- 1Chair of Forest Growth and Dendroecology, Institute of Forest Sciences, Albert Ludwig University of Freiburg, Freiburg, Germany
- 2Department of Botany, University of Innsbruck, Innsbruck, Austria
- 3Jahrringlabor Hofmann und Reichle, Nürtingen, Germany
- 4Bavarian State Department for Cultural Heritage, Thierhaupten, Germany
- 5Labor Dendron, Basel, Switzerland
- 6Museum of Archaeology, University of Stavanger, Stavanger, Norway
- 7Chair of Forest History, Institute of Forest Sciences, Albert Ludwig University of Freiburg, Freiburg, Germany
- 8Amt für Archäologie, Frauenfeld, Switzerland
- 9Generaldirektion Kulturelles Erbe Rheinland-Pfalz, Direktion Rheinisches Landesmuseum Trier, Trier, Germany
- 10Institut für Ur- und Frühgeschichte und Vorderasiatische Archäologie, Universität Heidelberg, Heidelberg, Germany
- 11Competence Center for Underwater Archaeology and Dendrochronology, Office for Urbanism, Zurich, Switzerland
Spruce is the most cultivated tree species in modern forestry in Central Europe, since it has the ability to grow on many soil types with profitable biomass accumulation. However, even-aged and uniform spruce forests are affected by recurring droughts and associated biotic stressors leading to large-scale diebacks across Central Europe causing controversies among foresters and nature conservationists. We investigate the role of spruce in historical woodlands by using 15666 spruce timbers from historical buildings and on the basis of pollen-based land cover estimates using the REVEALS model from 157 pollen sites in southern Central Europe. Start and end dates of the spruce timber samples and their dendrological characteristics (age, growth rates and stem diameters) were used to obtain information on past forest structures. Tree rings and REVEALS estimates are combined at a spatial scale of 1° × 1° resolution, grouped in four sub-regions, and a temporal resolution of 100-year time windows starting from 1150 to 1850 CE. We found that spruce dominates the species assemblage of construction timber with almost 41% and that the harvest age varies little through time, whereas a declining trend in growth rates and stem diameters are observed toward times before modern forestry. Temporal and regional differences in spruce abundance and building activity were found highlighting periods of (i) land abandonment and forest expansion in the 14th century, (ii) increased wood consumption during the 16th century due to population increase and beginning industrial developments, (iii) a forest recovery during and after the Thirty years' war, and (iv) afforestation efforts from the 1650s onwards. Furthermore, this study shows that spruce was constantly present in the study area in most studied sub-regions for the last 800 years. We demonstrate the need of combining tree-ring and pollen data to identify spatiotemporal patterns in spruce abundance and utilization.
Introduction
Norway spruce (Picea abies (L.) H. Karst.) is considered native to the boreal and subalpine conifer forests and covers mountain regions in Central Europe and vast areas in northern and eastern Europe (Caudullo et al., 2016). Its natural distribution ranges from the Balkan peninsula in the southeast, to the Scandinavian peninsula in the northwest, and east-west from the French Alps to the Ural Mountains. East of the Ural Mountains, Norway spruce (Picea abies spp. abies) is replaced by Siberian spruce (Picea abies spp. obovata), however, hybrid forms exist. In the Balkan peninsula, Serbian spruce (Picea omorika (Pančić) Purk.) is endemic and occurs in small populations in Serbia and Bosnia and Herzegovina.
In Europe, Norway spruce naturally dominates forests in the montane and subalpine zones. The altitudinal range spans from sea level in northern and eastern Europe to above 2300 m above sea level (asl) in the Italian Alps (Jansson et al., 2013). Spruce can grow and achieve good yields and high wood qualities at different site conditions and soil types but it is mostly found on acidic soils. However, the species prefers deep nutrient-rich and moist soils. It can occur as a pioneer species and as a partially shade-tolerant species it can be a natural main or secondary tree species. Spruce can compete with Scots pine (Pinus sylvestris L.) on dry conditions, European beech (Fagus sylvatica L.) and European silver fir (Abies alba Mill.) on cool conditions at mid elevations (800–1800 m asl), and with European larch (Larix decidua Mill.) and Swiss stone pine (Pinus cembra L.) at high elevations (1800–2100 m asl) (Caudullo et al., 2016).
Norway spruce likely spread from two late-glacial refugia, one in western Russia (region of Moscow) and one in the Alps and the Carpathians (Huntley and Birks, 1983), toward Fennoscandia (Russian population), and toward western and northern Europe (eastern European population), respectively (Björkman, 1996; Giesecke and Bennett, 2004; Seppä et al., 2009; Jansson et al., 2013; Giesecke et al., 2017). For the regions of Bavaria, southern Germany, and Bohemia, Czech Republic, first Holocene pollen of Norway spruce (and hereafter referred to as spruce) were recorded 10500 to 10000 years ago at high elevations (> 900 m asl), whereas the species appeared to be dominant in the forests at high- and mid-elevations (ca. 700–900 m asl) over the past 9000 years (Carter et al., 2018). According to pollen-based land cover models and in particular the REVEALS model (Sugita, 2007; see method chapter), the abundance of spruce in the Bohemian and Bavarian Forests increased to more than 50% of the total regional land-cover from 9000 cal. years BP (Carter et al., 2018). In southernmost Germany, at the borders between Germany, Austria and Switzerland, the grid-based REVEALS reconstructions show a regular increase of spruce abundance to 17% at about 6700–7200 cal. years BP (see grid 4 in Marquer et al., 2017). This is further supported by the work from Burga and Hussendörfer (2001) who first observed spruce pollen between 7500–6500 cal. BP in eastern Switzerland, and from Latałowa and van der Knaap (2006) who recorded the first spruce pollen from 7000–6000 cal. years BP in south-western Germany (southern Black Forest) and north-eastern Switzerland (Thurgau). In the southern Black Forest first pollen evidence starts 9000 BP, together with silver fir and beech, but spruce became a minor component of the forest after 3000 BP (Knopf et al., 2019). REVEALS reconstructions also show relatively low abundances (maximum of ca. 8 %) of spruce through the Holocene for the southern Black Forest and northern Switzerland (Marquer et al., 2017, grid 5) and the central and northern Black Forest (Marquer et al., 2017, grid 3), except for the last centuries when the abundances greatly increased (Marquer et al., 2017). Traditional pollen data demonstrate that the species colonized central and western Switzerland (south-western Jura mountains) at around 4500 cal. BP (Burga and Hussendörfer, 2001) while at the same time, it further expanded to central and eastern Germany (6000–5000 BP) (Latałowa and van der Knaap, 2006). During 3000–2000 BP it was established in the Vosges Mountain, France (Kalis, 1984; Burga and Hussendörfer, 2001; Latałowa and van der Knaap, 2006). In the northern Black Forest, spruce expanded much later, after the late medieval period (Rösch, 2012), whereas in the eastern Black Forest with a rather continental climate, spruce was established before silver fir and beech but was later replaced by those (Sudhaus, 2005). During 1000–0 BP the species was found in western Germany, Luxemburg, eastern Belgium, and northern Germany and only low occurrences throughout were observed in northern and eastern Germany, Poland, Denmark, Belgium (Latałowa and van der Knaap, 2006). Lang (1994) estimated for spruce a general migration rate of 60 to 130 meters per year. So far, our understanding of the history of spruce abundance mainly relies on traditional qualitative pollen data and only a few REVEALS reconstructions, which more realistically captures plant abundance in landscapes (Marquer et al., 2014).
In Central Europe, especially in Germany, spruce has been cultivated since the beginning of the 18th century and planted outside its natural distribution range in the lowlands (Spiecker, 2003; Jansen et al., 2017; Schmidt, 2017), which lead to a transformation of natural forests (Jansson et al., 2013). Nevertheless, a planned afforestation of coniferous species, including spruce, was conducted around Nuremberg (Bavaria) in as early as the 14th century (Warde, 2006; Enderle et al., 2021). Systematic large-scale spruce afforestation, however, was practiced from the 19th century onwards (Schmidt-Vogt, 1977; Schmidt, 2017).
Spruce wood has favorable mechanical properties such as good strength and elasticity, in relation to its low weight (mean density is 470 kg/m3) (e.g., Karopka, 2017). It is therefore suitable for a variety of purposes from construction timber to the production of furniture and instruments. With its long fibers (tracheids), it is furthermore important for manufacturing paper of high strength. This universal use in tandem with its fast growth made spruce the most commonly planted tree species in many European regions. Today, the importance of spruce in future silviculture is intensively discussed since the species suffers severely under recent climate change.
To better understand the history of spruce quantitative information are needed. For this purpose, new REVEALS reconstructions with a higher temporal resolution have been performed at a grid cell scale of one degree for southern Germany, north-eastern France, northern Switzerland, Austria, northern Italy, and western Czech Republic (Supplementary Figure S1) for centennial time windows. Results were compared with tree-ring data, i.e., start and end dates and tree growth characteristics, of absolutely dated spruce timber. This combination of tree rings and REVEALS reconstructions aims at providing new insights about a long-term context of spruce abundance and distribution, and its role in Central European forests in terms of forest structure and wood utilization.
