorous fish should be carried out immediately after artificial lakes are built and subsequently maintained for the long-term. Second, different types of piscivorous fish should be selected according to the characteristics of different habitats present within the lakes. For example, shallow habitats were more suitable for benthic-oriented piscivorous fish (e.g., S. chuatsi, C. gariepinus, and C. argus), while deep water habitats were more suitable for pelagic-oriented piscivores (e.g., C. alburnus). Third, the piscivorous species stocked should be native to avoid potential ecological hazards caused by non-native species and genetic hybridization. Although piscivorous fish played a key role in controlling small-bodied fish in Lake Yanlong, the utility of stocking piscivorous fish for improving water quality should be further verified in other artificial water supply lakes.
Conclusion
This study provided basic information on the composition, relative abundance and biomass, and diversity characteristics of fish communities occupying distinct habitat zones extending from the Mangshe River through to the outlet of Lake Yanlong. We confirmed that water depth, the amount of submerged aquatic vegetation and phytoplankton concentrations were the primary factors determining the spatial distribution of fishes along this gradient of artificially constructed habitat types. Based on the effects of stocking piscivorous fish in Lake Yanlong from 2017 to 2020, we suggest that the long-term stocking of piscivorous fish can effectively control small-bodied fishes. However, the species of piscivorous stocked should be tailored to habitat conditions present within the different purification zones of Lake Yanlong.
Data Availability Statement
The raw data supporting the conclusions of this article will be made available by the authors, without undue reservation.
Author Contributions
CG: investigation, data analysis, and writing – original draft. SL: investigation, resources, and data curation. WL: methodology, resources, and writing – review and editing. CL: methodology and writing – review and editing. TZ: resources and funding acquisition. JL: resources, writing – review and editing, and supervision. LL: resources and data curation. JS: investigation and data curation. XC: investigation and writing – review and Editing. AH: resources and writing – review and editing. All authors contributed to the article and approved the submitted version.
Funding
This study was supported by the National Key Research and Development Program of China (Grant No. 2020YFD0900500, 2019YFD0900601, and 2018YFE0110600), the Youth Innovation Promotion Association CAS (2019331), the Earmarked Fund for China Agriculture Research System (No. CARS-45), the Key Research and Development Program of Hubei Province (No. 2020BBB077), and the project “The monitoring and regulation of ecosystem of Lake Yanlong” funded by the Yancheng Water Affairs Group Co., Ltd.
Conflict of Interest
The authors declare that this study received funding from the Yancheng Water Affairs Group Co., LTD. The funder was not involved in the study design, collection, analysis, interpretation of data, the writing of this article or the decision to submit it for publication.
Publisher’s Note
All claims expressed in this article are solely those of the authors and do not necessarily represent those of their affiliated organizations, or those of the publisher, the editors and the reviewers. Any product that may be evaluated in this article, or claim that may be made by its manufacturer, is not guaranteed or endorsed by the publisher.
Acknowledgments
We are very grateful to Zhan Mai, Junfeng Li, Jie Ke, Yinzhe Zhang, Jiacheng wang, Bo Xu, and Lei Yang for their field sampling assistance. We would also like to thank Mantang Xiong, Xiang Ji, and Long Kong for their help in data analysis and creating figures for this manuscript.
Supplementary Material
The Supplementary Material for this article can be found online at: https://www.frontiersin.org/articles/10.3389/fevo.2022.921082/full#supplementary-material
References
Amarasinghe, L. D., Ganehiarachchi, G. A. S. M., Ruchirani, C., and Weerasinghe, T. (2012). “Phytoplankton and zooplankton community dynamics and aspects of food of two fish species in two streams and two lakes in presence and absence of Anophelese Mosquito Larvae in Mahaoya, Sri Lanka,” in Proceedings 13th Annual Research Symposium (Sarasavi Mawatha: University of Kelaniya).
American Public Health Association [APHA] (1992). Standard Methods for the Examination of Water and Wastewater, 18th Edn. Washington, DC: American Public Health Association.
Appelberg, M. (2000). Swedish Standard Methods for Sampling Freshwater Fish with Multi-Mesh Gillnets. Fiskeriverket Information. Goteborg: Institute for Freshwater Research.
