- 1Centre d’Etudes Biologiques de Chizé, Centre National de la Recherche Scientifique-La Rochelle Université, UMR 7372, Villiers en Bois, France
- 2Department of Neurobiology, Physiology and Behavior, University of California, Davis, CA, United States
In the current context of global change, there is evidence of a large inter-individual variability in the way animals physiologically respond to anthropogenic changes. In that context, the Hypothalamus-Pituitary-Adrenal (HPA) axis and the corticosterone stress response are of primary importance because they are thought to govern the ability of individuals to adjust to stress. Several studies have reported that this stress response is variable among adults and they have successfully linked this variability with abiotic and biotic factors. However, the inter-individual variability of the glucocorticoid stress response has rarely been examined during the developmental phase in wild vertebrates, and its potential ecological determinants remain unclear. In this study, we examined the ontogeny of the corticosterone stress response in an altricial seabird species (i.e., how corticosterone levels increase in response to a standardized stress protocol), the Snow petrel. We reported a strong heterogeneity of the corticosterone stress response at all ages in snow petrel chicks (11-, 20-, and 37-days old chicks). Although the magnitude of this corticosterone stress response decreases with the age of the chick, we also found that this corticosterone stress response was repeatable throughout the post-hatching developmental period (repeatability: r > 0.50 for stress-induced corticosterone levels after a 30-min restraint). Importantly, this glucocorticoid stress response was negatively associated with the body condition of the chicks (i.e., mass corrected for body size), and previous exposure to sampling was associated with a dampened corticosterone stress response. However, we did not find any link between parental traits (parental condition or parental corticosterone stress response), nest quality, hatching date, and the chick’s corticosterone stress response. Our study suggests that the corticosterone stress response is a consistent individual trait that is affected to some extent by post-natal conditions, and which differs among individuals very early in life.
Introduction
In the current context of global change, there is an urgent need to appropriately assess to what extent wild populations of vertebrates can persist when confronted to anthropogenic changes (Newbold, 2018; Warren et al., 2018). To achieve this goal, it is acknowledged that individual heterogeneity is of primary importance because different individuals may differ in the way they react to change and in the way they sustain the costs of environmental changes (Clutton-Brock and Sheldon, 2010; Wilson and Nussey, 2010; Cam et al., 2016; Vedder and Bouwhuis, 2018; Forsythe et al., 2021). Indeed, there is strong evidence that there is a large inter-individual variability in the way individuals respond to stress with important fitness consequences. In that context, the concept of allostasis is crucial to consider because it is involved in the ability of individuals to achieve stability through change (McEwen and Wingfield, 2003). Allostasis-related mechanisms allow the individual to adjust to environmental changes and they involve several organismal systems (Wingfield, 2003; Romero et al., 2009). Among those, the Hypothalamus-Pituitary-Adrenal (HPA) axis is commonly studied because it is classically involved in the so-called “stress response” (Wingfield et al., 1998; Wingfield, 2003), and it is thought to play a role in the ability of vertebrates to cope with global change (Angelier and Wingfield, 2013). In response to stressors or environmental constraints, this endocrine axis is activated and it results in the quick release of significant amounts of glucocorticoids in the bloodstream (Sapolsky et al., 2000). In turn, glucocorticoids–corticosterone in birds, amphibians, reptiles and rodents–mediate behavioral and physiological responses that aim to help the organism survive the stressor (Landys et al., 2006). The inter-individual variability of this corticosterone stress response has been extensively studied in birds (Cockrem, 2007, 2013a,b) and its role in mediating inter-species and inter-individual in key life-history decisions has been convincingly demonstrated (Wingfield and Sapolsky, 2003; Bokony et al., 2009; Hau et al., 2010). However, the origin of this inter-individual variability remains relatively overlooked despite its importance to understand to what extent individuals can adjust to environmental changes (Angelier and Wingfield, 2013; Romero and Wingfield, 2016).
Importantly, the corticosterone stress response seems repeatable within individuals during the adult life (Taff et al., 2018) and this suggests that the corticosterone stress response is an individual trait with a somewhat limited flexibility during adulthood (Rensel and Schoech, 2011). Accordingly, a few studies on wild birds have shown that genetic components could be important drivers of inter-individual differences in the corticosterone stress response (Satterlee and Johnson, 1988; Evans et al., 2006; Almasi et al., 2010; Angelier et al., 2011; Baugh et al., 2012; Jenkins et al., 2014; Béziers et al., 2019). Recent studies have clearly demonstrated that the developmental period is also crucial to consider when explaining inter-individual differences in the corticosterone stress response because it is a key stage for its ontogeny (Wada et al., 2009; Schoech et al., 2011). Indeed, developmental conditions can have significant effects on the HPA axis and the resulting corticosterone stress response that can persist until adulthood [e.g., Marasco et al. (2012) in the Japanese quail]. For example, developmental nutritional restrictions have been shown to alter the functioning of the HPA axis later in life (e.g., Jimeno et al., 2018), and experimental exposure to corticosterone levels during the developmental phase has also been shown to affect the magnitude of the corticosterone stress response later in life (e.g., Crino et al., 2014; Grace et al., 2020).
Despite these significant advances, most of these studies have focused either on the adult corticosterone stress response only or on a single measure of the response during the developmental phase, limiting our ability to understand individual trajectories in the ontogeny of the corticosterone stress response. Therefore, it remains unclear how the magnitude of the corticosterone stress response changes within individuals over the developmental phase, and what ecological factors could drive such changes. It remains also unclear whether the corticosterone stress response is repeatable within individuals during the developmental phase. In altricial chicks, a few studies have demonstrated that the corticosterone stress response is reduced or even suppressed in young chicks relative to juveniles or adults in altricial species (e.g., Sims and Holberton, 2000; Love et al., 2003; Wada et al., 2007; Rensel et al., 2010; Bebus et al., 2020; Jones et al., 2021) and this stress hypo-responsive period [SHRP, stress hyporesponsive period, sensu Wada (2008)] at an early age has also been demonstrated in an altricial laboratory bird model, the zebra finch (Wada et al., 2009). Opposite results were found in captive precocial species with an effective stress response at a very early age (e.g., Ericsson and Jensen, 2016) and it has been linked with the relative independence of precocial birds at young ages (Wada, 2008). Interestingly, the same pattern was found in two altricial bird species [gray faced petrels, Adams et al. (2008); green-rumped parrotlets, Berg et al. (2019)]: young chicks were able to mount a strong stress response—similar to the adult corticosterone stress response—almost immediately after hatching. Therefore, additional studies are necessary to better understand the ontogeny of the stress response in developing chicks of wild birds, and its ecological determinants.
In this study, we examined the ontogeny of the corticosterone stress response in an altricial seabird species, the Snow petrel (Pagodroma nivea) and we investigated the potential determinants of inter-individual variation in the corticosterone stress response in chicks. Snow petrel chicks were sampled three times to (1) investigate how the corticosterone stress response changes over the course of the post-hatching developmental period; (2) document the inter- and intra-individual variability of the corticosterone stress response; (3) determine the influence of abiotic and biotic factors on the ontogeny of the corticosterone stress response. According to previous studies conducted in altricial species [Wada et al., 2007, 2009; Rensel et al., 2010, but see Adams et al. (2008)], we predicted that the magnitude of the corticosterone stress response will increase over the course of the developmental period as the HPA system is developing (prediction 1). Because the corticosterone stress response is at least partly heritable (Evans et al., 2006; Béziers et al., 2019) and has been shown to be repeatable from the developmental period to adulthood (e.g., Wada et al., 2008), we also predicted that the corticosterone stress response will be correlated to that of the parents (prediction 2) although this link may be tenuous because of the modulation of the stress response by body condition and parental care in breeding snow petrels (Angelier et al., 2009, 2020). We also predicted that this corticosterone stress response will be consistent within individuals throughout the development (i.e., repeatable, prediction 3). Finally, we predicted that the corticosterone stress response will be modulated by the internal state of the chick [i.e., body mass corrected for body size, hereafter “body condition,” Lynn et al. (2003, 2010) and Angelier et al. (2015), prediction 4], by the post-hatching environmental conditions (i.e., nest site, repeated exposure to stress, prediction 5), and by proxies of parental quality [i.e., hatching date, Sauser et al. (2021), and parental body condition, Barbraud and Chastel (1999); prediction 6].
Materials and methods
Study species
Snow petrels were studied on Ile des Pétrels, Pointe Géologie Archipelago, Terre Adélie (66°40’S, 140°01’E), Antarctica. Snow petrels are long-lived birds with high survival probability and low fecundity (Chastel et al., 1993). They lay a single egg and parents alternate incubating the egg until hatching. Incubation period lasts by average 45 days. After a period of brooding that is limited to approximately 9 days (Barbraud et al., 2000), the chick is left unattended by both parents, which forage simultaneously at sea to feed their chick. Males and females provide roughly similar amounts of parental care in this species, and by average, the chick receives a meal every 17 h (Barbraud et al., 1999) but the timing of the last meal was not known in our study. The chick developmental period lasts approximately 42 days (Barbraud et al., 2000) and the chicks then fledge and leave the colony until their first breeding attempt at approximately 10 years old (Chastel et al., 1993).
Hatching date, body condition, and the corticosterone stress response
In January 2012, 27 nests were monitored for hatching date by daily visual inspection of the nests. After hatching these nests were followed to monitor the growth of the chick and the ontogeny and the repeatability of the corticosterone stress response (prediction 1 & 3). In snow petrels, hatching date has been linked with parental age, and fledging success (Sauser et al., 2021), suggesting that hatching date is a reliable proxy of parental quality (prediction 6). After hatching, all nests were left undisturbed for 11 days and 15 chicks were measured and sampled when they were 11-days old, 20-days old and 37-days old. In addition, groups of seven and five chicks were randomly selected and left undisturbed until 20-days old and 37-days old (instead of sampling at 11-days old), respectively. This allowed us to test if the corticosterone stress response was affected by repeated captures/sampling (i.e., repeated exposure to stress, prediction 5) through habituation or sensitization. When nests were visited, the tarsus and the weight of each chick was measured with a caliper (±0.01 mm) and a spring scale (±5 gr), respectively. This allowed us to calculate the scaled mass index of each chick, as a proxy of body condition (Peig and Green, 2009), and to test its relationship with the corticosterone stress response (prediction 4). In addition, their corticosterone stress response was monitored by using a standardized stress protocol (Wingfield et al., 1992). Specifically, a first blood sample was collected from the chick within 3 min of capture to monitor baseline corticosterone levels. Then, the chick was restrained in a cloth bag for 30 min. A second and a third blood samples were collected after 15 and 30 min of restraint, respectively, to monitor stress-induced corticosterone levels (hereafter, stress-induced corticosterone levels at 15 min and stress-induced corticosterone levels at 30 min). Four birds could not be sampled quickly enough to monitor baseline corticosterone levels at a given age (Romero and Reed, 2005).