Materials and Methods
Tree-Ring Data
We compiled 18031 dendrochronologically dated and georeferenced spruce timber samples, predominantly from historical buildings, covering areas within and outside the present species' natural distribution range in southern Germany, Switzerland and north-eastern France (Figure 1). From this data, we used start dates, the year of the first measured ring, and end dates, which is the year of the last measured ring. End dates were used as the majority corresponds to felling dates, that is, the last measured ring has a waney edge, and thus is the last formed ring before tree felling (Supplementary Figure S2). From this compilation, a total of 15666 end dates cover the 1150–1850 period and the region 7° to 13° E and 47° to 51°N, which were used for further analysis. We assume that the majority of the utilized spruce trees originate from regional forests. The study period is restricted to 1150–1850 due to insufficient and thus non-representative data replication before and after this time span.
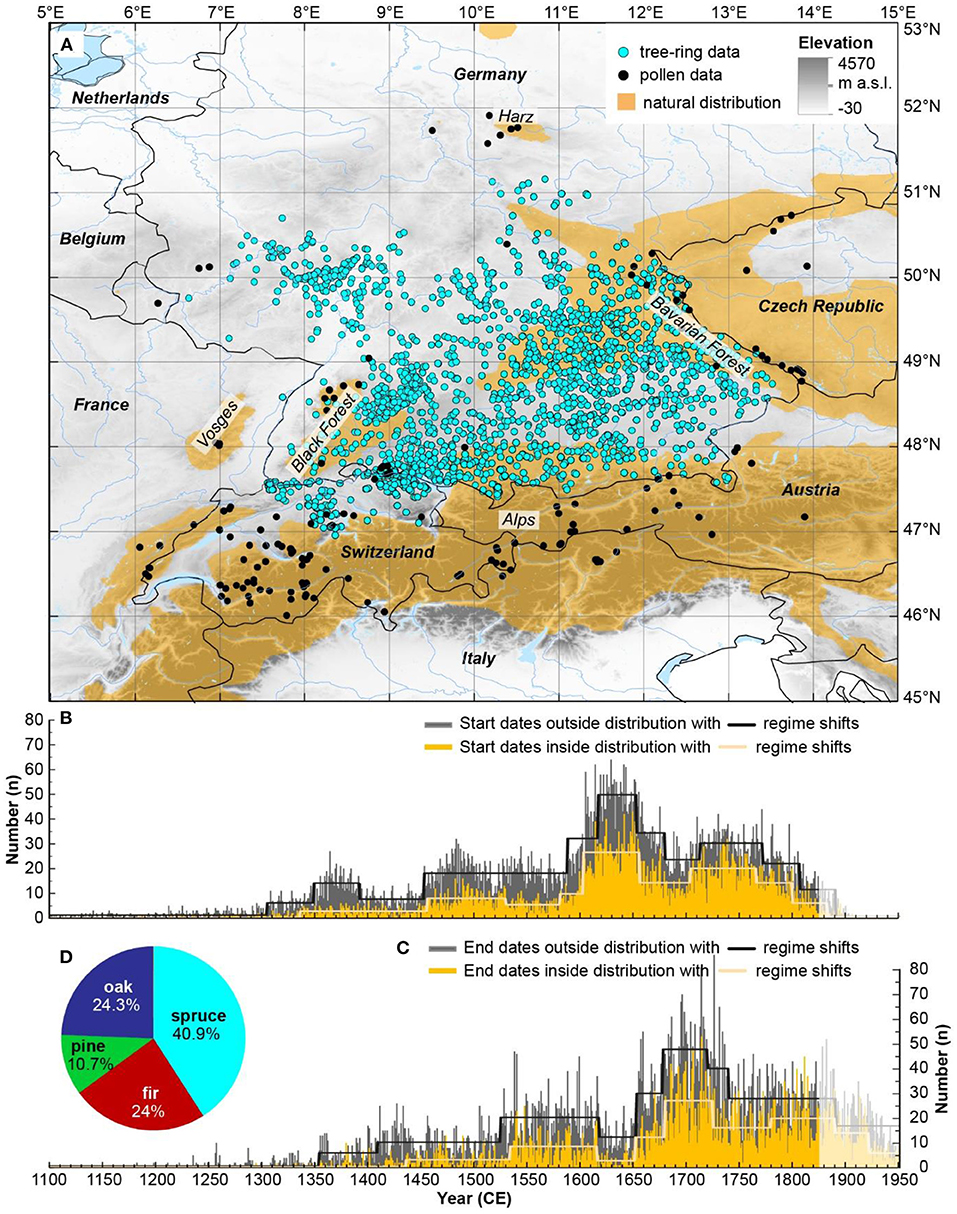
Figure 1. (A) Location of spruce tree-ring (turquois dots) and pollen data (black dots) used in this study. The elevation map (see legend for color) is superimposed by the natural distribution of Norway spruce (Picea abies) (orange areas) (source: http://www.euforgen.org/species/picea-abies/). (B) Start dates and (C) end dates with significant shifts in their temporal distribution (regime shifts) from historical spruce timber for the region within (orange bars, yellow line) and outside (gray bars, black line) the natural distribution of spruce. (D) Main tree species used as timber in constructions during the period 1150 – 1850.
To demonstrate the significance of spruce for construction wood, the end dates of all other important timber species for the same region and period (4086 pines, 9212 firs, 9315 oaks and 33 beech trees) were used for comparison.
Start and end dates were aggregated into periods of 100 years for comparison with the pollen-based land cover data. All data were made available by the authors whereas the data compilation for spruce was complemented with tree-ring data from Wilson et al. (2004), which was accessed from the International Tree-Ring Databank (ITRDB).
REVEALS: Pollen-Based Vegetation Estimates
The study area covers similar regions as the temporally continuous Holocene REVEALS reconstructions for Europe published by Marquer et al. (2017) and more recently by Githumbi et al. (2021). These reconstructions provide quantitative estimates of the regional plant abundance based on pollen records at a 1° × 1° spatial scale and for time slices of 500 years, except for the three most recent time slices covering the last 700 years. For a comparison with information based on tree rings (annual resolution), the temporal resolution of the pollen-based reconstructions should be increased (i.e., smaller time slices). Several tests have been performed to get a good compromise between an increase of the temporal resolution and a reliable pollen count per time slices. The higher the pollen count is, the lower the uncertainties in the REVEALS reconstructions are. The results show that an increase of the temporal resolution from 500 years to 100 years is reasonable. The minimum number of pollen grains per time slice is 400.
For the REVEALS runs, we used 157 pollen records (see Supplementary Table) selected from the NEOTOMA database (https://www.neotomadb.org/) and European Pollen Database (http://www.europeanpollendatabase.net/index.php). These pollen archives were grouped into 31 grid-cells of 1° × 1° (Supplementary Figure S1). REVEALS has been applied at a temporal resolution of 100 years per time slice for the last 2000 years. We considered 25 plant taxa and all available pollen records (mainly from lakes, bogs and mires) distributed within the 1° × 1° area of each grid. Note that pollen records from multiple sites (lakes or bogs; small and large) are suitable for reliable REVEALS reconstructions (Trondman et al., 2016). The age-depth models are all published and the site selection included the presence of a minimum of three radiocarbon dates. The radiocarbon dates can be situated within the time interval 1150 to 1850 and/or a larger time interval. In general, the higher the number of dates to build an age-depth model, the better the chronology is. All chronologies are transformed to years CE/BCE for the comparison with the tree-ring data.
The aim of the REVEALS model is to correct the non-linear nature of pollen-vegetation relationships when expressed as percentages, by considering the inter-taxonomic differences in pollen productivity, dispersal, and depositional characteristics. The methodological protocol for running REVEALS follows the ones used in the LANDCLIM I and II projects (Gaillard et al., 2010; Trondman et al., 2015; Marquer et al., 2017; Githumbi et al., 2021). The Relative Pollen Productivity estimates and Fall Speeds of Pollen used for the 25 plant taxa are those of the standard 2 published by Mazier et al. (2012) (Table 1). The spatial extent of the reconstruction (Z max) is fixed at 50 km radius around the central point of each grid cell, mean wind speed is 3 m/s, atmospheric conditions are defined as neutral (no turbulences) and a Gaussian Plume Model (GPM) is used as pollen dispersal model. The pros and cons of using a GPM as dispersal scheme for pollen can be found in Marquer et al. (2020). The resulting REVEALS estimates are the plant abundance of each of the 25 plant taxa expressed as proportion of the total land cover for each grid, the related standard errors are also provided by REVEALS. We used the REVEALS model version LRA.REVEALS.v6.2.4.exe (Sugita, unpublished).
Statistics and Comparative Analysis
The locations of the tree-ring spruce data were mapped using ArcGIS 10.6 (ESRI, 2017). The numbers of the dendrochronologically obtained start and end dates for each timber were aggregated for each year from 1150 to 1850. For the two sub-sets of data, i.e. within and outside the species' distribution limit, significant temporal changes in the number of start and end dates were assessed by using the sequential algorithm developed by Rodionov (2004, 2006), which considers autocorrelation and significant shifts at the 95% significance level. The Regime test shift software (version 6-2) is a macro code which runs in an Excel environment. Shifts, i.e., changes in trends, are identified using a cut-off length of 50 years by excluding the 10 first and last years of each series.