Bai, J., Huang, G., Jiang, C., Zhang, W., Wang, Q., and Yao, L. (2020). Characteristics and historical changes of the fish assemblage in the Danjiangkou Reservoir. Biodivers. Sci. 28, 1202–1212. doi: 10.17520/biods.2020009
Beklioglu, M., Ince, O., and Tuzun, I. (2003). Restoration of the eutrophic Lake Eymir, Turkey, by biomanipulation after a major external nutrient control I. Hydrobiologia 489, 93–105. doi: 10.1023/A:1023466629489
Bhagowati, B., and Ahamad, K. U. (2018). A review on lake eutrophication dynamics and recent developments in lake modeling. Ecohydrol. Hydrobiol. 19, 155–166. doi: 10.1016/j.ecohyd.2018.03.002
Bonsdorff, E. (2021). Eutrophication: early warning signals, ecosystem-level and societal responses, and ways forward. Ambio 50, 753–758. doi: 10.1007/s13280-020-01432-7
Brinsmead, J., and Fox, M. G. (2002). Morphological variation between lake- and stream-dwelling rock bass and pumpkinseed populations. J. Fish Biol. 61, 1619–1638. doi: 10.1006/jfbi.2002.2179
Buoro, M., Olden, J. D., and Cucherousset, J. (2016). Global salmonidae introductions reveal stronger ecological effects of changing intraspecific compared to interspecific diversity. Ecol. Lett. 19, 1363–1371. doi: 10.1111/ele.12673
Cai, X. (2017). “Studies on the characteristics of fish community structure and the manipulation strategies in three drinking water lakes,” in Institute of Hydrobiology, the Chinese Academy of Sciences, Wuhan. (In Chinese).
Cai, X., Ye, S., Li, W., Fan, H., Li, Z., Zhang, T., et al. (2019). Spatio-temporal variability of small fishes related with environmental factors in a typical domestic tap water lake, Eastern China. J. Oceanol. Limnol. 37, 278–289. doi: 10.1007/s00343-019-7283-1
Clark, K. L., Ruiz, G. M., and Hines, A. H. (2003). Diel variation in predator abundance, predation risk and prey distribution in shallow-water estuarine habitats. J. Exp. Mar. Biol. Ecol. 287, 37–55. doi: 10.1016/S0022-0981(02)00439-2
Cote, D., Gregory, R. S., Morris, C. J., Newton, B. H., and Schneider, D. C. (2013). Elevated habitat quality reduces variance in fish community composition. J. Exp. Mar. Biol. Ecol. 440, 22–28. doi: 10.1016/j.jembe.2012.11.006
Cucherousset, J., Lassus, R., Riepe, C., Millet, P., Santoul, F., Arlinghaus, R., et al. (2020). Quantitative estimates of freshwater fish stocking practices by recreational angling clubs in France. Fish. Manage. Ecol. 28, 295–304. doi: 10.1111/fme.12471
Dumont, H. J. F. (2002). Guides to the Identification of the Microinverebrates of the Continental Waters of the World. Hague: SPB Academic Publisher.
Fernandes, I. M., Machado, F. A., and Penha, J. (2010). Spatial pattern of a fish assemblage in a seasonal tropical wetland: effects of habitat, herbaceous plant biomass, water depth, and distance from species sources. Neotrop. Ichthyol. 8, 289–298. doi: 10.1590/S1679-62252010000200007
Geheber, A. D., and Piller, K. R. (2012). Spatio-temporal patterns of fish assemblage structure in a coastal plain stream: appropriate scales reveal historic tales. Ecol. Freshw. Fish 21, 627–639. doi: 10.1111/j.1600-0633.2012.00584.x
Gou, M., Li, L., Ouyang, S., Wang, N., La, L., Liu, C., et al. (2021). Identifying and analyzing ecosystem service bundles and their socioecological drivers in the Three Gorges Reservoir Area. J. Clean. Prod. 307:127208. doi: 10.1016/j.jclepro.2021.127208
Guo, C., Chen, Y., Xia, W., Qu, X., Yuan, H., Xie, S., et al. (2019). Eutrophication and heavy metal pollution patterns in the water suppling lakes of China’s south-to-north water diversion project. Sci. Total Environ. 711:134543. doi: 10.1016/j.scitotenv.2019.134543
Guo, C., Li, W., Li, S., Mai, Z., Zhang, T., Liu, J., et al. (2022). Manipulation of fish community structure effectively restores submerged aquatic vegetation in a shallow subtropical lake. Environ. Pollut. 292:118459. doi: 10.1016/j.envpol.2021.118459
Holm-Hansen, O., and Riemann, B. (1978). Chlorophyll a determination: improvements in methodology. Oikos 30, 438–447.