Nest quality
Nest quality was assessed according to the structure of the nest to test the link between the chick’s stress response and the quality of the nest (prediction 5). In snow petrels, the quality of the nest can be assessed according to the protection it offers to the chick against predators when the chick is left alone in the nest. In our study colony, South polar skuas (Catharacta maccormicki) often wander in the colony and opportunistically catch snow petrel chicks that are left alone in their nest. In that context, nest structure can play an important antipredator role by limiting the access to the chick by Antarctic skuas. In this study, we scored the nests that were monitored according to the protection it can offer to the chick against Antarctic skuas. A score of 1 referred to an open nest, which could be easily accessible to skuas. A score of 2 referred to a nest that is located between rocks but that remains partly accessible to skuas. A score of 3 referred to a nest that is located in a well-protected cavity and that is not accessible at all to skuas.
Parental corticosterone stress response and parental condition
During this field season (Austral summer 2011/2012), 35 breeding adult snow petrels were also captured during the incubation and the chick-rearing periods. All adults were captured while incubating their egg (N = 17) or brooding their chick (when the chicks were 5–20-days old, N = 18) at the nest. At capture, their tarsus and weight were measured with a caliper (±0.01 mm) and a spring scale (±5 gr), respectively. This allowed us to calculate the scaled mass index of each adult, as a proxy of body condition (Peig and Green, 2009) and to test the link between parental body condition and the chick’s stress response (prediction 6). The SMI was computed for each i individual as follows: SMIi = Mi × (Lo/Li)b where Mi and Li are, respectively, the body mass and the tarsus length of the individual i, Lo, the arithmetic mean value of tarsus length for the whole study population and b the slope estimate of a standardized major axis regression of log-transformed body mass on log-transformed tarsus length [see Peig and Green (2009)]. In addition, the corticosterone stress response of the parents was monitored by using a standardized stress protocol (Wingfield et al., 1992), as previously described for the chicks. Thus, we were able to measure their baseline corticosterone levels (within 3 min of capture), and their stress-induced corticosterone levels (after a 30 min restraint). Note that the adults were not sampled after 15 min of restraint. Importantly, all 18 sampled chick-rearing adults were a parent of a sampled chick, allowing us to test whether the corticosterone stress response of a chick is related to the corticosterone stress response of one of its parents (prediction 2). Only one parent per nest was captured and sampled to reduce nest disturbance.
Laboratory analyses
Blood samples were centrifuged and plasma was separated from red blood cells. Plasma samples were then stored at−20°C until analyzed at the lab. Plasma concentrations of corticosterone were determined by radioimmunoassay, as described previously (Angelier et al., 2015). All samples were run in one assay and three reference plasma were run in duplicates to calculate the intra-assay CV (intra-assay CV: 7.07%).
Statistical analyses
All statistical analyses were performed with SAS statistical software (SAS University edition). Firstly, General Linear Mixed Models (GLMM, proc glimmix) were run to test the influence of the “time” factor (three levels, baseline levels, stress-induced levels after 15 min of restraint, stress induced levels after 30 min of restraint), the “age” factor (three levels, 11-days old chicks, 20-days old chicks, and 37-days old chicks), and their interaction on corticosterone levels of chicks. Because each chick was sampled several times, “chick identity” was added as a random factor to control for repeated measures. Secondly, and to test the difference in corticosterone levels between chicks and adults, GLMM were run to test the influence of the “time” factor (two levels, baseline, stress-induced levels after 30 min of restraint), and the status factor (five levels, 11-days old chicks, 20-days old chicks, 37-days old chicks, incubating adults, chick-rearing adults) on corticosterone levels. This second set of models was run to compare chicks and adults because stress-induced corticosterone levels after 15 min of restraint were available for the chicks only. Thirdly, GLMMs were run to test the impact of previous exposure to capture and handling stress on corticosterone levels of 20-days old chicks and 37-days old chicks. These GLMMs aimed to test the influence of the “time” factor (three levels, baseline levels, stress-induced levels after 15 min of restraint, stress induced levels after 30 min of restraint), the “age” factor (two levels, 20-days old chicks, and 37-days old chicks), body condition, the “previous capture” factor (two levels, previously captured or not) and their interaction on corticosterone levels of chicks., Body condition index was standardized per age to allow building such models (transformation to a Z-distribution). Hatching date was also standardized (transformation to a Z-distribution). Fourthly, GLMMs were run to test the influence of the ‘time’ factor (three levels, baseline levels, stress-induced levels after 15 min of restraint, stress induced levels after 30 min of restraint), the “age” factor (three levels, 11-days old chick, 20-days old chicks, 37-days old chicks), body condition, “nest score,” “hatching date,” and their interactions on corticosterone levels of chicks. Because a specific data point had an important impact on the statistics (statistical outlier) and made some interactions almost significant (see Table 1), the same set of models was also run without this specific data point. Finally, GLMM were run to test the influence of the ‘age’ factor (three levels, 11-days old chicks, 20-days old chicks, and 37-days old chicks), parental baseline and stress-induced corticosterone levels, parental body condition, and their interaction on chick’s corticosterone levels. Because each chick was sampled several times, “chick identity” was added as a random factor. Model selections were conducted using a step wise approach and by eliminating progressively non-significant terms (Burnham and Anderson, 2002). Model assumptions were checked by using diagnostic plots (SAS, residual panels and box plots option) to visualize the distribution and the variance of residuals. Repeatability analyses (rptR package, R software) were run to assess the repeatability of (1) baseline corticosterone levels, (2) stress-induced corticosterone levels after 15 min of restraint, (3) stress-induced corticosterone levels after 30 min of restraint within individuals. Chick identity and the “age” factor were included as random and fixed factors, respectively. In addition, a second set of models were used to see if this repeatability could be related to the repeatability of body condition within individuals. In these additional models, the body condition index was added as an explanatory variable. In our data set, there was one data point with a very high corticosterone value (stress-induced corticosterone levels at 15 min: 60.15 ng/mL), which was potentially a statistical outlier. All statistics were run with and without this data point to make sure it did not affect our results and our interpretations.
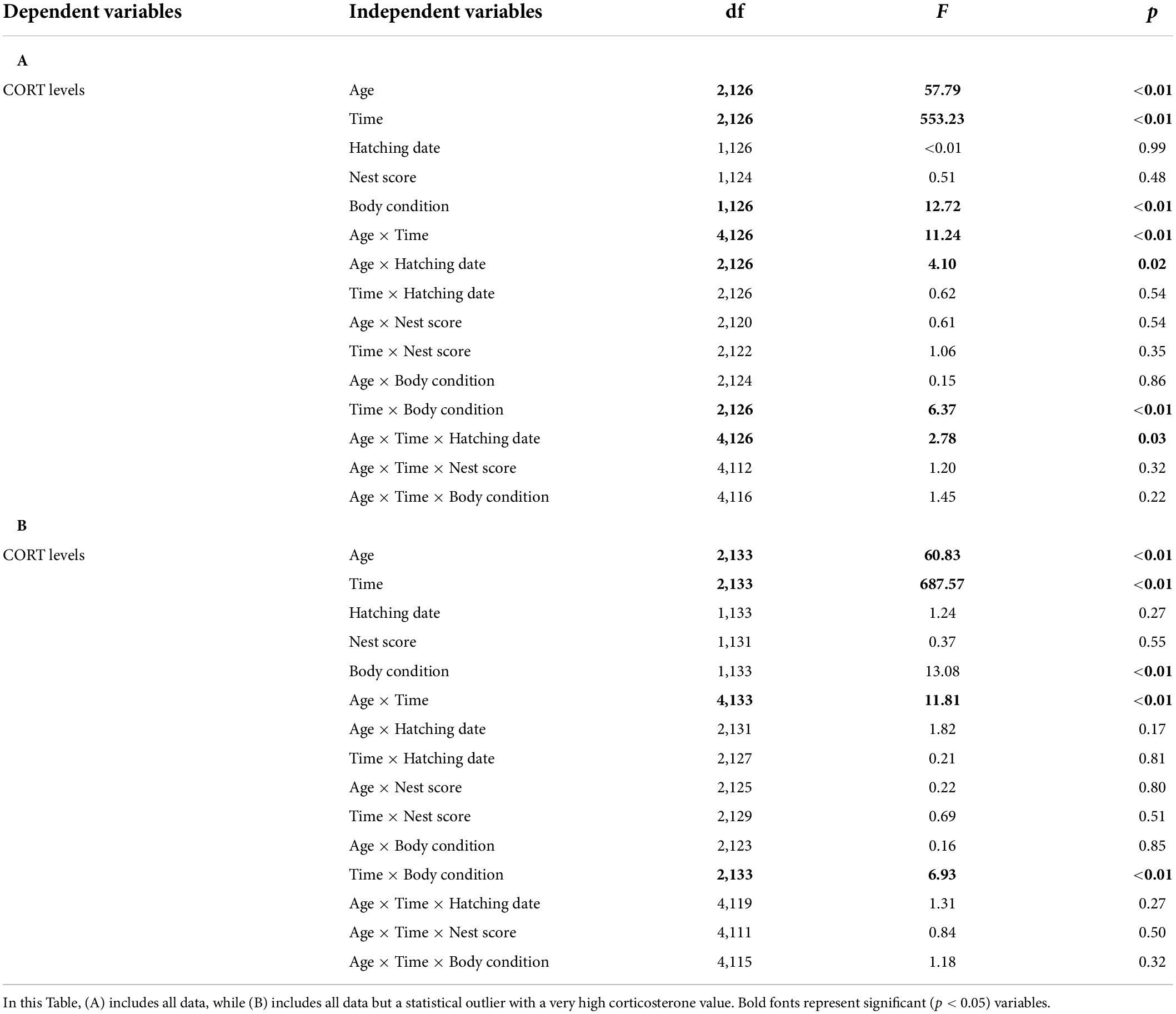
Table 1. Model selections to test the influence of the age of the chick (11-days old, 20-days old, 37-days old), time of sampling (baseline levels, stress-induced levels at 15 min, stress-induced levels at 30 min), body condition, hatching date, nest score, and all interactions on corticosterone levels.