To investigate past forest structures and harvest times, the mean tree age, the average growth rates (AGR), and mean tree diameter were calculated using the tree-ring width information of each timber. It has to be noted that the exact location of the sampling within the stem is unknown in historical material.
For the comparison, REVEALS estimates, as well as start and end dates of the spruce tree-ring data were mapped with a grid resolution of 1° × 1° for 100-year periods from 1150 to 1850 CE. Since the spatial distribution of the REVEALS estimates and tree-ring data do not entirely overlap (see Figure 1), we grouped the data into four larger sub-regions, enabling a direct comparison. For the four sub-regions (Northwest: 7°-10°E, 49°-51°N; Northeast: 10°-13°E, 49°-51°N; Southwest: 7°-10°E, 47°-49°N; Southeast: 10°-13°E, 47°-49°N) and the entire study region, REVEALS land-cover categories have been defined and end dates for the main construction timbers were calculated for 100-year periods beginning at 1,050 CE.
The REVEALS land-cover categories are spruce (Picea), other conifers (Abies, Pinus and Juniperus), broadleaves (Alnus, Betula, Carpinus, Corylus, Fagus, Fraxinus, Quercus, Salix, Tilia, Ulmus), open land (Artemisia, Calluna, Cyperaceae, Filipendula, Gramineae, Plantago lancelota, Plantago media, Plantago montana, Rumex acetosa) and arable land (Cerealia, Secale). We use the pollen types Picea, as it is not possible to identify the different species within this genus based on pollen morphology. However, as Picea abies is the only species present in our study region, it can be assumed that the pollen type Picea refers to Picea abies. Regarding tree-ring data, while spruce was kept separately, we developed the category conifers comprising of fir and pine and broadleaved species which includes predominantly oak and a few beech samples.
Results
Spatiotemporal Variations in Spruce Construction Timber
Within the study area, the majority of spruce tree-ring samples were obtained for southern Germany (southern Baden-Württemberg and Bavaria), and northern Switzerland with clusters around Basel, Zurich and Lake Constance, and north-eastern Switzerland (Figure 1A). The number of spruce timbers used for buildings slightly increased from the beginning of the 15th century, in particular outside its natural range, and remained stable until around the 1520s (Figures 1B,C). A new increase in the spruce utilization followed until the Thirty years' war (1618–1648), after which spruce as construction material was highly abundant. Generally, a higher number of spruce samples were recorded outside compared to within its natural distribution range. The general trends in construction activity are similar and the number of end dates lag by a few decades the number of start dates (Figures 1B,C). Following the period after the Black Death (1347–1351), an increase in the establishment of spruce can be observed particularly outside its natural distribution, which lasted about 50 years. An increase in spruce establishment occurred after ca. 1450, which remained high outside the species' distribution until the 1600s (Figure 1B). During the Thirty years' war, a reforestation occurred resulting in an increase in the spruce establishment across the entire study region.
Comparing the different species used in constructions from 1150 to 1850, spruce dominated the entire dendrochronological dataset with 40.9% (Figure 1D). Other frequently used species for construction in southern Germany and northern Switzerland were oak and fir with almost identical proportions of 24.3 and 24%, respectively, followed by pine (10.7%) and only few beech timbers (0.1%). Conifer wood (75.6%) clearly outweighed deciduous species (24.4%) in construction timber throughout the study period.
Spatiotemporal Variations in Spruce Timber Quality
For the entire study region, the utilized timber showed similar median (maximum) ages throughout the period 1150 to 1850 ranging from 70 (150) years during the period 1150–1250 to 62 (120) years during the period 1750–1850 (Figure 2A). For the period 1350–1450, the youngest spruce trees with a median (maximum) age of 55 (98) years were harvested. During this period, also the highest mean AGR (2.2 mm/year) was observed, whereas growth rates were otherwise constant from 1250 onwards (mean AGR during 1250–1850 is 1.9 mm/year) (Figure 2B). Only during the period 1150–1250, spruce grew on average 1.2 mm per year. Also, mean stem diameters are lowest (18.5 cm) during that early but low replicated period (Figure 2C). A general decreasing trend in median (maximum) stem diameter is found from 25.1 (44.5) cm during 1250–1350 to 22.7 (37.4) cm during 1750–1850.
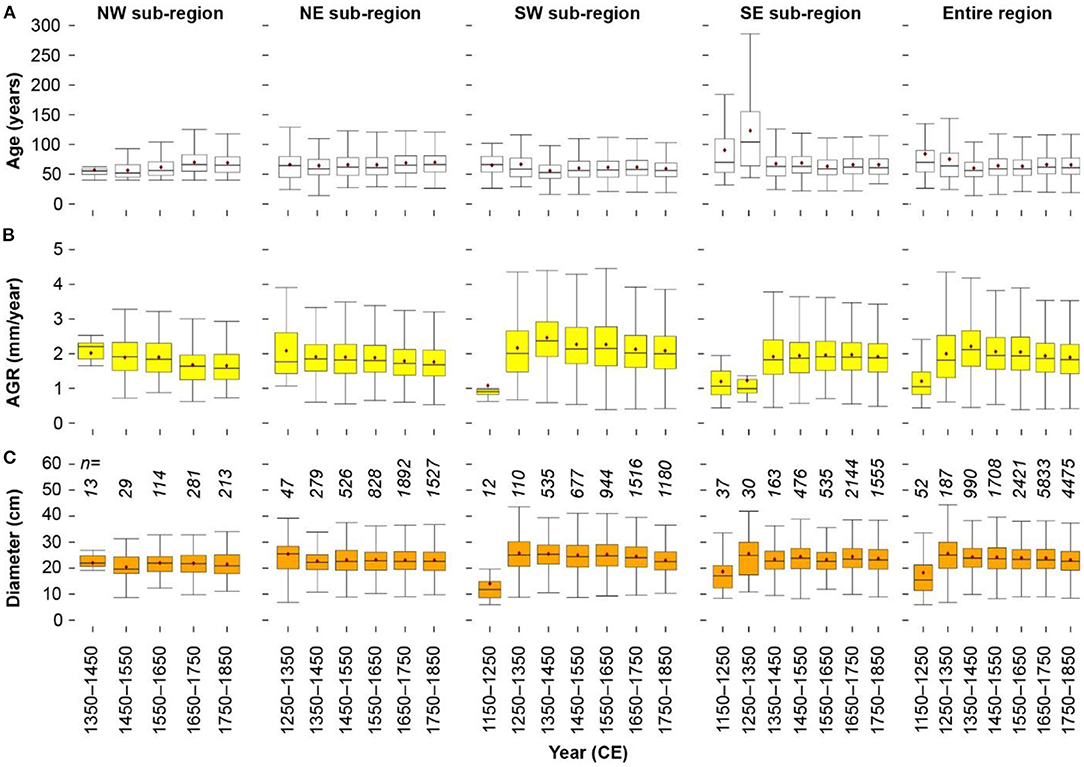
Figure 2. Temporal distribution of (A) tree age, (B) average growth rates (AGR), and (C) tree diameter for the dendrochronologically dated spruce timber shown for 100-year periods and for the four sub-regions [northwest (NW), northeast (NE), southwest (SW) and southeast (SE)] and the entire study region. Numbers of spruce samples for each 100-year period is indicated by numbers (in italic) in (C).
There are regional differences in mean tree age, AGR and mean tree diameter across the sub-regions and periods. For the north-western sub-region, except for the early 1350–1450 period, mean tree ages likely increased from 58 years from 1450–1550 to about 70 years during 1650–1850. This coincided with a general decreasing trend in mean AGR from 2 (1350–1450) to 1.65 mm/year (1750–1850), whereas the mean stem diameters remained at a constant level of about 21.6 cm (mean for 1350–1850).
For the north-eastern sub-region, mean tree ages remained stable during the period 1250–1850 with lowest ages during 1350–1450 (median of 56 years, maximum of 98 years) and highest ages during 1750–1850 (median of 71 years, maximum of 134 years). At the same time, mean (to a lesser extent also median) AGR values are slightly declining from 2.1 mm/year (1250–1350) to 1.8 mm/year (1750–1850). Median (maximum) stem diameters are constant throughout at ca. 22 (36) cm except for the early 1250–1350 period (median of 25.4 cm).
Similar trends as for the entire region are observed for the south-western sub-region. Here too, median (and maximum) ages for the spruce timbers were found to be declining from the period 1250–1350 (median 60 years, maximum 133 years) toward the period 1750–1850 (median of 56 years, maximum of 99 years). Mean AGR is increasing from the period 1150–1250 (1.1 mm/year) to the period 1350–1450 (2.5 mm/year) and subsequently declining until 1750–1850 (2.1 mm/year). Except for the period 1150–1250, mean stem diameters become smaller from the period 1250–1350 (26 cm) to the period 1750–1850 (23 cm).
For the south-eastern sub-region, except for the early periods from 1150 to 1350 where an increase in mean (median) age of up 124 (108) years, a comparable low mean (median) AGR of 1.2 (1) mm/year, and increasing mean (median) stem diameters of 25.7 (25) cm are observed, the timber properties barely vary until 1850 (mean age = 66 years, mean AGR = 1.9 mm/year, mean stem diameter = 23.8 cm) (Figures 2A–C).