Hothorn, T., Hornik, K., van de Wiel, M. A., and Zeileis, A. (2008). Implementing a class of permutation tests: the coin package. J. Stat. Softw. 28, 1–23. doi: 10.18637/jss.v028.i08
Huang, H. (2022). LinkET: Everything is Linkable. R Package Version 0.0.3.6. Available online at: https://github.com/Hy4m/linkET (accessed March 15, 2022).
James, F. C., and McCulloch, C. E. (1990). Multivariate analysis in ecology and systematics: panacea or Pandora’s box? Annu. Rev. Ecol. Syst. 21, 129–166.
Jeppesen, E., Jensen, J. P., Søndergaard, M., Lauridsen, T., Pedersen, L. J., and Jensen, L. (1997). Top-down control in freshwater lakes: the role of nutrient state, submerged macrophytes and water depth. Hydrobiologia 342, 151–164.
Jeppesen, E., Jensen, J. P., Kristensen, P., Søndergaard, M., Mortensen, E., Sortkjær, O., et al. (1990). Fish manipulation as a lake restoration tool in shallow, eutrophic, temperate lakes 2: threshold levels, long-term stability and conclusions. Hydrobiologia 200, 219–227. doi: 10.1007/BF02530341
Jeppesen, E., Meerhoff, M., Holmgren, K., González-Bergonzoni, I., Mello, F. T., Declerck, S. A. J., et al. (2010). Impacts of climate warming on lake fish community structure and potential effects on ecosystem function. Hydrobiologia 646, 73–90. doi: 10.1007/s10750-010-0171-5
Jeschke, J. M., Gabriel, W., and Kokko, H. (2019). R-Strategists/K-Strategists. Amsterdam: Elsevier.
Karr, J. R. (1991). Biological integrity: a long-neglected aspect of water resource management. Ecol. Appl. 1, 66–84. doi: 10.2307/1941848
Karr, J. R., Fausch, K. D., Angermeier, P. L., Yant, P. R., and Schlosser, I. J. (1986). Assessing Biological Integrity in Running Waters: a Method and its Rationale. Special Publication, 5. Champaign, IL: Illinois Natural History Survey.
Kassambara, A. (2021). rstatix: Pipe-Friendly Framework for Basic Statistical Tests. R Package Version 0.7.0. Available online at: https://CRAN.R-project.org/package=rstatix (accessed March 15, 2022).
Keast, A. (1978). Trophic and spatial interrelationships in the fish species of an Ontario temperate lake. Environ. Biol. Fish. 3, 7–31.
Kovalenko, K. E., Thomaz, S. M., and Warfe, D. M. (2012). Habitat complexity: approaches and future directions. Hydrobiologia 685, 1–17. doi: 10.1007/s10750-011-0974-z
Lai, J., Zou, Y., Zhang, J., and Peres-Neto, P. (2022). Generalizing hierarchical and variation partitioning in multiple regression and canonical analysis using the rdacca.hp R package. Methods Ecol. Evol. 13, 782–788. doi: 10.1111/2041-210X.13800
Li, B., Wang, Y., Tan, W., Saintilan, N., Lei, G., and Wen, L. (2021). Land cover alteration shifts ecological assembly processes in floodplain lakes: consequences for fish community dynamics. Sci. Total Environ. 782:146724. doi: 10.1016/j.scitotenv.2021.146724
Li, S., Li, W., Guo, C., Mai, Z., Liao, C., Liu, J., et al. (2021). Phytoplankton community structure and its relationships with environmental factors in a new urban land-scape lake (in Chinese). Biot. Resour. 43, 535–544.