Results
Age and the corticosterone stress response
Corticosterone levels were significantly linked to the “time factor” and corticosterone levels increased during the restraint stress protocol [F(2,137) = 483.67, p < 0.01; Figures 1A–C]. Importantly, corticosterone levels were also linked to the age of the chick [F(2,137) = 55.62, p < 0.01] and to the “age × time” interaction [F(4,137) = 9.52, p < 0.01], demonstrating that the corticosterone stress response was significantly correlated with the age of the chick (Figure 1). Specifically, baseline corticosterone levels did not significantly differ between 11-days old, 20-days old, and 37-days old chicks (post-hoc tests: all p > 0.30; Figures 1D–F). In contrast, stress-induced corticosterone levels at 15 min were higher in 11-days old chicks relative to 20-days old and 37-days old chicks (post hoc tests: all p < 0.01; Figures 1D–F) although stress-induced corticosterone levels at 15 min did not statistically differ between 20-days old and 37-days old chicks (post-hoc test: t = 1.01, p = 0.31; Figures 1E,F). Similarly, stress-induced corticosterone levels at 30 min were higher in 11 days old chicks relative to 20-days old and 37-days old chicks (post-hoc tests: all p < 0.01; Figures 1D–F) although stress-induced corticosterone levels at 30 min did not statistically differ between 20-days old and 37-days old chicks (post-hoc test: t = 0.97, p = 0.33; Figures 1E,F). The results remained similar if the potential statistical outlier was removed.
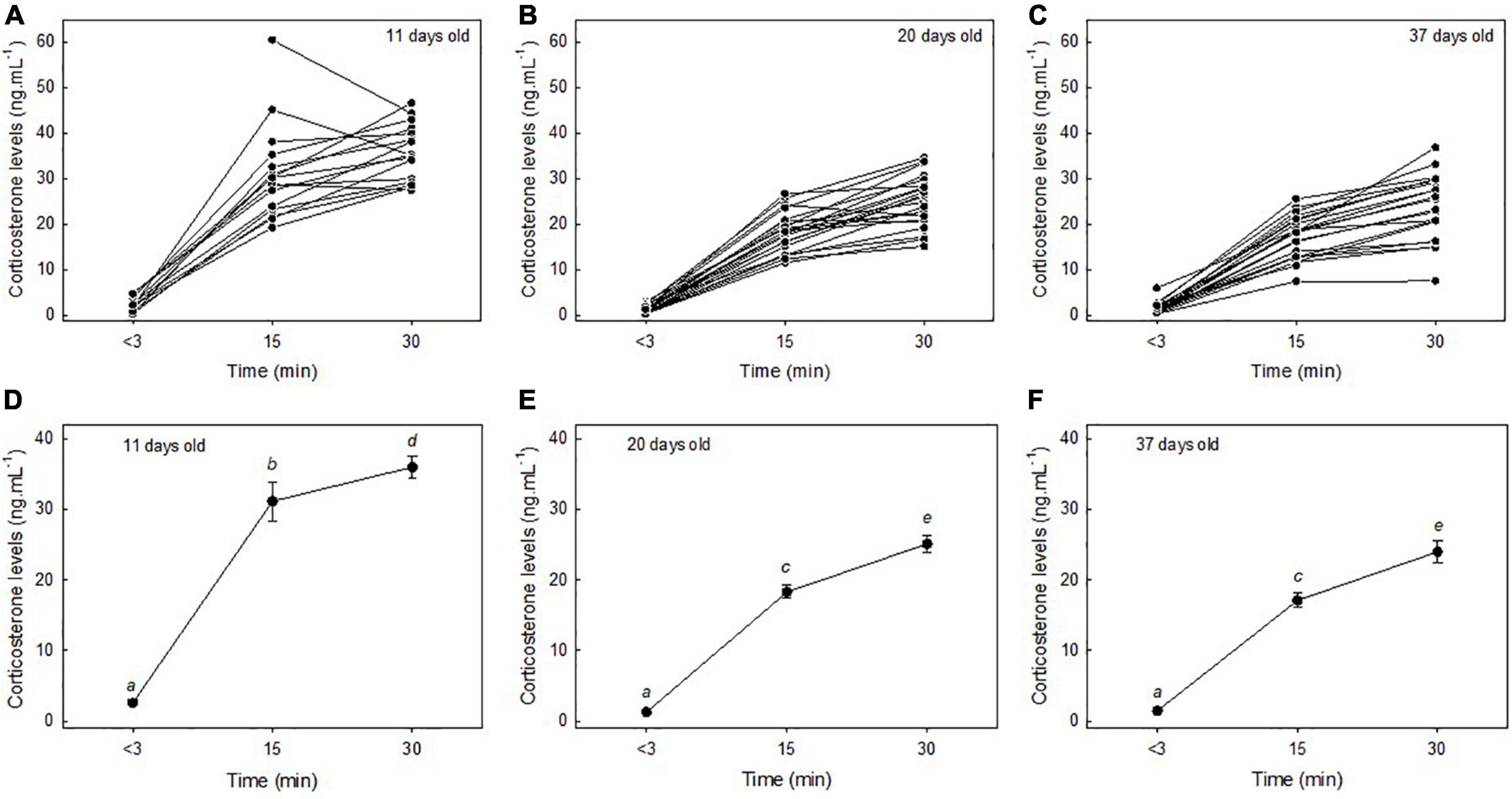
Figure 1. Inter-individual variability of the corticosterone stress response in snow petrel chicks at 11-days old (A), 20-days old (B), 37-days old (C), and influence of the age of the chick on the corticosterone stress response (D–F). Mean ± SE are represented and different letters indicate significant differences. Note that one data point with very high corticosterone levels was potentially a statistical outlier (stress-induced corticosterone levels at 15 min in 11-days old chicks: >60 ng/mL). All statistical tests remained similar if this data point was omitted from the data set.
When comparing the corticosterone stress response between chicks and adults, corticosterone levels were significantly linked to the “time factor” [F(1,114) = 1434.22, p < 0.01], the “status” factor [F(4,114) = 10.12, p < 0.01] and their interaction [F(4,114) = 6.15, p < 0.01], demonstrating that the corticosterone stress response significantly differed between individual statuses (11-, 20-, and 37-days old chick, and incubating and chick-rearing adults). For all statuses, corticosterone levels increased in response to the stress protocol. Baseline corticosterone levels did not differ between statuses (post-hoc tests: all p > 0.200). Stress-induced corticosterone levels of incubating and chick-rearing adults at 30 min were lower than those of the 11-days old chicks (post-hoc tests: all p < 0.05; Figure 2) but higher than those of 20- and 37-days old chicks (post-hoc tests: all p < 0.05; Figure 2). Stress-induced corticosterone levels of incubating and chick-rearing adults were similar (post-hoc test: p = 0.90; Figure 2). The results remained unchanged if the potential statistical outlier was removed.
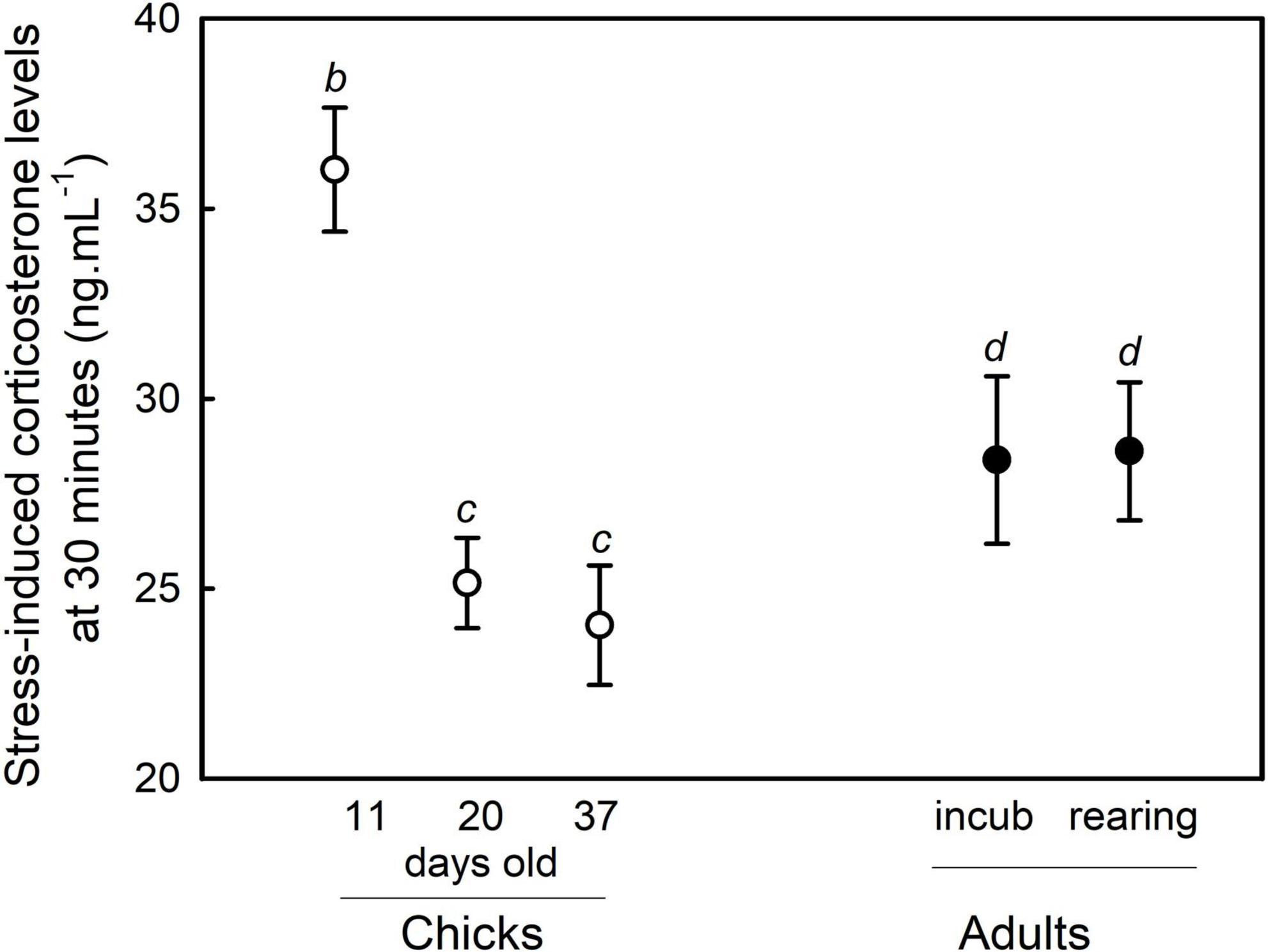
Figure 2. Difference in stress-induced corticosterone levels (after 30 min of restraint) between 11-days old chicks, 20-days old chicks, 37-days old chicks, incubating adults, and chick-rearing adults. Mean ± SE are represented and different letters indicate significant differences. White and black symbols represent chicks and adults, respectively.