Temporal Changes in Spruce Cover and Building Activities
REVEALS estimates for spruce and the main land cover categories are compared to spruce utilization (i.e., end dates of the dated timber) for the study area and four sub-regions from 1050 to 1950 (Figure 3). Regarding the entire study region, spruce abundance varies between 10 and 15% of the total regional land cover from 1050 onwards, whereas an increase in the last 200 years is observed (Figure 3B). At the same time, abundances of other coniferous and broadleaved trees show a declining trend from almost 60% to ca. 35% while areas of open land increased from ca. 30 to 50% (Figure 3B). Arable land varied slightly over time and decreased between 1850 and 1950. Regarding the tree-ring data, an increase in the number of end dates is observed for all species from 1250 onwards, whereas spruce accounts for almost half of all timber. A strong increase in the number of timbers is found from 1650 onwards.
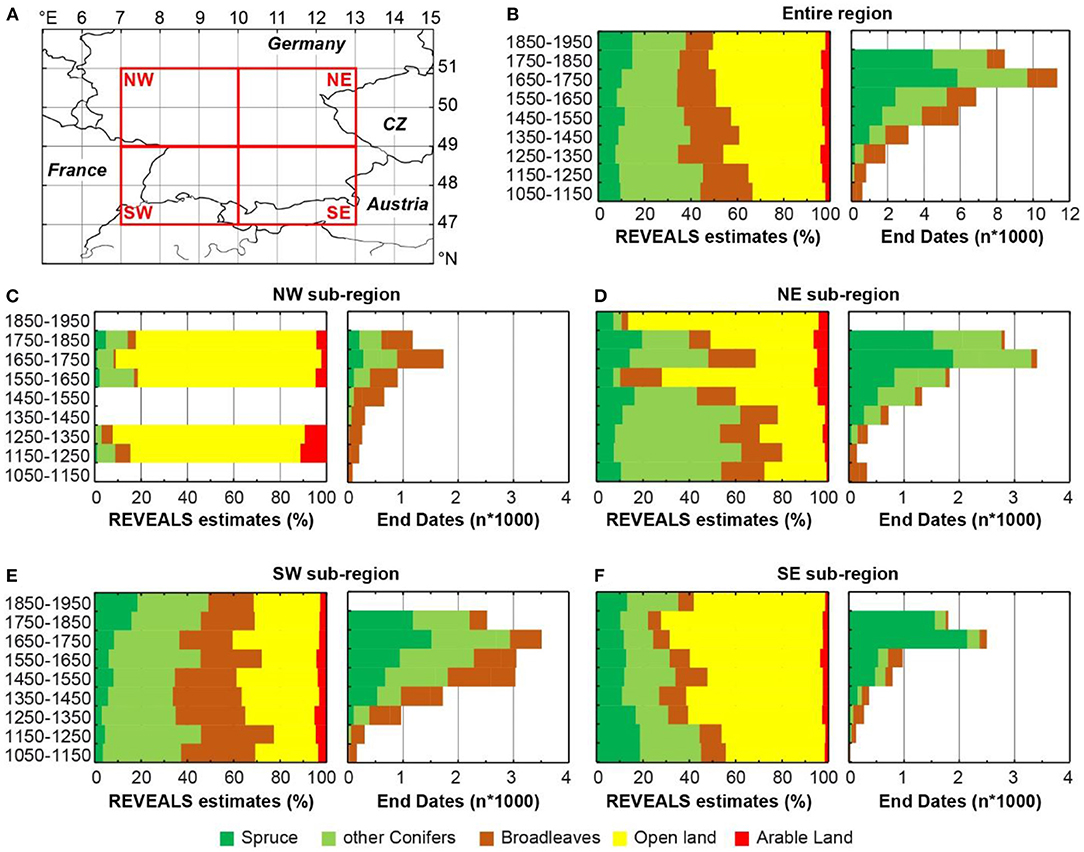
Figure 3. (A) Study area and its four sub-regions [northwest (NW), northeast (NE), southwest (SW) and southeast (SE)] for which (B–F) REVEALS estimates for spruce (left panels) are compared to the number of end dates from dendrochronologically dated spruce timber (right panels). REVEALS estimates for other coniferous and broadleaved plant covers, open land and arable land are also shown.
Taking regional variations into account, different trends can be observed for the four sub-regions (Figures 3C–F). For the north-western sub-region, spruce abundance and spruce timber was not found prior to 1550 (Figure 3C), whereas increasing building activity was recorded from 1250 onwards. Other coniferous and broadleaved species were used as building material in this region which is generally dominated by open and agricultural land cover.
The north-eastern sub-region displays strong changes in the vegetation cover from over 70% forest cover during 1050–1150 to ca. 80% open land during 1850–1950 (Figure 3D). Only during the period 1550–1650, the open land vegetation has almost the same extent as during the most recent period with ca. 60%. Agricultural activity showed a steady increase until 1750–1850 and decreased to ca. 5% during 1850–1950. Spruce timber was first used during the 1250–1350 period in low quantities (47 samples), followed by a continuous utilization as construction timber in buildings until 1850. After the Thirty years' war, the spruce consumption almost doubled, whereas spruce timber accounted for circa half of all other tree species.
In the south-western sub-region of the study area including the Black Forest and northern Switzerland, the forest cover remained rather stable over time with its lowest extent during 1650–1750 (Figure 3E). The forest species composition changed, however, and the abundance of deciduous trees declined from ca. 30% during 1050–1150 to 20% during 1850–1950 while conifers increased from ca. 37 to 49% at the same time. The extent of the open and agricultural areas varied only slightly. The abundance of spruce doubled from 1650–1750 to 1850–1950. The amount of spruce among construction timber was low in the 1250–1350 period but sharply increased already during the 1350–1450 period. Spruce made up ca. 30% of all construction timber until 1650–1750 and ca. half of it during the 1750–1850 period.
For the south-eastern sub-region, a declining trend in spruce cover was found from ca. 19% during 1050–1150 to around 13% during 1850–1950, which was accompanied by a decrease of the other coniferous and broadleaved trees, though less evenly, and an increasing trend in the extend of open land (Figure 3F). An increase in open land appeared to be severe particularly during 1250–1350, 1650–1750, and 1750–1850. From 1650 onwards, a sudden increase in spruce consumption is found in the tree-ring data, which accounted for ca. 85% of all timber since then.
Spatiotemporal Distribution of Spruce Abundances in Land Cover and Constructions
Gridded maps for seven 100-year periods from 1150 to 1850 show the abundance of spruce cover in comparison to arable land, the establishment of spruce trees in forests based on start dates, and the utilization of spruce trees for construction based on end dates (Figure 4).
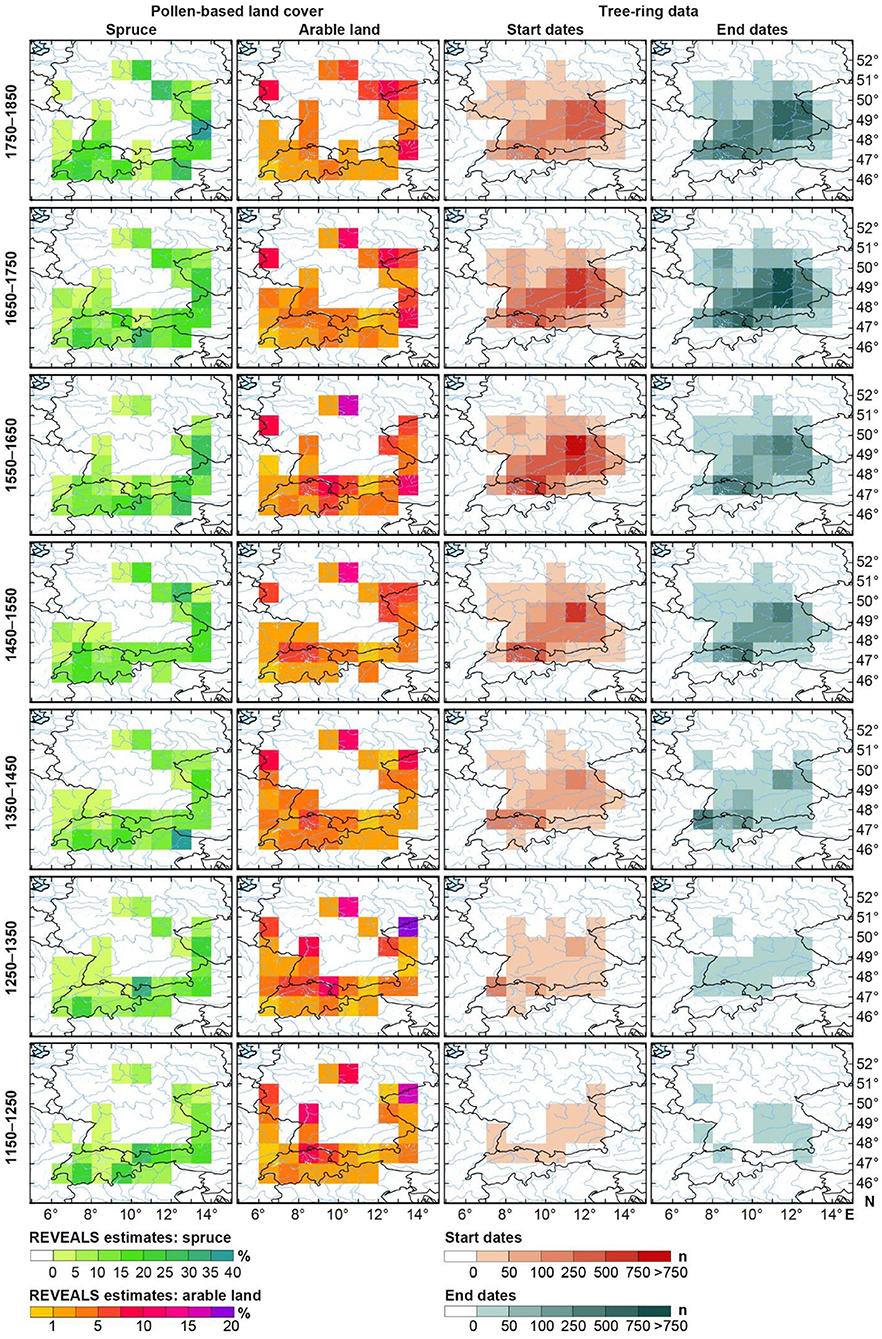
Figure 4. Gridded maps for seven 100-year periods from 1150 to 1850 showing regional abundance of spruce (REVEALS, left) in comparison to the regional abundance of arable land (REVEALS, second left), and the establishment of spruce trees in forests based on start dates (second right) and its utilization in buildings based on end dates (right).