Li, W., Gao, X., Liu, H., and Cao, W. (2020). Coexistence of two closely related cyprinid fishes (Hemiculter bleekeri and Hemiculter leucisculus) in the upper Yangtze River, China. Diversity 12:284. doi: 10.3390/d12070284
Li, W., Hicks, B. J., Lin, M. L., Guo, C., Zhang, T., Liu, J., et al. (2018). Impacts of hatchery-reared mandarin fish Siniperca chuatsi stocking on wild fish community and water quality in a shallow Yangtze lake. Sci. Rep. 8:11481. doi: 10.1038/s41598-018-29758-z
Li, W., Zhang, T., and Li, Z. (2010). Spatial distribution and abundance of small fishes in Xiaosihai Lake, a shallow lake along the Changjiang (Yangtze) River, China. J. Oceanol. Limnol. 28, 470–477. doi: 10.1007/s00343-010-9082-6
Liao, C., Chen, S., De Silva, S. S., Correa, S. B., Yuan, J., Zhang, T., et al. (2018). Spatial changes of fish assemblages in relation to filling stages of the Three Gorges Reservoir, China. J. Appl. Ichthyol. 34, 1293–1303. doi: 10.1111/jai.13798
Lima, A. C., Wrona, F. J., and Soares, A. M. V. M. (2016). Fish traits as an alternative tool for the assessment of impacted rivers. Rev. Fish Biol. Fish. 27, 31–42. doi: 10.1007/s11160-016-9446-x
Liu, E., Bao, C., and Wan, Q. (2008). The changes of the catch and age structure of lake anchovy and the relationship with environmental variation in Lake Taihu. J. Fish. China 32, 229–235.
Liu, X., Ao, X., Ning, Z., Hu, X., Wu, X., and Ouyang, S. (2017). Diversity of fish species in suichuan river and shushui river and conservation value, China. Environ. Biol. Fish. 100, 493–507. doi: 10.1007/s10641-017-0580-7
Mao, Z., Gu, X., Zeng, Q., Zhou, L., Wang, X., Wu, L., et al. (2011). Community structure and diversity of fish in Lake Taihu. Chin. J. Ecol. 30, 2836–2842. doi: 10.13292/j.1000-4890.2011.0435
Massicotte, P., Bertolo, A., Brodeur, P., Hudon, C., Mingelbier, M., and Magnan, P. (2015). Influence of the aquatic vegetation landscape on larval fish abundance. J. Great Lakes Res. 41, 873–880. doi: 10.1016/j.jglr.2015.05.010
McClatchie, S., Millar, R. B., Webster, F., Lester, P. J., Hurst, R., and Bagley, N. (1997). Demersal fish community diversity off New Zealand: is it related to depth, latitude and regional surface phytoplankton? Deep Sea Res. I Oceanogr. Res. Pap. 44, 647–667.
Mehner, T., Arlinghaus, R., Berg, S., Dörner, H., Jacobsen, L., Kasprzak, P., et al. (2004). How to link biomanipulation and sustainable fisheries management: a step-by-step guideline for lakes of the European temperate zone. Fish. Manage. Ecol. 11, 261–275. doi: 10.1111/j.1365-2400.2004.00401.x
Mehner, T., and Brucet, S. (2021). Structure of fish communities in lakes and its abiotic and biotic determinants. Ref. Module Earth Syst. Environ. Sci. 2, 77–88. doi: 10.1016/B978-0-12-819166-8.00004-9
Mehner, T., Diekmann, M., Brämick, U., and Lemcke, R. (2005). Composition of fish communities in German lakes as related to lake morphology, trophic state, shore structure and human-use intensity. Freshw. Biol. 50, 70–85. doi: 10.1111/j.1365-2427.2004.01294.x
Mello, F. T., Meerhoff, M., Pekcan-Hekim, Z., and Jeppesen, E. (2009). Substantial differences in littoral fish community structure and dynamics in subtropical and temperate shallow lakes. Freshw. Biol. 54, 1202–1215. doi: 10.1111/j.1365-2427.2009.02167.x
Ministry of Environmental Protection of China (2002). Envirinmental Protection Industry Standard of People Republic of China, Techical Specifications Requirements for Monitoring of Surface Water and Waste Water. Beijing: Ministry of Environmental Protection of China.