Body condition and corticosterone levels
Corticosterone levels were significantly linked to the “time factor” [F(2,134) = 525,88 p < 0.01], to the age of the chick [F(2,134) = 56.17, p < 0.01] and to the “age × time” interaction [F(4,134) = 10.70, p < 0.01]. In addition, corticosterone levels were linked to the body condition index [F(1,134) = 9.79, p < 0.01] and the “body condition index × time” interaction [F(2,134) = 5.32, p < 0.01], demonstrating that the correlation between body condition on corticosterone levels varied between baseline and stress-induced levels. Corticosterone levels were not significantly linked to any other interaction (all p > 0.60). Specifically, baseline corticosterone levels were not significantly correlated with the body condition index (t = 0.13, p = 0.90; Figure 3) while stress -induced corticosterone levels were negatively correlated with the body condition index (stress-induced corticosterone levels at 15 min: t = −3.06, p < 0.01; stress-induced corticosterone levels at 30 min: t = −3.63, p < 0.01; Figure 3). Corticosterone levels were not significantly linked to the “body condition × age” and the “body condition index × age × time” interactions (all p > 0.40), demonstrating that the correlation between body condition on corticosterone levels did not differ between 11-, 20-, and 37-days old chicks. The results remained similar if the potential statistical outlier was removed.
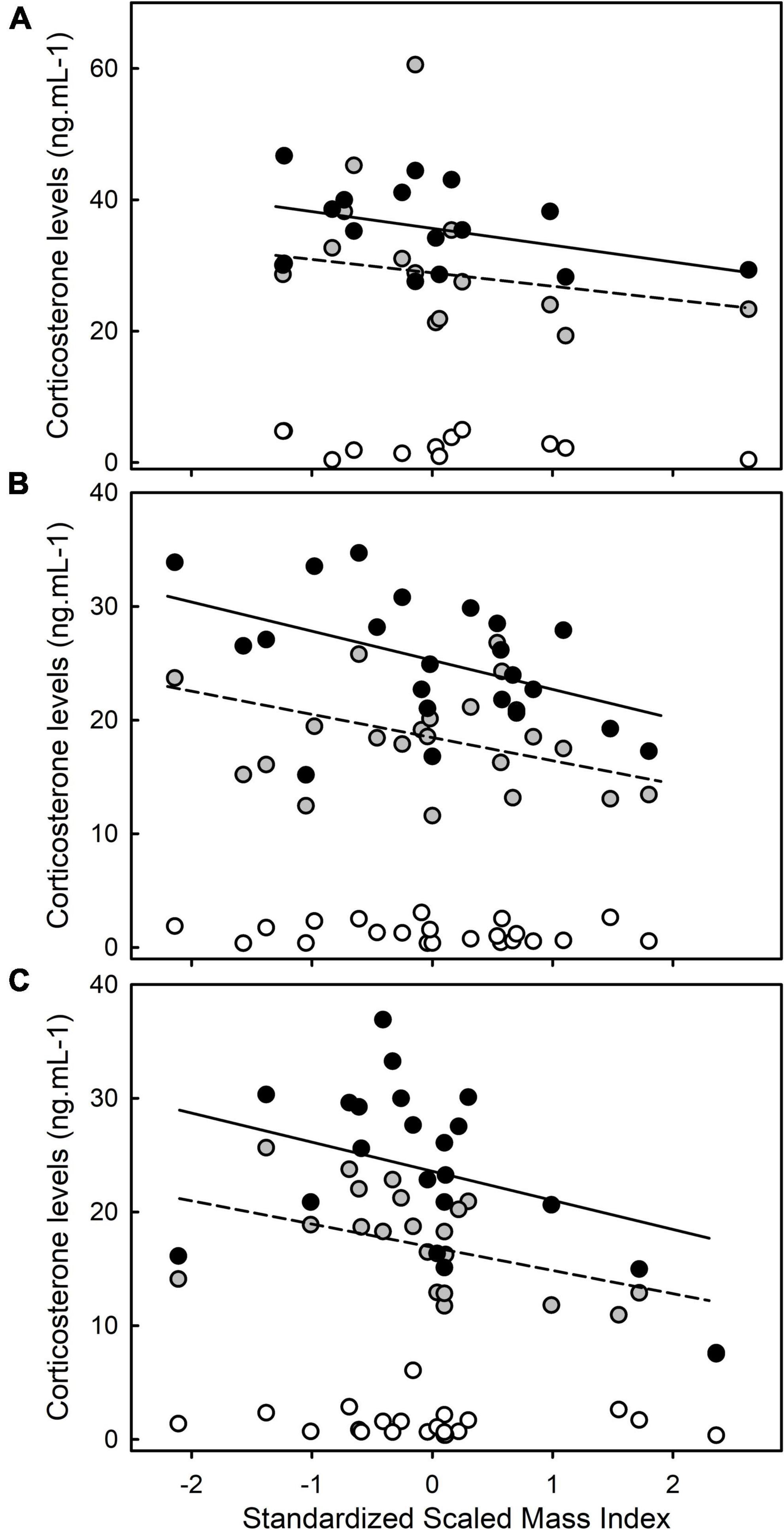
Figure 3. Influence of body condition (Scaled Mass Index) on corticosterone levels in 11-days old (A), 20-days old (B), and 37-days old (C) chicks. White, gray and black dots represent baseline corticosterone levels, stress-induced corticosterone levels after a 15 min restraint, and stress-induced corticosterone levels after a 30 min restraint, respectively. Solid and dashed lines represent significant relationships between body condition and corticosterone levels (dashed: stress-induced corticosterone levels at 15 min; solid: stress-induced corticosterone levels at 30 min).
Impact of multiple captures on the corticosterone stress response
In 20- and 37-days old chicks, corticosterone levels were linked to the “time factor” [F(2,97) = 527.81, p < 0.01] and to the age of the chick [F(1,97) = 4.23, p = 0.04] but not to the “age × time” interaction [F(2,92) = 0.81, p = 0.45]. Corticosterone levels were also linked to the body condition index [F(1,97) = 16.61, p < 0.01], and to the “body condition index × time” interaction [F(2,97) = 7.16, p < 0.01]. However, corticosterone levels were not significantly linked to the “previous capture” factor [F(1,96) = 0.01, p = 0.93, Figure 4], and any other interactions (all p > 0.10). The results remained similar if the potential statistical outlier was removed.
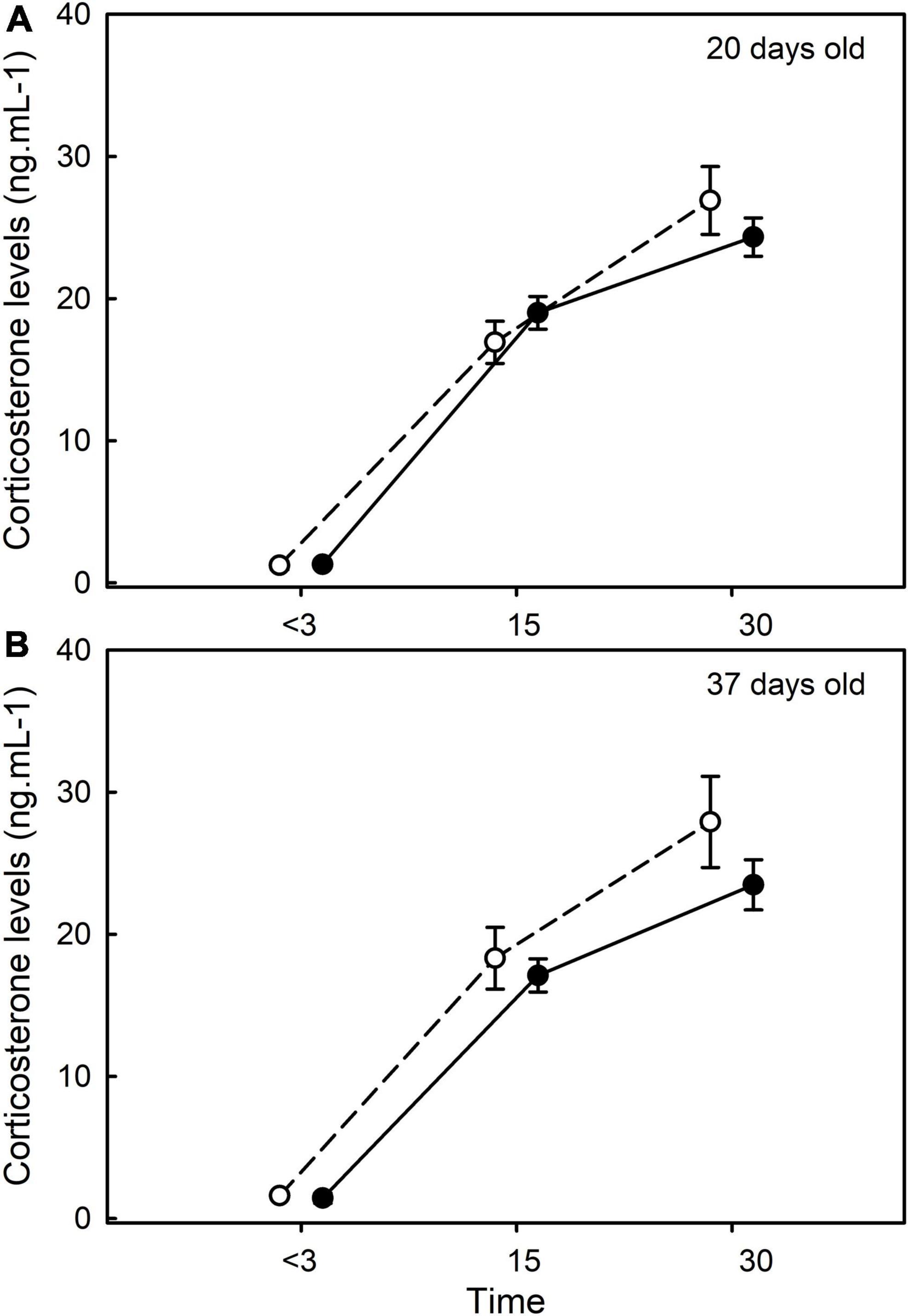
Figure 4. Influence of previous exposure to stress (i.e., capture/restraint protocol) on the corticosterone stress response of 20-days old (A) and 37-days old (B) chicks. Mean ± SE are represented and no significant differences were found. Black and white dots represent the petrels that have been previously captured or not, respectively.