In the early period 1150–1250, spruce was highly abundant with a coverage of 30 to 40 % in Switzerland and Austria, and in the Bavarian Forest, whereas arable land was highest in northern Switzerland (northern region close to Lake Constance), northern Black Forest, Harz mountain and eastern Germany/western Czech Republic (Figure 4). Spruce was already established in low quantities in the lowlands of the Alpine foothills, northern Switzerland and Bavarian Forest which was consequently found in building material in these regions.
In the period 1250–1350, while the arable land cover further increased across the study region, spruce started growing in higher abundances in northern Switzerland and southern Germany, in particular around Basel, Lake Constance and central Bavaria (Nuremberg). An increase in start dates are detected in these regions during the period 1350–1450, which is then followed by an increased use of spruce timbers for construction purposes in the subsequent investigation period.
Spruce cover increased to levels of ca. 25% locally in the north-eastern part of the study area during the period 1450–1550, while at the same time, arable land increased in most areas. Tree-ring data for spruce for this period are most abundant in northern Switzerland and in Bavaria with the highest concentrations found around Nuremberg and the Lake of Constance.
During the period 1550–1650, spruce cover increased in the southern and eastern grid cells, particularly in northern Italy and around the Bavarian Forest, for example, whereas arable land remained high but showed regional variations. At the same time, spruce establishments and tree fellings further increased and expanded toward central and western Germany.
During the periods 1650–1750 and 1750–1850, the abundance of spruce continued to be high across the study region, which is accompanied by a high intensity of land use, i.e., arable land, except in the Alpine region. The numbers of newly established and harvested spruce remained high especially in Germany (south of 50°N) and northern Switzerland.
Discussion
Tree-Ring Data
The majority of the studied timber are from historical buildings of the last millennium which are still standing. Roofs and half-timbered constructions dating prior to 1200 are rarely preserved, since older buildings or parts thereof were constantly replaced by younger constructions in the course of the centuries. Many of these simpler constructions, often made entirely from wood, were replaced during different waves of urban and rural development. Most of our data originate from roof framings and half-timbered constructions with only a few samples from foundations of buildings and infrastructures, which were excavated from archaeological contexts. Since different parts of timber constructions require different physical properties of the utilized species, we observe a selection of decay-resistant oak timber for structures in moist conditions (e.g., foundations) compared to the use of the lighter conifers for roof framings. In the upper floors and roofs of buildings, oak, if present, was only used for short structural elements, whereas the long-fibred conifers were preferred for the long structural beams often exposed to bending forces (Marstaller, 2012). In particular, the long straight stem growth of fir and spruce, combined with the relatively low gross density (i.e., low weight), make them ideal construction material for larger buildings, especially roofs and rooms of an unprecedented widths; likely causing a change from the basilica style to hall constructions for the larger churches during the late 15th century (Marstaller, 2012).
Our dataset captures the most important timber species on an evenly high spatial distribution (Figure 1) and, apart from a period of low replication prior to 1300, is representative for the entire study region. The number of spruce timbers used for buildings also reflect the general variations of building activity over time (Ljungqvist et al., 2022). However, it has to be noted that some species might be generally underrepresented in different regions such as beech in southern Germany or spruce in north-western Switzerland due to difficulties in dating. The large amount of tree-ring data also mitigate possible biases concerning correct ages or stem diameters, which is also addressed by Shindo et al. (2022).
Historical records (i.e. written sources) could provide insights into past forest history and composition, however, most available documents do not differentiate between taxa, making it impossible to distinguish between coniferous species (Sperber, 1968). Moreover, they hardly contain information on forest structures and management practices prior to the 16th century (Mantel, 1990). Also, the quantity and quality of historical records is not stable over time and becomes more fragmented for earlier periods (Muigg and Tegel, 2021). Here, tree-ring data provide the respective spatial and temporal coverage, thereby complementing the data from other sources.
REVEALS Reconstructions
The pollen archives used in this study essentially correspond to sediment cores retrieved from lakes, bogs and mires. Such sedimentary basins are not present everywhere, and are not distributed uniformly, as they depend on geomorphology and climate characteristics. Our results clearly show that most of the pollen data come from the Alps and south-western Germany, and a large gap in the data can be observed in south-eastern Germany. Basically, we observe the opposite geographical pattern than with the tree-ring data that are mostly located in the lowlands where the building activities mainly took place. In large urban, agriculture and cultivated areas, potential sedimentary basins for pollen analyses are lacking or have been most of the time disturbed.
The transformation of pollen data into land cover by using the REVEALS modeling scheme provide a spatial scale of pollen reconstructions, i.e., grids of one degree. REVEALS estimates correspond to the regional pattern of land cover change, where a region is represented by one grid cell. On the contrary, the tree-ring data refer to the grouping of local information (each building) for each grid. Consequently, we are focusing in this study on the regional changes in land cover and abundance of spruce timber.
There are pros and cons of using REVEALS at a grid cell scale of 1° × 1°. In particular, some sites located near the border of another grid could be more influenced by the vegetation pattern of the neighboring grid than the one the site is attributed to. This is of little concern when many sites are situated in the grid, large lakes are present and/or when the sites are uniformly distributed within it. However, it could be an issue, if only one small site is located in a corner of a grid. Nevertheless, the use of a grid pattern is generally reliable, in particular because we group many sites per grid (Supplementary Table S1) which reduces the uncertainties in the reconstructions (Trondman et al., 2016; Marquer et al., 2017).
Combination of Tree Rings and REVEALS Reconstructions
By combining tree ring and REVEALS estimates, we are grouping two different proxies at a same temporal and spatial scale. This is possible because we use a grid pattern, covering locations of both pollen and tree-ring data, as well as sub-regions to minimize the effect of the differences in the spatial availability of both proxies, and time slices of 100 years. As mentioned above, REVEALS reconstructions correspond to the regional plant/land cover, i.e., 1° × 1° grids, whereas the grouping of the tree-ring data also provided the regional pattern in building activity using spruce. The exact provenance of the timber from historical buildings is unknown and the material is not always of local/regional origin depending on topography and available transport routes. While large-scale long-distance overland transportation might be excluded due to excessive financial and logistical (infrastructural) expenses, riverine timber transport in form of rafting and log driving was widely used in our study area (Keßler, 1960; Keweloh, 1988; Eißing, 2010). Archaeological finds can trace riverine transport, both on large rivers like Rhine, Main, and Danube and their smaller tributaries, as far back as into Roman times (Ellmers, 1985). Early written evidences for timber rafting is documented for the Rhine river from the 13th century, e.g., 1208 in Koblenz, located at the confluence of Rhine and Moselle river, western Germany (Ellmers, 1985), or for the Lech river in Bavaria, which was used for timber transport from Tyrol, Austria (Filser, 1989). From the 14th century onwards, sophisticated rafting infrastructures on rivers was established in central Europe (Keweloh, 1988). Therefore, it is likely that some of the timber in our dataset have been transported from different locations, e.g. higher altitudes, on established waterways such as the river Rhine, Main, Danube and smaller tributaries thereof. While the proportion of rafted timber remains unknown, it can be assumed that coniferous wood, because of its lower density and better floating properties, was more frequently rafted than hardwood species. There are also regional differences as timber import was very important for urban centers without extensive municipal forest, and for periods of increased demand, e.g., for Augsburg, Bavaria, during the mid-16th century when the population increased (Watanabe, 2017), or for settlements in the Swabian Alb at the beginning 17th century (Marstaller, 2008). Nevertheless, considering the existence of locally, i.e., easily accessible and nearby, exploitable forests in rural areas, large parts of timber, especially in vernacular buildings, were harvested from local woodlands. Depending on terrain, different techniques were applied to transport the felled trees out of the forests (Johann, 2021).