Moal, M. L., Gascuel-Odoux, C., Ménesguen, A., Souchon, Y., Étrillard, C., Levain, A., et al. (2019). Eutrophication: a new wine in an old bottle? Sci. Total Environ. 651, 1–11. doi: 10.1016/j.scitotenv.2018.09.139
Oberdorff, T., Pont, D., Hugueny, B., and Cessel, D. (2001). A probabilistic model characterizing fish assemblages of French rivers: a framework for environmental assessment. Freshw. Biol. 46, 399–415. doi: 10.1046/j.1365-2427.2001.00669.x
Oksanen, J., Simpson, G. L., Blanchet, F. G., Kindt, R., Legendre, P., Minchin, P. R., et al. (2022). vegan: Community Ecology Package. R Package Version 2.6-2. Available online at: https://CRAN.R-project.org/package=vegan (accessed March 15, 2022).
Paredes, M. A., and Montecino, V. (2011). Size diversity as an expression of phytoplankton community structure and the identification of its patterns on the scale of fjords and channels. Cont. Shelf Res. 31, 272–281. doi: 10.1016/j.csr.2010.07.012
Pianka, E. R. (1971). Ecology of the agamid lizard Amphibolurus isolepis in western Australia. Copeia 3, 527–536.
Post, J. R., and McQueen, D. J. (1987). The impact of planktivorous flsh on the structure of a plankton community. Freshw. Biol. 17, 79–89.
Qin, B., Zhu, G., Gao, G., Zhang, Y., Li, W., Paerl, H. W., et al. (2010). A drinking water crisis in Lake Taihu, China: linkage to climatic variability and lake management. Environ. Manage. 45, 105–112. doi: 10.1007/s00267-009-9393-6
R Core Team (2021). R: A Language and Environment for Statistical Computing. Vienna: R Foundation for Statistical Computing.
Ren, X. (2021). Research on Water Purification and Spatial-Temporal Response of Phytoplankton in a Constructed Wetland-Reservior System (Yanlong Lake). Ph.D. dissertation. Beijing: University of Chinese Academy of sciences.
Schneck, F., Schwarzbold, A., and Melo, A. S. (2011). Substrate roughness affects stream benthic algal diversity, assemblage composition, and nestedness. J. North Am. Benthol. Soc. 30, 1049–1056. doi: 10.1899/11-044.1
Setubal, R. B., and Riccardi, N. (2020). Long-term effects of fish biomanipulation and macrophyte management on zooplankton functional diversity and production in a temperate shallow lake. Limnology 21, 305–317. doi: 10.1007/s10201-020-00617-z
Shannon, C. E., and Weaver, W. (1949). The Mathematical Theory of Communication. Urbana, IL: University of Illinois Press.
Smith, V. H., and Schindler, D. W. (2009). Eutrophication science: where do we go from here? Trends Ecol. Evol. 24, 201–207. doi: 10.1016/j.tree.2008.11.009
Søndergaard, M., Liboriussen, L., Pedersen, A. R., and Jeppesen, P. E. (2008). Lake restoration by fish removal: short- and long-term effects in 36 Danish lakes. Ecosystems 11, 1291–1305. doi: 10.1007/s10021-008-9193-5
Su, S., and Yao, W. (2002). A brief review on mutual relationship between submerged macrophytes and environment. Agro Environ. Prot. 21, 570–573.
Tang, X. (2020). Evolution, driving mechanism and control strategy for eutrophication in Changjiang River Basin (in Chinese). Yangtze River 51, 80–87.
Wang, J., Fu, Z., Qiao, H., and Liu, F. (2019). Assessment of eutrophication and water quality in the estuarine area of Lake Wuli, Lake Taihu, China. Sci. Total Environ. 650, 1392–1402. doi: 10.106/j.scitotenv.2018.09.137
Whiterod, N. S., Brown, L., Bachmann, M., Farrington, L., and Vilizzi, L. (2021). Long and lasting: spatial patterns and temporal trends in a fish community responding to landscape-scale hydrological restoration of a coastal freshwater wetland complex. Landsc. Ecol. 36, 1511–1532. doi: 10.1007/s10980-021-01219-5
Xie, P., and Liu, J. (2001). Practical success of biomanipulation using filter-feeding fish to control cyanobacteria blooms: a synthesis of decades of research and application in a subtropical hypereutrophic lake. Sci. World J. 1:276487. doi: 10.1100/tsw.2001.67
Yang, P., Zhou, T., Tang, W., Zhang, Y., and Liu, X. (2018). Abundance dynamic of catfishes in Jingjiang Section of the Yangtze River (in Chinese). Resour. Environ. Yangtze Basin 27, 135–144. doi: 10.11870/cjlyzyyhj201801016
Yu, J., Zhen, W., Kong, L., He, H., Zhang, Y., and Yang, X. (2021). Changes in pelagic fish community composition, abundance, and biomass along a productivity gradient in subtropical lakes. Waters 13:858. doi: 10.3390/w13060858
Zhang, Q., Dong, X., Yang, X., Odgaard, B. V., and Jeppesen, E. (2019). Hydrologic and anthropogenic influences on aquatic macrophyte development in a large, shallow lake in China. Freshw. Biol. 64, 799–812. doi: 10.1111/fwb.13263
Zhang, T. (2005). “Life-history strategies, trophic patterns and community structure in the fishes of Lake Biandantang,” in Institute of Hydrobiology, the Chinese Academy of Sciences, Wuhan. (In Chinese).