Nest score, hatching date, and corticosterone levels
Although corticosterone levels were linked to the “time factor,” the age of the chick, body condition, and the “age × time” and “time × body condition” interactions (Table 1), they were not linked to the nest score or any other interaction including the nest score (Table 1). Similarly, corticosterone levels were not significantly linked to hatching date or the “hatching date × time” interaction (Table 1A). There was a significant trend for an effect of the “hatching date × time” and the “hatching date × age × time” interactions (Table 1A), but this was the result of the potential statistical outlier and these interactions were far from being significant when this data point was removed (Table 1B).
Parental traits and corticosterone levels
Although corticosterone levels were linked to the “time factor,” the age of the chick, body condition, and to the “age × time” and “time × body condition” interactions (Table 2), they were not linked to parental body condition, parental baseline corticosterone levels, parental stress-induced corticosterone levels or any interaction (Table 2). The results remained unchanged if the potential statistical outlier was removed (no parental data was available for this specific data point).
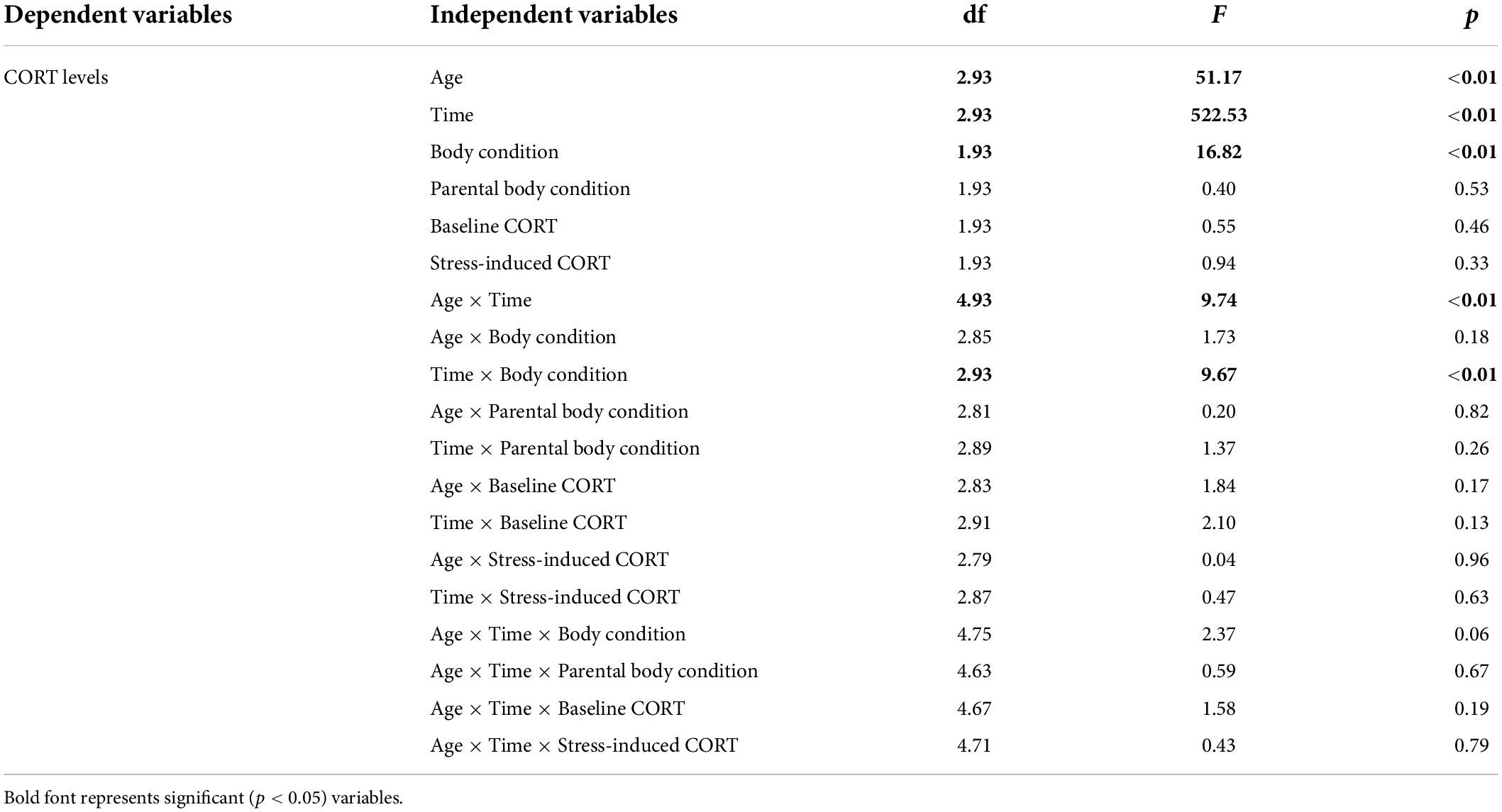
Table 2. Model selections to test the influence of the age of the chick (11-, 20-, and 37-days old), body condition of the chick, baseline and stress-induced corticosterone levels of the parent (“baseline CORT” and “stress-induced CORT”), body condition of the parent (“parental body condition”), and all interactions on corticosterone levels.
Repeatability of corticosterone levels and body condition
Baseline corticosterone levels were not repeatable (repeatability: r ± se = 0 ± 0.12, Figure 5). However, stress-induced corticosterone levels at 15 min were repeatable (repeatability: r = 0.23 ± 0.16, r = 0.38 ± 0.16 if the potential statistical outlier was removed, Figure 5). Stress-induced corticosterone levels at 30 min were also repeatable (repeatability: 30 min, r = 0.57 ± 0.13, Figure 5). Body condition was also repeatable (r = 0.51 ± 0.14). The repeatability of corticosterone levels was reduced when the body condition index was included in the models testing the repeatability of corticosterone levels. Specifically, baseline corticosterone levels were still not repeatable (repeatability: r ± se = 0 ± 0.12). Stress-induced corticosterone levels at 15 min were not repeatable (repeatability: r = 0 ± 0.10, r = 0.01 ± 0.12 if the potential statistical outlier was removed), while stress-induced corticosterone levels at 30 min remain repeatable (repeatability: 30 min, r = 0.43 ± 0.16).
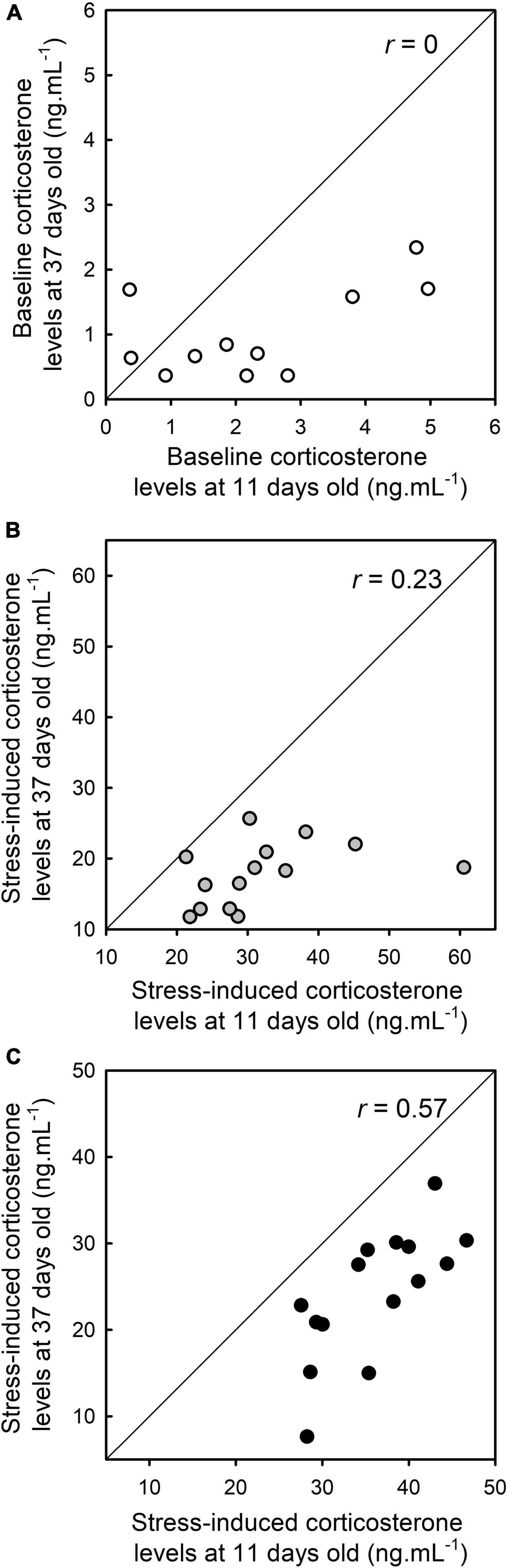
Figure 5. Repeatability (r) of baseline corticosterone levels (A), stress-induced corticosterone levels after a 15 min restraint (B), and stress-induced corticosterone levels after a 30 min restraint (C).
Discussion
In this study, we reported not only a strong heterogeneity of the corticosterone stress response at all ages in snow petrel chicks, but also a high repeatability of this corticosterone stress response throughout the post-hatching developmental period (e.g., repeatability: r = 0.57 for stress-induced corticosterone levels after a 30 min restraint). This suggests that this stress response is a consistent individual trait that shows a high inter-individual variability. Although this corticosterone stress response is linked to the internal state of the chick (age, body condition), we however did not find any strong evidence linking parental traits (parental condition or parental corticosterone stress response) and other ecological factors (nest quality, hatching date, previous exposure to sampling) with the chick corticosterone stress response. In addition, we found that the magnitude of the corticosterone stress response of snow petrel chicks is similar if not higher than that of breeding adults, suggesting that the chicks from this species do not show a stress hyporesponsive period at young ages [sensu Wada (2008)], as commonly found in altricial species. Our results emphasize that the corticosterone stress response shows a high-interindividual variability and future long-term studies are now required to understand whether this heterogeneity may play a role in the ability of developing individuals to cope with the perturbations that occur in Antarctica.