To secure local wood supply and building development, cities placed great importance on the ownership or the right to use forests belonging to the urban municipality and implemented regulations even as early as the 13th century for example for the city forest Sihlwald near Zurich (Irniger, 1991) or the municipal forest of Nuremberg, Bavaria (Mantel, 1968).
For more than 700 years, spruce has been significantly used in Central Europe both within and outside its natural distribution area, and in the foothill zones (mid-elevations; ca. 700 to 900 m asl) and lowland areas (<700 m asl) (Kolár et al., 2021). Although the exact provenance of spruce timber remains unknown, the use of 1° × 1° grids (i.e., respectively covering an area of 100 km2) is likely to reduce this bias; one can assume that in general spruce timber would be collected and used for construction in a same grid.
Spruce History in Central Europe
Spruce became the main timber species in building constructions from the 14th century onwards (Figure 1C). Using the REVEALS reconstructions, which provide quantitative information about the spruce cover, our results show that spruce abundance over the entire study region varies between 10 and 15% from 1050 CE onwards while an increase is observed over the last 300 years. The last two centuries correspond to large scale forest conversion toward spruce monoculture, while other coniferous and broadleaved trees show a decline from almost 60% to ca. 35%. The tree-ring data reveal a strong increase in the number of spruce timbers from 1650 onwards. After the Thirty years' war, open and arable land unused by the reduced population seems to have favored the distribution of spruce in lowland areas. This is particular evident when looking at regional differences within the study area (i.e., the four sub-regions) (Figure 3). For example, the north-eastern sub-region shows comprehensive changes in land cover from ca. 70% forest cover (1050–1150 CE) to ca. 80% of open land (1850–1950). In this region, spruce timber was first moderately used (1250–1350) and then it was continuously utilized as construction timber in buildings until 1850, in particular after the Thirty years' war when the spruce consumption almost doubled. In the south-western sub-region, the forest cover remained rather stable over time, although the abundance of deciduous trees declined from 1050 to 1950 while conifers, except silver fir or yew, increased at the same time. For the south-eastern sub-region, spruce cover was decreasing from 1050 to 1950, which was accompanied by an increase in the extend of open land. On the contrary, spruce consumption increases through time. In the north-western sub-region spruce was generally less used, compared to other coniferous and broadleaved species.
While humans likely modulated the abundance of spruce in our study region, detrimental climate conditions, as they were prevailing during the Little Ice Age, could have a beneficial effect in the expansion of spruce. Since spruce can tolerate colder climate conditions, it can outcompete other species such as fir (Schmidt-Vogt, 1977).
Similar tree ages were obtained for all investigated 100-year periods (except the low replicated early sub-periods) and across the four sub-regions, whereas in most sub-regions a declining mean AGR and stem diameter was found until 1850 (Figure 2). Despite that not all samples retained the piths, the similar temporal distribution of samples with pith and without pith (Supplementary Figure S2) and the aggregation into 100-year periods provides representative insights into timber quality characteristics.
In the 20th century, rotation cycles for spruce range between 80 and 140 years and are scheduled when the economic yield is highest and stems reached target diameters (Faustmann, 1849; Moog and Borchert, 2001; Beinhofer and Knoke, 2007). This is in accordance with our spruce data for the entire study area, when maximum ages are around 150 years during the period 1150–1350, 98 years during 1350–1450, 114 years during the period 1450–1650, and up to 120 years during 1750–1850 (Figure 2). Regional and temporal differences exist reflecting the regional forest structure, which is influenced by environmental factors and site conditions as well as by economic demands due to population increase. For example, our data show that in early periods, old spruce forest stands were available in the south-western region including the Black Forest. In comparison, relatively young spruce trees were used during the construction boom in the period 1350–1450 in the south-eastern region, likely indicating a high demand for constructions and thus, shorter harvest times. In the north-western region, older trees were available after the Thirty years' war (Figure 2). The changes in growth rates can likely be related to changes in exploitation of the regional forests.
Mean stem diameters show a slightly decreasing trend from around 30 cm (75% of all data) and a maximum of 44 cm during 1250–1350 to 26 cm (3rd Quartile) toward the most recent period 1750–1850, which is accompanied by a decrease in AGR. The decrease in AGR is likely a result of changing forest management, e.g., forests with higher stand density, or the intense practice of litter raking which caused the soil conditions to deteriorate and which was practiced until the 20th century (Bürgi et al., 2013; Vild et al., 2018). Here, clear-cutting further depleted the soils of humus and nutrients and in addition with forest grazing and game browsing led to a large-scale change in tree species, from hardwoods to spruce and pine.
Since spruce does not play a significant role in traditional forestry by supplying by-products such as fruits for pig masting or leaves for leaf litter raking (Stuber and Bürgi, 2002), its economic importance is attributed to its fast growth, straight stem and favorable mechanical properties for its use as timber.
Considering their use as timber, trees had to have a certain size and age. Therefore, it is likely that the trees come either from high forests or from the canopy layer of coppice-with-standards (CWS) forests. Especially for northern Switzerland, spruce, alongside oak, played an important role as dominant standards in this CWS forest management type (Bader et al., 2015). CWS forest structures allowed to simultaneously generate different wood products, from timber to fuelwood, which was important for subsistence economies, and found to have been applied in the Early Middle Ages and likely before (Muigg et al., 2020). Spruce can rejuvenate well in the understorey of sparse oak-dominated CWS forests. The observed increase in spruce abundance from the mid-14th century might be linked to an outgrowing of spruce over its competitors and a selective cutting of dense forests dominated by oak, beech or fir, and/or the growing promotion of the species for timber in evolving timber-framed architecture. Moreover, oak coppice was intensively extracted for fuelwood and tanning, whereas spruce was spared for the production of quality timber, forming soon upper forest layers and even dominant stands (Schmidt-Vogt, 1977). This development might have also favored the high abundance of spruce in subsequently formed CWS stands (Bader et al., 2015).
On the other hand, the 14th century was a period of desertification in which cultivated areas, i.e., arable and open land, were abandoned (Figures 3B, 4 period 1350–1450) (Abel, 1976). The more tolerant spruce was able to grow on nutrient depleted open lands and could establish forests. Lindbladh et al. (2014) described the processes when the agricultural practice of slash-and-burn cultivation (i.e., the land underwent a rotation of clear cutting, farming, grazing and natural forest regeneration) ceased in southern Sweden supporting the natural spread of spruce without active forest management. Especially, the removal of grazing pressure on spruce seedlings and the selection of species, contributed to the establishment of spruce forests (Lindbladh et al., 2014). This slash-and-burn management practice as well as other forms of agro-forestry were common across the study area (Mantel, 1968). During the early modern period (16th century), wood consumption intensified due to the development of large-scale consumers for various products (i.e., salt extraction, glass production and mining), resulting in severe forest degeneration. The 16th century was known as the era of forest regulations (Hasel, 1985), which subsequently led to a strong change in the forest composition thereby favoring spruce in the study area until present-day.
Today, however, spruce is confronted with multiple problems, including drought extremes, subsequent bark beetle infestations and large-scale dieback (Marini et al., 2017; Hlásny et al., 2021). This led to urging questions about the suitability of spruce in modern and future forests in the light of climate change. For this reason, spruce is now at a crossroad as to whether it is still suitable for future European forestry. More structured and mixed forest stands might hold an opportunity for this species, that has been crucially important for centuries. Additionally, such multi-aged and mixed forest stands are urgently needed for the future promotion of biodiversity (Oettel and Lapin, 2021).
Conclusion
Throughout the last millennium spruce was commonly used as timber in southern Germany and northern Switzerland, both within and outside its natural distribution area and for a region with regionally differing political and demographic histories. By investigating more than 38000 dendrochronological samples, coniferous wood (75.6%) is the dominating timber in constructions compared to hardwood species (24.4%). From this, spruce is the most abundant species (40.9%), followed by fir (24%) and pine (10.7%). We combined pollen and tree-ring data to investigate the abundance of spruce in the land cover in tandem with the utilization of spruce timbers in historical buildings at regional scale. It was shown that the spatial coverage of pollen and tree-ring data in some parts of the study area are still insufficient, thus (i) highlighting the complementarity of both archives to study the history of spruce in Central Europe and (ii) by developing sub-regions we could gain insights into the regional spruce abundance and utilization.
Besides identifying differences in the abundance of spruce across regions and during the past millennia we found an intense use and afforestation of spruce from the 1650s onwards.
Regarding future forestry, we highlight that spruce, long before its extensive monocultured planting, was valued as an important timber and should be included to generate diverse and resistant forests.
Data Availability Statement
The original contributions presented in the study are included in the article/Supplementary Material, further inquiries can be directed to the corresponding author.
Author Contributions
AS and LM designed the study and set up the experiments. LM and UB ran the REVEALS model. JH, FH, RK, BM, MN-E, AR, FW, JW, and WT provided tree-ring data. JL and MR generated the pollen data. All authors have contributed to the writing of the manuscript and approved the final version.