Zhen, W., Zhang, X., Guan, B., Yin, C., Yu, J., Jeppesen, E., et al. (2018). Stocking of herbivorous fishes in eutrophic shallow clear-water lakes to reduce standing height of submerged macrophytes while maintaining their biomass. Ecol. Eng. 113, 61–64. doi: 10.1016/j.ecoleng.2017.10.011
Zhou, J., Zhao, Y., Song, L., Bi, S., and Zhang, H. (2014). Assessing the effect of the Three Gorges reservoir impoundment on spawning habitat suitability of Chinese sturgeon (Acipenser sinensis) in Yangtze River, China. Ecol. Inf. 20, 33–46. doi: 10.1016/j.ecoinf.2014.01.008
Zhu, G., Xu, H., Zhu, M., Zou, W., Guo, C., Ji, P., et al. (2019). Changing characteristics and driving factors of trophic state of lakes in the middle and lower reaches of Yangtze River in the past 30 years (in Chinese). J. Lake Sci. 31, 1510–1524. doi: 10.18307/2019.0322
Zou, W., Zhu, G., Cai, Y., Vilmi, A., Xu, H., Zhu, M., et al. (2020). Relationships between nutrient, chlorophyll a and Secchi depth in lakes of the Chinese eastern plains ecoregion: implications for eutrophication management. J. Environ. Manage. 260:109923. doi: 10.1016/j.jenvman.2019.109923
Zuo, Z., Zhu, X., Hu, W., Cheng, B., Chen, Y., and Cang, J. (2015). Study on the quality characteristics of the raw water of the drinking water source, Mangshe River, Yancheng and the evaluation of the water quality purification effect of Yanlong Lake project (in Chinese). Environ. Pollut. Control 37, 61–65. doi: 10.15985/j.cnki.1001-3865.2015.05.012
Keywords: fish community, fish biodiversity, artificial water supply lake, Lake Yanlong, regulation strategies
Citation: Guo C, Li S, Li W, Liao C, Zhang T, Liu J, Li L, Sun J, Cai X and Hansen AG (2022) Spatial Variation in the Composition and Diversity of Fishes Inhabiting an Artificial Water Supply Lake, Eastern China. Front. Ecol. Evol. 10:921082. doi: 10.3389/fevo.2022.921082
Received: 15 April 2022; Accepted: 22 June 2022;
Published: 04 August 2022.
Edited by:
Tian Zhao, Key Laboratory of Mountain Ecological Rehabilitation and Biological Resource Utilization, Chengdu Institute of Biology (CAS), ChinaReviewed by:
Ratha Chea, National University of Battambang, CambodiaRenee Van Dorst, Leibniz-Institute of Freshwater Ecology and Inland Fisheries (IGB), Germany
Sven Matern, Leibniz-Institute of Freshwater Ecology and Inland Fisheries (IGB) Berlin, Germany, in collaboration with reviewer, RV
Copyright © 2022 Guo, Li, Li, Liao, Zhang, Liu, Li, Sun, Cai and Hansen. This is an open-access article distributed under the terms of the Creative Commons Attribution License (CC BY). The use, distribution or reproduction in other forums is permitted, provided the original author(s) and the copyright owner(s) are credited and that the original publication in this journal is cited, in accordance with accepted academic practice. No use, distribution or reproduction is permitted which does not comply with these terms.
*Correspondence: Wei Li, liwei@ihb.ac.cn