Baseline corticosterone levels
We found that baseline corticosterone levels were not affected by the age of the chick and they did not significantly differ between chicks and adults. In addition, baseline corticosterone levels of chicks were not repeatable throughout the post-hatching phase. These results are consistent with previous studies, which have shown that baseline corticosterone levels are labile and poorly repeatable [Romero and Reed, 2008; Rensel and Schoech, 2011; but see Ouyang et al. (2011)]. In their meta-analysis, Taff et al. (2018) reported that the repeatability of baseline corticosterone is low (r = 0.18, CI = 0.11–0.24) and we may have lacked statistical power to detect such a low repeatability in our study (sample size of 15 snow petrel chicks). In addition, baseline corticosterone levels were not related to body condition at any age (11-, 20-, and 37-days old). However, baseline corticosterone levels are classically elevated when individuals are in poor condition, as demonstrated in many bird species [e.g., adults: Lynn et al. (2003, 2010)], including seabirds [adults and chicks; e.g., Kitaysky et al. (2001) and Quilfeldt et al. (2006)], and even adult and chick snow petrels (Angelier et al., 2009, 2015; Dupont et al., 2021). Therefore, our results suggest that snow petrel chicks were not energetically constrained during the 2012 breeding season and this interpretation is indeed supported by the high fledging success of the 2012 season [see Sauser et al. (2021)]. Supporting further this interpretation, baseline corticosterone levels were not correlated with hatching date, and we did not find any evidence that baseline corticosterone levels were higher when the chicks were growing in a less protected nest. Inclement weather is known to increase baseline corticosterone levels in birds (Bize et al., 2010; de Bruijn and Romero, 2018; Crino et al., 2020) and hatching date and nest characteristics are known to be linked with local weather and fledging success. Specifically, the weather usually deteriorates as the breeding season progresses (snow fall and strong wind) and chicks from more sheltered nest are less affected by inclement weather (Dupont et al., 2020; Sauser et al., 2021). However, this was not the case in 2012, which was characterized by very little snow fall and no strong wind during the chick-rearing period (Sauser et al., 2021), and as a result, all chicks survived in our study. This specific mild weather may explain why baseline corticosterone levels were unrelated to nest score and hatching date.
Ontogeny of the corticosterone stress response during the post-hatching phase
We found that the chicks elicit a strong corticosterone stress response when they are as young as 11-days old. Indeed, the corticosterone stress response of 11-days old chicks is even greater than the corticosterone stress response of older chicks or even adults. This demonstrates that the HPA axis is fully operational at a very young age in this altricial seabird species. Although this result is not supported by several studies (Sims and Holberton, 2000; Love et al., 2003; Wada et al., 2007, 2009; Rensel et al., 2010; Bebus et al., 2020; Jones et al., 2021), two studies found similar results: Adams et al. (2008) found in another petrel species that chicks elicit a significant corticosterone stress response a few days after hatching. Similarly, Berg et al. (2019) recently found the same pattern in another altricial species, the green-rumped parrotlet. In our study, we sampled the chicks 11 days after hatching and it is possible that the HPA axis was not active before that age. However, 11 days represent only a fourth of the post-hatching developmental phase in the snow petrel and our results therefore demonstrate that snow petrel chicks are able to elicit a fully operational corticosterone stress response at a young age. It remains unclear why the ontogeny of the corticosterone stress response is rapid in a few altricial species (Adams et al., 2008; Berg et al., 2019; this study) but not others (Sims and Holberton, 2000; Love et al., 2003; Wada et al., 2007, 2009; Rensel et al., 2010; Bebus et al., 2020; Jones et al., 2021). A dampened stress response in young altricial chicks is thought to minimize the costs of bearing elevated corticosterone levels [slower growth, altered immunity, etc.; Kitaysky et al. (2003) and Martin et al. (2005)], but a functional HPA axis may be necessary to activate metabolic functions (Jimeno et al., 2018, 2020), which is crucial in precocial species and in a few altricial species living under extreme weather conditions such as the snow petrel. This could explain our results because snow petrel chicks can be left unattended by their parents at a very young age (Barbraud et al., 1999). Indeed, snow petrels, as many other seabird species, are often considered as semi-altricial because their chicks are covered with down at hatching and are able to maintain their body temperature at high levels soon after hatching. In our study, we measured the corticosterone stress response through plasma levels of corticosterone in response to a standardized stress protocol. Because the action of corticosterone is mediated through the binding to multiple types of receptors (Landys et al., 2006), it would be necessary to measure the location and the density of these glucocorticoid receptors to fully understand how the corticosterone stress response is influenced by age and post-hatching conditions. However, for ethical reasons (i.e., the study of receptors requires to euthanize the birds to collect organs), it was obviously not possible to study this question in long-lived Antarctic snow petrels.
We found in our study that snow petrel chicks show a high inter-individual variability in the magnitude of the corticosterone stress response (i.e., stress-induced corticosterone levels at 15 and 30 min). Importantly, we also reported for the first time in a wild bird species that this corticosterone stress response is repeatable throughout the post-hatching phase. Previous studies have reported that the corticosterone stress response is repeatable within individuals but most of these studies have focused on the adult life [reviewed in Taff et al. (2018)]. Interestingly, our repeatability coefficient is within the upper range that was reported by Taff et al. (2018) in their meta-analysis of dozens of species [i.e., Taff et al. (2018): r = 0.38, CI = 0.29–0.45], even after considering the potential effect of body condition on this repeatability measurement. This suggests that the corticosterone stress response could be a consistent physiological trait in snow petrels, as in many other bird species. In addition, this repeatability of the corticosterone stress response could also result from the circumstances in which the chicks are found rather than from a consistency of this physiological trait. Supporting this possibility, we found that the body condition of chicks is also repeatable through development (i.e., from 11-days old to 37-days old) and is correlated to the corticosterone stress response. Indeed, the corticosterone stress response of chicks is certainly determined simultaneously by the circumstances (i.e., rearing environment) and a genetic component (Jenkins et al., 2014) that both translate into a high repeatability of stress-induced corticosterone levels in our study. Supporting this idea, we found that body condition accounts for a part, but not all, of the repeatability of stress-induced corticosterone levels. This is consistent with the idea that the corticosterone stress response and the functioning of the HPA axis may be subject to selection (Angelier and Wingfield, 2013; Jenkins et al., 2014; Vitousek et al., 2014) and at least partly heritable (Satterlee and Johnson, 1988; Evans et al., 2006; Almasi et al., 2010; Angelier et al., 2011; Baugh et al., 2012; Jenkins et al., 2014; Béziers et al., 2019). Surprisingly, we did not find any evidence of such heritability in our study because the corticosterone stress response of the chick was not related to the corticosterone stress response of the parent. However, we only sampled one of the two parents to limit nest disturbance, and the parents were sampled during the chick-rearing phase when they are known to actively modulate their corticosterone stress response (Angelier et al., 2015, 2020), limiting therefore our ability to test the heritability of this stress response. To fully test the heritability of the corticosterone stress response, it would have been necessary to sample the parents and their chicks at the same life-history stage, but it is unpractical because the chicks return to the colony to breed only when they are 10 years old by average (Chastel et al., 1993).
Ecological determinants of the corticosterone stress response
As expected, we found that stress-induced corticosterone levels were affected by the internal state of the chicks. Specifically, the age of the chick had a strong influence on stress-induced corticosterone levels and chicks had higher stress-induced corticosterone levels at 11-days old relative to 20- or 37-days old. This difference may be explained by a smaller amount of body reserves at younger age because the ability of smaller chicks to store body reserves is usually limited by their smaller structural size. Indeed, the corticosterone stress response has been convincingly linked to the nutritional status of chicks in multiple species (e.g., Sockman and Schwabl, 2001), including seabirds (e.g., Kitaysky et al., 2001; Quilfeldt et al., 2006) and snow petrels (Dupont et al., 2021). Supporting further this idea, we found that stress-induced corticosterone levels were negatively correlated with body condition in 11-, 20-, and 37-days old chicks although it remains to be determined if this correlation results from a satiety effect (low corticosterone levels in recently fed chicks) or an effect of nutritional reserves (low corticosterone levels in chick with large body reserves).
In contrast, and contrary to our prediction, we did not find any evidence supporting an effect of nest quality on the corticosterone stress response. However, nest quality influences the exposure of snow petrel chicks to inclement weather and predation risk, two factors that could affect the corticosterone stress response [Cockrem and Silverin, 2002; de Bruijn and Romero, 2018; Crino et al., 2020; but see Rivers et al. (2011)]. This suggests that most of the inter-individual variability in the corticosterone stress response may be relatively independent of these specific abiotic factors during the post-hatching phase (at least in the study species). Instead, the corticosterone stress response may rather be an individually repeatable trait [reviewed in Taff et al. (2018)], which is determined by a genetic component and/or the nutritional status of the chick [see also Jenkins et al. (2014)]. Supporting this idea, we found that the corticosterone stress response was also independent of two proxies of parental quality (parental body condition and hatching date). More successful parents usually breed earlier and are in better condition in snow petrels (Barbraud and Chastel, 1999; Sauser et al., 2021), but hatching date and parental body condition were not related to the chick’s corticosterone stress response at any age in our study. Finally, we did not find any evidence for an effect of repeated exposure to capture and handling on the chick’s corticosterone stress response (Cyr and Romero, 2009), or on baseline corticosterone levels. This suggests that habituation of the corticosterone stress response is unlikely to occur in snow petrel chicks and that repeated capture and sampling may not detrimentally affect the development of the chicks in this petrel species [see also Dupont et al. (2020)]. Supporting this idea, all chicks successfully fledged in our study, suggesting that the fitness impact of repeated capture and sampling is probably limited, at least when environmental conditions are favorable [as it was the case during that breeding season, Sauser et al. (2021)]. However, we must remain cautious because our sample size of unmanipulated petrels was relatively limited (n = 5 for 20-days old petrels, n = 7 for 37 days old petrels). From a mechanistic point of view, repeated exposure to acute stressors may affect the functioning of the HPA axis in vertebrates (Cyr and Romero, 2009) but our results suggest that habituation and sensitization of the corticosterone stress response do not occur at that age in snow petrel chicks, except possibly in case of frequent exposure to stress [see Sagar et al. (2019)] but we could not test this because of limited sample size. Again, this argues for the idea that the corticosterone stress response is probably an individually consistent trait that shows a strong inter-individual variability during the post-hatching developmental phase.
Data availability statement
The raw data supporting the conclusions of this article will be made available by the authors, without undue reservation.
Ethics statement
Licences and permissions were granted by the Ethic Committee of the Institut Polaire Francais (IPEV).
Author contributions
FA: conceived the research, conducted field work, analyzed the data, and wrote the manuscript. JW and OC: conceived the research and provided comments on the manuscript. CB: provided comments on the manuscript. CP: conducted laboratory work and field work. CT: conducted laboratory work. All authors contributed to the article and approved the submitted version.