Funding
AS was funded by the German Research Foundation (DFG, SE 2802/1-1) and the Swedish Research Council (Vetenskapsrådet, grant no. 2018-01272). The article processing charge was funded by the Baden-Wuerttemberg Ministry of Science, Research and Art and the University of Freiburg in the funding program Open Access Publishing.
Conflict of Interest
The authors declare that the research was conducted in the absence of any commercial or financial relationships that could be construed as a potential conflict of interest.
Publisher's Note
All claims expressed in this article are solely those of the authors and do not necessarily represent those of their affiliated organizations, or those of the publisher, the editors and the reviewers. Any product that may be evaluated in this article, or claim that may be made by its manufacturer, is not guaranteed or endorsed by the publisher.
Acknowledgments
We thank Cheyenne Petinaud (University of Freiburg) for English editing and the two reviewers for valuable comments on an earlier version of the manuscript.
Supplementary Material
The Supplementary Material for this article can be found online at: https://www.frontiersin.org/articles/10.3389/fevo.2022.909453/full#supplementary-material
References
Abel, W. (1976). “Die Wüstungen des ausgehenden Mittelalters,” in Quellen und Forschungen zur Agrargeschichte, Bd. 1. (Stuttgart: De Gruyter Oldenbourg). doi: 10.1515/9783110506761
Bader, M., Gimmi, U., and Bürgi, M. (2015). Die Zürcher Wälder um 1823–Betriebsformen und Baumarten. Schweizerische Zeitschrift fur Forstwesen. 166, 24–31. doi: 10.3188/szf.2015.0024
Beinhofer, B., and Knoke, T. (2007). Umtriebszeit und Risiko der Fichte. Umweltvorsorge. Allg. Forst Z: Waldwirtsch. p. 110–113.
Björkman, L. (1996). The Late Holocene history of beech Fagus sylvatica and Norway spruce Picea abies at stand-scale in southern Sweden (Doctoral Dissertation). Lund University, Lund, Sweden.
Burga, C. A., and Hussendörfer, E. (2001). Vegetation history of Abies alba Mill. (silver fir) in Switzerland–pollen analytical and genetic surveys related to aspects of vegetation history of Picea abies (L.) H. Karsten (Norway spruce). Veg Hist Archaeobot. 10, 151–159. doi: 10.1007/PL00006927
Bürgi, M., Gimmi, U., and Stuber, M. (2013). Assessing traditional knowledge on forest uses to understand forest ecosystem dynamics. For. Ecol. Manage. 289:115–122. doi: 10.1016/j.foreco.2012.10.012
Carter, V. A., Chiverrell, R. C., Clear, J. L., Kuosmanen, N., Moravcová, A., Svoboda, M., et al. (2018). Quantitative Palynology Informing Conservation Ecology in the Bohemian/Bavarian Forests of Central Europe. Front. Plant Sci. 8:2268. doi: 10.3389/fpls.2017.02268
Caudullo, G., Tinner, W., and de Rigo, D. (2016). Picea abies in Europe: Distribution, Habitat, Usage and Threats. Luxembourg: Publ. Off. EU.
Eißing, T. (2010). “Zur Flößerei auf Rhein und Main.” Der Rheingau und seine historischen Häuser: zur Flößerei auf Rhein und Main/Klaus Freckmann; Burghart Schmidt (Hrsg.). Marburg, 2010, Schriftenreihe zur Dendrochronologie und Bauforschung (Marburg), p.154–178.
Ellmers, D. (1985). Flößerei in Vorgeschichte, Römerzeit und Mittelalter. Pages 12–33 in. Flösserei in Deutschland, Stuttgart.
Enderle, R., Bräsicke, N., and Berendes, K. H. (2021). Herausforderungen in der Waldschutzforschung: Ein forstentomologischer und-pathologischer Rück-und Ausblick. Journal für Kulturpflanzen. 261–269. doi: 10.5073/JfK.2021.07-08.10
Faustmann, M. (1849). Berechnung des Werthes, welchen Waldboden, sowie noch nicht haubare Holzbestande fur die Waldwirthschaft besitzen [Calculation of the value which forest land and immature stands possess for forestry]. Allgemeine Forst- und Jagd-Zeitung. 25, 441–455.
Filser, K. (1989). Die Rolle der Lech- und Illerflößerei im Handelsverkehr zwischen Tirol und Schwaben. Schwaben - Tirol: historische Beziehungen zwischen Schwaben und Tirol von der Römerzeit bis zur Gegenwart; Ausstellung der Stadt Augsburg und des Bezirks Schwaben, Augsburg, Zeughaus, Toskanische Säulenhalle. Band. 2, 233–239.
Gaillard, M.-J., Sugita, S., Mazier, F., Trondman, A.-K., Broström, A., Hickler, T., et al. (2010). Holocene land-cover reconstructions for studies on land cover-climate feedbacks. Climate of the Past. 6, 483–499. doi: 10.5194/cp-6-483-2010
Giesecke, T., and Bennett, K. D. (2004). The Holocene spread of Picea abies (L.) Karst. in Fennoscandia and adjacent areas. J. Biogeography. 31, 1523–1548. doi: 10.1111/j.1365-2699.2004.01095.x
Giesecke, T., Brewer, S., Finsinger, W., Leydet, M., and Bradshaw, R. H. W. (2017). Patterns and dynamics of European vegetation change over the last 15,000 years. J. Biogeogr. 44, 1441–1456. doi: 10.1111/jbi.12974
Githumbi, E., Fyfe, R., Gaillard, M.-J., Trondman, A.-K., Mazier, F., Nielsen, A.-B., et al. (2021). European pollen-based REVEALS land-cover reconstructions for the Holocene: methodology, mapping and potentials. Earth Syst. Sci. Data Discuss. 1–61. doi: 10.5194/essd-2021-269
Hlásny, T., König, L., Krokene, P., Lindner, M., Montagné-Huck, C., Müller, J., et al. (2021). Bark beetle outbreaks in Europe: state of knowledge and ways forward for management. Curr. Forestry Rep. 7, 138–165. doi: 10.1007/s40725-021-00142-x
Huntley, B., and Birks, H. J. B. (1983). Atlas of Past and Present Pollen Maps for Europe, 0-13,000 Years Ago. Cambridge: Cambridge University Press.
Irniger, M. (1991). Der Sihlwald und sein Umland: Waldnutzung, Viehzucht und Ackerbau im Albisgebiet von 1400-1600. Zurich: Hans Rohr.
Jansen, S., Konrad, H., and Geburek, T. (2017). The extent of historic translocation of Norway spruce forest reproductive material in Europe. Ann. For. Sci. 74. doi: 10.1007/s13595-017-0644-z
Jansson, G., Danusevičius, D., Grotehusman, H., Kowalczyk, J., Krajmerova, D., Skrøppa, T., et al. (2013). Norway Spruce (Picea abies (L.) H.Karst.). in Forest tree breeding in Europe. Current state-of-the-art and perspectives, Pâques L. E., (ed). Dordrecht, New York: Springer. doi: 10.1007/978-94-007-6146-9_3
Johann, E. (2021). Transportation of wood out of the forest (along Short Distances). Int. J. Wood Culture. 1, 80–111. doi: 10.1163/27723194-20210008
Kalis, A. J. (1984). L'indigenat de l'Epicea dans les Hautes Vosges. Revue de Paléobiologie vol sp Avril. p. 103–115.
Keweloh, H.-W. (1988). Auf den Spuren der Flößer. Wirtschafts-und Sozialgeschichte eines Gewerbes. Stuttgart. p. 286.
Knopf, T., Fischer, E., Kämpf, L., Wagner, H., Wick, L., Duprat-Qualid, F., et al. (2019). Archäologische und naturwissenschaftliche Untersuchungen zur Landnutzungsgeschichte des Südschwarzwalds. Fundberichte aus Baden-Württemberg. 39, 19–101.
Kolár, T., Dobrovolný, P., Szabó, P., Mikita, T., Kyncl, T., Kyncl, J., et al. (2021). Wood species utilization for timber constructions in the Czech lands over the period 1400–1900. Dendrochronologia. 70:125900. doi: 10.1016/j.dendro.2021.125900
Lang, G. (1994). Quartäre Vegetationsgeschichte Europas: Methoden und Ergebnisse. Jena, Stuttgart, New York: Gustav Fischer Verlag.