Funding
The present research project was performed at Dumont d’Urville Station in Antarctica and was supported by the French Polar Institute (IPEV, Prog. 109). FA was supported by the 7th research program of the European Community FP7/2007–2013 (Marie-Curie Fellowship, no. 237034).
Acknowledgments
We thank the “Service d’Analyses Biologiques” of the CEBC for its expertise and its technical help in conducting laboratory analyses. We thank Bertille Mohring for help with the statistical analyses.
Conflict of interest
The authors declare that the research was conducted in the absence of any commercial or financial relationships that could be construed as a potential conflict of interest.
The reviewer KB declared a past co-authorship with one of the authors JW to the handling editor.
Publisher’s note
All claims expressed in this article are solely those of the authors and do not necessarily represent those of their affiliated organizations, or those of the publisher, the editors and the reviewers. Any product that may be evaluated in this article, or claim that may be made by its manufacturer, is not guaranteed or endorsed by the publisher.
Supplementary material
The Supplementary Material for this article can be found online at: https://www.frontiersin.org/articles/10.3389/fevo.2022.902202/full#supplementary-material
References
Adams, N. J., Cockrem, J. F., Candy, E. J., and Taylor, G. A. (2008). Non-precocial grey-faced petrel chicks (Pterodroma macroptera gouldi) show no age-related variation in corticosterone responses to capture and handling. Gen. Comp. Endocrinol. 157, 86–90. doi: 10.1016/j.ygcen.2008.02.007
Almasi, B., Jenni, L., Jenni-Eiermann, S., and Roulin, A. (2010). Regulation of stress response is heritable and functionally linked to melanin-based coloration. J. Evol. Biol. 23, 987–996.
Angelier, F., and Wingfield, J. C. (2013). Importance of the glucocorticoid stress response in a changing world: Theory, hypotheses and perspectives. Gen. Comp. Endocrinol. 190, 118–128. doi: 10.1016/j.ygcen.2013.05.022
Angelier, F., Ballentine, B., Holberton, R. L., Marra, P. P., and Greenberg, R. (2011). What drives variation in the corticosterone stress response between subspecies? A common garden experiment of swamp sparrows (Melospiza georgiana). J. Evol. Biol. 24, 1274–1283. doi: 10.1111/j.1420-9101.2011.02260.x
Angelier, F., Chastel, O., Lendvai, A. Z., Parenteau, C., Weimerskirch, H., and Wingfield, J. C. (2020). When do older birds better resist stress? A study of the corticosterone stress response in snow petrels. Biol. Lett. 16:20190733. doi: 10.1098/rsbl.2019.0733
Angelier, F., Moe, B., Blanc, S., and Chastel, O. (2009). What factors drive prolactin and corticosterone responses to stress in a long-lived bird species (snow petrel Pagodroma nivea)? Physiol. Biochem. Zool. 82, 590–602. doi: 10.1086/603634
Angelier, F., Wingfield, J. C., Parenteau, C., Pellé, M., and Chastel, O. (2015). Does short-term fasting lead to stressed-out parents? A study of incubation commitment and the hormonal stress responses and recoveries in snow petrels. Horm. Behav. 67, 28–37. doi: 10.1016/j.yhbeh.2014.11.009
Barbraud, C., and Chastel, O. (1999). Early body condition and hatching success in the snow petrel Pagodroma nivea. Polar Biol. 21, 1–4.
Barbraud, C., Weimerskirch, H., Guinet, C., and Jouventin, P. (2000). Effect of sea-ice extent on adult survival of an Antarctic top predator: The snow petrel Pagodroma nivea. Oecologia 125, 483–488. doi: 10.1007/s004420000481
Barbraud, C., Weimerskirch, H., Robertson, G. G., and Jouventin, P. (1999). Size-related life history traits: Insights from a study of snow petrels (Pagodroma nivea). J. Anim. Ecol. 68, 1179–1192.
Baugh, A. T., Schaper, S. V., Hau, M., Cockrem, J. F., de Goede, P., and van Oers, K. (2012). Corticosterone responses differ between lines of great tits (Parus major) selected for divergent personalities. Gen. Comp. Endocrinol. 175, 488–494. doi: 10.1016/j.ygcen.2011.12.012
Bebus, S. E., Jones, B. C., and Anderson, R. C. (2020). Development of the corticosterone stress response differs among passerine species. Gen. Comp. Endocrinol. 291:113417.
Berg, K. S., Delgado, S., Mata-Betancourt, A., Krause, J. S., Wingfield, J. C., and Beissinger, S. R. (2019). Ontogeny of the adrenocortical response in an extremely altricial bird. J. Exp. Zool. Part A 331, 521–529. doi: 10.1002/jez.2317
Béziers, P., San-Jose, L. M., Almasi, B., Jenni, L., and Roulin, A. (2019). Baseline and stress-induced corticosterone levels are heritable and genetically correlated in a barn owl population. Heredity 123, 337–348. doi: 10.1038/s41437-019-0203-5
Bize, P., Stocker, A., Jenni-Eiermann, S., Gasparini, J., and Roulin, A. (2010). Sudden weather deterioration but not brood size affects baseline corticosterone levels in nestling Alpine swifts. Horm. Behav. 58, 591–598.
Bokony, V., Lendvai, A. Z., Liker, A., Angelier, F., Wingfield, J. C., and Chastel, O. (2009). Stress response and the value of reproduction: Are birds prudent parents? Am. Nat. 173, 589–598.
Burnham, K. P., and Anderson, D. R. (2002). Model Selection and Multimodel Inference. Berlin: Springer.
Cam, E., Aubry, L. M., and Authier, M. (2016). The conundrum of heterogeneities in life history studies. Trends Ecol. Evol. 31, 872–886.
Chastel, O., Weimerskirch, H., and Jouventin, P. (1993). High annual variability in reproductive success and survival of an Antarctic seabird, the snow petrel Pagodroma nivea. Oecologia 94, 278–285. doi: 10.1007/BF00341328
Clutton-Brock, T., and Sheldon, B. C. (2010). Individuals and populations: The role of long-term, individual-based studies of animals in ecology and evolutionary biology. Trends Ecol. Evol. 25, 562–573. doi: 10.1016/j.tree.2010.08.002
Cockrem, J. F. (2007). Stress, corticosterone responses and avian personalities. J. Ornithol. 148, 169–178.
Cockrem, J. F. (2013a). Corticosterone responses and personality in birds: Individual variation and the ability to cope with environmental changes due to climate change. Gen. Comp. Endocrinol. 190, 156–163. doi: 10.1016/j.ygcen.2013.02.021
Cockrem, J. F. (2013b). Individual variation in glucocorticoid stress responses in animals. Gen. Comp. Endocrinol. 181, 45–58.
Cockrem, J. F., and Silverin, B. (2002). Sight of a predator can stimulate a corticosterone response in the great tit (Parus major). Gen. Comp. Endocrinol. 125, 248–255. doi: 10.1006/gcen.2001.7749
Crino, O. L., Driscoll, S. C., and Breuner, C. W. (2014). Corticosterone exposure during development has sustained but not lifelong effects on body size and total and free corticosterone responses in the zebra finch. Gen. Comp. Endocrinol. 196, 123–129.
Crino, O. L., Driscoll, S. C., Brandl, H. B., Buchanan, K. L., and Griffith, S. C. (2020). Under the weather: Corticosterone levels in wild nestlings are associated with ambient temperature and wind. Gen. Comp. Endocrinol. 285:113247. doi: 10.1016/j.ygcen.2019.113247
Cyr, N. E., and Romero, L. M. (2009). Identifying hormonal habituation in field studies of stress. Gen. Comp. Endocrinol. 161, 295–303.
de Bruijn, R., and Romero, L. M. (2018). The role of glucocorticoids in the vertebrate response to weather. Gen. Comp. Endocrinol. 269, 11–32.
Dupont S., Barbraud, C., Chastel, O., Delord, K., Parenteau, C., Ribout, C., and Angelier, F. (2020). Do repeated captures and handling affect phenotype and survival of growing Snow Petrel (Pagodroma nivea)? Polar. Biol. 43, 637–646. doi: 10.1007/s00300-020-02666-7
Dupont, S. M., Barbraud, C., Chastel, O., Delord, K., Parenteau, C., Trouvé, C., et al. (2021). “Home alone!” influence of nest parental attendance on offspring behavioral and hormonal stress responses in an Antarctic seabird, the snow petrel (Pagodroma nivea). Horm. Behav. 131:104962. doi: 10.1016/j.yhbeh.2021.104962
Ericsson, M., and Jensen, P. (2016). Domestication and ontogeny effects on the stress response in young chickens (Gallus gallus). Sci. Rep. 6:35818.
Evans, M. R., Roberts, M. L., Buchanan, K. L., and Goldsmith, A. R. (2006). Heritability of corticosterone response and changes in life history traits during selection in the zebra finch. J. Evol. Biol. 19, 343–352. doi: 10.1111/j.1420-9101.2005.01034.x
Forsythe, A. B., Day, T., and Nelson, W. A. (2021). Demystifying individual heterogeneity. Ecol. Lett. 24, 2282–2297.
Grace, J. K., Parenteau, C., and Angelier, F. (2020). Post-natal corticosterone exposure downregulates the HPA axis through adulthood in a common passerine. Gen. Comp. Endocrinol. 292:113421. doi: 10.1016/j.ygcen.2020.113421
Hau, M., Ricklefs, R. E., Wikelski, M., Lee, K. A., and Brawn, J. D. (2010). Corticosterone, testosterone and life-history strategies of birds. Proc. R. Soc. B 277, 3203–3212.
Jenkins, B. R., Vitousek, M. N., Hubbard, J. K., and Safran, R. J. (2014). An experimental analysis of the heritability of variation in glucocorticoid concentrations in a wild avian population. Proc. R. Soc. B 281:20141302.
Jimeno, B., Hau, M., and Verhulst, S. (2018). Corticosterone levels reflect variation in metabolic rate, independent of ‘stress’. Sci. Rep. 8, 1–8. doi: 10.1038/s41598-018-31258-z
Jimeno, B., Prichard, M. R., Landry, D., Wolf, C., Larkin, B., Cheviron, Z., et al. (2020). Metabolic rates predict baseline corticosterone and reproductive output in a free-living passerine. Integr. Org. Biol. 2:obaa030. doi: 10.1093/iob/obaa030
Jones, B. C., Nguyen, L. T., and DuVal, E. H. (2021). Testing the developmental hypothesis of the HPA axis in a tropical passerine: Dampened corticosterone response and faster negative feedback in nestling lance-tailed manakins (Chiroxiphia lanceolata). Gen. Comp. Endocrinol. 300:113639. doi: 10.1016/j.ygcen.2020.113639
Kitaysky, A. S., Kitaiskaia, E. V., Piatt, J. F., and Wingfield, J. C. (2003). Benefits and costs of increased levels of corticosterone in seabird chicks. Horm. Behav. 43, 140–149.
Kitaysky, A. S., Kitaiskaia, E. V., Wingfield, J. C., and Piatt, J. F. (2001). Dietary restriction causes chronic elevation of corticosterone and enhances stress response in red-legged kittiwake chicks. J. Comp. Physiol. B 171, 701–709.
Landys, M. M., Ramenofsky, M., and Wingfield, J. C. (2006). Actions of glucocorticoids at a seasonal baseline as compared to stress-related levels in the regulation of periodic life processes. Gen. Comp. Endocrinol. 148, 132–149. doi: 10.1016/j.ygcen.2006.02.013
Love, O. P., Bird, D. M., and Shutt, L. J. (2003). Corticosterone levels during post-natal development in captive American kestrels (Falco sparverius). Gen. Comp. Endocrinol. 130, 135–141. doi: 10.1016/s0016-6480(02)00587-7
Lynn, S. E., Breuner, C. W., and Wingfield, J. C. (2003). Short-term fasting affects locomotor activity, corticosterone, and corticosterone binding globulin in a migratory songbird. Horm. Behav. 43, 150–157. doi: 10.1016/s0018-506x(02)00023-5
Lynn, S. E., Stamplis, T. B., Barrington, W. T., Weida, N., and Hudak, C. A. (2010). Food, stress, and reproduction: Short-term fasting alters endocrine physiology and reproductive behavior in the zebra finch. Horm. Behav. 58, 214–222. doi: 10.1016/j.yhbeh.2010.03.015
Marasco, V., Robinson, J., Herzyk, P., and Spencer, K. A. (2012). Pre-and post-natal stress in context: Effects on the stress physiology in a precocial bird. J. Exp. Biol. 215, 3955–3964. doi: 10.1242/jeb.071423
Martin, L. B. I. I., Gilliam, J., Han, P., Lee, K., and Wikelski, M. (2005). Corticosterone suppresses cutaneous immune function in temperate but not tropical House Sparrows, Passer domesticus. Gen. Comp. Endocrinol. 140, 126–135. doi: 10.1016/j.ygcen.2004.10.010
McEwen, B. S., and Wingfield, J. C. (2003). The concept of allostasis in biology and biomedicine. Horm. Behav. 43, 2–15.
Newbold, T. (2018). Future effects of climate and land-use change on terrestrial vertebrate community diversity under different scenarios. Proc. R. Soc. B 285:20180792. doi: 10.1098/rspb.2018.0792
Ouyang, J. Q., Hau, M., and Bonier, F. (2011). Within seasons and among years?: When are corticosterone levels repeatable? Horm. Behav. 60, 559–564.
Peig, J., and Green, A. J. (2009). New perspectives for estimating body condition from mass/length data?: The scaled mass index as an alternative method. Oikos 118, 1883–1891.
Quilfeldt, P., Poisbleau, M., Chastel, O., and Masello, J. F. (2006). Corticosterone in thin-billed prion Pachyptila belcheri chicks: Diel rhythm, timing of fledging and nutritional stress. Naturwissenschaften 94, 919–925. doi: 10.1007/s00114-007-0275-6
Rensel, M. A., and Schoech, S. J. (2011). Repeatability of baseline and stress-induced corticosterone levels across early life stages in the Florida scrub-jay (Aphelocoma coerulescens). Horm. Behav. 59, 497–502. doi: 10.1016/j.yhbeh.2011.01.010
Rensel, M. A., Boughton, R. K., and Schoech, S. J. (2010). Development of the adrenal stress response in the Florida scrub-jay (Aphelocoma coerulescens). Gen. Comp. Endocrinol. 165, 255–261. doi: 10.1016/j.ygcen.2009.07.002
Rivers, J. W., Martin, L. B., Liebl, A. L., and Betts, M. G. (2011). Parental Alarm Calls of the White-Crowned Sparrow Fail to Stimulate Corticosterone Production in Nest-Bound Offspring. Ethology 117, 374–384.
Romero, L. M., and Reed, J. M. (2005). Collecting baseline corticosterone samples in the field: Is under 3 min good enough? Comp. Biochem. Physiol. Part A 140, 73–79. doi: 10.1016/j.cbpb.2004.11.004
Romero, L. M., and Reed, J. M. (2008). Repeatability of baseline corticosterone concentrations. Gen. Comp. Endocrinol. 156, 27–33.
Romero, L. M., and Wingfield, J. C. (2016). Tempests, Poxes, Predators, and People: Stress in Wild Animals and how they Cope. Oxford: Oxford University Press.
Romero, L. M., Dickens, M. J., and Cyr, N. E. (2009). The reactive scope model—A new model integrating homeostasis, allostasis, and stress. Horm. Behav. 55, 375–389. doi: 10.1016/j.yhbeh.2008.12.009
Sagar, R. L., Cockrem, J., Rayner, M. J., Stanley, M. C., Welch, J., and Dunphy, B. J. (2019). Regular handling reduces corticosterone stress responses to handling but not condition of semi-precocial mottled petrel (Pterodroma inexpectata) chicks. Gen. Comp. Endocrinol. 272, 1–8.
Sapolsky, R. M., Romero, L. M., and Munck, A. U. (2000). How do glucocorticoids influence stress responses? Integrating permissive, suppressive, stimulatory, and preparative actions. Endocr. Rev. 21, 55–89. doi: 10.1210/edrv.21.1.0389
Satterlee, D. G., and Johnson, W. A. (1988). Selection of Japanese quail for contrasting blood corticosterone response to immobilization. Poult. Sci. 67, 25–32.
Sauser, C., Delord, K., and Barbraud, C. (2021). Sea ice and local weather affect reproductive phenology of a polar seabird with breeding consequences. Condor 123:duab032.
Schoech, S. J., Rensel, M. A., and Heiss, R. S. (2011). Short-and long-term effects of developmental corticosterone exposure on avian physiology, behavioral phenotype, cognition, and fitness: A review. Curr. Zool. 57, 514–530.
Sims, C. G., and Holberton, R. L. (2000). Development of the corticosterone stress response in young northern mockingbirds (Mimus polyglottos). Gen. Comp. Endocrinol. 119, 193–201. doi: 10.1006/gcen.2000.7506
Sockman, K. W., and Schwabl, H. (2001). Plasma Corticosterone in Nestling American Kestrels: Effects of Age, Handling Stress, Yolk Androgens, and Body Condition. Gen. Comp. Endocrinol. 122, 205–212. doi: 10.1006/gcen.2001.7626
Taff, C. C., Schoenle, L. A., and Vitousek, M. N. (2018). The repeatability of glucocorticoids: A review and meta-analysis. Gen. Comp. Endocrinol. 260, 136–145.
Vedder, O., and Bouwhuis, S. (2018). Heterogeneity in individual quality in birds: Overall patterns and insights from a study on common terns. Oikos 127, 719–727.
Vitousek, M. N., Jenkins, B. R., and Safran, R. J. (2014). Stress and success: Individual differences in the glucocorticoid stress response predict behavior and reproductive success under high predation risk. Horm. Behav. 66, 812–819. doi: 10.1016/j.yhbeh.2014.11.004
Wada, H. (2008). Glucocorticoids: Mediators of vertebrate ontogenetic transitions. Gen. Comp. Endocrinol. 156, 441–453.
Wada, H., Hahn, T. P., and Breuner, C. W. (2007). Development of stress reactivity in white-crowned sparrow nestlings: Total corticosterone response increases with age, while free corticosterone response remains low. Gen. Comp. Endocrinol. 150, 405–413. doi: 10.1016/j.ygcen.2006.10.002
Wada, H., Salvante, K. G., Wagner, E., Williams, T. D., and Breuner, C. W. (2009). Ontogeny and individual variation in the adrenocortical response of zebra finch (Taeniopygia guttata) nestlings. Physiol. Biochem. Zool. 82, 325–331. doi: 10.1086/599320
Wada, H., Salvante, K. G., Stables C., Wagner, E., Williams, T. D., Breuner, C. W. (2008). Adrenocortical responses in zebra finches (Taeniopygia guttata): Individual variation, repeatability, and relationship to phenotypic quality. Horm. Behav. 453, 472–480. doi: 10.1016/j.yhbeh.2007.11.018
Warren, R., Price, J., Graham, E., Forstenhaeusler, N., and VanDerWal, J. (2018). The projected effect on insects, vertebrates, and plants of limiting global warming to 1.5 C rather than 2 C. Science 360, 791–795. doi: 10.1126/science.aar3646
Wilson, A. J., and Nussey, D. H. (2010). What is individual quality? An evolutionary perspective. Trends Ecol. Evol. 25, 207–214.
Wingfield, J. C. (2003). Control of behavioural strategies for capricious environments. Anim. Behav. 66, 806–816.
Wingfield, J. C., and Sapolsky, R. M. (2003). Reproduction and resistance to stress: When and how. J. Neuroendocrinol. 15, 711–724.
Wingfield, J. C., Maney, D. L., Breuner, C. W., Jacobs, J. D., Lynn, S., Ramenofsky, M., et al. (1998). Ecological bases of hormone—Behavior interactions: The “emergency life history stage”. Am. Zool. 38, 191–206.
Keywords: stress, glucocorticoids, development, repeatability, HPA axis
Citation: Angelier F, Wingfield JC, Barbraud C, Parenteau C, Trouvé C and Chastel O (2022) Ontogeny and individual heterogeneity of the corticosterone stress response in a wild altricial seabird, the snow petrel (Pagodroma nivea). Front. Ecol. Evol. 10:902202. doi: 10.3389/fevo.2022.902202
Received: 22 March 2022; Accepted: 19 July 2022;
Published: 18 August 2022.
Edited by:
John Cockrem, Massey University, New ZealandReviewed by:
Karl Berg, The University of Texas Rio Grande Valley, United StatesSimon Verhulst, University of Groningen, Netherlands
Copyright © 2022 Angelier, Wingfield, Barbraud, Parenteau, Trouvé and Chastel. This is an open-access article distributed under the terms of the Creative Commons Attribution License (CC BY). The use, distribution or reproduction in other forums is permitted, provided the original author(s) and the copyright owner(s) are credited and that the original publication in this journal is cited, in accordance with accepted academic practice. No use, distribution or reproduction is permitted which does not comply with these terms.
*Correspondence: Frédéric Angelier, YW5nZWxpZXJAY2ViYy5jbnJzLmZy