Latałowa, M., and van der Knaap, W. O. (2006). Late Quaternary expansion of Norway spruce Picea abies (L.) Karst. in Europe according to pollen data. Quat. Sci. Rev. 25, 2780–2805. doi: 10.1016/j.quascirev.2006.06.007
Lindbladh, M., Axelsson, A.-L., Hultberg, T., Brunet, J., and Felton, A. (2014). From broadleaves to spruce – the borealization of southern Sweden. Scand. J. For. Res. 29:686–696. doi: 10.1080/02827581.2014.960893
Ljungqvist, F. C., Seim, A., Tegel, W., Krusic, P. J., Baittinger, C., Belingard, C., et al. (2022). Regional patterns of late medieval and early modern european building activity revealed by felling dates. Front. Ecol. Evol. 9. doi: 10.3389/fevo.2021.825751
Mantel, K. (1968). Die Anfänge der Waldpflege und Forstkultur im Mittelalter unter der Einwirkung der lokalen Waldordnung in Deutschland. Forstwissenschaftliches Centralblatt. 87, 75–100. doi: 10.1007/BF02735854
Marini, L., Økland, B., Jönsson, A. M., Bentz, B., Carroll, A., Forster, B., et al. (2017). Climate drivers of bark beetle outbreak dynamics in Norway spruce forests. Ecography. 40:1426–1435. doi: 10.1111/ecog.02769
Marquer, L., Gaillard, M.-J., Sugita, S., Poska, A., Trondman, A.-K., Mazier, F., et al. (2017). Quantifying the effects of land use and climate on Holocene vegetation in Europe. Quat. Sci. Rev. 171, 20–37. doi: 10.1016/j.quascirev.2017.07.001
Marquer, L., Gaillard, M. J., Sugita, S., Trondman, A. K., Mazier, F., Nielsen, A. B., et al. (2014). Holocene changes in vegetation composition in northern Europe: why quantitative pollen-based vegetation reconstructions matter. Quat. Sci. Rev. 90, 199–216. doi: 10.1016/j.quascirev.2014.02.013
Marquer, L., Mazier, F., Sugita, S., Galop, D., Houet, T., Faure, E., et al. (2020). Reply to Theuerkauf and Couwenberg.2020. comment on: “Pollen-based reconstruction of Holocene land-cover in mountain regions: evaluation of the Landscape Reconstruction Algorithm in the Vicdessos valley, Northern Pyrenees, France”. Quat. Sci. Rev. 244, 106462. doi: 10.1016/j.quascirev.2020.106462
Marstaller, T. (2008). Der Wald im Haus. Zum Wechselspiel von Holzressourcen und Hausbau. Das Mittelalter. 13. doi: 10.1524/mial.2008.0017
Marstaller, T. (2012). Zu Lande und zu Wasser. Bauholzimporte des 12.−17. Jahrhunderts im mittleren Neckarraum. Mitteilungen der Deutschen Gesellschaft für Archäologie des Mittelalters und der Neuzeit. 24, 61–70. doi: 10.11588/dgamn.2012.1.17139
Mazier, F., Gaillard, M.-J., Kuneš, P., Sugita, S., Trondman, A-K., and Broström, A. (2012). Testing the effect of site selection and parameter setting on REVEALS-model estimates of plant abundance using the Czech Quaternary Palynological Database. Rev. Palaeobot. Palynol. 187, 38–49. doi: 10.1016/j.revpalbo.2012.07.017
Moog, M., and Borchert, H. (2001). Increasing rotation periods during a time of decreasing profitability of forestry—a paradox? Forest Policy Econ. 2, 101–116. doi: 10.1016/S1389-9341(01)00047-8
Muigg, B., Skiadaresis, G., Tegel, W., Herzig, F., Krusic, P. J., Schmidt, U. E., et al. (2020). Tree rings reveal signs of Europe's sustainable forest management long before the first historical evidence. Sci. Rep. 10:1–11. doi: 10.1038/s41598-020-78933-8
Muigg, B., and Tegel, W. (2021). Forest history – new perspectives for an old discipline. Front. Ecol. Evolut. 9, 724775. doi: 10.3389/fevo.2021.724775
Oettel, J., and Lapin, K. (2021). Linking forest management and biodiversity indicators to strengthen sustainable forest management in Europe. Ecol. Indic. 122, 107275. doi: 10.1016/j.ecolind.2020.107275
Rodionov, S. N. (2006). Use of prewhitening in climate regime shift detection. Geophys. Res. Lett. 33, L12707. doi: 10.1029/2006GL025904
Rodionov, S. N. (2004). A sequential algorithm for testing climate regime shifts. Geophys. Res. Lett. 31, L09204. doi: 10.1029/2004GL019448
Rösch, M. (2012). Vegetation und Waldnutzung im Nordschwarzwald während sechs Jahrtausenden anhand von Profundalkernen aus dem Herrenwieser See. Standort Wald. Mitteilungen des Vereins für forstliche Standortskunde und Forstpflanzenzüchtung. 47, 43–64.
Schmidt, U.-E. (2017). Die Fichte in der Wald- und Forstgeschichte - eine soziokulturelle Betrachtung. LWF Wissen. 80, 35–41. Available online at: https://www.lwf.bayern.de/wissenstransfer/forstliche-informationsarbeit/172835/index.php
Schmidt-Vogt, H. (1977). Die Fichte, Band I. Taxonomie. Verbreitung. Morphologie. Ökologie. Waldgesellschaft. Hamburg und Berlin: Verlag Paul Parey. p. 647.
Seppä, H., Alenius, T., Bradshaw, R. H., Giesecke, T., Heikkilä, M., and Muukkonen, P. (2009). Invasion of Norway spruce (Picea abies) and the rise of the boreal ecosystem in Fennoscandia. J. Ecol. 97, 629–640. doi: 10.1111/j.1365-2745.2009.01505.x
Shindo, L., Blondel, F., and Labbas, V. (2022). Forest exploitation and wood supply: a dendroarchaeological approach between the massif central and the southern alps since the middle ages. Forests. 13, 275. doi: 10.3390/f13020275
Sperber, G. (1968). Die Reichswälder bei Nürnberg. Aus der Geschichte des ältesten Kunstforstes. München: Mitt. Staatsforstverwalt. Bay. p. 37.
Spiecker, H. (2003). Silvicultural management in maintaining biodiversity and resistance of forests in Europe—temperate zone. J. Environ. Manage. 67:55–65. doi: 10.1016/S0301-4797(02)00188-3
Stuber, M., and Bürgi, M. (2002). Agrarische Waldnutzungen in der Schweiz 1800–1950. Nadel-und Laubstreue| Agricultural use of forests in Switzerland 1800–1950. Needles and leaves for litter harvesting. Schweizerische Zeitschrift fur Forstwesen. 153, 397–410. doi: 10.3188/szf.2002.0397
Sudhaus, D. (2005). Paläoökologische Untersuchungen zur spätglazialen und holozänen Landschaftsgenese des Ostschwarzwaldes im Vergleich mit den Buntsandsteinvogesen. Freiburg i.Br: Freiburger Geographische Hefte 64.
Sugita, S. (2007). Theory of quantitative reconstruction of vegetation I: pollen from large sites REVEALS regional vegetation composition. The Holocene. 17, 229–241. doi: 10.1177/0959683607075837
Trondman, A.-K., Gaillard, M.-J., Mazier, F., Sugita, S., Fyfe, R., Nielsen, A. B., et al. (2015). Pollen-based quantitative reconstructions of Holocene regional vegetation cover (plant-functional types and land-cover types) in Europe suitable for climate modelling. Glob. Chang. Biol. 21, 676–697. doi: 10.1111/gcb.12737
Trondman, A.-K., Gaillard, M.-J., Sugita, S., Björkman, L., Greisman, A., Hultberg, T., et al. (2016). Are pollen records from small sites appropriate for REVEALS model-based quantitative reconstructions of past regional vegetation? An empirical test in southern Sweden. Veg. Hist. Archaeobot. 25:131–151. doi: 10.1007/s00334-015-0536-9
Vild, O., Šipoš, J., Szabó, P., Macek, M., Chudomelová, M., Kopecký, M., et al. (2018). Legacy of historical litter raking in temperate forest plant communities. J. Vegetat. Sci. 29, 596–606. doi: 10.1111/jvs.12642
Warde, P. (2006). Fear of wood shortage and the reality of the Woodland in Europe, c.1450-1850. Hist Workshop J. 62, 28–57. doi: 10.1093/hwj/dbl009
Watanabe, Y. (2017). Waldpolitik und Holzversorgung der Reichsstadt Augsburg im 16. Jahrhundert. Dissertation, Universität Augsburg. p. 274.
Keywords: historical forest utilization, construction timber, Picea abies, pollen-based land cover modeling, tree rings
Citation: Seim A, Marquer L, Bisson U, Hofmann J, Herzig F, Kontic R, Lechterbeck J, Muigg B, Neyses-Eiden M, Rzepecki A, Rösch M, Walder F, Weidemüller J and Tegel W (2022) Historical Spruce Abundance in Central Europe: A Combined Dendrochronological and Palynological Approach. Front. Ecol. Evol. 10:909453. doi: 10.3389/fevo.2022.909453
Received: 31 March 2022; Accepted: 02 June 2022;
Published: 11 July 2022.
Edited by:
Gianluca Piovesan, University of Tuscia, ItalyReviewed by:
Alessia Masi, Sapienza University of Rome, ItalyKatarina Cufar, University of Ljubljana, Slovenia
Copyright © 2022 Seim, Marquer, Bisson, Hofmann, Herzig, Kontic, Lechterbeck, Muigg, Neyses-Eiden, Rzepecki, Rösch, Walder, Weidemüller and Tegel. This is an open-access article distributed under the terms of the Creative Commons Attribution License (CC BY). The use, distribution or reproduction in other forums is permitted, provided the original author(s) and the copyright owner(s) are credited and that the original publication in this journal is cited, in accordance with accepted academic practice. No use, distribution or reproduction is permitted which does not comply with these terms.
*Correspondence: Andrea Seim, andrea.seim@iww.uni-freiburg.de